- Department of Biology, University of Louisiana at Lafayette, Lafayette, LA, United States
The northern Gulf of Mexico (nGOM) is a highly productive region and supports some of the world’s largest fisheries. Mesozooplankton represent a key linkage in coastal food webs for larval fish, both as food and as competition. While many studies have investigated seasonal patterns of mesozooplankton off the Louisiana coast and in the Mississippi Bight, there is little information about mesozooplankton communities on the Texas shelf. In this study, we investigated environmental drivers of mesozooplankton community variability over space and time. Samples were collected on the Texas shelf near Galveston Bay at seasonal intervals following Hurricane Harvey. Total mesozooplankton abundance were found to be highest in September. Diversity exhibited a hump-shaped pattern over the 6 months sampled, with the highest diversity occurring in October 2017. Taxa richness did not vary over the sampling period. Significant differences in mesozooplankton community structure were found only between September 2017 and March 2018. Community abundance was greatest nearshore, and zooplankton diversity was greatest on the shelf. Community structure was found to be driven by both temperature and salinity. Spatial and temporal patterns of specific larval fish prey are presented.
Introduction
The northern Gulf of Mexico (nGOM) is a highly productive region, referred to as the “fisheries fertile crescent” (Gunter, 1963). This area contributes just under a fifth of the United States’ annual landings and contains some of the most valuable fisheries in the country (Iverson and Martin, 2009; Keithly and Roberts, 2017). In marine ecosystems, mesozooplankton (0.2–2 mm) communities act as an important energy conduit between primary producers and higher trophic levels. Mesozooplankton production is tightly coupled with environmental conditions and can rapidly increase, or decrease, in response to changes in abiotic and biotic conditions such as salinity, temperature, and food availability. This in turn, alters the relative abundance, composition and overall diversity of the community (Quintana et al., 1998; Nielsen and Andersen, 2002; Leising et al., 2015; Cavole et al., 2016). Variability in mesozooplankton abundance, composition, and phenology have all been shown to impact higher trophic levels across all life stages, from fish larvae to adult forage fish (Cushing, 1990; Sullivan et al., 2001; Costello et al., 2006; Frederiksen et al., 2006; Robinson et al., 2015). The distribution of larval fish prey, such as chaetognaths, ostracods, or copepods, therefore, plays an important role in recruitment success (Govoni et al., 1983; Nip et al., 2003; Hoover, 2018). Many fisheries around the world, and within the Gulf of Mexico, have transitioned toward ecosystem-based fisheries management (EBFM), a strategy that incorporates the entire life cycle of the harvested species and accounts for complex interactions regarding food and habitat availability (Karnauskas et al., 2017). Resolving mesozooplankton distribution in space and time is crucial for the success of these management plans (Ortner et al., 1989).
Previous work investigating the spatial and seasonal trends of mesozooplankton in the nGOM have revealed general patterns. Nearshore communities have higher abundance and lower diversity than their shelf counterparts and are typically dominated by copepod nauplii and taxa with tolerances for lower salinity (12–23 ppt; Elliott et al., 2012; Roman et al., 2012; Dzwonkowski et al., 2017). As distance from shore increases, overall mesozooplankton abundance decreases and diversity increases (Ortner et al., 1989; Ditty et al., 2004; Dzwonkowski et al., 2017). These patterns have been shown across the nGOM with regional variations in magnitude (Ortner et al., 1989; Ditty et al., 2004). Among seasons, summer mesozooplankton communities generally have the highest abundance, followed by spring and fall (Ditty et al., 2004; Carassou et al., 2014). Winters typically have the lowest abundances (Ortner et al., 1989). While these studies provide general seasonal trends for the whole nGOM, variability of mesozooplankton community structure along coastal nGOM ecosystems is high and largely depends on local environmental drivers (Murawski et al., 2016; Criales et al., 2017; Dubickas, 2019).
It is important to note that the overwhelming majority of nGOM mesozooplankton studies focus on the eastern Louisiana shelf (Roman et al., 2012) and the Mississippi Bight (Greer et al., 2016, 2017; Criales et al., 2017; Parra et al., 2019) (but see Gomez et al., 2019). The oceanography of these areas is strongly controlled by the Mississippi-Atchafalaya River (MAR) discharge, where changes in temperature, salinity, and hypoxia are strongly coupled with seasonal changes in river flow (Spies et al., 2016). This relationship between MAR discharge and coastal oceanographic conditions is clearly reflected in the observed drivers of mesozooplankton community structure (Elliott et al., 2012; Roman et al., 2012), with temperature and salinity are the most commonly reported as seasonal drivers (Ortner et al., 1989; Elliott et al., 2012; Greer et al., 2016; Liu et al., 2017). Other MAR driven effects that are important in structuring mesozooplankton communities includes bottom water hypoxia due to stratificaiton (Elliott et al., 2012; Roman et al., 2012) and increased primary production (Dagg and Whitledge, 1991; Dagg and Breed, 2003). Additionally, mesozooplankton biomass is indirectly controlled through seasonal wind direction (Dubickas, 2019) through its impact on shelf circulation patterns (Gomez et al., 2019).
These environmental drivers act synergistically on individual taxonomic preferences, resulting in taxon-specific distributional patterns across the nGOM. Chaetognaths have been shown to be ubiquitously distributed between near- and offshore stations and across all depths, though some studies have found a correlation with colder, offshore waters (Dzwonkowski et al., 2017; Greer et al., 2017, 2016). Ostracods generally favor clear, salty offshore waters, far from terrestrial influence (Ortner et al., 1989; Dzwonkowski et al., 2017; Parra et al., 2019). Acartia tonsa is a widely reported calanoid copepod that is found nearshore in areas of high river influence, attributed to their ability to tolerate low salinities (Elliott et al., 2012; Roman et al., 2012; Liu et al., 2017). For predicting the survival of larval fish with mesozooplankton prey preferences, distributional information about these taxa are invaluable (Ortner et al., 1989). However, it is unknown if factors influencing mesozooplankton communities in the northwest nGOM are similar to those reported elsewhere in the nGOM, as the influence of MAR discharge is more ephemeral compared to the river dominated central nGOM and Mississippi Bight.
The northwestern GOM/western LATEX shelf is a wide, shallow-sloping continental shelf with limiting influence of seasonal MAR discharge (Du et al., 2019). Seasonal winds dictate nearshore surface currents with localized ephemeral influence from storm events (Nowlin et al., 2005) or ENSO-related anomalies (Gomez et al., 2019). For the majority of the year (September – April), the shelf is dominated by northernly surface currents (Cochrane and Kelly, 1986; Johnson, 2008). Wind direction changes during summer (May – August), resulting in a southerly current structure (Cochrane and Kelly, 1986; Nowlin et al., 2005). Episodic storm events such as fronts and hurricanes can reverse these seasonal dominant currents for up to 2 weeks (Nowlin et al., 2005). While estuaries of this region are well studied regarding both physical and biological dynamics (i.e., Galveston Bay; Liu et al., 2017, 2019; D’Sa et al., 2019), much less is known about planktonic systems on the shelf. The Texas area of the LATEX shelf is unique in the nGOM as it retains similar physical qualities of MAR influenced areas (broad shelf, muddy benthos) but with a lower connectedness to MAR discharge (Spies et al., 2016; Du et al., 2019). This lack of freshwater influence may alter hypothesized drivers of seasonal mesozooplankton structure.
Shortly following Hurricane Harvey (August 2017), we sampled a near- to offshore transect on the Texas shelf near Galveston Bay over a period of 6 months (September – March) in order to describe the distribution and temporal variability of mesozooplankton communities. In this paper, we quantify for the first-time patterns of mesozooplankton community abundance, diversity, and composition of northwestern Gulf of Mexico. We discuss our findings in the context of environmental drivers as well previous estimates of mesozooplankton abundance in the nGOM. Finally, we highlight the abundance and distributional patterns of select larval fish prey in order to understand food web implications of the observed spatial and temporal variability in mesozooplankton communities.
Materials and Methods
Study Area
We conducted bi-monthly cruises along an inshore to offshore transect from September 2017 to March 2018. We collected zooplankton samples at stations located off the coast of Galveston, Texas, and coincided with established SEAMAP stations (Figure 1). Our stations were arranged north-to-south to yield nearshore to offshore transects for the evaluation of spatial patterns. The first sampling effort was during a NOAA NFMS SEAMAP Survey cruise in September 2017, 3 weeks following the landfall of Hurricane Harvey. The following three sampling events occurred approximately at approximately 2-months intervals (October 2017, January, and March 2018) to allow us to track the temporal evolution of planktonic systems and food web processes. Nearshore stations were in water less than 60 m in depth. Shelf stations had depths between 60 and 150 m.
