- 1Division of Pathology, Faculty of Veterinary Medicine, Department of Biomolecular Health Sciences, Utrecht University, Utrecht, Netherlands
- 2Diagnostic Imaging, Faculty of Veterinary Medicine, Department of Clinical Sciences, Utrecht University, Utrecht, Netherlands
- 3Veterinary Microbiologic Diagnostic Centre, Faculty of Veterinary Medicine, Department of Biomolecular Health Sciences, Utrecht University, Utrecht, Netherlands
- 4SOS Dolfijn Foundation, The Hague, Netherlands
- 5Department of Medical Microbiology, Radboud University Medical Center, Nijmegen, Netherlands
- 6Center of Expertise in Mycology, Radboudumc/CWZ, Nijmegen, Netherlands
Studies on the occurrence of fungal communities in the marine environment are still scarce, but mycotic infections in cetaceans are increasingly reported. Fungal disease following infection with Aspergillus species is most frequently reported, with the respiratory tract commonly affected in cetaceans and other taxa, like humans and birds. Infection with Aspergillus spp. is dependent on the characteristics of the fungus as well as the hosts immune status, with dissemination into other organs being relatively common. Along the southern North Sea, harbor porpoise (Phocoena phocoena) strandings increased significantly since 2005 and necropsies to determine causes of death have been conducted since 2008. Here we describe the post-mortem findings in stranded, free-ranging harbor porpoises on the Dutch coast which were diagnosed with fungal disease, to determine the prevalence of mycotic infections, and to compare them to those described in other species. A total of 18/754 (2.4%) harbor porpoises showed lesions compatible with localized or disseminated fungal disease as confirmed by histological examination. The respiratory tract was most commonly affected (67%), followed by the central nervous system (CNS, 33%), and auditory system (AS, 17%). Aspergillosis was confirmed for 11/18, by fungal growth (as A. fumigatus species complex, n = 9) and PCR (as Aspergillus spp., n = 1, and as A. fumigatus sensu strictu by sequence analysis, n = 1). One live stranded and euthanized animal presented partial hemiplegia of the blowhole and therefore an MRI was conducted, which resulted in a unique image of the extensive, fungus-induced lesion in the left cerebellar hemisphere, deforming and displacing the brainstem, and additionally affected the AS. The gross- and histologic lesions in the 18 porpoises diagnosed with fungal disease were similar to changes described in other mammalian species. The prevalence of fungal disease in free-living harbor porpoises is lower than seen in captive and rehabilitated animals, suggesting that captivity increases the risk to develop mycotic infections. Finally, fungal infection in the CNS and AS are usually considered consequences of vascular dissemination originating from pulmonary foci. However, only 1/7 cases with otitis and/or encephalitis demonstrated pulmonary aspergillosis, suggesting a different pathogenesis.
Introduction
Mycotic infections in wild cetaceans are assumed to be rare, however, increasingly reported in the last decades. This is most often following infection with Aspergillus species, mostly A. fumigatus, which is a ubiquitous filamentous, spore-producing, saprotrophic terrestrial fungus, and opportunistic pathogen (Seyedmousavi et al., 2015). Aspergillosis occurs in many species, including human beings, terrestrial and marine mammalian and avian hosts (Lass-Flörl et al., 2005; Balajee et al., 2009). Disease due to Aspergillus infection is variable, with targeted organs varying between species, but with the respiratory tract most commonly affected in human beings, birds, and cetaceans (summarized in Seyedmousavi et al., 2015). However, regardless of the primary infection site, dissemination into other organs is a relatively common sequence (Reidarson et al., 2001; Abdo et al., 2012). Infection with Aspergillus species seems to be dependent on the characteristics of the fungus as well as the hosts immune status (Seyedmousavi et al., 2015).
Aspergillus-associated lesions in free-ranging cetaceans include: occlusive tracheobronchitis in a striped dolphin (Stenella coeruleoalba; Grattarola et al., 2018); pneumonia and a focus of malacia in the cerebrum of a bottlenose dolphin (Tursiops truncatus; Cassle et al., 2016); localized pulmonary disease in an Atlantic spotted dolphin (Stenella frontalis; Groch et al., 2018); intracranial granuloma in a harbor porpoise (Phocoena phocoena; Dagleish et al., 2006); encephalitis in a northern bottlenose whale (Hyperoodon ampullatus; Dagleish et al., 2008) and in striped dolphins (Domingo et al., 1992); and otitis media in harbor porpoises (Seibel et al., 2010; Prahl et al., 2011). From an epidemiologic perspective, aspergillosis in free-ranging delphinids is often occurring with co-infection of dolphin morbillivirus (DMV; Cassle et al., 2016; Terio et al., 2018), but for other species, including the harbor porpoise, morbillivirus infections are reported only infrequently (Kennedy et al., 1991; De Swart et al., 1995, Müller et al., 2000).
