- 1Leibniz-Institute for Baltic Sea Research Warnemünde, Rostock, Germany
- 2Danish Shellfish Center, National Institute of Aquatic Resources, Technical University of Denmark, Nykøbing Mors, Denmark
- 3Department of Marine Sciences, University of Gothenburg, Gothenburg, Sweden
- 4Marine Research Institute, Klaipėda, Lithuania
To support the ongoing discussion about mussel farming and the potential to extract nutrients from the sea, this study investigated the phosphorus (P) and nitrogen (N) content of blue mussels (Mytilus spp.) under different abiotic and biotic parameters. The focus of this survey was on the highly eutrophied Baltic Sea, where salinity ranges from 4 to 27 psu, and is a major contributing factor to differential mussel growth. We observed that nutrient content was not linearly correlated to salinity, but if categorized, decreased at higher salinities. Chlorophyll-a and temperature did not significantly correlate with nutrient content, but season of harvest and mussel size did. Furthermore, habitat was a strong driver of nutrient content, indicating higher nutrient density if mussels are grown in mussel farms (i.e., in the water column) instead of on mussel culture beds or harvested from wild beds (on the sea bed). Values of N and P averaged 5.85% N and 0.83% P of tissue dry weight in mussels at the sea bed and 9.43% N and 0.96% P of tissue dry weight in mussels from longline cultivation. These results will be useful in refining estimations about mussel farming as a nutrient mitigation measure and the extraction potential, as well as related costs.
Introduction
The persistence and magnitude of eutrophication in the Baltic Searequires cost-effective measures to reduce nutrients and to achieve agood ecological status (GES) based on the Water Framework Directive (WFD, European Parliament, 2000) and the Marine Strategy Framework Directive (MSFD, European Parliament, 2008). Extensive mussel aquaculture on longlines or tube-net systems (e.g., Smart Farm) is highly discussed as such a measure in the greater Baltic Sea (Lindahl and Kollberg, 2008; Stadmark and Conley, 2011; Petersen et al., 2012, 2014, 2019; Nielsen et al., 2016; Hedberg et al., 2018; Gren, 2019; Taylor et al., 2019; Kotta et al., 2020). The amount of nutrients that can hereby be removed depends on several parameters, such as nutrient content of the mussels, growth rates, harvesting time, and farm set up (Capillo et al., 2018; Taylor et al., 2019). These parameters drive the total mitigation potential and economic feasibility of mussel farming as a eutrophication measure in different areas of the Baltic Sea. However, to the extent which environmental parameters (salinity, chl-a, temperature) influence the nutrient content within mussels has not been exhaustively investigated. Previous studies (Hedberg et al., 2018; Buer et al., 2020; Holbach et al., 2020; Kotta et al., 2020) report a fluctuating nutrient content of blue mussels across the Baltic Sea but base their estimation of total mitigation potentials rather on different growth rates and an average nutrient content. Besides area-specific growth rates, it is important to evaluate the parameters that affect the actual nitrogen and phosphorus content stored in mussel tissue. Area-specific nutrient contents can support further studies on the mitigation potential, cost analyses and projections for Baltic-wide nutrient reduction.
The main objective of the present study was to document the relationship between environmental conditions and nutrient content in blue mussels across the Baltic Sea. Therefore, we analyzed the nutrient content (nitrogen, N and phosphorus, P) of blue mussels across the Baltic Sea in regards to different environmental parameters (salinity, maxima of water temperature, chlorophyll-a levels). Furthermore, nutrient contents were related to mussel size, habitat, and season of harvest. Providing the different environmental conditions across the greater Baltic Sea, our hypothesis was that environmental parameters would correlate with condition and nutrient contents of mussels, and an analytical approach to test for associations was followed.