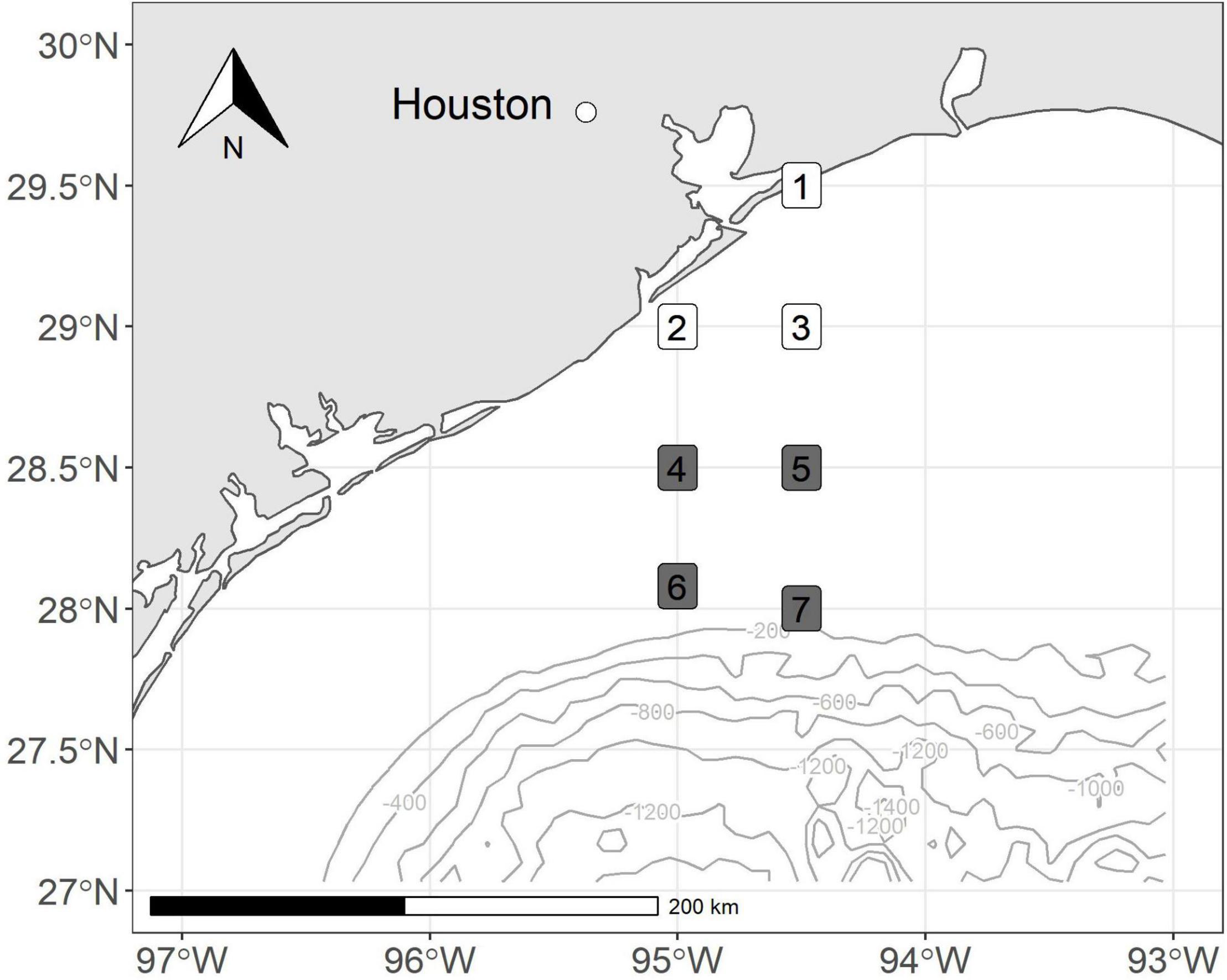
Figure 1. Map of stations sampled off the coast of Texas, United States. Colors correspond to depth bin (nearshore = white, shelf = gray).
Physical Data
At each of the six stations and for all 4 months, we collected physical data via vertical casts of a SEABIRD (9plus; SBE 11) CTD instrument suite to determine the influence of environmental conditions on mesozooplankton community structure. CTD data was recorded every 0.2 m and subsequently smoothed by removing any errant values (i.e., negative temperatures or salinities) using the “castr” package in R.1 We plotted all depth profiles to compare recorded parameter values against adjacent stations to control for outliers or sensor malfunctions. Parameter values were summarized between 0 and 150 m as this was the deepest any of the plankton nets were fished. Both average and median values were used in model parametrization.
Field Collection of Mesozooplankton
Mesozooplankton were collected in vertically integrated, oblique plankton tows at all stations. During October 2017, January 2018, and March 2018, a 1-m2, 333-μm mesh Multiple Opening/Closing Net Environmental Sampling System (MOCNESS) was used at stations with water depths >5 m. A single MOCNESS net was towed from either 2 m above sea floor in water depths <100 m or from 100 m at depths ≥100 m to the ocean’s surface. Samples were not collected from nearshore site B221 (Figure 1) in January or March due to gear malfunction. As the initial net of the MOCNESS integrates the water column fished, these methods allowed us to collect comparable data to the September 2017 SEAMAP Survey, which used a 0.5-m2 diameter, 333-μm mesh bongo net towed obliquely from 2 m above sea floor to the ocean’s surface (G. Zapfe, pers. comm.). For all gear types, we washed the outside bottom third of each net down into the cod-end with surface seawater using a hose. We then transferred the cod-end contents to a 5-gallon bucket and concentrated to one liter using a 200-μm mesh sieve. All samples were preserved in 5% buffered formalin and seawater solution.
Mesozooplankton Sample Processing
We identified and quantified mesozooplankton using workflow that included a ZooScan benchtop plankton imaging system (Hydroptic v3 ZSCA04; Gorsky et al., 2010). ZooScan produces individual shadow-graphic vignettes of particles from sizes of 200 μm and up (Supplementary Figure S1, Gorsky et al., 2010). Plankton samples settled for 20 min in a graduated cylinder prior to scanning. Internal laboratory tests revealed no statistical difference between the settled volumes of the same sample settled for 20 min versus 24 h. Samples were gently homogenized with an aquarium bubbler for 60 s. Following homogenization, a 5-ml aliquot was transferred to a ZooScan using a Henson-Stempel pipette. Each aliquot was scanned and the resultant TIFF image processed into particle-specific vignettes using the ZooProcess software (Hydroptic; Gorsky et al., 2010). Image processing included subtraction of the scanner-specific background blanks generated prior to the start of scanning each day. Three aliquots per sample were scanned. The vignettes of all three aliquots were then combined prior to classification. We classified the vignettes using the EcoTaxa platform.2 Plankton vignettes were manually sorted into 31 groups ranging from 200 μm to a max of 5 cm, by trained experts on the EcoTaxa platform to order or the lowest taxonomic level image resolution allowed. Subsamples of each sorter’s images were checked periodically for quality control by two other sorters to maintain accurate sorting delegations. For the full list of taxa categories used, see Supplementary Table S1. Counts of organisms were standardized to individual per cubic meter using the equation:
Community Analysis
To quantify variability in mesozooplankton community structure in space and over time, we calculated taxa richness, abundance (indv/m3), and Shannon-Weiner diversity metrics using the “vegan” package in R Statistical Software (Oksanen et al., 2019; R Core Team, 2019). Normality of the data was assessed using a Shapiro–Wilks test. Due to non-normality of the abundance data even after transformation, differences in mesozooplankton abundance were determined using a Kruskal–Wallis test. Differences in abundance between factors were assessed using a pairwise-Wilcox test to determine significance. P-values were adjusted following Benjamini and Hochberg (1995) in order to control for false discovery rate. Normally distributed responses (richness, diversity) were assessed using a two-way ANOVA followed by a post-hoc Tukey tests to determine significant differences between factors. Results were statistically significant at α ≤ 0.05.
The mesozooplankton community structure of each station and sampling event (n = 26) was evaluated using a Bray-Curtis dissimilarity matrix with a non-metric multidimensional scaling analysis (NMDS). We applied a Hellinger transformation to account for, and subsequently transform, high taxa abundances. We ran a permutational multivariate analysis of variance (PERMANOVAs) with “sampling event” (September, October, January, March), and “position” (nearshore, shelf) as grouping factors. A permutational post-hoc PERMANOVA was run (n = 9999) using a subset of the data to determine if differences between factor levels were statistically significant (pairwise.adonis, Martinez Arbizu, 2019). A similarity percentage analysis (SIMPR, “vegan” package, Oksanen et al., 2019) was used to determine taxa driving the differences between statistically different factor levels.
