- 1The Nature Conservancy, Carlton, VIC, Australia
- 2College of Science and Engineering, Flinders University, Bedford Park, SA, Australia
- 3Division of Marine Science and Conservation, Nicholas School of the Environment, Duke University, Beaufort, NC, United States
- 4TropWATER, The Centre for Tropical Water and Aquatic Ecosystem Research, James Cook University, Townsville, QLD, Australia
Over 85% of the world's oyster reefs have been lost in the past two centuries, triggering a global effort to restore shellfish reef ecosystems and the ecosystem services they provide. While there has been considerable success in re-establishing oyster reefs, many challenges remain. These include: high incidence of failed restoration, high cost of restoration per unit area, and increasing stress from climate change. In order to leverage our past successes and progress the field, we must increase restoration efficiencies that not only reduce cost per unit area, but also increase the resilience of restored ecosystems. To help address this need, we qualitatively review the literature associated with the structure and function of oyster reef ecosystems to identify key positive species interactions (i.e., those species interactions where at least one partner benefits and no partners are harmed). We classified positive inter- and intraspecific interactions between oysters and organisms associated with oyster ecosystems into the following seven functional categories: (1) physical reef creation, (2) positive density dependence, (3) refugia from physical stress, (4) refugia from biological stress, (5) biodiversity enhancement, (6) settlement improvement, and (7) long-distance facilitation. We discuss each category of positive interaction and how restoration practitioners can use knowledge of such processes to enhance restoration success. We propose that systematic incorporation of positive species interactions into restoration practice will both enhance ecological services provided by restored reefs and increase restoration success.
Introduction
Ecologists have long emphasized the importance of negative interactions (i.e., competition, predation, parasitism), physical disturbances, and physiological stress in driving species diversity and shaping ecological communities (Darwin, 1859; Connell, 1956; Paine, 1965). However, over the past three decades, research has shown that positive interactions (i.e., those where neither species is harmed and at least one species benefits) are equally important in shaping population and community-level structure (Bertness and Leonard, 1997; Stachowicz, 2001; Bruno et al., 2003; Silliman et al., 2011). Positive interactions occur when one organism makes the local environment more favorable for another organism. This can happen directly, such as when canopy-forming macroalgae shade the intertidal zone and reduce desiccation stress for other organisms (Thomsen et al., 2018). Alternatively it can happen indirectly, such as when predators consume grazers, indirectly facilitating plants by reducing stress from herbivory (Stachowicz, 2001; Bruno et al., 2003).
Research on positive interactions has established a theoretical framework that predicts the relative importance of positive interactions in space and time (Bertness and Callaway, 1994). The Stress Gradient Hypothesis (SGH) predicts that facilitation among neighboring individuals is more likely under high physical or biological stress. Contrastingly, according to the SGH, negative interactions such as competition or predation prevail under low stress conditions (Bertness and Callaway, 1994; Bertness and Leonard, 1997; Bruno et al., 2003; He et al., 2013). This idea has been tested over 1,000 times across marine, terrestrial and aquatic systems both qualitatively and quantitatively (He et al., 2013). The SGH is now considered a foundational theory in ecology that generally predicts when and where positive species interactions will drive species diversity patterns and ranges (Stachowicz, 2001; Silliman et al., 2013; Silliman and He, 2018). In addition to its contribution to ecological theory, the SGH offers an important theoretical framework when considering how to restore species or ecosystems and their functions (Halpern et al., 2007).
In the field of ecological restoration, practitioners aim to reassemble a species, population, community, or ecosystem and to re-establish the ecological interactions that occurred preceding degradation (Jordan et al., 1987). Since degraded systems in need of repair are often under high stress, the SGH suggests positive species interactions should be important in helping them recover, and thus harnessing these interactions could improve restoration outcomes. However, the foundational paradigm in coastal and marine restoration has been that competition limits an organism's regrowth. Accordingly, restoration projects have focused on minimizing negative interactions, while largely ignoring potential positive ones (Silliman et al., 2015; Renzi et al., 2019; Valdez et al., 2020). However, this “foundational” principle stating that negative interactions are paramount in restoration came directly from forestry science without first testing it in marine systems (Halpern et al., 2007). In contrast to forest restoration, recent work in salt marshes testing this paradigm in a restoration context found that the lack of positive species interactions incorporated into planting designs dramatically limited restoration success. Researchers found that outplants positioned next to, rather than further apart from other outplants—as called for by the current paradigm—increased restoration success by >100% with no extra cost (Silliman et al., 2015). Planting marsh grasses close to each other encouraged intraspecific facilitation whereby grass clumps were better able to resist wave erosion and low oxygen stress than individual outplants. Restoring other naturally occurring positive interactions may bring restored ecosystem functional processes closer to those of the target ecosystem, but for many coastal systems this has yet to be tested.
Several recent reviews have stressed the importance of incorporating positive species interactions into restoration because of their potential to increase project success. These reviews cover aquatic systems in general (Halpern et al., 2007) as well as mangrove (Gedan and Silliman, 2009; Renzi et al., 2019), coral (Shaver and Silliman, 2017), tidal marsh (Derksen-Hooijberg et al., 2018; Renzi et al., 2019), seagrass (Valdez et al., 2020), and terrestrial (Gómez-Aparicio et al., 2004; Gómez-Aparicio, 2009) systems. However, similar syntheses for oyster reef ecosystems are lacking.
Oyster reefs were once ubiquitous in many shallow water marine environments, replacing corals as the dominant reef forming ecosystem in higher latitude environments. However, over the past two centuries, more than 85% of oyster reefs around the world have been lost (Beck et al., 2011). In response, there has been a worldwide effort to restore shellfish reef ecosystems and the ecosystem services they provide, including water filtration, nutrient cycling, fish production, and shoreline protection (Fitzsimons et al., 2019; McLeod et al., 2019; Pogoda et al., 2019). Historically, restoration has focused on oyster population enhancement as a means to restore oyster reefs (Brumbaugh and Coen, 2009; Schulte et al., 2009), likely because oyster reefs were traditionally managed as a fishery rather than as an ecosystem (Coen and Luckenbach, 2000; Luckenbach et al., 2005). The field of oyster restoration primarily developed in the United States over the past 20 years (Luckenbach et al., 1999; Schrack et al., 2012) but has recently grown to encompass non-North American oyster species [e.g., Saccostrea glomerata, Ostrea edulis, Ostrea angasi, Magallana (Crassostrea) sikamea, Magallana (Crassostrea) hongkongensis], mussel species (e.g., Mytilus galloprovincialis and Perna canaliculus), and new geographic regions such as Australia, New Zealand, Europe, and Asia (Fitzsimons et al., 2019, 2020).
In this study, we systematically reviewed the scientific literature associated with the structure and function of oyster reef ecosystems to define and identify studies on positive species interactions that support key ecological processes. To do this, we took a whole system approach and examined both the benefits provided by other species to oysters and the benefits provided by oysters to other species, given that oyster reef restoration is not only undertaken to rejuvenate oyster populations themselves, but also for associated species that depend on oyster reefs. The ability of oysters to support other organisms is increasingly a primary reason why oyster reefs are restored, as oyster and associated species support ecosystem functions such as water filtration, fish production, and hard reef structure (Coen et al., 2007; Grabowski and Peterson, 2007; Grabowski et al., 2012; Kellogg et al., 2014; Zu Ermgassen et al., 2016). We define oyster ecosystems to include both structural forms of the ecosystem (i.e., largely two-dimensional oyster beds and higher profile reefs) and include all ecosystem-forming species described in the literature. The aim of this study was to synthesize any positive species interactions that have previously been identified in the oyster restoration literature to provide an overview of relevant positive interactions that could be useful to restoration practitioners. However, we do not rank the importance of these interactions, as each restoration project will have situational drivers that could result in different positive interactions being more or less important. We hope this systematic review will advance understanding of positive interactions and serve as a tool to improve ecosystem function outcomes of restoration projects and the management of wild oyster ecosystems.
Methods
We reviewed the scientific literature, following guidelines for preferred reporting items for systematic reviews and meta-analyses (PRISMA; Figure 1), by searching Web of Science and SCOPUS using the search string: TOPIC = [(oyster* OR “oyster reef*” “OR” “oyster bed*”) AND (restor* OR creat* OR rehabilit* OR reconstruct* OR recover* OR benefit* OR construct* OR “ecosystem service*” “OR” “ecosystem function*” OR facilitat*)]. Timespan: All Years (1945–2019); Indexes: SCI-EXPANDED, SSCI, A&HCI, ESCI, which returned 3,385 unique articles. We reviewed these articles at the title-, keyword-, and abstract-level to determine whether the article might involve a species interaction involving oysters. We only included studies that were field-, lab-, or modeling-based; reviews and meta-analyses were excluded (Figure 1—PRISMA flow diagram).
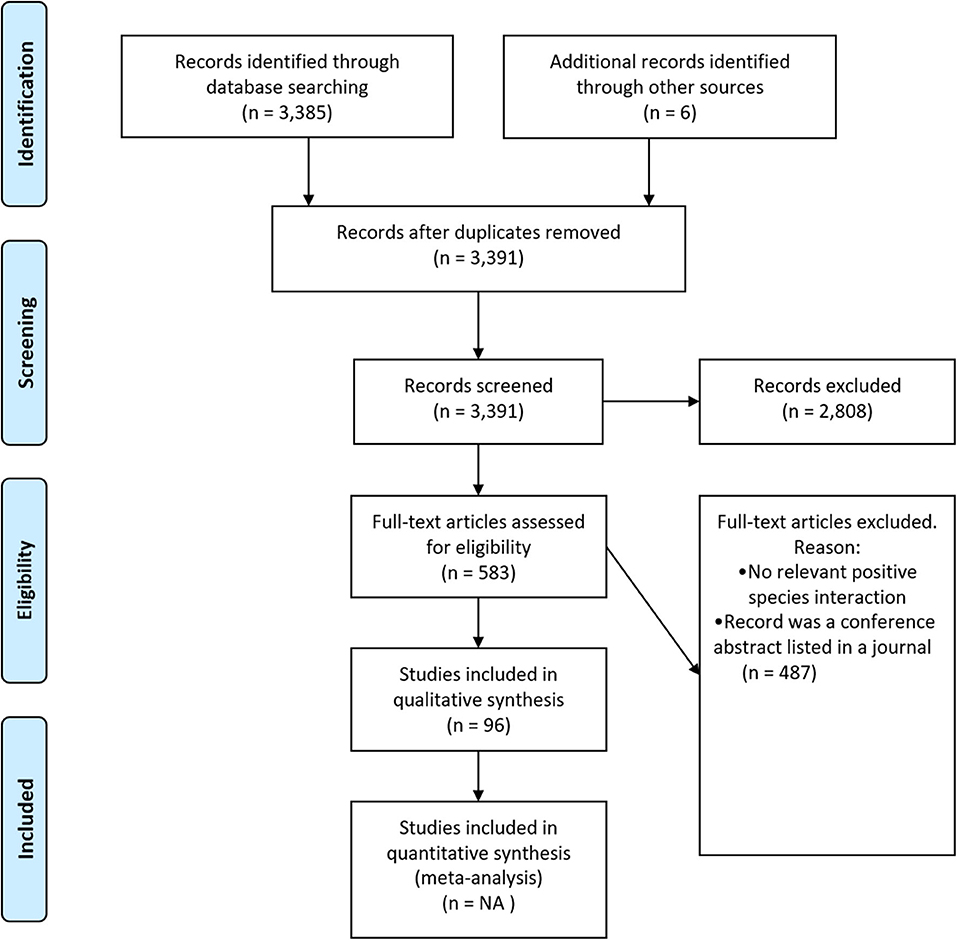
Figure 1. Systematic review flow diagram. The PRISMA (Preferred Reporting Items for Systematic Reviews and Meta-Analyses) flow diagram for the systematic review detailing the database searches, the number of abstracts screened, and the full texts retrieved.
