- 1School of Chemical Engineering and Technology, Tianjin University, Tianjin, China
- 2Tianjin Academy of Agricultural Sciences, Tianjin, China
Microalgae are a valuable and rich source of oil. By the means of using microalgae oil as raw materials or additives for edible oil will improve the nutritional and edible value of the latter. This paper compares the lipid content and fatty acid composition of microalgae and traditional oil crops, elaborates the lipid synthesis mechanism of microalgae cells and the strategies of promoting lipid accumulation both from environmental pressure and from molecular pressure, summarizes the methods of breaking microalgae cell walls and extracting oil, analyzes the nutritional value, toxicity, antioxidant, and economic feasibility issues to be considered in microalgae edible oil. It is hoped that this study could provide theoretical basis for the actual production of microalgae edible oil, and provide inspiration for the research on the synthesis, accumulation, extraction and preparation of microalgae oils.
Introduction
As population growth and the development of the biodiesel industry, traditional sources of edible oil have faced the challenge. Food researchers have focused on microalgae, an effective, sustainable and promising source of food and fuel supplies. Using microalgae as a source of edible oil save agricultural resources. They grow in non-cultivated land like seawater, salt water and even wastewater. With only sunlight and some nutrients, the autotrophic microalgae used for oil production can grow in large quantities in indoor photoreactors (Raheem et al., 2015; Chen et al., 2018). Microalgae are able to produce large amounts of oil per unit area, and their biomass productivity is 10 times higher than that of plankton and much higher than that of terrestrial biomass. Their fatty acid composition is the same as that of plant-derived oil, mainly C16 and C18 fatty acids. Some species are also rich in valuable ω-3 polyunsaturated fatty acids (PUFAs), which have antioxidant activity and cardiovascular benefits (Li X. et al., 2019; Suparmaniam et al., 2019; Katiyar and Arora, 2020). As a result, microalgae oil is considered as a functional oil with great commercial potential, and has the potential to be as a raw material for ordinary edible oil or as an added nutrient enhancer.
In recent years, there have been many studies on the production of biodiesel from microalgae (Ahmad et al., 2011b; Salam et al., 2016; Skorupskaite et al., 2016; Faried et al., 2017; Raheem et al., 2018; Sivaramakrishnan and Incharoensakdi, 2018; Srinuanpan et al., 2018a, b; Mofijur et al., 2019; Shomal et al., 2019). Biodiesel is a mixture of fatty acid alkyl esters (FAAE), most commonly methacrylate (FAME) or ethyl ester (FAEE), obtaining by alcoholysis of triacylglycerol (TAG) from microalgae oil (Halim et al., 2012). Studies are concentrated on the selection of oil-producing algae species (Nwokoagbara et al., 2015; Yu et al., 2015; Arroussi et al., 2017; Sadvakasova et al., 2019), fatty acid composition and fuel characteristics (Islam et al., 2017; Piloto-Rodríguez et al., 2017), upstream cultivation of microalgae for biodiesel production and downstream processing (Wang et al., 2016; Zhu et al., 2017, 2019; Kadir et al., 2018; Mathimani and Mallick, 2018; Tan et al., 2018; Goh et al., 2019; Yew et al., 2019; Yin et al., 2020), and analysis of economic feasibility and energy potential (Shin et al., 2018; Arcigni et al., 2019; Sun J. et al., 2019). Though there are few researches and evaluations on the production of microalgae edible oil.
This article studied the development prospect and preparation technology of edible oil from microalgae, compared the microalgae oil with vegetable oil to highlight the advantages of the former in the lipid productivity and fatty acid composition, introduced the microalgae cell lipid synthesis pathways and the method of the promoting intracellular lipid accumulation based on environmental pressure and molecular strategies, summarized the mechanical and non-mechanical methods to break microalgae cells, and analyzed organic solvent, ionic liquid, supercritical fluid, enzyme-assisted water and some other oil extraction methods, finally looked forward to the prospect of edible oils from microalgae.
The Oil Content and Fatty Acid Composition of Microalgae
Overview of Microalgae Lipids
Microalgae, one of the most primitive forms of plants, live in water systems. They are very small single-celled or simple multicellular photosynthetic microorganisms, having no leaves or roots, sizes <400 μm, and diameters usually 1–30 μm (Mata et al., 2010; Vassilev and Vassileva, 2016). Most algae are eukaryotes. The nuclei of eukaryotic microalgae are similar to those of higher plants, with intracellular organelles including chloroplasts for photosynthesis, endoplasmic reticulum (ER), golgi apparatus, mitochondria and vacuoles. The main biochemical components of microalgae are lipids, proteins, polysaccharides and nucleic acids (Sajjadi et al., 2018).
Microalgae cells typically contain 30–80% lipids (Japar et al., 2017; Deshmukh et al., 2019). Lipids are separate from polar and non-polar. Polar lipids (phospholipids and glycolipids etc.) are important components of cell membranes, while non-polar lipids (triglycerides and sterols etc.) are mostly used for intracellular energy storage (D’Alessandro and Antoniosi Filho, 2016). Microalgae have accumulated large amounts of non-polar lipid TAG, which are tiny droplets of three identical or different fatty acids molecules attached to glycerol. Moreover, in terms of fatty acid composition, microalga oil contains PUFAs with multiple double bonds. PUFAs are divided into different classes inclusive of ω-3 PUFAs and ω-6 PUFAs, which are, respectively, synthesized from linoleic acid and linolenic acid, and are essential for human health (Lorgeril and Salen, 2012; Jang and Park, 2019). The ω-3 PUFAs have received extensive attention owing to their physiological effects such as antioxidant activity, immune regulation, inflammation reduction, and prevention of neurological and cardiovascular diseases, especially the two most important α-linolenic acid derivatives, docosahexaenoic acid (DHA, C22: 6ω-3) and eicosapentaenoic acid (EPA, C20: 5ω-3) (Wu et al., 2015; Elagizi et al., 2018; Luo et al., 2018; Ferguson et al., 2019; Heshmati et al., 2019; Oikonomou et al., 2019; Ahmmed et al., 2020). It has been proved in animal experiments that intaking of diets rich in DHA and EPA in appropriate forms will enhance cognitive ability (Wen et al., 2016; Zhou et al., 2018), regulate metabolism (Asztalos et al., 2016; Bargut et al., 2017; Hussein et al., 2019) and have anti-inflammatory effects (Sierra et al., 2008; Calder, 2015; Alfaddagh et al., 2018). DHA is abundant in the brain and retina and plays a vital role in promoting the development of the brain and retina and maintaining membrane fluidity to ensure normal function (Bazan, 2007; Echeverría et al., 2017; Sun et al., 2017). DHA also ameliorates vascular health, participates in reducing inflammatory responses, prevents cancer, and protects the body from disease (Jung et al., 2013; Ozkan et al., 2016; Yum et al., 2016). EPA plays a beneficial role in fat metabolism, and prevents obesity, atherosclerosis and other diseases by improving fat metabolism (Laiglesia et al., 2016; Ding et al., 2017; Zhang et al., 2017).
Microalgae Lipids and Plant Oil
Compared with traditional vegetable oil, microalgae have become a potential new source of edible oil as its advantages in oil content and fatty acid composition. Table 1 (Balke and Diosady, 2000; Ogunniyi, 2006; Ong et al., 2011; Wang et al., 2012; Rondanini et al., 2014; Mukherjee and Ghosh, 2017; Dorni et al., 2018; Beyzi et al., 2019; Dehghan et al., 2019; Ebrahimian et al., 2019; Hossain et al., 2019; Xie et al., 2019, 2020; Liu et al., 2020; Tamagno et al., 2020) lists the oil content and fatty acid composition of some conventional oil crops. In general, the oil content of plant-derived crops is 20–60%, and the oil yield is generally less than 300 gal oil/acre (Sajjadi et al., 2018). Besides castor oil contain special up to about 87.0% of ricinoleic acid [C20:0 (OH)], palm oil and coconut oil, respectively, contain quite amount of palmitic acid (C16:0) and lauric acid (C12:0), most plant oil containing unsaturated fatty acids 70%, unsaturated fatty acids primarily oleic acid, linoleic acid, linolenic acid, and 20 carbon olefine acid. In addition to flaxseed oil, mustard oil and double-low rapeseed oil, vegetable oil contain relatively low ratios of ω-3 PUFAs, especially safflower oil, where the ratio of ω-6 PUFAs to ω-3 PUFAs reaches 631.59 (Ferreira et al., 2019).
The lipid content of microalgae varies according to different algae species and growth periods, usually between 20 and 50% biomass, and come up to 70% under certain culture conditions. Lipid productivity rather than lipid content is generally accepted as an indicator for evaluating the oil-producing performance of microalgae. Lipid content is the concentration of lipids in microalgae cells, regardless of the production of biomass; and lipid productivity depends on the production of biomass, and refers to the accumulation of lipids in cells in the total biomass produced. Microalgae with low lipid content have higher lipid productivity in the main, for instance, Chlorella sp. with only about 30% lipid content and lipid productivity exceeding 100 mg/L/day (Kiran et al., 2014). On account of the high lipid productivity of microalgae, the oil production of microalgae is able to achieve 6,000–15,000 gal oil/acre, far over that of vegetable oil (Sajjadi et al., 2018). Table 2 (Chisti, 2007; Bogen et al., 2013; Japar et al., 2017; Sajjadi et al., 2018; Shuba and Kifle, 2018; Deshmukh et al., 2019; Ferreira et al., 2019; Menegazzo and Fonseca, 2019). In terms of fatty acid composition, the content of PUFAs in microalgae is 20–60%. Some algal species, for example, Phaeodactylum tricornutum, Botryococcus braunii, and Dunaliella salina, contain DHA and EPA. Nevertheless, many vegetable oil contains only the precursor of both, α-linolenic acid, which has low conversion efficiency in human body (Gómez-Cortés and Camiña, 2019).
Lipid Synthesis and Accumulation in Microalgae
Metabolic Pathways for Microalgae Lipid Synthesis
The biosynthesis pathways of fatty acids and TAG in microalgae are similar to those in higher plants, including two stages of de novo synthesis of fatty acids and subsequent glyceride assembly (Supplementary Figure S1). The fatty acid synthesis begins in the chloroplasts in microalgae. Microalgae fix carbon dioxide into glycero-3-phosphate (G-3-P) through photosynthesis, and further convert it into acetyl coenzyme A (acetyl-CoA) through glycolysis, which is the direct precursor of fatty acid synthesis. In addition, the citric acid produced by sugar metabolism through the tricarboxylic acid (TCA) cycle in the mitochondria is also converted to acetyl-CoA under the action of ATP: citric acid cleavage synthase (ACL). In the cytoplasm, acetyl-CoA is activated by acetyl-CoA carboxylase (ACC) to generate malonyl coenzyme A (malonyl-CoA), which can be used for fatty acid elongation in the ER membrane. The conversion of acetyl-CoA to malonyl-CoA is the first step of fatty acid synthesis, which requires ATP. After the synthesis of malonyl-CoA in chloroplast, it is transferred to acyl carrier protein (ACP), and then goes through a four-step cycle of condensation, reduction, dehydration, and reduction (each cycle extends two carbon atoms), finally results in C16-ACP and C18-ACP. The elongation of C2 unit of each carbon chain demands 1 ATP and 2 NADPH molecules. On the one hand, acyl-ACP continues to synthesize lipids in chloroplast, which is considered as the prokaryotic pathway of lipid metabolism. On the other hand, acyl-ACP is dissolved into free fatty acids (FFA) under the action of fatty acyl-ACP thioesterase (FAT), FFA combine with the coenzyme A in the cytoplasm to regenerate the acyl-CoA, enter the ER with G-3-P through Kennedy pathway to generate lyso-phosphatidic acid (LPA), phosphatidic acid (PA), diacylglycerol (DAG) in turn, and become TAG in the end. There is a certain relationship between DAG, TAG, and membrane lipids (ML), this is eukaryotic way of lipid metabolism (Bellou et al., 2014; Arora et al., 2018; Mohan et al., 2019; Ran et al., 2019; Arif et al., 2020).
