- 1Department of Marine Biology, Texas A&M University Galveston Campus, Galveston, TX, United States
- 2Marine Sciences Institute, University of Texas at Austin, Port Aransas, TX, United States
- 3Department of Oceanography and Coastal Studies, Louisiana State University, Baton Rouge, LA, United States
The northern Gulf of Mexico has been an important source for crude oil and natural gas extraction since the 1930s. Thousands of fixed platforms and associated equipment have been installed on the Gulf of Mexico continental shelf, leading to a pervasive ‘ocean sprawl.’ After decommissioning, 100s of these structures have been converted to artificial reefs under the federal ‘Rigs-to-Reefs’ program, in addition to artificial reefs specifically designed to enhance fisheries and/or benefit the recreational diving industry. Apart from a few natural banks, which reach to approximately 55 ft below the surface, artificial reefs provide the only shallow-water hard substrate for benthic organisms in the deeper waters of the northern Gulf of Mexico. This vast expansion in available habitat has almost exclusively occurred over a relatively short span of time (∼50 years). The ecological interactions of artificial and natural reefs in the northern Gulf of Mexico are complex. Artificial reefs in general, and oil and gas structures in particular, have often been invoked as stepping stones for non-native and invasive species (e.g., Tubastrea cup corals, lionfish). The pilings are covered with fouling communities which remain largely unstudied. While the risks of these fouling organisms for invading natural reefs are being broadly discussed, other impacts on the ecological and economic health of the Gulf of Mexico, such as the potential to facilitate jellyfish blooms or increase the incidence of ciguatera fish poisoning, have received less attention. Artificial reefs also provide ecosystem services, particularly as habitat for economically important fish species like red snapper. Here we revisit the potential role of artificial reefs as ‘stepping stones’ for species invasions and for fisheries enhancement. Beyond concerns about ecological effects, some of these topics also raise public health concerns. We point out gaps in current knowledge and propose future research directions.
Introduction
The northern Gulf of Mexico (nGoM) is home to nearly 2,200 active oil and gas platforms (BSEE/BOEM Data Center, 2019). In addition, there are almost as many artificial structures not currently used for oil or gas extraction, including ‘reefed’ oil and gas platforms, submerged vessels, reef balls and others (Figure 1; Broughton, 2012). These-human made structures create so-called ‘ocean sprawl’ (Duarte et al., 2013; Firth et al., 2016), altering the habitat by creating hard substrate, forming barriers to movement for some organisms and changing predator-prey interactions (Bishop et al., 2017). In 2016, the total number of artificial reefs in the nGoM (including active and inactive) amounted to 4,176 (NOAA, 2016).
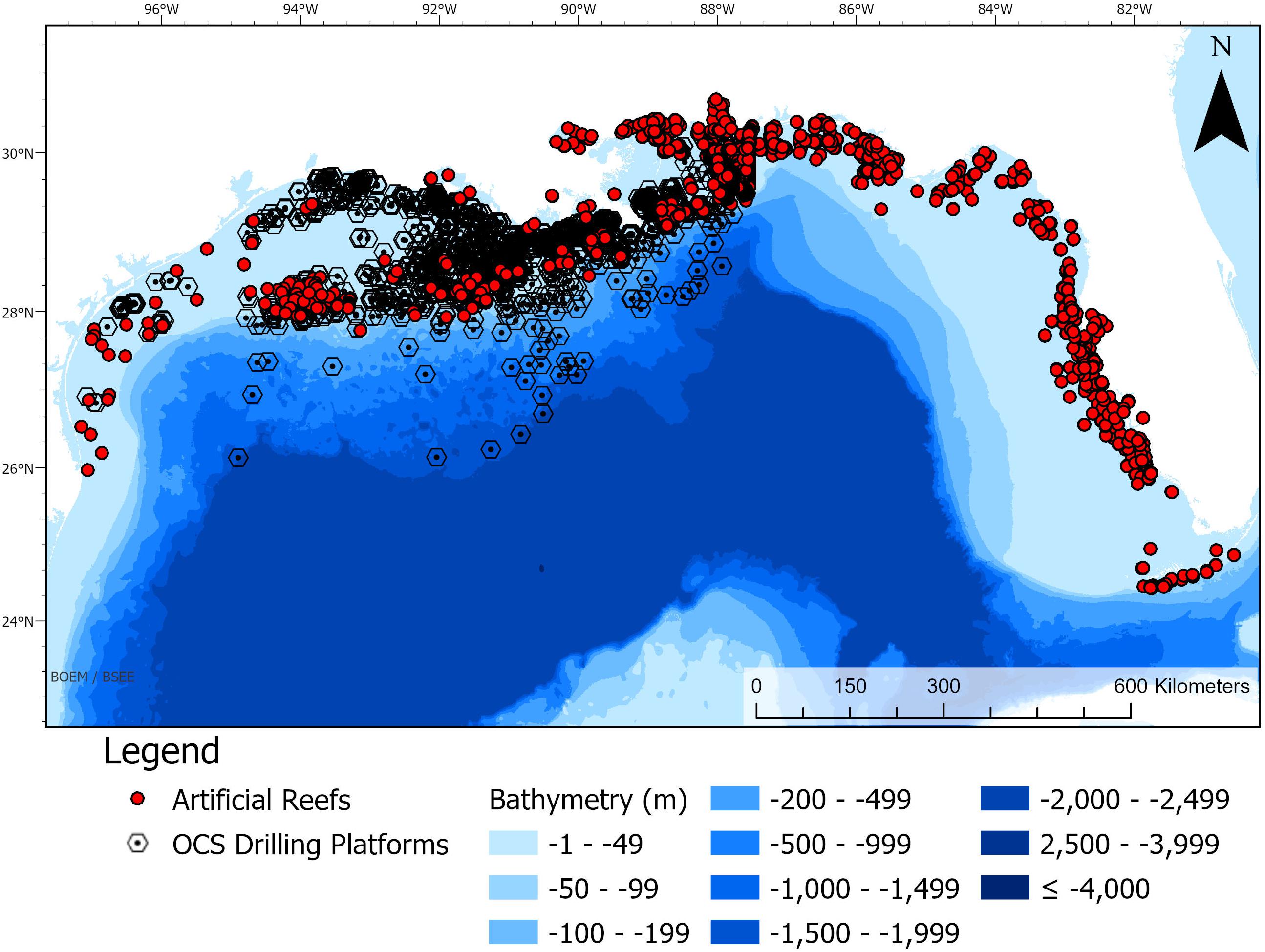
Figure 1. Total artificial reefs of nGoM (red) and outer continental shelf drilling platforms (black) shown with bathymetry in meters. Data from NOAA (2016) and BSEE/BOEM Data Center (2019).
Before oil and gas exploration, the seafloor was mostly sedimented, although the bathymetry of the continental slope is complex and frequently marked by domes, pockmarks, canyons, faults, and channels. The nGoM is additionally home to some of the best studied cold seep communities in the world (e.g., Fisher et al., 2007; Cordes et al., 2009) as well as coral banks and reefs. Known coral assemblages in the GoM range from phototrophic in the shallower portions to mesophotic and deep coral communities, down to more than 2,500 m in De Soto Canyon (Doughty et al., 2014). Shallower coral assemblages are often present on artificial structures (Bright et al., 1991; Sammarco et al., 2014a).
Near the edge of the continental slope off the coasts of Texas and Louisiana, multiple banks formed by underlying salt diapirs support natural reefs. The best known of these are the East and West Flower Garden Banks, which constitute the core of the Flower Garden Banks National Marine Sanctuary (FGBNMS). The Flower Garden Banks are not only the northernmost coral reefs of the greater Caribbean but also the most isolated and among the healthiest with regard to coral cover (Hickerson et al., 2012; Johnston et al., 2016a). Located within the sanctuary boundaries, High Island 389A (HI-389A) is a decommissioned platform installed in 1981 located on a 20-acre artificial reef site in a water depth of 410 feet (Figure 2). The structure has recently (July 2018) been reefed by removal of the top 65 ft below the water line. The FGBNMS further includes Stetson Bank, located closer to the shore. The FGBNMS Advisory Board recently voted to expand the sanctuary boundaries to include 14 additional banks. Approximately 150 platforms are located within 25 miles of the current sanctuary borders (US Department of Commerce, 2012). The proposed new boundaries as set out in the Sanctuary Expansion DEIS will incorporate three additional oil and gas production structures.
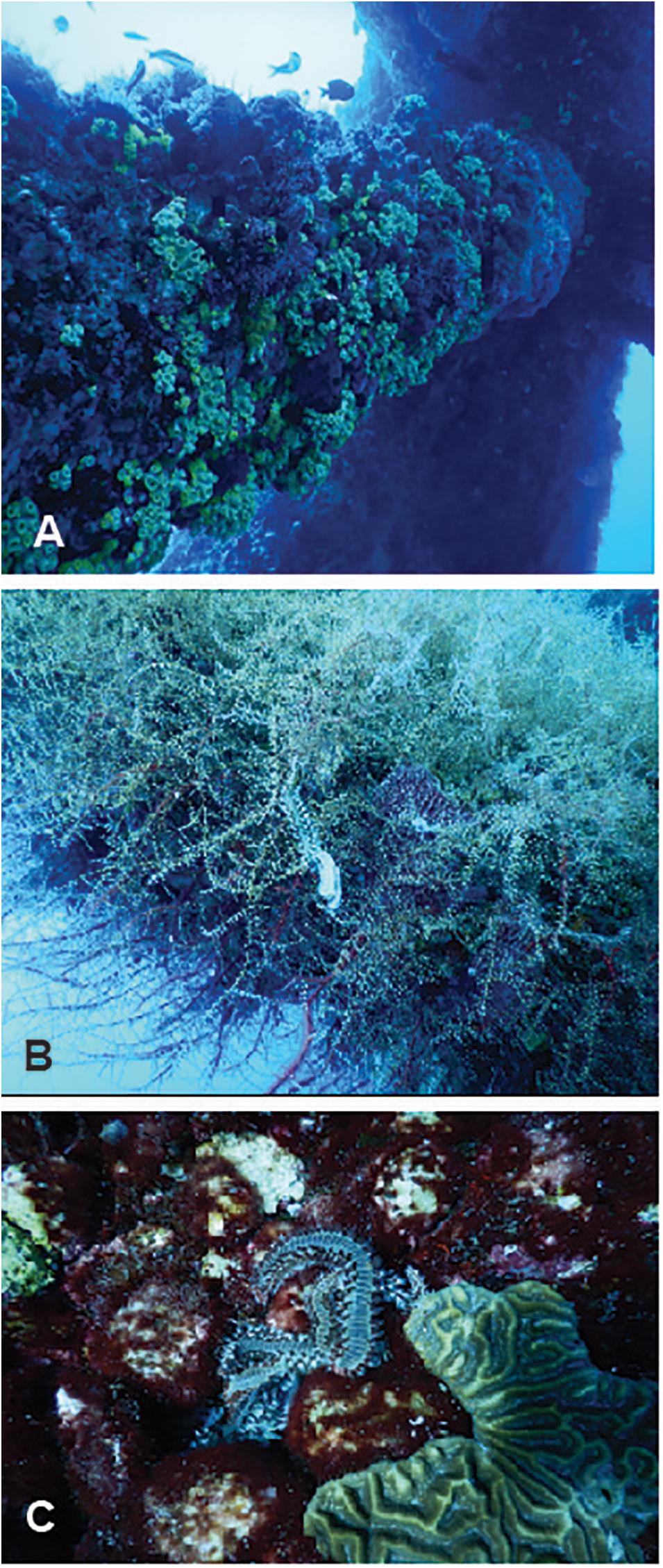
Figure 2. Examples of the fouling community and associated motile fauna on High Island 389A (HI-389A). (A) Numerous colonies of Tubastrea coccinea shown on the underside of a horizontal beam of at 37 m. (B) The hydroid, Thyroscyphus sp., with a bearded fireworm, Hermodice carunculata, on top on a diagonal structure support beam on HI389-A at 20 m. (C) Five bearded fireworms, H. carunculata, in close proximity to a scleractinian coral (Pseudodiploria strigosa) on a horizontal beam of HI389-A at 9 m.