Knowledge on the pathologic basis of disease and the understanding of causes of mortality in stranded marine mammals is vital for evaluating marine ecosystem health (Bossart, 2011). Studies regarding the occurrence of fungal communities and distribution in the marine environment are to date, however, still scarce and the presence of A. fumigatus in the North Sea is yet to be confirmed. Here, the harbor porpoise is the most abundant marine mammal (Hammond et al., 2002). A recent study has shown that stranding numbers in the southern North Sea raised significantly since 2005, with exact causes yet to be determined (IJsseldijk and ten Doeschate, 2019). Post-mortem examinations on stranded porpoises and the diagnosis of (emerging) infectious diseases, like aspergillosis, will aid in quantifying the health status of the harbor porpoises of the North Sea population. Therefore, the goals of this study were to describe the post-mortem findings in stranded harbor porpoises from the southern North Sea diagnosed with fungal disease, to determine the prevalence of mycotic infections, and to compare them to those described in other species.
Materials and Methods
Post-mortem Investigations
Necropsies were performed on 1282 harbor porpoises that were found dead along the Dutch coastline or were euthanized following live stranding based on welfare grounds from January 2009 to December 2019. Carcasses were transported to Utrecht University (UU), Faculty of Veterinary Medicine, Division of Pathology for immediate necropsy, or temporally stored at −20°C prior to further investigations. From live stranded animals, photo and video footage was collected at the location of stranding and prior to euthanasia. Animals that were rehabilitated following live stranding or those that were given any medication prior to euthanasia, were not included in this study.
A post-mortem examination was conducted following internationally standardized protocols (IJsseldijk et al., 2019). Each case was appointed a decomposition condition code (DCC), on a five-point scale with DCC1 representing very fresh carcasses, and DCC5 the skeletal remains of animals. Only animals in a relatively fresh condition (DCC1-3) for which histological examination were performed, were included in this study (n = 754). The minimal data collected of each case was: stranding date, location, sex, total body length, weight, and blubber thickness. The latter was measured immediately anterior to the dorsal fin at three locations (dorsal, lateral and ventral, in mm). Every individual was assigned a nutritional condition code (NCC), based on blubber thickness, muscularity, weight and the presence of subcutaneous and pleural fat, with NCC1 representing a very good nutritive condition, and NCC6 representing an extremely poor nutritional condition. Individuals were categorized into age classes based on total body length, with animals <90 cm classified as neonates, from 91 to 130 cm considered juveniles, and >130 cm classified as adults. Reproductive organs were assessed grossly in order to make a final differentiation between juveniles and adults.
Samples for histopathological evaluation varied on a case-to-case basis, but generally included skin, muscle, lung, heart, liver, adrenals, kidney, esophagus, stomach, intestine, pancreas, reproductive tissue, brain and spinal cord, spleen and various lymph nodes, and macroscopically identified lesions. Tissues were routinely fixed in 10% neutral buffered formalin and embedded in paraffin wax, sectioned at 5 μm, stained with hematoxylin and eosin (HE), and examined. Additional staining was performed, including periodic acid–Schiff (PAS) to confirm presence of fungal hyphae for respiratory tract tissue of fourteen cases (#1–10, #12, #14, #16, and #18), the central nervous system (CNS) of seven cases (#1, #7–8, #11–12, #15, and #17) and fourteen other tissue samples, including the skin (n = 3) and the auditory system (AS, n = 2), of nine cases (#1–4, #7, #9–10, and #13–15), and Grocott’s methenamine silver (GMS) stain of lung tissue from one case (#14) for better visualization of fungal organisms. In order to determine other pathological changes, Ziehl-Neelsen (ZN) stain on lung tissue (n = 5, #2, #9, #10, #12, and #14) and humerus (n = 1, after decalcification, and #14), Fite-Faraco (FF) stain on lung tissue of one animal (#2), and Von-Kossa (VK) stain of cerebellum of one animal (#2) were conducted (an overview is given in Supplementary Table 1).