The ω-3 PUFAs are synthesized by both plastid and ER in the presence of specific elongases and desaturatase enzymes (Bellou et al., 2014). Synthesis of microalgae PUFAs begins with oleic acid, which is achieved by carbon chain elongation and desaturation of linoleic acid and linolenic acid (Supplementary Figure S2). In the traditionalω-3 PUFAs synthesis process, the eicosatetraenoic acid is generated by the Δ6 desaturation enzyme and elongation enzyme, and this process could also be completed by the Δ8 substitution process with the assistance of the Δ9 elongation enzyme and the Δ8 desaturation enzyme. Next, EPA is synthesized by the eicosatetraenoic acid under the action of Δ5 desaturation enzyme, and then DHA is synthesized by EPA under the Δ5 elongation enzyme and Δ4 desaturation enzyme (Guschina and Harwood, 2006; Gong et al., 2014; Hamilton et al., 2014).
Different substrates lead to different lipids synthesis of plant plasmids and ER. Plastid synthesis takes acyl-ACP as the substrates, and ER takes acyl-CoA as the substrates. Thus, it is possible to determine whether the synthesis site of glycerides is plastid or ER according to whether the fatty acyl group occupying the sn-2 position is 16 or 18 carbonyl groups. Unfortunately, reaction-related enzymes of microalgae have not been studied in detail, and the prokaryotic and eukaryotic pathways mentioned above may not be fully applicable to microalgae (Klok et al., 2014). Moreover, studies have identified at least six genes in the Chlamydomonas genome that presumably encode DGAT, and microalgae lipid synthesis may be much more complex than plant lipid synthesis for the reason of the wider substrate specificity of proteins (Liu and Benning, 2013). With the study of genome sequencing of microalgae, the differences between microalgae and plant lipid biosynthesis is more and more obvious. For example, the synthesis of TAG in microalgae usually takes place in photosynthetic cells, while that in plants mainly occurs in developing embryos and fruits. TAG synthesis of microalgae showed regionalized characteristics. Plasma membrane lipids are synthesized by plasmids, while extracellular lipids are synthesized by ER only. Due to the synthesis of DHA and EPA by microalgae cells, some aspects of desaturation and elongation of fatty acids are different from that of plants. Microalgae and plants have different fatty acyl groups, and the overall subcellular tissues of glyceride metabolism are different (Merchant et al., 2012; Liu and Benning, 2013; Klok et al., 2014; Zienkiewicz et al., 2016). Consequently, the lipid metabolism pathway of microalgae needs to be further studied.
Strategies of Microalgae Lipid Accumulation
When algae are subjected to environmental stress and modified at the molecular level, lipid metabolism is affected and accumulates (Supplementary Figure S3). Thus, microalgae can obtain high lipid content by changing the external culture conditions like strong light, extreme temperature, high salt, chemical induction, plant hormone regulation, and co-culture by using the unique symbiotic relationship between bacteria, fungi, and microalgae. Different stress factors mainly induce lipid accumulation in microalgae by changing ROS balance, carbon flow conversion and stress hormone levels, and regulating lipid synthesis and conversion (Chung et al., 2017; Sajjadi et al., 2018; Goh et al., 2019; Ran et al., 2019; Sun X. et al., 2019; Yew et al., 2019; Zhao et al., 2019). One of the most common and effective means is nutritional stress, specially nitrogen stress (Breuer et al., 2015). On the basis of nutritional stress, a two-stage culture method is developed. In the first stage, microalgae achieve high biomass concentration in nutrient-rich culture, and after harvesting biomass, in the second stage, microalgae attain lipid accumulation under environmental stress (Aziz et al., 2020). Furthermore, genetically engineering microalgae by over-expression, heterologous expression, gene knockout, and inserting certain genes to increase the supply of acetyl-CoA and NADPH precursor substances, and controlling signaling pathways by transcription factor can make the balance transfer in the direction of lipid synthesis, thereby enhance the synthesis of fatty acids and TAG, inhibit lipid β-oxidation and hydrolysis, and reduce starch, sterol and other competition way (Singh et al., 2016; Tandon and Jin, 2017; Arora et al., 2018; Park et al., 2019; Arif et al., 2020).
For biodiesel, it is more meaningful to increase the content of microalgae TAG, because the existence of PUFAs is not conducive to the stability of fuel. However, as edible oil, the development and utilization of PUFAs should also be considered. In the early stage of nitrogen depletion, starch accumulates; while in the late depletion, lipid accumulation. Under the appropriate environment, the growth curve of microalgae consist of four periods, namely stagnation, logarithmic, linear and stable. The stable stage is of high lipid content (Aziz et al., 2020). Different lipids also accumulate at different times and under different conditions: PUFAs need to be produced by low illumination at the beginning of the stationary period, while TAG will be produced by high illumination at the end of the stationary period (Gifuni et al., 2019). Studies have shown that the establishment of a reasonable carbon and nitrogen supply strategy, the provision of sufficient dissolved oxygen in the medium, the control of light, radiation and the addition of chemical substances are conducive to the accumulation of PUFAs (Ling et al., 2015; Chua and Schenk, 2017; Chen and Yang, 2018; Hu H. et al., 2019).
Extraction and Preparation of Edible Microalgae Oil
Cell Disruption
Despite the many advantages of microalgae, the commercial application of oil meets with formidable challenges for the high cost of downstream processing including the process of cell wall breaking and oil extraction. Microalgae cells are small and have a dense cell wall. Conventional mechanical pressing methods, which are widely applied to extracting vegetable oil, are not suitable for microalga. Direct solvent extraction or other methods to extract oil are also less efficient. Microalgae cell crushing methods are comprised of mechanical and non-mechanical methods (Supplementary Figure S4) (Grimi et al., 2014; Günerken et al., 2015; Lee et al., 2017; Onumaegbu et al., 2018; Goh et al., 2019; Hu Y. et al., 2019; Li X. et al., 2019; Mathimani and Mallick, 2019; bin Azmi et al., 2020; Katiyar and Arora, 2020; Sankaran et al., 2020). Commonly used mechanical cells broken pretreatment methods are mainly physical methods, such as ball mill, homogeneous and cavitation, but non-mechanical methods include chemical method and biological method. When selecting different methods, energy consumption and oil extraction effect should be considered. Mechanical methods have high energy consumption, high-pressure homogenization, high-speed homogenization and ultrasonic methods may cause lipid oxidation and seriously affect product quality, while chemical and biological methods have low energy consumption but the possibility of pollution, and may produce some by-products (Grimi et al., 2014; Günerken et al., 2015; Onumaegbu et al., 2018; Menegazzo and Fonseca, 2019). Combining complementary advantages of two or more methods with each other often gains better oil extraction effect (Duan et al., 2018; Phong et al., 2018). After cell fragmentation, fatty acid composition may also be affected. Previous studies have shown that mechanically broken cells increase the release of amphiphilic substances (FFA, lysophosphatidylcholine, etc.), which is conducive to emulsification (Rivera et al., 2018). Under alkaline conditions, hydrolase activity was enhanced, anaerobic hydrolysis was significantly improved, microalgae cell wall was degraded, and long chain fatty acids (LCFA) were accumulated (Qiu et al., 2020).
Oil Extraction
The most usual and simplest method to extract microalgae oil from broken cells is organic solvent extraction. At present, hexane and ethanol have been widely used in extracting edible oil, but chloroform, methane, benzene and other organic solvents are toxic and therefore not applicable. According to the similarity compatibility principle, non-polar solvents dissolve and destroy non-polar lipids on microalgae cell membrane to extract oil. There are not only monophasic solvents (mixtures of one or more polar/non-polar solvents and water miscible, e.g., hexane/ethanol), but also biphasic solvents (mixtures of two or more insoluble substances of different polarity, e.g., hexane in water) effective for the extraction of lipids (Liu et al., 2013; Yap et al., 2014). In a two-phase solvent, the two phases play their respective roles. Non-polar organic solvents destroy the hydrophobic interaction between non-polar/neutral lipids. Polar organic solvents destroy polar lipids and the electrostatic forces and hydrogen bonds between lipids and proteins.
For the reason that organic solvents are toxic, volatile, and difficult to recycle, some green solvents are also used, such as bio-based solvents, ionic liquids, convertible solvents, supercritical fluids, subcritical water, and pressurized solvents. The most extensively used technology is supercritical fluid extraction (SFE). Supercritical fluid is a fluid whose temperature and pressure are higher than its critical point. The most commonly used supercritical fluid is CO2, which is in gas phase under atmospheric conditions, so the solvent can be largely removed to form a solvent-free extract (Molino et al., 2020). CO2 is a non-polar solvent to extract non-polar compounds, so substances with opposite polarity, such as water, methanol, ethanol and other polar solvents, can be added as co-solvents to enhance the extraction effect (Albarelli et al., 2018; Patil et al., 2018). Typical supercritical CO2 (SC-CO2) extraction process is the compression and transmission of liquid CO2 into the feed pump, then heat the oven and push CO2 in supercritical state into the lipid extraction reaction kettle in the oven, where is also a metering valve to decompression in SC-CO2. Once the decompression completes, CO2 will escape from the oven to the environment in the form of gas, and the extracted lipids are collected in a special container (Chen and Walker, 2012). SCF is non-toxic, easy to recycle, and runs at low temperatures, but because of the high price of equipment and production, it current main application is in the production of biodiesel. Now it have accomplished that using dissolved in oil microalgae feed directly in the supercritical fluid to enzymatic interesterification reactor to produce biodiesel, without other expensive method of pumping equipment (Reddy et al., 2014; Drira et al., 2016; Patil et al., 2017; Taher et al., 2020). SCF is an effective alternative method for extracting natural bioactive ingredients (such as astaxanthin, carotenoids, etc.) in the food industry, and has been applied to decaffeination of coffee and extraction of hops, etc. (Krichnavaruk et al., 2008; Goto et al., 2015; Kwan et al., 2018; Rammuni et al., 2019). SCF is conducive to the extraction of unsaturated fatty acids. The unsaturated fatty acids in SC-CO2 extract are about 40%, while the traditional solvent extract is only 10–15% (Srivastava et al., 2018; de Melo et al., 2020). However, in the production of edible oil, economic and feasible strategies should be formulated according to the cost.