Natural and artificial reefs are interspersed with each other in the nGoM. This network of habitable ‘islands’ separated by stretches of uninhabitable (or less preferred) ground, provides unprecedented opportunities for organismal movement between these two habitat types. In this context, the geographic isolation of the FGB is a double-edged sword. On the one hand, it may provide relative protection from some dangers that threaten other Caribbean reefs, such as coral pathogens, nearshore anthropogenic impacts, and invasive species. On the other hand, isolation may hinder recruitment and adult replacement, especially after cases of local coral mortality. Despite isolation, some invasive species, most notably lionfish, have become established at the FGB (Johnston et al., 2016b). Oil and gas structures and other artificial reefs are often regarded as ‘stepping stones’ for invasives (Fenner, 2001; Sammarco et al., 2004, 2012a). However, this stepping-stone theory is ecologically complex, as much of the fauna on platforms is a typical ‘fouling community’ as opposed to a true reef community (Page et al., 2010). For example, artificial structures are more often dominated by small, flexible hydroid colonies rather than reef building corals. However, some species overlap between the two habitat types does exist. Utilization of these different habitat types by organisms with different life histories and ecological characteristics has far-reaching implications for marine conservation as well as commercial and recreational fisheries.
Previous reviews on the ecological roles of artificial reefs either had a national (Broughton, 2012) or global (Bull and Love, 2019) scope. The purpose of this review is to focus on the nGoM and summarize our current state of knowledge of the habitat preferences of different organismal groups inhabiting artificial reefs as well as functional connectivity between artificial and natural reefs. We will discuss the implications of the existing knowledge for ecosystem health and society, and identify knowledge gaps and future research directions.
Functional connectivity is here defined as the movement of organisms or particles among different locations or habitats (Bishop et al., 2017). When focusing on populations of individual species, the term population connectivity is used; multiple connected populations form a metapopulation (Cowen and Spunaugle, 2009). We consider the area offshore from the coasts of Texas, Louisiana, Mississippi, Alabama and Florida between approximately longitude 97° and 81° W and latitude 24° and 30° N (Figure 1). This area mostly encompasses the coastal areas to the continental slope, with a maximum depth of about 3,000 m. The review does not cover the effects of platform installation, operation and removal on soft sediment benthic communities, or the toxicological impacts of accidental spills or produced water, as these aspects have been reviewed elsewhere (e.g., Broughton, 2012; Cordes et al., 2016).
Prior reviews on connectivity between natural and artificial reefs in the nGoM were generally focused on particular taxonomic groups, such as corals (e.g., Sammarco et al., 2004) or commercially important fish (e.g., Shipp and Bortone, 2009; Cowan et al., 2011). This review has a wider taxonomic scope and broader view of ecological implications, in particular highlighting emerging concerns for which we lack sufficient knowledge. In light of the continuing proliferation of artificial reefs in the region, ongoing fisheries trends and the recent detection of previously unreported species (e.g., Bennett et al., 2019; Figueroa et al., 2019), this review is timely and can serve as a baseline to evaluate future developments. It is our hope that the information provided will guide future research efforts and management decisions.
Connectivity: Stepping Stones, Metapopulations, Resilience, and Replacement
Connectivity and resilience in dispersal networks are generally correlated, as more densely connected habitats are more likely to avoid recruitment failures. The 1000s of artificial structures in the GoM expand the habitats for naturally occurring (Sammarco et al., 2004, 2012b; Kolian et al., 2017), invasive (Fenner and Banks, 2004; Sammarco et al., 2012b; Dahl and Patterson, 2014), and fouling communities in the region, and simultaneously increase the density of the dispersal networks of these communities.
The metapopulation effects of artificial structures have been investigated in other coastal oceans, and support the notion that these structures support benthic, epibenthic, and migratory communities in complex ways. Paxton et al. (2019) found that artificial reefs on the subtropical US Atlantic continental shelf disproportionately support highly mobile tropical planktivorous and piscivorous fishes when compared to natural reefs, and they expand the biogeographic ranges of those species into higher latitudes. In the North Sea, artificial structures can increase the resilience of threatened coral species, both within the connected communities on artificial structures, as well as in nearby naturally occurring communities (Henry et al., 2018). It is reasonable to assume that artificial structures in the GoM increase metapopulation resilience for a diverse set of species – including historical natives, native and non-native invaders, and those species unique to fouling communities.
However, artificial structures are inherently more ephemeral than most naturally occurring comparable habitats in the nGoM. These structures are routinely decommissioned and subsequently removed, or reefed. Even when reefed, artificial structures and associated communities may undergo physical degradation and/or community succession (Sammarco et al., 2014a), which suggests the populations found on artificial structures are dynamic in both space and time. Metapopulation resilience is sensitive not only to the density of the dispersal network, but also to the rates of colonization and extinction at individual patches (Hanski and Ovaskainen, 2000). Thus, the assumed density of connectivity among artificial structures must be weighed against these rates when assessing the persistence of metapopulations supported by artificial structures. To date, rates of colonization, extinction, and larval connectivity among artificial structures in the GoM are not well-understood; however, data are available on the rates of decommissioning of artificial structures, which could help to inform the persistence of these unique communities.
As noted elsewhere in this review and in previous studies, the artificial structure communities in the GoM are not identical to, and not entirely different from, adjacent natural habitats. The hypotheses are usually that, following local extinctions on natural habitats, recolonization may occur with larvae from nearby artificial structures (Sammarco et al., 2012b), or that fouling communities will not establish on naturally occurring hard substrates. Although invasives that occupy artificial structures are routinely found on the FGB (e.g., Tubastrea coccinea, Fenner and Banks, 2004), a possibility that has not been fully addressed to date is that invasive or competitive species that occupy artificial structures could supplant naturally occurring species on natural habitats following perturbation or local extinction. A specific example may be the potential for alternative stable states between coral and sponge communities (reviewed in Norström et al., 2009). Sponges of the genera Cliona and Chondrilla have been shown to supplant hard corals following coral mortality events and prevent the recolonization of coral. Sponge communities on artificial structures in the GoM can be diverse (Rützler et al., 2009), but the distributions of sponges in those genera on artificial structures is not currently known. Following a perturbation to the coral communities on the FGB, colonization from sponge communities on nearby artificial structures may be more likely than colonization of hard corals from reefs that are further away.
Fouling Communities
Fouling communities, defined as assemblages of sessile organisms and associated species with limited mobility growing on human-made structures, are vastly understudied on offshore artificial structures in the GoM. The term ‘fouling community’ has a negative connotation, implying that these organisms are unwanted and destructive. On vessel hulls, sessile organisms increase drag and thereby reduce vessel speed and fuel efficiency. On oil and gas platforms, fouling communities can greatly increase the weight of the structure, as well as the diameter and surface roughness of the platform members (Page et al., 2010) (Figure 2), affecting the hydrodynamic loading of the platform and interfering with visual inspection. However, fouling communities on platforms provide ecosystem services, as prey items and ecosystem engineers of complex habitat for other sessile and motile organisms (Daigle et al., 2013), including commercially important fish species. The establishment of fouling communities is largely driven by the relative concentrations of propagule stages present in the water column but varies depending on many physical characteristics, such as light, temperature, salinity, pressure, spatial orientation and current regimes, as well as the interactions between biotic and abiotic factors (Terlizzi and Faimali, 2010).
As the vast majority of oil and gas platforms worldwide are constructed in soft substrate, the fauna on the structure tends to be inherently different from the surrounding benthic fauna. However, even if platforms are erected in the vicinity of natural hard substrates, the fouling fauna is generally distinctive (Page et al., 2010). This may be a result, in part, of the structure reaching from the seafloor to the surface allowing for increased primary producer activity and bioaccumulation in a location where it would not otherwise be possible (Daigle et al., 2013). Regardless, artificial structures expand the habitat of distinct fouling communities into areas of both soft and hard substrates on the continental shelf of the GoM.
On oil and gas platforms, shallow fouling communities are generally dominated by a few taxa with hardened shells. In the GoM barnacles tend to dominate the nearshore structures, whereas bivalves are more prevalent on offshore structures, in addition to extensive growth of hydroids (Figures 2B, 3) (Lewbel et al., 1987; Bull and Kendall, 1994; Page et al., 2010).
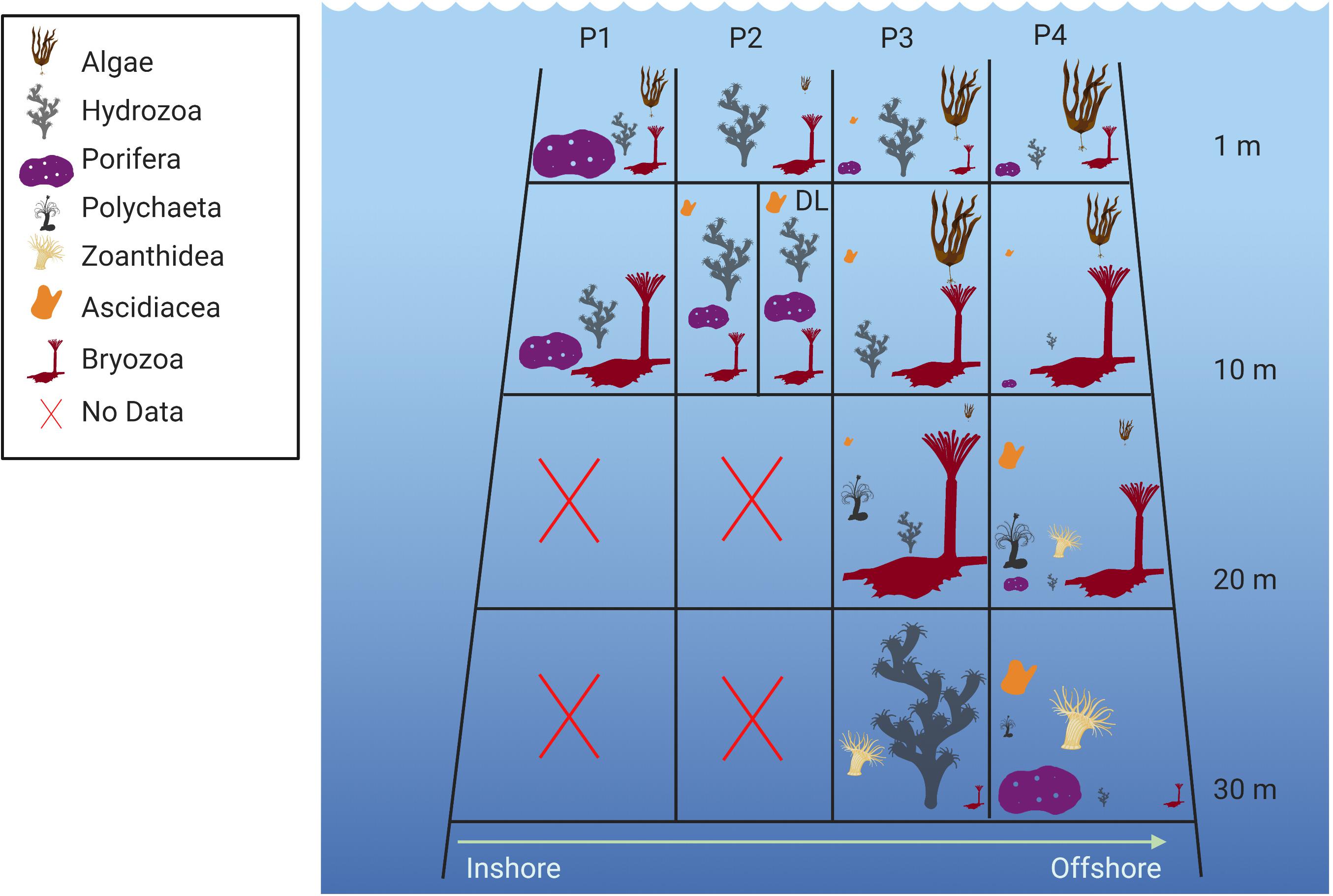
Figure 3. Zonation of fouling communities on four platforms (P1–P4) off the Louisiana coast from Lewbel et al. (1987). The size of the respective symbols represents the relative contribution of the taxon to the percent cover. The platforms were located in 12 to 46 m of water and fouling communities were sampled at 1 and 10 m depth at all platforms and additionally at 20 and 30 m depth at P3 and P4. At P2, an additional sampling station (P2-DL) was established above a produced water outlet. Note that scleractinian corals were not sampled on P1–P4 but were reported (but not quantitatively sampled) from other platforms in the area.
These structures can become colonized and integrated into fouling metacommunities fairly rapidly. Nearly 1 year after the installation of HI-389A, surveys were conducted from the surface to the 37 m horizontal supports and describe the establishment of fouling mats with hydroids and macroalgae (Boland, 2000). Bearded fireworms (Hermodice carunculata) and two species of sea urchin (Diadema antillarum and Arbacia punctulata) were present in noticeable amounts at this time (Boland, 2000). Hermatypic corals were also recorded on HI-389A in 1990, 9 years after installation, supporting the idea that these rigs provide settling surface for reef-builders (Boland, 2000).
