- 1Department of Estuarine and Delta Systems, Utrecht University, NIOZ Royal Netherlands Institute for Sea Research, Yerseke, Netherlands
- 2Department of Environmental Sciences, Copernicus Institute for Sustainable Development, Utrecht University, Utrecht, Netherlands
Cold-water corals build extensive reefs on the seafloor that are oases of biodiversity, biomass, and organic matter processing rates. The reefs baffle sediments, and when coral growth and sedimentation outweigh ambient sedimentation, carbonate mounds of tens to hundreds of meters high and several kilometers wide can form. Because coral mounds form over ten-thousands of years, their development process remains elusive. While several environmental factors influence mound development, the mounds also have a major impact on their environment. This feedback between environment and mounds, and how this drives mound development is the focus of this paper. Based on the similarity of spatial coral mound patterns and patterns in self-organized ecosystems, we provide a new perspective on coral mound development. In accordance with the theory of self-organization through scale-dependent feedbacks, we first elicit the processes that are known to affect mound development, and might cause scale-dependent feedbacks. Then we demonstrate this concept with model output from a study on the Logachev area, SW Rockall Trough margin. Spatial patterns in mound provinces are the result of a complex set of interacting processes. Spatial self-organization provides a framework in which to place and compare these processes, so as to assess if and how they contribute to pattern formation in coral mounds.
Introduction
Cold-water coral ecosystems are true oases amidst a deep-sea desert, with higher biodiversity (Henry and Roberts, 2007), biomass (Bongiorni et al., 2010; Demopoulos et al., 2014) and organic matter processing rates (Cathalot et al., 2015; de Froe et al., 2019) as compared to the off-reef soft-sediment seafloor at similar water depth.
When scleractinian cold-water corals grow, they build a structurally complex framework consisting of a mosaic of living corals, dead coral branches, associated fauna and coral rubble. The reef framework reduces the current velocity (Mienis et al., 2019) and thereby stimulates settling of fine particles between the coral branches (Dorschel et al., 2005; de Haas et al., 2009). When coral growth and sediment accumulation within the framework outweighs “ambient” sedimentation of the off-reef seafloor, carbonate mounds, of tens to hundreds of meters high and several kilometers wide, are formed on geological time scales (Dorschel et al., 2005; Douarin et al., 2014; Van der Land et al., 2014; Wienberg and Titschack, 2017). Hence, coral mound formation is tightly linked to coral growth and sediment infill (Roberts et al., 2006).
The remoteness of the deep sea and the slow growth rate of mounds make it difficult to obtain insight in the processes that govern mound formation through observations alone. Environmental parameters, such as hydrodynamics, organic matter concentration and particle supply can be measured directly (e.g., Mienis et al., 2007; Davies et al., 2009; Wagner et al., 2011), but often only at a few locations and for a limited amount of time. Long sediment cores reveal information on mound growth and non-growth periods, but do not convey information on the governing environmental parameters or from periods when erosion prevailed (Hebbeln et al., 2019). Multi-beam or side-scan sonar maps provide spatial configurations of coral mounds on the seafloor, but this retrieves little information on their temporal dynamics and the underlying processes. A conceptual framework in which insights from these various data sources can be integrated may represent an interesting step forward.
Here, we provide a new perspective on cold-water coral mound formation which could serve as such a conceptual framework. It is based on the theory of spatial self-organization, a theory that is well-rooted in terrestrial and coastal sciences (Rietkerk and van de Koppel, 2008), but that has found little application for deep-sea ecosystems so far. Specifically, we hypothesize that coral mounds form on the seafloor through the interplay of positive and negative feedbacks. Positive feedbacks are processes that increasingly stimulate mound growth as they grow bigger and we will argue that these positive feedbacks act primarily at the local scale. In contrast, negative feedbacks result in an inhibitory effect on mound formation and we will argue that these act at some distance from the mound. Provided that environmental conditions are suitable for coral growth and mound development, we propose that these scale-dependent positive and negative feedbacks shape the mounds and their orientation on the seafloor.
Theory of Spatial Self-Organization
How scale-dependent feedbacks shape the environment is described by Alan Turing, in his attempt to explain the breaking of spherical symmetry during embryonic development (Turing, 1990). Since then, scale-dependent feedbacks have been used to explain how a variety of biological systems are shaped (Camazine et al., 2001), such as the synchronized flashing of fireflies, beehives, the appearance of animal skin (Kondo, 2002) and shells (Meinhardt, 1995), and in many ecological systems (Rietkerk and van de Koppel, 2008).
Probably the best-studied ecological example is arid vegetation. Aerial photography shows that in dry climates, vegetation forms a conspicuous spatial pattern of alternating vegetation bands and bare soil (Figure 1A). The mechanisms driving this pattern formation is that when water runs down a slope it is caught by a tussock of vegetation. Vegetation increases water infiltration with its roots, that serve as channels in the soil, thereby stimulating its own growth; a local-scale positive feedback. At the same time, the enhanced water infiltration reduces water supply downslope of the tussock, so vegetation growth is inhibited behind the tussock; a negative feedback at some distance. The interplay of these feedbacks creates a patterned state: on sloping terrain alternating bands of vegetation and bare soil appear, and under other environmental conditions, vegetation spots, mazes and gap patterns exist (Rietkerk et al., 2002). The initial distribution of the organism is here insignificant for pattern formation, because the organisms takes over the process of pattern formation, through scale-dependent feedbacks.
