- Division of Sea Turtle Science and Recovery, Padre Island National Seashore, National Park Service, Corpus Christi, TX, United States
Understanding how populations are distributed spatially can be an effective tool for conservation and management planning. This is especially true for threatened species of mobile marine megafauna, which can use vast expanses of the ocean as foraging and reproductive habitat. Additionally, the broad distributions of these species expose them to a wide variety of threats across their range. Marine turtles are one of these mobile marine megafauna taxa, with individuals making extensive migrations between nesting beaches and foraging grounds. However, it is not well understood how many marine turtle populations distribute themselves spatially at the population or species level. In this study, 178 post-nesting female Kemp’s ridley sea turtles were concurrently satellite tracked from their primary and major secondary nesting beaches between 2010 and 2017 to determine population- and species-level distribution of females to foraging grounds. Filtered tracks and switching state-space models (SSMs) were used to determine the proportion of the adult female population dispersing to, and foraging in, the northern and southern Gulf of Mexico (GoM). Fidelity to Gulf regions and foraging areas were also assessed. The majority of females dispersed to northern GoM (nGoM) foraging grounds indicating 82% of the adult female population may use the region to forage. Additionally, individuals displayed fidelity to regions, key foraging areas, and migratory corridors over time. These results suggest that the nGoM may provide the most important foraging areas for reproductively active females of the species and threats in the region may have a disproportionately higher impact on the adult female population, a population critical to the perpetuation and recovery of the species. Results highlight that continued bi-national recovery efforts are essential for recovery of Kemp’s ridleys, as most females nest on Mexican beaches and forage in U.S. waters. The methodologies used could be applied to conservation and management efforts of other imperiled mobile marine megafauna.
Introduction
Determining distributions of mobile marine megafauna (e.g., marine turtles, elasmobranchs, cetaceans, and pinnipeds) is a fundamental concept for conservation and management of these imperiled species. However, determining these distributions, especially at the population level, poses significant challenges (Morales et al., 2010; Holdo and Roach, 2013; Jeffers and Godley, 2016). Specifically, mobile marine megafauna are often cryptic and their ranges can encompass entire ocean basins (Block et al., 2011; Reisinger et al., 2018) making systematic surveys impractical, both geographically and temporally. Beginning in the 1980s, telemetry studies using satellite transmitters were employed to investigate the detailed movement patterns and distributions of these species in lieu of surveys or the need to recapture an individual (Godley et al., 2008; Hart and Hyrenbach, 2009; Hays and Hawkes, 2018). However, these studies have often been plagued with low sample sizes (<10), short tracking durations, a lack of appropriate experimental design, high degrees of spatial error, and an absence of behavioral data to interpret how an animal is using the environment (Hart and Hyrenbach, 2009; Rutz and Hays, 2009).
Continued improvements in animal-borne telemetry and collaborative partnerships [e.g., Global Tagging of Pelagic Predators (GTOPP), Ocean Tracking Network (OTN), Integrated Marine Observing System Animal Tracking Facility (IMOS ATF)] have alleviated these shortcomings, allowing for increased numbers of individuals (10s to 1,000s) (Schofield et al., 2013; Fossette et al., 2014; Block et al., 2016; Queiroz et al., 2019) to be tracked over longer timeframes than in the past through data-sharing, reductions in cost, decreased transmitter size, and increased location acquisition and error processing (Rutz and Hays, 2009; Lopez and Malardé, 2011; Hays and Hawkes, 2018). These improvements, paired with advancements in quantitative techniques (e.g., state-space modeling, stable isotope analyses, genetics, remotely sensed environmental data) and computational power, allow the use of telemetry data to further our understanding of the spatial distributions of mobile marine megafauna and infer population-level dynamics (Godley et al., 2008; Hazen et al., 2012; Hays and Hawkes, 2018). Specifically, increased numbers of tracked individuals and behavioral-based modeling approaches can provide a link between individual animal movements and population metrics (Morales et al., 2010; Holdo and Roach, 2013; Schofield et al., 2013). For example, large-scale tracking studies have identified ocean basin-scale distributions (Block et al., 2011), classified migratory corridors and foraging areas (Costa et al., 2012; Shaver et al., 2013, 2016a), assessed inter-species overlap (Hart et al., 2018b), and determined exposure to anthropogenic threats (e.g., fisheries, energy production, pollution) (Maxwell et al., 2013; Hart et al., 2018a; Queiroz et al., 2019) of mobile marine megafauna. These insights can be effective tools for conservation planning and management of these species and highlight the need for continued large-scale collaborative data-sharing programs to further improve population-level knowledge.
Studies typically focus on assessing distributions and movements at the metapopulation (e.g., mixed-stock foraging aggregations, individuals from disparate breeding colonies) (Block et al., 2011; Queiroz et al., 2019) or local (e.g., specific nesting beaches or haul-out sites) (Oksanen et al., 2014; Dawson et al., 2017) level. These studies have provided significant information on how mobile marine megafauna are distributed at global and local scales and the potential broadscale and localized impacts of threats (e.g., fisheries bycatch, pollution, human development) (Maxwell et al., 2013; Hart et al., 2018a; Queiroz et al., 2019). However, implementing conservation strategies on a global scale may be hindered by differences between stakeholder priorities and cultural beliefs, while local-scale conservation may only protect a small percentage of a Regional Management Unit (RMU) or subpopulation, providing limited conservation benefit. In addition, these studies may not identify specific threats, important foraging regions, or delineate population boundaries at the subpopulation or RMU level, which can be effective targets for conservation goals. Thus, a spatial mismatch may exist between the units being studied (e.g., metapopulations, local stocks) and the units at which conservation efforts should be directed (i.e., RMUs) (Moritz, 1994; Hamann et al., 2010). Protecting RMUs can ensure genetic diversity and resiliency in metapopulations, while addressing specific regional threats and developing appropriate management plans, making them appropriate targets for short-term conservation goals (Moritz, 1994; Wallace et al., 2010).
Marine turtles are one guild of mobile marine megafauna which has received a significant amount of research focusing on delineating the movements and distributions of individuals and populations (Godley et al., 2008). To date, thousands of transmitters have been deployed on nesting and foraging marine turtles of all seven species, globally (Godley et al., 2008). Despite the level of effort that has been put into tracking individuals, there are still uncertainties in the boundaries and connections that exist within and between populations, where key foraging areas lie and the degree of site fidelity to foraging areas (Hays and Hawkes, 2018). In fact, these questions are consistently listed as priority items in marine turtle conservation and management reviews (Godley et al., 2008; Hamann et al., 2010; Rees et al., 2016; Hays and Hawkes, 2018). Generalized boundaries for marine turtle RMUs were outlined by Wallace et al. (2010) and recent progress has been made identifying key foraging regions for some RMUs (Schofield et al., 2013; Fossette et al., 2014). However, further investigations are needed to fully understand adult foraging areas and distributions. Future studies should also determine effective methods to identify the key foraging regions, specifically for RMUs (Rees et al., 2016) and identify the relative importance of each region to the conservation and/or recovery of a species.
A species of particular conservation concern is the critically endangered Kemp’s ridley sea turtle (Lepidochelys kempii), which nests almost exclusively along the western coast of the Gulf of Mexico (GoM) (Márquez-Millán et al., 2005; NMFS and USFWS, 2015). The majority of Kemp’s ridley nests are found near Rancho Nuevo, Tamaulipas, Mexico, which is considered to be the primary nesting beach for the species (Wibbels and Bevan, 2016). The majority of nests not laid at Rancho Nuevo are found at Padre Island National Seashore, Texas, United States, and Tecolutla, Veracruz, Mexico (NMFS and USFWS, 2015) which are considered here as major secondary nesting beaches. Recent pioneering work has identified foraging areas and migratory corridors within the GoM for this species through tracking post-nesting females from the primary and major secondary nesting beaches located in Mexico and the United States (Shaver and Rubio, 2008; Shaver et al., 2013, 2016a). Despite this work, the proportion of the population using identified key foraging areas is largely unknown, as is the fidelity of individuals to these foraging areas over time or the relative importance of each area. Uniquely, the Kemp’s ridley is managed under one RMU and is believed to be a single genetic stock (Wallace et al., 2010) allowing for population-, RMU-, and species-level determinations of distribution and foraging area use through tracking individuals from the primary and major secondary nesting beaches. In addition, nesting beaches of this species are heavily monitored annually to obtain accurate nest counts and nesting distributions to inform the proportion of the population each nesting beach represents (Márquez-Millán et al., 2005; NMFS and USFWS, 2015; Shaver et al., 2016b). These factors allow targeted, long-term tracking studies to be used to identify the preferred foraging areas of post-nesting females to infer population- and species-level spatial dynamics of reproductively active Kemp’s ridley females. This study aimed to: (1) assess the proportional distribution of the foraging adult female Kemp’s ridley population within the GoM through concurrent tracking of females from the primary and major secondary nesting beaches; (2) determine key foraging areas for the adult female population; and (3) determine fidelity of females to foraging areas over time through repeated tracking of individuals to the foraging grounds. Results from objectives 1–3 were then used collectively to determine the overall importance of each GoM region to the female population. This information can then be used to inform conservation and management planning, as well as serve as a model for identifying spatial population dynamics of other mobile marine megafauna species.
Materials and Methods
Tracking
A total of 178 adult female Kemp’s ridley sea turtles were outfitted with satellite transmitters after nesting at each of the major nesting beaches in the western GoM (NMFS and USFWS, 2015) between 2010 and 2017: Padre Island National Seashore, Texas, United States (PAIS, n = 76, 2010–2017); Rancho Nuevo, Tamaulipas, Mexico (RNMX, n = 44, 2010, 2011, 2014–2016); Tecolutla, Veracruz, Mexico (VCMX, n = 58, 2012–2017) (Table 1 and Supplementary Table S1). Each individual received a platform terminal transmitter (PTT) manufactured by Wildlife Computers (SPOT: n = 32, 2013–2015 or SPLASH: n = 24, 2010–2013) or Sirtrack (Kiwisat 101: n = 29, 2011–2013 or Kiwisat 202: n = 93, 2014–2017) (Table 1). Straight carapace lengths (nuchal notch to posterior tip, SCLs) were obtained at the time of PTT attachment for all turtles using straight, metal calipers or converted from curved carapace lengths (CCLs) using the equation developed by Schmid and Witzell (1997). PTTs were attached using the methods described in Shaver et al. (2013).
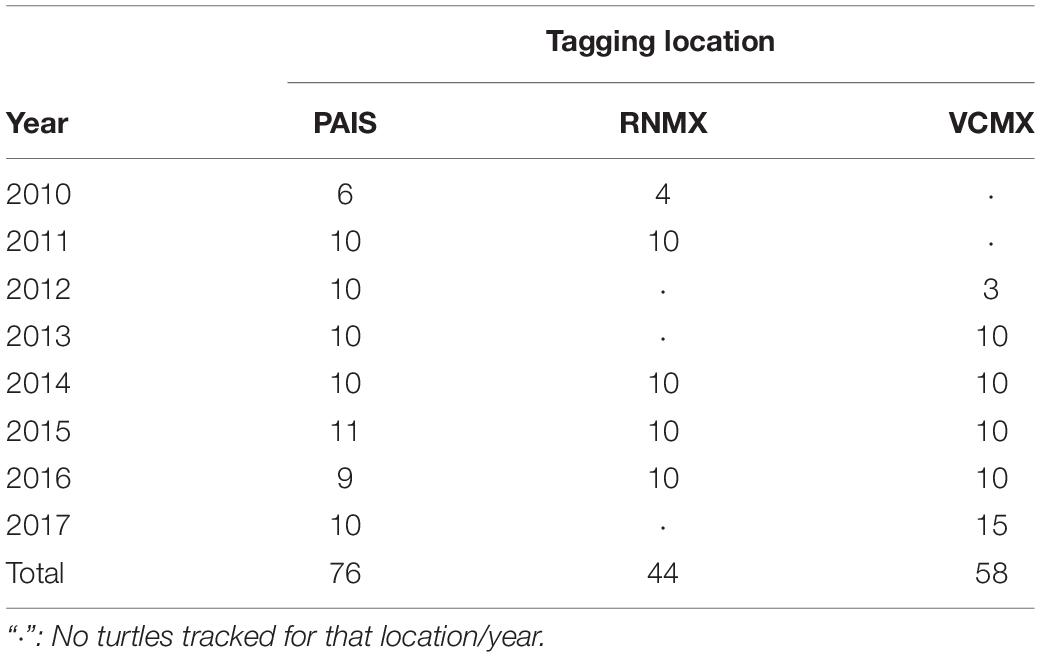
Table 1. Tagging locations and years for satellite transmitters deployed between 2010 and 2017 of 178 post-nesting female Kemp’s ridley sea turtles tracked from the primary and major secondary nesting beaches: Padre Island National Seashore, Texas, United States (PAIS); Rancho Nuevo, Tamaulipas, Mexico (RNMX); Tecolutla, Veracruz, Mexico (VCMX).
