- 1Instituto Oceanográfico, Universidade de São Paulo, São Paulo, Brazil
- 2Instituto Coral Vivo, Santa Cruz Cabrália, Brazil
- 3Centro de Biologia Marinha, Universidade de São Paulo, São Sebastião, Brazil
- 4Instituto de Biodiversidade e Sustentabilidade, Universidade Federal do Rio de Janeiro, Macaé, Brazil
- 5Programa de Pós-graduação em Biodiversidade e Biologia Evolutiva, Universidade Federal do Rio de Janeiro, Rio de Janeiro, Brazil
- 6Laboratório de Ecologia Marinha, Departamento de Oceanografia e Limnologia, Universidade Federal do Rio Grande do Norte, Natal, Brazil
Mass coral bleaching has increased in intensity and frequency and has severely impacted shallow tropical reefs worldwide. Although extensive investigation has been conducted on the resistance and resilience of coral reefs in the Indo-Pacific and Caribbean, the unique reefs of the South Atlantic remain largely unassessed. Here we compiled primary and literature data for reefs from three biogeographical regions: Indo-Pacific, Caribbean and South Atlantic and performed comparative analyses to investigate whether the latter may be more resistant to bleaching. Our findings show that South Atlantic corals display critical features that make them less susceptible to mass coral bleaching: (i) deeper bathymetric distribution, as species have a mean maximum depth of occurrence of 70 m; (ii) higher tolerance to turbidity, as nearly 60% of species are found in turbid conditions; (iii) higher tolerance to nutrient enrichment, as nitrate concentration in the South Atlantic is naturally elevated; (iv) higher morphological resistance, as massive growth forms are dominant and comprise two thirds of species; and (v) more flexible symbiotic associations, as 75% of corals and 60% of symbiont phylotypes are generalists. Such features were associated with occurrence of fewer bleaching episodes with coral mortality in the South Atlantic, approximately 60% less than the Indo-Pacific and 50% less than the Caribbean. In addition, no mass coral mortality episodes associated with the three global mass bleaching events have been reported for the South Atlantic, which suffered considerably less bleaching. These results show that South Atlantic reefs display several remarkable features for withstanding thermal stress. Together with a historic experience of lower heat stress, our findings may explain why climate change impacts in this region have been less intense. Given the large extension and latitudinal distribution of South Atlantic coral reefs and communities, the region may be recognized as a major refugium and likely to resist climate change impacts more effectively than Indo-Pacific and Caribbean reefs.
Introduction
Coral reefs are diverse marine ecosystems that harbor approximately 25% of marine eukaryotic and prokaryotic species in less than 1% of the ocean surface area (Spalding et al., 2001; Knowlton et al., 2010; Sheppard et al., 2018). However, coral reefs have suffered significantly from anthropogenic impacts such as global warming, ocean acidification, pollution and overfishing (Hoegh-Guldberg et al., 2007; Bellwood et al., 2012; Wenger et al., 2015; Hughes et al., 2018a). Marine heat waves in combination with background warming and consequential elevated sea surface temperatures are responsible for the bleaching phenomenon, in which the key symbiotic relationship between reef-building corals and photosynthetic Symbiodiniaceae dinoflagellates is disrupted (Glynn, 1993, 1996; Fitt et al., 2001). Thermal stress induces the dinoflagellates to produce reactive oxygen species, which leads the coral host to expel them and leave its white calcareous skeleton visible under a thin and transparent tissue layer (Glynn, 1993; Lesser, 1996, 2006). Because the symbiotic relationship is obligate for most shallow-water reef-building corals, bleaching frequently leads to coral mortality (Glynn, 1993; Brown, 1997; Hoegh-Guldberg, 1999).
Recurrent mass bleaching events have contributed to severe reduction in coral cover in both the Indo-Pacific and Caribbean regions (Sheppard, 2003; Eakin et al., 2010; De’ath et al., 2012; DeCarlo et al., 2017; Hughes et al., 2017, 2018b). Loss of coral cover is often followed by decline in carbonate budgets and habitat complexity, which in turn compromises overall reef biodiversity (Pratchett et al., 2011; Descombes et al., 2015; Perry and Morgan, 2017). Mass bleaching also dramatically affects several economic activities and ecosystem services that are associated with coral reefs, such as fisheries and tourism, on which millions of people depend (Moberg and Folke, 1999; Cesar et al., 2003; Graham, 2014). Furthermore, the loss of structural complexity reduces the ability of shallow reefs to absorb wave energy and protect coastal communities from storms and erosion (Sheppard et al., 2005).
Although the impacts of mass bleaching and climate change on reef-building fauna have been well documented in several areas of the Indo-Pacific and Caribbean provinces, coral reefs in the South Atlantic remain little investigated on this regard. South Atlantic reefs are separated from the Caribbean by the Amazon-Orinoco plume and are mostly restricted to the Brazilian coast in the Western Atlantic due to upwelling preventing reef development on the African side (Le Læuff and von Cosel, 1998; Leão et al., 2003, 2016). The consequence is a unique system (the “Brazilian Province,” sensu Castro and Pires, 2001; Floeter et al., 2008) with low diversity and high proportion of endemic reef-building corals distributed across approximately 3,000 km from equatorial to subtropical latitudes (Castro and Pires, 2001; Leão et al., 2003, 2016; Pereira-Filho et al., 2019). South Atlantic reefs are also distinctive because they are mostly coastal as atolls and oceanic archipelagos are very few (Leão et al., 2016). In addition, they encompass extensive mesophotic reefs (Meirelles et al., 2015; Francini-Filho et al., 2019; Soares et al., 2019) the largest rhodolith beds in the world (Pereira-Filho et al., 2011; Amado-Filho et al., 2012; Moura et al., 2016) and are significantly influenced by river discharge (Leão et al., 2003, 2016; Moura et al., 2016). Therefore, South Atlantic reefs display several features that are unique to them and not typically found in the more studied reef habitats of the Indo-Pacific and Caribbean.
To assess whether South Atlantic reefs are less susceptible to bleaching than those in the Indo-Pacific and Caribbean, we compiled data for the three regions and performed a comparative analysis to address six hypotheses: (i) South Atlantic coral species have a deeper bathymetric distribution; (ii) South Atlantic coral species occur more frequently in turbid waters; (iii) South Atlantic reefs occur in higher nutrient conditions; (iv) there is a greater proportion of coral species displaying resistant growth forms in the South Atlantic; (v) there is a greater proportion of generalist coral species and symbiont phylotypes in the South Atlantic; and (vi) South Atlantic reefs have fewer bleaching episodes with coral mortality. These hypotheses investigate if the following characteristics associated with thermal stress resistance are typical of South Atlantic coral populations: possibility of refuge in deeper and cooler conditions (Glynn, 1996); possibility of refuge in turbid areas that attenuate high irradiance and temperature (van Woesik et al., 2012); tolerance to local stressors that enhance bleaching effects, such as nutrient enrichment (Kuta and Richardson, 2002); robust morphology (Loya et al., 2001); and flexible symbiotic associations that may increase adaptability to environmental disturbances (Baker, 2003). Together, these topics allow for a comprehensive evaluation of whether South Atlantic corals are less susceptible to bleaching and if reefs in this area serve as climate change refugia. Addressing these topics for the South Atlantic may also bring awareness to one of the largest coral reef communities in the world, which is still relatively little investigated and neglected in most global studies.
Materials and Methods
To address the six hypotheses, data were compiled for four specific coral traits (lower bathymetric distribution limit, occurrence in turbid waters, typical growth morphology and diversity of symbiont associations), as well as for reef nutrient concentration (inferred using nitrate) and the frequency of coral mortality associated with bleaching episodes. Data were compiled for all three regions, Indo-Pacific, Caribbean and South Atlantic, which were considered in their entirety and by including both coastal and oceanic reefs. Only zooxanthellate scleractinian and hydrocoral species were considered. The three regions (i.e., biogeographical provinces) were delimited based on their distinctive phylogeographic characteristics and following a widely used classification (LaJeunesse, 2005; Floeter et al., 2008; Roff and Mumby, 2012).
Lower Bathymetric Limit, Occurrence in Turbid Waters and Growth Morphology
To assess the deepest occurrence record for Indo-Pacific, Caribbean and South Atlantic corals, whether they are found in turbid environments, and their primary growth morphology, coral trait data were compiled for 850 species from Veron (2000), Ruiz-Ramos et al. (2014), Madin et al. (2016), Veron et al. (2016). Information for South Atlantic species is often less available in some of these databases, therefore additional data were retrieved from Leão et al. (2003, 2010, 2016), Castro et al. (2006), Amaral et al. (2008), Souza et al. (2017). Coral morphology data were divided into four comprehensive categories: branching (including branching, corymbose, digitate and tabular growth forms), massive (massive, submassive, hemispherical, dome-shaped and nodule), plate (plate, laminar, and foliose), and others (encrusting, phacelloid, meandroid, and solitary).
Nutrient Conditions
Nitrate (NO3–) is widely used as a proxy for nutrient enrichment in marine environments (Kennedy et al., 2013). To assess whether South Atlantic reefs are found in higher nutrient conditions, we compiled nitrate (NO3–) data available from scientific publications for 15 reefs in each region (References 1–37 in Supplementary Reference List S1). For three reef sites in the South Atlantic (Araripe, Porto Seguro, and Recife de Fora), data obtained in situ were provided by the Coral Vivo Institute. In cases where nitrate concentration was given as an interval, the median value between the minimum and maximum values was considered.
Symbiotic Associations
A symbiotic organism is considered as generalist when found in association with a high diversity of partner phylotypes, whereas specialists associate with a restrictive pool of partners (Baker, 2003). To investigate if corals and Symbiodiniaceae dinoflagellates in Indo-Pacific, Caribbean and South Atlantic reefs are either generalists or specialists, we compiled the symbiont diversity for 339 scleractinian and hydrocoral species that have undergone assessment (references 38–78 in Supplementary Reference List S1 for Indo-Pacific corals; 41, 47, 48, 50, 54, 57, 79–95 for Caribbean; and 96–102 for South Atlantic). Additional primary data on South Atlantic coral symbiont diversity were provided by the Laboratório de Biodiversidade de Cnidaria at the Universidade Federal do Rio de Janeiro (authors CZ and AG). The diversity of Symbiodiniaceae dinoflagellates was considered at the Internal Transcribed Spacer 2 (ITS2) level, which is a non-coding region in the ribosomal operon that is widely used as a marker for identification and diversity assessments (LaJeunesse, 2001; Arif et al., 2014; Hume et al., 2018). For our analyses, symbiont phylotypes were considered generalists when associated with more than one coral genus. Coral species were considered generalists when associated with more than one symbiont clade, given that Symbiodiniaceae clades have recently been assigned generic status (LaJeunesse et al., 2018). Corals and symbionts associated with a single symbiont clade or host genus were considered specialists.
Mortality Associated With Bleaching Episodes
To evaluate if fewer bleaching episodes associated with coral mortality have been observed in the South Atlantic than in the Indo-Pacific and Caribbean, we compiled 775 independent bleaching records, spanning 1980 to 2018, from scientific articles available in PubMed, Scopus, Web of Knowledge and Google Scholar, and the Bleaching Database (Donner et al., 2017). Based on the information reported, bleaching records were divided into two groups, with or without associated mortality. The proportion of bleaching episodes associated with coral mortality was then calculated for each of the three regions.