Documented Invasions
There is an ongoing debate whether artificial reefs in the nGoM act as ‘stepping stones’ for non-indigenous or invasive species. In most cases, there is no definitive answer as to whether a non-indigenous species would be present in the area if there were no artificial reefs. Knowledge of a species’ life history and its behavior in other regions can provide some insight about the invasion history and may guide future management decisions. Here we review the evidence for a few non-indigenous taxa and their presence on natural and artificial structures in the nGoM.
Lionfish
Two species of Indo-Pacific lionfish, the red lionfish (Pterois volitans) and the devil firefish (Pterois miles) are established throughout the Caribbean, and their detrimental effects on native fauna are well documented (reviewed in Albins and Hixon, 2013; Côté et al., 2013). P. volitans and P. miles are morphologically similar and can only be distinguished by different numbers of dorsal and caudal spines, but their distinct genetic signatures confirm them as separate species, with P. volitans representing 93% of the sequenced specimens (Hamner et al., 2007). Genetic diversity within each species is low, indicating single, rapid invasions (Hamner et al., 2007).
Lionfish were first sighted in the nGoM in 2010 (Nuttall et al., 2014). By 2013, their numbers had increased exponentially on both natural and artificial reefs, but their densities on artificial reefs were two orders of magnitude higher and they exhibited a more varied diet than on natural reefs (Dahl and Patterson, 2014). Their rapid growth rates suggest that biomass is increasing even more rapidly than the numbers of individuals (Dahl and Patterson, 2014).
Some contributing factors to the success of Pterois spp. in the GoM include: buoyant egg masses and long-lived larvae [28 days (Ahrenholz and Morris, 2010; Morris et al., 2011)], limited number of natural predators (Mumby et al., 2011; Diller et al., 2014), rapid growth rate (Green et al., 2011), and high reproductive success (Albins and Hixon, 2013). Fogg et al. (2017) examined the reproductive biology of P. volitans in the nGoM. Comparative reproductive data from the native range of the species are surprisingly sparse, but it appears that reproductive output is higher in the nGoM than in the native range.
NOAA, partnering with several other organizations, has been conducting annual ‘Lionfish Invitationals’ since 2015 to document and capture lionfish in the FGBNMS. While this effort will greatly contribute to the scientific study of lionfish in the nGoM, it likely does not significantly reduce their populations. Nuttall et al. (2014) detected nearly 400 lionfish, including both species, in mesophotic environments down to 112 m on 14 banks surveyed by ROV, with the highest counts between 80 and 90 m depth. At the time of the study, the eastern banks were more heavily invaded than the western banks. Three of the western banks (Horseshoe Bank, 29 Fathom Bank and Bright Bank) seemed to still be free of lionfish.
Paxton et al. (2019) provide indirect support that artificial reefs have contributed to the lionfish invasion in the nGoM. Their survey of fish abundance and diversity on 30 artificial and natural reefs off the coast of North Carolina show that tropical fish at their distribution edge, particularly planktivorious and piscivorous species, have higher abundances on artificial than on natural reefs. They conclude that artificial structures probably act as stepping stones for northward expansion of motile tropical fish species in the face of climate change.
The Regal Demoiselle, Neopomacentrus cyanomos
This small (<10 cm) species of damselfish is the latest documented newcomer in the nGoM, including the FGBNMS (Bennett et al., 2019; Nuttall et al., 2019). The Indo-Pacific/Indian Ocean species was first reported from natural reefs in the southern GoM off the coast of Veracruz in 2013 (González-Gándara and de la Cruz-Francisco, 2014). Johnston and Akins (2016) modeled the invasive potential of this species and concluded that currents in the southern GoM were not conducive to long-distance transport of N. cyanomos larvae. Nonetheless, around the same time, N. cyanomos was reported from Cayo Arcas on the southwestern corner of Campeche Bank, ca. 350 km distant from the original sighting (Robertson et al., 2016). In this area it was reported as ‘superabundant’ on the coral reef as well as on an oil platform which housed ‘thousands’ of individuals (Robertson et al., 2016). Subsequent reviews of video footage showed that the species was already present in 2013, but remained unrecognized at the time (Robertson et al., 2016).
In 2017, Bennett et al. (2019) surveyed 138 sites, including natural and artificial reefs, off the Alabama coast. While no N. cyanomos were sighted on natural reefs, several hundred of the non- native damselfish were discovered in groups of 10–35, primarily juveniles, on five petroleum platforms and one concrete structure. It is unclear whether they reached these locations via ship ballast water (the Ports of Mobile, AL and Tampico, Veracruz are well-connected through shipping routes) or whether early life stages may have been transported there via the Loop Current. Although it was initially uncertain whether N. cyanomos would be able to survive the colder winter water temperatures in the nGoM, Bennett et al. (2019) confirmed their presence in 2018. In late June, 2018, NOAA reported the presence of N. cyanomos at Stetson Bank where schools of several 100 individuals were observed on multiple pinnacles and inside sponges (Nuttall et al., 2019). The ecological impact of this most recent invasion is difficult to predict, but the possible displacement of native damselfish species is a concern.
Cup Corals: Tubastrea spp.
Tubastrea coccinea, also known as the orange cup coral, is an Indo-Pacific non-zooxanthellate scleractinian stony coral (Sheehy and Vik, 2010) which was introduced to the Caribbean in 1943 and in the GoM in the 1960s. It now has a pantropical distribution and is considered the most abundant scleractinian species in both the tropical Pacific and Atlantic (Cairns, 1994). A second species, the green cup coral Tubastrea micranthus, was first detected in 2006 on a single platform (GI-93-B) off the coast of Louisiana (Sammarco et al., 2010), but had appeared on eight additional platforms within a 20 mile radius of GI-93-B by 2014 (Sammarco et al., 2014b). The two species show clear depth preferences: whereas T. coccinea is generally found above 78 m, T. micranthus occupies deeper portions of the platforms, down to 138 m (Sammarco et al., 2013). Recently, a third species, the Indo-Pacific T. tagusensis, has been reported for the first time on offshore plantforms in the GoM (Figueroa et al., 2019). Although sampling was limited, in some sites T. tagusensis seemed to outnumber T. coccinea. Morphologically, T. tagusensis is very similar to T. coccinea, and although it can be distinguished from its congener using molecular tools, misidentification of T. tagusensis as T. coccinea may have occurred in the past. This complicates attempts to understand the history and timing of T. tagusensis GoM invasion. If T. tagusensis has only recently invaded the GoM, Figueroa et al. (2019) argue it may be expanding rapidly and potentially outcompeting T. coccinea, thus posing a new threat to the GoM ecosystems.
Tubastrea coccinea exhibits a predominantly hermaphroditic reproduction, with typical non-feeding cnidarian larvae, planulae that settle on the appropriate substrate after 1 to 3 days. However, the planulae can survive and be competent for up to 100 days (Fenner, 2001), thus showing massive potential for dispersal. T. coccinea has multiple reproductive cycles per year, and can also reproduce asexually, all traits that generally favor invasive species success. Assemblages of T. coccinea have been reported fouling hulls of boats (Cairns, 2000) and the species is believed to have been introduced by shipping. In the GoM, after being reported on oil and gas platforms, it was reported on a range of human-made structures, such as sunken vessels and other artificial reefs (Shearer, 2009). Although there is evidence that T. coccinea takes advantage of newly formed habitat, it can sometimes be found on established reef communities, including in the FGBNMS. Removal experiments in the FGBNMS have shown that T. coccinea has a very rapid recolonization rate (Precht et al., 2014). Off the coasts of Mexico, Texas, and Louisiana, it has been shown to colonize artificial reefs and oil rigs within a few years of their installation (Fenner, 2001) and it has been rapidly expanding its invasive range (Creed et al., 2017). On oil and gas platforms in the nGoM, T. coccinea is most abundant in relatively shallow waters, peaking at about 17 m (Sammarco et al., 2004), although it can be found at deeper sites (Sammarco et al., 2013). Its depth distribution may be limited by environmental factors such as sedimentation and turbidity associated with river discharge (Sammarco et al., 2013).
Tubastrea coccinea is an excellent competitor for space and, in parts of its invasive range, it has been shown to outcompete local coral species (Creed, 2006; Lages et al., 2011; Riul et al., 2013) and cause tissue necrosis to colonies that come in contact with its polyps (dos Santos et al., 2013). It can also impact mussel beds (Mantelatto and Creed, 2015), and alter local community structure and biodiversity (Lages et al., 2011).
Acorn Barnacles
Anthropogenic transport of acorn barnacles (Cirripedia) probably dates back many centuries, as they are some of the most common fouling organisms on ship hulls. Additionally, their nauplius larvae are long-lived and able to survive long passages in ballast water (Cohen et al., 2014). As such, the native distributions of most species are hard to determine and species are often characterized as ‘cryptogenic’ (of unknown geographic origin). Like many other fouling organisms, most barnacle species are difficult to identify, especially because many characteristics of their shell and appendages can be plastic depending on environmental conditions (Cohen et al., 2014). Carlton et al. (2011) list four non-native species of barnacles in the GoM and reconstruct their invasion history. In chronological order, the four species are Balanus trigonus, Amphibalanus amphitrite, Amphibalanus reticulatus, and Megabalanus coccopoma. B. trigonus has been established the longest, probably introduced in the mid- to late 1800s on ship hulls and is now abundant throughout the nGoM (Gittings, 1985; Carlton et al., 2011). The two Amphibalanus species first appeared in the 1950s (Carlton et al., 2011). M. coccopoma is native to the eastern Pacific and was first reported in the GoM by Perrault (2004) from jetties in Louisiana. M. coccopoma is a large barnacle with a characteristically pink shell. Cohen et al. (2014) examined mitochondrial sequence divergence in this species throughout its native and invasive range. Their study revealed that there were likely multiple invasions in the Southeastern US and Brazil. The species is now common on oil and gas structures (Gittings, 2009). Other Megabalanus species may be established in the GoM as well.
The environmental implications of introduced barnacles are not well-understood because there are often no baseline data from before the invasion. Large barnacles like M. coccopoma may be particularly successful in competing with smaller species, as they are able to occupy space faster and possibly filter feed more efficiently. Fouling by large barnacles on artificial structures can also add substantial weight and volume to a structure and affect its hydrodynamic properties. The survival and northern expansion of the tropical M. coccopoma may be limited by water temperature (Crickenberger et al., 2017), but warming temperatures may facilitate its northward spread in the future.
Tunicates: Didemnum perlucidum
The invasion history of the colonial tunicate, Didemnum perlucidum, in the GoM remains poorly documented. Culbertson and Harper (2002) report that between 1998 and 2000, divers observed a thin white layer of this encrusting species that almost completely covered structure High Island A-532 from 27 m to least 42 m depth (the depth limit of the SCUBA surveys). High Island A-532 is located 12 nautical miles from Stetson Bank (part of the FGBNMS) in 58 m of water and was installed as an artificial reef in 1997. D. perlucidum was also observed on several nearby structures within 12 nautical miles (Culbertson and Harper, 2002). The species can be characterized as cryptogenic. It was originally described from the island of Guadeloupe (Monniot, 1983), but it is uncertain whether its native range included the Caribbean. D. perlucidum is distributed worldwide in tropical and subtropical waters and is most prevalent on artificial structures (Dias et al., 2016). Its most recent appearance has been in Western Australia where it was first detected in 2010 and has since spread to the Northern Territories (Smale and Childs, 2012; Bridgwood et al., 2014; Dias et al., 2016). Ascidians have very short-lived larval stages, and introductions are therefore likely a result of the propagation of adults from fouling communities on boat hulls or rafting debris. Dias et al. (2016) studied genetic diversity of D. perlucidum worldwide, including a population from the GoM (Veracruz, Mexico), using cytochrome c oxidase subunit I (COI) sequence data. They found that genetic diversity is low, with a single haplotype (Haplotype 1) present in most locations. This likely indicates that most of the populations are relatively recent introductions. However, as D. perlucidum reproduces both sexually and asexually through budding, the genetic uniformity may be partially attributable to clonal organization. The GoM population actually included Haplotypes 1 and 3 but it is unclear whether the two haplotypes represent two separate introductions or local speciation. More sensitive molecular markers are needed to resolve finer scale population differentiation for this species.
Emerging Concerns
Artificial reefs may not only provide habitat to newly arriving species, but may also promote habitat-limited native species which could lead to shifts in trophic structure and ecosystem function in the Gulf of Mexico. Furthermore, some of these species, notably jellyfish and Gambierdiscus species dinoflagellates, may raise public health concerns if their abundance increases, as is likely under predicted climate change scenarios.