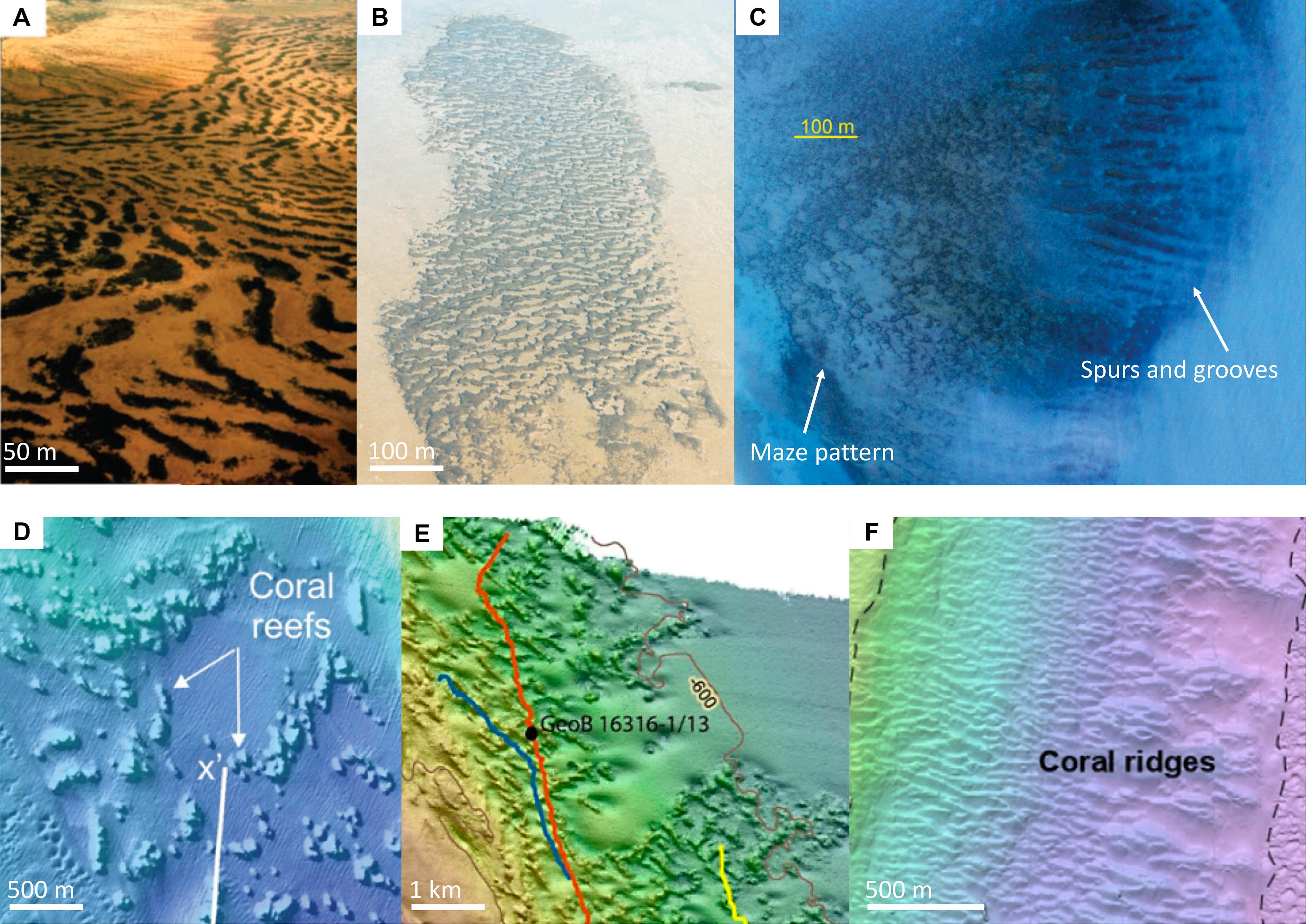
Figure 1. Spatial patterns in natural systems. (A) Arid vegetation forming regular bands (adapted from Rietkerk et al., 2004. Reprinted with permission from AAAS). (B) Regular bands in a young mussel bed (adapted from van de Koppel et al., 2005. ©2005 by University of Chicago Press). (C) Maze (or “reticulate”) patterns, and a banded pattern called “spurs and grooves,” in tropical coral reefs (adapted from Schlager and Purkis, 2015. ©2014 The Authors. Sedimentology. ©2014 International Association of Sedimentologists). (D) Coral reefs in northern Norway, Hola reef, clustering into bands (adapted from Bøe et al., 2016. ©2016 The Geological Society of London). The white line with X’ is the start of a sub-bottom profile transect. (E) Coral ridges in the Gulf of Mexico, Campeche coral mound Province, forming a maze pattern (adapted from Hebbeln et al., 2014. ©2014 The Authors. CC Attribution 3.0 License). Depth contours are shown for 500 m (left) and 600 m (right). Colored lines denote (parts of) ROV dives. GeoB is a station number. (F) Coral ridges near Miami (adapted from Correa et al., 2012. ©2012. With permission from Elsevier). Dashed lines mark the area of the slope, which is covered in coral ridges. Miami Terrace is on the left and the base on the right. The terrace also features coral ridges, but the base is covered in sand dunes. Approximate scales are indicated.
Regular patterns formed via spatial self-organization are also found in the marine environment, e.g., mussel beds (Figure 1B) and tropical coral reefs (Figure 1C). Settlement of young mussels is enhanced on existing mussel patches, as larvae need to attach to hard substrate with byssal threads (i.e., local-scale positive feedback). Downstream of a feeding mussel-patch however, water gets depleted of food, inhibiting mussel growth behind the patch (i.e., negative feedback at a distance). In a predominantly unidirectional current, these two feedbacks can cause the formation of bands of mussels from an initially random distribution of mussels (van de Koppel et al., 2005). Regular bands, called “spurs and grooves,” in tropical coral reefs are also thought to be caused by a positive and a negative feedback. The spurs are favorable habitat for corals and/or coralline algae, which heighten the spur by accretion, similar to cold-water coral mounds. In the grooves, however, sediment erodes (Gischler, 2010). Spots and maze (or “reticulate”) patterns are also seen in tropical coral reefs (Schlager and Purkis, 2015).
Interestingly, multi-beam and side-scan sonar topography maps of the deep seafloor of cold-water coral mound provinces from around the world, show regular bands, ridges and spots (Figures 1D–F) that are surprisingly similar to the systems that are known to be governed by spatial self-organization. For example, cold-water coral mounds on the Norwegian shelf, probably originating from the start of the Holocene, show ridge- and spot-like patterns (Figure 1D; Bøe et al., 2016). In the Gulf of Mexico, individual reefs align in a maze- and ridge-like pattern (Figures 1E,D; Hebbeln et al., 2014), where the mounds are subjected to a current that regularly shifts about 30 degrees. The shift in current direction is thought to be the cause of this maze pattern as the ridges appear to align in both current directions (Hebbeln et al., 2014). Finally, coral mound structures in the Florida Strait (Figure 1F; Correa et al., 2012) are subjected to a unidirectional current and bear strong resemblance to the bands in the mussel bed (Figure 1B), albeit at a different scale. Other examples of cold-water coral mound patterns are also mentioned in the literature. Somoza et al. (2014), for example, report coral mounds that are lined up in ridges, alternated by bare sediments, and White et al. (2007) found that mound clusters are oriented toward the strongest currents.