Statistical Analyses
To investigate if South Atlantic species have a deeper bathymetric distribution limit, a Kruskal-Wallis test was performed followed by a Steel-Dwass post-hoc test. For differences in nitrate content, a one-way analysis of variance (ANOVA) was performed, followed by Tukey’s HSD post-hoc test. One-tailed Fisher-Irwin tests were performed for proportion data (%) to assess if the South Atlantic has (a) more coral species found in turbid conditions, (b) more massive growth species, (c) proportionally fewer bleaching episodes with mortality, and a higher proportion of (d) generalist corals, and (e) generalist symbionts than the Indo-Pacific and Caribbean. Differences were considered significant at p < 0.05 for all tests.
Results
The results for all statistical tests comparing differences in traits associated with resistance to bleaching between Indo-Pacific, Caribbean and South Atlantic coral reefs are summarized in Table 1.
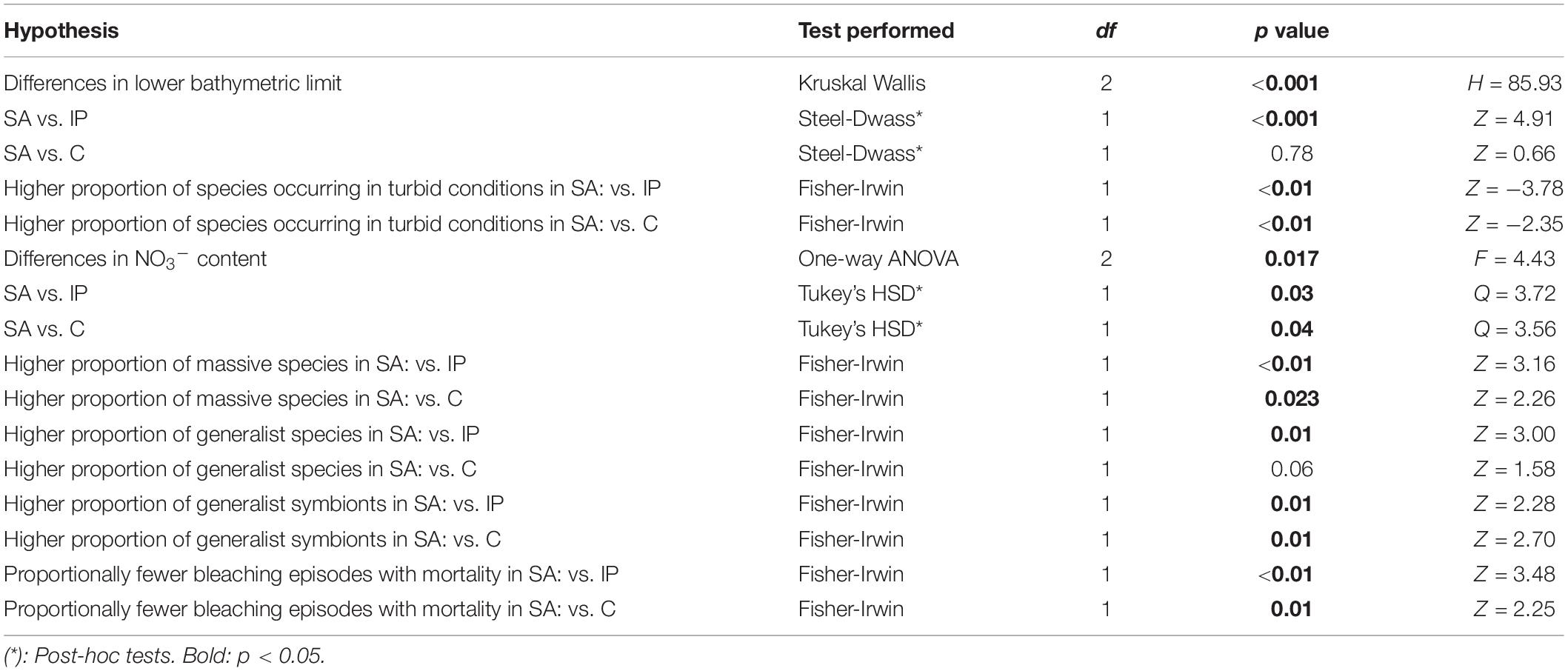
Table 1. Results for statistical tests investigating differences in six different aspects associated with thermal tolerance between South Atlantic (SA), Indo-Pacific (IP), and Caribbean (C) coral reefs.
Lower Bathymetric Limit
Analysis of the lower bathymetric limits shows that there are differences between Indo-Pacific, Caribbean and South Atlantic coral species (p < 0.001). Post-hoc tests demonstrate that corals in the South Atlantic and Caribbean have lower limits than in the Indo-Pacific (p < 0.001 for both cases). Depth limit for Indo-Pacific, Caribbean and South Atlantic species is 30.6 ± 0.7 (mean ± standard error), 59.5 ± 3.9 and 70.1 ± 9.0 m, respectively (Figure 1). Several species shared between the Caribbean and South Atlantic have been reported deeper than 100 m, such as Agaricia fragilis, Montastraea cavernosa, Madracis decactis, Meandrina brasiliensis, and Scolymia wellsii (Supplementary Table S1).
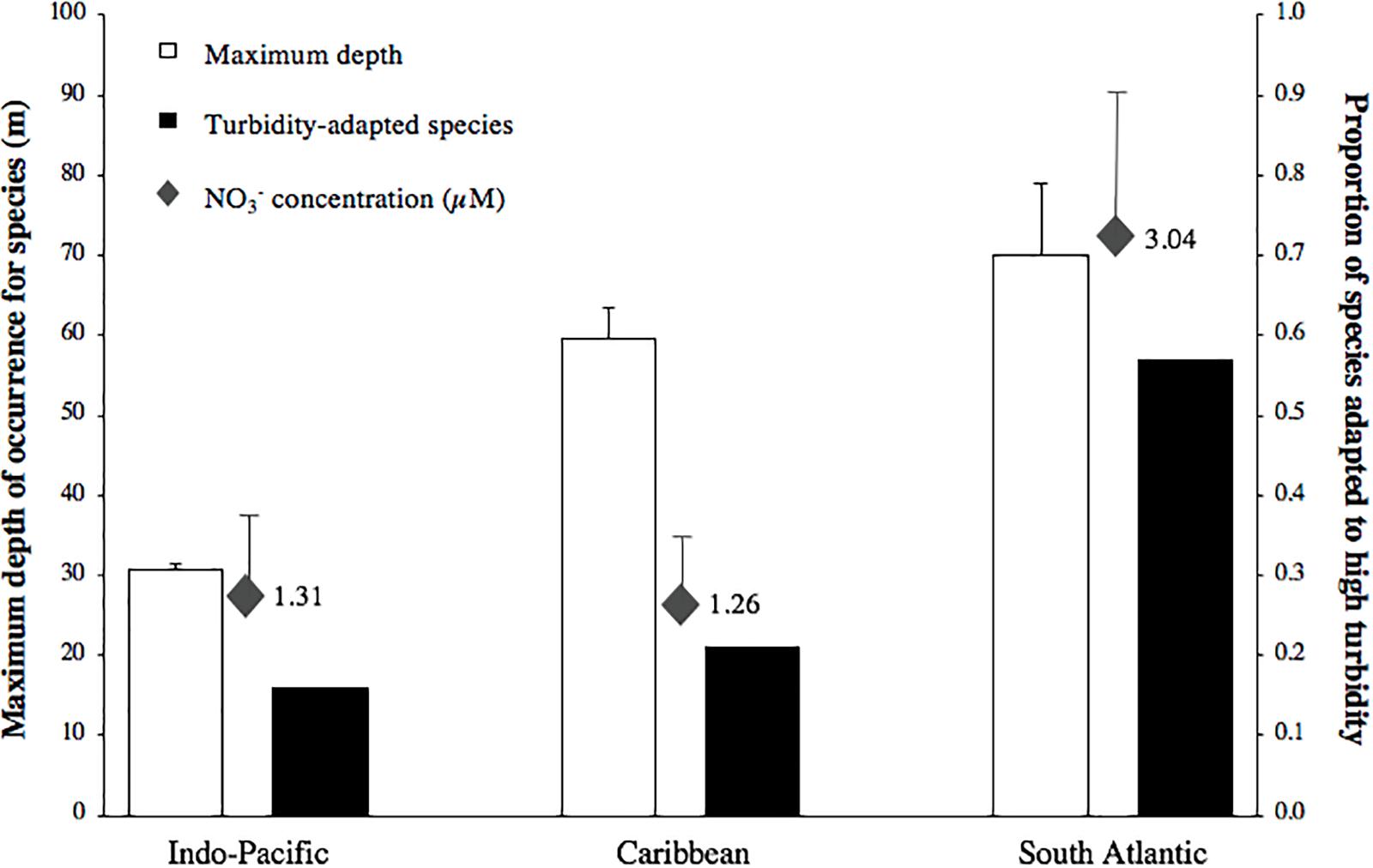
Figure 1. Mean maximum depth reported for zooxanthellate scleractinian and hydrocoral species, proportion of species that are tolerant to turbid waters and NO3– (a proxy for nutrient enrichment) concentration in Indo-Pacific, Caribbean and South Atlantic coral reefs. Bars denote standard error.
Turbidity Tolerance
South Atlantic reefs harbor a higher proportion of species that occur in turbid waters than both the Indo-Pacific (p < 0.01) and Caribbean (p < 0.01). The proportion of species that occur in turbid waters is 16, 21, and 57% for the Indo-Pacific, Caribbean and South Atlantic, respectively (Figure 1). Many South Atlantic species that are tolerant to high turbidity also display a deeper bathymetric limit, such as the cases of Mussismilia hispida, Scolymia wellsii, Siderastrea stellata, Montastraea cavernosa, and Stephanocoenia michelinii (Supplementary Table S1).
Nutrient Conditions
Nitrate content was different among Indo-Pacific, Caribbean and South Atlantic reefs (p = 0.017), with South Atlantic reefs presenting a higher nitrate content than both the Indo-Pacific (p = 0.03) and Caribbean (p = 0.04) (Figure 1). Several locations in the South Atlantic display remarkably high nitrate concentrations, occasionally above 5.0 μM (Supplementary Table S2).
Coral Growth Morphology
A higher proportion of species displaying massive growth forms are found in the South Atlantic than in both the Indo-Pacific (p < 0.01) and Caribbean (p = 0.023). Massive species comprise the majority of reef-building corals in the South Atlantic, but not in the Indo-Pacific and Caribbean (Figure 2). While South Atlantic reefs have the highest proportion of massive species, the Indo-Pacific has the lowest. For branching corals, the exact opposite pattern is observed, i.e., highest proportion in the Indo-Pacific and lowest proportion in the South Atlantic. Only three branching species occur in the South Atlantic, the milleporid hydrocorals Millepora alcicornis, M. braziliensis, and M. nitida (Supplementary Table S1).