We performed a degradation analysis for each community metric to determine if taxonomic level influenced our observed patterns. Taxa were degraded to both order and then class, at which all models were tested. Taxa that were impossible to identify to order due to image resolution (e.g., invertebrate larvae or ostracods) were maintained at the lowest taxonomic grouping image resolution allowed. All community metric trends, sans taxa richness over time, were recovered when degraded. All analysis, therefore, were performed using taxa identified to order. This approach maintained taxonomic consistency without compromising functionally important groups, such as copepods.
Environmental drivers of community structure were evaluated with a BIOENV analysis (Clarke and Ainsworth, 1993). This analysis correlates a community dissimilarity matrix with all possible combinations of an environmental matrix. The best correlations are produced as a subset of all the environmental parameters input. The environmental matrix is compared using Euclidean distance after standardizing the data by mean and standard deviation. The community matrix used was calculated as for the other multivariate analyses (Hellinger transformation, Bray-Curtis dissimilarity). Strengths of relationships between the community and environmental matrices were assessed with Spearman correlation. Mean temperature (°C), salinity (psu), dissolved oxygen (mL/L), and transmittance (%) of the water column at each station was included in the analysis as these parameters were collected at all stations over all cruises.
Results
Mesozooplankton density (indv/m3) showed a slight U-shape pattern over time (Figure 2A). Densities of mesozooplankton from September 2017 were significantly higher than all other months (Table 1, p < 0.01). Nearshore stations appeared to have higher densities than shelf stations (Supplementary Figure S2) but were not found to be statistically different (Table 1). Taxa richness was slightly higher in October relative to other months (Table 1 and Figure 2B). A difference in richness was also found between nearshore and shelf stations (Table 1, p = 0.01) with higher richness found at shelf stations (Supplementary Figure S2). Diversity followed a “hump-shaped” over the 6 months sampled. Diversity peaked during October 2017 (Figure 2C) and was found to be statistically different from September (Tukey HSD, p = 0.04) and March 2018 (Tukey HSD, p = 0.02). Shelf station diversity was elevated compared to nearshore stations (Supplementary Figure S2 and Table 1).
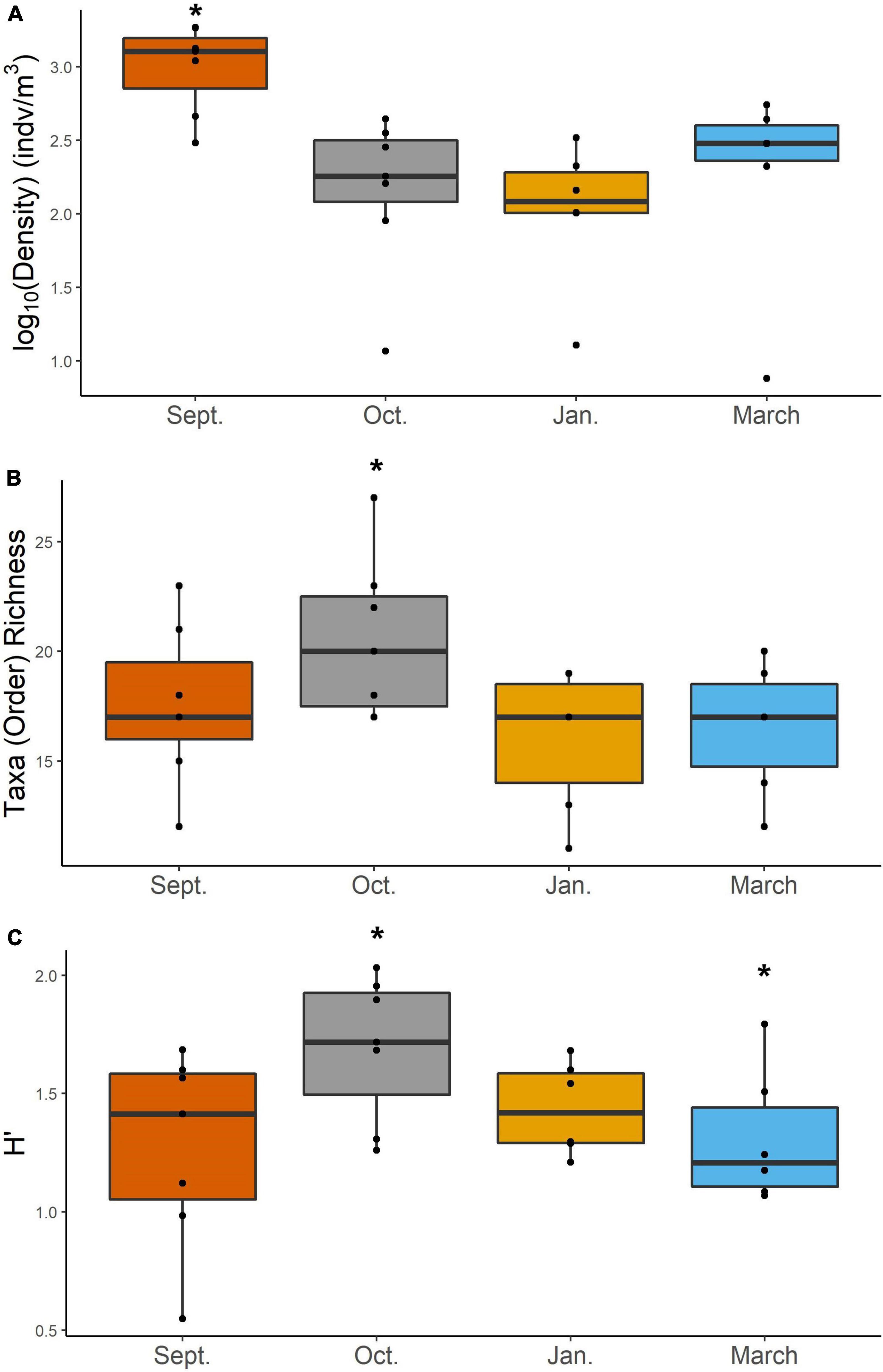
Figure 2. Density (log10 transformed) (A), taxa richness at order (B), and Shannon-Weiner diversity (H′) (C) of mesozooplankton for each cruise. Asterisk denotes groups that are significantly different based on a two-way ANOVA and post-hoc Tukey test. Differences in abundance were determined using a Kruskal–Wallis and pairwise-Wilcox test.
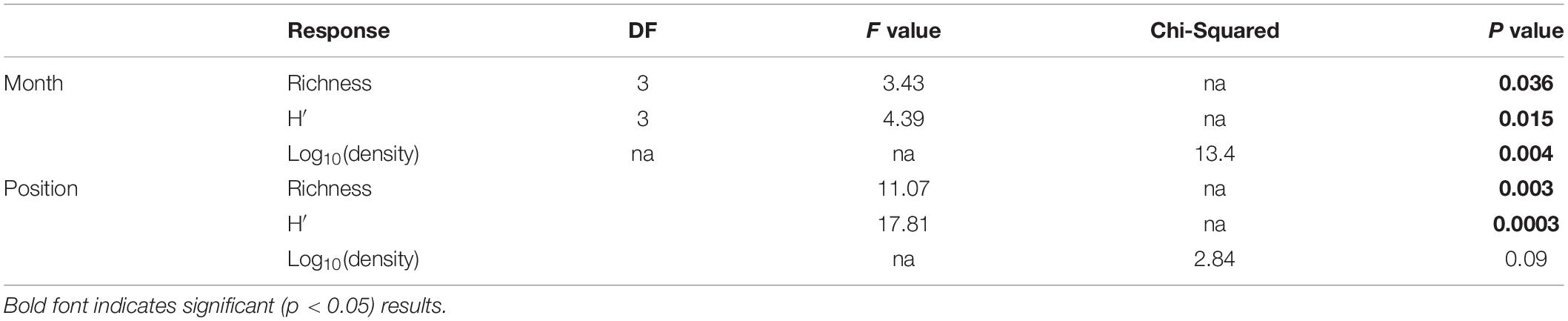
Table 1. Results of two-way ANOVAs (richness, H′) and Kruskal–Wallis test [log10(density)] against cruise and spatial position.
We found significant differences in the mesozooplankton community structure between sampling events (Table 2 and Figure 3). The post-hoc pairwise PERMANOVA, using a conservative Bonferroni corrected p-value, could not reveal any differences between the months. Raw p-values indicate differences between each month, except September and March (Table 2), but this should be interpreted carefully as Type I error is likely inflated. Closer inspection of the most abundant taxa revealed that all groups exhibited higher densities during the September cruise relative to other months, with calanoid copepods exhibiting an especially pronounced peak (Figure 4). Nearshore and shelf stations had significantly different mesozooplankton community structures (Df = 1, Sum Sq = 0.28, Pseudo-F = 6.15, R2 = 0.204, p < < 0.001) which was driven by ostracods (18%), calanoid copepods, cyclopoid copepods, salps, chaetognaths, and appendicularians (Figure 4). Combined, these six taxa accounted for over half the spatial variation in community structure (55%).