Spatial Self-Organization Theory Applied to Cold-Water Coral Mounds
If the coral mound patterns, as identified in the previous section, are indeed the result of spatial-self organization, then we should be able to identify positive feedbacks and negative feedbacks that act at different spatial scales. The positive and negative feedbacks can be visualized as in Figure 2A and are “scale-dependent,” as the feedback changes from positive (green) to negative (yellow) with increasing distance from the mound.
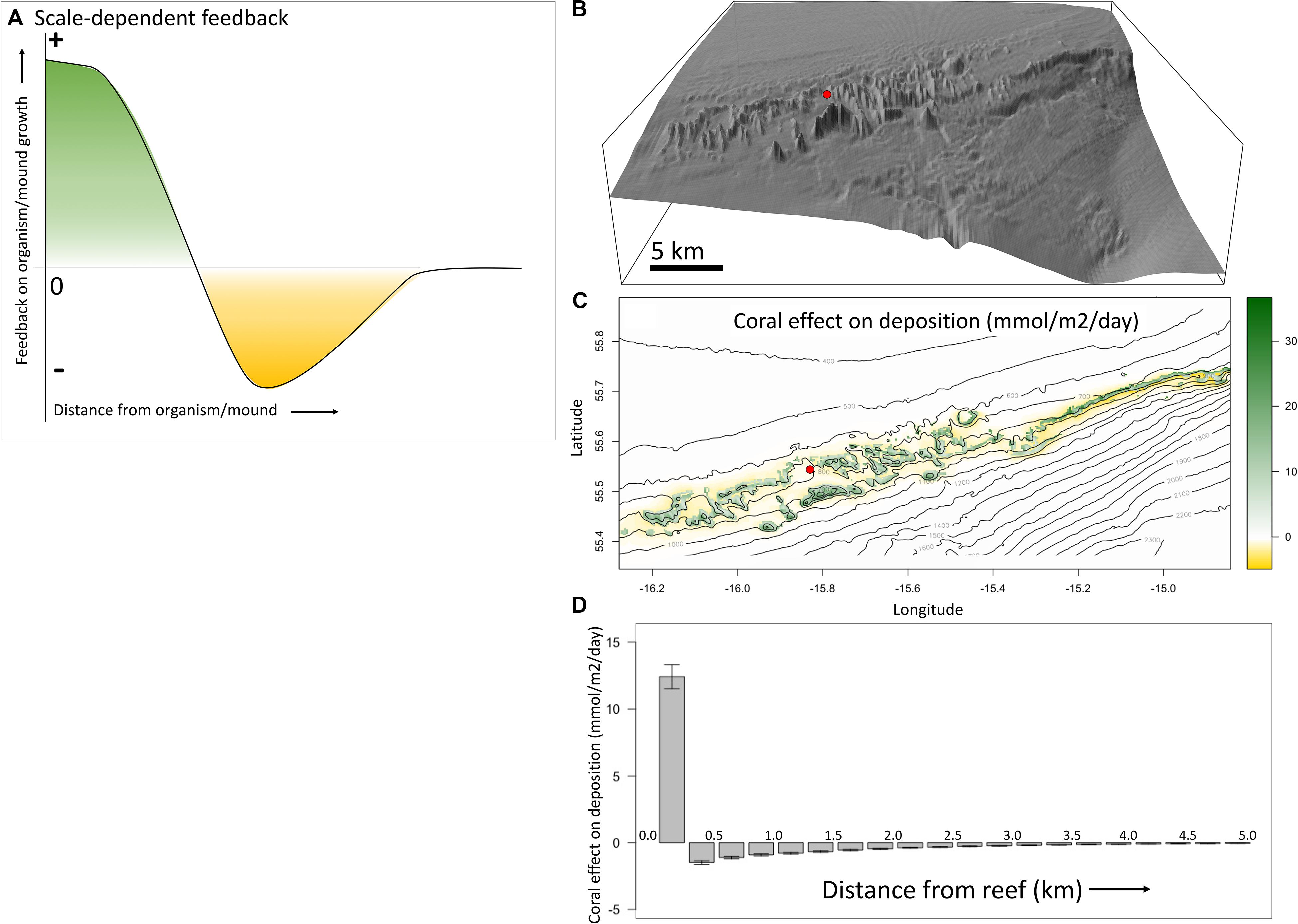
Figure 2. Scale-dependent feedbacks visualized. (A) Depicted are the proposed scale-dependent feedbacks as caused by the presence of coral mounds, in a classic scale-dependent feedback figure (e.g., Figure 1B in Rietkerk and van de Koppel, 2008). Near the mound there is a positive feedback (green) on reef-, and therefore mound-, growth, whereas there is a negative feedback (yellow) at a larger distance from the mound. (B) The study area of the Southwest Rockall Trough (Logachev) area. The red circle serves as a reference to the map in panel (C). (C) The effect of coral presence on organic carbon deposition (mmol m– 2 day– 1) is mapped, with positive effects in green and negative effects in yellow. It shows a positive effect of reefs on their food supply on the mounds, and a negative effect around the mounds. (D) This classic scale-dependent feedback plot shows the average effect of coral reefs on their food supply, with error bars showing the 95% confidence level. Locally (distance = 0) there is a mean positive effect on deposition and until about 3 km from the reefs there is a mean negative effect. These feedbacks are illustrated using model output from Soetaert et al. (2016), for the SW Rockall Trough margin.
Positive Feedbacks on Coral Mound Growth
Several mechanisms causing positive feedbacks can be found in the literature regarding coral mounds. The presence of coral reef framework has a positive effect on mound development, because it baffles suspended sediment and stimulates mound growth (Dorschel et al., 2007; Mienis et al., 2009a; Hebbeln et al., 2016) and stability (Titschack et al., 2015). Furthermore, settlement of coral larvae is greatly enhanced on coral mounds because of the presence of dead coral framework, shells and other debris from reef dwellers (Freiwald, 2002). Cold-water corals are passive suspension feeders and thus depend on water currents to intercept suspended food particles. Coral mounds have a positive effect on coral growth by accelerating currents and enhancing turbulence, because of the formation of lee waves and non-linear hydrodynamic features such as hydraulic jumps and internal bores (Mohn et al., 2014; Van Haren et al., 2014; Mienis et al., 2009b). Such internal waves can cause vertical water excursions of as much as 200 m (Mienis et al., 2007), which enhance downward transport of nutritious particles to the mounds (Duineveld et al., 2007; Davies et al., 2009; Kenchington et al., 2017). This effect becomes stronger for larger mounds, highlighting the positive feedback effect (Cyr et al., 2016). The accelerated currents and turbulent mixing increase the encounter rate of suspended particles with coral feeding tentacles, and thereby likely enhance coral growth.