Hydroenzymatic method is a new type of gentle, low energy consumption, highly specific method that does not produce harmful or volatile organic compounds but produce many bioactive substances, which has attracted the attention of scholars in recent years (Sekhon et al., 2008; Gai et al., 2013; Liu et al., 2016; Nadar et al., 2018). Although, direct enzymatic cell degradation is not only costly but also inefficient, so the pretreatment methods mentioned above are often needed before hydroenzymatic oil extraction, such as ultrasound-assisted hydroenzymatic method, which has achieved good results in oil extraction (Hou et al., 2018). The water enzymatic oil extraction technology also has a problem, that is, the emulsifying layer will be formed in the process. In order to improve the oil extraction rate of the water enzymatic method, it is necessary to study the composition and properties of the emulsifying layer and choose appropriate methods for demulsification (Yusoff et al., 2015). Currently, demulsification methods used in the research include centrifugation, phase transformation, freezing and thawing, shearing, microfiltration and other physical and chemical methods (Ahmad et al., 2011a; Zolfaghari et al., 2016; Cai et al., 2019). Wu et al. (2009) used PH regulation and enzyme treatment to provide an effective strategy to destroy the stability of oil-rich emulsions formed during water extraction. Qian et al. (2019) used petroleum ether to eliminate the emulsification in water enzymatic extraction of soybean oil and enriched nearly 99% of the oil in the emulsion. Protein is a natural emulsifier and stabilizer, which can stabilize the emulsion at the oil/water interface. Protein–lipid interaction in the emulsion is closely related to the stability of the emulsion, and relevant mechanism research is still underway. Zhang and Lu (2015) studied the hydroenzymatic extraction of proteins from peanut oil emulsions, revealing the unique structural characteristics of peanut proteins adsorbed on the oil/water interface, as well as the structure-function relationship between enzymatic hydrolysis of proteins and their emulsifying properties. In the water enzymatic extraction of microalgae edible oil, the composition, spatial structure and chemical properties of the emulsion still need to be further studied to reveal the enzymatic hydrolysis and demulsification mechanism, further improve the oil extraction rate and reduce the production cost. Besides, there are other mild oil extraction methods in recent years, such as the three-phase distribution method (TPP) (Panadare and Rathod, 2017). Table 3 Enamala et al. (2018), Baskar et al. (2019), Karmakar and Halder (2019), Li P. et al. (2019), and Nagappan et al. (2019) shows the comparison of various methods for extracting microalgae oil.
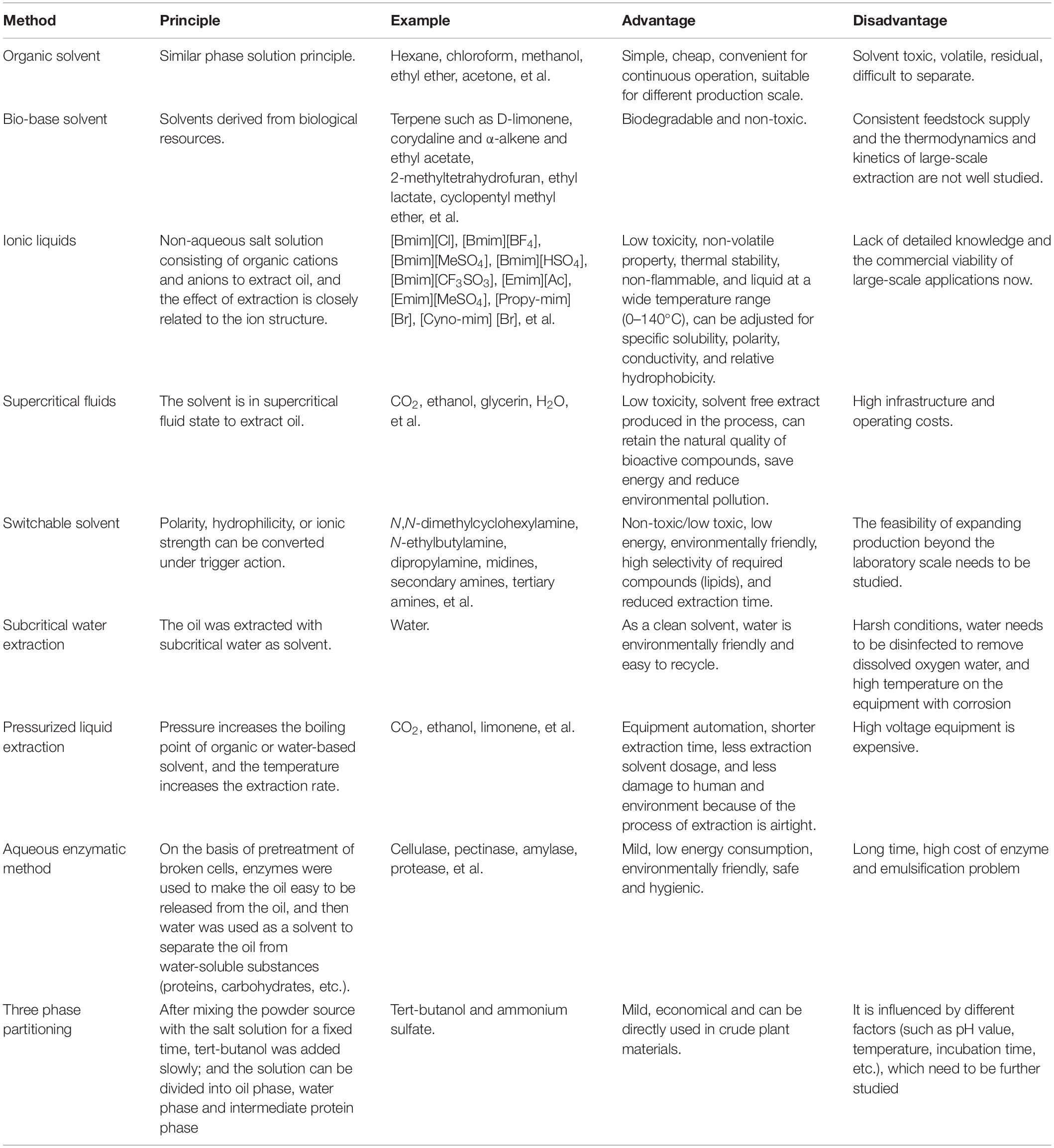
Table 3. Comparison of different lipid extraction methods of microalgae (Enamala et al., 2018; Baskar et al., 2019; Karmakar and Halder, 2019; Li P. et al., 2019; Nagappan et al., 2019).
Conclusion and Outlook
Firstly, the exploitation and utilization of microalgae in edible oil is mainly based on the nutritional value of microalgae. By comparing the fat content of some traditional oil materials and oil-producing algae strains above, and taking into account the lipid productivity of microalgae, we believe that microalgae is a sustainable and rich source of oil. In terms of fatty acid composition, as a result of the great difference of fatty acid composition in the cells of each algae species, some rich in ω-3 PUFAs and with reasonable composition of them can be selected for research and development of new food. To comprehensively evaluate the nutritional quality of microalgae, in addition to studying more biochemical components, it is also necessary to conduct in vitro simulated digestion experiments to test the digestibility of microalgae oil and evaluate its bioavailability (Niccolai et al., 2019). Currently, microalgae oil, particularly algal oil DHA and EPA, is mainly used as a nutritional fortifier for baby milk powder or health products, and is also added to candies, pasta and beverages (Wang et al., 2015; Rizwan et al., 2018). There are two applications of microalgae oil in edible oil: first, as the raw material of ordinary edible oil. The oil content and fatty acid composition of microalgae oil meet the raw material requirements of ordinary edible oil; Second, as a source of nutritional fortifiers in blending oils. The fatty acid composition of certain algal strains rich in ω-3 PUFAs should be studied in depth, and the processing methods of algal strains should be improved to purify and isolate physiological PUFAs such DHA and EPA as edible oil additives.
Additionally, we consider that the lipid synthesis pathway of microalgae cells is similar to but different from that of higher plants, and it has a unique PUFAs prolongation process. In present paper, the methods of promoting lipid accumulation, cell fragmentation and oil extraction in microalgae cells were introduced from the technical level. It is need to be considered the economic feasibility and commercial prospect of using microalgae as an alternative to conventional plant oil crops. Oleaginous microalgae with fast growth, high oil content, but the production cost is higher, and in the downstream processing, the costs of breaking the cell wall and extracting oil have increased than traditional plant source fuel. And we need improve the microalgae oil production technology from microalgae cultivation, cell broken, oil extraction, and oil processing to promote industrialization and application promotion of microalgae oil (Wase et al., 2018; Wang et al., 2019). A modern way to process microalgae is to build microalgae biorefineries, microalgae produce biodiesel and synthesis of a large number of high value compounds, such as pigment, vitamins, PUFAs, antioxidant and so on. Making full use of microalgae resources production is useful to meet energy, chemical, food, medicine, cosmetics, and other products in a wide range of industries. To reduce microalgae processing costs and achieve integrated microalgae product production, a comprehensive economic and environmental study on the production of high-value compounds from microalgae is required to develop a more economically feasible biorefining route (Lee and Chang, 2017; Mobin et al., 2019; Banu et al., 2020).
What’s more, the development of microalgae edible oil is different from biodiesel in that it is necessary to consider the edible safety of the oils, the chemical and physical properties of the products are very important, and toxicological studies of microalgae oils must be conducted to check for potential toxicity (including mutagenicity, systemic toxicity, reproductive and multigenerational toxicity), the possibility of risk levels of heavy metals and pathogenic microorganisms, and the safety of the oil production process (Draaisma et al., 2013). Several DHA rich algal species have been accepted as Generally Recognized as Safe (GRAS) by Food and Drug Administration (FDA), for an instance, Schizochytrium sp., Chlorella protothecoides, Ulkenia sp. SAM2179 et al. (Szabo et al., 2014). There have been several studies on the toxicological effects of microalgae oil (Kroes et al., 2003; Kagan and Matulka, 2015), but more evidence of safety is needed before microalgae oil can be widely used.
Last but not least, a common problem faced by edible oils is how to improve the oxidative stability and shelf life of algal oils. In particular, microalgae oil contain more PUFAs than normal oils, and thus have less antioxidant capacity. At present, the commonly used antioxidant means are adding natural or synthetic antioxidants to the oil, and the combined oxidants have better effect than the single oxidant, so further research should be conducted to optimize the formulation and mixture ratio of antioxidant combinations (Laguerre et al., 2007; Jacobsen et al., 2008; Gaffney et al., 2014; Shen et al., 2020). Ganiari et al. (2017) used edible and active films and coatings as carriers of natural antioxidants in lipid food, which provid a barrier for water, oxygen and solute movement in food and achieve good antioxidant effect. The latest research applied some nanotechnology to microencapsulate oil or make microemulsions, which not only plays a significant role in preventing oil oxidation, but also can improve the physical and nutritional properties of oil (Ziani et al., 2012; da Silva Santos et al., 2019; Sharma et al., 2019; Linke et al., 2020). Yet, as a new technical method, its production method, performance characterization, safety evaluation and many other aspects need to carry out extensive research.