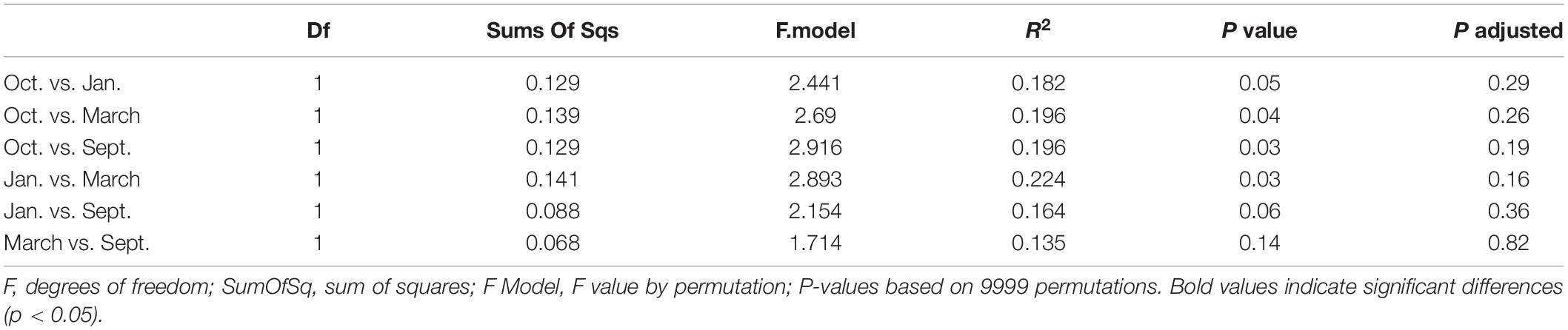
Table 2. Pairwise-PERMANOVA results based on Bray-Curtis dissimilarity on Hellinger standardized densities of zooplankton community structure in relation to cruise.
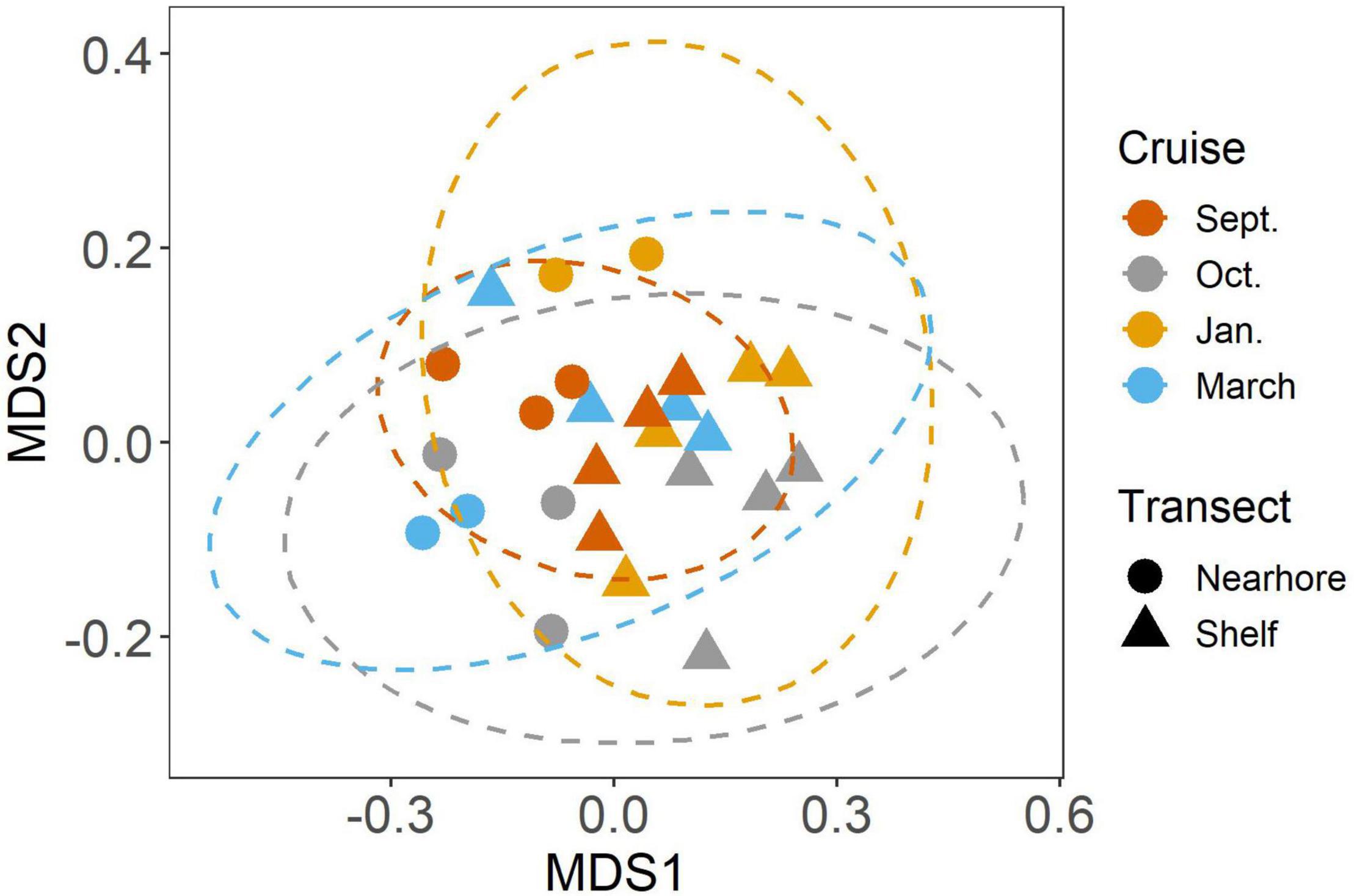
Figure 3. NMDS analysis of mesozooplankton community structure. Colors indicate cruise month. Ellipses represent 95% CI (Solid lines = significant differences in community structure from PERMANOVA, p < 0.05).
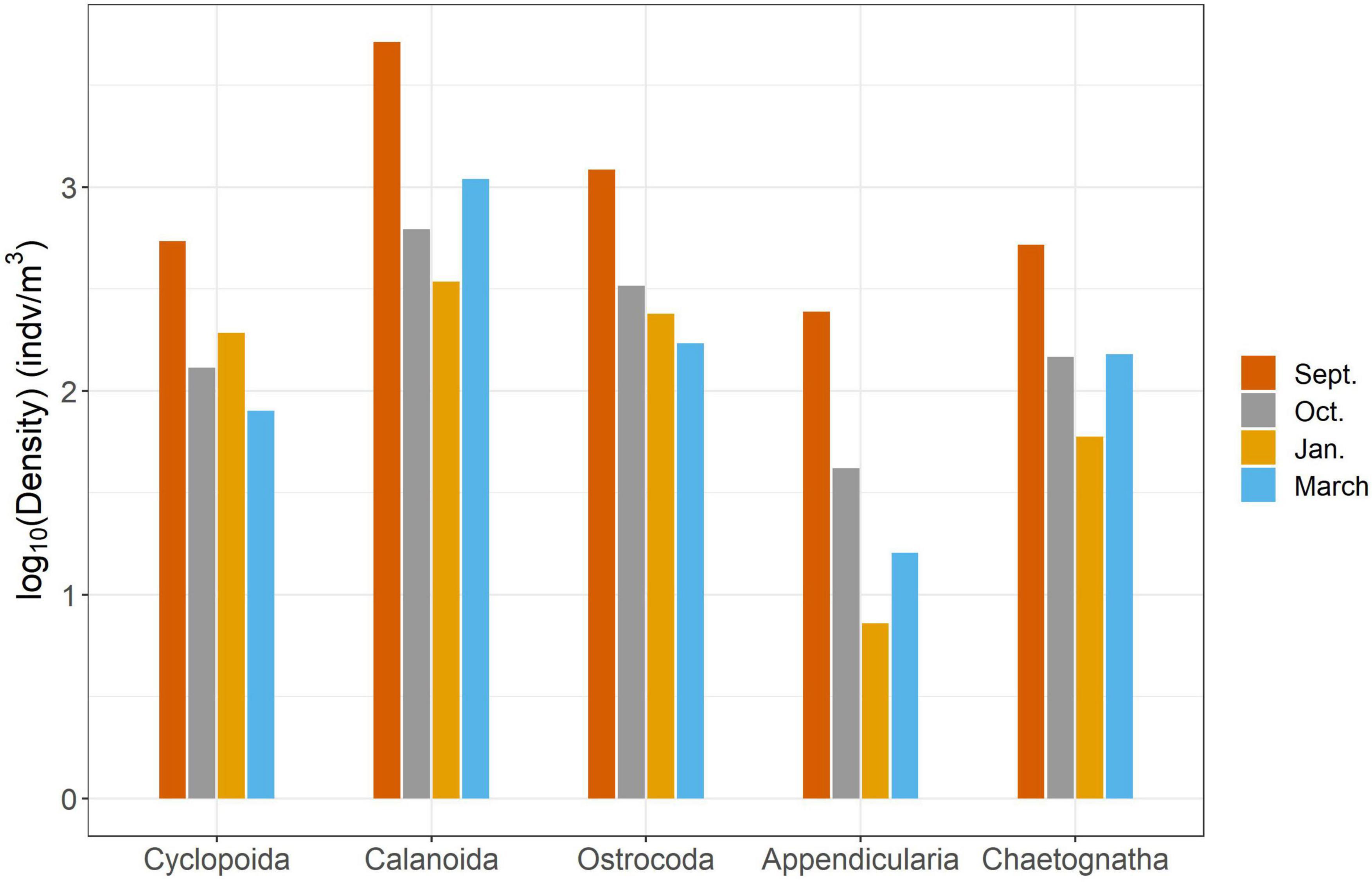
Figure 4. Results of SIMPER analysis between cruises. Top five highest contributing taxa to structural differences were chosen to explain over 50% of the variance.