Negative Feedbacks on Coral Mound Growth
Negative feedbacks are also reported in the literature, and, typically, negative feedbacks are dominant over positive feedbacks, at some distance from the mound. One such scale-dependent negative feedback is caused by the filtering activity of the cold-water coral reef community that depletes the water of food particles around or downstream of the reefs (Wagner et al., 2011; Soetaert et al., 2016). Another negative feedback might come from erosional forces such as scouring, the process whereby sediment is eroded away around the base of mounds, due to the acceleration of currents. Indeed, larger mounds have larger and deeper scour marks (Lim et al., 2018), suggesting that lateral mound development is increasingly hampered by these erosional forces. By inhibiting lateral mound development, such a scale-dependent feedback can shape mounds into self-organized patterns.
Another erosional force, hydraulic drag, may also be an important factor in shaping coral mounds. A model for the Eastern Rockall Trough mounds explained the elliptical shape of the mounds as well as the mound-size distribution, using hydraulic drag. The negative feedback from drag increases as mounds grow, preventing further mound growth as soon as it becomes stronger than reef growth (O’Reilly et al., 2003).
Within coral mound provinces, i.e., areas where several coral mounds exist together, it seems that mound tops are situated around the same depth (Mienis et al., 2006; Wheeler et al., 2007). Several mechanisms have been put forward to explain this observation. At the South-West Rockall Trough margin, the presence of intermediate nepheloid layers (INL) around the tops of the coral mounds has been linked to higher turbidity and increased food supply toward the corals (Kenyon et al., 2003; Mienis et al., 2007). The depth of mound-tops has also been linked to the presence of the permanent thermocline and water mass boundaries, which may provide favorable conditions by enhanced current speeds and vertical movement of isopycnals through internal waves (White and Dorschel, 2010; Hebbeln et al., 2014; Matos et al., 2017; Wang et al., 2019).
Visualizing Feedbacks Using the SW Rockall Trough Margin as Case-Study
The concept of scale-dependent feedbacks acting on coral mound formation can be demonstrated based on a model study on the Logachev area (Figure 2B), SW Rockall Trough margin (Soetaert et al., 2016). Here, food supply mechanisms to coral mounds were investigated by combining various modeling techniques. Hydrodynamics in the area, simulated in 3D (Mohn et al., 2014), was used to model organic matter dynamics in the water column, including export production, 3D-advective transport, decay, and sinking. The original paper considered two scenarios, one with organic matter uptake by reef organisms and one without uptake by reef organisms. We used the difference between both scenarios to demonstrate the net feedback caused by the suspension feeding activity of the reef community on organic matter deposition (Figures 2C,D).
In Figure 2C we plotted the effect of corals on their own food supply on a bathymetric map of the area. The mounds appear in green, because the reefs have a local positive effect on organic matter deposition. Around the mounds, depletion of organic matter by reef filtering is colored yellow. These effects can also be plotted in a classic scale-dependent feedback plot (Figure 2D). This shows the effect at increasing distance from the reefs, averaged over bins of 0.25 km. On the reefs, at a distance of 0–0.25 km from the reefs, the average effect is positive. At a distance of 0.25–0.5 km from the reefs the average effect is negative. The strength of the negative effect decreases until it is gone at about a distance of 3 km from the reefs.
Discussion
Inherited or Self-Organized Patterns
An alternative hypothesis that could explain patterns in cold-water coral mounds, is that corals colonized pre-existing (inherited) patterns. Such patterns can come from heterogeneity in landscape suitability. For example, in arid vegetation, the self-organized pattern can be distorted by patches of rock, where plants cannot settle (Sheffer et al., 2013). Sheffer et al. (2013) show that arid vegetation patterns in nature are likely a mixture of inherited and self-organized patterns and that the probability of occurrence of self-organized patterns increases as the area suitable for plant establishment increases. Coral larvae settle only on hard substrate and therefore seem bound to follow pre-existing patterns of hard substrate. Antecedent karst, for example, can explain patterns in some tropical coral reefs (Schlager and Purkis, 2015). However, as they grow into reefs, corals start to supply their own suitable substrate. This process is subject to positive and negative scale-dependent feedbacks, as described in this paper, that shape reefs into patterns. Indeed, Schlager and Purkis (2015) show that many patterns in tropical coral reefs cannot be explained by patterns in the underlying topography.
Pre-existing patterns could also be formed by physical processes, such as sand waves and ripples, which are often observed close to coral mounds (e.g., Mienis et al., 2006; Correa et al., 2012; Lim et al., 2018). However, an inherited pattern would require that cold-water corals follow the pre-existing topography without changing it. Correa et al. (2012) show that the shape and distribution of coral ridges cannot be explained by pre-existing patterns. Moreover, the coral ridges have their steepest slope facing the current, which is the reverse of sand ripples that have their steepest slope at the lee side. So, even if corals initially inherited the sand wave pattern, coral growth subsequently changed the bathymetry by stabilizing the sediment, exerting scale-dependent feedbacks and “self-organizing” the system into its current state. This is a known process in another self-organized system: dune development, in which crescent-shaped “barchan dunes” have their crest facing the wind, but reverse their shape when colonized by vegetation that stabilizes the sand (Tsoar and Blumberg, 2002).
From Initial Settlement to Full-Grown Coral Mound
A possible sequence of events leading to self-organized patterns, could be as follows. Cold-water corals initially settle on available hard substrate. If the environment is favorable enough, they form into bush-like coral colonies that grow outward and aggregate to form complex three-dimensional reefs. At this point, coral structures create their own suitable environment, and underlying hard substrate becomes inconsequential for further settlement and growth: new corals can settle on dead coral rubble and coral framework can grow outward from established coral colonies. If the environment permits, reefs can start forming mounds, a process that, we argue, is shaped by scale-dependent feedbacks that cause the formation of self-organized patterns, such as coral ridges.
At the early mound formation stage, the initial distribution pattern gets lost as positive and negative scale-dependent feedbacks start to dominate. Larger mounds would then negatively affect nearby, smaller mounds, inhibiting coral growth on the latter. This implies the co-occurrence of large mounds with thriving corals, next to smaller mounds with degraded coral cover. Evidence for such co-occurence is reported in literature (e.g., Foubert and Henriet, 2009; De Clippele et al., 2017), but is not ubiquitous. This may be, because observations have not yet been interpreted in light of this process. Another reason may be an observation bias, as larger mounds are surveyed preferentially above the seabed in between mounds. Furthermore, larger mounds may also outcompete smaller mounds, while leaving little evidence of the smaller, dead mounds, through three non-mutually exclusive processes: (1) erosion of the smaller mounds, (2) burial after they became inactive, and (3) clustering of smaller mounds into larger mounds.