Diagnostic Imaging
Post-mortem MRI examination of the head of one animal (#15) that was euthanized following live stranding was performed using a 1.5 Tesla scanner (Philips Ingenia; Philips Medical Systems Nederland B.V., Best, Netherlands) using a head coil. Images of the brain included transverse T2-weighted turbo spin echo [acquisition parameters repetition time (TR) 6852.36 ms, echo time (TE) 100 ms, flip angle 90°, matrix = 356 × 285, slice thickness/spacing = 5 mm/5 mm, field of view (FOV) 214 × 170 cm, and 34 interleaved axial slices]; transverse T1-weigthed spin echo (TR 450 ms, TE 15 ms, flip angle 69°, matrix = 284 × 271, slice thickness/spacing = 5 mm/5 mm, FOV = 214 × 170 cm, and 34 interleaved axial slices); T1 3D gradient echo (TR 9.37 ms, TE 4.61 ms, flip angle 8°, matrix = 232 × 230 slice thickness/spacing = 1 mm/1 mm, FOV = 230 × 171 cm, and 171 interleaved axial slices); transverse fluid attenuated inversion recovery (FLAIR; TR 11000 ms, TE = 140, flip angle 90°, matrix = 240 × 187, slice thickness/spacing = 5 mm/5 mm, FOV = 214 × 170 cm, and 34 interleaved axial slices); transverse T2∗ gradient echo (TR 947.76 ms, TE 13.81 ms, flip angle 18°, matrix = 328 × 266, slice thickness/spacing = 5 mm/5 mm, FOV = 214 × 170 cm, and 34 interleaved axial slices); and sagittal T2-weighted turbo spin echo (TR 4898.48 ms, TE 110 ms, flip angle 90°, matrix = 312 × 229, slice thickness/spacing = 2.5 mm/3.5 mm, FOV = 160 × 250 cm, and 44 interleaved axial slices). The MRI was evaluated by a veterinary diagnostic imaging resident (DSW) using a viewer of PACS (Impax, version 6.6.1.3004, N.V., Agfa Healthcare, Mortsel, Belgium).
Microbiological Investigation
Tissues from organs with macroscopic lesions related to suspect fungal disease, belonging to the respiratory tract, the CNS, the AS, along with liver, spleen, subcutis, and intestine, were analyzed at the Veterinary Microbiological Diagnostic Centre (VMDC) of UU. Tissue samples were cultured on Sabouraud and Malt agar plates (bioTRADING, Netherlands) and incubated at 30°C for up to 10 days. Fungal identification was based on colony characteristics of isolates and microscopic morphology on lactophenol cotton blue mount. When conventional identification was not possible, additional molecular analysis was performed by sequencing the nuclear ribosomal internal transcribed spacer (ITS) region using primers ITS1 (TCCGTAGGTGAACCTGCGG), and ITS4 (GGAAGTAAAAGTCGTAACAAGG; Schoch et al., 2012).
Culture negative samples were analyzed using a real-time PCR to detect Aspergillus DNA at the Department of Medical Microbiology of Radboudumc. Approximate 10 mm3 tissue samples were added to a 1.5 ml tube with MagNA Lyser Green Beads (Roche Diagnostics, Basel, Switzerland). To disrupt the fungal cell, tissue samples were lysed by bead beating twice. DNA was extracted with the MagNA Pure LC Total Nucleic Acid Kit (Roche Diagnostics, Basel, Switzerland). A real-time PCR was performed using Aspergillus specific primers (GCACGTGAAATTGTTGAAAGG and CAGGCTGGCCGCATTG) and probe (CATTCGTGCCGGTGTACTTCCCCG) using a LightCycler® 480 System (Roche Diagnostics, Basel, Switzerland) as previously described (Williamson et al., 2000; White et al., 2006).
Results
A total of 18/754 (2.4%) harbor porpoises were diagnosed with localized or disseminated fungal disease on the basis of gross findings correlating to histopathological mycotic changes, microbiological characterization, and molecular identification (Supplementary Tables 1, 2). These were twelve juveniles and six adult harbor porpoises, of which more males (n = 16) had fungal disease than females (n = 2). 15 animals were found dead, three animals live stranded, of which two were euthanized, and one animal died naturally. The first case was found in 2012, with yearly reports since: four cases in 2013, two in both 2014 and 2015, three in 2016, four in 2017, and one case in both 2018 and 2019 (Figure 1). Most animals were (very) fresh at the time of the necropsy, with six porpoises in DCC1 and eleven in DCC2, with the remaining animal in moderate state of decomposition (DCC3). Most animals were in a good nutritional condition (NCC1-2, n = 8), four presented a moderate nutritional condition (NCC3-4) and four porpoises a poor nutritive condition (NCC5) and the remaining two harbor porpoises were emaciated (NCC6; Table 1).
Gross Lesions
The respiratory tract was most commonly affected (12/18, 67%), followed by the CNS (6/18, 33%), and the AS (3/18, 17%; Table 2). Disease affecting multiple organ systems was seen in 3/18 animals (17%, #1, #8, and #13).