The BIOENV analysis revealed that mesozooplankton community structure was related to mean salinity, dissolved oxygen, and transmission (correlation = 0.261, Table 3).
Discussion
In this study, we investigated changes in mesozooplankton community structure over 6 months in a previously understudied region of the nGOM. September was characterized by significantly higher mesozooplankton abundances compared to other months (Figure 2A). Taxa richness was not found to vary over the 6 months (Figure 2B). Taxa richness and diversity were found to have higher values at shelf stations compared to nearshore stations (Supplementary Figure S2). Diversity showed a hump shaped pattern over the 6 months sampled, with the highest diversity peaking in October 2017 (Figure 2C). When investigating community structure, we found that only September 2017 and March 2018 were significantly different, driven by the high abundance of cyclopoid copepods during September (Figures 3, 4). Mesozooplankton community structure was shown to be related to both temperature and salinity (Table 3). These findings will inform our understanding of prey availability for larval fish as late summer and spring are key spawning times in the northwestern GOM for recreationally and ecologically important fishes, including Sciaenidae (drums and croakers, and spotted sea trout) as well as Gobiidae (S. Geist, pers. comm).
Abundance and Diversity Patterns in the nGOM
The spatial and temporal patterns we describe for the LATEX shelf mesozooplankton communities corroborate well with other studies performed in the nGOM (Ortner et al., 1989; Ditty et al., 2004; Dubickas, 2019). Both Ditty et al. (2004) and Liu et al. (2017) found higher abundances of zooplankton during “summer” sampling events compared to “spring” or “fall” across the nGOM. It is unknown how our January communities compare to other areas of the nGOM, as few studies have reported sampling during this time of year. The spatial patterns of zooplankton community reported in this study (high abundance, lower diversity nearshore) are consistent with studies performed throughout the nGOM (Ortner et al., 1989; Carassou et al., 2014; Dzwonkowski et al., 2017). The range of diversities we report are similar to those presented in Ortner et al. (1989). Both salinity and temperature are frequently reported as drivers of mesozooplankton community structure elsewhere in the nGOM (Elliott et al., 2012; Greer et al., 2016; Liu et al., 2017). However, unlike areas of heavy river influence (e.g., MS Bight), dissolved oxygen did not appear to play as significant a role in driving zooplankton communities on the LATEX shelf (Kimmel et al., 2010; Elliott et al., 2012; Roman et al., 2012).
While temporal and spatial patterns of mesozooplankton community structure in our sampling area of the LATEX shelf match well with other areas of the nGOM, there are large discrepancies regarding total mesozooplankton abundance. Reported abundances for the nGOM have been shown to have large spatial and temporal variability, making it difficult to make broad generalities across the region (Carassou et al., 2014; Dubickas, 2019). Abundance, measured in volume standardized counts or biovolume, were lower than some of the abundances reported for the Louisiana shelf (Table 4; Kimmel et al., 2010; Elliott et al., 2012; Roman et al., 2012). Specifically, our abundances were an order of magnitude smaller compared to the values reported in Elliott et al. (2012) and Roman et al. (2012). This extreme difference in abundance could be attributed to differences in sampling gear and environmental conditions as both these studies used smaller mesh sizes (202 μm) and incorporated areas of high Mississippi River influence. Kimmel et al. (2010) reported twice as much zooplankton for their nearshore stations, but their offshore values were comparable. September and October zooplankton abundances from this study were found to similar to those reported for the continental shelf near Alabama, Louisiana, and across the nGOM for similar months (Ditty et al., 2004; Kimmel et al., 2010; Di Mauro et al., 2017; Dzwonkowski et al., 2017). When we compare our abundances to Galveston Bay, we find that nearshore abundances can be up to ten times greater than what is found at the mouth of the bay (Liu et al., 2017). Overall, the zooplankton abundances reported over the 6 months sampled fell well within the range presented for the nGOM. While there is extreme variation in zooplankton abundance across the entire nGOM, the conservation of spatial and seasonal patterns suggests that local mechanisms regulate the magnitude of zooplankton abundance (e.g., productivity; Dagg and Whitledge, 1991; Dagg and Breed, 2003). Because a rigorous comparison of mesozooplankton abundance across the nGOM is beyond the scope of this study, we suggest that further investigation of community structure and potential environmental drivers over a greater spatial scale could provide insight on the variation in local importance of community governing mechanisms.
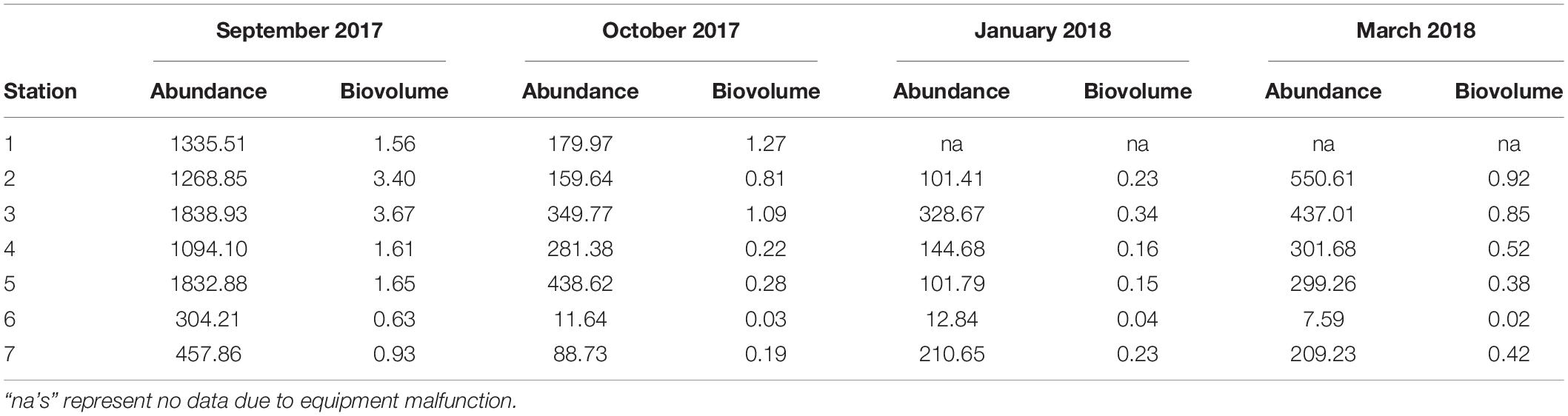
Table 4. Abundance (indv m–3) and biovolume (mL m–3) of mesozooplankton collected at each station over the 6 months following Hurricane Harvey on LATEX shelf near Galveston Bay.
Food Web Implications
Changes in mesozooplankton community structure has broad implications for energy transfer to higher order consumers such as fish and seabirds. Recruitment success of major fishery species can also be impacted by the suitability of prey items within zooplanktonic communities. Late fall is the spawning time for Scienidae fishes in nearshore waters of the northwestern GOM. Diet analysis of these larvae, collected simultaneously with our mesozooplankton in September nearshore, revealed the importance of calanoid and cyclopoid copepods and chaetognaths as prey (McAskill et al., in prep). Because of the tight coupling between marine copepod production and temperature (Huntley and Lopez, 1992), regional, long-term increases in sea surface temperature in both nGOM nearshore (1°C per decade) (Fodrie et al., 2010) and oceanic waters (+0.17°C to +0.3°C per decade) (Muller-Karger et al., 2015) may induce phenological shifts that result in a mismatch with these larval fishes. These warming trends may also accelerate the seasonal production of plankton predators like ctenophores and scyphozoans that selectivity feed on small copepods (e.g., Cyclopoida) as well as larval fish themselves during late fall (Purcell, 1985; Graham and Kroutil, 2001; Shiplett, 2011; Robinson and Graham, 2013, Robinson and Graham, 2014). It is important to also note that mesozooplankton do not only modify food web production as food but also via direct competition for resources or through predation (Robinson et al., 2015).