Evidence of mounds eroded away would be hard to find, but is not unlikely given that large mounds form on time scales of thousands to millions of years. Mound burial is reported, with several studies showing the presence of living mounds close to buried mounds, e.g., on the Moroccan margin (Vandorpe et al., 2017), the Magellan province (Huvenne et al., 2007), West Melilla mound Province (Iacono et al., 2014), and the SW Rockall Trough margin (Mienis et al., 2006). Clustering of smaller mounds to form larger mounds, has also been observed (e.g., De Mol et al., 2002; de Haas et al., 2009; Lim et al., 2018). Vandorpe et al. (2017) even write that, by clustering into larger features, small coral mounds can enhance coral mound growth, by intensifying bottom currents locally. They describe a scale-dependent feedback, supporting the idea of coral mound self-organization.
Contribution of Processes to Scale-Dependent Feedbacks
Coral mound development is the outcome of a complex set of interacting processes. However, for some processes it may be unclear whether they are influenced by the mounds, or even whether they have a positive or negative effect. For instance, sedimentation is usually seen as an external factor, but it might be enhanced by mound presence, similarly as food particles. While sedimentation is necessary for mound growth and thus enhances mound development in most cases, if cold-water coral growth declines, it could also limit mound development by burying the coral reefs (De Mol et al., 2002; Foubert et al., 2008). Coral mounds are often situated in a high energy regime. The mobility of the sediments in such environments can restrict lateral mound development by burying the corals at the base (Dorschel et al., 2007; Mienis et al., 2007). While scour is a process that can restrict lateral mound growth (see above), it may also have a positive effect on mound development, as the accelerated currents can uncover hard substrates such as rocks in the sediment. Hard substrates are necessary for initial coral settlement and can therefore stimulate coral seabed colonization (Lim et al., 2018).
There are also processes that influence coral growth and coral mound development, but that do not trigger feedbacks because they are not affected by the presence of mounds or corals. An example is the type of sediment that is deposited on the coral mounds (Foubert et al., 2008; Pirlet et al., 2011; Hebbeln et al., 2016). Other external factors that influence cold-water coral growth, and that are beyond control of corals, are temperature (Freiwald and Roberts, 2005), water density (Flögel et al., 2014), oxygen (Dodds et al., 2007; Wienberg et al., 2018), and aragonite saturation state (Guinotte et al., 2006; Roberts et al., 2006). Although these factors play a major role, they merely set the scene, i.e. they determine whether or not corals can possibly occur in a region. Based on our hypothesis, it is only when presence of coral or mounds themselves can affect environmental factors, that interesting patterns may emerge.
Outlook
Rapid cold-water coral extinction events are frequently observed in geological records. Such “tipping-points” are a well-known (theoretical) response of self-organized systems to a gradual change in environmental conditions. However, how self-organized systems respond to changing environmental conditions and how spatial patterns link to resilience, has recently become a topic of debate. Current belief is that the resilience of self-organized systems is not governed by a single tipping point, but by a cascade of destabilizations.
Coral mounds may provide an interesting new case to study resilience of systems governed by scale-dependent feedbacks. Mound structures remain for thousands of years even after coral reef extinction. This may facilitate recolonization as is indeed demonstrated by long cores drilled in the mounds that document sequences of individual coral growth periods, interrupted by periods without coral growth. An interesting topic is then to study the impact that scale-dependent feedbacks have for the resilience of coral systems, and how this will affect these enigmatic deep-sea environments in the near and far future.
Data Availability Statement
Publicly available datasets were analyzed in this study. This data can be found here: https://doi.org/10.1594/PANGAEA.911414.
Author Contributions
JK, DO, and KS initiated the study idea. KS provided the model output data. A-SK analyzed the data and wrote the manuscript. All authors contributed with in-depth knowledge from their respective fields and experience, contributed to manuscript revision, read and approved the submitted version.
Funding
This study was made possible through collaboration funding between Utrecht University and the Royal Netherlands Institute for Sea Research (NIOZ). DO was supported by the European Union’s Horizon 2020 Research and Innovation Program under grant agreement no. 678760 (ATLAS) and the Innovational Research Incentives Scheme of the Netherlands Organization for Scientific Research (NWO), under grant agreement no. 864.13.007. This output reflects only author’s view and the European Union cannot be held responsible for any use that may be made of the information contained therein. The funders had no role in study design, data collection, and analysis, decision to publish, or preparation of the manuscript.
Conflict of Interest
The authors declare that the research was conducted in the absence of any commercial or financial relationships that could be construed as a potential conflict of interest.
The reviewer, DH declared a past co-authorship with one of the authors, DO, to the handling Editor.
References
Bøe, R., Bellec, V. K., Dolan, M. F. J., Buhl-Mortensen, P., Rise, L., and Buhl-Mortensen, L. (2016). “Cold-water coral reefs in the hola glacial trough off Vesterålen, North Norway,” in Atlas of Submarine Glacial Landforms: Modern, Quaternary and Ancient, eds J. A. Dowdeswell, M. Canals, M. Jakobsson, B. J. Todd, E. K. Dowdeswell, and K. A. Hogan (London: Geological Society of London), 309–310. doi: 10.1144/M46.8
Bongiorni, L., Mea, M., Gambi, C., Pusceddu, A., Taviani, M., and Danovaro, R. (2010). Deep-water scleractinian corals promote higher biodiversity in deep-sea meiofaunal assemblages along Continental margins. Biol. Conserv. 143, 1687–1700. doi: 10.1016/j.biocon.2010.04.009
Camazine, S., Deneubourg, J., Franks, N. R., Sneyd, J., Theraulaz, G., and Bonabeau, E. (2001). Self-Organization in Biological Systems, 1st Edn. Princeton, NJ: Princeton University Press.