Following the initial review, 583 articles remained, and these articles were screened at the full-text level (Figure 1—PRISMA flow diagram). Articles were included in the final database if: they examined a positive species interaction where oysters were involved directly or indirectly [e.g., oysters acting as refugia for other species (direct)/fish facilitate oysters by consuming shell crushing crabs (indirect)]. From each of those articles, we extracted: (1) the species of oyster involved; (2) where the study was conducted; (3) whether the research was a lab, field, or modeling study; (4) whether the study was intertidal or subtidal; (5) the life stages of the species involved; (6) whether the species involved were native or non-native; (7) what metric was used to assess species' benefit; (8) whether the interaction was mutually beneficial; (9) whether the authors found the relationship to be context-dependent (i.e., a positive relationship in some contexts and not in others); and (10) a short description of the interaction. An article could have multiple observations of positive interactions, if for example a positive interaction was studied in multiple experimental systems (i.e., a laboratory and field study) or if there were multiple positive interactions within a study. Each article was screened by at least two different authors for consistency.
After creating the database, we qualitatively screened the observations into seven categories of positive interactions (Figure 2, Table 1: Interaction category): (1) physical reef creation, (2) positive density dependence, (3) refugia from physical stress, (4) refugia from biological stress, (5) biodiversity enhancement, (6) settlement improvement, and (7) long-distance facilitation. For three of the categories (settlement improvement, refugia from physical stress, and refugia from biological stress), we also sub-categorized the positive interaction mechanism to capture additional information (Table 1: Interaction sub-category). “Settlement improvement” was broken down into two further sub-categories: substrate type and settlement cues. “Refugia from physical stress” was sub-categorized into five specific types of physical stress: thermal, flow, sediment, water chemistry, and water stress; and “refugia from biological stress” was broken down to trophic, competition, disease and nutrient stress. We also identified ten benefit outcomes from the partnerships (Table 1): (i) enhanced survival, (ii) enhanced growth, (iii) enhanced abundance of a single species or of many species (i.e., biodiversity), (iv) increased settlement, (v) improved filtration, (vi) reduced stress response, (vii) enhanced reproduction, (viii) reduced disease prevalence, (ix) improved reef structural integrity and (x) enhanced foraging. For a small number of studies, it was difficult to define a single positive interaction mechanism. For example, Aquino-Thomas and Proffitt (2014) found that red mangrove prop roots provided a good settlement surface for oysters, as prop roots provided a refuge from sedimentation and wave exposure during storms, improving survival of oysters. From this study three mechanisms were identified underlying two positive interactions: (1) “settlement improvement” by mangrove prop roots resulting in enhanced settlement; and (2) “refugia from physical stress” due to (a) refugia from sedimentation and (b) refugia from water flow both resulting in enhanced survival. As a result, the number of observations of positive interactions and mechanisms underlying these positive interactions may be greater than the total number of studies included in our review.
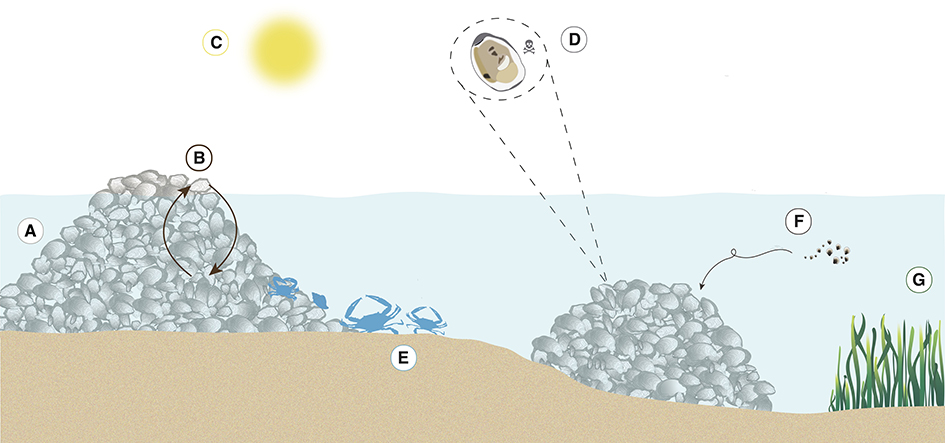
Figure 2. Schematic depiction of the seven categories of positive interactions on oyster reefs: (A) physical reef creation, (B) positive density dependence, (C) refugia from abiotic stress, (D) refugia from biotic stress, (E) biodiversity enhancement, (F) settlement improvement, and (G) long-distance facilitation.
Results and Discussion
Out of the 3,391 studies initially identified, we found 96 studies that reported positive interactions associated with oyster reef ecosystems, a result that is consistent with many similar syntheses on positive interactions from marsh, mangrove, kelp, and coral reef systems showing that positive interactions are relatively understudied (Halpern et al., 2007; Gedan and Silliman, 2009; Shaver and Silliman, 2017; Renzi et al., 2019; Valdez et al., 2020). Through these studies, we identified 199 observations of positive intra- and interspecific interactions between pairs of organisms. The effects of these positive species interactions included: enhanced survival and growth of species, increased abundance of species, promotion of settlement, improved water filtration, reduced stress to organisms, enhanced reproduction, reduced disease prevalence, improved reef structural integrity, and enhanced foraging.
Positive interactions were reported for oyster ecosystems in most geographic regions, with the notable exception of Africa, which had no relevant studies (Figure 3). There was a geographic concentration in the dataset, with the largest number of observations from North America (72%) and the fewest from South America (1%), excluding Africa (0%). Even when split into sub-regions (North Atlantic, Atlantic Bight, Chesapeake, Gulf of Mexico and West Coast), four of the five North American sub-regions still had more observations than any other continent. The Atlantic Bight region of the east coast of North America had the most observations (44 obs. from North Carolina, South Carolina, Georgia and Atlantic coast of Florida) followed by the Chesapeake Bay (32 obs. from Virginia and Maryland). This geographic bias may be in part because much of the research comes from “developed economies” with greater amounts of government funding available for conservation/restoration research and practice, in comparison to low/lower-middle income economies with fewer resources (James, 1999; Waldron et al., 2013). The geographic distribution of our results may also be skewed because we only used English language articles, which may underrepresent studies from non-English speaking regions that are published in native languages. Given the predominance of non-English languages in the regions outside of North America and Australia (i.e., South America, Europe, Asia, and Africa), and the bias of online databases to English language articles (Gates, 2002), the potential likelihood of missing articles is high. Encompassing non-English language studies, consulting with experts from all regions and including information on oyster restoration from the gray literature (e.g., monitoring and technical reports) would likely further improve our understanding of species interactions globally.
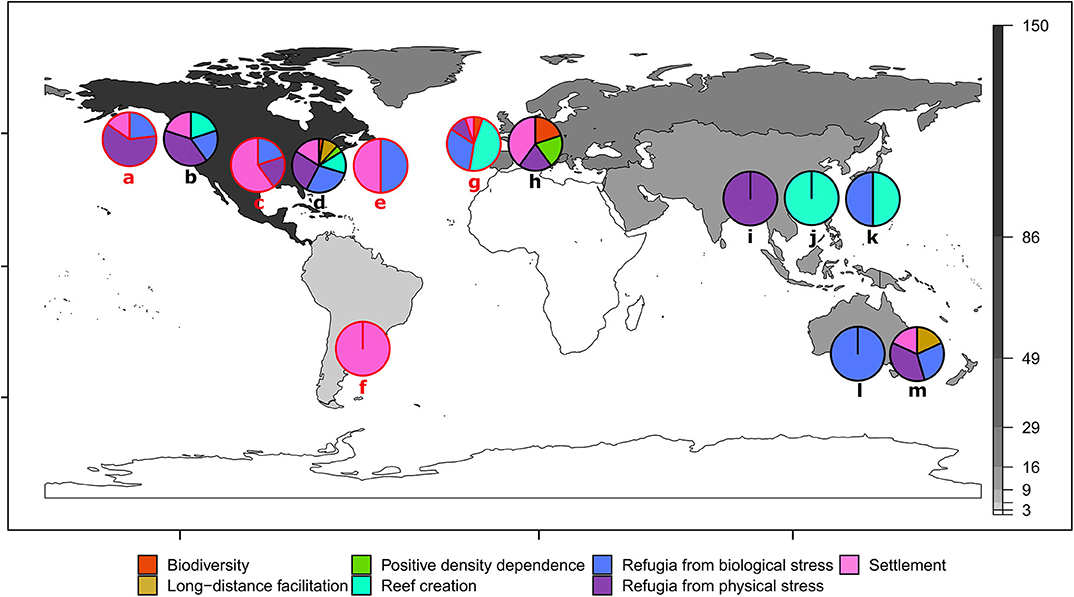
Figure 3. Positive interactions for shellfish restoration summarized for 10 ecosystem-forming oyster species. Continents are color coded by the number of positive species interactions synthesized from the review (e.g., black = 86–150 observations; and white = zero observations). Lower case letters indicate oyster species. Pie charts and letters in red (a, c, e, f, g) show non-native oyster taxa for that region. West coast of North America: (a) Crassostrea (Magallana) gigas, Pacific oyster–non-native (13 obs.); (b) O. lurida, Olympia oyster—native (10 obs.). East coast of North America: (c) Crassostrea (Magallana) ariakensis, Suminoe oyster–non-native (5 obs.); (d) Crassostrea virginica, Eastern oyster–native (118 obs.); (e) C. gigas, Pacific oyster–non-native (4 obs.). South America: (f) C. gigas, Pacific oyster–non-native (3 obs.). Europe: (g) C. gigas, Pacific oyster–non-native (19 obs.); (h) Ostrea edulis, European flat oyster (5 obs.). Asia: (i) Saccostrea culccullata, Natal oyster–native (6 obs.), (j) Crassostrea (Magallana) angulata, Portugeuse oyster–native (2 obs.); (k) C. gigas, Pacific oyster–native (2 obs.). Australasia and Oceania: (l) O. angasi, Australian flat oyster–native (1 obs.); (m) Saccostrea glomerata, Sydney rock oyster–native (11 obs.).