Implications of Oil and Gas Structures for Jellyfish Blooms
Jellyfish (Scyphozoa, Cnidaria) go through seasonal pulses in response to environmental triggers, and rapidly produce enormous biomass that impacts the marine food chain. Three jellyfish species have formed massive blooms in the GoM: the moon jelly, Aurelia sp. 9; the Atlantic sea nettle, Chrysaora quinquecirrha, and the Australian spotted jellyfish, Phyllorhiza punctata (Graham, 2001; Robinson and Graham, 2013; Chiaverano et al., 2016; Frolova and Miglietta, 2020). Patches of P. punctata covering up to 150 km and composed of an estimated 5.6 × 106 medusae have been reported. These super swarms threaten large commercial fisheries and can cause millions of dollars in damage (Graham et al., 2003).
In contrast to the relatively large pelagic medusa stages, the benthic scyphozoan polyps are microscopic and have never been detected in the GoM. Because most of the naturally available benthic habitat in the GoM is soft sediment and unsuitable for polyp attachment, it has been hypothesized that the polyps have successfully colonized artificial substrates, such as oil and gas platforms (Graham et al., 2003; Duarte et al., 2013). A recent body of literature indicates that artificial substrates, including oil and gas platforms, are suitable substrate for scyphozoan polyps (van Walraven et al., 2016; Dong et al., 2018) and play an important role in the frequency and magnitude of jellyfish blooms (Duarte et al., 2013). Moreover, there is evidence that the removal of artificial structures may limit jellyfish bloom occurrence (Jin et al., 2017) and that artificial platform-originating planulae (cnidarian larval stage) play an important role in sustaining local jellyfish populations (Vodopivec et al., 2017).
Occurrence of Ciguatera Toxin-Producing Dinoflagellates
The complex fouling communities on artificial structures also represent potential habitat for benthic/epiphytic dinoflagellates of the genus Gambierdiscus, the causative agent of ciguatera fish poisoning (CFP). Ciguatera is the most common foodborne illness related to finfish consumption (Friedman et al., 2017), with estimates of up to 500,000 poisonings per year (Fleming et al., 1998) in tropical and subtropical regions. Gambierdiscus dinoflagellates are well-known from coral reef areas, where they are found primarily on the surface of macroalgae. They are consumed by herbivores and detritivores, and the toxins they produce persist through successive trophic levels, eventually contaminating large, mobile, predatory finfish species that are targeted in many recreational and commercial fisheries. Economic impacts associated with ciguatera, ranging from loss of fishing revenue to morbidity from illness, can be considerable; the annual impact of ciguatera in the U.S. was estimated to be in excess of $20 million (Anderson et al., 2000).
While several island regions of the U.S. and its territories (Puerto Rico, the U.S. Virgin Islands, Hawaii) are considered to be hyperendemic for ciguatera, the only ciguatera-endemic mainland region is the Florida coast (Lehane and Lewis, 2000). In contrast, the nGoM has been considered to be at low or unknown risk for ciguatera (Lewis, 2001) because it comprises mainly soft sediment benthos that is not hospitable for Gambierdiscus spp. and water temperatures that are thought to be too low for vigorous growth (Kibler et al., 2017). In addition to the ongoing proliferation of artificial hard substrate, there has also been a steady increase in sea surface temperatures over the past decades (Muller-Karger et al., 2015). Villareal et al. (2007) reported the first findings of Gambierdiscus spp. in the northern GoM, from six oil platforms and also on floating Sargassum seaweed. The authors also hypothesized that the increase in artificial structure in the GoM would facilitate colonization of the area by Gambierdiscus spp., thereby aiding range expansion as sea surface temperatures warmed.
Since that first record in the nGoM, diverse Gambierdiscus communities have been reported within the FGBNMS in the nGoM (Tester et al., 2013). Five of the seven species known from the Caribbean were found at the east and west Banks, at depths > 45 m, and the authors estimate that conditions at the FGBNMS would provide 200 days of optimal growth conditions annually. The diversity and widespread distribution across the Banks suggests that these communities are able to persist throughout the year. Sea surface temperatures are predicted to continue increasing in the region (Moore et al., 2008), which would increase the number of optimal growing days for Gambierdiscus spp. in the nGoM (Tester et al., 2013), and therefore the risk for ciguatera (Tester et al., 2010; Gingold et al., 2014). Another risk factor is range expansion due to warmer waters. As sea surface temperatures increase, Gambierdiscus species distributions may shift northward (Hallegraeff, 2010; Parsons et al., 2012), facilitated by the presence of artificial structures in the GoM, which can act as ‘stepping stones’ for expansion.
Ciguatera cases are routinely reported from the nGOM region. A review of case reports and surveys in Florida estimated the annual incidence at 5.6 cases per 100,000 population, although the incidence rates are higher for the counties in which it is most common (Radke et al., 2015). Export of fish means that ciguatera cases are not always restricted to the source area (CDC, 2009). In the northern GoM, ciguatera cases were reported in 1998 from fish caught off of an oil platform in the region (CDC, 2006) and in 2007 from a gag grouper caught at the FGBNMS (FGBNMS, 2019). The 2007 event was one cause for the FDA to issue a 2008 ciguatera toxin alert for fish caught in the nGoM near the FGBNMS. This later resulted in an industry guidance document for seafood processors, cautioning them about purchasing reef fish caught in areas that were considered to be at risk for ciguatera, including the GoM and the FGBNMS specifically (U.S FDA, 2013). This guidance remains in effect today.
It is clear that the causative agents of ciguatera, Gambierdiscus spp., are present throughout the GoM, and that they become sufficiently abundant, at least periodically, to result in toxic fish and human poisonings. Predictions of future risk are difficult because we have so little information on Gambierdiscus spp. in the nGoM, which harbors the greatest density of artificial structures. Gambierdiscus dinoflagellates occur in multispecies assemblages, and the level of toxicity varies between species (Pisapia et al., 2017), thus both community composition and relative abundance affect ciguatera risk. However, there have been no quantitative measures of Gambierdiscus species diversity and abundance from the GoM. We also do not know whether nGoM populations are long-term residents or recent immigrants, and if they are locally sustaining or depend on dispersal from hydrographically connected regions. Populations of Gambierdiscus spp. in the US Virgin Islands and the Florida Keys show significant genetic differentiation (Sassenhagen et al., 2018), which suggests that Gambierdiscus spp. populations may be fairly isolated. To understand the likelihood and consequences of range expansion in the region, we need to understand the current community composition and distribution within the GoM, the occurrence and routes of dispersal, and the connectivity of nGoM populations to other ‘pockets’ of Gambierdiscus spp. in the Gulf and Caribbean.
Fisheries Enhancement
The primary argument in favor of artificial reefs is the enhancement of fisheries, including the recovery of overfished populations (Pickering and Whitmarsh, 1997; Shipp and Bortone, 2009; Bull and Love, 2019). There are two competing hypotheses about the role of artificial reefs for fish stocks (Bohnsack and Sutherland, 1985; Bohnsack, 1989; Pickering and Whitmarsh, 1997): the production hypothesis states that artificial structures increase fish stocks in an ecosystem by providing additional shelter and food, while the attraction hypothesis posits that artificial structures attract fish from nearby areas by providing shelter and food, thus only leading to a redistribution of the existing fish stock.
The red snapper (Lutjanus campechanus) fishery, dating back to the mid 1800s, is one of the most important in the GoM, and its history has been reviewed in several recent publications (Shipp and Bortone, 2009; Cowan et al., 2011; Bull and Love, 2019). Other commonly fished species associated with artificial reefs in the nGoM are gray snapper (a.k.a. mangrove snapper, Lutjanus griseus, Fischer et al., 2005); vermillion snapper (Rhomboplites aurorubens; Allman, 2007), red grouper (Epinephelus morio, Bull and Kendall, 1994), greater amberjack (Seriola dumerilii, Stanley and Wilson, 1989) and gray triggerfish (Herbig and Szedlmayer, 2016). Artificial reefs are often cited as the primary reason for the recovery of red snapper stocks after the collapse of the commercial fishery in the 1980s (e.g., Shipp and Bortone, 2009), but this notion has repeatedly been called into question. Stanley and Wilson (2000) estimated that artificial reefs have increased the available snapper habitat in the nGoM by only 4.1%, and (Cowan et al., 2011) argued that the recovery only commenced recently, although artificial reefs have existed for decades prior.
When evaluating the impact of artificial reefs on fish populations in the nGoM, the type of reef, location, density, depth and vicinity to other natural and artificial reefs are important factors to consider. There is evidence (Ajemian et al., 2015) that standing platforms support the highest species richness. In this context, a platform depth of around 50 m has been reported to be the most conducive to enhancing fish stocks (Ajemian et al., 2015), likely because this depth represents a transition between coastal and offshore zones. However, there is also evidence of a negative correlation between fish biomass and reef density (Strelcheck et al., 2005). Fish population surveys conducted at HI-389A, a recently reefed platform near the east FGB, found significant overlap in fish community composition between the natural and artificial reefs, but species richness on the artificial structure remained lower than on the natural reef, despite their geographic vicinity (Rooker et al., 1997). Similarly, new artificial reefs deployed near existing natural habitats housed fewer juvenile red snapper than those deployed at larger distances (Mudrak and Szedlmayer, 2012).
These findings show that there is evidence for both production and attraction of fish to artificial structures. Which process dominates can greatly vary temporally and spatially. Broughton (2012) argues that rather than focusing on the dichotomy between production and attraction, a broader evaluation of the ecosystem function of artificial structures and their wide-ranging effects on fisheries is necessary (see Research Priority 3); but there is little doubt that artificial structures have become important components of fisheries species demographies in the GoM.
Future Research Priorities and Conclusion
We have reviewed the current literature on the roles of artificial reefs in the nGoM in facilitating the establishment of non-native species, promoting population increases of previously habitat-limited species, and their impact on fisheries. There are still large gaps in our understanding of the organismal communities inhabiting the artificial structures that are a prominent part of the nGoM ecosystem, and their interactions with established natural communities like those at the FGBNMS. Based on our review, we have identified three research priorities aimed at improving our understanding of the community ecology of artificial reefs in the nGoM (Table 1). For each, we outline potential research approaches, with an emphasis on novel techniques that can be used to provide insight to long-standing questions.
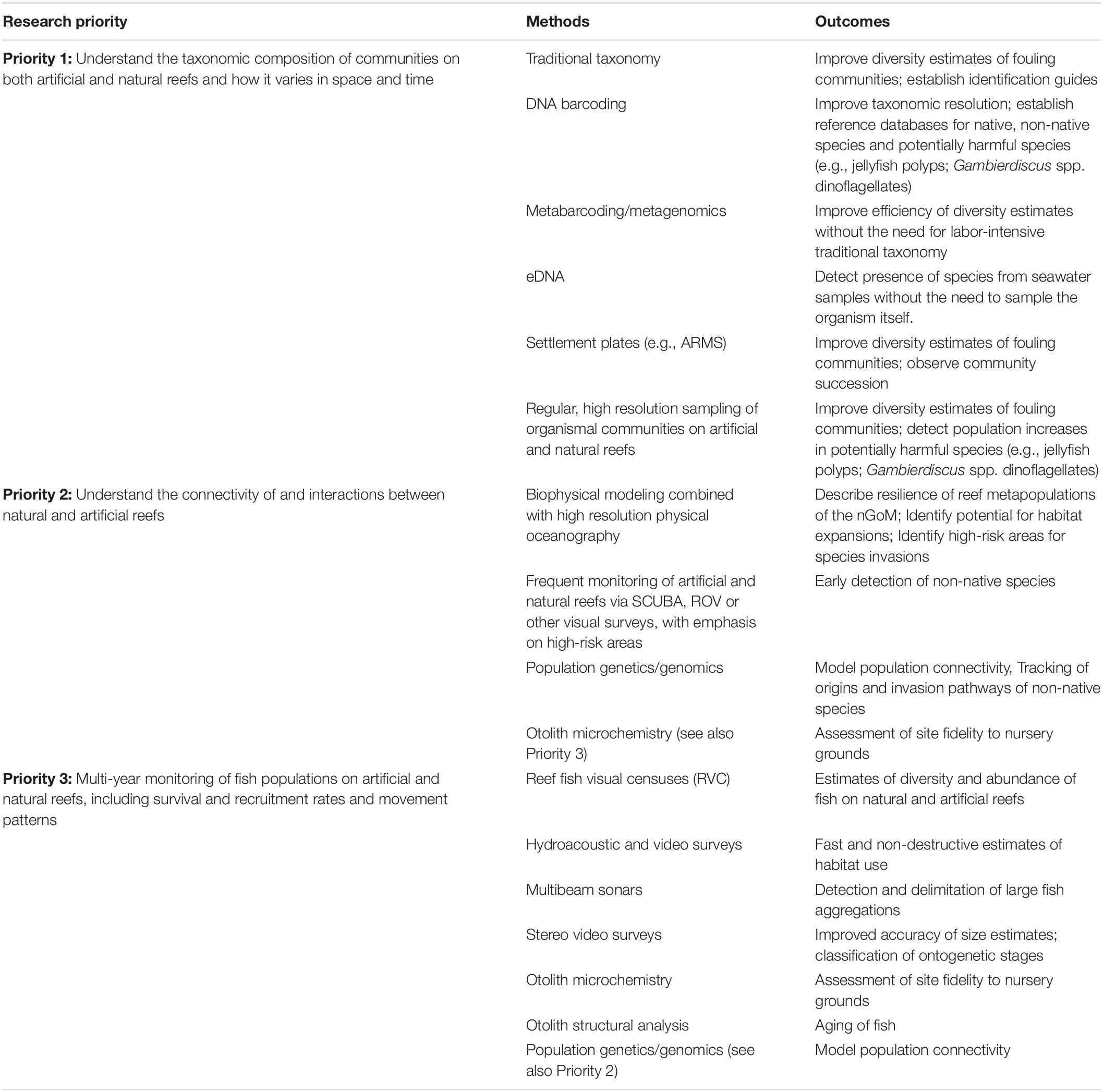
Table 1. Proposed research priorities for artificial reefs in the nGoM, methods to address the priorities and their prospective outcomes.