Platform terminal transmitters were programmed in the following ways: continuously on (n = 44, 2010–2014), 24-h on/24-h off (n = 10, 2013) and 6-h on/6-h off (n = 75, 2010–2017). PTTs transmitted data using the ARGOS system, which estimates each location using the following classes: 3, <250 m; 2, 250 to <500 m; 1, 500 to <1500 m; 0, >1500 m; A and B, unknown; Z, failed plausibility tests (CLS, 2011). Six PTTs deployed in 2010 were processed using least-squares analysis. The remaining PTTs were processed using the Kalman filter, which provides improved accuracy and increases the number of estimated positions (Lopez and Malardé, 2011). Kalman filtering was unavailable for the six PTTs deployed in 2010 processed using least-squares analysis. To ensure there were enough locations to identify primary foraging regions, only individuals tracked for at least 30 days after the mean last date of nesting for each specific nesting beach were used in analyses. Any tracks eliminated from analysis were confirmed to still be within or near the inter-nesting area at the last transmission. Mean last date of nesting was calculated separately for each of the three beaches using the last nesting date of each beach for each of the PTT deployment years to account for temporal differences in nesting seasons for each nesting beach. Eleven individuals were recaptured and tracked up to three times. In these instances, all tracking data were used if tracking durations were long enough. All activities were carried out according to protocols approved by the National Park Service Institutional Animal Care and Use Committee.
Modeling
A switching state-space model (SSM) was used to estimate locations and behavioral modes of each individual to determine area-restricted-search-type movements (ARS; i.e., “foraging” or “inter-nesting”) and migratory-type movements (i.e., “exploratory” or “transit”). Because satellite-location data are often received at irregular time intervals and can contain large positional errors and temporal gaps, SSMs provide a means to estimate the most likely movement patterns of an animal and account for these errors while using the specific dynamics of a species’ movement patterns (Jonsen et al., 2005). Location data for each individual were first fit with a continuous-time correlated random walk (CTCRW) model to predict temporally regular locations in R using the “crawl” package (Johnson, 2018; R Core Team, 2019) with an initial value centered on their release location. The CTCRW model was fit using a prior distribution and estimated the location error from ARGOS estimates. For PTTs processed using least-squares, variance parameters were fixed using ARGOS provided error estimates for the three highest quality location classes (3, 2, and 1) to estimate the error parameters. For PTTs processed using Kalman filtering, ARGOS provided error ellipse information was used (McClintock et al., 2015). Locations for each individual were simulated at 6-h time-steps over 1,000 imputations. SSMs were then modeled using a two-state continuous-time hidden Markov model using the R package ‘momentuHMM’ from the simulated CTCRW tracks over 1,000 imputations (McClintock and Michelot, 2018). Model parameters were set using the gamma distribution for step lengths and the wrapped Cauchy distribution for turning angles. Parameter values were set at 750 ± 200 m (ARS) and 2,000 ± 750 m (migratory) for step lengths and π ± 0.1 rad (ARS) and 0 ± 0.7 rad (migratory) for turning angles.
Primary Foraging Regions and Dispersal
After defining movement types, migratory and inter-nesting movements, locations on land and those interpolated during tracking gaps ≥ 7 days were removed from the SSM-derived locations for foraging area analyses. Inter-nesting locations were determined from the ARS locations using the mean last date of nesting for each nesting beach. For individuals that remigrated back to the nesting beach, inter-nesting was considered to begin after a migration that coincided with the nesting season (March–July) (NMFS and USFWS, 2015). For dispersal analyses, migratory movements after the mean last date of nesting were retained in SSM-derived location data.
For PTT data that failed to converge using SSM, locations were filtered by removing locations on land, that exceeded 5 km/h, had turning angles greater than 25° or were in depths greater than 100 m. The 100 m isobath was used as greater depths have been shown to be biologically implausible for Kemp’s ridleys (Shaver and Rubio, 2008; Seney and Landry, 2011). Filtered locations were used to include those individuals whose data failed to converge using SSM, but met all other criteria (e.g., tracking duration), in dispersal analyses. Mean daily locations (MDLs) were then calculated from SSM-derived and filtered locations using a custom script in R to normalize the data for all analyses. MDLs represented the centroid location of all SSM-derived or filtered locations for each day locations were available.
To determine primary foraging regions, the GoM was divided into two major areas: northern GoM (nGoM) and southern GoM (sGoM). The Exclusive Economic Zone (EEZ) boundary between the United States and Mexico demarcated the division between the nGoM and sGoM. The EEZ was chosen as the boundary as it provided a known, defined border between different environmental, anthropogenic, and political factors that may affect marine turtle turtles in the GoM. Each region included all marine waters from the coast out to the 100 m isobath in the corresponding direction from the EEZ boundary. The Atlantic coasts of Florida and Georgia were included as part of the nGoM to simplify the region.
A 25 km hex-bin grid was used to calculate the number of foraging days and identify areas of increased use throughout the Gulf using SSM-derived MDLs of ARS locations identified as foraging. This grid size was chosen as a compromise between improved data visualization and matching the spatial error of MDLs. A 10 km hex-bin grid was also investigated for comparisons to previous studies of female Kemp’s ridley movements (Shaver et al., 2016a, 2017; Hart et al., 2018a, b) and is presented in the Supplementary Material. The ratio of SSM-derived or filtered MDLs in each GoM region was also calculated for each individual to determine regional dispersal of females from the primary and major secondary nesting beaches throughout the Gulf. Individuals with ≥70% of filtered or SSM-derived MDLs within a region were assigned to that region. The percentage of the total adult female Kemp’s ridley population that forages in the nGoM and sGoM was then calculated using the relative proportion of the nesting population that each nesting beach represented. Relative proportions were calculated using the mean percentage of nests laid in each nesting region (Texas, Tamaulipas, and Veracruz states) between 2010 and 2017 as a proxy for adult female population size.
Regional and Foraging Area Fidelity
For individuals tracked multiple times and those tracked remigrating to the nesting beach, and then returning to foraging areas, tracks were compared between deployments and migrations to determine fidelity to Gulf regions. Utilization distributions (UDs) were then calculated using 95% kernel-density estimates (KDEs) for PTTs with SSM-derived foraging MDLs for all deployments, or during remigrations, to assess fidelity to specific foraging areas. A fixed-kernel least-squares cross-validation smoothing factor (hcv) was used to calculate each 95% KDE. KDEs were compared for each turtle using the UD overlap index (UDOI) (Fieberg and Kochanny, 2005) and Bhattacharyya’s affinity (BAs) (Bhattacharyya, 1943). The UDOI and BA are statistics used to determine the amount of spatial overlap in three-dimensional UDs. Values of zero describe UDs with no overlap, while values of one indicate 100% overlap. The UDOI value can be greater than one if UDs overlap significantly, but are not uniformly distributed (Fieberg and Kochanny, 2005). The UDOI has been found to be the best estimator for describing the degree of space sharing and the BA is better suited for comparing the overall similarity between UDs (Fieberg and Kochanny, 2005); thus, both are reported here. KDEs, UDOIs, and BAs were calculated in R using the package “adehabitatHR” (Calenge, 2006). All other spatial analyses were conducted in ArcGIS 10.7.
Results
Tracking
Individuals measured between 58.0 and 75.2 cm SCL (mean ± SD: 63.9 ± 2.7 cm). Turtles were tracked from three to 1,554 days (mean ± SD: 368 ± 313 days) for a total of 65,466 tracking days. Overall, 150 PTTs (PAIS: n = 68; RNMX: n = 37; VCMX: n = 45) deployed at the primary and major secondary nesting beaches between 2010 and 2017 provided enough data to identify regional dispersal of individuals using SSM-derived MDLs or, for datasets that did not converge using SSM, filtered MDLs. Of these 150 PTTs, foraging areas were modeled for 117 PTTs (PAIS: n = 55; RNMX: n = 27; VCMX: n = 35) using SSM (Figure 1). Data from 33 PTTs did not converge using SSM and 28 PTTs had deployments too short in time for analyses. During the study period, 10 turtles were tracked twice, and one turtle was tracked three times from their respective nesting beaches. Eight of these individuals tracked multiple times had tracking durations long enough (≥30 days) for analyses. In addition, seven turtles were tracked migrating back to their nesting beaches during deployments. All remigrant turtles had tracking durations long enough for analyses. No individuals remigrated to nesting beaches other than the beach they were initially tracked from.
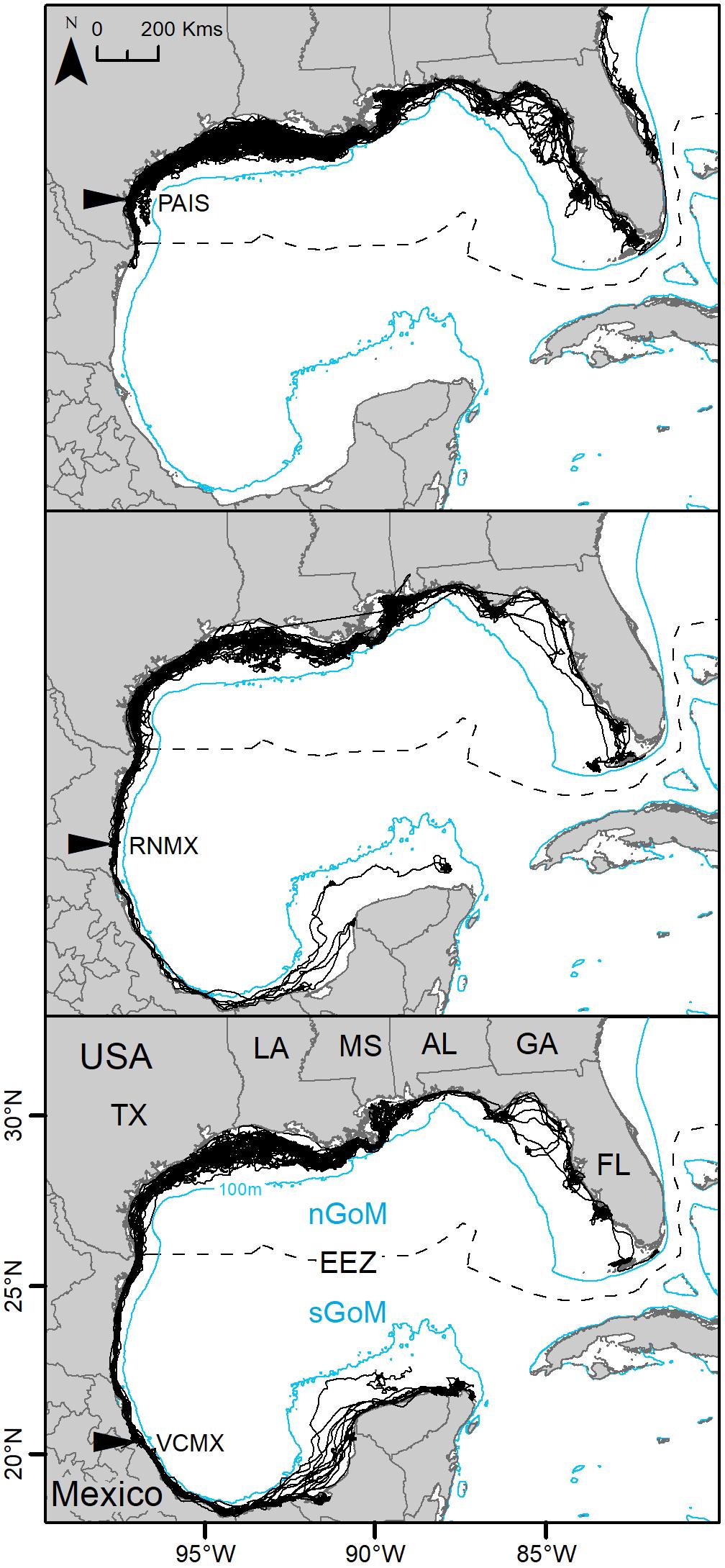
Figure 1. Switching state-space model-derived tracks for 117 post-nesting female Kemp’s ridley sea turtles tracked from the primary and major secondary nesting beaches: Padre Island National Seashore, Texas, United States (PAIS); Rancho Nuevo, Tamaulipas, Mexico (RNMX); Tecolutla, Veracruz, Mexico (VCMX). The Exclusive Economic Zone (EEZ) demarcates the division between the northern Gulf of Mexico (nGoM) and southern Gulf of Mexico (sGoM). Transmitters were deployed between 2010 and 2017.