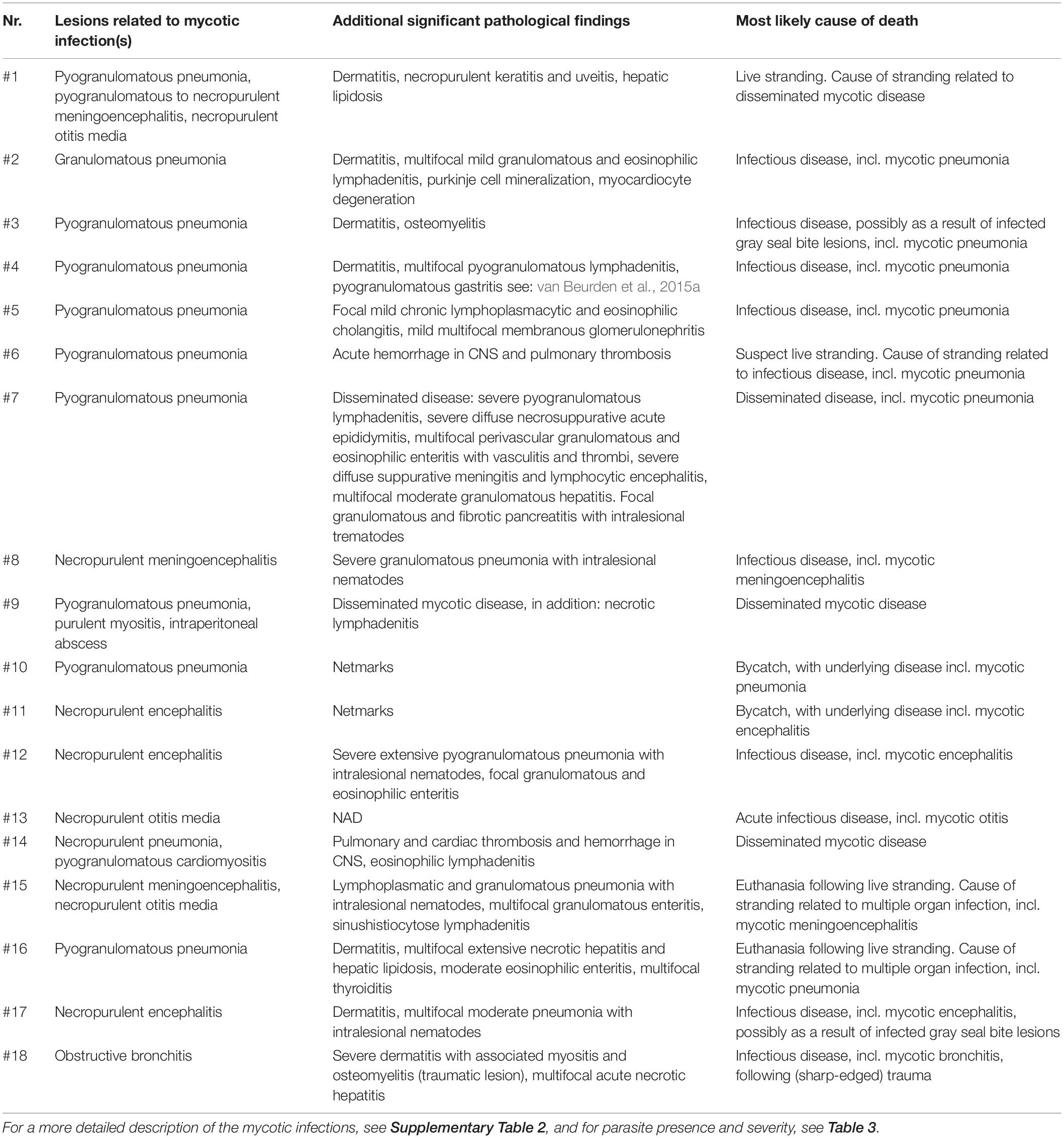
Table 2. An overview of lesions related to mycotic infections, additional significant pathological findings and most likely cause of death.
Nine cases (#1–3, #5–6, #9–10, #14, and #16) showed focal to multifocal granulomatous pneumonia with condensed foci varying in size (from pinpoint to 5 cm in diameter) and distribution. Two animals (#4, #7) presented a bilateral bronchopneumonia and one case (#18) showed severe narrowing of bronchi by granular, irregular plaques (Figure 2A). In all cases with respiratory involvement, the pulmonary and prescapular lymph nodes appeared edematous, and variably enlarged (2:1–3:1).