Priority 1
Understand the taxonomic composition of communities on both artificial and natural reefs and how it varies in space and time.
Rationale
We have summarized the evidence for the invasion of some species, but other invasives may have gone unnoticed, as thorough taxonomic surveys have been and continue to be rare. Repeated and continual sampling is critical for detecting new invasions and expansions of existing ones, as well as the appearance of native taxa at new sites. This is especially important for taxa of public health and economic concern, such as jellyfish (see section “Implications of Oil and Gas Structures for Jellyfish Blooms”) and Gambierdiscus spp. dinoflagellates (see section “Occurrence of Ciguatera Toxin-Producing Dinoflagellates”). Systematic spatial sampling will help us to understand how structure density and proximity to different habitat types may influence community composition, population density, and ecosystem function, as well as their potential as stepping stones for habitat expansion.
Research Approaches
Although time and labor-intensive, traditional taxonomy remains an important tool for the characterization of organismal communities. Standardized identification guides are a prerequisite to accurately detect changes in community composition and assess their downstream effects. In addition to traditional taxonomy, genetic and genomic methods are becoming more cost and time efficient as technology advances, and they are particularly useful for the detection of microscopic or cryptic taxa and life stages. They allow the taxonomic identification of individual organisms (DNA barcoding) (Hebert et al., 2003; Miller, 2007) as well as the analysis of community composition (metabarcoding or metagenomics). Environmental DNA (eDNA) from seawater can provide broad coverage of community membership that circumvents the limits of discrete sampling of organisms from substrate (Thomsen et al., 2012). Sampling can also be standardized by deployment of settlement plates. An excellent example of this is the Autonomous Reef Monitoring Systems (ARMS), simple stacks of PVC plates built using a standardized design. Settling organisms are processed using standardized procedures, allowing for direct comparisons between different habitat types or geographic regions (Leray and Knowlton, 2015). If multiple ARMS are deployed on the same structure for different lengths of time, they can also be used to study community succession. In addition, the ARMS provide information on the potential for invasions. Taxa that have dispersed to the area but would otherwise be unable to settle in the adjacent occupied habitat could colonize the ARMS, thus providing information on migration and dispersal. These same samples could be utilized as described in Priority 2 to examine connectivity across the region. Regular monitoring of natural reefs, with emphasis on predicted high risk areas and with increased vigilance after disturbance events such as the sudden mortality event in the East Flower Garden Banks in 2016 (Johnston et al., 2019) may help mitigate any negative effects, e.g., by active removal of invasive species.
Priority 2
Understand the connectivity of and interactions between natural and artificial reefs.
Rationale
Although the debate is still ongoing, there is increasing evidence that artificial reefs can act as stepping stones to facilitate species invasions and range expansions into the nGoM. Non-native and invasive species may negatively affect the health and resiliency of the natural reef communities. Further, the linkages between natural and artificial habitats are not clear – do they act as source or sink? Do artificial habitats promote or reduce genetic diversity within metapopulations? Do they provide trophic subsidies? This is important knowledge for not just introduced or non-native species, but also for indigenous taxa.
Research Approaches
The composition of natural and artificial reef communities is a crucial determinant of their ecosystem function. Understanding the connections between and within these two habitat types is essential for understanding how the current ecosystem state developed, and how it may change in response to disturbance. Biophysical modeling can be used to assess the likelihood of non-native species colonizing natural reefs from nearby artificial structures and to identify high risk focus areas for increased monitoring. In conjunction with high-resolution physical oceanography, biophysical modeling has become an important tool in the estimation of larval dispersal and connectivity in the ocean (Kool et al., 2012), and is especially useful when assessing the metapopulation effects of habitat expansion or removal (Henry et al., 2018), or for designing protected areas (White et al., 2014; Chollett et al., 2017). Biophysical dispersal models can be especially powerful when coupled with population genetics/genomics studies (e.g., Lee et al., 2013; Baltazar-Soares et al., 2018). Population genetic methods can reveal the extent of connectivity or isolation between habitats, provide insight into the source and history of a population, and even assess the extent and direction of migration between sites. Coupled biophysical-genetic models have been applied to habitats on the continental shelf of the nGoM (Garavelli et al., 2018; Studivan and Voss, 2018) but need to be extended to include more taxa with varied life histories.
Priority 3
Examine the broader role of artificial habitats in fisheries, past attraction and production, including more accurate estimates of survival and recruitment rates and movement patterns.
Rationale
The processes of attraction and production of fish to artificial reefs can vary greatly temporally and spatially. Rather than focusing on the attraction/production dichotomy, multi-year monitoring of fish populations on artificial and natural reefs can generate a more nuanced view of the role of artificial reefs for overall ecosystem health and fisheries.
Research Approaches
Continuation of the Reef Fish Visual Censuses (RVC) (Bohnsack and Bannerot, 1986) conducted in the nGoM (e.g., Rooker et al., 1997; Hickerson et al., 2012) would be beneficial to continue monitoring the fish populations, but these methods are expensive (both in time and cost). Otolith microchemistry can be used to determine nursery areas of fish and thus track site fidelity (Patterson et al., 1998; Zapp Sluis et al., 2012). Otolith structural analysis is well-established to age fish, including red snapper (Bortone and Hollingsworth, 1980; Patterson et al., 2001; Wilson and Nieland, 2001). Combining hydroacoustic and video technologies hold great promise for fast and non-destructive surveys of fish populations to identify and monitor habitat use at these sites (Reynolds et al., 2018). Novel stereo video techniques enable more accurate length estimates and allow for improved classification of ontogenetic stages (Davis et al., 2015; Elliott et al., 2017). In addition, the modification and utilization of multibeam sonars provides a new avenue for monitoring and characterizing larger fish populations (Melvin, 2016) although the methods may be limited by depth and adverse weather conditions.
In summary, the nGoM hosts a remarkable diversity of distinct habitats with their associated biological communities, ranging from thriving coral reefs to chemosynthesis-driven cold seep assemblages. Among these diverse habitats, artificial reefs are the newest additions and have led to ongoing ‘ocean sprawl’ (Duarte et al., 2013; Bishop et al., 2017). While some artificial structures have been in place for about three centuries (Horrell and Borgens, 2014), the majority are active or reefed oil and gas extraction platforms that were deployed over the past 50 years. Other anthropogenic activities, such as shrimp trawling, have long fundamentally altered the GoM ecosystem. It could be argued that the GoM ecosystem has reached a point of no return (Sommer et al., 2019) and the best solution may be to support the services provided by the ‘novel ecosystems’ of artificial structures (Hobbs et al., 2014) rather than trying to restore the GoM its original condition. Whether we choose to protect the current ecosystem or attempt restoration, it is critical to understand the function of artificial structures.
Author Contributions
AS, DE, and MM conceived the idea for this review article. AS wrote the sections “Introduction,” “Fouling Communities,” “Documented Invasions,” “Lionfish,” “The Regal Demoiselle, Neopomacentrus cyanomos,” “Acorn Barnacles,” “Tunicates: Didemnum perlucidum,” and “Fisheries Enhancement.” MM contributed to the sections “Cup Corals: Tubastrea spp.,” and “Implications of Oil and Gas Structures for Jellyfish Blooms.” DE contributed to the section “Occurrence of Ciguatera Toxin-Producing Dinoflagellates.” DH contributed to the section “Connectivity: Stepping Stones, Metapopulations, Resilience, and Replacement.” CG provided the figures and contributed the additional text for several sections. All authors contributed to the section “Future Research Priorities and Conclusion,” Table 1, the final editing, and revisions of the manuscript.
Funding
The symposium “Gulf of Mexico Reefs: Past, Present, and Future” was funded by the Paleontological Society and the Rice University Creative Ventures Fund. This work was partially funded by the National Science Foundation PIRE (1743802) and Centers for Oceans and Human Health (OCE-1841811) programs (DE) and the National Oceanic and Atmospheric Administration ECOHAB program (CiguaHAB NA11NOS4780061; DE). ECOHAB publication number 954.
Conflict of Interest
The authors declare that the research was conducted in the absence of any commercial or financial relationships that could be construed as a potential conflict of interest.
Acknowledgments
We thank the organizers of the symposium “Gulf of Mexico Reefs: Past, Present and Future” Adrienne Correa, Rowan Martindale, and Anna Weiss for catalyzing this contribution.
References
Ahrenholz, D. W., and Morris, J. A. (2010). Larval duration of the lionfish, Pterois volitans along the Bahamian Archipelago. Environ. Biol. Fishes 88, 305–309. doi: 10.1007/s10641-010-9647-4
Ajemian, M. J., Wetz, J. J., Shipley-Lozano, B., Shively, J. D., and Stunz, G. W. (2015). An analysis of artificial reef fish community structure along the northwestern Gulf of Mexico shelf: potential ompacts of “Rigs-to-Reefs” programs. PLoS One 10:e0126354. doi: 10.1371/journal.pone.0126354
Albins, M. A., and Hixon, M. A. (2013). Worst case scenario: potential long-term effects of invasive predatory lionfish (Pterois volitans) on Atlantic and Caribbean coral-reef communities. Environ. Biol. Fishes 96, 1151–1157. doi: 10.1007/s10641-011-9795-1
Allman, R. J. (2007). Small-scale spatial variation in the population structure of vermilion snapper (Rhomboplites aurorubens) from the northeast Gulf of Mexico. Fish. Res. 88, 88–99. doi: 10.1016/j.fishres.2007.07.017
Anderson, D. M., Hoagland, P., Kaoru, Y., and White, A. W. (2000). Estimated Annual Economic Impacts from Harmful Algal Blooms (HABs) in the United States. WHOI-2000-11. Woods Hole, MA: Woods Hole Oceanographic Institution. doi: 10.1575/1912/96
Baltazar-Soares, M., Hinrichsen, H.-H., and Eizaguirre, C. (2018). Integrating population genomics and biophysical models towards evolutionary-based fisheries management. ICES J. Mar. Sci. 75, 1245–1257. doi: 10.1093/icesjms/fsx24
Bennett, C. T., Robertson, A., and Patterson, W. F. III (2019). First record of the non-indigenous Indo-Pacific damselfish, Neopomacentrus cyanomos (Bleeker, 1856) in the northern Gulf of Mexico. Bioinvasions Rec. 8, 154–166. doi: 10.3391/bir.2019.8.1.17
Bishop, M. J., Mayer-Pinto, M., Airoldi, L., Firth, L. B., Morris, R. L., Loke, L. H. I., et al. (2017). Effects of ocean sprawl on ecological connectivity: impacts and solutions. J. Exp. Mar. Biol. Ecol. 492, 7–30. doi: 10.1016/j.jembe.2017.01.021
Bohnsack, J. A. (1989). Are high densities of fishes at artificial reefs the result of habitat limitation or behavioral preference? Bull. Mar. Sci. 44, 631–645.
Bohnsack, J. A., and Bannerot, S. P. (1986). A stationary visual census technique for quantitatively assessing community structure of coral reef fishes. NOAA Tech. Rep. NMFS 41, 1–15.
Bohnsack, J. A., and Sutherland, D. L. (1985). Artificial reef research: a review with recommendations for future priorities. Bull. Mar. Sci. 37, 11–39.