Materials and Methods
Mussel samples were collected from aquaculture farms and wild stock as indicated in Figure 1.
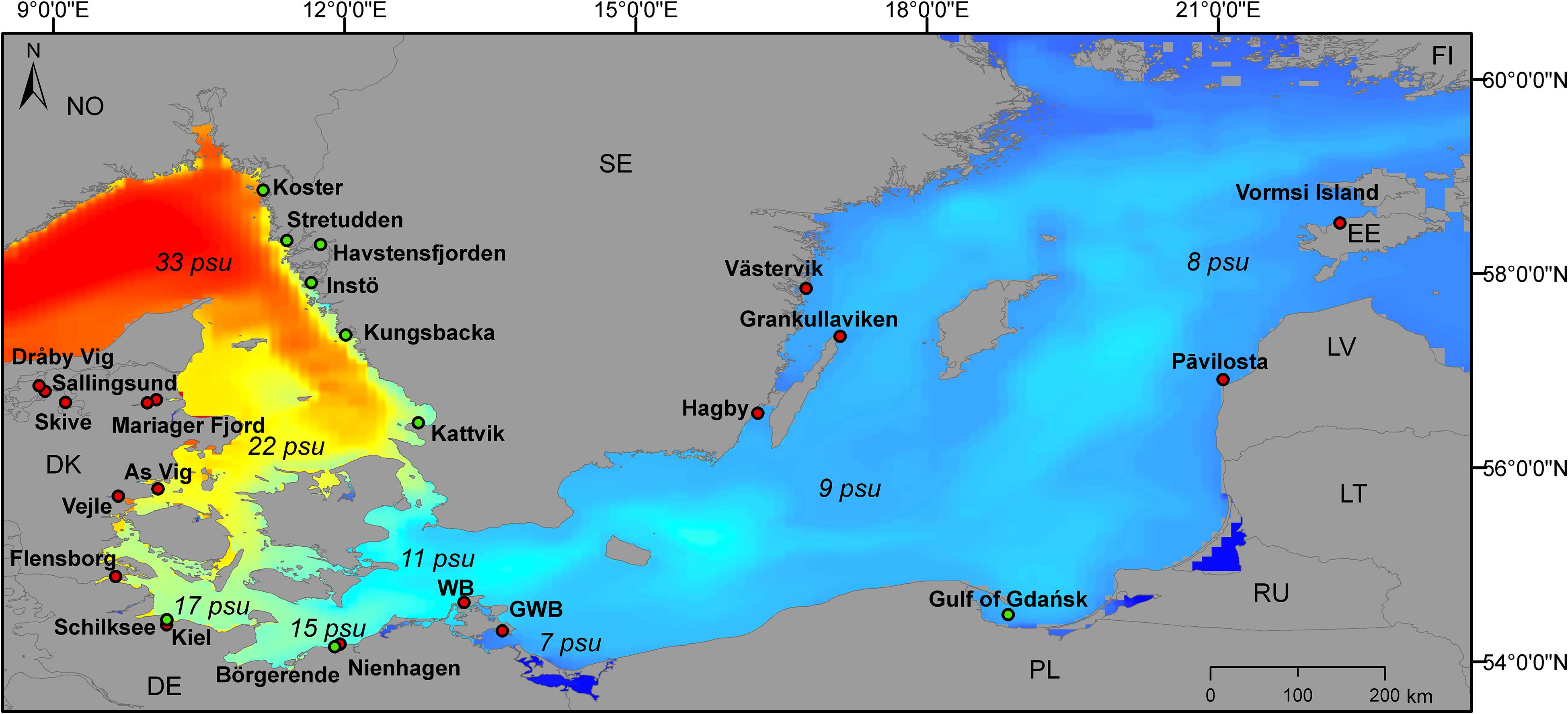
Figure 1. Sampling sites along the Baltic Sea coast. Red circles indicate samples from longline collectors (in the water column), green circles indicate sampling from wild stocks (off the ground).
Samples along the Swedish west coast were collected at six sites from natural mussel beds, taken at 0.5 m water depth, in the spring of 2014. Mussels were divided into three size classes (5–15, 20–30, and 40–50 mm) and the dry tissue of each individual (n = 178) was analyzed separately for N and P content. After freeze drying, a persulfate oxidation method was used for analysis of nitrogen and phosphorous (Valderrama, 1995). The age of the mussels was estimated based on the mussel shell length.
Samples from the eastern Swedish coast (Grankullaviken, Västervik and Hagby), the Latvian coast (Pâvilosta), and Estonia (Vormsi Island) were harvested from test mussel farms within the Interreg project Baltic Blue Growth (BBG). Mussel samples from Greifswald Bay (GWB), Wieker Bay (WB), Nienhagen, Kiel, and Flensburg (Germany), as well as the Danish sites (Sallingsund, Skive, Dråby Vig, Mariager mid- and inner-fjord, Vejle, As Vig), were collected at mussel farms within the BONUS Optimus project. In Börgerende and Schilksee, DE, as well as Gulf of Gdańsk, PL, mussels were collected from natural hard substrate. For all sites, mussels of different size, age and season were sampled and frozen in sealed containers (−20°C) for further analyses. Subsequently, the shell length and total wet weight of a minimum of 10–30 individuals was measured. After dissection, tissue and shell of all individuals was pooled, freeze-dried, and ground, before carbon and nitrogen were analyzed in an auto-analyzer (Elementaranalysator EA 3000). Phosphorous was determined in mussel ash using an alkaline persulfate oxidation after Hansen and Koroleff (1999). Danish samples cover seven sites, while some sites were sampled in different seasons (n = 100 for each sampling event). The mussels were processed after ISO (1998) 6491 (1998) and ISO (2009) 5983-2 (2009) for phosphorus and nitrogen determination, respectively.
The tissue-shell-ratio, also known as the condition index, was calculated based on the dry weight of mussel tissue and shell.
Environmental data (salinity, temperature and chlorophyll-a concentration) was collected by state authority monitoring programs or modeled data (for Poland) for the upper water layers (0.5 m depth)1. Chlorophyll-a was analyzed fluorometric (665 nm) after filtration (GF/F, 0.7 μm), extraction with ethanol and acidification (ISO 10260:19922) following HELCOM – Guidelines for monitoring of chlorophyll-a2.
Correlations of biometric data and environmental values were evaluated by bootstrapping ordinary least squares linear regression and mixed model effect leveraging by minimizing AICc. Confidence intervals were determined by bootstrapping and categorical comparison (habitat, season) was performed by permutation ANOVA (1E5 permutations) with the vegan package in R (Oksanen et al., 2019). Full factorial mixed models were bootstrapped and used to evaluate interactions with environmental variables. Normality of residuals was checked by a normal QQ plot and the Shapiro-Wilk test. Finally, for nutrient content of dry tissues, a full factorial interaction model of all environmental parameters, habitat, and season was reduced by elastic net regularization in the glmnet package in R by setting the alpha parameter to 0.5 (Friedman et al., 2010).