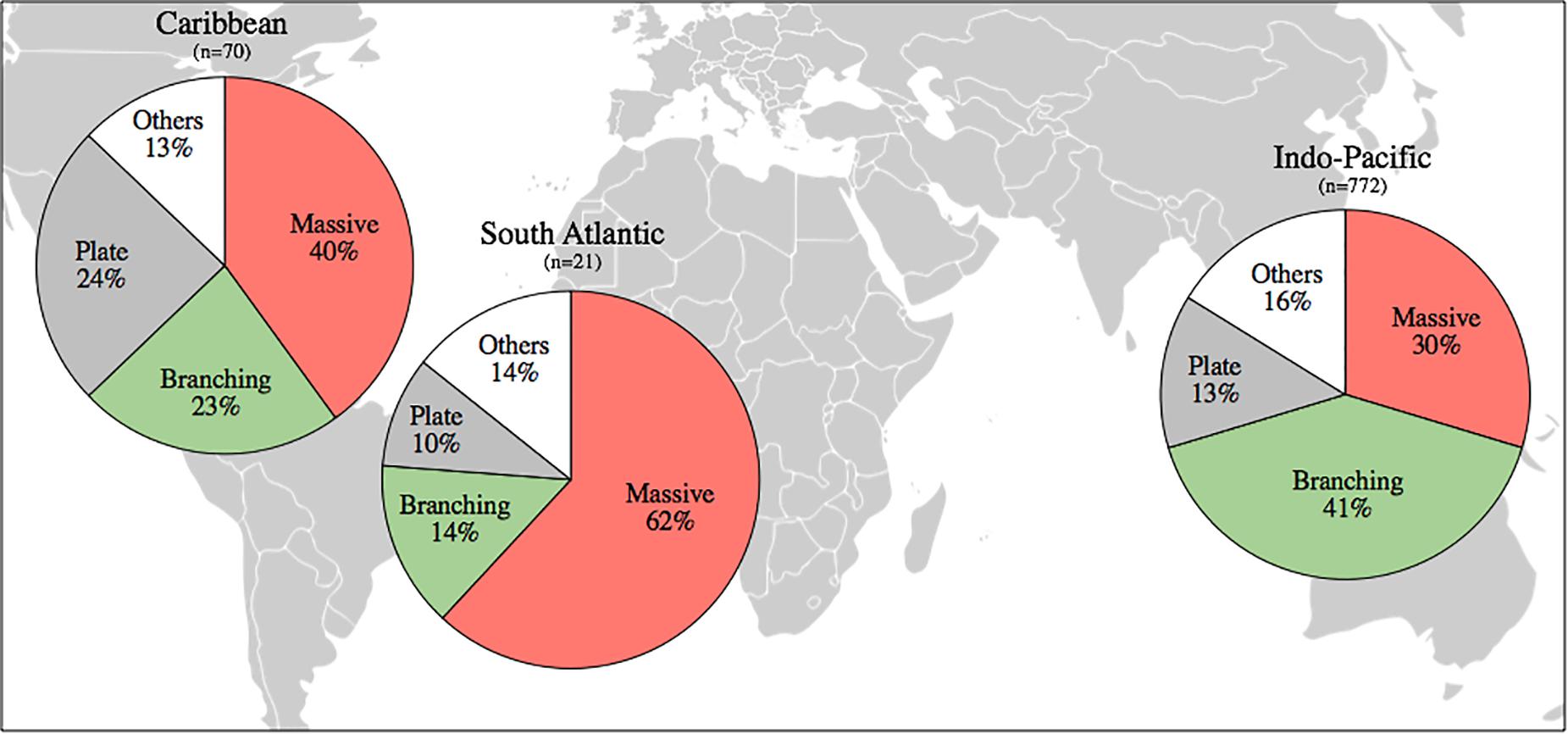
Figure 2. Composition of the main growth forms displayed by corals in Indo-Pacific, Caribbean and South Atlantic reefs. Primary growth morphology data were collected for 850 zooxanthellate scleractinian and hydrocoral species and were divided into four main categories: branching (branching, corymbose, digitate and tabular forms), massive (massive, submassive, hemispherical, dome-shaped and nodule), plate (plate, laminar and foliose) and others (encrusting, phacelloid, meandroid and solitary).
Symbiotic Associations
South Atlantic reefs have a higher proportion of generalist coral species than the Indo-Pacific (p = 0.01 – see Table 1). However, no statistical differences were found between the South Atlantic and Caribbean. From the symbiont perspective, South Atlantic reefs have a higher proportion of generalist Symbiodiniaceae phylotypes than both the Indo-Pacific (p = 0.01) and Caribbean (p = 0.01). For species that have been evaluated for symbiont diversity, three quarters of coral species and approximately 60% of Symbiodiniaceae phylotypes in the South Atlantic are generalists (Figure 3a and Supplementary Table S3). Among the South Atlantic coral species that host the highest diversity of symbionts are Mussismilia hispida, Mussismilia harttii, Favia gravida, Millepora alcicornis, and Siderastrea spp., all of which host at least three different Symbiodiniaceae genera (Supplementary Table S3). Amongst the generalist symbiont phylotypes in the South Atlantic are A4 (Symbiodinium linucheae), B1 (Breviolum minutum), B19, B30, C1 (Cladocopium goreaui), and C3, which associate with several distinct coral genera (Supplementary Table S4).
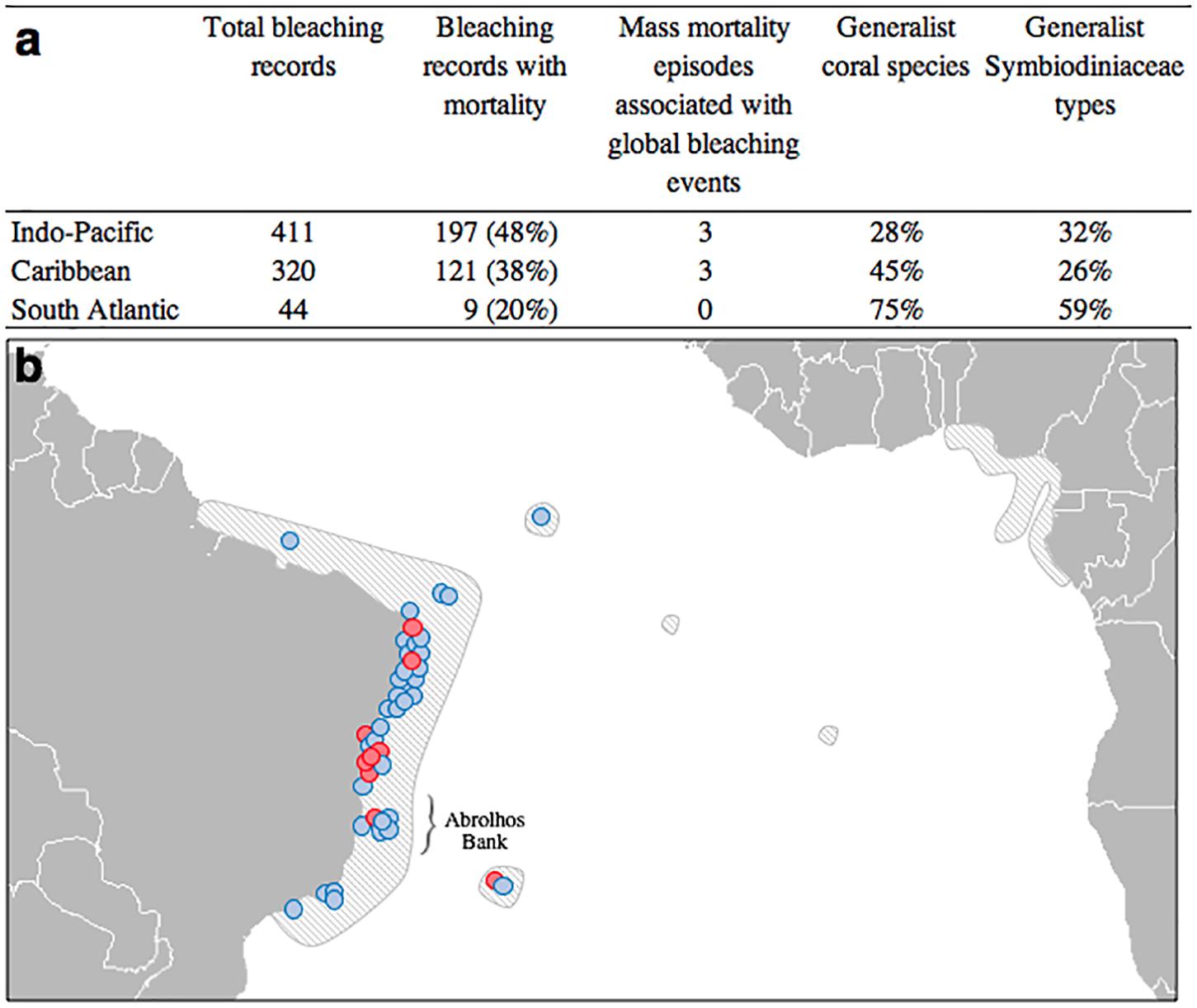
Figure 3. Severity of bleaching episodes in Indo-Pacific, Caribbean and South Atlantic reefs: (a) Bleaching-associated mortality records, proportion of generalist reef-building coral species and proportion of generalist symbiont phylotypes in Indo-Pacific, Caribbean and South Atlantic coral reefs. (b) Geographical distribution of all documented South Atlantic coral bleaching episodes as of 2018. Red and blue circles indicate records with and without mortality, respectively. Distribution of coral reefs and large coral communities is within the shaded areas. South Atlantic bleaching episodes references: 23, 103–148 in Supplementary Material Reference List S1.
Mortality Associated With Bleaching Episodes
South Atlantic reefs have experienced proportionally fewer bleaching episodes with coral mortality than both the Indo-Pacific (p < 0.01) and Caribbean (p = 0.01). Most South Atlantic bleaching episodes occurred in Northeastern Brazil, particularly in the coast of Bahia State (Figure 3b). No mortality was observed in 80% of bleaching episodes in the South Atlantic (Figure 3a). Additionally, no mass mortality episodes (>20% at the community level) were observed in the South Atlantic so far, including the three global mass bleaching events (Leão et al., 2016; Teixeira et al., 2019).
Discussion
The analyses performed in this study confirmed six hypotheses that together argue that South Atlantic reefs are less susceptible to mass coral bleaching episodes. Below, we discuss in detail each of the six lines of investigation performed comparatively between Indo-Pacific, Caribbean and South Atlantic reefs.
Lower Bathymetric Limit
Deep and mesophotic reefs have been widely hypothesized as refugia for reef-building corals against elevated temperatures (Glynn, 1996; Riegl and Piller, 2003; Bongaerts et al., 2010; Smith et al., 2014; Semmler et al., 2017; Baird et al., 2018). These reefs typically undergo less bleaching, although exceptions have been documented (Eakin et al., 2019). Deeper habitats are cooler and less susceptible to rapid temperature variations because of a lower incidence of solar radiation and light penetration (Glynn, 1996). As a consequence, most coral bleaching episodes worldwide occur in depths shallower than 20 m (Donner et al., 2017).