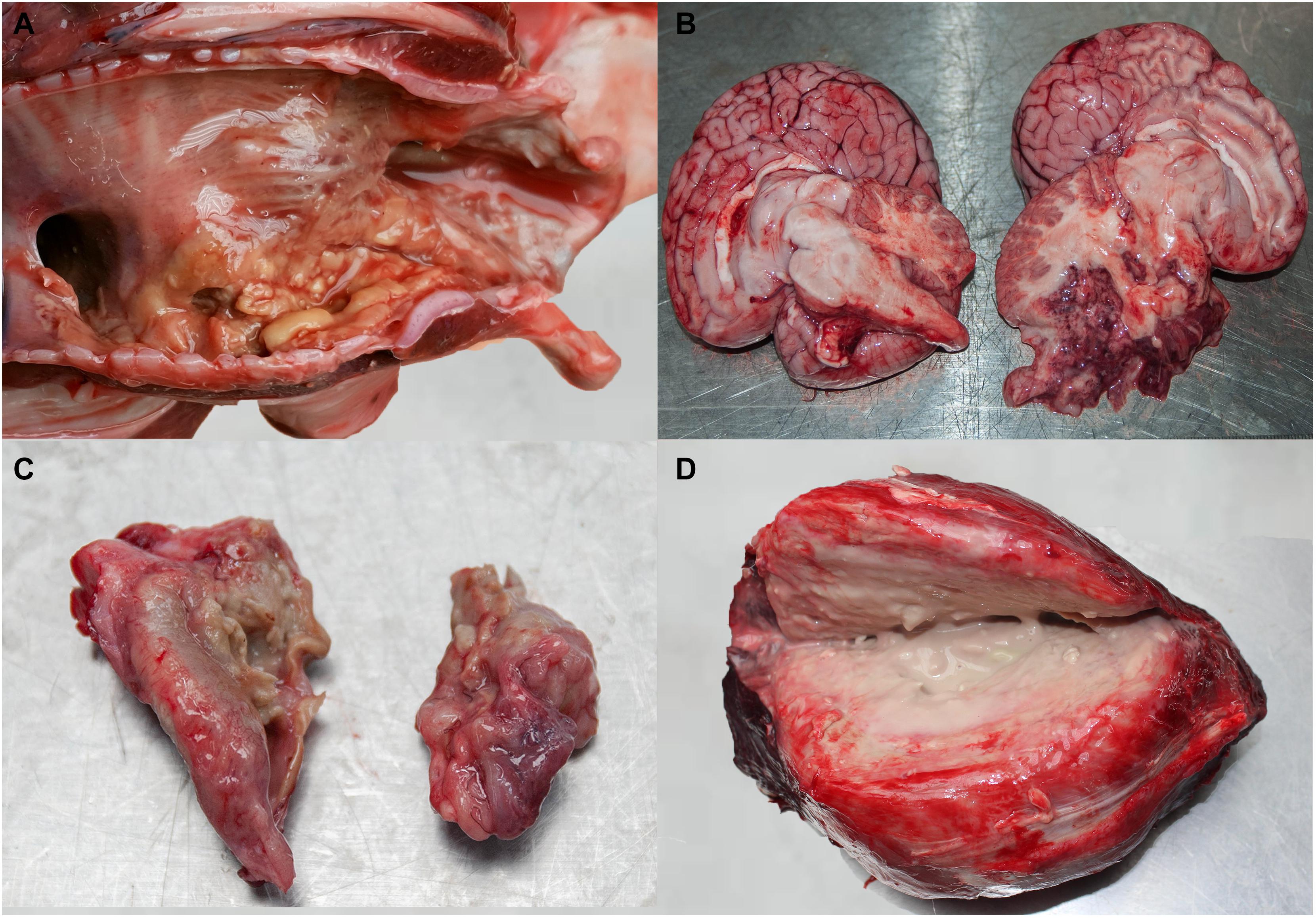
Figure 2. (A) Granular, irregular plaques in the bronchi of case #18. (B) Purulent meningoencephalitis demonstrated by purulent exudate in the cranium after removal of the brain in case #1. (C) Severe necro-purulent otitis in case #15 (left side). (D) Large, purulent and necrotic mass from abdominal cavity of case #9 (size: 20 × 20 × 15 cm).
Unilateral hemorrhage and lytic necrosis of the caudal part of the cerebrum (#11–12) and brainstem (#17) was visible in three animals. Purulent meningoencephalitis involving the cerebellum was evident in three cases (#1, #8, #15; Figure 2B). In two of these animals (#1, #15), as well as in another (#13) there was a severe necro-purulent otitis media (Figure 2C). One of these animals (#15) live stranded and during respiration, asymmetry of the blowhole musculature and a partial hemiplegia of the blowhole was observed (Supplementary Video).
In the three cases with disseminated disease (#1, #9, and #14), granulomatous foci and hemorrhages were present in heart, lungs, epaxial muscles, and the subcutis. One case (#9) had a large abscess (20 × 20 × 15 cm) in the abdominal cavity, dorsal to the intestine, which most likely originated from lymph nodes (Figure 2D and Supplementary Table 2). Parasitic presence related to respiratory tract, AS, liver, and gastrointestinal tract is presented in Table 3.