Boland, G. (2000). “Fish and epifaunal community observations at an artificial reef near a natural coral reef: Nineteen years at High Island platform A-389A, from bare steel to coral habitat,” in Proceedings of the Gulf of Mexico Fish and Fisheries: Bringing Together New and Recent Research, New Orleans, LA.
Bortone, S. A., and Hollingsworth, C. L. (1980). Ageing red snapper, Lutjanus campechanus, with otoliths, scales and vertebrae. Northeast Gulf Sci. 4, 1–4. doi: 10.18785/negs.0401.09
Bridgwood, S. D., Muñoz, J., and McDonald, J. I. (2014). Catch me if you can! The story of a colonial ascidian’s takeover bid in Western Australia. Bioinvasions Rec. 3, 217–223. doi: 10.3391/bir.2014.3.4.02
Bright, T. J., Gittings, S. R., and Zingula, R. (1991). Occurrence of Atlantic reef corals on offshore platforms in the northwestern Gulf of Mexico. Northeast Gulf Sci. 12, 55–60. doi: 10.18785/negs.1201.06
Broughton, K. (2012). Office of National Marine Sanctuaries Science Review of Artificial Reefs. US Department of Commerce, National Oceanic and Atmospheric Administration, Office of National Marine Sanctuaries, Silver Spring Marine Sanctuaries Conservation Series ONMS-12-05. Available online at: http://sanctuaries.noaa.gov/science/conservation/pdfs/artificial_reef.pdf (accessed April 7, 2020).
BSEE/BOEM Data Center (2019). Available online at: https://www.data.bsee.gov (accessed July 9, 2019).
Bull, A. S., and Kendall, J. J. J. (1994). An indication of the process: offshore platforms as artificial reefs in the Gulf of Mexico. Bull. Mar. Sci. 55, 1086–1098.
Bull, A. S., and Love, M. S. (2019). Worldwide oil and gas platform decommissioning: a review of practices and reefing options. Ocean Coast. Manage. 168, 274–306. doi: 10.1016/j.ocecoaman.2018.10.024
Cairns, S. (1994). Scleractinia of the temperate North Pacific. Smithson. Contrib. Zool. 557, 1–150.
Cairns, S. (2000). A Revision of the Shllow-Water Azooxanthellate Scleractinia of the Western Atlantic. Hilversum: Uitgeverij Verloren.
Carlton, J. T., Newman, W. A., and Pitombo, F. B. (2011). “Barnacle invasions: introduced, cryptogenic, and range expanding cirripedia of North and South America,” in The Wrong Place - Alien Marine Crustaceans: Distribution, Biology and Impacts, eds B. S. Galil, P. F. Clark, and J. T. Carlton (Dordrecht: Springer), 159–213. doi: 10.1007/978-94-007-0591-3_5
Chiaverano, L. M., Bayha, K. W., and Graham, W. M. (2016). Local versus generalized phenotypes in two sympatric Aurelia species: understanding jellyfish ecology using genetics and morphometrics. PLoS One 11:e0156588. doi: 10.1371/journal.pone.0156588
Chollett, I., Garavelli, L., Holstein, D., Cherubin, L., Fulton, S., and Box, S. J. (2017). A case for redefining the boundaries of the Mesoamerican Reef Ecoregion. Coral Reefs 36, 1039–1046. doi: 10.1007/s00338-017-1595-4
Cohen, O. R., Walters, L. J., and Hoffman, E. A. (2014). Clash of the titans: a multi-species invasion with high gene flow in the globally invasive titan acorn barnacle. Biol. Invasions 16, 1743–1756. doi: 10.1007/s10530-013-0624-7
Cordes, E. E., Bergquist, D. C., and Fisher, C. R. (2009). Macro-ecology of gulf of Mexico cold seeps. Annu. Rev. Mar. Sci. 1, 143–168. doi: 10.1146/annurev.marine.010908.163912
Cordes, E. E., Jones, D. O. B., Schlacher, T. A., Amon, D. J., Bernardino, A. F., Brooke, S., et al. (2016). Environmental impacts of the deep-water oil and gas industry: a review to guide management strategies. Front. Environ. Sci. 4:58. doi: 10.3389/fenvs.2016.00058
Côté, I. M., Green, S. J., and Hixon, M. A. (2013). Predatory fish invaders: Insights from Indo-Pacific lionfish in the western Atlantic and Caribbean. Biol. Conserv. 164, 50–61. doi: 10.1016/j.biocon.2013.04.014
Cowan, J. H. Jr., Grimes, C. B., Patterson, W. F. III, Walters, C. J., Jones, A. C., Lindberg, W. J., et al. (2011). Red snapper management in the Gulf of Mexico: science- or faith-based? Rev. Fish. Biol. 21, 187–204. doi: 10.1007/s11160-010-9165-7
Cowen, R. K., and Spunaugle, S. (2009). Larval dispersal and marine population connectivity. Annu. Rev. Mar. Sci. 1, 443–466. doi: 10.1146/annurev.marine.010908.163757
Creed, J. C. (2006). Two invasive alien azooxanthellate corals, Tubastraea coccinea and Tubastraea tagusensis, dominate the native zooxanthellate Mussismilia hispida in Brazil. Coral Reefs 25, 350–350. doi: 10.1007/s00338-006-0105-x
Creed, J. C., Fenner, D., Sammarco, P., Cairns, S., Capel, K., Junqueira, A. O. R., et al. (2017). The invasion of the azooxanthellate coral Tubastraea (Scleractinia: Dendrophylliidae) throughout the world: history, pathways and vectors. Biol. Invasions 19, 283–305. doi: 10.1007/s10530-016-1279-y
Crickenberger, S., Walther, K., and Moran, A. L. (2017). Lower thermal limits to larval development do not predict poleward range limits of the introduced tropical barnacle Megabalanus coccopoma. Invertebr. Biol. 136, 37–49. doi: 10.1111/ivb.12160
Culbertson, J., and Harper, D. E. (2002). “Settlement of a colonial ascidian on an artificial reef in the Gulf of Mexico,” in Gulf of Mexico Fish and Fisheries: Bringing Together New and Recent Research (MMS Contract 1435-00-01-CA-31060), eds M. McKay, J. Nides, and D. Vigil (Washington, DC: Minerals Management Service, Gulf of Mexico OCS Region), 614–630.
Dahl, K. A., and Patterson, W. F. III (2014). Habitat-specific density and diet of rapidly expanding invasive red lionfish, Pterois volitans, populations in the northern Gulf of Mexico. PLoS One 9:e105852. doi: 10.1371/journal.pone.0105852
Daigle, S. T., Fleeger, J. W., Cowan, J. H., and Pascal, P.-Y. (2013). What is the relative importance of phytoplankton and attached macroalgae and epiphytes to food webs on offshore oil platforms? Mar. Coast. Fish. 5, 53–64. doi: 10.1080/19425120.2013.774301
Davis, T., Harasti, D., and Smith, S. D. (2015). Compensating for length biases in underwater visual census of fishes using stereo video measurements. Mar. Freshw. Res. 66, 286–291. doi: 10.1071/MF14076
Dias, J., Rocha, R., Godwin, S., Tovar-Hernandez, M. A., Delahoz, M., McKirdy, S., et al. (2016). Investigating the cryptogenic status of the sea squirt Didemnum perlucidum (Tunicata, Ascidiacea) in Australia based on a molecular study of its global distribution. Aquat. Invasions 11, 239–245. doi: 10.3391/ai.2016.11.3.02
Diller, J. L., Frazer, T. K., and Jacoby, C. A. (2014). Coping with the lionfish invasion: evidence that naïve, native predators can learn to help. J. Exp. Mar. Biol. Ecol. 455, 45–49. doi: 10.1016/j.jembe.2014.02.014
Dong, Z., Wang, L., Sun, T., Liu, Q., and Sun, Y. (2018). Artificial reefs for sea cucumber aquaculture confirmed as settlement substrates of the moon jellyfish Aurelia coerulea. Hydrobiologia 818, 223–234. doi: 10.1007/s10750-018-3615-y
dos Santos, L. A. H., Ribeiro, F. V., and Creed, J. C. (2013). Antagonism between invasive pest corals Tubastraea spp. and the native reef-builder Mussismilia hispida in the southwest Atlantic. J. Exp. Mar. Biol. Ecol. 449, 69–76. doi: 10.1016/j.jembe.2013.08.017
Doughty, C. L., Quattrini, A. M., and Cordes, E. E. (2014). Insights into the population dynamics of the deep-sea coral genus Paramuricea in the Gulf of Mexico. Deep Sea Res. II Top. Stud. Oceanogr. 99, 71–82. doi: 10.1016/j.dsr2.2013.05.023
Duarte, C. M., Pitt, K. A., Lucas, C. H., Purcell, J. E., Uye, S., Robinson, K., et al. (2013). Is global ocean sprawl a cause of jellyfish blooms? Front. Ecol. Environ. 11, 91–97. doi: 10.1890/110246
Elliott, S. A., Turrell, W. R., Heath, M. R., and Bailey, D. M. (2017). Juvenile gadoid habitat and ontogenetic shift observations using stereo-video baited cameras. Mar. Ecol. Prog. Ser. 568, 123–135. doi: 10.3354/meps12068
Fenner, D. (2001). Biogeography of three Caribbean corals (Scleractinia) and the invasion of Tubastraea coccinea into the Gulf of Mexico. Bull. Mar. Sci. 69, 1175–1189.
Fenner, D., and Banks, K. (2004). Orange cup coral Tubastraea coccinea invades Florida and the Flower Garden Banks, Northwestern Gulf of Mexico. Coral Reefs 23, 505–507. doi: 10.1007/s00338-004-0422-x
FGBNMS (2019). FGBNMS Human Health: Flower Garden Banks. Available online at: https://sanctuaries.noaa.gov/science/sentinel-site-program/flower-garden-banks/human-health.html (accessed June 27, 2019).
Figueroa, D. F., McClure, A., Figueroa, N. J., and Hicks, D. W. (2019). Hiding in plain sight: invasive coral Tubastraea tagusensis (Scleractinia:Hexacorallia) in the Gulf of Mexico. Coral Reefs 38, 395–403. doi: 10.1007/s00338-019-01807-7
Firth, L. B., Knights, A. M., Bridger, D., Evans, A. J., Mieszkowska, N., Moore, P. J., et al. (2016). Ocean sprawl: challenges and opportunities for biodiversity management in a changing world. Oceanogr. Mar. Biol. 54, 189–262.
Fischer, A. J., Baker, M. S. Jr., Wilson, C. A., and Nieland, D. L. (2005). Age, growth, mortality, and radiometric age validation of gray snapper (Lutjanus griseus) from Louisiana. Fish. Bull. 103, 307–319.
Fisher, C., Roberts, H., Cordes, E., and Bernard, B. (2007). Cold seeps and associated communities of the Gulf of Mexico. Oceanography 20, 118–129. doi: 10.5670/oceanog.2007.12
Fleming, L. E., Baden, D. G., Bean, J. A., Weisman, R., and Blythe, D. G. (1998). “Seafood toxin diseases: issues in epidemiology and community outreach,” in Seafood Toxin Diseases: Issues in Epidemiology and Community Outreach, eds B. Reguera, J. Blanco, M. L. Fernandez, and T. Wyatt (Paris: Zunta de Galicia and Intergovernmental Oceanographic Commission of UNESCO), 245–248.
Fogg, A. Q., Brown-Peterson, N. J., and Peterson, M. S. (2017). Reproductive life history characteristics of invasive red lionfish (Pterois volitans) in the northern Gulf of Mexico. Bull. Mar. Sci. 93, 791–813. doi: 10.5343/bms.2016.1095
Friedman, M. A., Fernandez, M., Backer, L. C., Dickey, R. W., Bernstein, J., Schrank, K., et al. (2017). An updated review of Ciguatera Fish Poisoning: clinical, epidemiological, environmental, and public health management. Mar. Drugs 15:72. doi: 10.3390/md15030072
Frolova, A., and Miglietta, M. P. (2020). Insights on bloom forming jellyfish (Class: Scyphozoa) in the Gulf of Mexico: environmental tolerance ranges and limits suggest differences in habitat preference and resistance to climate change among congeners. Front. Mar. Sci. 7:93. doi: 10.3389/fmars.2020.00093
Garavelli, L., Studivan, M. S., Voss, J. D., Kuba, A., Figueiredo, J., and Chérubin, L. M. (2018). Assessment of mesophotic coral ecosystem connectivity for proposed expansion of a marine sanctuary in the northwest Gulf of Mexico: larval dynamics. Front. Mar. Sci. 5:174. doi: 10.3389/fmars.2018.00174
Gingold, D. B., Strickland, M. J., and Hess, J. J. (2014). Ciguatera fish poisoning and climate change: analysis of National Poison Center Data in the United States, 2001-2011. Environ. Health Perspect. 122, 580–586. doi: 10.1289/ehp.1307196
Gittings, S. R. (1985). Notes on barnacles (Cirripedia: Thoracica) from the Gulf of Mexico. Gulf Res. Rep. 8, 35–41. doi: 10.18785/grr.0801.06
Gittings, S. R. (2009). “Cirripedia (Crustacea) of the Gulf of Mexico,” in Gulf of Mexico–Origins, Waters, and Biota. Biodiversity, eds D. L. Felder and D. K. Camp (College Station, TX: Texas A&M University Press), 827–836.