Our analysis on coral bathymetric distribution shows that South Atlantic and Caribbean coral species extend into deeper waters than those in the Indo-Pacific (Figure 1). This does not necessarily mean that South Atlantic reefs are deeper, but that most coral species are tolerant to the relatively low light and temperature conditions found at greater depths. The refugium potential for a geographic region may largely depend on species and context (Bongaerts et al., 2010; Smith et al., 2016) but populations of depth-generalist species tend to benefit more and become established more successfully in deeper areas (Holstein et al., 2016). In the South Atlantic, most dominant reef-building species (e.g. Mussismilia spp., Siderastrea spp., Montastraea cavernosa) are depth-generalists found in both shallow (<5 m) and deep (>30 m) environments (Leão et al., 2003, 2010, 2016; Francini-Filho et al. (2019) Supplementary Table S1). In addition, many South Atlantic species thrive in conditions of significant light attenuation (Freitas et al., 2019). Therefore, many South Atlantic coral species are already adapted to and occur in depth zones that may shelter them from bleaching caused by global warming impacts.
Turbidity Tolerance
Corals adapted to lower light levels are also likely to tolerate turbid waters. Turbid reef environments may also serve as refugia from climate change impacts, as the suspended particles protect corals from high irradiance and reduce the intensity of thermal stress (Iglesias-Prieto and Trench, 1994; van Woesik et al., 2012; Cacciapaglia and van Woesik, 2016; Sully and van Woesik, 2020). During the third global mass bleaching event, turbid sections of the Great Barrier Reef showed remarkable tolerance and underwent little or no bleaching (Morgan et al., 2017). South Atlantic reefs are naturally turbid and associated with high sediment loads, mostly because of resuspension of fine sediments and terrigenous discharges from large rivers such as the Amazon, São Francisco, Doce and their many affluent streams (Dutra et al., 2006; Castro et al., 2012; Silva et al., 2013; Omachi et al., 2019). For instance, the Abrolhos reefs, which are the largest coral reefs in the South Atlantic, are associated with a high content of siliciclastic sand and mud, which is atypical for Caribbean and Indo-Pacific reefs (Leão and Kikuchi, 2001).
Our analysis shows that more than half of South Atlantic coral species are tolerant to turbid environments, which is more than twice than the proportion recorded for the Caribbean and more than three times higher than that for the Indo-Pacific (Figure 1). An emblematic example is Mussismilia braziliensis, an endemic species which is one of the main South Atlantic reef-builders, that displays higher growth rate and reproduction effort when found in more turbid conditions (Pires et al., 2011; Ribeiro et al., 2018). In addition, turbid inshore reefs in the South Atlantic often display higher coral cover and abundance than less turbid offshore reefs, particularly Montastraea cavernosa (Francini-Filho et al., 2013; Loiola et al., 2019). Therefore, unlike for the Indo-Pacific and Caribbean, most South Atlantic coral species have already adapted for thriving in turbid environments that may prove critical for surviving the increasing impacts of climate change and mass bleaching episodes.
Nutrient Conditions
Along with sediment, river run-off also releases considerable nutrient quantities. Nutrification often works in tandem with climate change and typically triggers negative impacts on coral reefs such as reduction in bleaching temperature threshold and disease outbreaks (Kuta and Richardson, 2002; Schlöder and D’Croz, 2004; Wiedenmann et al., 2013). However, local environmental stressors such as high nutrient concentration may also favor and select coral species or genotypes that are stress-tolerant, leading to more resistant species and populations over evolutionary time scales (Côté and Darling, 2010).
Our findings show that the mean nitrate concentration in South Atlantic reefs is approximately 2.5 times greater in comparison to those in the Indo-Pacific and Caribbean (Figure 1). South Atlantic coral reefs are typically found and develop under higher nitrogen, phosphorus and chlorophyll-a content (Leipe et al., 1999; Costa et al., 2002, 2006). In fact, in several South Atlantic reef areas, the soluble reactive phosphorus content is among the highest in all of the world’s reefs (Costa et al., 2008). While urban pollution has been hypothesized as a driver for disease outbreaks in the South Atlantic (Francini-Filho et al., 2008) there have been no reports of disease or mortality associated with the naturally higher nutrient concentration. In addition, South Atlantic corals seem to benefit from high nutrient levels by increasing their abundance and reproductive output (Pires et al., 2011). Therefore, South Atlantic corals appear to be more tolerant to nutrification. Furthermore, South Atlantic reefs are also farther from nutrient depletion. The increase in sea surface temperature is likely to produce intense stratification in the next decades and limit nutrient availability in coral reefs (Moore et al., 2013). Because nutrient depletion (<0.05 μM of both nitrate and phosphate) is associated with decrease in photosynthetic activity and enhanced bleaching effects (Ezzat et al., 2019). South Atlantic reefs are also less vulnerable to impacts connected to nutrient starvation.
Coral Growth Morphology
Corals may also display morphological adaptations that reduce the impacts of high temperature and irradiance. Mostly because of their skeletal architecture, thicker tissues and high metabolic rates, massive corals are more resistant to thermal stress and other impacts than branching ones (Gates and Edmunds, 1999; Loya et al., 2001; Schlöder and D’Croz, 2004). Their higher content of soluble proteins and photosynthetically-fixed carbon inside tissues are also key factors in their adaptation to exacerbated environmental disturbances (Gates and Edmunds, 1999).
Our findings show that massive growth forms comprise the majority of reef-building species in the South Atlantic, unlike the Indo-Pacific and Caribbean (Figure 2). Almost two thirds of South Atlantic coral species are massive, with reefs largely formed by genera such as Siderastrea, Montastraea and Mussismilia. The latter is the most widespread in the South Atlantic, and an endemic relict genus dating back to the Tertiary (Leão et al., 2016). Mussismilia spp. have been widely reported as stress-tolerant organisms (Leão et al., 2003; Loiola et al., 2013; Mies et al., 2018). Among branching corals, only three species occur in South Atlantic reefs, while several species within the Millepora, Acropora and Porites genera are found in the Caribbean. In the Indo-Pacific, branching acroporids and pocilloporids account for most of the coral cover (Clark et al., 2017) and less than one third of species in that region is massive. The higher proportion of massive and the lower number of branching species compared to other regions is another factor suggesting that South Atlantic reefs are relatively more tolerant to thermal stress.
Flexibility of Symbiotic Associations
Another critical component in the coral response to thermal stress is the photosynthetic symbiont community that resides within its tissue. Symbiont identity itself is a major predictor of thermal tolerance (Berkelmans and van Oppen, 2006; Hume et al., 2015; Swain et al., 2017) but the flexibility of the symbiotic relationship is also a key factor. The capacity of corals to establish a relationship with a wide symbiont diversity is an important mechanism for rapid acclimation and adaptation to environmental disturbances (Baker, 2003; Baker et al., 2004; Little et al., 2004; Baird et al., 2007).
Our analysis shows that South Atlantic and Caribbean reefs have proportionally more generalist coral species than the Indo-Pacific (Figure 3a). South Atlantic reefs also have more generalist symbiont phylotypes than both the Indo-Pacific and Caribbean. So far, only Symbiodiniaceae genera Symbiodinium, Breviolum, Cladocopium, Fugacium, and Gerakladium (formerly clades A, B, C, F, and G, respectively – see LaJeunesse et al., 2018) have been reported for the South Atlantic (Silva-Lima et al., 2015; Picciani et al., 2016; Teschima et al., 2019; Supplementary Table S4), but a remarkable 60% of local phylotypes are generalists. In addition, the most abundant phylotypes occur in a wide range of environmental conditions along the Southwestern Atlantic coast (Silva-Lima et al., 2015) reinforcing their physiological plasticity. Generalist phylotypes such as A4 (Symbiodinium linucheae), C1 (Cladocopium goreaui), and C3 are commonly found in South Atlantic locations that undergo drastic changes in temperature and other abiotic factors (Teschima et al., 2019). Generalist phylotypes also predominate in turbid Caribbean and Indo-Pacific reefs, especially Durusdinium trenchii (D1a) (LaJeunesse et al., 2014; Pettay et al., 2015). This species has recently invaded Caribbean reefs and are distributed in several reefs that underwent disturbances (Pettay et al., 2015; Chen et al., 2020). However, the Durusdinium genus has not yet been recorded for the South Atlantic and other generalist phylotypes within multiple genera dominate. Therefore, South Atlantic corals are thus not only typically generalists, but also tend to associate with generalist symbionts. This symbiotic flexibility allows for adaptive shifts in the symbiont community, which can be critical in increasing resistance and resilience to bleaching (Baker et al., 2004). Still, symbiont diversity data are missing for several species and this is imperative for further understanding the response of South Atlantic corals to climate change and other disturbances.
Mortality Associated With Bleaching Episodes
The five traits addressed above are likely major contributing factors for South Atlantic reef corals to suffer fewer bleaching episodes with associated mortality than those in the Indo-Pacific and Caribbean. Until 2018, there were only 44 independent bleaching records for the entire South Atlantic (Figures 3a,b), most of them from the 1990’s onward, when more systematic monitoring programs began by dozens of independent Brazilian research groups. Only 20% of those records reported mortality, which is approximately 60 and 50% lower than the number of coral mortality reports due to bleaching for the Indo-Pacific and Caribbean, respectively (Figure 3a). Mortality reports in the SA are in most cases associated with hydrocoral species (Millepora spp.), which are typically branching and inhabit very shallow depths (Amaral et al., 2006; Santos et al., 2016; Teixeira et al., 2019). Only a single mass coral mortality episode has been recorded for the South Atlantic reefs (also mostly associated with Millepora spp., despite occurring in turbid conditions Duarte et al., 2020) and none of the three major global mass bleaching events that affected the Indo-Pacific and Caribbean had comparable effects in the South Atlantic (Kelmo et al., 2003; Teixeira et al., 2019). Further evidence for the resilience of South Atlantic reefs is that a heat stress of 7.7°C-weeks (degree heating weeks, which accounts for the accumulation of temperature anomalies exceeding the monthly maximum mean Liu et al., 2006; Kayanne, 2017) caused 20% coral mortality in the Caribbean (Florida Keys) and 13°C-weeks caused 80% mortality in Indo-Pacific (Australia) reefs, however, a remarkable value of 20°C-weeks, ranking among the highest ever recorded, resulted in less than 2% mortality in the marginal reefs of the Southwestern Atlantic (Gilmour et al., 2013; Gintert et al., 2018; Banha et al., 2019). And even more recently, in late 2019, 19.6°C-weeks recorded for the diverse Abrolhos reefs caused no significant mortality for any reef-building species with the exception of the branching and fast-growing hydrocoral Millepora alcicornis and, to a lesser extent, the phacelloid Mussismilia harttii, further highlighting the resilience of South Atlantic reefs to heat stress (Duarte et al., 2020). Two additional advantages are that the South Atlantic is historically and currently less exposed to heat waves (Skirving et al., 2019) and that the largest reefs in the South Atlantic are found in the Abrolhos Bank, which is systematically influenced by cooler-water eddies that reduce thermal stress (Ghisolfi et al., 2015). Therefore, it is likely that the relatively smaller impacts of bleaching are a consequence of both the resistance and resilience of local coral species and the lower intensity and frequency of heat waves. In fact, specific physical oceanography studies on South Atlantic heat waves are warranted for further context.