Cathalot, C., Van Oevelen, D., Cox, T. J. S., Kutti, T., Lavaleye, M., Duineveld, G., et al. (2015). Cold-water coral reefs and adjacent sponge grounds: hotspots of benthic respiration and organic carbon cycling in the deep sea. Front. Mar. Sci. 2:37. doi: 10.3389/fmars.2015.00037
Correa, T. B. S., Eberli, G. P., Grasmueck, M., Reed, J. K., and Correa, A. M. S. (2012). Genesis and morphology of cold-water coral ridges in a unidirectional current regime. Mar. Geol. 32, 14–27. doi: 10.1016/j.margeo.2012.06.008
Cyr, F., Van Haren, H., Mienis, F., Duineveld, G., and Bourgault, D. (2016). On the influence of cold-water coral mound size on flow hydrodynamics, and vice versa. Geophys. Res. Lett. 43, 1–9. doi: 10.1002/2015GL067038
Davies, A. J., Duineveld, G. C. A., Lavaleye, M. S. S., Bergman, M. J. N., van Haren, H., and Roberts, J. M. (2009). Downwelling and deep-water bottom currents as food supply mechanisms to the cold-water coral lophelia pertusa (Scleractinia) at the mingulay reef complex. Limnol. Oceanogr. 54, 620–629. doi: 10.4319/lo.2009.54.2.0620
De Clippele, L. H., Gafeira, J., Robert, K., Hennige, S., Lavaleye, M. S., Duineveld, G. C. A., et al. (2017). Using novel acoustic and visual mapping tools to predict the small-scale spatial distribution of live biogenic reef framework in cold-water coral habitats. Coral Reefs 36, 255–268. doi: 10.1007/s00338-016-1519-8
de Froe, E., Rovelli, L., Glud, R. N., Maier, S. R., Duineveld, G., Mienis, F., et al. (2019). Benthic oxygen and nitrogen exchange on a cold-water coral reef in the North-East Atlantic Ocean. Front. Mar. Sci. 6:665. doi: 10.3389/fmars.2019.00665
de Haas, H., Mienis, F., Frank, N., Richter, T. O., Steinacher, R., de Stigter, H., et al. (2009). Morphology and sedimentology of (Clustered) cold-water coral mounds at the south Rockall trough margins, NE Atlantic Ocean. Facies 55, 1–26. doi: 10.1007/s10347-008-0157-1
De Mol, B., Van Rensbergen, P., Pillen, S., Van Herreweghe, K., Van Rooij, D., McDonnell, A., et al. (2002). Large deep-water coral banks in the porcupine basin, Southwest of Ireland. Mar. Geol. 188, 193–231. doi: 10.1016/S0025-3227(02)00281-5
Demopoulos, A. W. J., Bourque, J. R., and Frometa, J. (2014). Biodiversity and community composition of sediment macrofauna associated with deep-sea lophelia pertusa habitats in the Gulf of Mexico. Deep Sea Res. I Oceanogr. Res. Pap. 93, 91–103. doi: 10.1016/j.dsr.2014.07.014
Dodds, L. A., Roberts, J. M., Taylor, A. C., and Marubini, F. (2007). Metabolic tolerance of the cold-water coral lophelia pertusa (Scleractinia) to temperature and dissolved oxygen change. J. Exp. Mar. Biol. Ecol. 349, 205–214. doi: 10.1016/j.jembe.2007.05.013
Dorschel, B., Hebbeln, D., Foubert, A., White, M., and Wheeler, A. J. (2007). Hydrodynamics and cold-water coral facies distribution related to recent sedimentary processes at Galway Mound West of Ireland. Mar. Geol. 244, 184–195. doi: 10.1016/j.margeo.2007.06.010
Dorschel, B., Hebbeln, D., Ruggeberg, A., and Dullo, C. (2005). Carbonate budget of a cold-water coral carbonate mound: propeller mound, porcupine seabight. Int. J. Earth Sci. 96, 73–83. doi: 10.1007/s00531-005-0493-0
Douarin, M., Sinclair, D. J., Elliot, M., Henry, L., Long, D., Mitchison, F., et al. (2014). Changes in fossil assemblage in sediment cores from mingulay reef complex (NE Atlantic): implications for coral reef build-up. Deep Sea Res. II 99, 286–296. doi: 10.1016/j.dsr2.2013.07.022
Duineveld, G. C. A., Lavaleye, M. S. S., Bergman, M. J. N., de Stigter, H., and Mienis, F. (2007). Trophic structure of a cold-water coral mound community (Rockall Bank, NE Atlantic) in relation to the near-bottom particle supply and current regime. Bull. Mar. Sci. 81, 449–467.
Flögel, S., Dullo, W. C., Pfannkuche, O., Kiriakoulakis, K., and Rüggeberg, A. (2014). Geochemical and physical constraints for the occurrence of living cold-water corals. Deep Sea Res. II Top. Stud. Oceanogr. 99, 19–26. doi: 10.1016/j.dsr2.2013.06.006
Foubert, A., Depreiter, D., Beck, T., Maignien, L., Pannemans, B., Frank, N., et al. (2008). Carbonate mounds in a Mud volcano province off North-West Morocco: key to processes and controls. Mar. Geol. 248, 74–96. doi: 10.1016/j.margeo.2007.10.012
Foubert, A., and Henriet, J. P. (2009). Nature and Significance of the Recent Carbonate Mound Record. Lecture Notes in Earth Sciences, Vol. 126. Berlin: Springer-Verlag.
Freiwald, A. (2002). “Reef-forming cold-water corals,” in Ocean Margin Systems, eds G. Wefer, D. Billett, D. Hebbeln, B. B. Jørgensen, M. Schlüter, and T. C. E. van Weering (Berlin: Springer), 365–385. doi: 10.1016/B978-012374473-9.00666-4
Freiwald, A., and Roberts, J. M. (2005). Cold-Water Corals and Ecosystems, 1st Edn. Berlin: Springer-Verlag.