Author Contributions
ZX and WY generally guided the topic selection and research content of the manuscript. YY undertook the main writing work of the manuscript. XG and YZ provided specific guidance and detailed revisions. All authors contributed to the article and approved the submitted version.
Conflict of Interest
The authors declare that the research was conducted in the absence of any commercial or financial relationships that could be construed as a potential conflict of interest.
Acknowledgments
Thanks to the contributions of Tianjin University and Tianjin Academy of Agricultural Science to assist the author.
Supplementary Material
The Supplementary Material for this article can be found online at: https://www.frontiersin.org/articles/10.3389/fmars.2020.00402/full#supplementary-material
References
Ahmad, A. L., Kusumastuti, A., Derek, C. J. C., and Ooi, B. S. (2011a). Emulsion liquid membrane for heavy metal removal: an overview on emulsion stabilization and destabilization. Chem. Eng. J. 171, 870–882. doi: 10.1016/j.cej.2011.05.102
Ahmad, A. L., Yasin, N. H. M., Derek, C. J. C., and Lim, J. K. (2011b). Microalgae as a sustainable energy source for biodiesel production: a review. Renew. Sustain. Energy Rev. 15, 584–593. doi: 10.1016/j.rser.2010.09.018
Ahmmed, M. K., Ahmmed, F., Tian, H., Carne, A., and Bekhit, A. E. D. (2020). Marine omega-3 (n-3) phospholipids: a comprehensive review of their properties, sources, bioavailability, and relation to brain health. Compr. Rev. Food Sci. Food Saf. 19, 64–123. doi: 10.1111/1541-4337.12510
Albarelli, Q. J., Santos, T. D., Ensinas, V. A., Maréchal, F., Cocero, M. J., and Meireles, M. A. A. (2018). Comparison of extraction techniques for product diversification in a supercritical water gasification-based sugarcane-wet microalgae biorefinery: thermoeconomic and environmental analysis. J. Clean. Prod. 201, 697–705. doi: 10.1016/j.jclepro.2018.08.137
Alfaddagh, A., Elajami, K. T., Saleh, M., Elajami, M., Bistrian, B. R., and Welty, F. K. (2018). The effect of eicosapentaenoic and docosahexaenoic acids on physical function, exercise, and joint replacement in patients with coronary artery disease: a secondary analysis of a randomized clinical trial. J. Clin. Lipidol. 12, 937–947. doi: 10.1016/j.jacl.2018.03.080
Arcigni, F., Friso, R., Collu, M., and Venturini, M. (2019). Harmonized and systematic assessment of microalgae energy potential for biodiesel production. Renew. Sustain. Energy Rev. 101, 614–624. doi: 10.1016/j.rser.2018.11.024
Arif, M., Bai, Y., Usman, M., Jalalah, M., Harraz, F. A., Al-Assiri, M. S., et al. (2020). Highest accumulated microalgal lipids (polar and non-polar) for biodiesel production with advanced wastewater treatment: role of lipidomics. Bioresour. Technol. 298:122299. doi: 10.1016/j.biortech.2019.122299
Arora, N., Pienkos, T. P., Pruthi, V., Poluri, K. M., and Guarnieri, M. T. (2018). Leveraging algal omics to reveal potential targets for augmenting TAG accumulation. Biotechnol. Adv. 36, 1274–1292. doi: 10.1016/j.biotechadv.2018.04.005
Arroussi, E. H., Benhima, R., Mernissi, E. N., Bouhfid, R., Tilsaghani, C., Bennis, I., et al. (2017). Screening of marine microalgae strains from Moroccan coasts for biodiesel production. Renew. Energy 113, 1515–1522. doi: 10.1016/j.renene.2017.07.035
Asztalos, B. I., Gleason, A. J., Sever, S., Gedik, R., Asztalos, B. F., Horvath, K. V., et al. (2016). Effects of eicosapentaenoic acid and docosahexaenoic acid on cardiovascular disease risk factors: a randomized clinical trial. Metabolism 65, 1636–1645. doi: 10.1016/j.metabol.2016.07.010
Aziz, M. M. A., Kassim, A. K., Shokravi, Z., Jakarni, F. M., Liu, H. Y., and Zaini, N. (2020). Two-stage cultivation strategy for simultaneous increases in growth rate and lipid content of microalgae: a review. Renew. Sustain. Energy Rev. 119:109621. doi: 10.1016/j.rser.2019.109621
Balke, T. D., and Diosady, L. L. (2000). Rapid aqueous extraction of mucilage from whole white mustard seed. Food Res. Int. 33, 347–356. doi: 10.1016/S0963-9969(00)00055-7
Banu, R. J., Kavitha, S. P., Gunasekaran, M., and Kumar, G. (2020). Microalgae based biorefinery promoting circular bioeconomy-techno economic and life-cycle analysis. Bioresour. Technol. 302:122822. doi: 10.1016/j.biortech.2020.122822
Bargut, T. C. L., Santos, L. P., Machado, D. G. L., Aguila, M. B., and Mandarim-de-Lacerda, C. A. (2017). Eicosapentaenoic acid (EPA) vs. docosahexaenoic acid (DHA): effects in epididymal white adipose tissue of mice fed a high-fructose diet. Prostaglandins Leukot. Essent. Fatty Acids 123, 14–24. doi: 10.1016/j.plefa.2017.07.004
Baskar, G., Kalavathy, G., Aiswarya, R., and Selvakumari, I. A. (2019). “7 – Advances in bio-oil extraction from nonedible oil seeds and algal biomass,” in Advances in Eco-Fuels for a Sustainable Environment, ed. A. K. Azad (Sawston: Woodhead Publishing), 187–210. doi: 10.1016/B978-0-08-102728-8.00007-3
Bazan, N. G. (2007). Homeostatic regulation of photoreceptor cell integrity: significance of the potent mediator neuroprotectin D1 biosynthesized from docosahexaenoic acid: the proctor lecture. Invest. Ophthalmol. Vis. Sci. 48, 4866–4881. doi: 10.1167/iovs.07-0918
Bellou, S., Baeshen, N. M., Elazzazy, M. A., Aggeli, D., Sayegh, F., and Aggelis, G. (2014). Microalgal lipids biochemistry and biotechnological perspectives. Biotechnol. Adv. 32, 1476–1493. doi: 10.1016/j.biotechadv.2014.10.003
Beyzi, E., Gunes, A., Beyzi, B. S., and Konca, Y. (2019). Changes in fatty acid and mineral composition of rapeseed (Brassica napus ssp. oleifera L.) oil with seed sizes. Ind. Crops Prod. 129, 10–14. doi: 10.1016/j.indcrop.2018.11.064
bin Azmi, A. A., Sankaran, R., Show, P. L., Ling, T. C., Tao, Y., Munawaroh, H. S. H., et al. (2020). Current application of electrical pre-treatment for enhanced microalgal biomolecules extraction. Bioresour. Technol. 302:122874. doi: 10.1016/j.biortech.2020.122874
Bogen, C., Klassen, V., Wichmann, J., Russa, M. L., Doebbe, A., Grundmann, M., et al. (2013). Identification of monoraphidium contortum as a promising species for liquid biofuel production. Bioresour. Technol. 133, 622–626. doi: 10.1016/j.biortech.2013.01.164
Breuer, G., Lamers, P. P., Janssen, M., Wijffels, R. H., and Martens, D. H. (2015). Opportunities to improve the areal oil productivity of microalgae. Bioresour. Technol. 186, 294–302. doi: 10.1016/j.biortech.2015.03.085
Cai, Q., Zhu, Z., Chen, B., and Zhang, B. (2019). Oil-in-water emulsion breaking marine bacteria for demulsifying oily wastewater. Water Res. 149, 292–301. doi: 10.1016/j.watres.2018.11.023
Calder, P. C. (2015). Marine omega-3 fatty acids and inflammatory processes: effects, mechanisms and clinical relevance. Biochim. Biophys. Acta 1851, 469–484. doi: 10.1016/j.bbalip.2014.08.010
Chen, C., and Yang, Y. (2018). Combining engineering strategies and fermentation technology to enhance docosahexaenoic acid (DHA) production from an indigenous Thraustochytrium sp. BM2 strain. Biochem. Eng. J. 133, 179–185. doi: 10.1016/j.bej.2018.02.010
Chen, J., Li, J., Dong, W., Zhang, X., Tyagi, D. R., Drogui, P., et al. (2018). The potential of microalgae in biodiesel production. Renew. Sustain. Energy Rev. 90, 336–346. doi: 10.1016/j.rser.2018.03.073
Chen, Y. H., and Walker, H. T. (2012). Fed-batch fermentation and supercritical fluid extraction of heterotrophic microalgal Chlorella protothecoides lipids. Bioresour. Technol. 114, 512–517. doi: 10.1016/j.biortech.2012.03.026
Chisti, Y. (2007). Biodiesel from microalgae. Biotechnol. Adv. 25, 294–306. doi: 10.1016/j.biotechadv.2007.02.001
Chua, T. E., and Schenk, M. P. (2017). A biorefinery for Nannochloropsis: induction, harvesting, and extraction of EPA-rich oil and high-value protein. Bioresour. Technol. 244, 1416–1424. doi: 10.1016/j.biortech.2017.05.124
Chung, Y. S., Lee, J. W., and Chung, C. H. (2017). Molecular challenges in microalgae towards cost-effective production of quality biodiesel. Renew. Sustain. Energy Rev. 74, 139–144. doi: 10.1016/j.rser.2017.02.048
da Silva Santos, V., Ribeiro, A. P. B., and Santana, M. H. A. (2019). Solid lipid nanoparticles as carriers for lipophilic compounds for applications in foods. Food Res. Int. 122, 610–626. doi: 10.1016/j.foodres.2019.01.032
D’Alessandro, B. E., and Antoniosi Filho, R. N. (2016). Concepts and studies on lipid and pigments of microalgae: a review. Renew. Sustain. Energy Rev. 58, 832–841. doi: 10.1016/j.rser.2015.12.162
de Melo, M. M. R., Sapatinha, M., Pinheiro, J., Lemos, M. F. L., Bandarra, H. M., Batista, I., et al. (2020). Supercritical CO2 extraction of Aurantiochytrium sp. biomass for the enhanced recovery of omega-3 fatty acids and phenolic compounds. J. CO2 Util. 38, 24–31. doi: 10.1016/j.jcou.2020.01.014
Dehghan, L., Golmakani, M. T., and Hosseini, S. M. H. (2019). Optimization of microwave-assisted accelerated transesterification of inedible olive oil for biodiesel production. Renew. Energy 138, 915–922. doi: 10.1016/j.renene.2019.02.017