The resistance of South Atlantic coral fauna to bleaching was first proposed by Leão et al. (2003, 2010) likely as a consequence of biogeographic and evolutionary processes. Caribbean and South Atlantic coral fauna were separated probably during the late Cretaceous, when continental elevation in western South America and changes in ocean circulation directed the flow of the Amazon River to the Atlantic Ocean (Frost, 1977; Leão, 1983; Leão et al., 2003). These events resulted in an adverse oceanographical environment for the development of coral reefs, thereby selecting resistant species (Leão et al., 2003, 2008; Vasconcelos et al., 2018). This kept acroporids entirely out of the South Atlantic and favored massive species with large corallites, which aid in sediment cleaning and heterotrophic feeding (Logan, 1988; Crabbe and Smith, 2002; Mies et al., 2018; Marangoni et al., 2019). Therefore, South Atlantic corals may represent something of a resistant subset of Caribbean coral diversity.
Perspective and Conclusion
Coral bleaching is intensifying in the Indo-Pacific and Caribbean as large-scale episodes are becoming more frequent and longer-lasting (Hughes et al., 2019). However, this does not seem to be the case for the South Atlantic. Our results show that South Atlantic corals occupy greater depth zones, are tolerant to higher turbidity and nutrification, are morphologically resistant, engaged in flexible symbiotic associations, and most importantly, are less affected by bleaching (Figures 4A,B). In addition, they harbor numerous mesophotic reefs, possibly more than anywhere else in the world, that may serve as additional refugia (Soares et al., 2019). This qualifies South Atlantic reefs, especially its largest reefs in the Abrolhos Bank, as bioclimatic units (BCUs). BCUs are defined as large reef areas with lower vulnerability to climate disturbances and well-connected to surrounding marine ecosystems (Hoegh-Guldberg et al., 2018). However, it is important to note that although South Atlantic reefs have been historically less affected by bleaching, they are still vulnerable to increases in sea surface temperature (Kelmo et al., 2003).
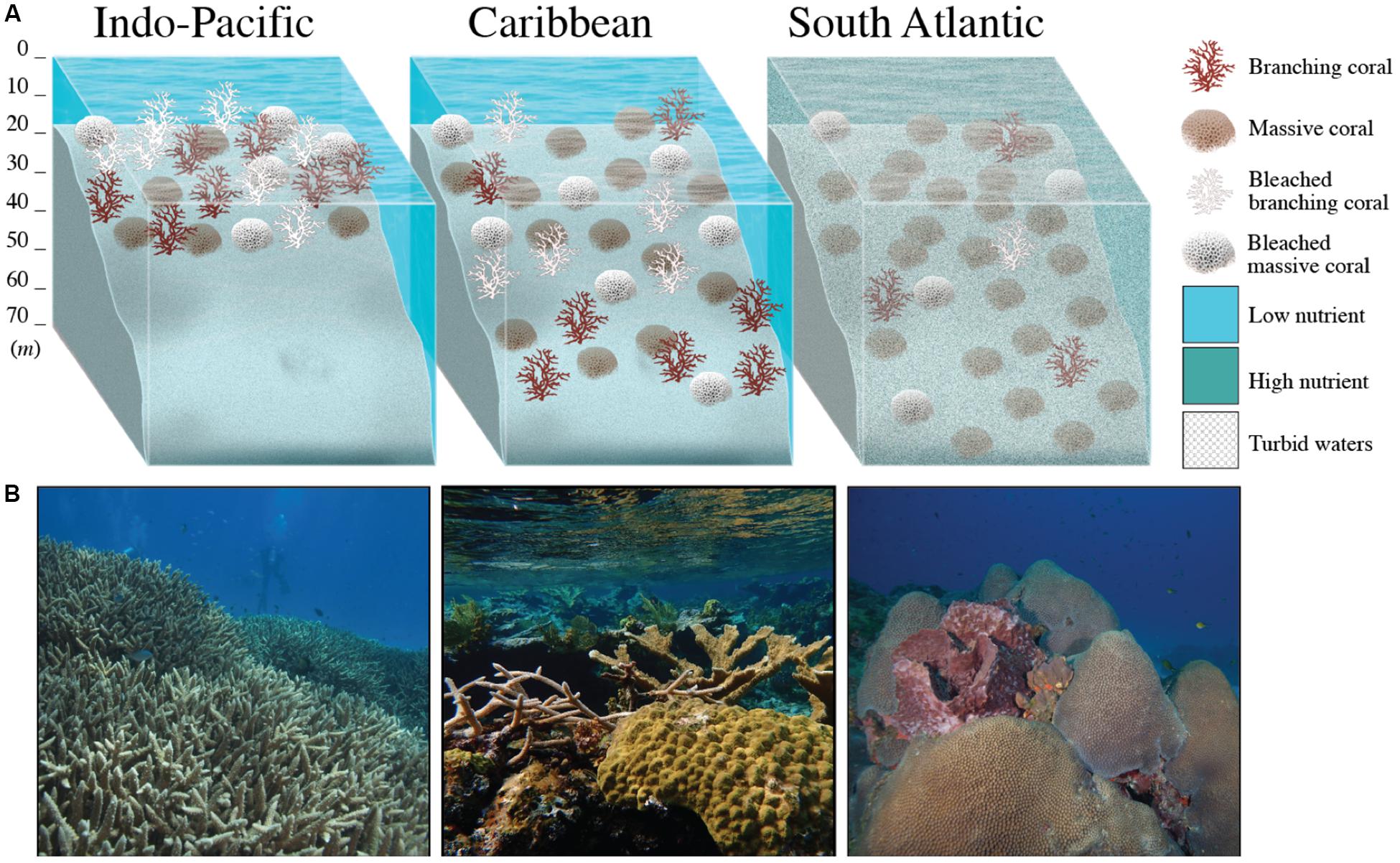
Figure 4. General comparison of Indo-Pacific, Caribbean and South Atlantic reefs: (A) Depiction of main characteristics that make South Atlantic reefs more resistant: (i) deeper mean distribution limit for coral species, (ii) tolerance of coral species to turbid conditions, (iii) reefs found in higher nutrient conditions, (iv) predominance of massive coral species (with proportion of massive and branching forms displayed according to Figure 2), (v) prevalence of generalist corals and symbionts (not shown in figure), and (vi) lower incidence of severe bleaching episodes (with proportion of bleached colonies according to Figure 3). (B) In situ photographs of typical coral reef morphology in the Indo-Pacific (branching forms at Milln Reef, Great Barrier Reef), Caribbean (mixed branching and massive forms at Andros Island, Bahamas) and South Atlantic (massive forms at Fernando de Noronha Archipelago, Brazil).
The global disparity in coral reef resistance and resilience to climate change has already been demonstrated for the Indo-Pacific and Caribbean (Roff and Mumby, 2012; Cinner et al., 2016) but never assessed comprehensively for the South Atlantic. Besides the vertical (bathymetric) refugium that South Atlantic reefs may offer, their extended latitudinal distribution of approximately 3,000 km makes them one of the largest coral reef refugia in the world. However, it remains to be seen how these reefs will respond under continuing ocean warming. Unfortunately, South Atlantic reefs and its marine protected areas still lack adequate management programs and enforcement. In face of the increasing magnitude of climate change, local management is often ineffective for oligotrophic reefs dominated by bleaching-susceptible species (Bruno et al., 2019). However, this may not be the case for the South Atlantic, where climate change impacts on corals are less dramatic due to intrinsic characteristics. Therefore, enhancing local conservation policies is warranted. Climate refugia may offer habitat persistence and increase biodiversity over evolutionary time scales (Pellissier et al., 2014) highlighting the importance of considering South Atlantic reefs as priorities for conservation.
Data Availability Statement
All datasets generated for this study are included in the article/Supplementary Material.
Author Contributions
MM, RF-F, CZ, AGG, GL, PS, and TB designed the work. MM, RF-F, CZ, AGG, GL, EL, and TB collected data. MM, RF-F, AGG, AZG, and TB analyzed the data. All authors contributed to the manuscript.
Funding
GL acknowledges funding from Serrapilheira Institute (Serra1708-15364). PS and GL acknowledge CNPq research productivity provided by CNPq (grant numbers 301089/2016-7 and 310517/2019-2, respectively).
Conflict of Interest
The authors declare that the research was conducted in the absence of any commercial or financial relationships that could be construed as a potential conflict of interest.
Acknowledgments
We thank Coral Vivo Institute, Petrobras (through the Petrobras Environmental Program) and Arraial d’Ajuda Eco Parque for data provided, Linda Waters and Christian Voolstra for reviewing the manuscript and Fernando Saraiva, Gayle Plaia, and Zaira Matheus for illustrations and pictures.
Supplementary Material
The Supplementary Material for this article can be found online at: https://www.frontiersin.org/articles/10.3389/fmars.2020.00514/full#supplementary-material
References
Amado-Filho, G. M., Moura, R. L., Bastos, A. C., Salgado, L. T., Sumida, P. Y. G., Güth, A. Z., et al. (2012). Rhodolith beds are major CaCO3 bio-factories in the tropical South West Atlantic. PLoS One 7:e35171. doi: 10.1371/journal.pone.0035171
Amaral, F. D., Hudson, M., and Steiner, A. (2006). Note on the widespread bleaching observed at the Manuel Luiz Marine State Park, Maranhão, Brazil. Arq. Ciênc. Mar 39, 138–141.
Amaral, F. D., Steiner, A. Q., Broadhurst, M. K., and Cairns, S. D. (2008). An overview of the shallow-water calcified hydroids from Brazil (Hydrozoa: Cnidaria), including the description of a new species. Zootaxa 1930, 56–68. doi: 10.11646/zootaxa.1930.1.4
Arif, C., Daniels, C., Bayer, T., Banguera-Hinestroza, E., Barbrook, A., Howe, C. J., et al. (2014). Assessing Symbiodinium diversity in scleractinian corals via next-generation sequencing-based genotyping of the ITS2 rDNA region. Mol. Ecol. 23, 4418–4433. doi: 10.1111/mec.12869
Baird, A. H., Cumbo, V. R., Leggat, W., and Rodriguez-Lanetty, M. (2007). Fidelity and flexibility in coral symbioses. Mar. Ecol. Prog. Ser. 347, 307–309.
Baird, A. H., Madin, J. S., Álvarez-Noriega, M., Fontoura, L., Kerry, J. T., Kuo, C.-Y., et al. (2018). A decline in bleaching suggest that depth can provide a refuge from global warming in most coral taxa. Mar. Ecol. Prog. Ser. 603, 257–264. doi: 10.3354/meps12732
Baker, A. C. (2003). Flexibility and specificity in coral-algal symbiosis: diversity, ecology, and biogeography of Symbiodinium. Annu. Rev. Ecol. Evol. Syst. 34, 661–689. doi: 10.1146/annurev.ecolsys.34.011802.132417
Baker, A. C., Starger, C. J., McClanahan, T. R., and Glynn, P. W. (2004). Corals’ adaptive response to climate change. Nature 430:741. doi: 10.1038/430741a
Banha, T. N. S., Capel, K. C. C., Kitahara, M. V., Francini-Filho, R. B., Francini, C. L. B., Sumida, P. Y. G., et al. (2019). Low coral mortality during the most intense bleaching event ever recorded in subtropical Southwestern Atlantic reefs. Coral Reefs. doi: 10.1007/s00338-019-01856-y
Bellwood, D. R., Hoey, A. S., and Hughes, T. P. (2012). Human activity selectively impacts the ecosystem roles of parrotfishes on coral reefs. Proc. R. Soc. B 279, 1621–1629. doi: 10.1098/rspb.2011.1906
Berkelmans, R., and van Oppen, M. J. H. (2006). The role of zooxanthellae in the thermal tolerance of corals: a ‘nugget of hope’ for coral reefs in an era of climate change. Proc. R. Soc. B 273, 2305–2312. doi: 10.1098/rspb.2006.3567
Bongaerts, P., Ridgway, T., Sampayo, E. M., and Hoegh-Guldberg, O. (2010). Assesing the ‘deep reef refugia’ hypothesis: focus on Caribbean reefs. Coral Reefs 29, 309–327. doi: 10.1007/s00338-009-0581-x
Bruno, J. F., Côté, I. M., and Toth, L. T. (2019). Climate change, coral loss, and the curious case of the parrotfish paradigm: Why don’t marine protected areas improve reef resilience? Annu. Rev. Mar. Sci. 11, 307–334. doi: 10.1146/annurev-marine-010318-095300
Cacciapaglia, C., and van Woesik, R. (2016). Climate-change refugia: shading reef corals by turbidity. Glob. Change Biol. 22, 1145–1154. doi: 10.1111/gcb.13166
Castro, C. B., and Pires, D. O. (2001). Brazilian coral reefs: what we already know and what is still missing. Bull. Mar. Sci. 69, 357–371.