Primary Foraging Regions and Dispersal
Gulf of Mexico regions were assigned to all PTTs that provided enough data for analyses (n = 150). In total, 41,591 foraging days were modeled with 86.1% in the nGoM and 13.9% in the sGoM. Both regions of the Gulf contained areas with high numbers of foraging days with hotspots near the Yucatán Peninsula, northern and southern Gulf coasts of Florida, including the Florida Keys, the Mississippi River Delta, and the Texas-Louisiana Shelf (Figure 2 and Supplementary Figure S1). Almost all PAIS turtles foraged in the nGoM with 99.8% of SSM-derived foraging days in the region and 98.5% of individuals dispersed to northern Gulf waters (Figure 3, Supplementary Figure S2, and Table 2). Only one individual tracked from PAIS (PTT 117512) migrated to the sGoM with its track ending offshore of the Yucatán Peninsula. Two turtles (PTTs 117515/152803 and 152804), one of which was tracked twice, foraged offshore of PAIS for the entirety of their tracking. One turtle (PTT 152808) foraged along the Atlantic coasts of Florida and Georgia (Figure 4). Individuals tracked from RNMX also primarily foraged in the nGoM with 90.7% of SSM-derived foraging days in the region and 83.8% of individuals dispersed to the nGoM (Figure 3, Supplementary Figure S2, and Table 2). Conversely, individuals tracked from VCMX were almost evenly split with 57.8% dispersing to the nGoM and 65.5% of SSM-derived foraging locations were located there (Figure 3, Supplementary Figure S2, and Table 2). No turtles from RNMX or VCMX foraged near the nesting beach they were tracked from.
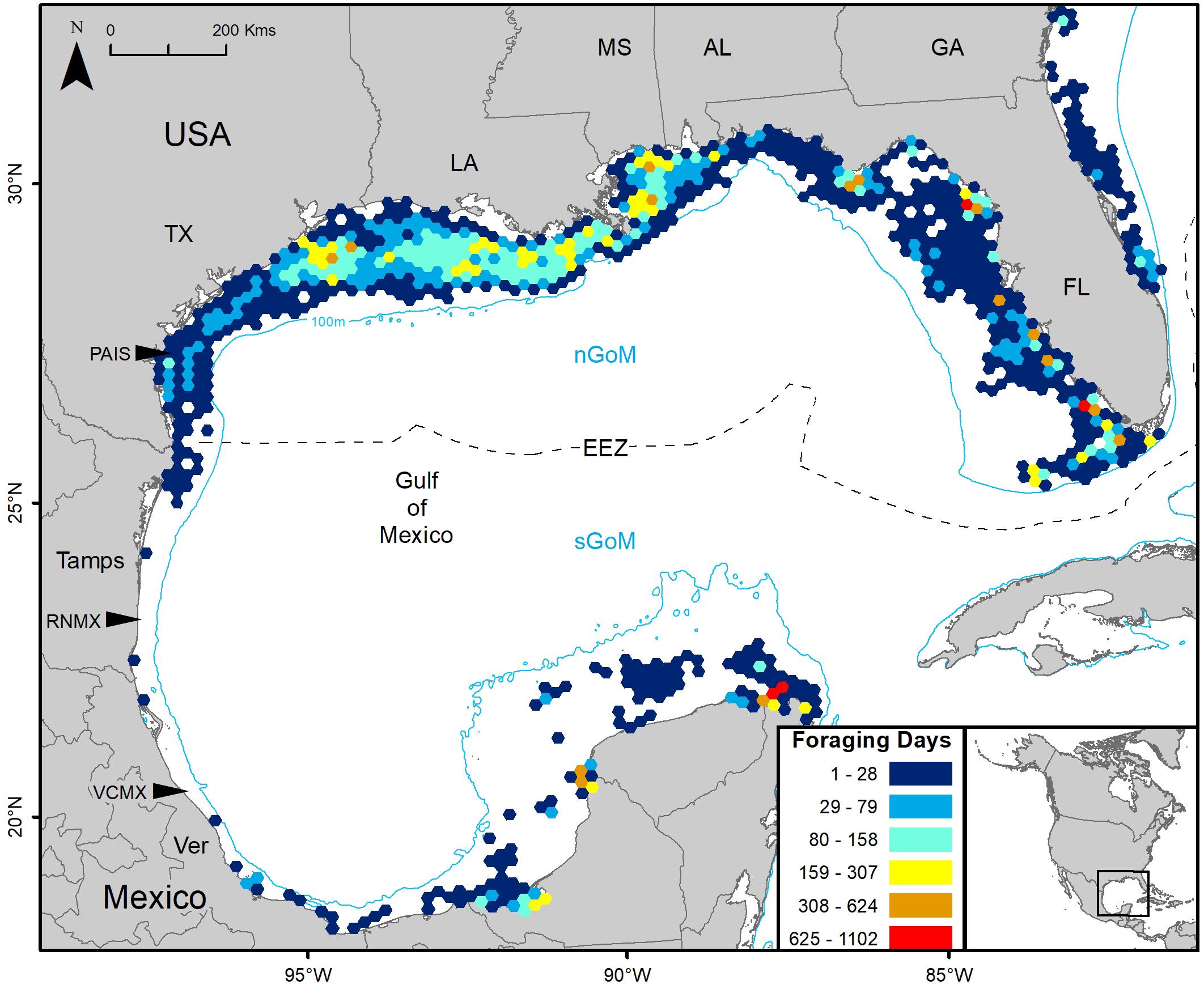
Figure 2. Switching state-space model-derived foraging days for 117 post-nesting female Kemp’s ridley sea turtles tracked from the primary and major secondary nesting beaches summarized in 25 km hex-bin cells: Padre Island National Seashore, Texas, United States (PAIS); Rancho Nuevo, Tamaulipas (Tamps), Mexico (RNMX); Tecolutla, Veracruz (Ver), Mexico (VCMX). The Exclusive Economic Zone (EEZ) demarcates the division between the northern Gulf of Mexico (nGoM) and southern Gulf of Mexico (sGoM). Transmitters were deployed between 2010 and 2017.
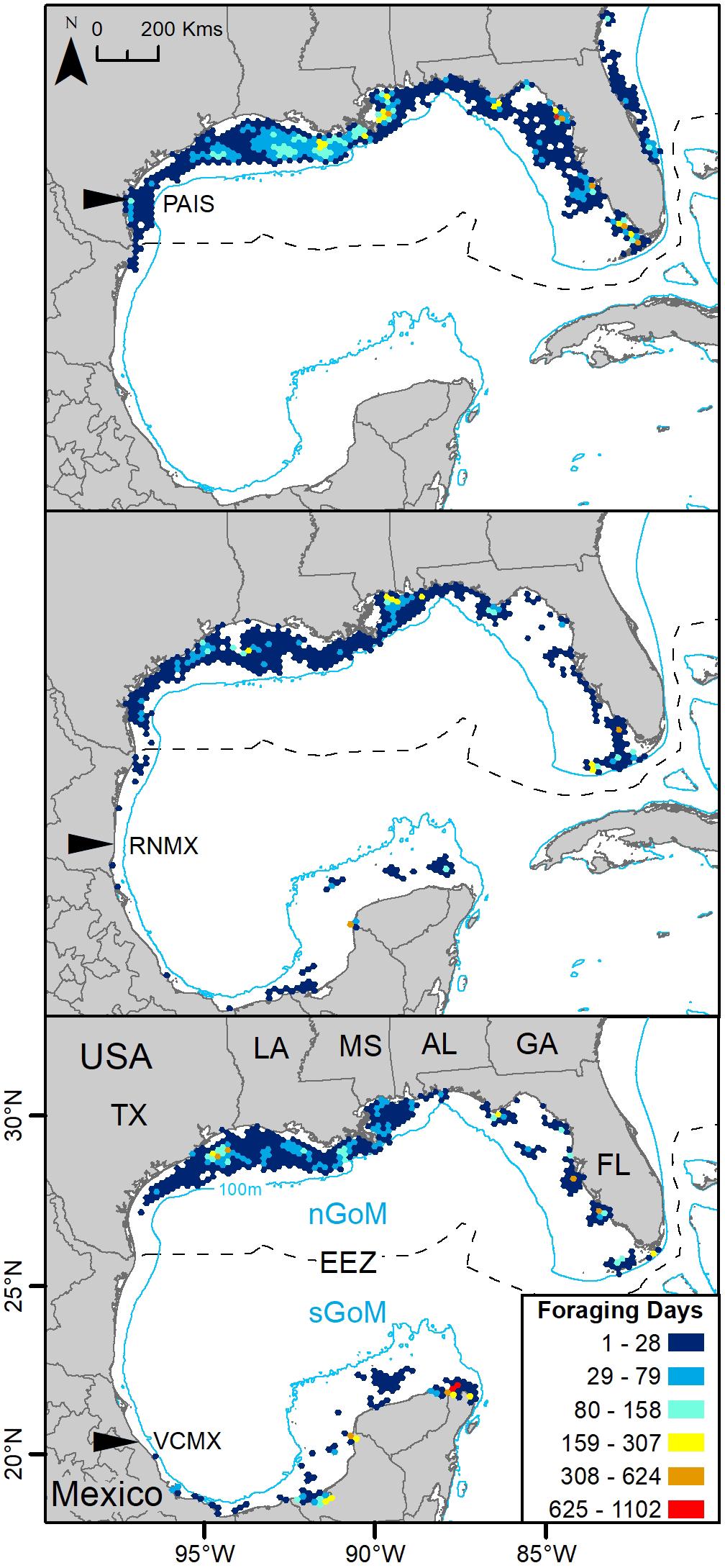
Figure 3. Switching state-space model-derived foraging days for 117 post-nesting female Kemp’s ridley sea turtles tracked from the primary and major secondary nesting beaches summarized in 25 km hex-bin cells and divided by deployment location: Padre Island National Seashore, Texas, United States (PAIS); Rancho Nuevo, Tamaulipas, Mexico (RNMX); Tecolutla, Veracruz, Mexico (VCMX). The Exclusive Economic Zone (EEZ) demarcates the division between the northern Gulf of Mexico (nGoM) and southern Gulf of Mexico (sGoM). Transmitters were deployed between 2010 and 2017.