Additionally, data was pooled into three salinity categories: below 15 psu (6–10 psu, based on sample availability), above 15 psu and above 20 psu. Salinities below 15 psu were expected to have a strong effect on mussel condition and water content (DW/WW-ratio) (Maar et al., 2015). As a bottleneck for water exchange between the Kattegat and Baltic proper, the Great Belt area provides a natural border between salinities above and below 20 psu and thereby, as a natural divider of samples (Feistel et al., 2009; Riisgård et al., 2012). These categories were used to detect potential differences in mussel N and P content (in tissue DW and total WW) as well as differences in the tissue-shell-ratio.
Results
Nutrients accumulate primarily in the bivalve tissues, only a minor fraction is stored in the shell (ratio of 0.16 ± 0.04 N in DW). Therefore, phosphorous and nitrogen concentrations of the mussel shell was not measured in all samples. To estimate N and P-values of the total mussel wet weight (including shell), measured N and P-values of the dry tissue were combined with literature data for N and P in the dry shell, averaged water contents and averaged shell-tissue-ratios. 0.04% P and 1% N of dry shell mass were assumed for all sites based on literature and our own data (Haamer, 1996; Ek Henning and Åslund, 2012; Petersen et al., 2014; Bucefalos, 2015; Palm et al., 2015; Hedberg et al., 2018). Water contents and shell-tissue-ratios were averaged for samples at each site. Results are presented in Table 1.
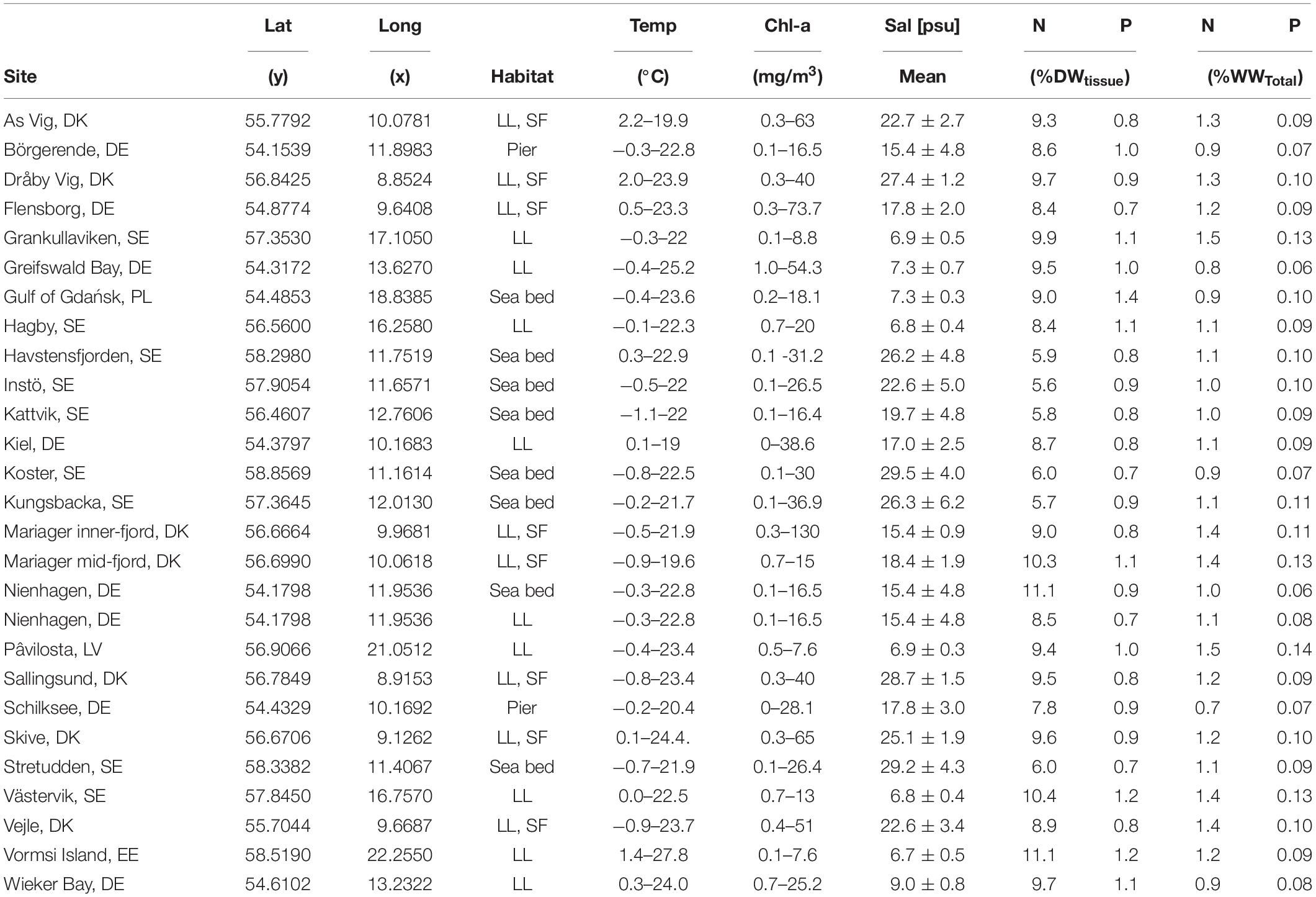
Table 1. Sample locations, bivalve habitat (LL, longline; SF,Smartfarm), environmental parameters, and N&P contents in dry tissue mass (DW) as well as total wet weight (WW).