Castro, C. B., Pires, D. O., Medeiros, M. S., Loiola, L. L., Arantes, R. C. M., Thiago, C. M., et al. (2006). “Filo Cnidaria,” in Biodiversidade Bentônica da Região Central da Zona Econômica Exclusiva Brasileira, eds H. P. Lavrado and B. L. Ignacio (Rio de Janeiro: Museu Nacional).
Castro, C. B., Segal, B., Negrão, F., and Calderon, E. N. (2012). Four-year monthly sediment deposition on turbid Southwestern Atlantic coral reefs, with a comparison of benthic assemblages. Braz. J. Oceanogr. 60, 49–63. doi: 10.1590/s1679-87592012000100006
Cesar, H., Burke, L., and Pet-Soede, L. (2003). The Economics of Worldwide Coral Reef Degradation. Arnhem: Cesar Environmental Economics Consulting (CEEC).
Chen, B., Yu, K., Qin, Z., Liang, J., Wang, G., Huang, X., et al. (2020). Dispersal, genetic variation, and symbiont interaction network of heat-tolerant endosymbiont Durusdinium trenchii: insights into the adaptive potential of coral to climate change. Sci. Total Environ. 723, 138026. doi: 10.1016/j.scitotenv.2020.138026
Cinner, J. E., Huchery, C., MacNeil, M. A., Graham, N. A. J., McClanahan, T. R., Maina, J., et al. (2016). Bright spots among the world’s coral reefs. Nature 535, 416–419.
Clark, T. R., Roff, G., Zhao, J.-X., Feng, Y.-X., Done, T. J., McCook, L. J., et al. (2017). U-Th dating reveals regional-scale decline of branching Acropora corals on the Great Barrier Reef over the past century. Proc. Natl. Acad. Sci. U.S.A. 114, 10350–10355. doi: 10.1073/pnas.1705351114
Costa, O. S. Jr., Attrill, M. J., and Nimmo, M. (2006). Seasonal and spatial controls on the delivery of excess nutrients to nearshore and offshore coral reefs of Brazil. J. Mar. Syst. 60, 63–74. doi: 10.1016/j.jmarsys.2005.11.006
Costa, O. S. Jr., Attrill, M. J., Pedrini, A. G., and de Paula, J. C. (2002). Spatial and seasonal distribution of seaweeds on coral reefs from Southern Bahia, Brazil. Bot. Mar. 45, 346–355.
Costa, O. S. Jr., Nimmo, M., and Attrill, M. J. (2008). Coastal nitrification in Brazil: a review of the role of nutrient excess on coral reef demise. J. S. Am. Earth Sci. 25, 257–270. doi: 10.1016/j.jsames.2007.10.002
Côté, I. M., and Darling, E. S. (2010). Rethinking ecosystem resilience in the face of climate change. PLoS Biol. 8:e1000438. doi: 10.1371/journal.pbio.1000438
Crabbe, M. J. C., and Smith, D. J. (2002). Comparison of two reef sites in the Wakatobi Marine National Park (S. E. Sulawesi) using digital image analysis. Coral Reefs 21, 242–244. doi: 10.1007/s00338-002-0250-9
De’ath, G., Fabricius, K. E., Sweatman, H., and Puotinen, M. (2012). The 27-year decline of coral cover on the Great Barrier Reef and its causes. Proc. Natl. Acad. Sci. U.S.A. 109, 17995–17999. doi: 10.1073/pnas.1208909109
DeCarlo, T. M., Cohen, A. L., Wong, G. T. F., Davis, K. A., Lohmann, P., and Soong, K. (2017). Mass coral mortality under local amplification of 2°C ocean warming. Sci. Rep. 7:44586.
Descombes, P., Wisz, M. S., Leprieur, F., Parravicini, V., Heine, C., Olsen, S. M., et al. (2015). Forecasted coral reef decline in marine biodiversity hotspots under climate change. Glob. Change Biol. 21, 2479–2487. doi: 10.1111/gcb.12868
Donner, S. D., Rickbeil, G. J. M., and Heron, S. F. (2017). A new, high-resolution global mass coral bleaching database. PLoS One 12:e0175490. doi: 10.1371/journal.pone.0175490
Duarte, G. A. S., Villela, H. D. M., Deocleciano, M., Silva, D., Barno, A., Cardoso, P. M., et al. (2020). Heat waves are a major threat to turboid coral reefs in Brazil. Front. Mar. Sci. 7:179. doi: 10.3389/fmars.2020.00179
Dutra, L. X. C., Kikuchi, R. K. P., and Leão, Z. M. A. N. (2006). Sediment accumulation on reef corals from Abrolhos, Bahia, Brazil. J. Coast. Res. 39, 639–644.
Eakin, C. M., Morgan, J. A., Heron, S. F., Smith, T. B., Liu, G., Alvarez-Filip, L., et al. (2010). Caribbean corals in crisis: record thermal stress, bleaching, and mortality in 2005. PLoS One 5:e13969. doi: 10.1371/journal.pone.0013969
Eakin, C. M., Sweatman, H. P. A., and Brainard, R. E. (2019). The 2014–2017 global-scale coral bleaching event: insights and impacts. Coral Reefs 38, 539–545. doi: 10.1007/s00338-019-01844-2
Ezzat, L., Maguer, J.-F., Grover, R., Rottier, C., Tremblay, P., and Ferrier-Pagès, C. (2019). Nutrient starvation impairs the trophic plasticity of reef-building corals under ocean warming. Funct. Ecol. 33, 643–653. doi: 10.1111/1365-2435.13285
Fitt, W. K., Brown, B. E., Warner, M. E., and Dunne, R. P. (2001). Coral bleaching: interpretation of thermal tolerance limits and thermal thresholds in tropical corals. Coral Reefs 20, 51–65. doi: 10.1007/s003380100146
Floeter, S. R., Rocha, L. A., Robertson, D. R., Joyeux, J. C., Smith-Vaniz, W. F., Wirtz, P., et al. (2008). Atlantic reef fish biogeography and evolution. J. Biogeogr. 35, 22–47.
Francini-Filho, R. B., Coni, E. O., Meirelles, P. M., Amado-Filho, G. M., Thompson, F. L., Pereira-Filho, G. H., et al. (2013). Dynamics of coral reef benthic assemblages of the Abrolhos Bank, Eastern Brazil: inferences on the natural and anthropogenic drivers. PLoS One 8:e54260. doi: 10.1371/journal.pone.0054260
Francini-Filho, R. B., Moura, R. L., Thompson, F. L., Reis, R. M., Kaufman, L., Kikuchi, R. K. P., et al. (2008). Diseases leading to accelerated decline of reef corals in the largest South Atlantic reef complex (Abrolhos Bank, eastern Brazil). Mar. Pollut. Bull. 56, 1008–1014. doi: 10.1016/j.marpolbul.2008.02.013
Francini-Filho, R. B., Velásquez, V. M., Silva, M. B., Rosa, M. R., Sumida, P. Y. G., Pinheiro, H. T., et al. (2019). “Brazil,” in Mesophotic Coral Ecosystems, eds Y. Loya, K. A. Puglise, and T. C. L. Bridge (Cham: Springer), 163–198.
Freitas, L. M., Oliveira, M. D. M., Leão, Z. M. A. N., and Kikuchi, R. K. P. (2019). Effects of turbidity and depth on the bioconstruction of the Abrolhos reefs. Coral Reefs 38, 241–253. doi: 10.1007/s00338-019-01770-3
Frost, S. H. (1977). “Cenozoic reef system of the Caribbean – Prospects for paleoecologic synthesis,” in Reef and Related Carbonates, Ecology and Sedimentology, eds S. H. Frost et al. (Tulsa, OK: American Association of Petroleum Geologists), 93–110. doi: 10.1306/st4393c8
Gates, R. D., and Edmunds, P. J. (1999). The physiological mechanisms of acclimatization in tropical reef corals. Integr. Comp. Biol. 39, 30–43. doi: 10.1093/icb/39.1.30
Ghisolfi, R. D., Silva, M. P., Santos, F. T., Servino, R. N., Cirano, M., and Thompson, F. L. (2015). Physical forcing mechanisms controlling the variability of chlorophyll-a over the Royal-Charlotte and Abrolhos Banks — Eastern Brazilian Shelf. PLoS One 10:e0117082. doi: 10.1371/journal.pone.0117082
Gilmour, J. P., Smith, L. D., Heyward, A. J., Baird, A. H., and Pratchett, M. S. (2013). Recovery of an isolated coral reef system following severe disturbance. Science 340, 69–71. doi: 10.1126/science.1232310
Gintert, B. E., Manzello, D. P., Enochs, I. C., Kolodziej, G., Carlton, R., Gleason, A. C. R., et al. (2018). Marked annual coral bleaching resilience of an inshore patch reef in the Florida Keys: a nugget of hope, aberrance, or last man standing? Coral Reefs 37, 533–547. doi: 10.1007/s00338-018-1678-x
Glynn, P. W. (1993). Coral reef bleaching: ecological perspectives. Coral Reefs 12, 1–17. doi: 10.1007/bf00303779
Glynn, P. W. (1996). Coral reef bleaching: facts, hypotheses and implications. Glob. Change Biol. 2, 495–509. doi: 10.1111/j.1365-2486.1996.tb00063.x
Graham, N. A. J. (2014). Habitat complexity: coral structural loss leads to fisheries declines. Curr. Biol. 24, R359–R361.
Hoegh-Guldberg, O. (1999). Climate change, coral bleaching and the future of world’s coral reefs. Mar. Freshw. Res. 50, 839–866.
Hoegh-Guldberg, O., Kennedy, E. V., Beyer, H. L., McClennen, C., and Possingham, H. P. (2018). Securing a long-term future for coral reefs. Trends Ecol. Evol. 33, 936–944. doi: 10.1016/j.tree.2018.09.006
Hoegh-Guldberg, O., Mumby, P. J., Hooten, A. J., Steneck, R. D., Greenfield, P., Gomez, E. D., et al. (2007). Coral reefs under rapid climate change and ocean acidification. Science 318, 1737–1742.