Broad Patterns in Positive Interactions for Oyster Reef Restoration
The oyster species with the greatest number of positive interactions reported was Crassostrea virginica (Figure 3d), which is likely due to the proportionally greater research effort for this species (56% of studies in the review). Comparatively, the species with the next greatest diversity of positive interactions and research effort was Magallena (Crossostrea) gigas–the Pacific oyster, which accounted for 25% of studies (Figures 3a,e–g). Most positive interactions observed for M. gigas were outside of its native range (e.g., North America and Europe). Our review shows that M. gigas, like other invasive foundation species (Ramus et al., 2017), can generate positive interactions outside of its native range, such as providing native bivalves in Europe (e.g., O. edulis and Mytilus edulis) with settlement substrate (Christianen et al., 2018) and refuge from predation (Markert et al., 2009). Positive interactions occur in oyster ecosystems globally and are evident for many reef-building oyster species, irrespective of whether the oyster is native or introduced (Figure 3).
Seventy-two percent (69 studies) of studies were undertaken directly in the field, as opposed to in the laboratory or modeled (Figure 4A). Out of the studies where tidal zonation was defined, 70% of observations were from intertidal studies (Figure 4B). This could support the ecological theory that positive interactions should be more prevalent under stressful physical conditions, such as in the intertidal where there are extreme daily temperature, light, and water level fluctuations (i.e., the stress gradient hypothesis SGH) (Bertness and Callaway, 1994; Stachowicz, 2001). Alternatively, it may simply be that intertidal shellfish systems are more accessible and more easily manipulated than subtidal systems, resulting in a greater number of intertidal studies. However, if positive interactions are indeed more important in the intertidal, which is supported by theory, incorporating positive interactions in the intertidal may be more important when restoring intertidal shellfish reefs than in the subtidal.
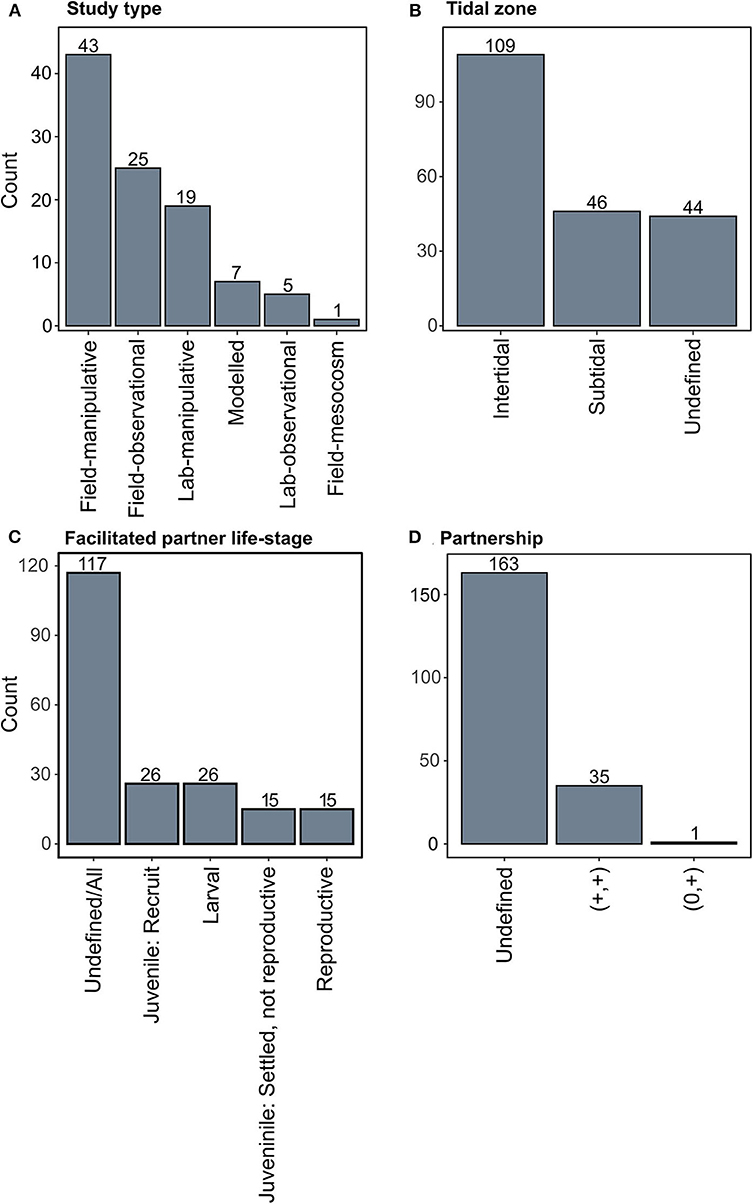
Figure 4. Summary of key parameters for positive interactions from oyster beds and reefs. (A) Studies that identified positive interactions summarized by study type, (B) the tidal zone where the positive species interaction occurred, (C) the life-stage of the facilitated partner, and (D) the type of partnership for each species interaction.
While many of the studies did not define the life-stage of the organism being facilitated, a large proportion of the observations that did identify the life-stage were sub-adults (i.e., larvae or recruits; Figure 4C). This could reflect a study bias toward early life stages, however it may also be that species early in their ontogeny will benefit more from positive interactions, as the benefits to early life stages are thought to diminish as juveniles become more hardy and resistant to physical stress, competition, or predation (Callaway and Walker, 1997; Stachowicz, 2001). Given that restoration projects try to promote target species settlement, an improved understanding of how positive species interactions affect early ontogenetic stages to promote the settlement of both the primary ecosystem engineers (i.e., oysters), and associated species will likely enhance the overall success of reef development.
Our review only found 35 studies that identified mutualistic relationships in oyster reef ecosystems (+,+; Figure 4D), which is likely because the majority of the studies reviewed only defined the effect of an interaction on one species. However, our review demonstrates that oysters facilitate many taxonomic groups, and in return are also facilitated by these groups (Figure 5). This under-identification of mutualisms could result from studies often focusing on understanding a single target species as opposed to understanding a two-way interaction between species. Alternatively, this finding could result from varied interaction outcomes at different life-stages, which can be difficult to observe in a single study. Further, if facilitations occur over spatial or temporal scales outside the range of an individual study, these mutualistic relationships could be missed and may only become apparent through synthesizing findings across multiple studies, as done here. Our results suggest that mutualistic relationships may be more prevalent in oyster ecosystems than previously accounted for and future research may uncover a greater diversity of mechanisms underpinning these mutualisms. Below we discuss in detail the importance of positive species interactions in oyster reef restoration using the seven categories defined in our study.
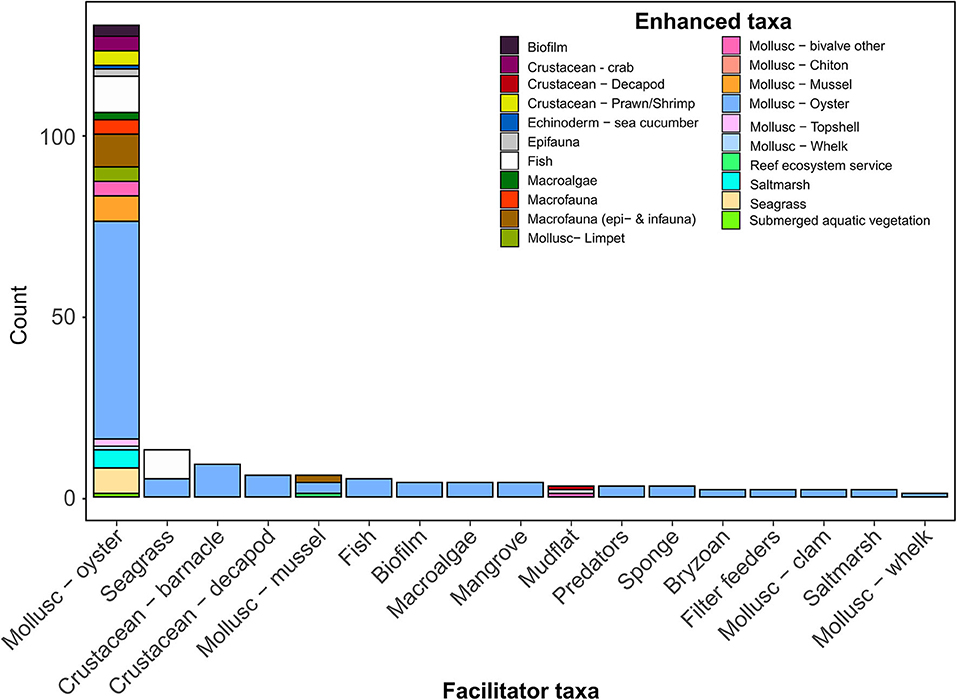
Figure 5. Positive species interactions on oyster reef ecosystems. Taxa on the x-axis facilitate and enhance other taxa identified by the colored fill of the stacked bars (Enhanced taxa), with the number of observations for each facilitator-enhanced taxa pairing (species interaction) on the y-axis. For example, the Mollusc-whelk and Mollusc-Oyster pairing (far right bar) shows one observation where this interaction was reported.
Intraspecific Facilitation: Oysters Helping Oysters
Our review found strong evidence that oysters facilitate the presence of other oysters (Figure 5), primarily through providing settlement cues (Tamburri et al., 2007, 2008), providing settlement substrate (Tamburri et al., 2008; Whitman and Reidenbach, 2012), and other positive density-dependent effects (Guy et al., 2019). Oysters, like other marine benthic invertebrates, are gregarious settlers. By preferentially settling onto aggregations of adult conspecifics, individuals increase their likelihood of successful fertilization and increase the likelihood that they settle in an area with favorable environmental conditions. Dense aggregations of oysters also perform better: they experience reduced predation pressure and exhibit higher filter-feeding efficiency. These fitness benefits of gregarious living can outweigh the negative effects of competition for limited resources (i.e., light, food, or space). Below we identify examples where positive intraspecific interactions led to improved fecundity and amelioration of external stressors, resulting in enhanced reproductive output, recruitment and survival of oysters (Figure 6).