Zooplankton compositional changes can dictate food quality for adult-stage fish that are valuable Gulf of Mexico fisheries (e.g., Gulf menhaden, Brevoortia sp.). These changes in community structure can occur via direct consumption of the adults or through recruitment success of larval fish. Heneghan et al. (2016) quantitatively showed that shifts in zooplankton community structure from specialist herbivores to generalist carnivores, impacts forage fish production, abundance, and the overall stability of the food web against perturbations. There was a distinct change in zooplankton assemblage over the 6 months we sampled (Figure 4). Smaller cyclopoid copepods dominated the composition of the September cruise compared to the later March communities (Figure 5). It has been shown that shifts toward smaller dominant copepods can have an important role in the organization of higher trophic levels such as forage fish (anchovies to sardines; Alheit and Niquen, 2004, jack mackerel to redbait; McLeod et al., 2012). These shifts in dominant forage fish taxa are driven by changes in nutritional quality of their prey (Brett et al., 2009). As their carbon sources are derived more from detrital pathways, small copepods and other small zooplankton typically have less carbon compared to larger copepods and zooplankton (Bouley and Kimmerer, 2006). This results in lowered nutritional benefit for consumers (Brett et al., 2009) and altered dominance patterns in planktivorous fishes (Winder and Jassby, 2011).
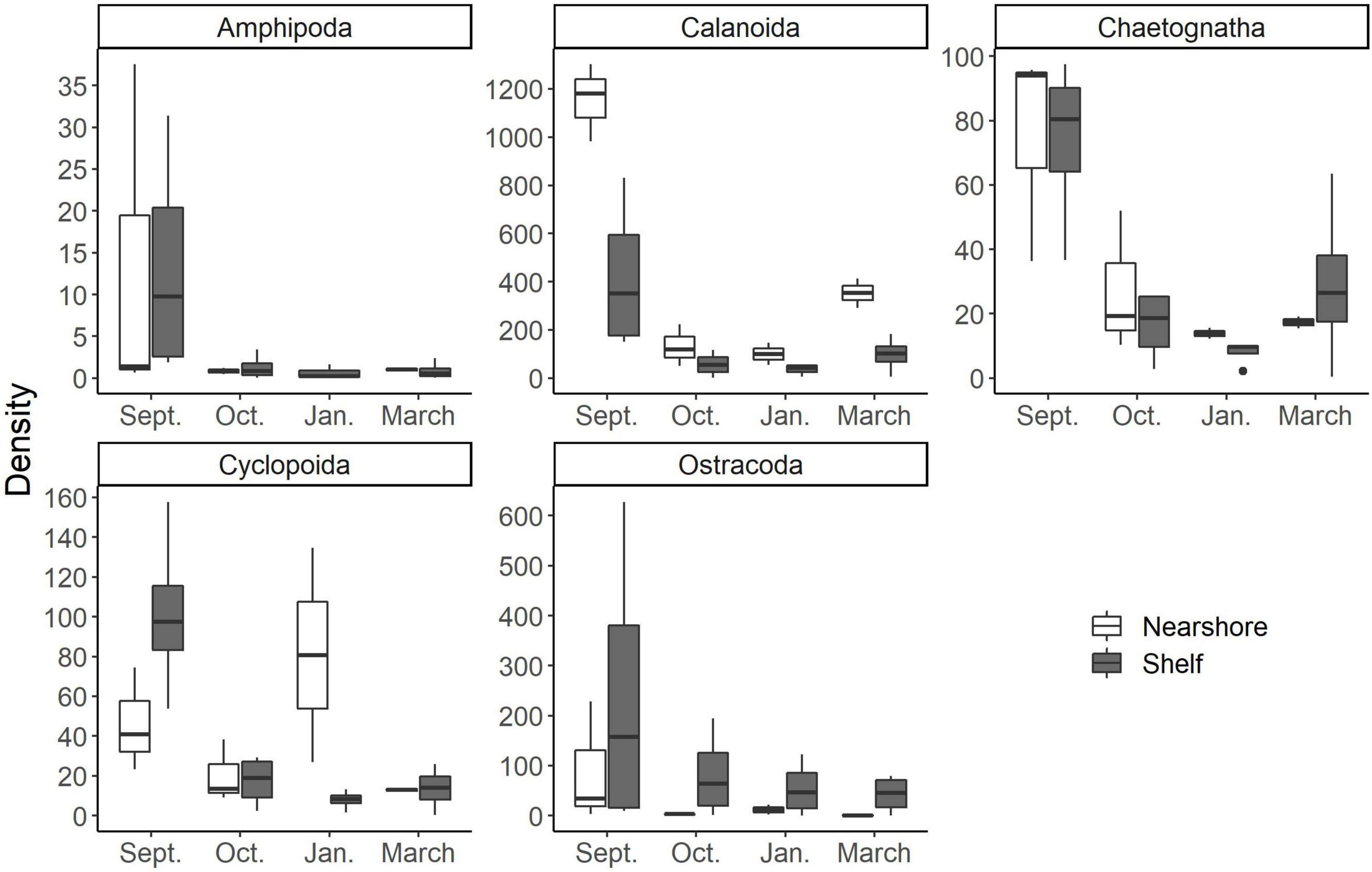
Figure 5. Common mesozooplankton taxa found in larval fish gut contents at the study area according to McAskill et al. (in prep). Note differences in scale of the y axis (density; indv m–3). Nearshore stations are white and shelf stations are gray.
Examples of zooplankton compositional changes in the Gulf of Mexico have also been explored using ecosystem modeling. Manipulated shifts in GOM zooplankton communities toward gelatinous dominated communities was shown to severely reduced trophic transfer efficiency and overall biomass production of higher trophic levels such as pelagic sportfish and birds (Robinson et al., 2015). In systems such as the nGOM where planktivores such as gulf menhaden are a major energy pathway to higher trophic levels, understanding how compositional changes of lower trophic levels like mesozooplankton is critical for understanding how our coastal food webs could be impacted by disturbances.
Limitations
Understanding interannual variability is important in order to contextualize our abundance and biovolume data for this area of the nGOM. This leaves a wide avenue for consolidating and exploring what constitutes a “normal” zooplankton community structure for this area of the LATEX shelf. Further comparison of this study’s zooplankton communities to historic samples will provide essential context for our results. Another limitation of this study is our interpretation of community patterns at a coarse taxonomic resolution. While our taxonomic degradation analysis did not distinguish any significant differences between our results when resolution was mixed, spanning family to class, a more refined resolution may highlight specific taxa within groups that are driving our observed patterns of mesozooplankton community change.
Broad Implications and Summary
Investigating patterns in mesozooplankton community structure over space and time is critical for understanding how pelagic food webs will react in a world of change. Understanding drivers of these compositional changes is especially pertinent in a climate regime where disturbances, like that from hurricanes, are projected to increase in frequency and intensity (Risser and Wehner, 2017). For an area such as the nGOM which heavily relies on forage fish production, knowledge of how these storms may impact coastal food webs and larval fish survival is crucial for proper analysis of management techniques and policy. To our knowledge, this paper described the first spatial and temporal patterns in mesozooplankton community structure on the LATEX shelf off Galveston, TX. We observed strong seasonal differences in abundance and unimodal patterns in diversity over the 6-months sampling period driven by changes in temperature and salinity. Peak abundance of important larval fish prey items varied in both in space and time, which may have control over recruitment success.
Data Availability Statement
The datasets generated for this study are available on request to the corresponding author.
Author Contributions
KR and ZT conceptualized the study, wrote the manuscript, and collected the samples. AT processed and identified the samples. ZT ran the analysis. All authors contributed to the article and approved the submitted version.
Funding
This project was funded by NSF RAPID grant number 1760704.
Conflict of Interest
The authors declare that the research was conducted in the absence of any commercial or financial relationships that could be construed as a potential conflict of interest.
Acknowledgments
We would like to thank the crew of the R/V Point Sur and R/V Pelican for assistance in sample collection. We would also like to thank Simon Geist and the crew of the R/V Gordon Gunter for their September zooplankton sampling effort. Additional thanks are directed toward the Stauffer lab for their processing of the CTD data. Finally, an enormous amount of gratitude is imparted to the undergraduate workers, including Aidyn Creson and Sara Moran, who helped process samples and sort images.