Tissue-shell-ratios were not linearly correlated to average salinity (R2 = 1.7e-4, p = 0.84), although a weak trend toward higher ratios in higher salinities appeared if categorically pooled (Figure 2A). Average site temperature was equally poor in explaining variation (R2 = 0.007, p = 0.12) as well as chlorophyll-a (R2 = 0.01, p = 0.05). Comparison of habitats exhibits a higher ratio in mussels grown in the water column [Figure 2B, F(1,214) = 56.61, p < 0.001]. This suggests that mussels grown on suspended substrate in the water column have either thinner shells or higher somatic tissue mass compared to mussels from the sea bed. Nitrogen [F(1,213) = 610, p < 0.001] and phosphorus [F(1,213) = 11.54, p < 0.001] contents were similarly significantly higher in samples from mussel farms [9.55 ± 0.73 (95%CI:9.30–9.78)% N DWtissue, 0.96 ± 0.17 (95%CI:0.89–1.01]% P DWtissue] compared to mussels from the sea bottom [5.85 ± 0.83 (95%CI:5.72–5.96)% N DWtissue, 0.82 ± 0.22 (95%CI:0.79–0.85)% P DWtissue] (Figure 2C). In a mixed model analysis of salinity and habitat, habitat exhibited significant leverage (R2 = 0.50, p < 0.001), but interaction between habitat and salinity was not significant (p = 0.21). These results suggest position in the water column or mode of attachment influences condition index to a greater degree than salinity alone. Crossing all environmental site averages was relatively effective in describing variability in the tissue-shell ratio (R2 = 0.38, p < 0.001), where chlorophyll-a, temperature, and their interaction were the only significant effects (p < 0.01).
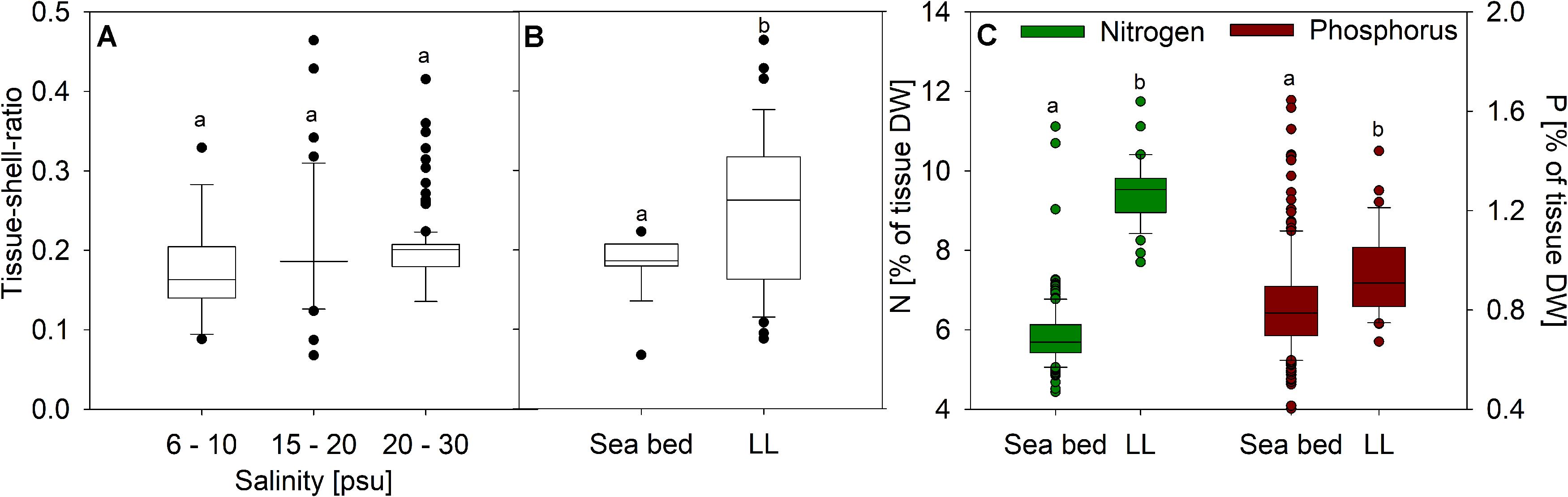
Figure 2. Ratio of dry tissue to shell in regards to (A) salinity and (B) habitat of the mussel as well as (C) nitrogen and phosphorus content in dry tissue of mussels from the sea bed and cultivated on longlines (LL).
The nutrient content (N&P) within mussel dry tissue was weakly correlated to average salinity (R2N = 0.28, R2P = 0.08), both exhibited negative slopes, indicating a general inverse trend of nutrient content to salinity. After samples were pooled into salinity categories, the phosphorus content showed significant differences between the lowest (6–10 psu) and the intermediate (15–20 psu) as well as the highest (20–30 psu) salinity (p < 0.001) but not between the latter (p = 1.000). The nitrogen content followed the same pattern, and showed significant differences between the lowest salinity and the two higher (p < 0.001) but not between the intermediate and high salinity categories (p = 0.179) (Figure 3A). Differences in nitrogen in total wet weight were similar, but not significant (p > 0.05). Phosphorus concentrations in total wet weight were not significantly different based on salinity (p > 0.05) (Figure 3B). No correlation was observed between nutrient content in tissue dry weight to mean values of chlorophyll-a concentration (Figure 3C) nor to maximal water temperatures (Figure 3D) at each site. However, differences were observed between seasons (Figure 3E, samples of all study sites included), indicating a significant lower nitrogen content in spring compared to all other seasons [F(3,211) = 80.76, p < 0.001]. Phosphorus contents on the other hand were not different between seasons [F(3,211) = 1.805, p = 0.1418] (Figure 3E). Furthermore, nutrient content also differed between mussel sizes (Figure 3F, pooled samples of Swedish sites). Small mussels (5–15 mm) had a significant higher nitrogen content in the dry tissue compared to medium (20–30 mm, p = 0.009) and large (20–50 mm, p < 0.001) mussels. On the contrary, the phosphorus content increased with mussel size (p > 0.001 between each group).