Histopathology
Intralesional hyphae were observed histologically in all eighteen harbor porpoises. Granulomatous to pyogranulomatous pneumonia with centrally located intralesional fungal hyphae was observed in ten animals (#1–7, #9–10, and #16). Intact and degenerated neutrophils and macrophages, surrounded by neutrophils and numerous epithelioid macrophages with small numbers of multinucleated giant cells often around a tertiary bronchus, were the predominant cell types in granulomas. The tissue reaction was purulent without obvious macrophages in one harbor porpoise (#14). In the case of obstructive bronchitis (#18; Figure 3A), the fungal invasion was restricted to the airways. The epithelium of affected bronchi was extensively necrotic and fungal hyphae had massively invaded the bronchial cartilage. In most cases, there were also cross sections of parasites present, notably not in the case with occlusive bronchitis (#18). The draining lymph nodes in all cases were reactive with follicle formation and sinusoidal histiocytosis.
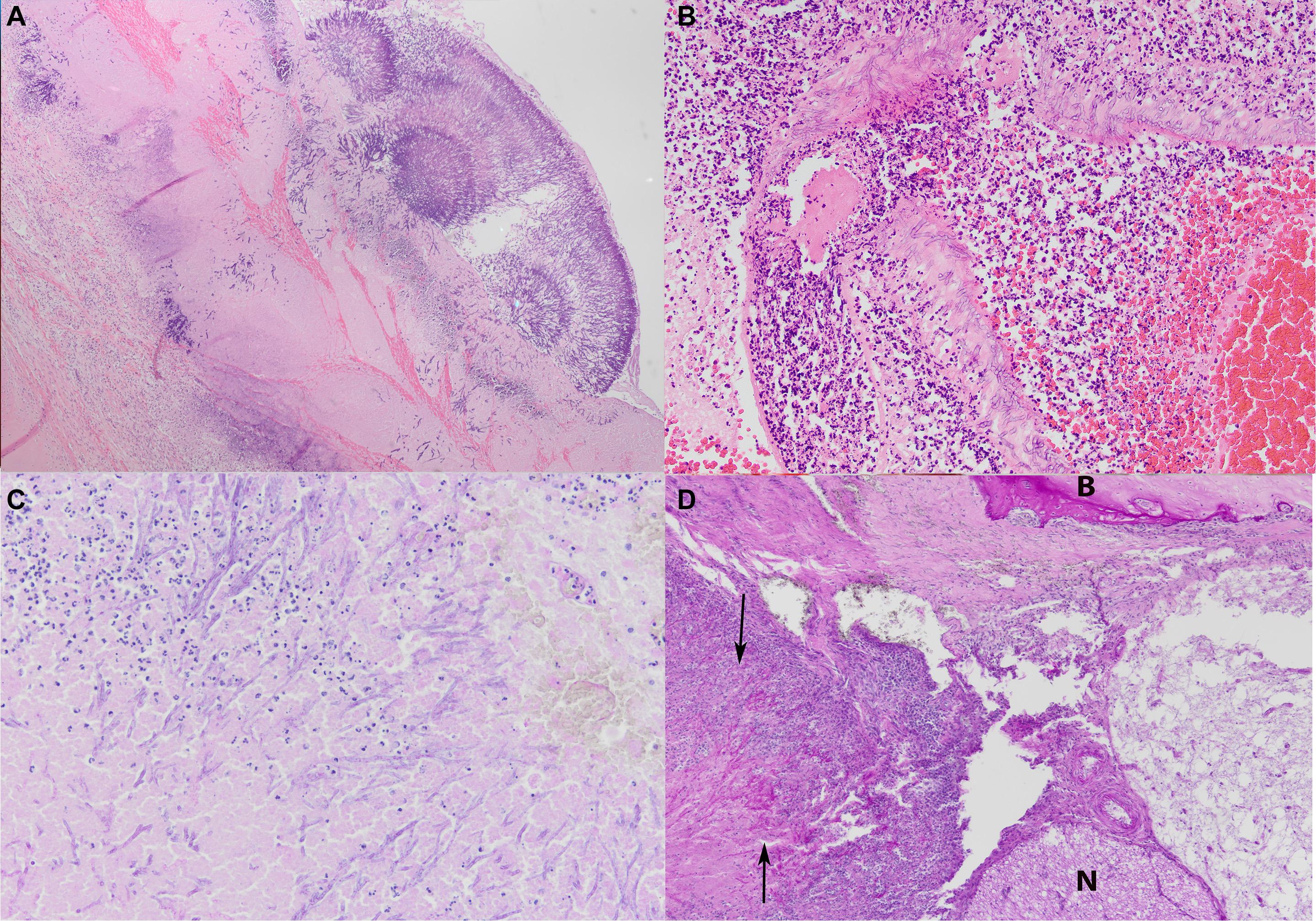
Figure 3. (A) Occlusive bronchitis in case #18 with extensive necrosis of the bronchial epithelium and presence of fungal hyphae into the bronchial cartilage (HE stain, microscopic magnification ×4). (B) Extensive necropurulent to pyogranulomatous vasculitis in cerebrum of case #17 (HE stain, microscopic magnification ×20). (C) Extensive necropurulent to pyogranulomatous meningoencephalitis of case #15 (PAS stain, microscopic magnification ×20). (D) Severe necropurulent otitis media with necrosis and erosion of the periotic bone, extensive fibrosis and inflammatory infiltrates (arrows) in case #15, with B indicating the bone tissue and N the nervous tissue (HE stain, microscopic magnification ×10).