Holstein, D. M., Paris, C. B., Vaz, A. C., and Smith, T. B. (2016). Modeling vertical connectivity and mesophotic refugia. Coral Reefs 35, 23–37. doi: 10.1007/s00338-015-1339-2
Hughes, T. P., Anderson, K. D., Connolly, S. R., Heron, S. F., Kerry, J. T., Lough, J. M., et al. (2018a). Spatial and temporal patterns of mass bleaching of corals in the Anthropocene. Science 359, 80–83. doi: 10.1126/science.aan8048
Hughes, T. P., Kerry, J. T., Álvarez-Noriega, M., Álvarez-Romero, J. G., Anderson, K. D., Baird, A. H., et al. (2017). Global warming and recurrent mass bleaching of corals. Nature 543, 373–377.
Hughes, T. P., Kerry, J. T., Baird, A. H., Connolly, S. R., Chase, T. J., Dietzel, A., et al. (2019). Global warming impairs stock-recruitment dynamics of corals. Nature 568, 387–390. doi: 10.1038/s41586-019-1081-y
Hughes, T. P., Kerry, J. T., Baird, A. H., Connolly, S. R., Dietzel, A., Eakin, C. M., et al. (2018b). Global warming transforms coral reef assemblages. Nature 556, 492–496. doi: 10.1038/s41586-018-0041-2
Hume, B. C. C., D’Angelo, C., Smith, E. G., Stevens, J. R., Burt, J. A., and Wiedenmann, J. (2015). Symbiodinium thermophilum sp. nov., a thermotolerant symbiotic alga prevalent in corals of the world’s hottest sea, the Persian/Arabian Gulf. Sci. Rep. 5:8562.
Hume, B. C. C., Ziegler, M., Poulain, J., Pochon, X., Romac, S., Boissin, E., et al. (2018). An improved primer set and amplification protocol with increased specificity and sensitivity targeting the Symbiodinium ITS2 region. PeerJ 6:e4816. doi: 10.7717/peerj.4816
Iglesias-Prieto, R., and Trench, R. K. (1994). Acclimation and adaptation to irradiance in symbiotic dinoflagellates. I. Responses of the photosynthetic unit to changes in photon flux density. Mar. Ecol. Prog. Ser. 113, 163–175. doi: 10.3354/meps113163
Kayanne, H. (2017). Validation of degree heating weeks as a coral bleaching index in the northwestern Pacific. Coral Reefs 36, 63–70. doi: 10.1007/s00338-016-1524-y
Kelmo, F., Attrill, M. J., and Jones, M. B. (2003). Effects of the 1997-1998 El Niño on the cnidarian community of a high turbidity coral reef system (northern Bahia, Brazil). Coral Reefs 22, 541–550.
Kennedy, E. V., Perry, C. T., Halloran, P. R., Iglesias-Prieto, R., Schönberg, C. H. L., Wisshak, M., et al. (2013). Avoiding coral reef functional collapse requires local and global action. Curr. Biol. 23, 912–918. doi: 10.1016/j.cub.2013.04.020
Knowlton, N., Brainard, R. E., Fisher, R., Moews, M., Plaisance, L., and Caley, M. J. (2010). “Coral reef biodiversity,” in Life in the World’s Oceans: Diversity, Distribution, and Abundance, ed. A. D. McIntyre (Oxford: Blackwell Publishing), 65–77.
Kuta, K., and Richardson, L. (2002). Ecological aspects of black band disease of corals: relationships between disease incidence and environmental factors. Coral Reefs 21, 393–398. doi: 10.1007/s00338-002-0261-6
LaJeunesse, T. C. (2001). Investigating the biodiversity, ecology, and phylogeny of endosymbiotic dinoflagellates in the genus Symbiodinium using the ITS region: in search of a “species” level marker. J. Phycol. 37, 866–880. doi: 10.1046/j.1529-8817.2001.01031.x
LaJeunesse, T. C. (2005). “Species” radiations of symbiotic dinoflagellates in the Atlantic and Indo-Pacific since the Miocene-Pliocene transition. Mol. Biol. Evol. 22, 570–581. doi: 10.1093/molbev/msi042
LaJeunesse, T. C., Parkinson, J. E., Gabrielson, P. W., Jeong, H. J., Reimer, J. D., Voolstra, C. R., et al. (2018). Systematic revision of Symbiodiniaceae highlights the antiquity and diversity of coral endosymbionts. Curr. Biol. 28, 2570–2580.e6. doi: 10.1016/j.cub.2018.07.008
LaJeunesse, T. C., Wham, D. C., Pettay, D. T., Parkinson, J. E., Keshavmurthy, S., and Chen, C. A. (2014). Ecologically differentiated stress-tolerant endosymbionts in the dinoflagellate genus Symbiodinium (Dinophyceae) Clade D are different species. Phycologia 53, 305–319. doi: 10.2216/13-186.1
Le Læuff, P., and von Cosel, R. (1998). Biodiversity patterns of the marine benthic fauna on the Atlantic coast of tropical Africa in relation to hydroclimatic conditions and paleogeographic events. Acta Oecol. 19, 309–321. doi: 10.1016/s1146-609x(98)80035-0
Leão, Z. M. A. N. (1983). Abrolhos: o refúgio pleistocênico de uma fauna terciária de corais. Rev. Ciênc. Terra 8, 22–24.
Leão, Z. M. A. N., and Kikuchi, R. K. P. (2001). “The Abrolhos reefs of Brazil,” in Coastal Marine Ecosystems of Latin America, eds U. Seeliger and B. Kjerfve (Berlin: Springer-Verlag), 83–96. doi: 10.1007/978-3-662-04482-7_7
Leão, Z. M. A. N., Kikuchi, R. K. P., Ferreira, B. P., Neves, E. G., Sovierzoski, H. H., Oliveira, M. D., et al. (2016). Brazilian coral reefs in a period of global change: a synthesis. Braz. J. Oceanogr. 64, 97–116. doi: 10.1590/s1679-875920160916064sp2
Leão, Z. M. A. N., Kikuchi, R. K. P., and Oliveira, M. D. M. (2008). Coral bleaching in Bahia reefs and its relation with sea surface temperature anomalies. Biota Neotrop. 8, 69–82.
Leão, Z. M. A. N., Kikuchi, R. K. P., Oliveira, M. D. M., and Vasconcellos, V. (2010). Status of eastern Brazilian coral reefs in time of climate changes. Pan Am. J. Aquat. Sci. 5, 224–235.
Leão, Z. M. A. N., Kikuchi, R. K. P., and Testa, V. (2003). “Corals and coral reefs of Brazil,” in Latin American Coral Reefs, ed. J. Cortés (Amsterdam: Elsevier), 9–52. doi: 10.1016/b978-044451388-5/50003-5
Leipe, T., Knoppers, B., Marone, E., and Camargo, R. (1999). Suspended matter transport in coral reefs waters of the Abrolhos Bank, Brazil. Geo Mar. Lett. 19, 186–195. doi: 10.1007/s003670050108
Lesser, M. P. (1996). Elevated temperatures and ultraviolet radiation cause oxidative stress and inhibit photosynthesis in symbiotic dinoflagellates. Limnol. Oceanogr. 41, 271–283. doi: 10.4319/lo.1996.41.2.0271
Lesser, M. P. (2006). Oxidative stress in marine environments: biochemistry and physiological ecology. Annu. Rev. Physiol. 68, 253–278. doi: 10.1146/annurev.physiol.68.040104.110001
Little, A. F., van Oppen, M. J. H., and Willis, B. (2004). Flexibility in algal endo-symbioses shapes growth in reef corals. Science 304, 1492–1494. doi: 10.1126/science.1095733
Liu, G., Strong, A. E., Skirving, W. J., and Arzayus, L. F. (2006). “Overview of NOAA Coral Reef Watch Program’s near-real-time satellite global coral bleaching monitoring activities,” in Proceedings of the 10th International Coral Reef Symposium, Okinawa, 1783–1793.
Logan, A. (1988). Sediment-shifting capability in the recent solitary coral Scolymia cubensis (Milne-Edwards and Haime) from Bermuda. Bull. Mar. Sci. 43, 241–248.
Loiola, M., Cruz, I. C. S., Lisboa, D. S., Mariano-Neto, E., Leão, Z. M. A. N., Oliveira, M. D. M., et al. (2019). Structure of marginal coral reef assemblages under different turbidity regime. Mar. Environ. Res. 147, 138–148. doi: 10.1016/j.marenvres.2019.03.013
Loiola, M., Oliveira, M. D. M., and Kikuchi, R. K. P. (2013). Tolerance of Brazilian brain coral Mussismilia braziliensis to sediment and organic matter inputs. Mar. Pollut. Bull. 77, 55–62. doi: 10.1016/j.marpolbul.2013.10.033
Loya, Y., Sakai, K., Yamazato, K., Nakano, Y., Sambali, H., and van Woesik, R. (2001). Coral bleaching: the winners and the losers. Ecol. Lett. 4, 122–131. doi: 10.1046/j.1461-0248.2001.00203.x
Madin, J. S., Hoogenboom, M. O., Connolly, S. R., Darling, E. S., Falster, D. S., Huang, D., et al. (2016). A trait-based approach to advance coral reef science. Trends Ecol. Evol. 31, 419–428. doi: 10.1016/j.tree.2016.02.012
Marangoni, L. F. B., Mies, M., Güth, A. Z., Banha, T. N. S., Inague, A., Fonseca, J. S., et al. (2019). Peroxynitrite generation and increased heterotrophic capacity are linked to the disruption of the coral-dinoflagellate symbiosis in scleractinian and hydrocoral species. Microorganisms 7:426. doi: 10.3390/microorganisms7100426
Meirelles, P. M., Amado-Filho, G. M., Pereira-Filho, G. H., Pinheiro, H. T., Moura, R. L., Joyeux, J.-C., et al. (2015). Baseline assessment of mesophotic reefs of the Vitória-Trindade seamount chain based on water quality, microbial diversity, benthic cover and fish biomass data. PLoS One 10:e0130084. doi: 10.1371/journal.pone.0130084
Mies, M., Güth, A. Z., Tenório, A. A., Banha, T. N. S., Waters, L. G., Polito, P. S., et al. (2018). In situ shifts of predominance between autotrophic and heterotrophic feeding in the reef-building coral Mussismilia hispida: an approach using fatty acid trophic markers. Coral Reefs 37, 677–689. doi: 10.1007/s00338-018-1692-z
Moberg, F., and Folke, C. (1999). Ecological good and services of coral reef ecosystems. Ecol. Econ. 29, 215–233. doi: 10.1016/s0921-8009(99)00009-9
Moore, C. M., Mills, M. M., Arrigo, K. R., Berman-Frank, I., Bopp, L., Boyd, P. W., et al. (2013). Processes and patterns of oceanic nutrient limitation. Nat. Geosci. 6, 701–710.
Morgan, K. M., Perry, C. T., Johnson, J., and Smithers, S. G. (2017). Nearshore turbid-zone corals exhibit high bleaching tolerance on the Great Barrier Reef following the 2016 ocean warming event. Front. Mar. Sci. 4:224. doi: 10.3389/fmars.2017.00224
Moura, R. L., Amado-Filho, G. M., Moraes, F. C., Brasileiro, P. S., Salomon, P. S., Mahiques, M. M., et al. (2016). An extensive reef system at the Amazon River mouth. Sci. Adv. 2:e1501252.