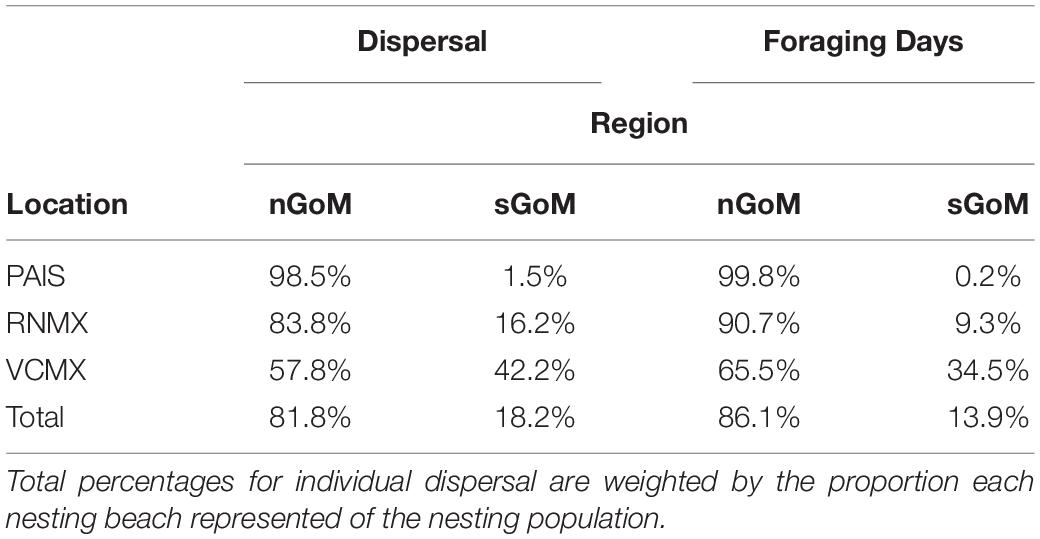
Table 2. Proportions of individual dispersal (Dispersal) and switching state-space model-derived foraging days (Foraging Days) of post-nesting female Kemp’s ridley sea turtles in the northern Gulf of Mexico (nGoM) and southern Gulf of Mexico (sGoM) tracked from the primary and major secondary nesting beaches: Padre Island National Seashore, Texas, United States (PAIS); Rancho Nuevo, Tamaulipas, Mexico (RNMX); Tecolutla, Veracruz, Mexico (VCMX).
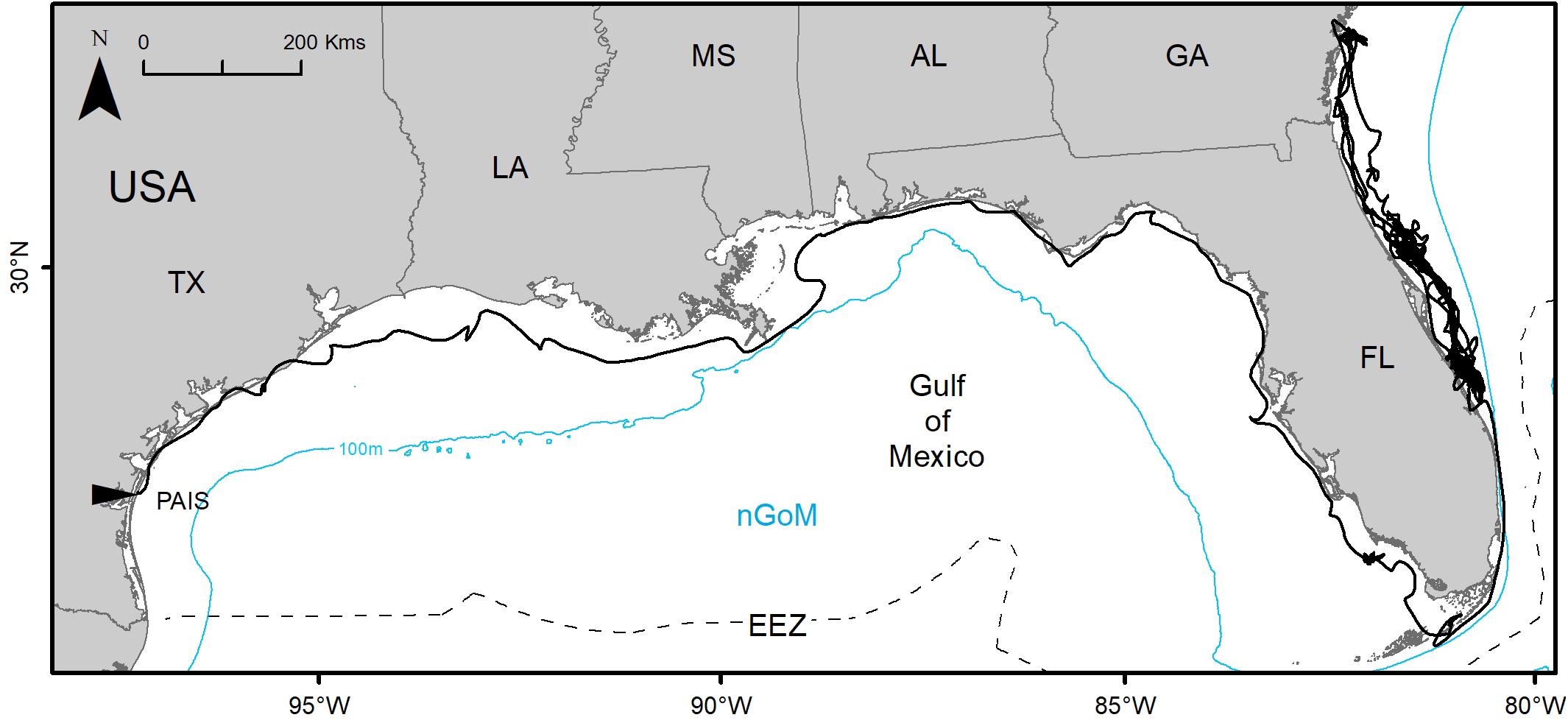
Figure 4. Switching state-space model-derived track of PTT 152808 tracked between 02 July 2016 and 24 March 2019 (995 days) from Padre Island National Seashore, Texas, United States (PAIS) to the Atlantic coasts of Florida and Georgia, United States. The Exclusive Economic Zone (EEZ) demarcates the division between the northern Gulf of Mexico (nGoM) and southern Gulf of Mexico.
All SSM-derived turtle tracks remained within the nearshore waters (≤100 m depth) of the GoM and Atlantic coast of the United States (Figure 1). In general, individuals foraged in either the nGoM or sGoM, but not both, and did not transition between regions over time to forage. However, there were two exceptions to this: (1) some individuals who remained within the coastal waters of southern Texas to forage and (2) individuals displaying short-duration (≤10 days) ARS-type behavior during migrations from nesting beaches in Mexico. One individual (PTT 152804) tracked from PAIS, which primarily foraged offshore of PAIS in the nGoM during its 387-day tracking period, briefly foraged in sGoM waters for 45 days before returning to its main foraging area off the south Texas coast. This individual had a maximum incursion distance into sGoM waters of 76.2 km (mean ± SD: 46.0 ± 25.7 km) from the EEZ border. Additionally, two individuals (PTTs 126252 and 126257) tracked from RNMX displayed ARS-type behavior within the sGoM near the United States/Mexico border (mean ± SD: 47.2 ± 24.4 km south of the United States/Mexico EEZ) during their migrations to nGoM foraging areas. These individuals undertook ARS-type behaviors in the sGoM during periods just after the completion of nesting seasons in Mexico (August–September). However, these individuals displayed ARS-type behavior for ≤10 days before continuing their migrations north into the nGoM where they remained for the duration of their tracking periods.
The majority of Kemp’s ridley nests (90.8%) were laid on the beaches of Tamaulipas, Mexico, concentrated at RNMX, between 2010 and 2017. The beaches of Veracruz, Mexico and Texas, United States accounted for relatively minor proportions of the nesting population with 8.2 and 1.0% of nests, respectively. Nesting in both of these states was concentrated at VCMX and PAIS. Using these proportions to weight the dispersal of tracked females from their nesting grounds to foraging areas indicated that 81.8% of the adult female population may use the nGoM as their primary foraging area (Table 2).
Regional and Foraging Area Fidelity
Eight of the 11 turtles that were tracked more than once (2X: n = 7, 3X: n = 1) had tracking durations long enough (≥30 days) during each deployment to assess regional fidelity over time. All eight individuals used the nGoM, following similar migratory corridors each time they were tracked, displaying strong fidelity to the region (Figure 5). One individual foraged offshore from PAIS during both deployments. All other turtles migrated away from PAIS. The mean time between repeat tracking was 4.0 ± 1.3 (mean ± SD) years.
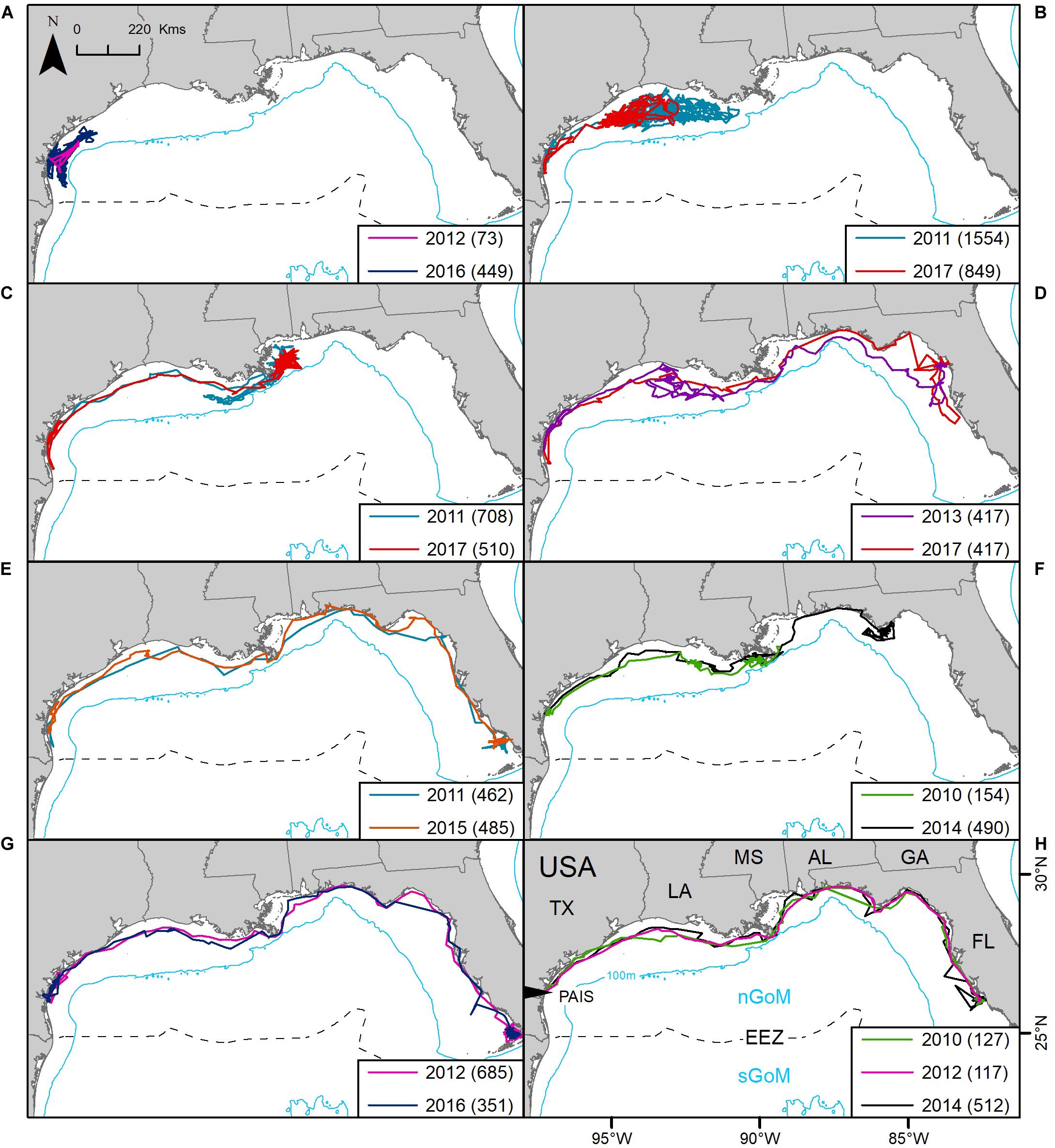
Figure 5. Repeat tracking of eight post-nesting female Kemp’s ridley sea turtles in the northern Gulf of Mexico tracked from Padre Island National Seashore, Texas, United States (PAIS): (A) platform terminal transmitters (PTTs) 117515 and 152803; (B) PTTs 101137 and 152828; (C) PTTs 101138 and 152827; (D) PTTs 112763 and 152829; (E) PTTs 106343 and 141781; (F) PTTs 47562 and 133286; (G) PTTs 112758 and 152802; (H) PTTs 47524, 112762 and 133285. The Exclusive Economic Zone (EEZ) demarcates the division between the northern Gulf of Mexico (nGoM) and southern Gulf of Mexico (sGoM). Numbers in parentheses equal the number of tracking days for each deployment. Tracks were reconstructed from mean daily locations of filtered location data. Transmitters were deployed between 2010 and 2017.