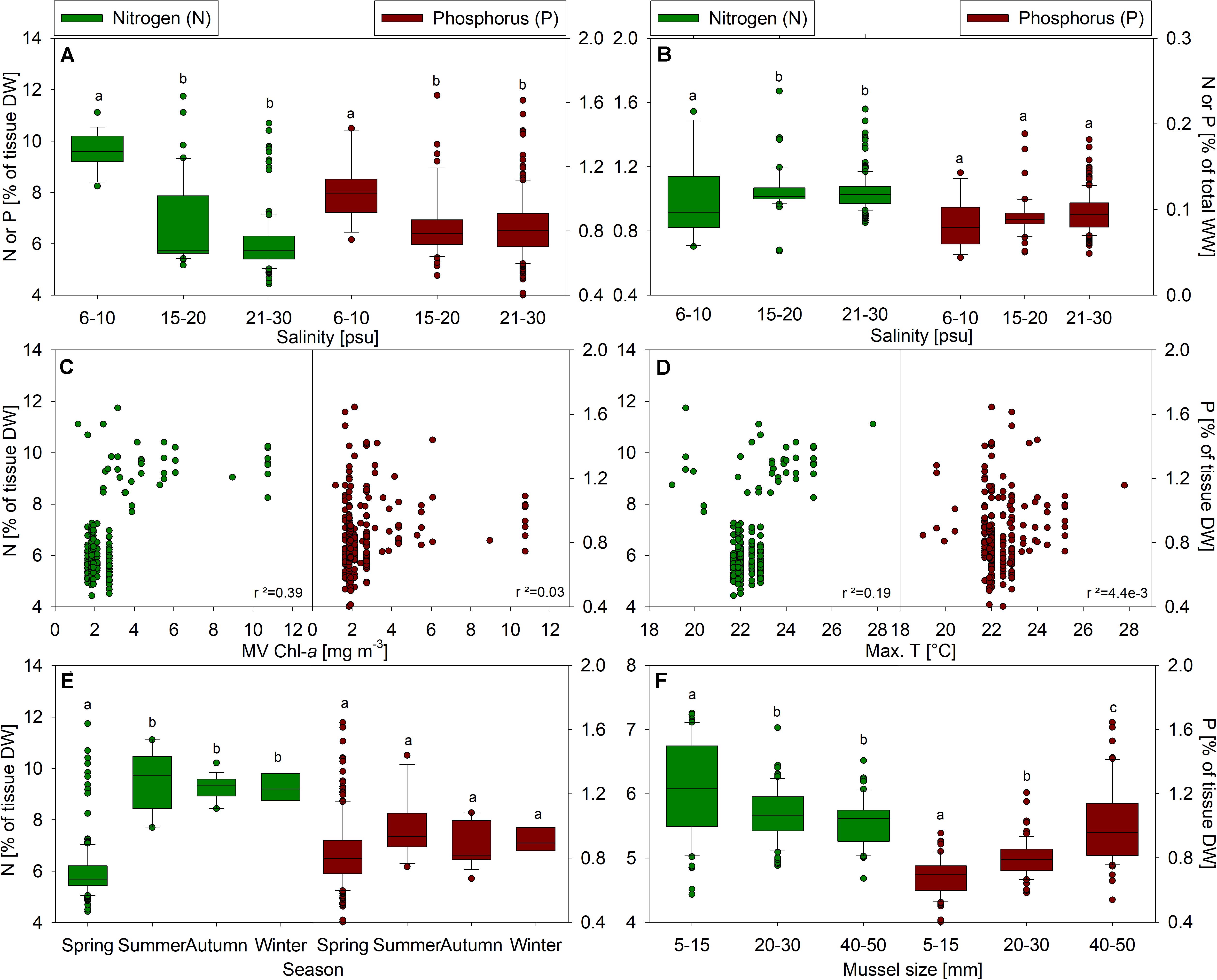
Figure 3. Nitrogen (N, green, on the left) and phosphorus (P, red, on the right) content in tissue dry weight (DW) and total wet weight (WW) plotted over different environmental parameters: (A) salinity categories (DW), (B) salinity categories (WW), (C) mean value chlorophyll-a (DW) and (D) maximal water temperatures (DW); as well as nutrients over (E) season of harvest and (F) mussel size (in shell length, only Swedish samples).
Reduction of the full factorial model for N in DW tissue, with mean environmental parameters, habitat, and season, included season∗habitat, season∗temperature∗chlorophyll-a, season∗salinity, and habitat as significant effects (R2 = 0.83, p < 0.05). For P in DW tissue, only habitat (p = 0.003) and season (p = 0.006) had significant effects, but were weakly correlated with the data (R2 = 0.15). It should be noted that habitat is correlated with chlorophyll-a, as longline (LL) exhibited higher mean values [5.80 ± 3.06 (95%CI:4.79–6.77)] than sea bed sites [2.05 ± 0.39 (95%CI:1.99–2.11)] [F(1,213) = 253.2, p < 0.001]. Furthermore, LL sites had higher mean temperatures [10.45 ± 0.99 (95%CI:10.14–10.78)] than sea bed sites [9.91 ± 0.31 (95%CI:9.87–9.96)] [F(1,213) = 35.45, p = 0.003] and lower salinity [15.59 ± 8.55 (95%CI:12.87–18.29)] than sea bed sites [25.07 ± 3.65 (95%CI:24.55–25.61)] [F(1,213) = 116.6, p < 0.001]. In sum, season and habitat were the most influential effects on the variation in N and P in DW tissue. When limiting to LL, as will be the habitat of mitigation culture under current recommendations, none of the environmental parameters were correlated with N or P content in DW tissue, with the exception of weak negative correlation of salinity and P content (R2 = 0.1, p = 0.04). Season as a mixed effect did not contribute to variation.