Supplementary Material
The Supplementary Material for this article can be found online at: https://www.frontiersin.org/articles/10.3389/fmars.2020.00462/full#supplementary-material
Footnotes
References
Alheit, J., and Niquen, M. (2004). Regime shifts in the humboldt current ecosystem. Prog. Oceanogr. 60, 201–222. doi: 10.1016/j.pocean.2004.02.006
Benjamini, Y., and Hochberg, Y. (1995). Controlling the false discovery rate: a practical and powerful approach to multiple testing. J. R. Stat. Soc. Ser. B 57, 289–300. doi: 10.1111/j.2517-6161.1995.tb02031.x
Bouley, P., and Kimmerer, W. J. (2006). Ecology of a highly abundant, introduced cyclopoid copepod in a temperate estuary. Mar. Ecol. Prog. Ser. 324, 219–228. doi: 10.3354/meps324219
Brett, M. T., Kainz, M. J., Taipalea, S. M., and Seshana, H. (2009). Phytoplankton, not allochthonous carbon, sustains herbivorous zooplankton production. Proc. Natl. Acad. Sci. U.S.A. 106, 21197–21201. doi: 10.1073/pnas.0904129106
Carassou, L., Hernandez, F. J., and Graham, W. M. (2014). Change and recovery of coastal mesozooplankton community structure during the Deepwater Horizon oil spill. Environ. Res. Lett. 9:124003. doi: 10.1088/1748-9326/9/12/124003
Cavole, L. M., Demko, A. M., Diner, R. E., Giddings, A., Koester, I., Pagniello, C. M., et al. (2016). Biological impacts of the 2013–2015 warm-water anomaly in the Northeast Pacific: winners, losers, and the future. Oceanography 29, 273–285.
Clarke, K. R., and Ainsworth, M. (1993). A method of linking multivariate community structure to environmental variables. Mar. Ecol. Prog. Ser. 92, 205–205.
Cochrane, J. D., and Kelly, F. J. (1986). Low-frequency circulation on the Texas-Louisiana continental shelf. J. Geophys. Res. 91, 10645–10659.
Costello, J. H., Sullivan, B. K., and Gifford, D. J. (2006). A physical–biological interaction underlying variable phenological responses to climate change by coastal zooplankton. J. Plankton Res. 28, 1099–1105. doi: 10.1093/plankt/fbl042
Criales, M. M., Shiplett, R. M., Thompson, N., and Kulpa, K. A. (2017). Abundance and distribution of planktonic decapods in the northern Gulf of Mexico before and after the Deepwater Horizon oil spill. Bull. Mar. Sci. 93, 829–856. doi: 10.5343/bms.2016.1050
Cushing, D. H. (1990). Plankton production and year-class strength in fish populations: an update of the match/mismatch hypothesis. Adv. Mar. Biol. 26, 249–293. doi: 10.1016/s0065-2881(08)60202-3
Dagg, M. J., and Breed, G. A. (2003). Biological effects of Mississippi river nitrogen on the northern Gulf of Mexico–a review and synthesis. J. Mar. Syst. 43, 133–152. doi: 10.1016/j.jmarsys.2003.09.002
Dagg, M. J., and Whitledge, T. E. (1991). Concentrations of copepod nauplii associated with the nutrient-rich plume of the Mississippi River. Cont. Shelf Res. 11, 1409–1423. doi: 10.1016/0278-4343(91)90043-6
Di Mauro, R., Kupchick, M. J., and Benfield, M. C. (2017). Abundant plankton-sized microplastic particles in shelf waters of the northern Gulf of Mexico. Environ. Pollut. 230, 798–809. doi: 10.1016/j.envpol.2017.07.030
Ditty, J. G., Shaw, R. F., and Cope, J. S. (2004). Distribution of carangid larvae (Teleostei: Carangidae) and concentrations of zooplankton in the northern Gulf of Mexico, with illustrations of early Hemicaranx amblyrhynchus and Caranx spp. larvae. Mar. Biol. 145, 1001–1014. doi: 10.1007/s00227-004-1381-z
D’Sa, E. J., Joshi, I. D., Liu, B., Ko, D. S., Osburn, C., and Bianchi, T. S. (2019). Biogeochemical response of apalachicola bay and the shelf waters to hurricane michael using ocean color semi-analytic/inversion and hydrodynamic models. Front. Mar. Sci. 6:523. doi: 10.3389/fmars.2019.00523
Du, J., Park, K., Shen, J., Zhang, Y. J., Yu, X., Ye, F., et al. (2019). A hydrodynamic model for Galveston Bay and the shelf inthe northern Gulf of Mexico. Ocean Sci. 15, 951–966. doi: 10.5194/os-15-951-2019
Dubickas, K. M. (2019). Zooplankton Community Structure in the NE Gulf of Mexico: Impacts of Environmental Variability and the Deepwater Horizon Oil Spill. Ph.D. thesis, University of South Florida, Tampa, FL.
Dzwonkowski, B., Greer, A. T., Briseño-Avena, C., Krause, J. W., Soto, I. M., Hernandez, F. J., et al. (2017). Estuarine influence on biogeochemical properties of the Alabama shelf during the fall season. Cont. Shelf Res. 140, 96–109. doi: 10.1016/j.csr.2017.05.001
Elliott, D. T., Pierson, J. J., and Roman, M. R. (2012). Relationship between environmental conditions and zooplankton community structure during summer hypoxia in the northern Gulf of Mexico. J. Plankton Res. 34, 602–613. doi: 10.1093/plankt/fbs029
Fodrie, F. J., Heck, K. L., Powers, S. P., Graham, W. M., and Robinson, K. L. (2010). Climate-related, decadal-scale assemblage changes of seagrass-associated fishes in the northern Gulf of Mexico. Glob. Change Biol. 16, 48–59. doi: 10.1111/j.1365-2486.2009.01889.x
Frederiksen, M., Edwards, M., Richardson, A. J., Halliday, N. C., and Wanless, S. (2006). From plankton to top predators: bottom−up control of a marine food web across four trophic levels. J. Anim. Ecol. 75, 1259–1268. doi: 10.1111/j.1365-2656.2006.01148.x
Gomez, F. A., Lee, S., Hernandez, F. J., Chiaverano, L. M., Muller-Karger, F. E., Liu, Y., et al. (2019). ENSO-induced co-variability of salinity, plankton biomass and coastal currents in the Northern Gulf of Mexico. Sci. Rep. 9:178. doi: 10.1038/s41598-018-36655-y
Gorsky, G., Ohman, M. D., Picheral, M., Gasparini, S., Stemmann, L., Romagnan, J. B., et al. (2010). Digital zooplankton image analysis using the ZooScan integrated system. J. Plankton Res. 32, 285–303. doi: 10.1093/plankt/fbp124
Govoni, J. J., Hoss, D. E., and Chester, A. J. (1983). Comparative feeding of three species of larval fishes in the northern Gulf of Mexico: Brevoortia patronus, Leiostomus xanthurus, and Micropogonias undulatus. Mar. Ecol. Prog. Ser. 13, 189–199. doi: 10.3354/meps013189
Graham, W. M., and Kroutil, R. M. (2001). Size-based prey selectivity and dietary shifts in the jellyfish, Aurelia aurita. J. Plankton Res. 23, 67–74. doi: 10.1093/plankt/23.1.67
Greer, A. T., Briseño-Avena, C., Deary, A. L., Cowen, R. K., Hernandez, F. J., and Graham, W. M. (2017). Associations between lobster phyllosoma and gelatinous zooplankton in relation to oceanographic properties in the northern Gulf of Mexico. Fish. Oceanogr. 26, 693–704. doi: 10.1111/fog.12228
Greer, A. T., Woodson, C. B., Smith, C. E., Guigand, C. M., and Cowen, R. K. (2016). Examining mesozooplankton patch structure and its implications for trophic interactions in the northern Gulf of Mexico. J. Plankton Res. 38, 1115–1134. doi: 10.1093/plankt/fbw033
Gunter, G. (1963). The fertile fisheries crescent. Mississippi academy of sciences journal 9: 286-290. 1967. Some relationships of estuaries to the fisheries of the Gulf of Mexico. Estuaries Am. Assoc. Adv. Sci. 83, 621–638.
Heneghan, R. F., Everett, J. D., Blanchard, J. L., and Richardson, A. J. (2016). Zooplankton are not fish: improving zooplankton realism in size-spectrum models mediates energy transfer in food webs. Front. Mar. Sci. 3:201. doi: 10.3389/fmars.2016.00201
Hoover, A. (2018). Influence of Natural and Anthropogenic Environmental Variability on Larval Fish Diet, Growth, and Condition in the Northcentral Gulf of Mexico. Ph.D. thesis, University of Southern Mississippi, Hattiesburg, MS.
Huntley, M. E., and Lopez, M. D. G. (1992). Temperature-dependent production of marine copepods: a global synthesis. Am. Nat. 140, 202–242.