Focally extensive necropurulent to pyogranulomatous inflammation affecting the cerebrum and/or the cerebellum was present in three cases with meningitis, that was characterized by the presence of thick hyphal plaques (#1, #8, and #15; Figure 3C). The cerebrum (#11–12) and brainstem (#17) in three cases presented with necropurulent encephalitis and extensive bleeding. There was abscess formation and extensive hemorrhage and intralesional fungal hyphae with vasculitis and thrombosis. Hyphae often invaded the vascular wall (Figure 3B) and vasculitis was commonly observed.
The AS of three animals (#1, #13, and #15) presented severe necropurulent otitis media with necrosis and erosion of the tympanoperiotic bone, extensive fibrosis and inflammatory infiltrates. The latter consisted of mainly degenerate neutrophils, with fewer lymphocytes, and occasionally many plasma cells. In two animals (#1, #15) this inflammation extended into the adjacent cerebellum. The expansion into adjacent bone was characterized by extensive osteolysis and hemorrhage admixed with fungal hyphae (Figure 3D).
The large abdominal abscess in one case (#9), which presumably originated from lymph nodes, presented histopathological changes comparable to those seen in the other infected organs, with extensive necrosis and presence of large amounts of fungal hyphae. Secondary findings, including the presence and severity of parasites, are given in Tables 2,3.
Diagnostic Imaging
The post-mortem MRI images (#15) revealed an extensive, ill-defined intra-axial lesion in the left cerebellar hemisphere Figure 4. The lesion was T2 hyperintense and T1 hypointense involving gray and white matter of almost the entire hemisphere. Multiple small signal voids (bleedings) were noted in the left hemisphere on the T2∗ sequence. An ill-defined T2-hyperintense zone was noted in the parenchyma of the adjacent brain stem. The lesion had a mass effect, deforming and displacing the brainstem to the right. The cerebellar midline was displaced to the right. The left tympanic bulla was filled with heterogeneous T2 hyperintense material, versus mainly hypo-intense content of the right middle ear. There was diffuse loss of bone in the region between the cerebellar lesion and the left ear, as observed during the gross pathological assessment. The bony loss was replaced by a rounded accumulation of heterogeneous tissue (2–2.5 cm), that was isointense to white matter. From these imaging findings it was concluded that the animal had suffered from an otitis media and interna with secondary granulomatous meningoencephalitis and osteomyelitis of the adjacent bone and cerebellum.