Discussion and Conclusion
Generally, the nutrient content within mussel tissue was not strongly related to the environmental parameters: salinity, average chlorophyll-a levels, or maximum water temperatures. A lower dry weight nutrient content in mussels of the eastern Baltic Sea by decreasing salinities was not observed. Consequently, our original hypothesis that these environmental parameters would account for most of the variability in mussel nutrient content, was rejected. On the other hand, it was evident that mussels cultivated on suspended substrate in the upper water column exhibited considerably higher nutrient contents than mussels on the sea bed. It has previously been demonstrated that cultivation mode, or in general terms, position in the water column, significantly influences the biochemical composition of mussel tissues (Colombo et al., 2016). Interactions of parameters suggested that simply season and habitat encompassed much of the variability in N and P content; while it is acknowledged that some of the associated variability within the environmental parameters are built into habitat and season. Vertical gradients in phytoplankton concentrations and stratification can exacerbate food limitation in mussels at the sea bed (Wiles et al., 2006; Filgueira et al., 2018). Prior work has demonstrated a relationship between food concentration, composition, and mussel tissue biochemistry (Pleissner et al., 2012). Two potentially important implications of this habitat differentiation are: (1) samples from wild mussel beds do not serve as reliable surrogates of nutrient content when estimating potential nutrient yields in mitigation culture; (2) nutrient content of DW tissue does not appear to vary much in response to the salinity gradient.
Harvest timing (seasonality) is an important parameter when considering the potential nutrient extraction potential. Low spring nitrogen contents in cultivated blue mussels are consistent with primary gamete release (Pieters et al., 1980; Okumus and Stirling, 1998; Fernández et al., 2015). Taylor et al. (2019) recommended late autumn through winter harvests in order to maximize nutrient extraction efficiency. This will additionally avoid potential loss of nutrient content overlapping with gamete release, and permit new spat collection in the late spring/early summer.
Due to the low resolution of environmental parameters in time and space, it was difficult to draw definitive conclusions from observed relationships of nutrient content and environmental conditions. These analyses could be improved by evaluating interannual variation relative to position-specific high resolution environmental data. Supplementing interpretations of nutrient extraction potentials by energetic models may corroborate the importance of environmental conditions to nutrient content (Buer et al., 2020).
In sum, these results suggest that variability in proportional mussel nutrient content will be nominal when cultivated in the water column if there is sufficient food available, and harvesting timed to avoid the reproduction period. Total biomass, therefore, will be the meaningful metric when estimating nutrient extraction potential. Furthermore, although there is reduced growth of blue mussels at low salinities, proportional nutrient content is not significantly reduced in the summary harvested dry materials.
Data Availability Statement
All datasets generated for this study are included in the article/Supplementary Material.
Author Contributions
A-LB was responsible for overall structure and writing of the manuscript, data collection, and sample processing. DT and LR contributed to data curation and manuscript writing. PB and DT provided the data for nutrients in mussels of Sweden’s west coast and Denmark, respectively, who also contributed to the text of the manuscript. AK was involved in sample processing. All authors contributed to the article and approved the submitted version.
Funding
The work was financially supported by the project BONUS OPTIMUS (03A0020A). The project has received funding from BONUS (Art 185) funded jointly by the European Union’s Seventh Programme for research, technological development and demonstration, and from Baltic Sea national funding institutions. The publication of this article was funded by the Open Access Fund of the Leibniz Association.
Conflict of Interest
The authors declare that the research was conducted in the absence of any commercial or financial relationships that could be construed as a potential conflict of interest.
Acknowledgments
We would like to thank the people who provided mussel samples from around the Baltic: Juris Aigars (Latvian Institute of Aquatic Ecology), Jonne Kotta (University of Tartu), Susanna Minnhagen (Kalmar Municipality), Ksenia Pazdro (IOPAN), Tim Staufenberger (Kieler Meeresfarm), and Florian Peine (LFA). For the provision of environmental long-term data, we thank SMHI, Latvian Institute of Aquatic Ecology, Estonian Marine Institute, Danish Overfladevandsdatabasen, IOPAN, LLUR, and LUNG. Furthermore, Regina Hansen, Anne Köhler, and Ines Scherff for their support on CNP analyses, as well as Ivar Lund and Ulla Sproegel for Danish nutrient analyses. Additionally, we would like to thank the editor and the three referees for their critical review and very valuable input.
Supplementary Material
The Supplementary Material for this article can be found online at: https://www.frontiersin.org/articles/10.3389/fmars.2020.00705/full#supplementary-material
Footnotes
- ^ Swedish Meteorological and Hydrological Institute (SMHI, www.smhi.se.data); Latvian Institute of Aquatic Ecology; Estonian Marine Institute; Dansih Overfladevandsdatabasen (ODA, https://oda.dk); SatBaltyk IOPAN (satbaltyk.iopan.gda.pl); Landesamt für Landwirtschaft, Umwelt und ländliche Räume des Landes Schleswig-Holstein (LLUR); Landesamt für Umwelt, Naturschutz und Geologie Mecklenburg-Vorpommern (LUNG).