González-Gándara, C., and de la Cruz-Francisco, V. (2014). Unusual record of the Indo-Pacific pomacentrid Neopomacentrus cyanomos (Bleeker, 1856) on coral reefs of the Gulf of Mexico. Bioinvasions Rec. 3, 49–52. doi: 10.3391/bir.2014.3.1.09
Graham, W. M. (2001). “Numerical increases and distributional shifts of Chrysaora quinquecirrha (Desor) and Aurelia aurita (Linné)(Cnidaria: Scyphozoa) in the northern Gulf of Mexico,” in Jellyfish Blooms: Ecological and Societal Importance, eds J. E. Purcell, W. M. Graham, and H. J. Dumont (Dordrecht: Springer), 97–111. doi: 10.1007/978-94-010-0722-1
Graham, W. M., Martin, D. L., Felder, D. L., Asper, V. L., and Perry, H. M. (2003). “Ecological and economic implications of a tropical jellyfish invader in the Gulf of Mexico,” in Marine Bioinvasions: Patterns, Processes and Perspectives, ed. J. Pederson (Dordrecht: Springer), 53–69. doi: 10.1007/978-94-010-0169-4
Green, S. J., Akins, J. L., and Côté, I. M. (2011). Foraging behaviour and prey consumption in the Indo-Pacific lionfish on Bahamian coral reefs. Mar. Ecol. Prog. Ser. 433, 159–167. doi: 10.3354/meps09208
Hallegraeff, G. M. (2010). Ocean climate change, phytoplankton community responses, and harmful algal blooms: a formidable predictive challenge. J. Phycol. 46, 220–235. doi: 10.1111/j.1529-8817.2010.00815.x
Hamner, R. M., Freshwater, D. W., and Whitfield, P. E. (2007). Mitochondrial cytochrome b analysis reveals two invasive lionfish species with strong founder effects in the western Atlantic. J. Fish Biol. 71, 214–222. doi: 10.1111/j.1095-8649.2007.01575.x
Hanski, I., and Ovaskainen, O. (2000). The metapopulation capacity of a fragmented landscape. Nature 404, 755–758. doi: 10.1038/35008063
Hebert, P. D. N., Cywinska, A., Ball, S. L., and deWaard, J. R. (2003). Biological identifications through DNA barcodes. Proc. R. Soc. Lond. B 270, 313–322. doi: 10.1098/rspb.2002.2218
Henry, L.-A., Mayorga-Adame, C. G., Fox, A. D., Polton, J. A., Ferris, J. S., McLellan, F., et al. (2018). Ocean sprawl facilitates dispersal and connectivity of protected species. Sci. Rep. 8:11346. doi: 10.1038/s41598-018-29575-4
Herbig, J. L., and Szedlmayer, S. T. (2016). Movement patterns of gray triggerfish, Balistes capriscus, around artificial reefs in the northern Gulf of Mexico. Fish. Manage. Ecol. 23, 418–427. doi: 10.1111/fme.12190
Hickerson, E. L., Schmahl, G. P., Johnston, M. A., Nuttall, M. F., Embesi, J. A., and Eckert, R. J. (2012). “Flower Garden Banks – A Refuge in the Gulf of Mexico?” in Proceedings of the 12th International Coral Reef Symposium, Cairns.
Hobbs, R. J., Higgs, E., Hall, C. M., Bridgewater, P., Chapin, F. S. III, Ellis, E. C., et al. (2014). Managing the whole landscape: historical, hybrid, and novel ecosystems. Front. Ecol. Environ. 12, 557–564. doi: 10.1890/130300
Horrell, C. E., and Borgens, A. A. (2014). “Interpreting the past by exploring the abyss: archaeological investigations of an early nineteenth-century shipwreck in the Gulf of Mexico,” in Proceedings of the Offshore Technology Conference, (Houston, TX: Offshore Technology Conference).
Jin, H. S., Han, D., Kim, J.-H., Shin, H.-J., Yoon, Y. H., and Han, C.-H. (2017). Simulations of the population dynamics of jellyfish polyps living on artificial substrates in coastal areas. Thalassas 33, 43–50. doi: 10.1007/s41208-016-0019-5
Johnston, M. A., Embesi, J. A., Eckert, R. J., Nuttall, M. F., Hickerson, E. L., and Schmahl, G. P. (2016a). Persistence of coral assemblages at East and West Flower Garden Banks, Gulf of Mexico. Coral Reefs 35, 821–826. doi: 10.1007/s00338-016-1452-x
Johnston, M. A., Nuttall, M. F., Eckert, R. J., Blakeway, R. D., Sterne, T. K., Hickerson, E. L., et al. (2019). Localized coral reef mortality event at East Flower Garden Bank, Gulf of Mexico. Bull. Mar. Sci. 95, 239–250. doi: 10.5343/bms.2018.0057
Johnston, M. A., Nuttall, M. F., Eckert, R. J., Embesi, J. A., Sterne, T. K., Hickerson, E. L., et al. (2016b). Rapid invasion of Indo-Pacific lionfishes Pterois volitans (Linnaeus, 1758) and P. miles (Bennett, 1828) in the Flower Garden Banks National Marine Sanctuary, Gulf of Mexico, documented in multiple data sets. Bioinvasions Rec. 5, 115–125. doi: 10.3391/bir.2016.5.2.09
Johnston, M. W., and Akins, J. L. (2016). The non-native royal damsel (Neopomacentrus cyanomos) in the southern Gulf of Mexico: an invasion risk? Mar. Biol. 163:12. doi: 10.1007/s00227-015-2777-7
Kibler, S. R., Davenport, E. D., Tester, P. A., Hardison, D. R., Holland, W. C., and Litaker, R. W. (2017). Gambierdiscus and Fukuyoa species in the greater Caribbean: regional growth projections for ciguatera-associated dinoflagellates. Ecol. Modell. 360, 204–218. doi: 10.1016/j.ecolmodel.2017.07.007
Kolian, S. R., Sammarco, P. W., and Porter, S. A. (2017). Abundance of corals on offshore oil and gas platforms in the Gulf of Mexico. Environ. Manage. 60, 357–366. doi: 10.1007/s00267-017-0862-z
Kool, J. T., Moilanen, A., and Treml, E. (2012). Population connectivity: recent advances and new perspectives. Landsc. Ecol. 28, 165–185. doi: 10.1007/s10980-012-9819-z
Lages, B. G., Fleury, B. G., Menegola, C., and Creed, J. C. (2011). Change in tropical rocky shore communities due to an alien coral invasion. Mar. Ecol. Prog. Ser. 438, 85–96. doi: 10.3354/meps09290
Lee, P. L. M., Dawson, M. N., Neill, S. P., Robins, P. E., Houghton, J. D. R., Doyle, T. K., et al. (2013). Identification of genetically and oceanographically distinct blooms of jellyfish. J. R. Soc. Interface 10:20120920. doi: 10.1098/rsif.2012.0920
Lehane, L., and Lewis, R. J. (2000). Ciguatera: recent advances but the risk remains. Int. J. Food Microbiol. 61, 91–125. doi: 10.1016/s0168-1605(00)00382-2
Leray, M., and Knowlton, N. (2015). Diversity patterns in cryptic benthic communities. Proc. Natl. Acad. Sci. U.S.A. 112, 2076–2081. doi: 10.1073/pnas.1424997112
Lewbel, G. S., Howard, R. L., and Gallaway, B. J. (1987). Zonation of dominant fouling organisms on northern Gulf of Mexico petroleum platforms. Mar. Environ. Res. 21, 199–224. doi: 10.1016/0141-1136(87)90066-3
Lewis, R. J. (2001). The changing face of ciguatera. Toxicon 39, 97–106. doi: 10.1016/s0041-0101(00)00161-6
Mantelatto, M. C., and Creed, J. C. (2015). Non-indigenous sun corals invade mussel beds in Brazil. Mar. Biodivers. 45, 605–606. doi: 10.1007/s12526-014-0282-8
Melvin, G. D. (2016). Observations of in situ Atlantic bluefin tuna (Thunnus thynnus) with 500-kHz multibeam sonar. ICES J. Mar. Sci. 73, 1975–1986. doi: 10.1093/icesjms/fsw077
Miller, S. E. (2007). DNA barcoding and the renaissance of taxonomy. Proc. Natl. Acad. Sci. U.S.A. 104, 4775–4776. doi: 10.1073/pnas.0700466104
Monniot, F. (1983). Ascidies littorales de Guadeloupe I. Didemnidae. Bull. Mus. Natl. D Hist. Nat. 5, 5–49.
Moore, S. K., Trainer, V. L., Mantua, N. J., Parker, M. S., Laws, E. A., Backer, L. C., et al. (2008). Impacts of climate variability and future climate change on harmful algal blooms and human health. Environ. Health 7(Suppl. 2):S4. doi: 10.1186/1476-069X-7-S2-S4
Morris, J. A. Jr., Shertzer, K. W., and Rice, J. A. (2011). A stage-based matrix population model of invasive lionfish with implications for control. Biol. Invasions 13, 7–12. doi: 10.1007/s10530-010-9786-8
Mudrak, P. A., and Szedlmayer, S. T. (2012). Proximity effects of larger resident fishes on recruitment of age-0 red snapper in the northern Gulf of Mexico. Trans. Am. Fish. Soc. 141, 487–494. doi: 10.1080/00028487.2012.667045
Muller-Karger, F. E., Smith, J. P., Werner, S., Chen, R., Roffer, M., Liu, Y., et al. (2015). Natural variability of surface oceanographic conditions in the offshore Gulf of Mexico. Prog. Oceanogr. 134, 54–76. doi: 10.1016/j.pocean.2014.12.007
Mumby, P. J., Harborne, A. R., and Brumbaugh, D. R. (2011). Grouper as a natural biocontrol of invasive lionfish. PLoS One 6:e21510. doi: 10.1371/journal.pone.0021510
NOAA (2016). US Artificial Reefs. Available online at: https://koordinates.com/layer/20868-us-artificial-reefs/ (accessed July 9, 2019).
Norström, A. V., Nyström, M., Lokrantz, J., and Folke, C. (2009). Alternative states on coral reefs: beyond coral–macroalgal phase shifts. Mar. Ecol. Prog. Ser. 376, 295–306. doi: 10.3354/meps07815
Nuttall, M. F., Blakeway, R. D., MacMillan, J., O’Connell, K., Hu, X., Embesi, J. A., et al. (2019). Stetson Bank Long-Term Monitoring: 2018 Annual Report. National Marine Sanctuaries Conservation Series ONMS-19-05. Galveston, TX: U.S. Department of Commerce, 43.
Nuttall, M. F., Johnston, M. A., Eckert, R. J., Embesi, J. A., Hickerson, E., and Schmahl, G. P. (2014). Lionfish (Pterois volitans [Linnaeus, 1758] and P. miles [Bennett, 1828]) records within mesophotic depth ranges on natural banks in the Northwestern Gulf of Mexico. Bioinvasions Rec. 3, 111–115. doi: 10.3391/bir.2014.3.2.09
Page, H. M., Dugan, J. E., and Piltz, F. (2010). “Fouling and antifouling in oil and other offshore industries,” in Biofouling, eds S. Dürr and J. C. Thomason (London: Wiley-Blackwell), 252–266. doi: 10.1002/9781444315462
Parsons, M. L., Aligizaki, K., Bottein, M.-Y. D., Fraga, S., Morton, S. L., Penna, A., et al. (2012). Gambierdiscus and Ostreopsis: reassessment of the state of knowledge of their taxonomy, geography, ecophysiology, and toxicology. Harmful Algae 14, 107–129. doi: 10.1016/j.hal.2011.10.017
Patterson, W. F. III, Cowan, J. H. Jr., Graham, E. Y., and Lyons, W. (1998). Otolith microchemical fingerprints of age-0 red snapper, Lutjanus campechanus, from the Northern Gulf of Mexico. Gulf Mexico Sci. 16, 83–91. doi: 10.18785/goms.1601.12
Patterson, W. F. III, Cowan, J. H. Jr., Wilson, C. A., and Shipp, R. L. (2001). Age and growth of red snapper, Lutjanus campechanus, from an artificial reef area off Alabama in the northern Gulf of Mexico. Fish. Bull. 66, 617–727.