Deshmukh, S., Kumar, R., and Bala, K. (2019). Microalgae biodiesel: a review on oil extraction, fatty acid composition, properties and effect on engine performance and emissions. Fuel Process. Technol. 191, 232–247. doi: 10.1016/j.fuproc.2019.03.013
Ding, L., Zhang, L., Wen, M., Che, H., Du, L., Wang, J., et al. (2017). Eicosapentaenoic acid-enriched phospholipids improve atherosclerosis by mediating cholesterol metabolism. J. Funct. Foods 32, 90–97. doi: 10.1016/j.jff.2017.02.020
Dorni, C., Sharma, P., Saikia, G., and Longvah, T. (2018). Fatty acid profile of edible oils and fats consumed in India. Food Chem. 238, 9–15. doi: 10.1016/j.foodchem.2017.05.072
Draaisma, B. R., Wijffels, H. R., Slegers, P. M. E., Brentner, L. B., Roy, A., and Barbosa, M. J. (2013). Food commodities from microalgae. Curr. Opin. Biotechnol. 24, 169–177. doi: 10.1016/j.copbio.2012.09.012
Drira, N., Piras, A., Rosa, A., Porcedda, S., and Dhaouadi, H. (2016). Microalgae from domestic wastewater facility’s high rate algal pond: lipids extraction, characterization and biodiesel production. Bioresour. Technol. 206, 239–244. doi: 10.1016/j.biortech.2016.01.082
Duan, P., Yang, S., Xu, Y., Wang, F., Zhao, D., Weng, Y. J., et al. (2018). Integration of hydrothermal liquefaction and supercritical water gasification for improvement of energy recovery from algal biomass. Energy 155, 734–745. doi: 10.1016/j.energy.2018.05.044
Ebrahimian, E., Seyyedi, M. S., Bybordi, A., and Damalas, C. A. (2019). Seed yield and oil quality of sunflower, safflower, and sesame under different levels of irrigation water availability. Agric. Water Manage. 218, 149–157. doi: 10.1016/j.agwat.2019.03.031
Echeverría, F., Valenzuela, R., Hernandez-Rodas, C. M., and Valenzuela, A. (2017). Docosahexaenoic acid (DHA), a fundamental fatty acid for the brain: new dietary sources. Prostaglandins Leukot. Essent. Fatty Acids 124, 1–10. doi: 10.1016/j.plefa.2017.08.001
Elagizi, A., Lavie, C. J., Marshall, K., DiNicolantonio, J. J., O’Keefe, J. H., and Milani, R. V. (2018). Omega-3 polyunsaturated fatty acids and cardiovascular health: a comprehensive review. Prog. Cardiovasc. Dis. 61, 76–85. doi: 10.1016/j.pcad.2018.03.006
Enamala, K. M., Enamala, S., Chavali, M., Donepudi, J., Yadavalli, R., Kolapalli, B., et al. (2018). Production of biofuels from microalgae – a review on cultivation, harvesting, lipid extraction, and numerous applications of microalgae. Renew. Sustain. Energy Rev. 94, 49–68. doi: 10.1016/j.rser.2018.05.012
Faried, M., Samer, M., Abdelsalam, E., Yousef, R., Attia, Y., and Ali, A. (2017). Biodiesel production from microalgae: processes, technologies and recent advancements. Renew. Sustain. Energy Rev. 79, 893–913. doi: 10.1016/j.rser.2017.05.199
Ferguson, J. F., Roberts-Lee, K., Borcea, C., Smith, H. M., Midgette, Y., and Shah, R. (2019). Omega-3 polyunsaturated fatty acids attenuate inflammatory activation and alter differentiation in human adipocytes. J. Nutr. Biochem. 64, 45–49. doi: 10.1016/j.jnutbio.2018.09.027
Ferreira, G. F., Ríos Pinto, L. F., Filho, R. M., and Fregolente, L. V. (2019). A review on lipid production from microalgae: association between cultivation using waste streams and fatty acid profiles. Renew. Sustain. Energy Rev. 109, 448–466. doi: 10.1016/j.rser.2019.04.052
Gaffney, M., O’Rourke, R., and Murphy, R. (2014). Manipulation of fatty acid and antioxidant profiles of the microalgae Schizochytrium sp. through flaxseed oil supplementation. Algal Res. 6, 195–200. doi: 10.1016/j.algal.2014.03.005
Gai, Q., Jiao, J., Wei, F., Luo, M., Wang, W., Zu, Y. G., et al. (2013). Enzyme-assisted aqueous extraction of oil from Forsythia suspense seed and its physicochemical property and antioxidant activity. Ind. Crops Prod. 51, 274–278. doi: 10.1016/j.indcrop.2013.09.014
Ganiari, S., Choulitoudi, E., and Oreopoulou, V. (2017). Edible and active films and coatings as carriers of natural antioxidants for lipid food. Trends Food Sci. Technol. 68, 70–82. doi: 10.1016/j.tifs.2017.08.009
Gifuni, I., Pollio, G., Safi, C., Marzocchella, A., and Olivieri, G. (2019). Current bottlenecks and challenges of the microalgal biorefinery. Trends Biotechnol. 37, 242–252. doi: 10.1016/j.tibtech.2018.09.006
Goh, B. H. H., Ong, H. C., Cheah, M. Y., Chen, W. H., Yu, K. L., and Mahlia, T. M. I. (2019). Sustainability of direct biodiesel synthesis from microalgae biomass: a critical review. Renew. Sustain. Energy Rev. 107, 59–74. doi: 10.1016/j.rser.2019.02.012
Gómez-Cortés, P., and Camiña, M. J. (2019). Oxidomics on the omega-3 volatile degradation pattern to determine differences between vegetable and marine oils. Food Res. Int. 122, 10–15. doi: 10.1016/j.foodres.2019.03.064
Gong, Y., Wan, X., Jiang, M., Hu, C., Hu, H., and Huang, F. (2014). Metabolic engineering of microorganisms to produce omega-3 very long-chain polyunsaturated fatty acids. Prog. Lipid Res. 56, 19–35. doi: 10.1016/j.plipres.2014.07.001
Goto, M., Kanda, H., Wahyu, D., and Machmudah, S. (2015). Extraction of carotenoids and lipids from algae by supercritical CO2 and subcritical dimethyl ether. J. Supercrit. Fluids 96, 245–251. doi: 10.1016/j.supflu.2014.10.003
Grimi, N., Dubois, A., Marchal, L., Lebovka, N. I., and Vorobiev, E. (2014). Selective extraction from microalgae Nannochloropsis sp. using different methods of cell disruption. Bioresour. Technol. 153, 254–259. doi: 10.1016/j.biortech.2013.12.011
Günerken, E., D’Hondt, E., Eppink, M. H. M., Garcia-Gonzalez, L., Elst, K., and Wijffels, R. H. (2015). Cell disruption for microalgae biorefineries. Biotechnol. Adv. 33, 243–260. doi: 10.1016/j.biotechadv.2015.01.008
Guschina, A. I., and Harwood, L. J. (2006). Lipids and lipid metabolism in eukaryotic algae. Prog. Lipid Res. 45, 160–186. doi: 10.1016/j.plipres.2006.01.001
Halim, R., Danquah, K. M., and Webley, A. P. (2012). Extraction of oil from microalgae for biodiesel production: a review. Biotechnol. Adv. 30, 709–732. doi: 10.1016/j.biotechadv.2012.01.001
Hamilton, L. M., Haslam, P. R., Napier, A. J., and Sayanova, O. (2014). Metabolic engineering of Phaeodactylum tricornutum for the enhanced accumulation of omega-3 long chain polyunsaturated fatty acids. Metab. Eng. 22, 3–9. doi: 10.1016/j.ymben.2013.12.003
Heshmati, J., Morvaridzadeh, M., Maroufizadeh, S., Akbari, A., Yavari, M., Amirinejad, A., et al. (2019). Omega-3 fatty acids supplementation and oxidative stress parameters: a systematic review and meta-analysis of clinical trials. Pharmacol. Res. 149:104462. doi: 10.1016/j.phrs.2019.104462
Hossain, Z., Johnson, N. E., Wang, L., Blackshaw, R. E., and Gan, Y. (2019). Comparative analysis of oil and protein content and seed yield of five Brassicaceae oilseeds on the Canadian prairie. Ind. Crops Prod. 136, 77–86. doi: 10.1016/j.indcrop.2019.05.001
Hou, K., Yang, X., Bao, M., Chen, F., Tian, H., and Yang, L. (2018). Composition, characteristics and antioxidant activities of fruit oils from Idesia polycarpa using homogenate-circulating ultrasound-assisted aqueous enzymatic extraction. Ind. Crops Prod. 117, 205–215. doi: 10.1016/j.indcrop.2018.03.001
Hu, H., Li, J., Pan, X., Zhang, F., Ma, L. L., Wang, H. J., et al. (2019). Different DHA or EPA production responses to nutrient stress in the marine microalga Tisochrysis lutea and the freshwater microalga Monodus subterraneus. Sci. Total Environ. 656, 140–149. doi: 10.1016/j.scitotenv.2018.11.346
Hu, Y., Gong, M., Feng, S., Xu, C., and Bassi, A. (2019). A review of recent developments of pre-treatment technologies and hydrothermal liquefaction of microalgae for bio-crude oil production. Renew. Sustain. Energy Rev. 101, 476–492. doi: 10.1016/j.rser.2018.11.037
Hussein, J., Attia, F. M., Bana, E. M., El-Daly, S. M., Mohamed, N., El-Khayat, Z., et al. (2019). Solid state synthesis of docosahexaenoic acid-loaded zinc oxide nanoparticles as a potential antidiabetic agent in rats. Int. J. Biol. Macromol. 140, 1305–1314. doi: 10.1016/j.ijbiomac.2019.08.201
Islam, A. M., Heimann, K., and Brown, J. R. (2017). Microalgae biodiesel: current status and future needs for engine performance and emissions. Renew. Sustain. Energy Rev. 79, 1160–1170. doi: 10.1016/j.rser.2017.05.041
Jacobsen, C., Let, M. B., Nielsen, S. N., and Meyer, A. S. (2008). Antioxidant strategies for preventing oxidative flavour deterioration of foods enriched with n-3 polyunsaturated lipids: a comparative evaluation. Trends Food Sci. Technol. 19, 76–93. doi: 10.1016/j.tifs.2007.08.001
Jang, H., and Park, K. (2019). Omega-3 and omega-6 polyunsaturated fatty acids and metabolic syndrome: a systematic review and meta-analysis. Clin. Nutr. 39, 765–773. doi: 10.1016/j.clnu.2019.03.032
Japar, S. A., Takriff, S. M., and Yasin, N. H. M. (2017). Harvesting microalgal biomass and lipid extraction for potential biofuel production: a review. J. Environ. Chem. Eng. 5, 555–563. doi: 10.1016/j.jece.2016.12.016
Jung, S. B., Kwon, S. K., Kwon, M., Nagar, H., Jeon, B. H., Irani, K., et al. (2013). Docosahexaenoic acid improves vascular function via up-regulation of SIRT1 expression in endothelial cells. Biochem. Biophys. Res. Commun. 437, 114–119. doi: 10.1016/j.bbrc.2013.06.049