Gischler, E. (2010). Indo-pacific and atlantic spurs and grooves revisited: the possible effects of different holocene sea-level history, exposure, and reef accretion rate in the shallow fore reef. Facies 56, 173–177. doi: 10.1007/s10347-010-0218-0
Guinotte, J. M., Orr, J., Cairns, S., Freiwald, A., Morgan, L., and George, R. (2006). Will human-induced changes in seawater chemistry alter the distribution of deep-sea scleractinian corals. Front. Ecol. Environ. 4, 141–146. doi: 10.1890/1540-92952006004[0141:WHCISC]2.0.CO;2
Hebbeln, D., Portilho-Ramos, R. C., Wienberg, C., and Titschack, J. (2019). The fate of cold-water corals in a changing world: a geological perspective. Front. Mar. Sci. 6:119. doi: 10.3389/fmars.2019.00119
Hebbeln, D., Van Rooij, D., and Wienberg, C. (2016). Good neighbours shaped by vigorous currents: cold-water coral mounds and contourites in the North Atlantic. Mar. Geol. 378, 171–185. doi: 10.1016/j.margeo.2016.01.014
Hebbeln, D., Wienberg, C., Wintersteller, P., Freiwald, A., Becker, M., Beuck, L., et al. (2014). Environmental forcing of the campeche cold-water coral province, Southern Gulf of Mexico. Biogeosciences 11, 1799–1815. doi: 10.5194/bg-11-1799-2014
Henry, L., and Roberts, J. M. (2007). Biodiversity and ecological composition of macrobenthos on cold-water coral mounds and adjacent off-mound habitat in the bathyal porcupine seabight, NE Atlantic. Deep Sea Res. I Oceanogr. Res. Pap. 54, 654–672. doi: 10.1016/j.dsr.2007.01.005
Huvenne, V. A. I., Bailey, W. R., Shannon, P. M., Naeth, J., di Primio, R., Henriet, J. P., et al. (2007). The magellan mound province in the porcupine basin. Int. J. Earth Sci. 96, 85–101. doi: 10.1007/s00531-005-0494-z
Iacono, C. L., Gràcia, E., Ranero, C. R., Emelianov, M., Huvenne, V. A. I., Bartolomé, R., et al. (2014). The west melilla cold water coral mounds, Eastern Alboran Sea: morphological characterization and environmental context. Deep Sea Res. II Top. Stud. Oceanogr. 99, 316–326. doi: 10.1016/j.dsr2.2013.07.006
Kenchington, E., Yashayaev, I., Tendal, O. S., and Jørgensbye, H. (2017). Water mass characteristics and associated fauna of a recently discovered Lophelia pertusa (Scleractinia: Anthozoa) reef in greenlandic waters. Polar Biol. 40, 321–337. doi: 10.1007/s00300-016-1957-3
Kenyon, N. H., Akhmetzhanov, A. M., Wheeler, A. J., van Weering, T. C. E., de Haas, H., and Ivanov, M. K. (2003). Giant carbonate mud mounds in the southern rockall trough. Mar. Geol. 195, 5–30. doi: 10.1016/S0025-3227(02)00680-1
Kondo, S. (2002). The reaction-diffusion system: a mechanism for autonomous pattern formation in the animal skin. Genes Cells 7, 535–541. doi: 10.1046/j.1365-2443.2002.00543.x
Lim, A., Huvenne, V. A. I., Vertino, A., Spezzaferri, S., and Wheeler, A. J. (2018). New insights on coral mound development from groundtruthed high-resolution ROV-mounted multibeam imaging. Mar. Geol. 403, 225–237. doi: 10.1016/j.margeo.2018.06.006
Matos, L., Wienberg, C., Titschack, J., Schmiedl, G., Frank, N., Abrantes, F., et al. (2017). Coral mound development at the campeche cold-water coral province, Southern Gulf of Mexico: implications of antarctic intermediate water increased influence during interglacials. Mar. Geol. 392, 53–65. doi: 10.1016/j.margeo.2017.08.012
Meinhardt, H. (1995). The Algorithmic Beauty of Sea Shells, 4th Edn. Berlin: Springer-Verlag, doi: 10.1007/s13398-014-0173-7.2
Mienis, F., Bouma, T. J., Witbaard, R., van Oevelen, D., and Duineveld, G. C. A. (2019). Experimental assessment of the effects of cold-water coral patches on water flow. Mar. Ecol. Prog. Ser. 609, 101–117. doi: 10.3354/meps12815
Mienis, F., de Stigter, H. C., de Haas, H., and van Weering, T. C. E. (2009a). Near-bed particle deposition and resuspension in a cold-water coral mound area at the southwest Rockall trough margin, NE Atlantic. Deep Sea Res. I Oceanogr. Res. Pap. 56, 1026–1038. doi: 10.1016/j.dsr.2009.01.006
Mienis, F., de Stigter, H. C., White, M., Duineveld, G., de Haas, H., and van Weering, T. C. E. (2007). Hydrodynamic controls on cold-water coral growth and carbonate-mound development at the SW and SE Rockall trough margin, NE Atlantic Ocean. Deep Sea Res. I Oceanogr. Res. Pap. 54, 1655–1674. doi: 10.1016/j.dsr.2007.05.013
Mienis, F., van der Land, C., de Stigter, H. C., van de Vorstenbosch, M., de Haas, H., Richter, T., et al. (2009b). Sediment accumulation on a cold-water carbonate mound at the Southwest Rockall trough margin. Mar. Geol. 265, 40–50. doi: 10.1016/j.margeo.2009.06.014
Mienis, F., van Weering, T., de Haas, H., de Stigter, H., Huvenne, V., and Wheeler, A. (2006). Carbonate mound development at the SW Rockall trough margin based on high resolution TOBI and seismic recording. Mar. Geol. 233, 1–19. doi: 10.1016/j.margeo.2006.08.003
Mohn, C., Rengstorf, A., White, M., Duineveld, G., Mienis, F., Soetaert, K., et al. (2014). Linking benthic hydrodynamics and cold-water coral occurrences: a high-resolution model study at three cold-water coral provinces in the NE Atlantic. Prog. Oceanogr. 122, 92–104. doi: 10.1016/j.pocean.2013.12.003
O’Reilly, B. M., Readman, P. W., Shannon, P. M., and Jacob, A. W. B. (2003). A model for the development of a carbonate mound population in the rockall trough based on deep-towed sidescan sonar data. Mar. Geol. 198, 55–66. doi: 10.1016/S0025-3227(03)00094-X
Pirlet, H., Colin, C., Thierens, M., Latruwe, K., Van Rooij, D., Foubert, A., et al. (2011). The importance of the terrigenous fraction within a cold-water coral mound: a case study. Mar. Geol. 282, 13–25. doi: 10.1016/j.margeo.2010.05.008
Rietkerk, M., Dekker, S. C., de Ruiter, P. C., and van de Koppel, J. (2004). Self-organized patchiness and catastrophic shifts in ecosystems. Science 305, 1926–1929. doi: 10.1126/science.1101867