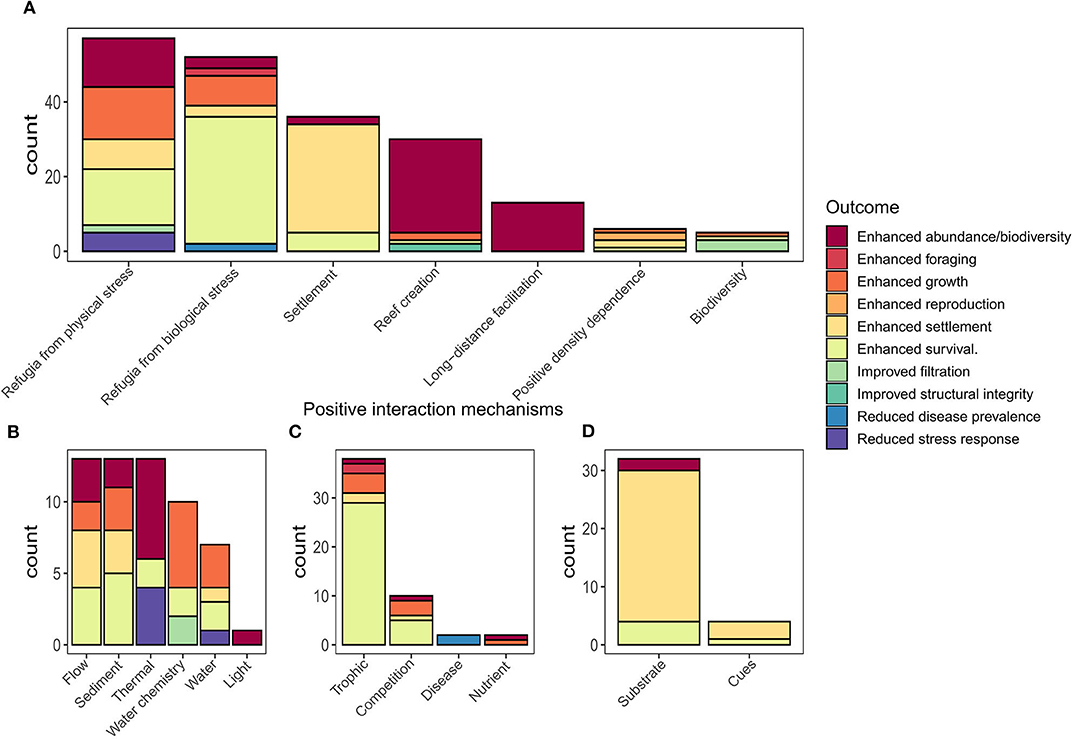
Figure 6. Outcomes of positive species interactions summarized by interaction mechanisms: (A) overview of positive interaction mechanisms by category, (B–D) display sub-categories for (B) refugia from physical stress; (C) refugia from biological stress; and (D) settlement categories individually summarized for sub-categories of positive species interactions. Note y-axis scales differ between plots.
Settlement Substrate
Restoration of degraded oyster reef habitat generally begins with the addition of substrate that serves as a reef base and site for oyster spat attachment. Years of oyster restoration practice has shown that selecting the right substrate is important for oyster recruitment and retention. Practitioners often use conspecific oyster or other bivalve shells as settlement substrate, as they emit chemical cues to attract and induce settlement of oysters and provide high surface complexity that is critical for survival of oyster larvae (Tamburri et al., 2008; Barnes et al., 2010; George et al., 2015). Our review identified 19 studies with 33 observations of positive interactions relating to substrate choice. In the majority of cases, the use of oyster shell material as a restoration substrate resulted in greater oyster settlement (Tamburri et al., 2008; White et al., 2009; Whitman and Reidenbach, 2012), and in fewer cases, enhanced survival of spat (Ruesink, 2007; Barnes et al., 2010) (Figure 6D).
Settlement Cues
Gregarious settlement in oysters is induced by settlement cues that enable pelagic larvae to recognize reef habitat and conspecifics, by sight, sound, or smell. Our review identified six studies with 11 observations of positive outcomes from provision of settlement cues (Figure 6D). Five of these studies identified positive interactions from conspecific olfactory cues, which enhanced settlement of oyster larvae. For example, Tamburri et al. (2007) showed that oyster larvae were attracted to the scent of conspecific adults. In still-water trials, larvae moved downward and settled after contacting a waterborne, adult chemical cue. For benthic filter feeders, this may seem to be a perilous choice given the potential risk of being cannibalized by adult conspecifics. However, for Pacific oysters, ciliary feeding currents were too weak to entrain conspecific larvae and larvae were only ingested when they landed directly on the gape region of a feeding oyster. This gape area only represents a small proportion of the total surface area of a reef, and thus the end result was that larvae responding to adult chemical cues were more likely to settle within hospitable habitat on the reef then be at risk of conspecific predation (Tamburri et al., 2007).
Our review also identified one study that examined the importance of auditory cues, (i.e., oyster reef soundscapes) for oyster settlement (Lillis et al., 2015). Many marine vertebrates (Simpson et al., 2005; Montgomery et al., 2006; Huijbers et al., 2012; Parmentier et al., 2015) and invertebrates (Vermeij et al., 2010; Lillis et al., 2015; Stanley et al., 2015) use sound as a directional cue to navigate toward settlement habitats or away from undesired habitats. Because of this reliance on auditory cues by many marine organisms, degraded soundscapes could hamper the recovery of ecosystems by directly attracting a lower number of propagules from critical foundation species (Rossi et al., 2017), such as coral and oyster larvae (Vermeij et al., 2010; Lillis et al., 2015). However, soundscapes can also play a role in restoring ecosystem function and recovering degraded systems. Lillis et al. (2015) demonstrated the utility of using recordings of oyster reef soundscapes (intermixed conspecific and heterospecific sounds) to attract larvae, induce settlement, and enhance recruitment of C. virginica.
Both olfactory and auditory cues from conspecifics are likely important for orientation and habitat selection by oyster larvae at different temporal and spatial scales. For this reason, restoration projects may benefit from manipulating both types of cues at restoration sites. For example, playback of healthy oyster soundscapes at restoration sites may enhance natural settlement of oysters and other reef organisms and facilitate recovery. Additionally, using oyster shell as substrate and seeding the target reef with a small number of adult oysters prior to spatfall, may ensure the presence of chemical conspecific cues important for attracting oyster larvae. Identifying the relative importance of substrate complexity, olfactory cues, and auditory cues for promoting settlement may further optimize restoration success, but more research is needed to determine the relative importance of these factors, as they may not generalize across oyster species.
Positive Density-Dependence
Seeding restoration sites with sufficient densities of adult oysters could improve restoration success in more ways than one. In many of the studies reviewed, we found the presence of adult oysters and the density of conspecifics facilitated oyster production, recruitment, and survival (Figure 6). Our review identified six examples of these positive density-dependent effects for oysters, where a positive relationship existed between the density of oysters on an established reef and the outcome measured (Figure 6). For example, for Ostrea edulis, Guy et al. (2019) identified a maximum distance to nearest conspecific neighbors of <1.5 m that resulted in greater production of brooded larvae compared to nearest neighbors >1.5 m. Considering the benefit of increased production on oyster restoration outcomes, this suggests a minimum stocking density should be applied for restoration of O. edulis, which may also be applicable for other brooding oyster species (e.g., O. angasi and O. chilensis). Given the importance of positive density dependence, where available, oyster restoration efforts should look to mix live oysters with dead shell instead of using dead shell alone.
Oysters Facilitate Biodiversity and Reef Communities Through Reef Creation
Positive interactions have the potential to cascade throughout the community, changing the presence and abundance of important species (e.g., increases in important fish species or decreases in oyster predators) (Stachowicz, 2001). Our study found that oysters are important for facilitating ecological assemblages through several processes defined below. While it is clear oysters increase diversity, it is less clear whether this diversity feeds back to facilitate oysters.
Creating Structure
Our review identified 21 studies with 37 observations (Figure 6) of positive interactions associated with the creation of reef structure. Two out of the 21 studies showed the importance of an inorganic-organic adhesive produced by live oysters that binds oyster shells to substrate, which is fundamental for creating a resilient and persistent reef structure (Burkett et al., 2010; Alberts et al., 2015). The remaining 19 studies demonstrated that using oyster shells as substrate enhanced resident invertebrates and fishes in reference to mudflats, tidal marshes, and seagrasses (Grabowski et al., 2005). For example, Lejart and Hily (2011) found that shell reefs added 3.97 and 3.87 m2 of additional surface area for rock and mudflats respectively, increasing habitat area for colonization by epifauna as compared to habitats without shell reefs.
Refuge From Physical Stress
The physical structure created by the oyster shell/live oyster matrix influences local environmental conditions, facilitating a suite of species by providing refugia from abiotic (i.e., thermal, flow, sediments, and water chemistry; Figure 6B) and biotic (i.e., competition, predation, and disease; Figure 6C) stressors. Our review identified 16 studies encompassing 57 interactions demonstrating facilitation of oysters and other taxa by the provision of refugia from abiotic stressors, including hydrodynamic, heat, sediment, salinity, oxygen, pH, light, and water stress (Figure 6D). The majority of these studies considered how altering the physical structure (orientation to flow, height, rugosity, and shape) of oyster shell reefs influenced these stressors and altered outcomes such as spat settlement density, oyster growth, and survival, as well as physiological stress and survival of associated invertebrates.
For instance, Lenihan (1999) examined how the height of shell reefs influenced water movement around reefs and oyster performance. This study found that flow speed increased, and sedimentation decreased, with elevation on a reef. Oyster recruitment was greatest at the front of reefs where flow was lowest, whilst growth, condition and survival of oysters increased with flow and elevation on a reef. Similarly, Colden et al. (2016) manipulated the orientation of oyster shell reefs to flow and found that reefs perpendicular to current flow produced conditions that were more favorable for reef persistence. Both Lenihan (1999) and Colden et al. (2016) stressed the importance of crests and vertical relief on reefs, suggesting that heterogeneous reefs will create favorable hydrodynamic conditions. They note that structure is critical for increasing flow, resuspending sediments, contributing to reef growth and ultimately determines whether reefs persist or degrade (Lenihan and Peterson, 1998; Lipcius et al., 2015; Colden et al., 2017). These studies show that intraspecific interactions between oysters (i.e., oyster density/height, reef shape) are important for oyster growth and should be considered in restoration designs.