Seven turtles were tracked remigrating to the nesting beach and then returning to forage during their deployments, including one of the turtles that was tracked twice. All individuals used the same region of the Gulf during successive migrations to their preferred foraging grounds, using the same migratory corridor each time (Figure 6). The mean time between remigrations to the nesting beach was 1.4 ± 0.5 (mean ± SD) years, but remigration intervals reported here only represent those individuals whose transmitters remained active long enough to capture a remigration during their tracking period. Seventy-six females were tracked ≥ 1 year, with seven of those tracked through a remigration, and 38 females were tracked for ≥ 1.5 years, with five of those remigrating within that period (Supplementary Table S1). Remigrating turtles in the nGoM initiated returns to the nesting beach during November and December while individuals returning from the sGoM began their migrations in February and March.
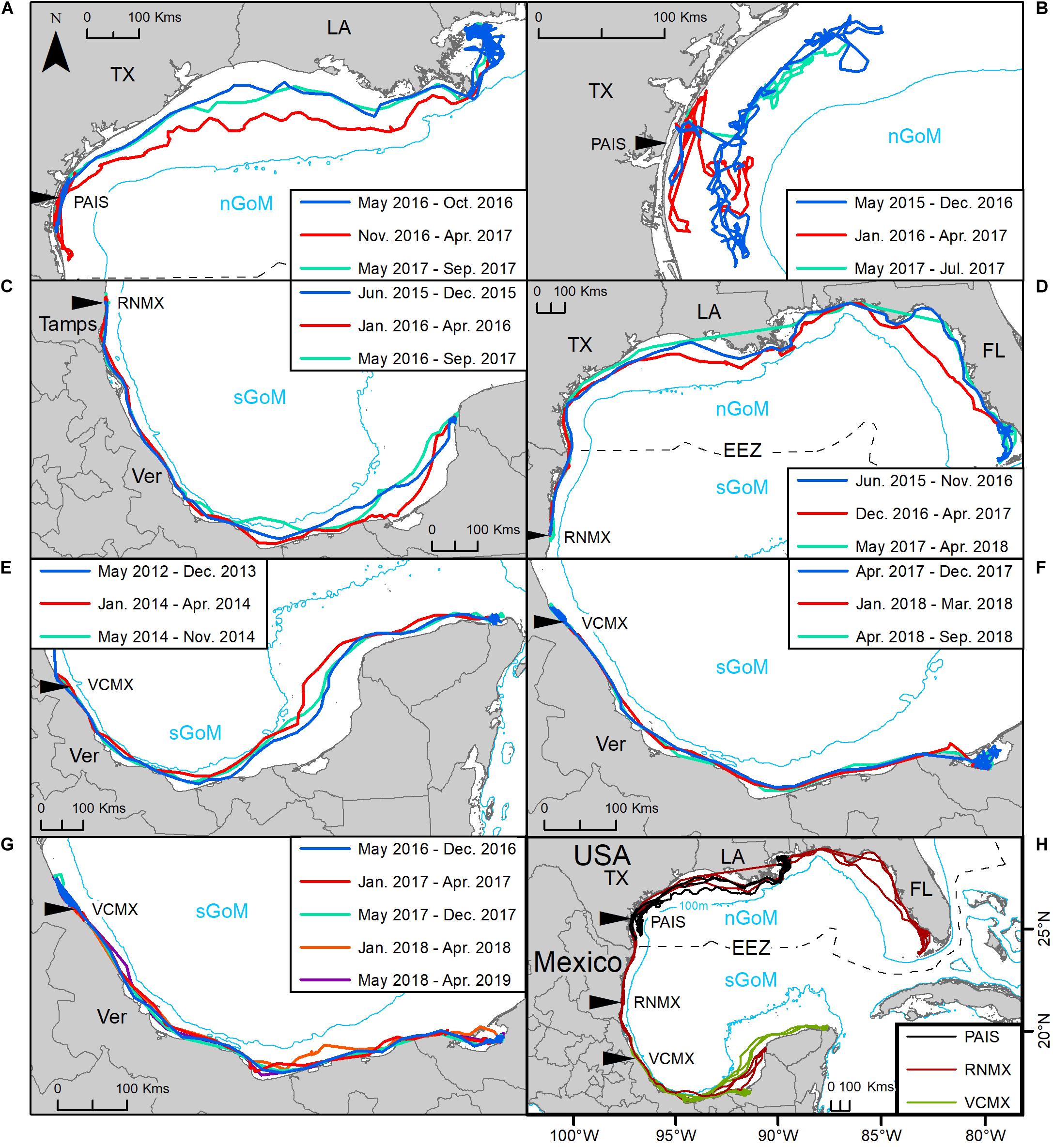
Figure 6. Tracking of seven post-nesting female Kemp’s ridley sea turtles from the primary and major secondary nesting beaches that remigrated from foraging areas to their nesting beach: (A,B) Padre Island National Seashore, Texas, United States (PAIS); (C,D) Rancho Nuevo, Tamaulipas (Tamps), Mexico (RNMX); (E–G) Tecolutla, Veracruz (Ver), Mexico (VCMX). (H) Tracks of all individuals. The Exclusive Economic Zone (EEZ) demarcates the division between the northern Gulf of Mexico (nGoM) and southern Gulf of Mexico (sGoM). Tracks were reconstructed from mean daily locations of switching state-space model-derived location data. Transmitters were deployed between 2010 and 2017. Line colors correspond to each migration; blue: first migration from the nesting beach to foraging grounds; red: first return migration to the nesting beach; turquoise: second return migration from the nesting beach to foraging grounds; orange: second return migration to the nesting beach; purple: third migration from the nesting beach to foraging grounds.
Individuals also showed fidelity to specific foraging areas. Twelve individuals that were tracked multiple times (n = 5), remigrated to the nesting beach and then back to foraging grounds during their initial deployment (n = 6) or both (n = 1) had data robust enough for SSM analysis to identify specific foraging areas and determine recurring use. For all of these individuals, overlap indices (i.e., UDOI and BA) indicated spatial overlap in their preferred foraging areas after each migration from the nesting beach (mean ± SD: 0.8 ± 0.7 UDOI; 0.6 ± 0.2 BA) (Figure 7). Turtles that remigrated during tracking displayed fidelity over successive nesting years (mean ± SD: 0.9 ± 0.8 UDOI; 0.6 ± 0.2 BA) and turtles that were tracked more than once displayed fidelity to foraging grounds over time (mean ± SD: 0.7 ± 0.6 UDOI; 0.6 ± 0.2 BA).
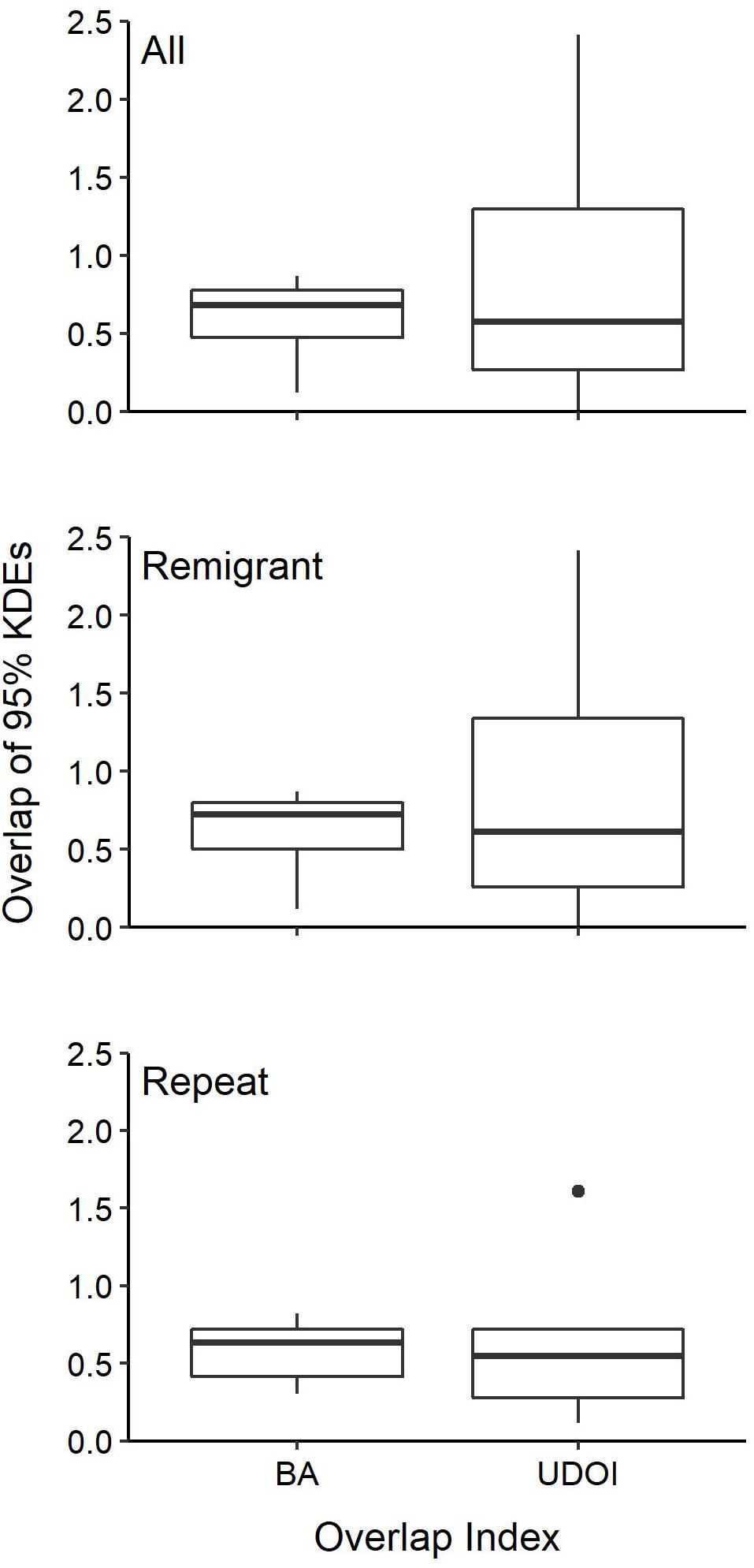
Figure 7. Kernel-density estimates (KDEs) of foraging area overlap for individual post-nesting female Kemp’s ridley sea turtles with transmitters deployed between 2010 and 2017. Individuals were tracked multiple times (Repeat) or tracked remigrating back to the nesting beach and then returning to the foraging grounds during their deployment (Remigrant). Remigrant and Repeat tracks were also combined (All) to show overall intra-individual overlap of foraging areas. BA, Bhattacharyya’s affinity; UDOI, utilization distribution overlap index. Boxes bound the 25th and 75th percentiles, the solid line indicates the median, dots represent outliers, and the whiskers extend 1.5 times the interquartile range.
Discussion
Long-term, concurrent tracking of adult female Kemp’s ridley sea turtles from the primary and major secondary nesting beaches identified dispersal patterns to key foraging areas within the GoM. This study adds an additional five years of tracking data and 115 adult female Kemp’s ridleys not previously described in the literature, with a significantly increased sample size from VCMX. Female Kemp’s ridleys showed preferential selection for nearshore nGoM foraging areas, with the majority of the female population likely using these areas to forage. The proportion of the female population from each of the primary and major secondary nesting beaches using the nGoM to forage decreased with latitude, with more individuals dispersing to the sGoM from more southerly nesting beaches. Individuals showed fidelity to foraging regions over time, with animals migrating back to the same foraging regions (nGoM or sGoM) they used previously after each nesting season and when tracked more than once. Turtles also displayed strong fidelity to previously used foraging grounds, with large overlaps between individual foraging areas after repeat migrations or additional PTT deployments. Furthermore, migratory routes remained consistent for individuals between migrations and deployments. No individuals were observed nesting in areas other than the one they were initially tracked from, indicating fidelity to nesting beaches. These results are consistent with patterns seen in Kemp’s ridleys and other marine megafauna species, showing strong fidelity to foraging sites, migratory corridors, and nesting/breeding areas (Shaver and Rubio, 2008; Kelly et al., 2010; Horton et al., 2017; Shimada et al., 2019). Because large numbers of individuals were tracked from regions representing nearly 100% of the nesting population (NMFS et al., 2011), these results have the potential to be applied to the entire adult female Kemp’s ridley population to infer population-level and species-level spatial dynamics.