Omachi, C. Y., Asp, N. E., Siegle, E., Couceiro, M. A. A., Francini-Filho, R. B., and Thompson, F. L. (2019). Light availability for reef-building organisms in a plume-influenced shelf. Cont. Shelf Res. 181, 25–33. doi: 10.1016/j.csr.2019.05.005
Pellissier, L., Leprieur, F., Parravicini, V., Cowman, P. F., Kulbicki, M., Litsios, G., et al. (2014). Quaternary coral reef refugia preserved fish diversity. Science 344, 1016–1019. doi: 10.1126/science.1249853
Pereira-Filho, G. H., Amado-Filho, G. M., Moura, R. L., Bastos, A. C., Guimarães, S. M. P. B., Salgado, L. T., et al. (2011). Extensive rhodolith beds cover the summits of Southwestern Atlantic Ocean seamounts. J. Coast. Res. 28, 261–269. doi: 10.2112/11t-00007.1
Pereira-Filho, G. H., Shintate, G. S. I., Kitahara, M. V., Moura, R. L., Amado-Filho, G. M., Bahia, R. G., et al. (2019). The southernmost Atlantic coral reef is off the subtropical island of Queimada Grande (24°S), Brazil. Bull. Mar. Sci. 1:1. doi: 10.5343/bms
Perry, C. T., and Morgan, K. M. (2017). Bleaching drives collapse in reef carbonate budgets and reef growth potential on southern Maldives reefs. Sci. Rep. 7:40581.
Pettay, D. T., Wham, D. C., Smith, R. T., Iglesias-Prieto, R., and LaJeunesse, T. C. (2015). Microbial invasion of the Caribbean by an Indo-Pacific zooxanthella. Proc. Natl. Acad. Sci. U.S.A. 112, 7513–7518. doi: 10.1073/pnas.1502283112
Picciani, N., Seiblitz, I. G. L., Paiva, P. C., Castro, C. B., and Zilberberg, C. (2016). Geographic patterns of Symbiodinium diversity associated with the coral Mussismilia hispida (Cnidaria, Scleractinia) correlate with major reef regions in the Southwestern Atlantic Ocean. Mar. Biol. 163:236.
Pires, D. O., Segal, B., and Caparelli, A. C. (2011). Reproductive effort of an endemic major reef builder along an inshore-offshore gradient in south-western Atlantic. J. Mar. Biol. Assoc. U.K. 91, 1613–1616. doi: 10.1017/s0025315410000767
Pratchett, M. S., Bay, L. K., Gehrke, P. C., Koehn, J. D., Osborne, K., Pressey, R. L., et al. (2011). Contribution of climate change to degradation and loss of critical fish habitats in Australian marine and freshwater environments. Mar. Freshw. Res. 62, 1062–1081.
Ribeiro, F. V., Sá, J. A., Fistarol, G. O., Salomon, P. S., Pereira, R. C., Souza, M. L. A. M., et al. (2018). Long-term effects of competition and environmental driver on the growth of the endangered coral Mussismilia braziliensis (Verrill, 1867). PeerJ 6:e5419. doi: 10.7717/peerj.5419
Riegl, B., and Piller, W. E. (2003). Possible refugia for reefs in time of environmental stress. Int. J. Earth Sci. 92, 520–531. doi: 10.1007/s00531-003-0328-9
Roff, G., and Mumby, P. J. (2012). Global disparity in the resilience of coral reefs. Trends Ecol. Evol. 27, 404–413. doi: 10.1016/j.tree.2012.04.007
Ruiz-Ramos, D. V., Weil, E., and Schizas, N. V. (2014). Morphological and genetic evaluation of the hydrocoral Millepora species complex in the Caribbean. Zool. Stud. 53:4. doi: 10.1186/1810-522x-53-4
Santos, H. F., Carmo, F. L., Martirez, N., Duarte, G. A. S., Calderon, E. N., Castro, C. B., et al. (2016). Cyanobacterial and microeukaryotic profile of healthy, diseased, and dead Millepora alcicornis from the South Atlantic. Dis. Aquat. Org. 119, 163–172. doi: 10.3354/dao02972
Schlöder, C., and D’Croz, L. (2004). Responses of massive and branching coral species to the combined effects of water temperature and nitrate enrichment. J. Exp. Mar. Biol. Ecol. 313, 255–268. doi: 10.1016/j.jembe.2004.08.012
Semmler, R. F., Hoot, W. C., and Reaka, M. L. (2017). Are mesophotic coral ecosystems distinct communities and can they serve as refugia for shallow reefs? Coral Reefs 36, 433–444. doi: 10.1007/s00338-016-1530-0
Sheppard, C., Dixon, D. J., Gourlay, M., Sheppard, A., and Payet, R. (2005). Coral mortality increases wave energy reaching shores protected by reef flats: examples from the Seychelles. Estuar. Coast. Shelf Sci. 64, 223–234. doi: 10.1016/j.ecss.2005.02.016
Sheppard, C. R. C. (2003). Predicted recurrences of mass coral mortality in the Indian Ocean. Nature 425, 294–297. doi: 10.1038/nature01987
Sheppard, C. R. C., Davy, S. K., Pilling, G. M., and Graham, N. A. J. (2018). The Biology of Coral Reefs. Oxford: Oxford University Press.
Silva, A. S., Leão, Z. M. A. N., Kikuchi, R. K. P., Costa, A. B., and Souza, J. R. B. (2013). Sedimentation in the coastal reefs of Abrolhos over the last decades. Cont. Shelf Res. 70, 159–167. doi: 10.1016/j.csr.2013.06.002
Silva-Lima, A. W., Walter, J. M., Garcia, G. D., Ramires, N., Ank, G., Meirelles, P. M., et al. (2015). Multiple Symbiodinium strains are hosted by the Brazilian endemic corals Mussismilia spp. Microb. Ecol. 70, 301–310. doi: 10.1007/s00248-015-0573-z
Skirving, W. J., Heron, S. F., Marsh, B. L., Liu, G., De La Cour, J. L., Geiger, E. F., et al. (2019). The relentless march of mass coral bleaching: a global perspective of changing heat stress. Coral Reefs 38, 547–557. doi: 10.1007/s00338-019-01799-4
Smith, T. B., Glynn, P. W., Maté, J. L., Toth, L. T., and Gyory, J. (2014). A depth refugium from catastrophic coral bleaching prevents regional extinction. Ecology 95, 1663–1673. doi: 10.1890/13-0468.1
Smith, T. B., Gyory, J., Brandt, M. E., Miller, W. J., Jossart, J., and Nemeth, R. S. (2016). Caribbean Mesophotic Coral Ecosystems Are Unlikely Climate Change Refugia. Glob. Change Biol. 22, 2756–2765. doi: 10.1111/gcb.13175
Soares, M. O., Tavares, T. C. L., and Carneiro, P. B. M. D. (2019). Mesophotic ecosystems: distribution, impacts and conservation in the South Atlantic. Divers. Distrib. 25, 255–268.
Souza, J. N., Nunes, F. L. D., Zilberberg, C., Sanchez, J. A., Migotto, A. E., Hoeksema, B. W., et al. (2017). Contrasting patterns of connectivity among endemic and widespread fire coral species (Millepora spp.) in the tropical Southwestern Atlantic. Coral Reefs 36, 701–716. doi: 10.1007/s00338-017-1562-0
Spalding, M. D., Ravilious, C., and Green, E. P. (2001). World Atlas of Coral Reefs. Berkeley, CA: University of California Press.
Sully, S., and van Woesik, R. (2020). Turbid reefs moderate coral bleaching under climate-related temperature stress. Glob. Chang. Biol. 26, 1367–1373. doi: 10.1111/gcb.14948
Swain, T. D., Chandler, J., Backman, V., and Marcelino, L. (2017). Consensus thermotolerance ranking for 110 Symbiodinium phylotypes: an exemplar utilization of a novel iterative partial-rank aggregation tool with broad application potential. Funct. Ecol. 31, 172–183. doi: 10.1111/1365-2435.12694
Teixeira, C. D., Leitão, R. L. L., Ribeiro, F. V., Moraes, F. C., Neves, L. M., Bastos, A. C., et al. (2019). Sustained mass coral bleaching (2016–2017) in Brazilian turbid-zone reefs: taxonomic, cross-shelf and habitat-related trends. Coral Reefs 38, 801–813. doi: 10.1007/s00338-019-01789-6
Teschima, M. M., Garrido, A. G., Paris, A., Nunes, F. L. D., and Zilberberg, C. (2019). Biogeography of the endosymbiotic dinoflagellates (Symbiodiniaceae) community associated with the brooding coral Favia gravida in the Atlantic Ocean. PLoS One 14:e0215167. doi: 10.1371/journal.pone.0215167
van Woesik, R., Houk, P., Isechal, A. L., Idechong, J. W., Victor, S., and Golbuu, Y. (2012). Climate-change refugia in the sheltered bays of Palau: analogs of future reefs. Ecol. Evol. 2, 2474–2484. doi: 10.1002/ece3.363
Vasconcelos, M. J. O., Leão, Z. M. A. N., and Kikuchi, R. K. P. (2018). Coral reef growth pattern in eastern Brazil has not changed since the Holocene. Quat. Environ. Geosci. 9, 49–61.
Veron, J. E., Stafford-Smith, M. G., Turak, E., and DeVantier, L. M. (2016). Corals of the World. Available online at: http://www.coralsoftheworld.org/page/home/ (accessed May 20, 2019).
Wenger, A., Fabricius, K. E., Jones, G. P., and Brodie, J. E. (2015). “Effects of sedimentation, eutrophication and chemical pollution on coral reef fishes,” in Ecology of Fishes on Coral Reefs, ed. C. Mora (Cambridge: Cambridge University Press), 145–153. doi: 10.1017/cbo9781316105412.017
Keywords: Brazil, climate change, nutrients, reef-building, resilience, symbiosis, tolerance, turbidity
Citation: Mies M, Francini-Filho RB, Zilberberg C, Garrido AG, Longo GO, Laurentino E, Güth AZ, Sumida PYG and Banha TNS (2020) South Atlantic Coral Reefs Are Major Global Warming Refugia and Less Susceptible to Bleaching. Front. Mar. Sci. 7:514. doi: 10.3389/fmars.2020.00514
Received: 13 December 2019; Accepted: 04 June 2020;
Published: 25 June 2020.
Edited by:
Michael Sweet, University of Derby, United KingdomReviewed by:
Fabiano Thompson, Alberto Luiz Coimbra Institute for Graduate Studies and Engineering Research, Federal University of Rio de Janeiro, BrazilVerena Schoepf, University of Amsterdam, Netherlands
Copyright © 2020 Mies, Francini-Filho, Zilberberg, Garrido, Longo, Laurentino, Güth, Sumida and Banha. This is an open-access article distributed under the terms of the Creative Commons Attribution License (CC BY). The use, distribution or reproduction in other forums is permitted, provided the original author(s) and the copyright owner(s) are credited and that the original publication in this journal is cited, in accordance with accepted academic practice. No use, distribution or reproduction is permitted which does not comply with these terms.
*Correspondence: Miguel Mies, bWlndWVsLm1pZXNAdXNwLmJy