Substrate orientation at the scale of individuals is also important for providing physical refugia. A number of studies identified the importance of shading by oysters, which influences the thermal conditions experienced by oysters and associated invertebrates (Padilla, 2010; McAfee et al., 2016, 2017, 2018; McAfee and Bishop, 2019). For instance, vertically oriented oysters provide greater shading than horizontally oriented oysters, which reduces heat and desiccation stress for other organisms (McAfee et al., 2018; McAfee and Bishop, 2019). These small-scale substrate orientation factors may be increasingly important in intertidal restoration settings where heat and desiccation stress will be highest.
Knowledge of how these positive interactions vary along an environmental gradient can help inform restoration practice. For example, across a 900 km latitudinal gradient, McAfee and Bishop (2019) identified that in warmer estuaries, shading by intertidal oysters had a larger effect on biodiversity, whereas in cooler estuaries, the provision of a predation refuge by oysters played a more important role. This demonstrates that context plays an important role in restoration planning and a project may benefit more or less from consideration of positive interactions depending on the type and amount of stress encountered in a system (e.g., an intertidal oyster restoration project in a hot arid climate vs. one in a cool temperate climate).
Interspecific Facilitation
Settlement
We found evidence that several species facilitate the presence of oysters and that the sequence of species colonization on reefs affects reef communities. For instance, Shelamoff et al. (2019) demonstrated that kelps can control turfing algae by abrading algae and reducing light levels, freeing space on the substrate and enhancing settlement density of Ostrea angasi. A number of other studies also reported that barnacles (Barnes et al., 2010), mangrove prop roots (Aquino-Thomas and Proffitt, 2014), kelps (Shelamoff et al., 2019), biofilms (Campbell et al., 2011), mobile invertebrates (Kimbro et al., 2014) and fishes can facilitate other species by enhancing surfaces for larval settlement (Table 1).
Refuge From Biological Stress
The stress gradient hypothesis predicts that refugia from biological stress—such as competition, predation, and disease—may be more important in more physically benign systems such as sub-tidal oyster reefs. Our review identified 29 studies encompassing 52 observations of organisms providing other organisms with refugia from biological stress (Figure 6). Of these observations 73% (38 obs.) involved trophic facilitations, 17% (10 obs.) involved refugia from competition, 3% (2 obs.) involved disease reduction, and 3% (2 obs.) involved the bio-availability of nutrients [i.e., oysters enriching nutrients in benthic sediments; Booth and Heck (2009)]. These positive interactions resulted in a diverse array of outcomes. Enhanced survival was most common 61% (28 obs.), followed by enhanced abundance/biodiversity 13% (6 obs.), enhanced growth (11%; 5 obs.), enhanced water quality 7% (3 obs.), enhanced settlement 4% (2 obs.), and reduced disease prevalence 4% (2 obs.) (Figure 6D).
Trophic facilitation is a form of indirect positive interaction, where one species is positively impacted through the feeding activities of another species. Many of the examples in our review were examples of trophic cascades where predators suppressed densities of primary consumers, resulting in increased densities of basal prey species. For example, oyster toadfish (Opsanus tau) enhance juvenile oyster survival by reducing mud crab (Panopeus herbstii) predation via non-consumptive (i.e., predation threat) and consumptive (i.e., predation) pathways. In this example the oyster toadfish indirectly increased oyster survivorship through reducing the number of oysters consumed by mud crabs (Grabowski, 2004; Grabowski et al., 2008; Kimbro et al., 2014, 2017). Similarly, native rock crabs control oyster drill populations, limiting oyster drill predation on Ostrea lurida and resulting in enhanced survival of O. lurida (Cheng and Grosholz, 2016). In addition to fishes and crabs, nudibranchs and sea urchins that eat boring sponges [e.g., the lemon drop nudibranch (Doriopsilla pharpa), and sea urchins (Arbucia punctulata and Lytechinus variegatus) (Lamarck), sea urchin Lytechinus variegatus (Lamarck)] can indirectly facilitate oysters by reducing boring sponge pressure on reefs (Guida, 1976).
Long-Distance Facilitation
Positive interactions can also occur at much larger spatial scales and involve species that are not in direct contact with oyster reefs. These long-distance positive interactions are often generated by the amelioration of physical and/or biological stress across landscapes (Van De Koppel et al., 2015). At the reef scale, oysters can have a significant impact on water quality by filter feeding, which in turn enriches surrounding benthic habitats with the deposition of organic rich bio-deposits such as feces and pseudofeces. For this reason, the potential of oyster reefs to enhance water quality has been the subject of extensive research and a motivating force for large-scale oyster restorations (Grizzle et al., 2008; Zu Ermgassen et al., 2013). Two studies in our review identified the capacity of oysters to filter pathogens out of the water column, reducing the impact of disease on oysters (Ben-Horin et al., 2018) and seagrasses (Groner et al., 2018). Benthic-pelagic coupling by oysters also enables the removal of nutrients from the water column and subsequent enrichment of sediments, which increases seagrass growth (Booth and Heck, 2009). Furthermore, our review also found examples of long distance interspecific facilitation enhancing the abundance of fish and invertebrate fauna on shellfish reefs when constructed in close proximity to seagrass, tidal marsh, and mudflat systems (Grabowski et al., 2005; Gilby et al., 2019), suggesting that a seascape view of habitat restoration could be beneficial for maximizing restoration success and outcomes for any particular ecosystem.
Modeling and field studies have demonstrated that oysters reduce suspended sediment concentrations dramatically, increasing water clarity and enhancing seagrass growth (Newell and Koch, 2004; Sharma et al., 2016). However, most restoration permitting frameworks have yet to recognize this potential interaction and restrict shellfish restoration in areas with seagrass. For example, development approval for shellfish restoration in many Australian states requires deployment of reef base to avoid areas of seagrass (i.e., >40% cover of Posidonia spp.) to limit potentially negative impacts to these vulnerable ecosystems. However, this results in restoration sites being sited in areas away from existing structured habitat (e.g., seagrass beds, rocky reefs), reducing the likelihood of long distance facilitation (e.g., water filtration and wave attenuation). Future research should focus on determining the value of co-restoring neighboring structured habitats and quantifying the value of long-distance facilitation between habitats. This research will build a knowledge base that can support restoration permitting frameworks to allow co-locating habitats and promote a seascape view of habitat restoration (i.e., restoration of multiple types of habitat patches across a seascape).
Biodiversity and Enhanced Ecosystem Function
Biodiversity encompasses the species, genetic, functional, and ecological diversity of living things (Shaver and Silliman, 2017). Recent evidence suggests that biodiversity often enhances ecosystem function, ecosystem service provisioning and ecosystem resilience (Van Wesenbeeck et al., 2013; Angelini et al., 2015). Ecologists refer to this as the portfolio effect, analogous to the portfolio concept in finance. In finance, the portfolio effect notes that diversified financial investment portfolios tend to produce more stable returns than simple portfolios. Similarly, in ecology the portfolio effect states that diversified ecosystems tend to produce higher functioning, more resilient ecosystems (Tilman et al., 1998; Schindler et al., 2015). This is partially because as diversity increases in an ecosystem, the functional redundancy (i.e., multiple species performing the same ecological function) often increases as well, which can lead to higher ecosystem resilience if one or a few species are lost (the insurance hypothesis: Yachi and Loreau, 1999).
While a number of key studies have documented both the loss of oyster reefs (Beck et al., 2011) and their value in terms of ecosystem services (Coen et al., 2007; Grabowski and Peterson, 2007; Grabowski et al., 2012; Zu Ermgassen et al., 2013, 2016), few have considered the role of multiple foundation species in supporting those ecosystem services. If multiple foundation species increase ecosystem services and/or biodiversity, accounting for their effects could alter the projected approach and benefits of restoration (Gedan et al., 2014). Several studies have shown positive relationships between hooked mussels (Ischadium recurvum) and eastern oysters (C. virginica) (Gedan et al., 2014; Lipcius and Burke, 2018). In terms of filtration, the hooked mussel shows some niche overlap with oysters, particularly for larger size classes of phytoplankton, but the hooked mussel can filter picoplankton more efficiently than the eastern oyster (Fulford et al., 2007; Gedan et al., 2014). Thus, while they may compete at times or in places where phytoplankton resources are limiting, both species can contribute significantly to the improvement of water quality by oyster reef restoration (Gedan et al., 2014). Multiple foundation species also produce habitat on oyster reefs, as associated sessile marine taxa (i.e., sponges, barnacles and algae) aid in binding the reef together and in supplementing the reef matrix, facilitating oyster settlement and enhancing the diversity of reef associated species (Manley et al., 2010).
Ecological restoration often focuses on a single ecosystem engineering species, with many restoration projects using a single species to form an entire ecological community. Oyster restoration traditionally focuses on the restoration of a single species of oyster to create an entire reef and deliver a suite of ecosystem services. However, our review suggests that considering multiple native foundation species will help support a self-sustaining oyster population, control oyster competitors, and boost filtration. The resulting increases in diversity should also support resilient ecosystem functioning and enhance the delivery of ecosystem services.
Future research should examine the role of multiple foundation species in accelerating the rate or trajectory of restoration projects [i.e., Williams et al. (2017) for tropical seagrass] and the importance of multiple taxa in delivering ecosystem services. However, care has to be taken in the selection of the multiple foundations species, as the aim is not to create a novel ecosystem by creating communities of foundation species that do not naturally co-exist, but to restore an ecosystem that occurred in the past. Achieving this outcome is aided by the use of a model system for the local native ecosystem being restored (McDonald et al., 2016; Gillies et al., 2017).
Conclusion
Our review intentionally focused on positive interactions and therefore did not report neutral or negative interactions. We acknowledge that in order to understand functioning of ecosystems both positive and negative interactions should be considered collectively. Yet in order to do so, further research on positive interactions is needed to provide a more complete picture of species interactions and complement existing research on negative interactions. While we make several recommendations for improving restoration practice in this review, we acknowledge further research may be needed to justify their wide-spread use (Table 1; e.g., playback of healthy oyster soundscapes at restoration sites to enhance natural settlement of oysters). In all instances, relevance to the particular restoration context should be considered and tested by appropriate experimental designs (i.e., Before After Control Impact—BACI) before scaling such novel enhancement methods. Further, future work might benefit from understanding the magnitude and effect size of positive interactions as this may aid outcomes of restoration projects by enabling prioritization of practical interventions that apply positive interactions.