Kadir, W. N. A., Lam, K. M., Uemura, Y., Lim, J. W., and Lee, K. T. (2018). Harvesting and pre-treatment of microalgae cultivated in wastewater for biodiesel production: a review. Energy Convers. Manag. 171, 1416–1429. doi: 10.1016/j.enconman.2018.06.074
Kagan, L. M., and Matulka, A. R. (2015). Safety assessment of the microalgae Nannochloropsis oculata. Toxicol. Rep. 2, 617–623. doi: 10.1016/j.toxrep.2015.03.008
Karmakar, B., and Halder, G. (2019). Progress and future of biodiesel synthesis: advancements in oil extraction and conversion technologies. Energy Convers. Manag. 182, 307–339. doi: 10.1016/j.enconman.2018.12.066
Katiyar, R., and Arora, A. (2020). Health promoting functional lipids from microalgae pool: a review. Algal Res. 46:101800. doi: 10.1016/j.algal.2020.101800
Kiran, B., Kumar, R., and Deshmukh, D. (2014). Perspectives of microalgal biofuels as a renewable source of energy. Energy Convers. Manag. 88, 1228–1244. doi: 10.1016/j.enconman.2014.06.022
Klok, A. J., Lamers, P. P., Martens, D. E., Draaisma, R. B., and Wijffels, R. H. (2014). Edible oils from microalgae: insights in TAG accumulation. Trends Biotechnol. 32, 521–528. doi: 10.1016/j.tibtech.2014.07.004
Krichnavaruk, S., Shotipruk, A., Goto, M., and Pavasant, P. (2008). Supercritical carbon dioxide extraction of astaxanthin from Haematococcus pluvialis with vegetable oils as co-solvent. Bioresour. Technol. 99, 5556–5560. doi: 10.1016/j.biortech.2007.10.049
Kroes, R., Schaefer, J. E., Squire, A. R., and Williams, G. M. (2003). A review of the safety of DHA45-oil. Food Chem. Toxicol. 41, 1433–1446. doi: 10.1016/S0278-6915(03)00163-7
Kwan, A. T., Kwan, E. S., Peccia, J., and Zimmerman, J. B. (2018). Selectively biorefining astaxanthin and triacylglycerol co-products from microalgae with supercritical carbon dioxide extraction. Bioresour. Technol. 269, 81–88. doi: 10.1016/j.biortech.2018.08.081
Laguerre, M., Lecomte, J., and Villeneuve, P. (2007). Evaluation of the ability of antioxidants to counteract lipid oxidation: existing methods, new trends and challenges. Prog. Lipid Res. 46, 244–282. doi: 10.1016/j.plipres.2007.05.002
Laiglesia, L. M., Lorente-Cebrián, S., Prieto-Hontoria, P. L., Fernández-Galilea, M., Ribeiro, S. M. R., Sáinz, N., et al. (2016). Eicosapentaenoic acid promotes mitochondrial biogenesis and beige-like features in subcutaneous adipocytes from overweight subjects. J. Nutr. Biochem. 37, 76–82. doi: 10.1016/j.jnutbio.2016.07.019
Lee, D. J., and Chang, J. S. (2017). Microalgae biorefinery: high value products perspectives. Bioresour. Technol. 229, 53–62. doi: 10.1016/j.biortech.2017.01.006
Lee, Y. S., Cho, M. J., Chang, K. Y., and Oh, Y. K. (2017). Cell disruption and lipid extraction for microalgal biorefineries: a review. Bioresour. Technol. 244, 1317–1328. doi: 10.1016/j.biortech.2017.06.038
Li, P., Sakuragi, K., and Makino, H. (2019). Extraction techniques in sustainable biofuel production: a concise review. Fuel Process. Technol. 193, 295–303. doi: 10.1016/j.fuproc.2019.05.009
Li, X., Liu, J., Chen, G., Zhang, J., Wang, C., and Liu, B. (2019). Extraction and purification of eicosapentaenoic acid and docosahexaenoic acid from microalgae: a critical review. Algal Res. 43:101619. doi: 10.1016/j.algal.2019.101619
Ling, X., Guo, J., Liu, X., Zhang, X., Wang, N., Lu, Y., et al. (2015). Impact of carbon and nitrogen feeding strategy on high production of biomass and docosahexaenoic acid (DHA) by Schizochytrium sp. LU310. Bioresour. Technol. 184, 139–147. doi: 10.1016/j.biortech.2014.09.130
Linke, A., Weiss, J., and Kohlus, R. (2020). Oxidation rate of the non-encapsulated- and encapsulated oil and their contribution to the overall oxidation of microencapsulated fish oil particles. Food Res. Int. 127:108705. doi: 10.1016/j.foodres.2019.108705
Liu, B., and Benning, C. (2013). Lipid metabolism in microalgae distinguishes itself. Curr. Opin. Biotechnol. 24, 300–309. doi: 10.1016/j.copbio.2012.08.008
Liu, C., Zheng, S., Xu, L., Wang, F., and Guo, C. (2013). Algal oil extraction from wet biomass of Botryococcus braunii by 1,2-dimethoxyethane. Appl. Energy 102, 971–974. doi: 10.1016/j.apenergy.2012.08.016
Liu, H., Zhang, L., Mei, L., Quampah, A., He, Q., Zhang, B., et al. (2020). qOil-3, a major QTL identification for oil content in cottonseed across genomes and its candidate gene analysis. Ind. Crops Prod. 145:112070. doi: 10.1016/j.indcrop.2019.112070
Liu, J., Gasmalla, M. A. A., Li, P., and Yang, R. (2016). Enzyme-assisted extraction processing from oilseeds: principle, processing and application. Innov. Food Sci. Emerg. Technol. 35, 184–193. doi: 10.1016/j.ifset.2016.05.002
Lorgeril, M. D., and Salen, P. (2012). New insights into the health effects of dietary saturated and omega-6 and omega-3 polyunsaturated fatty acids. BMC Med. 10:50. doi: 10.1186/1741-7015-10-50
Luo, C., Ren, H., Yao, X., Shi, Z., Liang, F., Kang, J. X., et al. (2018). Enriched brain omega-3 polyunsaturated fatty acids confer neuroprotection against microinfarction. EBioMedicine 32, 50–61. doi: 10.1016/j.ebiom.2018.05.028
Mata, M. T., Martins, A. A., and Caetano, S. N. (2010). Microalgae for biodiesel production and other applications: a review. Renew. Sustain. Energy Rev. 14, 217–232. doi: 10.1016/j.rser.2009.07.020
Mathimani, T., and Mallick, N. (2018). A comprehensive review on harvesting of microalgae for biodiesel – key challenges and future directions. Renew. Sustain. Energy Rev. 91, 1103–1120.
Mathimani, T., and Mallick, N. (2019). A review on the hydrothermal processing of microalgal biomass to bio-oil - knowledge gaps and recent advances. J. Clean. Prod. 217, 69–84. doi: 10.1016/j.jclepro.2019.01.129
Menegazzo, L. M., and Fonseca, G. G. (2019). Biomass recovery and lipid extraction processes for microalgae biofuels production: a review. Renew. Sustain. Energy Rev. 107, 87–107. doi: 10.1016/j.rser.2019.01.064
Merchant, S. S., Kropat, J., Liu, B., Shaw, J., and Warakanont, J. (2012). TAG, you’re it! Chlamydomonas as a reference organism for understanding algal triacylglycerol accumulation. Curr. Opin. Biotechnol. 23, 352–363. doi: 10.1016/j.copbio.2011.12.001
Mobin, S. M. A., Chowdhury, H., and Alam, F. (2019). Commercially important bioproducts from microalgae and their current applications – a review. Energy Procedia 160, 752–760. doi: 10.1016/j.egypro.2019.02.183
Mofijur, M., Rasul, M. G., and Hassan, N. M. S. (2019). Recent development in the production of third generation biodiesel from microalgae. Energy Procedia 156, 53–58. doi: 10.1016/j.egypro.2018.11.088
Mohan, S. V., Rohit, M. V., Subhash, G. V., Chandra, R., Devi, M. P., Butti, S. K., et al. (2019). “Chapter 12 – algal oils as biodiesel,” in Biomass, Biofuels, Biochemicals, Biofuels from Algae, 2nd Edn, eds A. Pandey, J.-S. Chang, C. R. Soccol, D.-J. Lee, and Y. Chisti (Amsterdam: Elsevier), 287–323. doi: 10.1016/B978-0-444-64192-2.00012-3
Molino, A., Mehariya, S., Sanzo, D. G., Larocca, V., Martino, M., Leone, G. P., et al. (2020). Recent developments in supercritical fluid extraction of bioactive compounds from microalgae: role of key parameters, technological achievements and challenges. J. CO2 Util. 36, 196–209. doi: 10.1016/j.jcou.2019.11.014
Mukherjee, S., and Ghosh, M. (2017). Studies on performance evaluation of a green plasticizer made by enzymatic esterification of furfuryl alcohol and castor oil fatty acid. Carbohydr. Polym. 157, 1076–1084. doi: 10.1016/j.carbpol.2016.10.075
Nadar, S. S., Rao, P., and Rathod, K. V. (2018). Enzyme assisted extraction of biomolecules as an approach to novel extraction technology: a review. Food Res. Int. 108, 309–330. doi: 10.1016/j.foodres.2018.03.006
Nagappan, S., Devendran, S., Tsai, P. C., Dinakaran, S., Dahms, H. U., and Ponnusamy, V. K. (2019). Passive cell disruption lipid extraction methods of microalgae for biofuel production – a review. Fuel 252, 699–709. doi: 10.1016/j.fuel.2019.04.092
Niccolai, A., Zittelli, C. G., Rodolfi, L., Biondi, N., and Tredici, M. R. (2019). Microalgae of interest as food source: biochemical composition and digestibility. Algal Res. 42:101617. doi: 10.1016/j.algal.2019.101617
Nwokoagbara, E., Olaleye, K. A., and Wang, M. (2015). Biodiesel from microalgae: the use of multi-criteria decision analysis for strain selection. Fuel 159, 241–249. doi: 10.1016/j.fuel.2015.06.074
Ogunniyi, D. S. (2006). Castor oil: a vital industrial raw material. Bioresour. Technol. 97, 1086–1091. doi: 10.1016/j.biortech.2005.03.028
Oikonomou, E., Vogiatzi, G., Karlis, D., Siasos, G., Chrysohoou, C., Zografos, T., et al. (2019). Effects of omega-3 polyunsaturated fatty acids on fibrosis, endothelial function and myocardial performance, in ischemic heart failure patients. Clin. Nutr. 38, 1188–1197. doi: 10.1016/j.clnu.2018.04.017
Ong, H. C., Mahlia, T. M. I., Masjuki, H. H., and Norhasyima, R. S. (2011). Comparison of palm oil, Jatropha curcas and Calophyllum inophyllum for biodiesel: a review. Renew. Sustain. Energy Rev. 15, 3501–3515. doi: 10.1016/j.rser.2011.05.005