Paxton, A. B., Peterson, C. H., Taylor, J. C., Adler, A. M., Pickering, E. A., and Silliman, B. R. (2019). Artificial reefs facilitate tropical fish at their range edge. Commun. Biol. 2:168. doi: 10.1038/s42003-019-0398-2
Perrault, R. T. (2004). An exotic tropical barnacle Megabalanus coccopoma (Darwin, 1854) in Louisiana: its probable arrival and environmental implications. Proc. Louis. Acad. Sci. 66, 13–16.
Pickering, H., and Whitmarsh, D. (1997). Artificial reefs and fisheries exploitation: a review of the ‘attraction versus production’ debate, the influence of design and its significance for policy. Fish. Res. 31, 39–59. doi: 10.1016/S0165-7836(97)00019-2
Pisapia, F., Holland, W. C., Hardison, D. R., Litaker, R. W., Fraga, S., Nishimura, T., et al. (2017). Toxicity screening of 13 Gambierdiscus strains using neuro-2a and erythrocyte lysis bioassays. Harmful Algae 63, 173–183. doi: 10.1016/j.hal.2017.02.005
Precht, W. F., Hickerson, E. L., Schmahl, G. P., and Aronson, R. B. (2014). The invasive coral Tubastraea coccinea (Lesson, 1829): implications for natural habitats in the Gulf of Mexico and the Florida Keys. Gulf Mexico Sci. 32:5. doi: 10.18785/goms.3201.05
Radke, E. G., Reich, A., and Morris, J. G. (2015). Epidemiology of ciguatera in Florida. Am. J. Trop. Med. Hyg. 93, 425–432. doi: 10.4269/ajtmh.14-0400
Reynolds, E. M., Cohen, J. H., Lewis, K. A., and Simonsen, K. A. (2018). Method for estimating relative abundance and species composition around oil and gas platforms in the northern Gulf of Mexico, U.S.A. Fish. Res. 201, 44–55. doi: 10.1016/j.fishres.2018.01.002
Riul, P., Targino, C. H., Júnior, L. A. C., Creed, J. C., Horta, P. A., and Costa, G. C. (2013). Invasive potential of the coral Tubastraea coccinea in the southwest Atlantic. Mar. Ecol. Prog. Ser. 480, 73–81. doi: 10.3354/meps10200
Robertson, D. R., Perez-España, H., Nuñez Lara, E., Puc Itza, F., and Simões, N. (2016). The fishes of Cayo Arcas (Campeche Bank, Gulf of Mexico): an updated checklist. ZooKeys 640, 139–155. doi: 10.3897/zookeys.640.10862
Robinson, K. L., and Graham, W. M. (2013). Long-term change in the abundances of northern Gulf of Mexico scyphomedusae Chrysaora sp. and Aurelia spp. with links to climate variability. Limnol. Oceanogr. 58, 235–253. doi: 10.4319/lo.2013.58.1.0235
Rooker, J. R., Dokken, Q. R., Pattengill, C. V., and Holt, G. J. (1997). Fish assemblages on artificial and natural reefs in the Flower Garden Banks National Marine Sanctuary, USA. Coral Reefs 16, 83–92. doi: 10.1007/s003380050
Rützler, K., van Soest, R. W. M., and Piantoni, C. (2009). “Sponges (Porifera) in the Gulf of Mexico,” in Gulf of Mexico – Origin, Waters, and Biota, eds D. L. Felder and D. K. Camp (College Station, TX: Texas A&M University Press), 285–313.
Sammarco, P. W., Atchison, A. D., and Boland, G. S. (2004). Expansion of coral communities within the Northern Gulf of Mexico via offshore oil and gas platforms. Mar. Ecol. Prog. Ser. 280, 129–143. doi: 10.3354/meps280129
Sammarco, P. W., Atchison, A. D., Boland, G. S., Sinclair, J., and Lirette, A. (2012a). Geographic expansion of hermatypic and ahermatypic corals in the Gulf of Mexico, and implications for dispersal and recruitment. J. Exp. Mar. Biol. Ecol. 436–437, 36–49. doi: 10.1016/j.jembe.2012.08.009
Sammarco, P. W., Brazeau, D. A., and Sinclair, J. (2012b). Genetic connectivity in scleractinian corals across the northern Gulf of Mexico: oil/gas platforms, and relationship to the Flower Garden Banks. PLoS One 7:e30144. doi: 10.1371/journal.pone.0030144
Sammarco, P. W., Lirette, A., Porter, S. A., Sinclair, J., and Genazzio, M. (2013). Depth distribution of a new invasive coral (Gulf of Mexico) –Tubastraea micranthus, comparisons with T. coccinea, and implications for control. Manage. Biol. Invasions 4, 291–303. doi: 10.3391/mbi.2013.4.4.04
Sammarco, P. W., Lirette, A., Tung, Y. F., Boland, G. S., Genazzio, M., and Sinclair, J. (2014a). Coral communities on artificial reefs in the Gulf of Mexico: standing vs. toppled oil platforms. ICES J. Mar. Sci. 71, 417–426. doi: 10.1093/icesjms/fst140
Sammarco, P. W., Porter, S., and Cairns, S. D. (2010). A new coral species introduced into the Atlantic Ocean - Tubastraea micranthus (Ehrenberg 1834) (Cnidaria, Anthozoa, Scleractinia): An invasive threat? Aquat. Invasions 5, 131–140. doi: 10.3391/ai.2010.5.2.02
Sammarco, P. W., Porter, S., Sinclair, J., and Genazzio, M. (2014b). Population expansion of a new invasive coral species, Tubastraea micranthus, in the northern Gulf of Mexico. Mar. Ecol. Prog. Ser. 495, 161–173. doi: 10.3354/meps10576
Sassenhagen, I., Gao, Y., Lozano-Duque, Y., Parsons, M. L., Smith, T. B., and Erdner, D. L. (2018). Comparison of spatial and temporal genetic differentiation in a harmful dinoflagellate species emphasizes impact of local processes. Front. Mar. Sci. 5:393. doi: 10.3389/fmars.2018.00393
Shearer, T. L. (2009). “Population analysis of an introduced coral species, Tubastraea coccinea, in Florida,” in Proceedings of the28th Symposium American Academy of Underwater Sciences, (Dauphin Island, AL: AAUS), 229–236.
Sheehy, D. J., and Vik, S. F. (2010). The role of constructed reefs in non-indigenous species introductions and range expansions. Ecol. Eng. 36, 1–11. doi: 10.1016/j.ecoleng.2009.09.012
Shipp, A. L., and Bortone, S. A. (2009). A Perspective of the importance of artificial habitat on the management of red snapper in the Gulf of Mexico. Rev. Fish. Sci. 17, 41–47. doi: 10.1080/10641260802104244
Smale, D. A., and Childs, S. (2012). The occurrence of a widespread marine invader, Didemnum perlucidum (Tunicata, Ascidiacea) in Western Australia. Biol. Invasions 14, 1325–1330. doi: 10.1007/s10530-011-0167-8
Sommer, B., Fowler, A. M., Macreadie, P. I., Palandro, D. A., Aziz, A. C., and Booth, D. J. (2019). Decommissioning of offshore oil and gas structures - environmental opportunities and challenges. Sci. Total Environ. 658, 973–981. doi: 10.1016/j.scitotenv.2018.12.193
Stanley, D. R., and Wilson, C. A. (1989). Utilization of offshore platforms by recreational fishermen and SCUBA divers off the Louisiana coast. Bull. Mar. Sci. 44, 767–775.
Stanley, D. R., and Wilson, C. A. (2000). Seasonal and spatial variation in the biomass and size frequency distribution of the fish associated with oil and gas platforms in the northern Gulf of Mexico. OCS Study MMS 2000-005. New Orleans, LA: U.S. Dept. of the Interior, 252.
Strelcheck, A. J., Cowan, J. H., and Shah, A. (2005). Influence of reef location on artificial-reef fish assemblages in the northcentral Gulf of Mexico. Bull. Mar. Sci. 77, 425–440.
Studivan, M. S., and Voss, J. D. (2018). Assessment of mesophotic coral ecosystem connectivity for proposed expansion of a marine sanctuary in the northwest Gulf of Mexico: population genetics. Front. Mar. Sci. 5:152. doi: 10.3389/fmars.2018.00152
Terlizzi, A., and Faimali, M. (2010). “Fouling on artificial substrates,” in Biofouling, eds S. Dürr and J. C. Thomason (Oxford: Wiley-Blackwell), 170–184. doi: 10.1002/9781444315462.ch12
Tester, P. A., Feldman, R. L., Nau, A. W., Kibler, S. R., and Wayne Litaker, R. (2010). Ciguatera fish poisoning and sea surface temperatures in the Caribbean Sea and the West Indies. Toxicon 56, 698–710. doi: 10.1016/j.toxicon.2010.02.026
Tester, P. A., Vandersea, M. W., Buckel, C. A., Kibler, S. R., Holland, W. C., Davenport, E. D., et al. (2013). Gambierdiscus (Dinophyceae) species diversity in the Flower Garden Banks National Marine Sanctuary, Northern Gulf of Mexico, USA. Harmful Algae 29, 1–9. doi: 10.1016/j.hal.2013.07.001
Thomsen, P. F., Kielgast, J., Iversen, L. L., Møller, P. R., Rasmussen, M., and Willerslev, E. (2012). Detection of a diverse marine fish fauna using environmental DNA from seawater samples. PLoS One 7:e41732. doi: 10.1371/journal.pone.0041732
US Department of Commerce (2012). Flower Garden Banks National Marine Sanctuary Final Management Plan. Silver Spring, MD: U.S Department of Commerce.
U.S FDA (2013). Guidance for Industry: Purchasing Reef Fish Species Associated with the Hazard of Ciguatera Fish Poisoning. College Park, MD: FDA.
van Walraven, L., Driessen, F., van Bleijswijk, J., Bol, A., Luttikhuizen, P. C., Coolen, J. W. P., et al. (2016). Where are the polyps? Molecular identification, distribution and population differentiation of Aurelia aurita jellyfish polyps in the southern North Sea area. Mar. Biol. 163:172. doi: 10.1007/s00227-016-2945-4
Villareal, T. A., Hanson, S., Qualia, S., Jester, E. L. E., Granade, H. R., and Dickey, R. W. (2007). Petroleum production platforms as sites for the expansion of ciguatera in the northwestern Gulf of Mexico. Harmful Algae 6, 253–259. doi: 10.1016/j.hal.2006.08.008
Vodopivec, M., Peliz, Á. J., and Malej, A. (2017). Offshore marine constructions as propagators of moon jellyfish dispersal. Environ. Res. Lett. 12:084003. doi: 10.1088/1748-9326/aa75d9
White, W. J., Schroeger, J., Drake, P. T., and Edwards, C. A. (2014). The value of larval connectivity information in the static optimization of marine reserve design. Conserv. Lett. 7, 533–544. doi: 10.1111/conl.12097
Wilson, C. A., and Nieland, D. L. (2001). Age and growth of red snapper, Lutjanus campechanus, from the Northern Gulf of Mexico off Louisiana. Fish. Bull. 99, 653–664.
Keywords: oil and gas platforms, red snapper, lionfish, regal demoiselle, Tubastrea, jellyfish blooms, ciguatera, fouling communities
Citation: Schulze A, Erdner DL, Grimes CJ, Holstein DM and Miglietta MP (2020) Artificial Reefs in the Northern Gulf of Mexico: Community Ecology Amid the “Ocean Sprawl”. Front. Mar. Sci. 7:447. doi: 10.3389/fmars.2020.00447
Received: 16 January 2020; Accepted: 20 May 2020;
Published: 12 June 2020.
Edited by:
Susana Carvalho, King Abdullah University of Science and Technology, Saudi ArabiaReviewed by:
Joanna Maria Pitt, Department of Environment and Natural Resources, BermudaNadav Shashar, Ben-Gurion University of the Negev, Israel
Copyright © 2020 Schulze, Erdner, Grimes, Holstein and Miglietta. This is an open-access article distributed under the terms of the Creative Commons Attribution License (CC BY). The use, distribution or reproduction in other forums is permitted, provided the original author(s) and the copyright owner(s) are credited and that the original publication in this journal is cited, in accordance with accepted academic practice. No use, distribution or reproduction is permitted which does not comply with these terms.
*Correspondence: Anja Schulze, c2NodWx6ZWFAdGFtdWcuZWR1