Reef building oysters are autogenic ecosystem engineers that create their own habitat. Reef-building oysters make the environment less stressful for themselves, but also for many other species, creating a diverse ecological community that collectively produces important ecosystem services like coastal protection, water filtration, and fish production. To keep oyster predators, competitors and abiotic stressors low, in turn, the oysters depend on the reef inhabitants that they shelter. This review emphasizes the importance of positive interactions for not only oyster populations, but also for the biodiversity and resultant ecosystem functions and services that oysters engineer. By exploring nearly 200 examples of positive species interactions from 96 scientific studies, our review and database (available at the Knowledge Network for Biocomplexity. doi: 10.5063/F1125R1W) provides a tool for oyster reef restoration practitioners and scientists to explore the importance of positive interactions for creating and maintaining oyster ecosystems. These results can be used to improve our understanding of how these interactions can be applied to enhance outcomes of restoration projects and the management of wild oyster ecosystems.
Data Availability Statement
Publicly available datasets were analyzed in this study. This data can be found here: The dataset generated for this study can be found in the Knowledge Network for Biocomplexity “doi: 10.5063/F1125R1W”.
Author Contributions
SR, JR, CG, BH, and BS conceptualized the manuscript. SR, JR, and EF undertook the review. SR analysed the database and produced Figures 1, 3–6. JR produced Figure 2. SR, JR, EF, and CG wrote the initial draft and all authors edited and reviewed the manuscript.
Funding
BS was supported by a Lenfest Ocean Program Grant and also received support as the CSIRO Distinguished Fulbright Chair in Science and Technology. JR was supported by a US National Science Foundation Graduate Research Fellowship (NSF Grant DGE-1644868) and Duke University.
Conflict of Interest
The authors declare that the research was conducted in the absence of any commercial or financial relationships that could be construed as a potential conflict of interest.
Supplementary Material
The Supplementary Material for this article can be found online at: https://www.frontiersin.org/articles/10.3389/fmars.2020.00656/full#supplementary-material
References
Alberts, E. M., Taylor, S. D., Edwards, S. L., Sherman, D. M., Huang, C. P., Kenny, P., et al. (2015). Structural and compositional characterization of the adhesive produced by reef building oysters. ACS Appl. Mater. Interfaces 7, 8533–8538. doi: 10.1021/acsami.5b00287
Angelini, C., Van Der Heide, T., Griffin, J. N., Morton, J. P., Derksen-Hooijberg, M., Lamers, L. P., et al. (2015). Foundation species' overlap enhances biodiversity and multifunctionality from the patch to landscape scale in southeastern United States salt marshes. Proc. R. Soc. B Biol. Sci. 282:20150421. doi: 10.1098/rspb.2015.0421
Aquino-Thomas, J., and Proffitt, C. E. (2014). Oysters Crassostrea virginica on red mangrove Rhizophora mangle prop roots: facilitation of one foundation species by another. Mar. Ecol. Prog. Ser. 503, 177–194. doi: 10.3354/meps10742
Barnes, B. B., Luckenbach, M. W., and Kingsley-Smith, P. R. (2010). Oyster reef community interactions: the effect of resident fauna on oyster (Crassostrea spp.) larval recruitment. J. Exp. Mar. Biol. Ecol. 391, 169–177. doi: 10.1016/j.jembe.2010.06.026
Beck, M. W., Brumbaugh, R. D., Airoldi, L., Carranza, A., Coen, L. D., Crawford, C., et al. (2011). Oyster reefs at risk and recommendations for conservation, restoration, and management. Bioscience 61, 107–116. doi: 10.1525/bio.2011.61.2.5
Ben-Horin, T., Burge, C. A., Bushek, D., Groner, M. L., Proestou, D. A., Huey, L. I., et al. (2018). Intensive oyster aquaculture can reduce disease impacts on sympatric wild oysters. Aquacult. Environ. Interact. 10, 557–567. doi: 10.3354/aei00290
Bertness, M. D., and Callaway, R. (1994). Positive interactions in communities. Trends Ecol. Evol. 9, 191–193. doi: 10.1016/0169-5347(94)90088-4
Bertness, M. D., and Leonard, G. H. (1997). The role of positive interactions in communities: lessons from intertidal habitats. Ecology 78, 1976–1989. doi: 10.1890/0012-9658(1997)078[1976:TROPII]2.0.CO;2
Booth, D. M., and Heck, K. L. (2009). Effects of the American oyster Crassostrea virginica on growth rates of the seagrass Halodule wrightii. Mar. Ecol. Prog. Ser. 389, 117–126. doi: 10.3354/meps08163
Brumbaugh, R. D., and Coen, L. D. (2009). Contemporary approaches for small-scale oyster reef restoration to address substrate versus recruitment limitation: a review and comments relevant for the olympia oyster, Ostrea lurida carpenter 1864. J. Shellfish Res. 28, 147–161. doi: 10.2983/035.028.0105
Bruno, J. F., Stachowicz, J. J., and Bertness, M. D. (2003). Inclusion of facilitation into ecological theory. Trends Ecol. Evol. 18, 119–125. doi: 10.1016/S0169-5347(02)00045-9
Burkett, J. R., Hight, L. M., Kenny, P., and Wilker, J. J. (2010). Oysters produce an organic-inorganic adhesive for intertidal reef construction. J. Am. Chem. Soc. 132, 12531–12533. doi: 10.1021/ja104996y
Callaway, R. M., and Walker, L. R. (1997). Competition and facilitation: a synthetic approach to interactions in plant communities. Ecology 78, 1958–1965. doi: 10.1890/0012-9658(1997)078[1958:CAFASA]2.0.CO;2
Campbell, A. H., Meritt, D. W., Franklin, R. B., Boone, E. L., Nicely, C. T., and Brown, B. L. (2011). Effects of age and composition of field-produced biofilms on oyster larval setting. Biofouling 27, 255–265. doi: 10.1080/08927014.2011.560384
Cheng, B. S., and Grosholz, E. D. (2016). Environmental stress mediates trophic cascade strength and resistance to invasion. Ecosphere 7:e01247. doi: 10.1002/ecs2.1247
Christianen, M. J. A., Lengkeek, W., Bergsma, J. H., Coolen, J. W. P., Didderen, K., Dorenbosch, M., et al. (2018). Return of the native facilitated by the invasive? Population composition, substrate preferences and epibenthic species richness of a recently discovered shellfish reef with native European flat oysters (Ostrea edulis) in the North Sea. Mar. Biol. Res. 14, 590–597. doi: 10.1080/17451000.2018.1498520
Coen, L. D., Brumbaugh, R. D., Bushek, D., Grizzle, R., Luckenbach, M. W., Posey, M. H., et al. (2007). Ecosystem services related to oyster restoration. Mar. Ecol. Prog. Ser. 341, 303–307. doi: 10.3354/meps341303
Coen, L. D., and Luckenbach, M. W. (2000). Developing success criteria and goals for evaluating oyster reef restoration: ecological function or resource exploitation? Ecol. Eng. 15, 323–343. doi: 10.1016/S0925-8574(00)00084-7
Colden, A. M., Fall, K. A., Cartwright, G. M., and Friedrichs, C. T. (2016). Sediment suspension and deposition across restored oyster reefs of varying orientation to flow: implications for restoration. Estuaries Coasts 39, 1435–1448. doi: 10.1007/s12237-016-0096-y
Colden, A. M., Latour, R. J., and Lipcius, R. N. (2017). Reef height drives threshold dynamics of restored oyster reefs. Mar. Ecol. Prog. Ser. 582, 1–13. doi: 10.3354/meps12362
Connell, J. H. (1956). A study of some of the factors which determine the density and survival of natural populations of the intertidal barnacle, Balanus balanoides (L.) (Ph.D. thesis). University of Glasgow, Glasgow, Scotland.
Darwin, C. (1859). On the Origin of Species by Means of Natural Selection or the Preservation of Favoured Races in the Struggle for Life. London: H. Milford, Oxford University Press. doi: 10.5962/bhl.title.68064
Derksen-Hooijberg, M., Angelini, C., Lamers, L. P. M., Borst, A., Smolders, A., Hoogveld, J. R. H., et al. (2018). Mutualistic interactions amplify saltmarsh restoration success. J. Appl. Ecol. 55, 405–414. doi: 10.1111/1365-2664.12960
Fitzsimons, J. A., Branigan, S., Brumbaugh, R. D., McDonald, T., and Zu Ermgassen, P. S. E. (Eds.). (2019). Restoration Guidelines for Shellfish Reefs. Arlington, VA: The Nature Conservancy.