Johnson, D. R. (2008). Ocean Surface Current Climatology in the Northern Gulf of Mexico. Ocean Springs, MS: Gulf Coast Research Laboratory.
Karnauskas, M., Kelble, C. R., Regan, S., Quenée, C., Allee, R., Jepson, M., et al. (2017). Ecosystem Status Report Update for The Gulf of Mexico. Technical Report no. NOAA Technical Memorandum NMFS-SEFSC, 706. Silver Spring, MD: National Oceanic and Atmospheric Administration, 51.
Keithly, W. R., and Roberts, K. J. (2017). “Commercial and recreational fisheries of the Gulf of Mexico,” in Habitats and Biota of the Gulf of Mexico: Before the Deepwater Horizon Oil Spill, ed. C. Ward (New York, NY: Springer), 1039–1188. doi: 10.1007/978-1-4939-3456-0_2
Kimmel, D. G., Boicourt, W. C., Pierson, J. J., Roman, M. R., and Zhang, X. (2010). The vertical distribution and diel variability of mesozooplankton biomass, abundance and size in response to hypoxia in the northern Gulf of Mexico USA. J. Plankton Res. 32, 1185–1202. doi: 10.1093/plankt/fbp136
Leising, A. W., Schroeder, I. D., Bograd, S. J., Abell, J., Durazo, R., Gaxiola-Castro, G., et al. (2015). State of the California current 2014-15: impacts of the Warm-Water “Blob”. Cal. Coop. Ocean. Fish. Invest. Rep. 56, 31–68.
Liu, B., D’Sa, E. J., and Joshi, I. D. (2019). Floodwater impact on Galveston Bay phytoplankton taxonomy, pigment composition and photo-physiological state following Hurricane Harvey from field and ocean color (Sentinel-3A OLCI) observations. Biogeosciences 16:1975. doi: 10.5194/bg-16-1975-2019
Liu, H., Zhang, X., Yang, Q., Zuo, T., and Quigg, A. (2017). Mesozooplankton dynamics in relation to environmental factors and juvenile fish in a subtropical estuary of the Gulf of Mexico. J. Coast. Res. 33, 1038–1050. doi: 10.2112/jcoastres-d-16-00155.1
Martinez Arbizu, P. (2019). pairwiseAdonis: Pairwise Multilevel Comparison Using Adonis. R Package Version 0.3.
McLeod, D. J., Hobday, A. J., Lyle, J. M., and Welsford, D. C. (2012). A prey-related shift in the abundance of small pelagic fish in eastern Tasmania? ICES J. Mar. Sci. 69, 953–960. doi: 10.1093/icesjms/fss069
Muller-Karger, F. E., Smith, J. P., Werner, S., Chen, R., Roffer, M., Liu, Y., et al. (2015). Natural variability of surface oceanographic conditions in the offshore Gulf of Mexico. Prog. Oceanogr. 134, 54–76. doi: 10.1016/j.pocean.2014.12.007
Murawski, S., Fleeger, J. E., Patterson, W. F. III, Hu, C., Daly, K. L., Romero, I. C., et al. (2016). How did the Deepwater Horizon oil spill affect coastal and continental shelf ecosystems of the Gulf of Mexico? Oceanography 29, 160–173. doi: 10.5670/oceanog.2016.80
Nielsen, T., and Andersen, C. (2002). Plankton community structure and production along a freshwater-influenced Norwegian fjord system. Mar. Biol. 141, 707–724. doi: 10.1007/s00227-002-0868-8
Nip, T. H., Ho, W. Y., and Wong, C. K. (2003). Feeding ecology of larval and juvenile black seabream (Acanthopagrus schlegeli) and Japanese seaperch (Lateolabrax japonicus) in Tolo Harbour, Hong Kong. Environ. Biol. Fish. 66, 197–209.
Nowlin, W. D., Jochens, A. E., DiMarco, S. F., Reid, R. O., and Howard, M. K. (2005). Low-frequency circulation over the Texas-Louisiana continental shelf. Geophys. Monogr. Am. Geophys. Union 161:219. doi: 10.1029/161gm17
Oksanen, J., Blanchet, F. G., Friendly, M., Kindt, R., Legendre, P., McGlinn, D., et al. (2019). vegan: Community Ecology Package. R Package Version 2.5-5. Available online at: https://CRAN.R-project.org/package=vegan (accessed September 1, 2019).
Ortner, P. B., Hill, L. C., and Cummings, S. R. (1989). Zooplankton community structure and copepod species composition in the northern Gulf of Mexico. Cont. Shelf Res. 9, 387–402. doi: 10.1016/0278-4343(89)90040-x
Parra, S. M., Greer, A. T., Book, J. W., Deary, A. L., Soto, I. M., Culpepper, C., et al. (2019). Acoustic detection of zooplankton diel vertical migration behaviors on the northern Gulf of Mexico shelf. Limnol. Oceanogr. 64, 2092–2113. doi: 10.1002/lno.11171
Purcell, J. E. (1985). Predation on fish eggs and larvae by pelagic cnidarians and ctenophores. Bull. Mar. Sci. 37, 739–755.
Quintana, X. D., Comiín, F. A., and Moreno-Amich, R. (1998). Nutrient and plankton dynamics in a Mediterranean salt marsh dominated by incidents of flooding. Part 2: response of the zooplankton community to disturbances. J. Plankton Res. 20, 2109–2127. doi: 10.1093/plankt/20.11.2109
R Core Team (2019). R: A Language and Environment for Statistical Computing. Vienna: R Foundation for Statistical Computing.
Risser, M. D., and Wehner, M. F. (2017). Attributable human−induced changes in the likelihood and magnitude of the observed extreme precipitation during hurricane Harvey. Geophys. Res. Lett. 44, 12–457.
Robinson, K. L., and Graham, W. M. (2013). Long-term change in the abundances of northern Gulf of Mexico scyphomedusae Chysaora sp. and Aurelia spp., with link to climate variability. Limnol. Oceanogr. 58, 235–253. doi: 10.4319/lo.2013.58.1.0235
Robinson, K. L., and Graham, W. M. (2014). Warming of subtropical coastal waters accelerates Mnemiopsis leidyi growth and alters timing of spring ctenophore blooms. Mar. Ecol. Prog. Ser. 502, 105–115. doi: 10.3354/meps10739
Robinson, K. L., Ruzicka, J. J., Hernandez, F. J., Graham, W. M., Decker, M. B., Brodeur, R. D., et al. (2015). Evaluating energy flows through jellyfish and gulf menhaden (Brevoortia patronus) and the effects of fishing on the northern Gulf of Mexico ecosystem. ICES J. Mar. Sci. 72, 2301–2312. doi: 10.1093/icesjms/fsv088
Roman, M. R., Pierson, J. J., Kimmel, D. G., Boicourt, W. C., and Zhang, X. (2012). Impacts of hypoxia on zooplankton spatial distributions in the northern Gulf of Mexico. Estuaries Coasts 35, 1261–1269. doi: 10.1007/s12237-012-9531-x
Shiplett, R. (2011). Selective Feeding and Icthyoplankton Predation by Scyphomedusae in the Northern Gulf of Mexico, 124. M.Sc. thesis, University of South Alabama, Mobile, AL.
Spies, R. B., Senner, S., and Robbins, C. S. (2016). An overview of the Northern Gulf of Mexico ecosystem. Gulf Mexico Sci. 33:9.
Sullivan, B. K., Van Keuren, D., and Clancy, M. (2001). Timing and size of blooms of the ctenophore Mnemiopsis leidyi in relation to temperature in Narragansett Bay, RI. Hydrobiologia 451, 113–120. doi: 10.1023/A:1011848327684
Keywords: Gulf of Mexico, zooplankton, hurricane, tropical cyclone, flood, community, diversity, disturbance
Citation: Topor ZM, Robinson KL and Turcu A (2020) Investigating Seasonal Succession Patterns in Mesozooplankton Community Structure Following Hurricane Harvey. Front. Mar. Sci. 7:462. doi: 10.3389/fmars.2020.00462
Received: 13 January 2020; Accepted: 25 May 2020;
Published: 17 June 2020.
Edited by:
Xosé Anxelu G. Morán, King Abdullah University of Science and Technology, Saudi ArabiaReviewed by:
Ulisses Miranda Azeiteiro, University of Aveiro, PortugalLeocadio Blanco Bercial, Bermuda Institute of Ocean Sciences, Bermuda
Copyright © 2020 Topor, Robinson and Turcu. This is an open-access article distributed under the terms of the Creative Commons Attribution License (CC BY). The use, distribution or reproduction in other forums is permitted, provided the original author(s) and the copyright owner(s) are credited and that the original publication in this journal is cited, in accordance with accepted academic practice. No use, distribution or reproduction is permitted which does not comply with these terms.
*Correspondence: Zachary M. Topor, dG9wb3Iuem1AZ21haWwuY29t