Rietkerk, M., Maarten, C., Boerlijst, F., van Langevelde, R., HilleRisLambers, J., van de Koppel, L., et al. (2002). Self−organization of vegetation in arid ecosystems. Am. Nat. 160, 524–530. doi: 10.1086/342078
Rietkerk, M., and van de Koppel, J. (2008). Regular pattern formation in real ecosystems. Trends Ecol. Evol. 23, 169–175. doi: 10.1016/j.tree.2007.10.013
Roberts, J. M., Wheeler, A. J., and Freiwald, A. (2006). Reefs of the deep: the biology and geology of cold-water coral ecosystems. Science 312, 543–547. doi: 10.1126/science.1119861
Schlager, W., and Purkis, S. (2015). Reticulate reef patterns – antecedent karst versus self-organization. Sedimentology 62, 501–515. doi: 10.1111/sed.12172
Sheffer, E., von Hardenberg, J., Yizhaq, H., Shachak, M., and Meron, E. (2013). Emerged or imposed: a theory on the role of physical templates and self-organisation for vegetation patchiness. Ecol. Lett. 16, 127–139. doi: 10.1111/ele.12027
Soetaert, K., Mohn, C., Rengstorf, A., Grehan, A., and Van Oevelen, D. (2016). Ecosystem engineering creates a direct nutritional link between 600-m deep cold-water coral mounds and surface productivity. Sci. Rep. 6, 1–9. doi: 10.1038/srep35057
Somoza, L., Ercilla, G., Urgorri, V., León, R., Medialdea, T., Paredes, M., et al. (2014). Detection and mapping of cold-water coral mounds and living lophelia reefs in the Galicia Bank, Atlantic NW Iberia margin. Mar. Geol. 349, 73–90. doi: 10.1016/j.margeo.2013.12.017
Titschack, J., Baum, D., de Pol-Holz, R., ópez Correa, M. L., Forster, N., Flögel, S., et al. (2015). Aggradation and carbonate accumulation of holocene norwegian cold-water coral reefs. Sedimentology 62, 1–26. doi: 10.1111/sed.12206
Tsoar, H., and Blumberg, D. G. (2002). Formation of parabolic dunes from barchan and transverse dunes along israel’s mediterranean coast. Earth Surf. Process. Landf. 27, 1147–1161. doi: 10.1002/esp.417
Turing, A. M. (1990). The chemical basis of morphogenesis. Bull. Math. Biol. 52, 153–197. doi: 10.1016/s0092-8240(05)80008-4
van de Koppel, J., Rietkerk, M., Dankers, N., and Herman, P. M. J. (2005). Scale-dependent feedback and regular spatial patterns in young mussel beds. Am. Nat. 165, E66–E77.
Van der Land, C., Eisele, M., Mienis, F., de Haas, H., Hebbeln, D., Reijmer, J. J. G., et al. (2014). Carbonate mound development in contrasting settings on the Irish margin. Deep Sea Res. II Top. Stud. Oceanogr. 99, 297–306. doi: 10.1016/j.dsr2.2013.10.004
Van Haren, H., Mienis, F., Duineveld, G. C. A., and Lavaleye, M. S. S. (2014). High-resolution temperature observations of a trapped nonlinear diurnal tide influencing cold-water corals on the Logachev mounds. Prog. Oceanogr. 125, 16–25. doi: 10.1016/j.pocean.2014.04.021
Vandorpe, T., Wienberg, C., Hebbeln, D., Van den Berghe, M., Gaide, S., Wintersteller, P., et al. (2017). Multiple generations of buried cold-water coral mounds since the early-middle pleistocene transition in the Atlantic Moroccan Coral Province, Southern Gulf of Cádiz. Palaeogeogr. Palaeoclimatol. Palaeoecol. 485, 293–304. doi: 10.1016/j.palaeo.2017.06.021
Wagner, H., Purser, A., Thomsen, L., Jesus, C. C., and Lundälv, T. (2011). Particulate organic matter fluxes and hydrodynamics at the tisler cold-water coral reef. J. Mar. Syst. 85, 19–29. doi: 10.1016/j.jmarsys.2010.11.003
Wang, H., Lo Iacono, C., Wienberg, C., Titschack, J., and Hebbeln, D. (2019). Cold-water coral mounds in the Southern Alboran Sea (Western Mediterranean Sea): internal waves as an important driver for mound formation since the last deglaciation. Mar. Geol. 412, 1–18. doi: 10.1016/j.margeo.2019.02.007
Wheeler, A. J., Beyer, A., Freiwald, A., de Haas, H., Huvenne, V. A. I., Kozachenko, M., et al. (2007). Morphology and environment of cold-water coral carbonate mounds on the NW European margin. Int. J. Earth Sci. 96, 37–56. doi: 10.1007/s00531-006-0130-6
White, M., and Dorschel, B. (2010). The importance of the permanent thermocline to the cold water coral carbonate mound distribution in the NE Atlantic. Earth Planet. Sci. Lett. 296, 395–402. doi: 10.1016/j.epsl.2010.05.025
White, M., Roberts, J. M., and van Weering, T. (2007). Do bottom-intensified diurnal tidal currents shape the alignment of carbonate mounds in the NE Atlantic? Geo Mar. Lett. 27, 391–397. doi: 10.1007/s00367-007-0060-8
Wienberg, C., and Titschack, J. (2017). “Framework-forming scleractinian cold-water corals through space and time: a late Quaternary North Atlantic Perspective,” in Marine Animal Forests, ed. S. Rossi (Cham: Springer International Publishing), 699–732. doi: 10.1007/978-3-319-21012-4_16
Keywords: oceanography, pattern-formation, self-organization, cold-water corals, carbonate mounds
Citation: van der Kaaden A-S, van Oevelen D, Rietkerk M, Soetaert K and van de Koppel J (2020) Spatial Self-Organization as a New Perspective on Cold-Water Coral Mound Development. Front. Mar. Sci. 7:631. doi: 10.3389/fmars.2020.00631
Received: 18 November 2019; Accepted: 09 July 2020;
Published: 04 August 2020.
Edited by:
J. Murray Roberts, The University of Edinburgh, United KingdomReviewed by:
Andres Rüggeberg, Université de Fribourg, SwitzerlandDierk Hebbeln, Center for Marine Environmental Sciences, University of Bremen, Germany
Copyright © 2020 van der Kaaden, van Oevelen, Rietkerk, Soetaert and van de Koppel. This is an open-access article distributed under the terms of the Creative Commons Attribution License (CC BY). The use, distribution or reproduction in other forums is permitted, provided the original author(s) and the copyright owner(s) are credited and that the original publication in this journal is cited, in accordance with accepted academic practice. No use, distribution or reproduction is permitted which does not comply with these terms.
*Correspondence: Anna-Selma van der Kaaden, anna.van.der.kaaden@nioz.nl