Fitzsimons, J. A., Branigan, S., Gillies, C. L., Brumbaugh, R. D., Cheng, J., Deangelis, B. M., et al. (2020). Restoring shellfish reefs: global guidelines for practitioners and scientists. Conserv. Sci. Prac. 2:e198. doi: 10.1111/csp2.198
Fulford, R. S., Breitburg, D. L., Newell, R. I., Kemp, W. M., and Luckenbach, M. (2007). Effects of oyster population restoration strategies on phytoplankton biomass in chesapeake bay: a flexible modeling approach. Mar. Ecol. Prog. Ser. 336, 43–61. doi: 10.3354/meps336043
Gates, S. (2002). Review of methodology of quantitative reviews using meta-analysis in ecology. J. Anim. Ecol. 71, 547–557. doi: 10.1046/j.1365-2656.2002.00634.x
Gedan, K. B., Kellogg, L., and Breitburg, D. L. (2014). Accounting for multiple foundation species in oyster reef restoration benefits. Restor. Ecol. 22, 517–524. doi: 10.1111/rec.12107
Gedan, K. B., and Silliman, B. R. (2009). Using facilitation theory to enhance mangrove restoration. AMBIO 38:109. doi: 10.1579/0044-7447-38.2.109
George, L. M., De Santiago, K., Palmer, T. A., and Pollack, J. B. (2015). Oyster reef restoration: effect of alternative substrates on oyster recruitment and nekton habitat use. J. Coastal Conserv. 19, 13–22. doi: 10.1007/s11852-014-0351-y
Gilby, B., Olds, A. D., Henderson, C. J., Ortodossi, N. L., Connolly, R., and Schlacher, T. A. (2019). Seascape context modifies how fish respond to restored oyster reef structures. ICES J. Mar. Sci. 76, 1131–1139. doi: 10.1093/icesjms/fsz019
Gillies, C. L., Crawford, C., and Hancock, B. (2017). Restoring angasi oyster reefs: what is the endpoint ecosystem we are aiming for and how do we get there? Ecol. Manag. Restor. 18, 214–222. doi: 10.1111/emr.12278
Gómez-Aparicio, L. (2009). The role of plant interactions in the restoration of degraded ecosystems: a meta-analysis across life-forms and ecosystems. J. Ecol. 97, 1202–1214. doi: 10.1111/j.1365-2745.2009.01573.x
Gómez-Aparicio, L., Zamora, R., Gómez, J. M., Hódar, J. A., Castro, J., and Baraza, E. (2004). Applying plant facilitation to forest restoration: a meta-analysis of the use of shrubs as nurse plants. Ecol. Appl. 14, 1128–1138. doi: 10.1890/03-5084
Grabowski, J. H. (2004). Habitat complexity disrupts predator-prey interactions but not the trophic cascade on oyster reefs. Ecology 85, 995–1004. doi: 10.1890/03-0067
Grabowski, J. H., Brumbaugh, R. D., Conrad, R. F., Keeler, A. G., Opaluch, J. J., Peterson, C. H., et al. (2012). Economic valuation of ecosystem services provided by oyster reefs. BioScience 62, 900–909. doi: 10.1525/bio.2012.62.10.10
Grabowski, J. H., Hughes, A. R., and Kimbro, D. L. (2008). Habitat complexity influences cascading effects of multiple predators. Ecology 89, 3413–3422. doi: 10.1890/07-1057.1
Grabowski, J. H., Hughes, A. R., Kimbro, D. L., and Dolan, M. A. (2005). How habitat setting influences restored oyster reef communities. Ecology 86, 1926–1935. doi: 10.1890/04-0690
Grabowski, J. H., and Peterson, C. H. (2007). Restoring oyster reefs to recover ecosystem services. Ecosys. Eng. Plants Protists 4, 281–298. doi: 10.1016/S1875-306X(07)80017-7
Grizzle, R. E., Greene, J. K., and Coen, L. D. (2008). Seston removal by natural and constructed intertidal eastern oyster (Crassostrea virginica) reefs: a comparison with previous laboratory studies, and the value of in situ methods. Estuaries Coasts 31, 1208–1220. doi: 10.1007/s12237-008-9098-8
Groner, M. L., Burge, C. A., Cox, R., Rivlin, N. D., Turner, M., Van Alstyne, K. L., et al. (2018). Oysters and eelgrass: potential partners in a high pCO2 ocean. Ecology 99, 1802–1814. doi: 10.1002/ecy.2393
Guida, V. G. (1976). Sponge predation in the oyster reef community as demonstrated with Cliona celata grant. J. Exp. Mar. Biol. Ecol. 25, 109–122. doi: 10.1016/0022-0981(76)90012-5
Guy, C., Smyth, D., and Roberts, D. (2019). The importance of population density and inter-individual distance in conserving the European oyster Ostrea edulis. J. Mar. Biol. Assoc. U. K. 99, 587–593. doi: 10.1017/S0025315418000395
Halpern, B. S., Silliman, B. R., Olden, J. D., Bruno, J. P., and Bertness, M. D. (2007). Incorporating positive interactions in aquatic restoration and conservation. Front. Ecol. Environ. 5, 153–160. doi: 10.1890/1540-9295(2007)5[153:IPIIAR]2.0.CO;2
He, Q., Bertness, M. D., and Altieri, A. H. (2013). Global shifts towards positive species interactions with increasing environmental stress. Ecol. Lett. 16, 695–706. doi: 10.1111/ele.12080
Hughes, A. R., Hanley, T. C., Byers, J. E., Grabowski, J. H., Mccrudden, T., Piehler, M. F., et al. (2019). Genetic diversity and phenotypic variation within hatchery-produced oyster cohorts predict size and success in the field. Ecol. Appl. 29:e01940. doi: 10.1002/eap.1940
Huijbers, C. M., Nagelkerken, I., Lössbroek, P. A., Schulten, I. E., Siegenthaler, A., Holderied, M. W., et al. (2012). A test of the senses: fish select novel habitats by responding to multiple cues. Ecology 93, 46–55. doi: 10.1890/10-2236.1
Jordan, W. R., Gilpin, M. E., and Aber, J. D. (1987). “Restoration ecology: ecological restoration as a technique for basic research,” in Restoration Ecology: A Synthetic Approach to Ecological Research, ed W. R. Jordan (Cambridge: Cambridge University Press), 3–23.
Kellogg, M. L., Smyth, A. R., Luckenbach, M. W., Carmichael, R. H., Brown, B. L., Cornwell, J. C., et al. (2014). Use of oysters to mitigate eutrophication in coastal waters. Estuarine Coastal Shelf Sci. 151, 156–168. doi: 10.1016/j.ecss.2014.09.025
Kimbro, D. L., Byers, J. E., Grabowski, J. H., Hughes, A. R., and Piehler, M. F. (2014). The biogeography of trophic cascades on US oyster reefs. Ecol. Lett. 17, 845–854. doi: 10.1111/ele.12293
Kimbro, D. L., Grabowski, J. H., Hughes, A. R., Piehler, M. F., and White, J. W. (2017). Nonconsumptive effects of a predator weaken then rebound over time. Ecology 98, 656–667. doi: 10.1002/ecy.1702
Lejart, M., and Hily, C. (2011). Differential response of benthic macrofauna to the formation of novel oyster reefs (Crassostrea gigas, Thunberg) on soft and rocky substrate in the intertidal of the Bay of Brest, France. J. Sea Res. 65, 84–93. doi: 10.1016/j.seares.2010.07.004
Lenihan, H. S. (1999). Physical–biological coupling on oyster reefs: how habitat structure influences individual performance. Ecol. Monogr. 69, 251–275. doi: 10.1890/0012-9615(1999)069[0251:PBCOOR]2.0.CO;2
Lenihan, H. S., and Peterson, C. H. (1998). How habitat degradation through fishery disturbance enhances impacts of hypoxia on oyster reefs. Ecol. Appl. 8, 128–140. doi: 10.1890/1051-0761(1998)008[0128:HHDTFD]2.0.CO;2
Lillis, A., Bohnenstiehl, D. R., and Eggleston, D. B. (2015). Soundscape manipulation enhances larval recruitment of a reef-building mollusk. PeerJ. 3:e999. doi: 10.7717/peerj.999
Lipcius, R., Burke, R., McCulloch, D., Schreiber, S., Schulte, D., Seitz, R., et al. (2015). Overcoming restoration paradigms: value of the historical record and metapopulation dynamics in native oyster restoration. Front. Mar. Sci. 2:65. doi: 10.3389/fmars.2015.00065
Lipcius, R. N., and Burke, R. P. (2018). Successful recruitment, survival and long-term persistence of eastern oyster and hooked mussel on a subtidal, artificial restoration reef system in Chesapeake Bay. PLoS ONE 13:e0204329. doi: 10.1371/journal.pone.0204329
Luckenbach, M. W., Coen, L. D., Ross, P. G., and Stephen, J. A. (2005). Oyster reef habitat restoration: relationships between oyster abundance and community development based on two studies in Virginia and South Carolina. J. Coastal Res. 40, 64–78. Available online at: www.jstor.org/stable/25736616
Luckenbach, M. W., Mann, R., and Wesson, J. A. (1999). Oyster Reef Habitat Restoration: A Synopsis and Synthesis of Approaches; Proceedings From the Symposium, Williamsburg, Virginia, April 1995. Virginia Institute of Marine Science, College of William and Mary.
Manley, J., Power, A., Walker, R., Hurley, D., Belcher, C., and Richardson, J. (2010). Ecological succession on restored intertidal oyster habitat in the tidal creeks of coastal Georgia. J. Shellfish Res. 29, 917–926. doi: 10.2983/035.029.0424
Markert, A., Wehrmann, A., and Kröncke, I. (2009). Recently established Crassostrea-reefs versus native Mytilus-beds: differences in ecosystem engineering affects the macrofaunal communities (Wadden Sea of Lower Saxony, southern German Bight). Biol. Invasions 12:15. doi: 10.1007/s10530-009-9425-4
McAfee, D., and Bishop, M. J. (2019). The mechanisms by which oysters facilitate invertebrates vary across environmental gradients. Oecologia 189, 1095–1106. doi: 10.1007/s00442-019-04359-3
McAfee, D., Bishop, M. J., Yu, T. N., and Williams, G. A. (2018). Structural traits dictate abiotic stress amelioration by intertidal oysters. Funct. Ecol. 32, 2666–2677. doi: 10.1111/1365-2435.13210
McAfee, D., Cole, V. J., and Bishop, M. J. (2016). Latitudinal gradients in ecosystem engineering by oysters vary across habitats. Ecology 97, 929–939. doi: 10.1890/15-0651.1
McAfee, D., O'connor, W. A., and Bishop, M. J. (2017). Fast-growing oysters show reduced capacity to provide a thermal refuge to intertidal biodiversity at high temperatures. J. Anim. Ecol. 86, 1352–1362. doi: 10.1111/1365-2656.12757
McDonald, T., Gann, G., Jonson, J., and Dixon, K. (2016). International Standards for the Practice of Ecological Restoration–Including Principles and Key Concepts. Society for Ecological Restoration, Washington, DC. doi: 10.1111/rec.12359
McLeod, I. M., Zu Ermgassen, P. S. E., Gillies, C. L., Hancock, B., and Humphries, A. (2019). “Chapter 25: Can bivalve habitat restoration improve degraded estuaries?” in Coasts and Estuaries, eds E. Wolanski, J. W. Day, M. Elliott, and R. Ramachandran (Elsevier), 427–442. doi: 10.1016/B978-0-12-814003-1.00025-3
Montgomery, J. C., Jeffs, A., Simpson, S. D., Meekan, M., and Tindle, C. (2006). Sound as an orientation cue for the pelagic larvae of reef fishes and decapod crustaceans. Adv. Mar. Biol. 51, 143–196. doi: 10.1016/S0065-2881(06)51003-X
Newell, R. I., and Koch, E. W. (2004). Modeling seagrass density and distribution in response to changes in turbidity stemming from bivalve filtration and seagrass sediment stabilization. Estuaries 27, 793–806. doi: 10.1007/BF02912041
Padilla, D. K. (2010). Context-dependent impacts of a non-native ecosystem engineer, the Pacific oyster Crassostrea gigas. Integr. Comp. Biol. 50, 213–225. doi: 10.1093/icb/icq080
Paine, R. T. (1965). Natural history, limiting factors and energetics of the opisthobranch Navanax inermis. Ecology 46, 603–619. doi: 10.2307/1935000
Parmentier, E., Berten, L., Rigo, P., Aubrun, F., Nedelec, S., Simpson, S., et al. (2015). The influence of various reef sounds on coral-fish larvae behaviour. J. Fish Biol. 86, 1507–1518. doi: 10.1111/jfb.12651
Pogoda, B., Brown, J., Hancock, B., Preston, J., Pouvreau, S., Kamermans, P., et al. (2019). The Native oyster restoration alliance (NORA) and the Berlin oyster recommendation: bringing back a key ecosystem engineer by developing and supporting best practice in Europe. Aquat. Living Resour. 32:13. doi: 10.1051/alr/2019012
Ramus, A. P., Silliman, B. R., Thomsen, M. S., and Long, Z. T. (2017). An invasive foundation species enhances multifunctionality in a coastal ecosystem. Proc. Natl. Acad. Sci. U.S.A. 114, 8580–8585. doi: 10.1073/pnas.1700353114
Renzi, J. J., He, Q., and Silliman, B. R. (2019). Harnessing positive species interactions to enhance coastal wetland restoration. Front. Ecol. Evol. 7:131. doi: 10.3389/fevo.2019.00131
Rossi, T., Connell, S. D., and Nagelkerken, I. (2017). The sounds of silence: regime shifts impoverish marine soundscapes. Landscape Ecol. 32, 239–248. doi: 10.1007/s10980-016-0439-x
Ruesink, J. L. (2007). Biotic resistance and facilitation of a non-native oyster on rocky shores. Mar. Ecol. Prog. Ser. 331, 1–9. doi: 10.3354/meps331001
Schindler, D. E., Armstrong, J. B., and Reed, T. E. (2015). The portfolio concept in ecology and evolution. Front. Ecol. Environ. 13:140275. doi: 10.1890/140275
Schrack, E., Beck, M. W., Brumbaugh, R. D., Crisley, K., and Hancock, B. (2012). Restoration Works: Highlights From a Decade of Partnership Between the Nature Conservancy and the National Oceanic and Atmospheric Administration's Restoration Center. The Nature Conservancy, Arlington, VA.