Results complement and add to findings from previous work identifying key foraging areas and migratory corridors of female Kemp’s ridleys, showing consistency over 22 years of satellite tracking (Seney and Landry, 2008, 2011; Shaver and Rubio, 2008; Shaver et al., 2013, 2016a; Hart et al., 2018a, b). SSM identified additional foraging areas along the panhandle and central coast of Florida and the Yucatán Peninsula, adding to previously identified foraging areas in these regions (Shaver et al., 2013; Hart et al., 2018a, b). Furthermore, foraging areas were identified along the Atlantic coasts of Florida and Georgia that had previously not been described. The importance of the U.S. Atlantic coast to adult Kemp’s ridleys is still largely unknown, with only one confirmed migration from the nesting beaches to the region (PTT 152808), as are the mechanisms leading to these migrations or if these individuals return to the GoM to nest in later years. These additional foraging areas may be the result of increased tracking of individuals, which were not identified in previous studies, indicate a shifting range of female Kemp’s ridleys over time or are controlled by other environmental factors (e.g., seasonal currents, resource availability). Further analysis is warranted to describe these differences. However, foraging areas on the Texas-Louisiana Shelf and off of the Mississippi River Delta in the nGoM remain the most heavily used areas (Shaver and Rubio, 2008; Shaver et al., 2013; Hart et al., 2018a, b).
It is still unknown how Kemp’s ridleys, and marine turtles in general, select their key foraging areas and distribute themselves spatially. However, it has been suggested that dispersal of hatchlings by oceanic circulation patterns may play a role in determining where individuals recruit to forage as adults. Specifically, individuals recruiting to neritic foraging areas from the pelagic zone may retain fidelity to productive or known areas as they mature, either through imprinting to geomagnetic fields, memory or other factors (Hays et al., 2010; Putman et al., 2015a, b; Shimada et al., 2019). Consequently, oceanic circulation patterns may explain preferential use of the nGoM. In fact, dispersal modeling of hatchlings from the primary and major secondary Kemp’s ridley nesting beaches indicates a strong relationship between where hatchlings are predicted to disperse and where identified key adult foraging areas are located, with most hatchlings predicted to disperse to U.S. nGoM waters (Putman et al., 2010, 2020). Other modeling work has suggested that the location of the major nesting beaches correlates to areas with circulation patterns which are more likely to transport hatchlings to productive foraging grounds (Putman et al., 2010). Thus, the distribution of key foraging areas may be interlinked between hatchling dispersal patterns, major nesting beach locations, and resource availability. In light of this, key foraging areas may have the potential to shift due to predicted changes in ocean circulation patterns and resource distributions caused by climate change (Pikesley et al., 2015; Willis-Norton et al., 2015). For example, sGoM sites appear to provide suitable habitat for juvenile and adult Kemp’s ridleys (Carr, 1980; Márquez-Millán, 1994). However, these areas are seemingly underused by the species, with approximately 18% of the female population foraging in the region.
This study focused only on the movements and distributions of reproductively active female Kemp’s ridleys from the primary and major secondary nesting beaches who had successfully nested at least once during their lifetime. While Kemp’s ridleys have been reported to nest in other areas along the GoM and U.S. Atlantic coast, these events are rare, accounting for only a few individuals annually, and are not considered significant portions of the population (NMFS et al., 2011; Shaver and Caillouet, 2015). However, it is unknown if additional foraging areas are used by females on the verge of reaching maturity, newly mature individuals who have not yet nested in their lifetime or those that are no longer in the breeding population (e.g., post-reproductive, chronically ill, or disabled individuals). Thus, these portions of the female population and the foraging areas they use, if different, were not captured in this study. Further investigations are needed to identify potential differences in foraging distributions of other life-stages.
In addition, only a few studies have investigated the movement patterns of adult males, with comparatively low numbers of individuals tracked (Shaver et al., 2005; Hughes and Landry, 2016). Nevertheless, tracking has shown that some males appear to overlap with female distributions in the nGoM, specifically on the Texas-Louisiana Shelf, and use the same migratory corridor as females (Shaver et al., 2005; Hughes and Landry, 2016). The spatial overlap of some males and females in this area of the northwestern GoM reinforces the importance of the region as key foraging habitat for the species and spatial protections and policies may encompass both genders. However, many of the males tracked remained in the vicinity of the primary and major secondary nesting beaches throughout the year (Shaver et al., 2005). This is in sharp contrast to female migration patterns, where very few individuals remain near the nesting beaches outside of the nesting season. The results of the current and previous studies suggest that additional research and conservation actions may be needed to protect male Kemp’s ridleys in their foraging grounds. Specifically, key areas are still undescribed, and males may not follow the same patterns as females for site selection, making spatial protections for females potentially inadequate for males. In fact, increased protections of males are becoming progressively more important due to the predicted feminization of marine turtle populations as a result of climate change (Jensen et al., 2018; Patrício et al., 2019). Thus, additional studies should be undertaken to determine key foraging areas and distributions of males to inform a complete picture of adult Kemp’s ridley spatial population dynamics. In addition, spatial segregation of genders has also been shown in other mobile marine megafauna species such as blue sharks (Prionace glauca) (Maxwell et al., 2019), Indo-Pacific bottlenose dolphins (Tursiops aduncus) (Fury et al., 2013), and Australian fur seals (Arctocephalus pusillus doriferus) (Kernaléguen et al., 2015) highlighting the need for research across genders to determine the spatial dynamics of a species.
Conservation Implications
Preferential selection of nGoM waters as foraging grounds, fidelity to foraging regions, and narrow migratory corridors across the Gulf indicate that the adult female Kemp’s ridley nesting population may be more susceptible to regional threats and that the nGoM may be the most significant foraging region for females of the species. Importantly, point source events have the potential to significantly affect a high proportion of the nesting population in the foraging grounds or along migratory routes. For example, the Deepwater Horizon oil spill in 2010 may have had a population-wide effect on nesting females due to its centralized location within the nGoM, overlap with key foraging areas and lying directly within the migratory corridor (Beyer et al., 2016; Shaver et al., 2016a; Hart et al., 2018a). Due to its location, any individuals migrating to central or eastern nGoM foraging grounds, or returning to the nesting beaches, would have had to pass directly through the oiled area. In fact, it has been estimated that >50% of Kemp’s ridleys that forage in the region may have been exposed to oil (Reich et al., 2017) and it has been hypothesized that the spill may have affected, in part, the recovery of the species (Caillouet, 2014; Gallaway et al., 2016a, b).
The nGoM region is also used heavily by a variety of anthropogenic activities, including commercial fisheries, commercial shipping traffic, and oil and gas production (Hart et al., 2018a). Shrimp trawling has been linked with high incidents of marine turtle bycatch, with large numbers of mortalities of Kemp’s ridleys in the nGoM (Lewison et al., 2003; Finkbeiner et al., 2011) and the fishery has been plagued with compliance issues in the past (Lewison et al., 2003; Cox et al., 2007). Vessel traffic has been connected with marine turtle mortalities (Hazel and Gyuris, 2006; Casale et al., 2010) and mortalities in a host of other threatened mobile marine megafauna [e.g., North Atlantic right whales (Eubalaena glacialis) (van der Hoop et al., 2012) dugongs (Dugong dugon) (Marsh et al., 2011) manatees (Trichechus manatus) (Lightsey et al., 2006)]. Vessels have also been identified as a significant threat to marine turtles in the nGoM (Hart et al., 2018a). Hypoxic events and harmful algal blooms are becoming more common in the GoM and are predicted to increase (Brand and Compton, 2007; Justić et al., 2007) which can affect individuals, including marine turtles, and their prey resources (Landsberg et al., 2009; Capper et al., 2013; Walker et al., 2018; Foley et al., 2019).
Not only is a high proportion of the nesting population exposed to varying threats within their foraging regions, there are increasing threats along their migratory corridor, both in United States and Mexican waters. Illegal, unreported and unregulated (IUU) fishing using long-line and gill net gear, primarily targeting red snapper (Lutjanus campechanus) and sharks, may be the most significant inadequately addressed threat and marine turtles are frequently incidentally caught and killed by these operations. Large numbers of stranded marine turtles have been reported in south Texas, including adult female Kemp’s ridleys, during recent years, presumably a result of incidental capture from IUU fishing vessels, called lanchas, operating out of Mexico (Oliver and Jacobs, 2019; Donna Shaver, unpublished data). The U.S. Coast Guard reported seizing 74 of the 175 detected lanchas from south Texas waters in 2019, a new record, and have noted a significant increase of vessels since 2017 (USCG, 2019). Additionally, only an estimated 5–15% of total lancha incursions are detected annually, suggesting the total volume of illegal fishing activity in the GoM may be much higher than what has been reported (Frazer, 2020). These interactions have the potential to impact a high proportion of mature females as these operations occur directly in the primary migratory corridor leading from the nGoM to the major nesting beaches (Shaver and Rubio, 2008; Shaver et al., 2016a).
Conclusion
The nGoM appears to be the most important foraging area for adult female Kemp’s ridleys and management of threats in the region may play a significant role in the conservation and recovery of the species. Preferential use of foraging regions, consistency in foraging region selection, and fidelity to migratory corridors highlights the need for a continued bi-national cooperation between the United States and Mexico. These cooperative actions are needed for effective management and conservation of Kemp’s ridleys, with most females foraging in U.S. waters, migrating across the Mexico–United States border and nesting on Mexican beaches. This calls for maintaining and building upon existing nest and marine protection efforts in Mexico and marine protections and policies in the United States.
Data Availability Statement
All datasets presented in this study are included in the article/Supplementary Material.
Ethics Statement
The animal study was reviewed and approved by the National Park Service Institutional Animal Care and Use Committee.
Author Contributions
CG and DS contributed to the conception and design of the study and revised and wrote the final manuscript. CG conducted the modeling, performed the statistical analyses, wrote the first draft of the manuscript, and produced the figures and tables. All authors contributed to the article and approved the submitted version.
Funding
Transmitters and transmitter time were funded by the National Park Service, the Deepwater Horizon Natural Resource Damage Assessment (NRDA), and the National Fish and Wildlife Foundation. Funding for the field studies and data analyses was provided in part by the National Park Service and Deepwater Horizon NRDA. Acuario de Veracruz, Campamento Tortuguero de Rancho Nuevo, Campamento Tortuguero del Totonacapan, Campamento Tortuguero Vida Milenaria, Conservación y Desarrollo de Espacios Naturales, S.C., Gladys Porter Zoo, and NOAA-Fisheries provided equipment, funding, and/or logistical assistance.
Disclaimer
The statements and findings are those of the authors and do not necessarily reflect the views of the National Park Service or the Department of the Interior. Any use of trade, product, or firm names is for descriptive purposes only and does not imply endorsement by the U.S. Government.
Conflict of Interest
The authors declare that the research was conducted in the absence of any commercial or financial relationships that could be construed as a potential conflict of interest.
Acknowledgments
We thank the individuals and organizations that aided with detection, monitoring, and reporting of the nests included in this analysis. C. Rubio, J. S. Walker, and D. Guerrero from Padre Island National Seashore were instrumental in assisting with and organizing transmitter deployments, procurements, and initial data organization. T. Wibbels from the University of Alabama at Birmingham provided information on Rancho Nuevo, Tamaulipas, Mexico nesting dates. J. Peña from Gladys Porter Zoo deployed transmitters and provided additional information on Rancho Nuevo nesting dates. E. A. Cuevas Flores from Centro de Investigación y de Estudios Avanzados del IPN (CINVESTAV-IPN) and B. M. Zapata Najera from Cómision Nacional de Áreas Naturales Protegidas (CONANP) facilitated deployment of some transmitters in Rancho Nuevo. R. de Jesús González Díaz Mirón from the Acuario de Veracruz deployed transmitters and provided information on Tecolutla, Veracruz, Mexico, nesting dates and numbers. D. S. Johnson and B. T. McClintock from the National Marine Mammal Laboratory provided guidance and help with state-space modeling using “crawl” and “momentuHMM.” We acknowledge the use of the satellite-tracking and analysis tool (STAT) and telemetry data generated as part of the Deepwater Horizon NRDA (publicly available from www.seaturtle.org).