Onumaegbu, C., Mooney, J., Alaswad, A., and Olabi, A. G. (2018). Pre-treatment methods for production of biofuel from microalgae biomass. Renew. Sustain. Energy Rev. 93, 16–26. doi: 10.1016/j.rser.2018.04.015
Ozkan, A., Parlak, H., Tanriover, G., Dilmac, S., Ulker, S. N., Birsen, I., et al. (2016). The protective mechanism of docosahexaenoic acid in mouse model of Parkinson: the role of heme oxygenase. Neurochem. Int. 101, 110–119. doi: 10.1016/j.neuint.2016.10.012
Panadare, D. C., and Rathod, V. K. (2017). Three phase partitioning for extraction of oil: a review. Trends Food Sci. Technol. 68, 145–151. doi: 10.1016/j.tifs.2017.08.004
Park, S., Nguyen, T. H. T., and Jin, E. (2019). Improving lipid production by strain development in microalgae: strategies, challenges and perspectives. Bioresour. Technol. 292:121953. doi: 10.1016/j.biortech.2019.121953
Patil, D. P., Dandamudi, K. P. R., Wang, J., Deng, Q., and Deng, S. (2018). Extraction of bio-oils from algae with supercritical carbon dioxide and co-solvents. J. Supercrit. Fluids 135, 60–68. doi: 10.1016/j.supflu.2017.12.019
Patil, D. P., Reddy, H., Muppaneni, T., and Deng, S. (2017). Biodiesel fuel production from algal lipids using supercritical methyl acetate (glycerin-free) technology. Fuel 195, 201–207. doi: 10.1016/j.fuel.2016.12.060
Phong, N. W., Show, L. P., Le, F. C., Tao, Y., Chang, J. S., and Ling, T. C. (2018). Improving cell disruption efficiency to facilitate protein release from microalgae using chemical and mechanical integrated method. Biochem. Eng. J. 135, 83–90. doi: 10.1016/j.bej.2018.04.002
Piloto-Rodríguez, R., Sánchez-Borroto, Y., Melo-Espinosa, A. E., and Verhelst, S. (2017). Assessment of diesel engine performance when fueled with biodiesel from algae and microalgae: an overview. Renew. Sustain. Energy Rev. 69, 833–842. doi: 10.1016/j.rser.2016.11.015
Qian, J., Tong, J., Chen, Y., Yao, S., Guo, H., and Yang, L. (2019). Study on lipids transfer in aqueous enzyme hydrolysis soybean protein and oil extraction process. Ind. Crops Prod. 137, 203–207. doi: 10.1016/j.indcrop.2019.04.063
Qiu, Y., Frear, C., Chen, S., Ndegwa, P., Harrison, J., Yao, Y., et al. (2020). Accumulation of long-chain fatty acids from Nannochloropsis salina enhanced by breaking microalgae cell wall under alkaline digestion. Renew. Energy 149, 691–700. doi: 10.1016/j.renene.2019.12.093
Raheem, A., Prinsen, P., Vuppaladadiyam, K. A., Zhao, M., and Luque, R. (2018). A review on sustainable microalgae based biofuel and bioenergy production: recent developments. J. Clean. Prod. 181, 42–59. doi: 10.1016/j.jclepro.2018.01.125
Raheem, A., Wan Azlina, W. A. K. G., Taufiq Yap, Y. H., Danquah, K. M., and Harun, R. (2015). Thermochemical conversion of microalgal biomass for biofuel production. Renew. Sustain. Energy Rev. 49, 990–999. doi: 10.1016/j.rser.2015.04.186
Rammuni, M. N., Ariyadasa, U. T., Nimarshana, P. H. V., and Attalage, R. A. (2019). Comparative assessment on the extraction of carotenoids from microalgal sources: astaxanthin from H. pluvialis and β-carotene from D. salina. Food Chem. 277, 128–134. doi: 10.1016/j.foodchem.2018.10.066
Ran, W., Wang, H., Liu, Y., Qi, M., Xiang, Q., Yao, C., et al. (2019). Storage of starch and lipids in microalgae: biosynthesis and manipulation by nutrients. Bioresour. Technol. 291:121894. doi: 10.1016/j.biortech.2019.121894
Reddy, K. H., Muppaneni, T., Patil, D. P., Ponnusamy, S., Cooke, P., Schaub, T., et al. (2014). Direct conversion of wet algae to crude biodiesel under supercritical ethanol conditions. Fuel 115, 720–726. doi: 10.1016/j.fuel.2013.07.090
Rivera, C. E., Montalescot, V., Viau, M., Drouin, D., Bourseau, P., Frappart, M., et al. (2018). Mechanical cell disruption of Parachlorella kessleri microalgae: impact on lipid fraction composition. Bioresour. Technol. 256, 77–85. doi: 10.1016/j.biortech.2018.01.148
Rizwan, M., Mujtaba, G., Memon, A. S., Lee, K., and Rashid, N. (2018). Exploring the potential of microalgae for new biotechnology applications and beyond: a review. Renew. Sustain. Energy Rev. 92, 394–404. doi: 10.1016/j.rser.2018.04.034
Rondanini, P. D., Castro, N. D., Searles, S. P., and Rousseaux, M. C. (2014). Contrasting patterns of fatty acid composition and oil accumulation during fruit growth in several olive varieties and locations in a non-Mediterranean region. Eur. J. Agron. 52, 237–246. doi: 10.1016/j.eja.2013.09.002
Sadvakasova, K. A., Akmukhanova, R. N., Bolatkhan, K., Zayadan, B., Usserbayeva, A., Bauenova, M., et al. (2019). Search for new strains of microalgae-producers of lipids from natural sources for biodiesel production. Int. J. Hydrogen Energy 44, 5844–5853. doi: 10.1016/j.ijhydene.2019.01.093
Sajjadi, B., Chen, W., Abdul, R. A. A., and Ibrahim, S. (2018). Microalgae lipid and biomass for biofuel production: a comprehensive review on lipid enhancement strategies and their effects on fatty acid composition. Renew. Sustain. Energy Rev. 97, 200–232. doi: 10.1016/j.rser.2018.07.050
Salam, A. K., Velasquez-Orta, B. S., and Harvey, P. A. (2016). A sustainable integrated in situ transesterification of microalgae for biodiesel production and associated co-product: a review. Renew. Sustain. Energy Rev. 65, 1179–1198. doi: 10.1016/j.rser.2016.07.068
Sankaran, R., Cruz, R. A. P., Pakalapati, H., Show, P. L., Ling, T. C., Chen, W. H., et al. (2020). Recent advances in the pretreatment of microalgal and lignocellulosic biomass: a comprehensive review. Bioresour. Technol. 298:122476. doi: 10.1016/j.biortech.2019.122476
Sekhon, K. J., Rosentrater, A. K., Jung, S., and Wang, T. (2008). Effect of co-products of enzyme-assisted aqueous extraction of soybeans, enzymes, and surfactant on oil recovery from integrated corn-soy fermentation. Ind. Crops Prod. 121, 441–451. doi: 10.1016/j.indcrop.2018.05.033
Sharma, S., Cheng, S., Bhattacharya, B., and Chakkaravarthi, S. (2019). Efficacy of free and encapsulated natural antioxidants in oxidative stability of edible oil: special emphasis on nanoemulsion-based encapsulation. Trends Food Sci. Technol. 91, 305–318. doi: 10.1016/j.tifs.2019.07.030
Shen, Y., Lu, T., Liu, X., Zhao, M. T., Yin, F. W., Rakariyatham, K., et al. (2020). Improving the oxidative stability and lengthening the shelf life of DHA algae oil with composite antioxidants. Food Chem. 313:126139. doi: 10.1016/j.foodchem.2019.126139
Shin, S. Y., Choi, I. H., Choi, W. J., Lee, J. S., Sung, Y. S., and Sim, S. J. (2018). Multilateral approach on enhancing economic viability of lipid production from microalgae: a review. Bioresour. Technol. 258, 335–344. doi: 10.1016/j.biortech.2018.03.002
Shomal, R., Hisham, H., Mlhem, A., Hassan, R., and Sulaiman, A. Z. (2019). Simultaneous extraction–reaction process for biodiesel production from microalgae. Energy Rep. 5, 37–40. doi: 10.1016/j.egyr.2018.11.003
Shuba, S. E., and Kifle, D. (2018). Microalgae to biofuels: ‘promising’ alternative and renewable energy, review. Renew. Sustain. Energy Rev. 81, 743–755. doi: 10.1016/j.rser.2017.08.042
Sierra, S., Lara-Villoslada, F., Comalada, M., Olivares, M., and Xaus, J. (2008). Dietary eicosapentaenoic acid and docosahexaenoic acid equally incorporate as decosahexaenoic acid but differ in inflammatory effects. Nutrition 24, 245–254. doi: 10.1016/j.nut.2007.11.005
Singh, P., Kumari, S., Guldhe, A., Misra, R., Rawat, I., and Bux, F. (2016). Trends and novel strategies for enhancing lipid accumulation and quality in microalgae. Renew. Sustain. Energy Rev. 55, 1–16. doi: 10.1016/j.rser.2015.11.001
Sivaramakrishnan, R., and Incharoensakdi, A. (2018). Microalgae as feedstock for biodiesel production under ultrasound treatment – a review. Bioresour. Technol. 250, 877–887. doi: 10.1016/j.biortech.2017.11.095
Skorupskaite, V., Makareviciene, V., and Gumbyte, M. (2016). Opportunities for simultaneous oil extraction and transesterification during biodiesel fuel production from microalgae: a review. Fuel Process. Technol. 150, 78–87. doi: 10.1016/j.fuproc.2016.05.002
Srinuanpan, S., Cheirsilp, B., and Prasertsan, P. (2018a). Effective biogas upgrading and production of biodiesel feedstocks by strategic cultivation of oleaginous microalgae. Energy 148, 766–774. doi: 10.1016/j.energy.2018.02.010
Srinuanpan, S., Cheirsilp, B., Prasertsan, P., Kato, Y., and Asano, Y. (2018b). Strategies to increase the potential use of oleaginous microalgae as biodiesel feedstocks: nutrient starvations and cost-effective harvesting process. Renew. Energy 122, 507–516. doi: 10.1016/j.renene.2018.01.121
Srivastava, G., Paul, K. A., and Goud, V. V. (2018). Optimization of non-catalytic transesterification of microalgae oil to biodiesel under supercritical methanol condition. Energy Convers. Manag. 156, 269–278. doi: 10.1016/j.enconman.2017.10.093
Sun, G. Y., Simonyi, A., Fritsche, K. L., Chuang, D. Y., Hannink, M., Gu, Z., et al. (2017). Docosahexaenoic acid (DHA): an essential nutrient and a nutraceutical for brain health and diseases. Prostaglandins Leukot. Essent. Fatty Acids 136, 3–13. doi: 10.1016/j.plefa.2017.03.006
Sun, J., Xiong, X., Wang, M., Du, H., Li, J., Zhou, D., et al. (2019). Microalgae biodiesel production in China: a preliminary economic analysis. Renew. Sustain. Energy Rev. 104, 296–306. doi: 10.1016/j.rser.2019.01.021