- ^ https://helcom.fi/media/publications/Guidelines-for-measuring-chlorophyll-a.pdf
References
Bucefalos (2015). Design of Full Scale Blue Mussel Cultivation Site in Öresund. Report from the Bucefalos Project BUCEFALOS/LIFE11/ENV/SE/839. New York, NY: Bucefalos
Buer, A. L., Maar, M., Nepf, M., Ritzenhofen, L., Dahlke, S., Friedland, R., et al. (2020). Potential and feasibility of Mytilus spp. farming along a salinity gradient. Front. Mar. Sci. 7:371. doi: 10.3389/fmars.2020.00371
Capillo, G., Silvestro, S., Sanfilippo, M., Fiorino, E., Giangrosso, G., Ferrantelli, V., et al. (2018). Assessment of electrolytes and metals profile of the Faro Lake (Capo Peloro Lagoon, Sicily, Italy) and its impact on Mytilus galloprovincialis. Chem. Biodivers. 15:e1800044. doi: 10.1002/cbdv.201800044
Colombo, J., Varisco, M., Isola, T., Crovetto, C., Rost, E., and Risso, S. (2016). Composición química proximal y perfil de ácidos grasos del mejillón Mytilus edulis provenientes de cultivos y bancos naturales en el Golfo San Jorge, Argentina. Rev. Biol. Mar. Oceanogr. 51, 293–299. doi: 10.4067/S0718-19572016000200007
Ek Henning, H., and Åslund, M. (2012). Pilotmusselodlingar i Östergötlands Skärgård – Kunskapsunderlag för Storskaliga Musselodlingar. Östergötland: Länsstyrelsen Östergötland.
European Parliament (2000). Directive 2000/60/EC of the European Parliament and of the Council of 23 October 2000 establishing a framework for community action in the field of water policy. Off. J. Eur. Parliam. 327, 1–82. doi: 10.1039/ap9842100196
European Parliament (2008). Directive 2008/56/EC of the European Parliament and of the Council of 17 June 2008 establishing a framework for community action in the field of marine environmental policy (Marine Strategy Framework Directive). Off. J. Eur. Parliam. 164, 19–40.
Feistel, R., Weinreben, S., Wolf, H., Seitz, S., Spitzer, P., Adel, B., et al. (2009). Density and absolute salinity of the Baltic Sea 2006–2009. Ocean Sci. Discuss. 6, 1757–1817. doi: 10.5194/osd-6-1757-2009
Fernández, A., Grienke, U., Soler-Vila, A., Guihéneuf, F., Stengel, D. B., and Tasdemir, D. (2015). Seasonal and geographical variations in the biochemical composition of the blue mussel (Mytilus edulis L.) from Ireland. Food Chem. 177, 43–52. doi: 10.1016/j.foodchem.2014.12.062
Filgueira, R., Grant, J., and Kjerulf Petersen, J. (2018). Identifying the optimal depth for mussel suspended culture in shallow and turbid environments. J. Sea Res. 132, 15–23. doi: 10.1016/j.seares.2017.11.006
Friedman, J., Hastie, T., and Tibshirani, R. (2010). Regularization paths for generalized linear models via coordinate descent. J. Stat. Softw. 33, 1–22. doi: 10.1016/0196-0709(89)90135-X
Gren, I. M. (2019). The economic value of mussel farming for uncertain nutrient removal in the Baltic Sea. PLoS One 14:e0218023. doi: 10.1371/journal.pone.0218023
Haamer, J. (1996). Improving water quality in a eutrophied fjord system with mussel farming. Ambio 25, 356–362.
Hansen, H. P., and Koroleff, F. (1999). “Determination of nutrients,” in Methods of Seawater Analysis, 3rd Edn, eds K. Grasshoff, K. Krem-ling, and M. Ehrhardt (Weinheim: Wiley-VCH Verlag).
Hedberg, N., Kautsky, N., Kumblad, L., and Wikström, S. A. (2018). Limitations of Using Blue Mussel Farms as a Nutrient Reduction Measure in the Baltic Sea. Report number: 2/2018. Stockholm: Stockholm University.
Holbach, A., Maar, M., Timmermann, K., and Taylor, D. (2020). A spatial model for nutrient mitigation potential of blue mussel farms in the western Baltic Sea. Sci. Total Environ. 736:139624. doi: 10.1016/j.scitotenv.2020.139624
ISO (1998). Animal Feeding Stuffs — Determination of Phosphorus Content — Spectrometric Method, ISO 6491:1998. Geneva: International Organisation for Standardization.
ISO (2009). Animal Feeding Stuffs — Determination of Nitrogen Content and Calculation of Crude Protein Content — Part 2: Block Digestion/Steam Distillation Method, ISO 5983-2:2009. Geneva: International Organisation for Standardization.
Kotta, J., Futter, M., Kaasik, A., Liversage, K., Rätsep, M., Barboza, F. R., et al. (2020). Cleaning up seas using blue growth initiatives: mussel farming for eutrophication control in the Baltic Sea. Sci. Total Environ. 709:136144. doi: 10.1016/j.scitotenv.2019.136144
Lindahl, O., and Kollberg, S. (2008). How mussels can improve coastal water quality: mussel farming - a way to combat eutrophication. Biosci. Explain 5, 1–14.