Supplementary Material
The Supplementary Material for this article can be found online at: https://www.frontiersin.org/articles/10.3389/fmars.2020.00559/full#supplementary-material
References
Beyer, J., Trannum, H. C., Bakke, T., Hodson, P. V., and Collier, T. K. (2016). Environmental effects of the Deepwater Horizon oil spill: a review. Mar. Pollut. Bull. 110, 28–51. doi: 10.1016/j.marpolbul.2016.06.027
Bhattacharyya, A. (1943). On a measure of divergence between two statistical populations defined by their probability distributions. Bull. Calcutta Math. Soc. 35, 99–109.
Block, B. A., Holbrook, C., Simmons, S., Holland, K., Ault, J., Costa, D., et al. (2016). Toward a national animal telemetry network for aquatic observations in the United States. Anim. Biotelemet. 4:6. doi: 10.1186/s40317-015-0092-91
Block, B. A., Jonsen, I. D., Jorgensen, S. J., Winship, A. J., Shaffer, S. A., Bograd, S. J., et al. (2011). Tracking apex marine predator movements in a dynamic ocean. Nature 475, 86–90. doi: 10.1038/nature10082
Brand, L. E., and Compton, A. (2007). Long-term increase in Karenia brevis abundance along the southwest Florida Coast. Harmful Algae 6, 232–252. doi: 10.1016/j.hal.2006.08.005
Caillouet, C. W. (2014). Interruption of the Kemp’s ridley population’s pre-2010 exponential growth in the Gulf of Mexico and its aftermath: one hypothesis. Mar. Turtle Newslett. 143, 1–7.
Calenge, C. (2006). The package “adehabitat” for the R software: a tool for the analysis of space and habitat use by animals. Ecol. Model. 197, 516–519. doi: 10.1016/j.ecolmodel.2006.03.017
Capper, A., Flewelling, L. J., and Arthur, K. (2013). Dietary exposure to harmful algal bloom (HAB) toxins in the endangered manatee (Trichechus manatus latirostris) and green sea turtle (Chelonia mydas) in Florida, USA. Harmful Algae 28, 1–9. doi: 10.1016/j.hal.2013.04.009
Carr, A. F. (1980). Some problems of sea turtle ecology. Am. Zool. 20, 489–498. doi: 10.1093/icb/20.3.489
Casale, P., Affronte, M., Insacco, G., Freggi, D., Vallini, C., Pino d’Astore, P., et al. (2010). Sea turtle strandings reveal high anthropogenic mortality in Italian waters. Aquat. Conserv. Mar. Freshw. Ecosyst. 20, 611–620. doi: 10.1002/aqc.1133
Costa, D. P., Breed, G. A., and Robinson, P. W. (2012). New insights into pelagic migrations: implications for ecology and conservation. Annu. Rev. Ecol.Evol. Syst. 43, 73–96. doi: 10.1146/annurev-ecolsys-102710-145045
Cox, T. M., Lewison, R. L., Žydelis, R., Crowder, L. B., Safina, C., and Read, A. J. (2007). Comparing effectiveness of experimental and implemented bycatch reduction measures: the ideal and the real. Conserv. Biol. 21, 1155–1164. doi: 10.1111/j.1523-1739.2007.00772.x
Dawson, T. M., Formia, A., Agamboué, P. D., Asseko, G. M., Boussamba, F., Cardiec, F., et al. (2017). Informing marine protected area designation and management for nesting olive ridley sea turtles using satellite tracking. Front. Mar. Sci. 4:312. doi: 10.3389/fmars.2017.00312
Fieberg, J., and Kochanny, C. O. (2005). Quantifying home-range overlap: the importance of the utilization distribution. J. Wildl. Manag. 69, 1346–1359. doi: 10.2193/0022-541x(2005)69[1346:qhotio]2.0.co;2
Finkbeiner, E. M., Wallace, B. P., Moore, J. E., Lewison, R. L., Crowder, L. B., and Read, A. J. (2011). Cumulative estimates of sea turtle bycatch and mortality in USA fisheries between 1990 and 2007. Biol. Conserv. 144, 2719–2727. doi: 10.1016/j.biocon.2011.07.033
Foley, A. M., Stacy, B. A., Schueller, P., Flewelling, L. J., Schroeder, B. A., Minch, K., et al. (2019). Assessing Karenia brevis red tide as a mortality factor of sea turtles in Florida. Dis. Aquat. Organ 132, 109–124. doi: 10.3354/dao03308
Fossette, S., Witt, M. J., Miller, P., Nalovic, M. A., Albareda, D., Almeida, A. P., et al. (2014). Pan-Atlantic analysis of the overlap of a highly migratory species, the leatherback turtle, with pelagic longline fisheries. Proc. R. Soc. B Biol. Sci. 281:20133065. doi: 10.1098/rspb.2013.3065
Frazer, T. K. (2020). Letter to the Secretary of Commerce from the Gulf of Mexico Fishery Management Council Regarding IUU Fishing Activities and Red Snapper Management in Gulf of Mexico. Tampa, FL: Gulf of Mexico Fishery Management Council.
Fury, C. A., Ruckstuhl, K. E., and Harrison, P. L. (2013). Spatial and social sexual segregation patterns in Indo-Pacific bottlenose dolphins (Tursiops aduncus). PLoS One 8:e52987. doi: 10.1371/journal.pone.0052987
Gallaway, B. J., Gazey, W. J., Caillouet, C. W. Jr., Plotkin, P. T., and Abreu Grobois, F. A. (2016a). Development of a Kemp’s ridley sea turtle stock assessment model. Gulf Mexico Sci. 33, 138–157. doi: 10.18785/goms.3302.03
Gallaway, B. J., Gazey, W. J., Wibbels, T., Bevan, E. M., Shaver, D. J., and George, J. (2016b). Evaluation of the status of the Kemp’s ridley sea turtle after the 2010 Deepwater Horizon oil spill. Gulf Mexico Sci. 2016, 192–205. doi: 10.18785/goms.3302.06
Godley, B. J., Blumenthal, J. M., Broderick, A. C., Coyne, M. S., Godfrey, M. H., Hawkes, L. A., et al. (2008). Satellite tracking of sea turtles: where have we been and where do we go next? Endangered Spec. Res. 4, 3–22. doi: 10.3354/esr00060
Hamann, M., Godfrey, M. H., Seminoff, J. A., Arthur, K., Carreras, C., Casale, P., et al. (2010). Global research priorities for sea turtles: informing management and conservation in the 21st century. Endangered Spec. Res. 11, 245–269. doi: 10.3354/esr00279
Hart, K. M., and Hyrenbach, K. D. (2009). Satellite telemetry of marine megavertebrates: the coming of age of an experimental science. Endangered Spec. Res. 10, 9–20. doi: 10.3354/esr00238
Hart, K. M., Iverson, A. R., Fujisaki, I., Lamont, M. M., Bucklin, D., and Shaver, D. J. (2018a). Marine threats overlap key foraging habitat for two imperiled sea turtle species in the Gulf of Mexico. Front. Mar. Sci. 5:336. doi: 10.3389/fmars.2018.00336
Hart, K. M., Iverson, A. R., Fujisaki, I., Lamont, M. M., Bucklin, D., and Shaver, D. J. (2018b). Sympatry or syntopy? Investigating drivers of distribution and co-occurrence for two imperiled sea turtle species in Gulf of Mexico neritic waters. Ecol. Evol. 8, 12656–12669. doi: 10.1002/ece3.4691
Hays, G. C., Fossette, S., Katselidis, K. A., Mariani, P., and Schofield, G. (2010). Ontogenetic development of migration: lagrangian drift trajectories suggest a new paradigm for sea turtles. J. R. Soc. Interface 7, 1319–1327. doi: 10.1098/rsif.2010.0009
Hays, G. C., and Hawkes, L. A. (2018). Satellite tracking sea turtles: opportunities and challenges to address key questions. Front. Mar. Sci. 5:432. doi: 10.3389/fmars.2018.00432
Hazel, J., and Gyuris, E. (2006). Vessel-related mortality of sea turtles in Queensland, Australia. Wildl. Res. 33, 149–154. doi: 10.1071/wr04097
Hazen, E. L., Maxwell, S. M., Bailey, H., Bograd, S. J., Hamann, M., Gaspar, P., et al. (2012). Ontogeny in marine tagging and tracking science: technologies and data gaps. Mar. Ecol. Prog. Ser. 457, 221–240. doi: 10.3354/meps09857
Holdo, R. M., and Roach, R. R. (2013). Inferring animal population distributions from individual tracking data: theoretical insights and potential pitfalls. J. Anim. Ecol. 82, 175–181. doi: 10.1111/j.1365-2656.2012.02031.x
Horton, T. W., Hauser, N., Zerbini, A. N., Francis, M. P., Domeier, M. L., Andriolo, A., et al. (2017). Route fidelity during marine megafauna migration. Front. Mar. Sci. 4:422. doi: 10.3389/fmars.2017.00422
Hughes, C. L., and Landry, A. M. (2016). Long-term movements of an adult male Kemp’s ridley sea turtle (Lepidochelys kempii) in the northwestern Gulf of Mexico. Gulf Mexico Sci. 33, 206–213. doi: 10.18785/goms.3302.07
Jeffers, V. F., and Godley, B. J. (2016). Satellite tracking in sea turtles: how do we find our way to the conservation dividends? Biol. Conserv. 199, 172–184. doi: 10.1016/j.biocon.2016.04.032
Jensen, M. P., Allen, C. D., Eguchi, T., Bell, I. P., LaCasella, E. L., Hilton, W. A., et al. (2018). Environmental warming and feminization of one of the largest sea turtle populations in the world. Curr. Biol. 28, 154–159. doi: 10.1016/j.cub.2017.11.057
Johnson, D. S. (2018). crawl: Fit continuous-time correlated random walk models to animal movement data. R package version 2.2.1.
Jonsen, I. D., Flemming, J. M., and Myers, R. A. (2005). Robust state-space modeling of animal movement data. Ecology 86, 2874–2880. doi: 10.1890/04-1852
Justić, D., Bierman, V. J., Scavia, D., and Hetland, R. D. (2007). Forecasting Gulf’s hypoxia: the next 50 years? Estua. Coasts 30, 791–801. doi: 10.1007/BF02841334
Kelly, B. P., Badajos, O. H., Kunnasranta, M., Moran, J. R., Martinez-Bakker, M., Wartzok, D., et al. (2010). Seasonal home ranges and fidelity to breeding sites among ringed seals. Polar Biol. 33, 1095–1109. doi: 10.1007/s00300-010-0796-x
Kernaléguen, L., Cherel, Y., Knox, T. C., Baylis, A. M. M., and Arnould, J. P. Y. (2015). Sexual niche segregation and gender-specific individual specialisation in a highly dimorphic marine mammal. PLoS One 10:e0133018. doi: 10.1371/journal.pone.0133018
Landsberg, J. H., Flewelling, L. J., and Naar, J. (2009). Karenia brevis red tides, brevetoxins in the food web, and impacts on natural resources: decadal advancements. Bot. Mar. 8, 598–607. doi: 10.1016/j.hal.2008.11.010
Lewison, R. L., Crowder, L. B., and Shaver, D. J. (2003). The impact of turtle excluder devices and fisheries closures on loggerhead and Kemp’s ridley strandings in the western Gulf of Mexico. Conserv. Biol. 17, 1089–1097. doi: 10.1046/j.1523-1739.2003.02057.x
Lightsey, J. D., Rommel, S. A., Costidis, A. M., and Pitchford, T. D. (2006). Methods used during gross necropsy to determine watercraft-related mortality in the Florida manatee (Trichechus manatus latirostris). J. Zool. Wildl. Med. 37, 262–275. doi: 10.1638/04-095.1
Lopez, R., and Malardé, J.-P. (2011). Improving ARGOS Doppler Location using Kalman Filtering. Ramonville Saint-Agne: CLS.
Márquez-Millán, R. (1994). Synopsis of Biological Data on the Kemp’s Ridley Turtle, Lepidochelys Kempi (Garman, 1880). Miami, FL: U.S. Department of Commerce, National Oceanic and Atmospheric Administration, National Marine Fisheries Service, Southeast Fisheries Science Center.