Sun, X., Ren, L., Zhao, Q., Zhang, L., and Huang, H. (2019). Application of chemicals for enhancing lipid production in microalgae-a short review. Bioresour. Technol. 293:122135. doi: 10.1016/j.biortech.2019.122135
Suparmaniam, U., Lam, K. M., Uemura, Y., Shuit, S., Lim, J. W., and Lee, K. T. (2019). Insights into the microalgae cultivation technology and harvesting process for biofuel production: a review. Renew. Sustain. Energy Rev. 115:109361. doi: 10.1016/j.rser.2019.109361
Szabo, N. J., Matulka, R. A., Marone, P. A., Bauter, M. R., Chan, T., Franklin, S., et al. (2014). Safety evaluation of oleic-rich triglyceride oil produced by a heterotrophic microalgal fermentation process. Food Chem. Toxicol. 65, 301–311. doi: 10.1016/j.fct.2013.12.048
Taher, H., Giwa, A., Abusabiekeh, H., and Al-Zuhair, S. (2020). Biodiesel production from Nannochloropsis gaditana using supercritical CO2 for lipid extraction and immobilized lipase transesterification: economic and environmental impact assessments. Fuel Process. Technol. 198:106249. doi: 10.1016/j.fuproc.2019.106249
Tamagno, S., Aznar-Moreno, A. J., Durrett, P. T., Prasad, P. V. V., Rotundo, J. L., and Ciampitti, I. O. (2020). Dynamics of oil and fatty acid accumulation during seed development in historical soybean varieties. Field Crops Res. 248:107719. doi: 10.1016/j.fcr.2020.107719
Tan, X. B., Lam, M. K., Uemura, Y., Lim, J. W., Wong, C. Y., and Lee, K. T. (2018). Cultivation of microalgae for biodiesel production: a review on upstream and downstream processing. Chin. J. Chem. Eng. 26, 17–30. doi: 10.1016/j.cjche.2017.08.010
Tandon, P., and Jin, Q. (2017). Microalgae culture enhancement through key microbial approaches. Renew. Sustain. Energy Rev. 80, 1089–1099. doi: 10.1016/j.rser.2017.05.260
Vassilev, V. S., and Vassileva, G. C. (2016). Composition, properties and challenges of algae biomass for biofuel application: an overview. Fuel 181, 1–33. doi: 10.1016/j.fuel.2016.04.106
Wang, J., Wang, X., Zhao, X., Liu, X., Dong, T., and Wu, F. A. (2015). From microalgae oil to produce novel structured triacylglycerols enriched with unsaturated fatty acids. Bioresour. Technol. 184, 405–414. doi: 10.1016/j.biortech.2014.09.133
Wang, J., Zhang, M., and Fang, Z. (2019). Recent development in efficient processing technology for edible algae: a review. Trends Food Sci. Technol. 88, 251–259. doi: 10.1016/j.tifs.2019.03.032
Wang, L., Raymer, P., Chinnan, M., and Pittman, R. N. (2012). Screening of the USDA peanut germplasm for oil content and fatty acid composition. Biomass Bioenergy 39, 336–343. doi: 10.1016/j.biombioe.2012.01.025
Wang, S., Zhu, J., Dai, L., Zhao, X., Liu, D., and Du, W. (2016). A novel process on lipid extraction from microalgae for biodiesel production. Energy 115, 963–968. doi: 10.1016/j.energy.2016.09.078
Wase, N., Black, P., and DiRusso, C. (2018). Innovations in improving lipid production: algal chemical genetics. Prog. Lipid Res. 71, 101–123. doi: 10.1016/j.plipres.2018.07.001
Wen, M., Xu, J., Ding, L., Zhang, L., Du, L., Wang, J., et al. (2016). Eicosapentaenoic acid-enriched phospholipids improve Aβ1–40-induced cognitive deficiency in a rat model of Alzheimer’s disease. J. Funct. Foods 24, 537–548. doi: 10.1016/j.jff.2016.04.034
Wu, J., Johnson, L. A., and Jung, S. (2009). Demulsification of oil-rich emulsion from enzyme-assisted aqueous extraction of extruded soybean flakes. Bioresour. Technol. 100, 527–533. doi: 10.1016/j.biortech.2008.05.057
Wu, Q., Zhou, T., Ma, L., Yuan, D., and Peng, Y. (2015). Protective effects of dietary supplementation with natural ω-3 polyunsaturated fatty acids on the visual acuity of school-age children with lower IQ or attention-deficit hyperactivity disorder. Nutrition 31, 7–8. doi: 10.1016/j.nut.2014.12.026
Xie, Y., Wei, F., Xu, S., Wu, B., Zheng, C., Lv, X., et al. (2019). Profiling and quantification of lipids in cold-pressed rapeseed oils based on direct infusion electrospray ionization tandem mass spectrometry. Food Chem. 285, 194–203. doi: 10.1016/j.foodchem.2019.01.146
Xie, Y., Yan, Z., Niu, Z., Coulter, J. A., Niu, J., Zhang, J., et al. (2020). Yield, oil content, and fatty acid profile of flax (Linum usitatissimum L.) as affected by phosphorus rate and seeding rate. Ind. Crops Prod. 145:112087. doi: 10.1016/j.indcrop.2020.112087
Yap, B. H. J., Crawford, A. S., Dumsday, J. G., Scales, P. J., and Martin, G. J. O. (2014). A mechanistic study of algal cell disruption and its effect on lipid recovery by solvent extraction. Algal Res. 5, 112–120. doi: 10.1016/j.algal.2014.07.001
Yew, G. Y., Lee, S. Y., Show, P. L., Tao, Y., Law, C. L., Nguyen, T. T. C., et al. (2019). Recent advances in algae biodiesel production: from upstream cultivation to downstream processing. Bioresour. Technol. Rep. 7:100227. doi: 10.1016/j.biteb.2019.100227
Yin, Z., Zhu, L., Li, S., Hu, T., Chu, R., Mo, F., et al. (2020). A comprehensive review on cultivation and harvesting of microalgae for biodiesel production: environmental pollution control and future directions. Bioresour. Technol. 301:122804. doi: 10.1016/j.biortech.2020.122804
Yu, N., Dieu, L. T. J., Harvey, S., and Lee, D. Y. (2015). Optimization of process configuration and strain selection for microalgae-based biodiesel production. Bioresour. Technol. 193, 25–34. doi: 10.1016/j.biortech.2015.05.101
Yum, H. W., Na, H. K., and Surh, Y. J. (2016). Anti-inflammatory effects of docosahexaenoic acid: implications for its cancer chemopreventive potential. Semin. Cancer Biol. 40, 141–159. doi: 10.1016/j.semcancer.2016.08.004
Yusoff, M. M., Gordon, H. M., and Niranjan, K. (2015). Aqueous enzyme assisted oil extraction from oilseeds and emulsion de-emulsifying methods: a review. Trends Food Sci. Technol. 41, 60–82. doi: 10.1016/j.tifs.2014.09.003
Zhang, L., Wang, D., Wen, M., Du, L., Xue, C., Wang, J., et al. (2017). Rapid modulation of lipid metabolism in C57BL/6J mice induced by eicosapentaenoic acid-enriched phospholipid from Cucumaria frondosa. J. Funct. Foods 28, 28–35. doi: 10.1016/j.jff.2016.10.022
Zhang, S., and Lu, Q. (2015). Characterizing the structural and surface properties of proteins isolated before and after enzymatic demulsification of the aqueous extract emulsion of peanut seeds. Food Hydrocoll. 47, 51–60. doi: 10.1016/j.foodhyd.2015.01.007
Zhao, Y., Wang, H., Han, B., and Yu, X. (2019). Coupling of abiotic stresses and phytohormones for the production of lipids and high-value by-products by microalgae: a review. Bioresour. Technol. 274, 549–556. doi: 10.1016/j.biortech.2018.12.030
Zhou, M., Ding, L., Wen, M., Che, H., Huang, J., Zhang, T., et al. (2018). Mechanisms of DHA-enriched phospholipids in improving cognitive deficits in aged SAMP8 mice with high-fat diet. J. Nutr. Biochem. 59, 64–75. doi: 10.1016/j.jnutbio.2018.05.009
Zhu, L., Li, S., Hu, T., Nugroho, Y. K., Yin, Z., Hu, D., et al. (2019). Effects of nitrogen source heterogeneity on nutrient removal and biodiesel production of mono- and mix-cultured microalgae. Energy Convers. Manag. 201:112144. doi: 10.1016/j.enconman.2019.112144
Zhu, L., Nugroho, Y. K., Shakeel, S. R., Li, Z., Martinkauppi, B., and Hiltunen, E. (2017). Using microalgae to produce liquid transportation biodiesel: what is next? Renew. Sustain. Energy Rev. 78, 391–400. doi: 10.1016/j.rser.2017.04.089
Ziani, K., Fang, Y., and McClements, J. D. (2012). Encapsulation of functional lipophilic components in surfactant-based colloidal delivery systems: vitamin E, vitamin D, and lemon oil. Food Chem. 134, 1106–1112. doi: 10.1016/j.foodchem.2012.03.027
Zienkiewicz, K., Du, Z., Ma, W., Vollheyde, K., and Benning, C. (2016). Stress-induced neutral lipid biosynthesis in microalgae-molecular, cellular and physiological insights. Biochim. Biophys. Acta 1861, 1269–1281. doi: 10.1016/j.bbalip.2016.02.008
Keywords: microalgae oil, edible oil, omega-3 PUFAs, oil accumulation, oil extraction
Citation: Xue Z, Yu Y, Yu W, Gao X, Zhang Y and Kou X (2020) Development Prospect and Preparation Technology of Edible Oil From Microalgae. Front. Mar. Sci. 7:402. doi: 10.3389/fmars.2020.00402
Received: 10 March 2020; Accepted: 08 May 2020;
Published: 18 June 2020.
Edited by:
Carla C. C. R. de Carvalho, Instituto Superior Técnico, PortugalReviewed by:
Tianzhong Liu, Qingdao Institute of Bioenergy and Bioprocess Technology (CAS), ChinaChanghong Yao, Sichuan University, China
Copyright © 2020 Xue, Yu, Yu, Gao, Zhang and Kou. This is an open-access article distributed under the terms of the Creative Commons Attribution License (CC BY). The use, distribution or reproduction in other forums is permitted, provided the original author(s) and the copyright owner(s) are credited and that the original publication in this journal is cited, in accordance with accepted academic practice. No use, distribution or reproduction is permitted which does not comply with these terms.
*Correspondence: Xiaohong Kou, kouxiaohong@tju.edu.cn
†These authors have contributed equally to this work