Schulte, D. M., Burke, R. P., and Lipcius, R. N. (2009). Unprecedented restoration of a native oyster metapopulation. Science 325, 1124–1128. doi: 10.1126/science.1176516
Sharma, S., Goff, J., Moody, R. M., Byron, D., Heck, K. L. Jr., Powers, S. P., et al. (2016). Do restored oyster reefs benefit seagrasses? An experimental study in the Northern Gulf of Mexico. Restor. Ecol. 24, 306–313. doi: 10.1111/rec.12329
Shaver, E. C., and Silliman, B. R. (2017). Time to cash in on positive interactions for coral restoration. PeerJ. 5:e3499. doi: 10.7717/peerj.3499
Shelamoff, V., Layton, C., Tatsumi, M., Cameron, M. J., Wright, J. T., and Johnson, C. R. (2019). Ecosystem engineering by a canopy-forming kelp facilitates the recruitment of native oysters. Restor. Ecol. 27, 1442–1451. doi: 10.1111/rec.13019
Silliman, B. R., Bertness, M. D., Altieri, A. H., Griffin, J. N., Bazterrica, M. C., Hidalgo, F. J., et al. (2011). Whole-community facilitation regulates biodiversity on patagonian rocky shores. PLoS ONE 6:e24502. doi: 10.1371/journal.pone.0024502
Silliman, B. R., and He, Q. (2018). Physical stress, consumer control, and new theory in ecology. Trends Ecol. Evol. 33, 492–503. doi: 10.1016/j.tree.2018.04.015
Silliman, B. R., McCoy, M. W., Angelini, C., Holt, R. D., Griffin, J. N., and Van De Koppel, J. (2013). Consumer fronts, global change, and runaway collapse in ecosystems. Annu. Rev. Ecol. Evol. Syst. 44, 503–538. doi: 10.1146/annurev-ecolsys-110512-135753
Silliman, B. R., Schrack, E., He, Q., Cope, R., Santoni, A., Van Der Heide, T., et al. (2015). Facilitation shifts paradigms and can amplify coastal restoration efforts. Proc. Natl. Acad. Sci. U.S.A. 112, 14295–14300. doi: 10.1073/pnas.1515297112
Simpson, S. D., Meekan, M., Montgomery, J., McCauley, R., and Jeffs, A. (2005). Homeward sound. Science 308:221. doi: 10.1126/science.1107406
Stachowicz, J. J. (2001). Mutualism, facilitation, and the structure of ecological communities: positive interactions play a critical, but underappreciated, role in ecological communities by reducing physical or biotic stresses in existing habitats and by creating new habitats on which many species depend. Bioscience 51, 235–246. doi: 10.1641/0006-3568(2001)051[0235:MFATSO]2.0.CO;2
Stanley, J. A., Hesse, J., Hinojosa, I. A., and Jeffs, A. G. (2015). Inducers of settlement and moulting in post-larval spiny lobster. Oecologia 178, 685–697. doi: 10.1007/s00442-015-3251-4
Tamburri, M. N., Luckenbach, M. W., Breitburg, D. L., and Bonniwell, S. M. (2008). Settlement of Crassostrea ariakensis larvae: effects of substrate, biofilms, sediment and adult chemical cues. J. Shellfish Res. 27, 601–608. doi: 10.2983/0730-8000(2008)27[601:SOCALE]2.0.CO;2
Tamburri, M. N., Zimmer, R. K., and Zimmer, C. A. (2007). Mechanisms reconciling gregarious larval settlement with adult cannibalism. Ecol. Monogr. 77, 255–268. doi: 10.1890/06-1074
Thomsen, M. S., Altieri, A. H., Angelini, C., Bishop, M. J., Gribben, P. E., Lear, G., et al. (2018). Secondary foundation species enhance biodiversity. Nature Ecol. Evol. 2, 634–639. doi: 10.1038/s41559-018-0487-5
Tilman, D., Lehman, C. L., and Bristow, C. E. (1998). Diversity-stability relationships: statistical inevitability or ecological consequence? Am. Nat. 151, 277–282. doi: 10.1086/286118
Valdez, S. R., Zhang, Y. S., Van Der Heide, T., Vanderklift, M. A., Tarquinio, F., Orth, R. J., et al. (2020). Positive ecological interactions and the success of seagrass restoration. Front. Mar. Sci. 7:91. doi: 10.3389/fmars.2020.00091
Van De Koppel, J., Van Der Heide, T., Altieri, A. H., Eriksson, B. K., Bouma, T. J., Olff, H., et al. (2015). Long-distance interactions regulate the structure and resilience of coastal ecosystems. Ann. Rev. Mar. Sci. 7, 139–158. doi: 10.1146/annurev-marine-010814-015805
Van Wesenbeeck, B. K., Griffin, J. N., Van Koningsveld, M., Gedan, K. B., McCoy, M. W., and Silliman, B. R. (2013). “Nature-based coastal defenses: can biodiversity help,” in Encyclopedia of Biodiversity, ed S. Levin (Cambridge: Academic Press), 451–458. doi: 10.1016/B978-0-12-384719-5.00323-3
Vermeij, M. J., Marhaver, K. L., Huijbers, C. M., Nagelkerken, I., and Simpson, S. D. (2010). Coral larvae move toward reef sounds. PloS ONE 5:e10660. doi: 10.1371/journal.pone.0010660
Waldron, A., Mooers, A. O., Miller, D. C., Nibbelink, N., Redding, D., Kuhn, T. S., et al. (2013). Targeting global conservation funding to limit immediate biodiversity declines. Proc. Natl. Acad. Sci. U.S.A. 110, 12144–12148. doi: 10.1073/pnas.1221370110
White, J. M., Buhle, E. R., Ruesink, J. L., and Trimble, A. C. (2009). Evaluation of olympia oyster (Ostrea lurida carpenter 1864) status and restoration techniques in puget sound, Washington, United States. J. Shellfish Res. 28, 107–112. doi: 10.2983/035.028.0101
Whitman, E. R., and Reidenbach, M. A. (2012). Benthic flow environments affect recruitment of Crassostrea virginica larvae to an intertidal oyster reef. Mar. Ecol. Prog. Ser. 463, 177–191. doi: 10.3354/meps09882
Williams, S. L., Ambo-Rappe, R., Sur, C., Abbott, J. M., and Limbong, S. R. (2017). Species richness accelerates marine ecosystem restoration in the coral triangle. Proc. Natl. Acad. Sci. U.S.A. 114, 11986–11991. doi: 10.1073/pnas.1707962114
Yachi, S., and Loreau, M. (1999). Biodiversity and ecosystem productivity in a fluctuating environment: the insurance hypothesis. Proc. Natl. Acad. Sci. U.S.A. 96, 1463–1468. doi: 10.1073/pnas.96.4.1463
Young, C. S., and Gobler, C. J. (2018). The ability of macroalgae to mitigate the negative effects of ocean acidification on four species of North Atlantic bivalve. Biogeosciences 15, 6167–6183. doi: 10.5194/bg-15-6167-2018
Zu Ermgassen, P., Grabowski, J. H., Gair, J. R., and Powers, S. P. (2016). Quantifying fish and mobile invertebrate production from a threatened nursery habitat. J. Appl. Ecol. 53, 596–606. doi: 10.1111/1365-2664.12576
Keywords: mutualism, facilitation, restoration, oyster reef, ecosystem services, systematic review, positive interactions
Citation: Reeves SE, Renzi JJ, Fobert EK, Silliman BR, Hancock B and Gillies CL (2020) Facilitating Better Outcomes: How Positive Species Interactions Can Improve Oyster Reef Restoration. Front. Mar. Sci. 7:656. doi: 10.3389/fmars.2020.00656
Received: 29 March 2020; Accepted: 20 July 2020;
Published: 03 September 2020.
Edited by:
Romuald Lipcius, College of William & Mary, United StatesReviewed by:
Donna Marie Bilkovic, College of William & Mary, United StatesElisabeth Marijke Anne Strain, University of Tasmania, Australia
Copyright © 2020 Reeves, Renzi, Fobert, Silliman, Hancock and Gillies. This is an open-access article distributed under the terms of the Creative Commons Attribution License (CC BY). The use, distribution or reproduction in other forums is permitted, provided the original author(s) and the copyright owner(s) are credited and that the original publication in this journal is cited, in accordance with accepted academic practice. No use, distribution or reproduction is permitted which does not comply with these terms.
*Correspondence: Simon E. Reeves, c2ltb24ucmVldmVzQHRuYy5vcmc=