Márquez-Millán, R., Burchfield, P. M., Diaz, J., Sanchez, M., Carrasco, M., Jimenez, C., et al. (2005). Status of the Kemp’s ridley sea turtle, Lepidochelys kempii. Chelonian Conserv. Biol. 4, 761–766.
Marsh, H., O’Shea, T. J., and Reynolds, J. E. (2011). Ecology and Conservation of the Sirenia: Dugongs and Manatees. Cambridge: Cambridge University Press.
Maxwell, S. M., Hazen, E. L., Bograd, S. J., Halpern, B. S., Breed, G. A., Nickel, B., et al. (2013). Cumulative human impacts on marine predators. Nat. Commun. 4:2688. doi: 10.1038/ncomms3688
Maxwell, S. M., Scales, K. L., Bograd, S. J., Briscoe, D. K., Dewar, H., Hazen, E. L., et al. (2019). Seasonal spatial segregation in blue sharks (Prionace glauca) by sex and size class in the northeast Pacific Ocean. Divers. Distrib. 25, 1304–1317. doi: 10.1111/ddi.12941
McClintock, B. T., London, J. M., Cameron, M. F., and Boveng, P. L. (2015). Modelling animal movement using the Argos satellite telemetry location error ellipse. Methods Ecol. Evol. 6, 266–277. doi: 10.1111/2041-210X.12311
McClintock, B. T., and Michelot, T. (2018). momentuHMM: R package for generalized hidden Markov models of animal movement. Methods Ecol. Evol. 9, 1518–1530. doi: 10.1111/2041-210X.12995
Morales, J. M., Moorcroft, P. R., Matthiopoulos, J., Frair, J. L., Kie, J. G., Powell, R. A., et al. (2010). Building the bridge between animal movement and population dynamics. Philos. Trans. R. Soc. B Biol. Sci. 365, 2289–2301. doi: 10.1098/rstb.2010.0082
Moritz, C. (1994). Defining ‘Evolutionarily Significant Units’ for conservation. Trends Ecol. Evol. 9, 373–375. doi: 10.1016/0169-5347(94)90057-90054
NMFS, and USFWS (2015). Kemp’s Ridley Sea Turtle (Lepidochelys kempii) 5-Year Review: Summary and Evaluation. Silver Spring, MD: National Marine Fisheries Service Office of Protected Resources & U.S. Fish and Wildlife Service Southwest Region.
NMFS, USFWS, and SEMARNAT (2011). Bi-National Recovery Plan for the Kemp’s Ridley Sea Turtle (Lepidochelys kempii). Second revision. Silver Spring, MD: National Marine Fisheries Service.
Oksanen, S. M., Ahola, M. P., Lehtonen, E., and Kunnasranta, M. (2014). Using movement data of Baltic grey seals to examine foraging-site fidelity: implications for seal-fishery conflict mitigation. Mar. Ecol. Prog. Ser. 507, 297–308. doi: 10.3354/meps10846
Oliver, C., and Jacobs, N. A. (2019). Improving International Fisheries Management: 2019 Report to Congress. Silver Spring, MD: NOAA.
Patrício, A. R., Varela, M. R., Barbosa, C., Broderick, A. C., Catry, P., Hawkes, L. A., et al. (2019). Climate change resilience of a globally important sea turtle nesting population. Glob. Change Biol. 25, 522–535. doi: 10.1111/gcb.14520
Pikesley, S. K., Broderick, A. C., Cejudo, D., Coyne, M. S., Godfrey, M. H., Godley, B. J., et al. (2015). Modelling the niche for a marine vertebrate: a case study incorporating behavioural plasticity, proximate threats and climate change. Ecography 38, 803–812. doi: 10.1111/ecog.01245
Putman, N. F., Abreu-Grobois, F. A., Iturbe-Darkistade, I., Putman, E. M., Richards, P. M., and Verley, P. (2015a). Deepwater Horizon oil spill impacts on sea turtles could span the Atlantic. Biol. Lett. 11:20150596. doi: 10.1098/rsbl.2015.0596
Putman, N. F., Seney, E. E., Verley, P., Shaver, D. J., López-Castro, M. C., Cook, M., et al. (2020). Predicted distributions and abundances of the sea turtle ‘lost years’ in the western North Atlantic Ocean. Ecography 43, 506–517. doi: 10.1111/ecog.04929
Putman, N. F., Shay, T. J., and Lohmann, K. J. (2010). Is the geographic distribution of nesting in the Kemp’s ridley turtle shaped by the migratory needs of offspring? Integr. Comp. Biol. 50, 305–314. doi: 10.1093/icb/icq041
Putman, N. F., Verley, P., Endres, C. S., and Lohmann, K. J. (2015b). Magnetic navigation behavior and the oceanic ecology of young loggerhead sea turtles. J. Exp. Biol. 218, 1044–1050. doi: 10.1242/jeb.109975
Queiroz, N., Humphries, N. E., Couto, A., Vedor, M., da Costa, I., Sequeira, A. M. M., et al. (2019). Global spatial risk assessment of sharks under the footprint of fisheries. Nature 572, 461–466. doi: 10.1038/s41586-019-1444-1444
R Core Team (2019). R: A Language and Environment for Statistical Computing. Vienna: R Foundation for Statistical Computing.
Rees, A. L. F., Alfaro-Shigueto, J., Barata, P. C. R., Bjorndal, K. A., Bolten, A. B., Bourjea, J., et al. (2016). Are we working towards global research priorities for management and conservation of sea turtles? Endangered Spec. Res. 31, 337–382. doi: 10.3354/esr00801
Reich, K. J., López-Castro, M. C., Shaver, D. J., Iseton, C., Hart, K. M., Hooper, M. J., et al. (2017). δ13C and d15N in the endangered Kemp’s ridley sea turtle Lepidochelys kempii after the Deepwater Horizon oil spill. Endangered Spec. Res. 33, 281–289. doi: 10.3354/esr00819
Reisinger, R. R., Raymond, B., Hindell, M. A., Bester, M. N., Crawford, R. J. M., Davies, D., et al. (2018). Habitat modelling of tracking data from multiple marine predators identifies important areas in the southern Indian Ocean. Divers. Distrib. 24, 535–550. doi: 10.1111/ddi.12702
Rutz, C., and Hays, G. C. (2009). New frontiers in biologging science. Biol. Lett. 5, 289–292. doi: 10.1098/rsbl.2009.0089
Schmid, J. R., and Witzell, W. N. (1997). Age and growth of wild Kemp’s ridley turtles (Lepidochelys kempi): cumulative results of tagging studies in Florida. Chelonian Conserv. Biol. 2, 532–537.
Schofield, G., Dimadi, A., Fossette, S., Katselidis, K. A., Koutsoubas, D., Lilley, M. K. S., et al. (2013). Satellite tracking large numbers of individuals to infer population level dispersal and core areas for the protection of an endangered species. Divers. Distrib. 19, 834–844. doi: 10.1111/ddi.12077
Seney, E. E., and Landry, A. M. (2008). Movements of Kemp’s ridley sea turtles nesting on the upper Texas coast: implications for management. Endangered Spec. Res. 4, 73–84. doi: 10.3354/esr00077
Seney, E. E., and Landry, A. M. (2011). Movement patterns of immature and adult female Kemp’s ridley sea turtles in the northwestern Gulf of Mexico. Mar. Ecol. Prog. Ser. 440, 241–254. doi: 10.3354/meps09380
Shaver, D. J., and Caillouet, C. W. (2015). Reintroduction of Kemp’s Ridley (Lepidochelys kempii) sea turtle to Padre Island National Seashore, Texas and its connection to head-starting. Herpetol. Conserv. Biol. 10, 378–435.
Shaver, D. J., Hart, K. M., Fujisaki, I., Bucklin, D., Iverson, A. R., Rubio, C., et al. (2017). Inter-nesting movements and habitat-use of adult female Kemp’s ridley turtles in the Gulf of Mexico. PLoS One 12:e0174248. doi: 10.1371/journal.pone.0174248
Shaver, D. J., Hart, K. M., Fujisaki, I., Rubio, C., Sartain, A. R., Peña, J., et al. (2013). Foraging area fidelity for Kemp’s ridleys in the Gulf of Mexico. Ecol. Evol. 3, 2002–2012. doi: 10.1002/ece3.594
Shaver, D. J., Hart, K. M., Fujisaki, I., Rubio, C., Sartain-Iverson, A. R., Peña, J., et al. (2016a). Migratory corridors of adult female Kemp’s ridley turtles in the Gulf of Mexico. Biol. Conserv. 194, 158–167. doi: 10.1016/j.biocon.2015.12.014
Shaver, D. J., and Rubio, C. (2008). Post-nesting movement of wild and head-started Kemp’s ridley sea turtles Lepidochelys kempii in the Gulf of Mexico. Endangered Spec. Res. 4, 43–55. doi: 10.3354/esr00061
Shaver, D. J., Rubio, C., Walker, J. S., George, J., Amos, A. F., Reich, K. J., et al. (2016b). Kemp’s ridley sea turtle (Lepidochelys kempii) nesting on the Texas coast: geographic, temporal, and demographic trends through 2014. Gulf Mexico Sci. 33, 158–178. doi: 10.18785/goms.3302.04
Shaver, D. J., Schroeder, B. A., Byles, R. A., Burchfield, P. M., Peña, J., Márquez-Millán, R., et al. (2005). Movements and home ranges of adult male Kemp’s ridley sea turtles (Lepidochelys kempii) in the Gulf of Mexico investigated by satellite telemetry. Chelonian Conserv. Biol. 4, 817–827.
Shimada, T., Limpus, C. J., Hamann, M., Bell, I., Esteban, N., Groom, R., et al. (2019). Fidelity to foraging sites after long migrations. J. Anim. Ecol. 89, 1008–1016. doi: 10.1111/1365-2656.13157
USCG (2019). News Release: Coast Guard sets new record for illegal fishing vessel interdictions. Washington, DC: U.S. Coast Guard.
van der Hoop, J. M., Vanderlaan, A. S. M., and Taggart, C. T. (2012). Absolute probability estimates of lethal vessel strikes to North Atlantic right whales in Roseway Basin, Scotian Shelf. Ecol. Appl. 22, 2021–2033. doi: 10.1890/11-1841.1
Walker, J. S., Shaver, D. J., Stacy, B. A., Broadwater, M. H., and Wang, Z. (2018). Brevetoxin exposure in sea turtles in south Texas (USA) during Karenia brevis red tide. Dis. Aquat. Organ 127, 145–150. doi: 10.3354/dao03194
Wallace, B. P., DiMatteo, A. D., Hurley, B. J., Finkbeiner, E. M., Bolten, A. B., Chaloupka, M. Y., et al. (2010). Regional management units for marine turtles: a novel framework for prioritizing conservation and research across multiple scales. PLoS One 5:e15465. doi: 10.1371/journal.pone.0015465
Wibbels, T., and Bevan, E. M. (2016). A historical perspective of the biology and conservation of the Kemp’s ridley sea turtle. Gulf Mexico Sci. 33, 129–137. doi: 10.18785/goms.3302.02
Keywords: Gulf of Mexico, Lepidochelys kempii, conservation, distribution, state-space modeling
Citation: Gredzens C and Shaver DJ (2020) Satellite Tracking Can Inform Population-Level Dispersal to Foraging Grounds of Post-nesting Kemp’s Ridley Sea Turtles. Front. Mar. Sci. 7:559. doi: 10.3389/fmars.2020.00559
Received: 10 April 2020; Accepted: 17 June 2020;
Published: 09 July 2020.
Edited by:
Rob Harcourt, Macquarie University, AustraliaReviewed by:
Tiffany Marie Dawson, Old Dominion University, United StatesNuno Queiroz, University of Porto, Portugal
Copyright © 2020 Gredzens and Shaver. This is an open-access article distributed under the terms of the Creative Commons Attribution License (CC BY). The use, distribution or reproduction in other forums is permitted, provided the original author(s) and the copyright owner(s) are credited and that the original publication in this journal is cited, in accordance with accepted academic practice. No use, distribution or reproduction is permitted which does not comply with these terms.
*Correspondence: Donna J. Shaver, donna_shaver@nps.gov