Maar, M., Saurel, C., Landes, A., Dolmer, P., and Petersen, J. K. (2015). Growth potential of blue mussels (M. edulis) exposed to different salinities evaluated by a Dynamic Energy Budget model. J. Mar. Syst. 148, 48–55. doi: 10.1016/j.jmarsys.2015.02.003
Nielsen, P., Cranford, P. J., Maar, M., and Petersen, J. K. (2016). Magnitude, spatial scale and optimization of ecosystem services from a nutrient extraction mussel farm in the eutrophic Skive fjord, Denmark. Aquac. Environ. Interact. 8, 312–329. doi: 10.3354/aei00175
Oksanen, J., Guillaume, F., Blanchet, R. K., Legendre, P., Minchin, P. R., O’Hara, R. B., et al. (2019). Package ‘vegan.’ Community Ecol. Packag R Package Version 2.5-6.
Okumus, I., and Stirling, H. P. (1998). Seasonal variations in the meat weight, condition index and biochemical composition of mussels (Mytilus edulis L.) in suspended culture in two Scottish sea lochs. Aquaculture 159, 249–261. doi: 10.1016/s0044-8486(97)00206-8
Palm, H. W., Graf, G., Paschen, M., and Schubert, H. (2015). Abschlussbericht: Verfahrensentwicklung einer Integrierten Multi Trophischen Aquakultur für die Küstengewässer Mecklenburg-Vorpommerns (Baltic IMTA). Available online at: http://www.aquakultur-mv.de/static/AQUA/Dokumente/Forschen/Abschlussbericht_IMTA_31_10_2015_UNIRO_(1).pdf (accessed October 1, 2017).
Petersen, J. K., Hasler, B., Timmermann, K., Nielsen, P., Tørring, D. B., Larsen, M. M., et al. (2014). Mussels as a tool for mitigation of nutrients in the marine environment. Mar. Pollut. Bull. 82, 137–143. doi: 10.1016/j.marpolbul.2014.03.006
Petersen, J. K., Holmer, M., Termansen, M., and Hasler, B. (2019). “Nutrient extraction through bivalves,” in Goods and Services of Marine Bivalves, eds A. Smaal, J. Ferreira, J. Grant, J. Petersen, and Ø. Strand (Cham: Springer), 179–208. doi: 10.1007/978-3-319-96776-9_10
Petersen, J. K., Timmermann, K., Carlsson, M., Holmer, M., Maar, M., and Lindahl, O. (2012). Mussel farming can be used as a mitigation tool - A reply. Mar. Pollut. Bull. 64, 452–454. doi: 10.1016/j.marpolbul.2011.11.027
Pieters, H., Kluytmans, J. H., Zandee, D. I., and Cadée, G. (1980). Tissue composition and reproduction of Mytilus edulis in relation to food availability. Netherlands J. Sea Res. 14, 349–361. doi: 10.1016/0077-7579(80)90008-3
Pleissner, D., Eriksen, N. T., Lundgreen, K., and Riisgård, H. U. (2012). Biomass composition of blue mussels, Mytilus edulis, is affected by living site and species of ingested microalgae. ISRN Zool. 2012, 1–12. doi: 10.5402/2012/902152
Riisgård, H. U., Bøttiger, L., and Pleissner, D. (2012). Effect of salinity on growth of mussels, Mytilus edulis, with special reference to Great Belt (Denmark). Open J. Mar. Sci. 2, 167–176. doi: 10.4236/ojms.2012.24020
Stadmark, J., and Conley, D. J. (2011). Mussel farming as a nutrient reduction measure in the Baltic Sea: consideration of nutrient biogeochemical cycles. Mar. Pollut. Bull. 62, 1385–1388. doi: 10.1016/j.marpolbul.2011.05.001
Taylor, D., Saurel, C., Nielsen, P., and Petersen, J. K. (2019). Production characteristics and optimization of mitigation mussel culture. Front. Mar. Sci. 6:698. doi: 10.3389/FMARS.2019.00698
Valderrama, J. C. (1995). “Methods of nutrient analysis,” in Manual of Harmful Marine Microalgae. IOC Manuals and Guides No 33, eds G. M. Hallegraeff, D. M. Anderson, and A. D. Cembella (Paris: UNESCO), 251–268.
Keywords: Mytilus spp., Baltic Sea, nitrogen, phosphorus, salinity
Citation: Buer A-L, Taylor D, Bergström P, Ritzenhofen L and Klemmstein A (2020) Nitrogen and Phosphorous Content in Blue Mussels (Mytilus spp.) Across the Baltic Sea. Front. Mar. Sci. 7:705. doi: 10.3389/fmars.2020.00705
Received: 28 March 2020; Accepted: 03 August 2020;
Published: 21 August 2020.
Edited by:
Jose Luis Iriarte, Institute of Aquaculture, Austral University of Chile, ChileReviewed by:
Caterina Faggio, University of Messina, ItalyHuang Wei, Second Institute of Oceanography, Ministry of Natural Resources, China
Martyn Futter, Swedish University of Agricultural Sciences, Sweden
Copyright © 2020 Buer, Taylor, Bergström, Ritzenhofen and Klemmstein. This is an open-access article distributed under the terms of the Creative Commons Attribution License (CC BY). The use, distribution or reproduction in other forums is permitted, provided the original author(s) and the copyright owner(s) are credited and that the original publication in this journal is cited, in accordance with accepted academic practice. No use, distribution or reproduction is permitted which does not comply with these terms.
*Correspondence: Anna-Lucia Buer, anna-lucia.buer@io-warnemuende.de