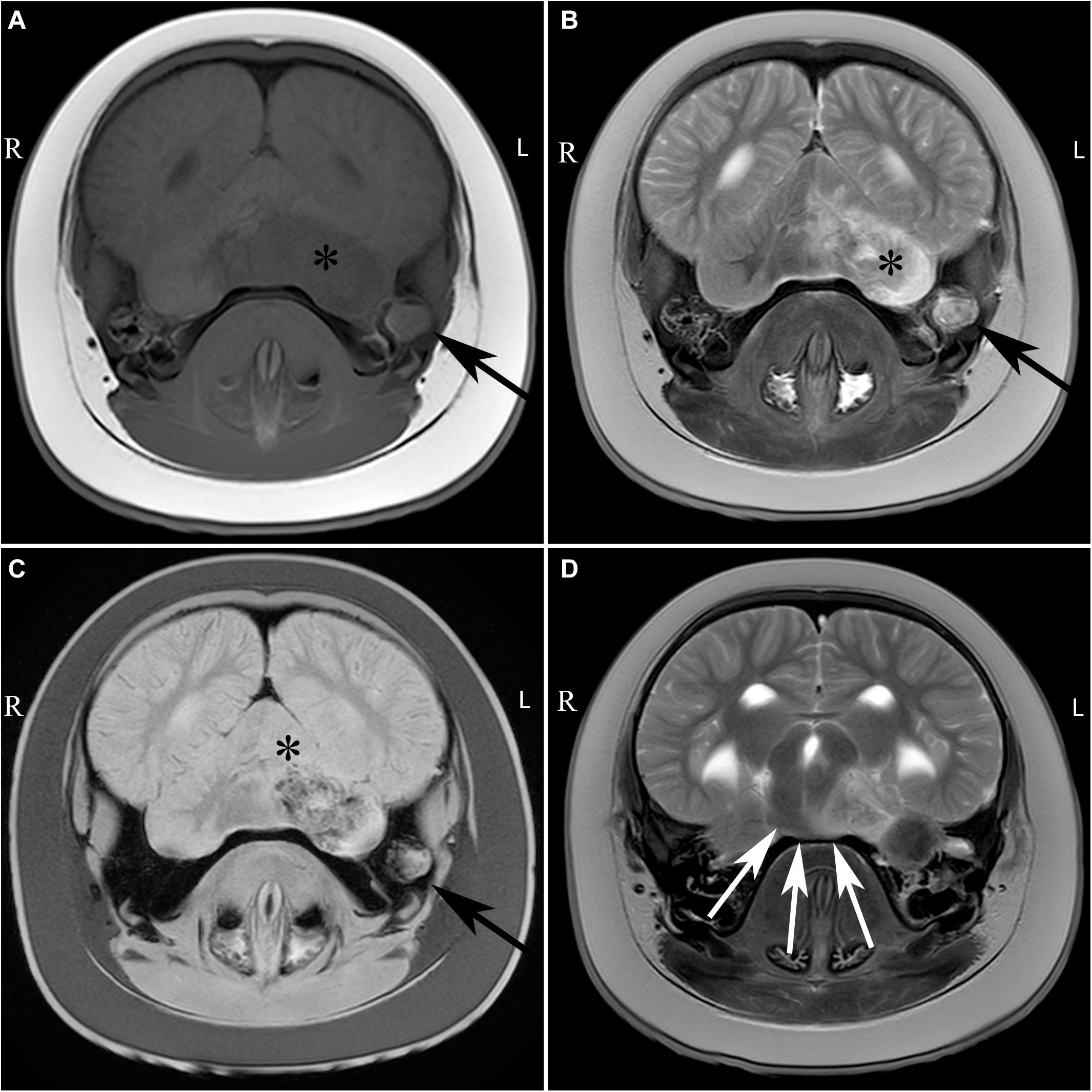
Figure 4. Transverse images of the brain of case #15 at the level of the cerebellum (A–C) and transverse image at the level of the mesencephalon (D), including T1-weigthed (A), T2 weighted (B,D) and T2∗ weighted (C) images. Large, ill-defined T2 hyperintense lesion affecting the left middle ear (black arrow), brainstem (white arrows), and cerebellum (asterisk) with T2∗ signal voids in the left cerebellar hemisphere. L, left; R, right.
Fungal Identification
The diagnosis of fungal disease was based on the occurrence of intralesional fungal elements in microscopic sections, which were present in all 18 cases. Fungal elements where characterized uniformly by hyphae of 6 to15 μm in diameter, that had parallel walls, were septated and branched acutely in a dichotomous manner. The hyphae appeared pale and eosinophilic in HE microscopic sections (Supplementary Table 1). In PAS-stained sections, hyphae appeared intensely positive with the exception of one case, where the fungus formed faintly stained spherules (#14). Here, a GMS stain was performed, in which the fungus stained black with multiple thick round to oval spherules of 6 to 12 μm, round to lemon-shaped yeast-like bodies with thick, refractile walls commonly arranged in rows and frequently germinated.
Culture was performed in 17/18 of the cases and was positive in 10/17. Nine fungal isolates showed rapid growth with green colored conidia. Microscopy showed a smooth conidiophore with a pear-shaped vesical. The upper 2/3 was covered with uniseriate phialides and round to oval spores specific for A. fumigatus species complex (Lamoth, 2016). One case (lung of #14) yielded an aspecific growth which could not be identified based on the macroscopic and microscopic characteristics and was therefore submitted for additional molecular analysis. The isolate was identified as A. fumigatus sensu strictu by sequence analysis of the nuclear ribosomal ITS region (Schoch et al., 2012). In addition, the cases that yielded negative culture results and one case for which no culture was attempted were submitted for molecular analysis. By Aspergillus PCR, one additional case of aspergillosis was confirmed: the cerebrum of case #8 (Table 4).
Discussion
Fungal infections were diagnosed on the basis of histopathological evaluation in 18/754 free-living harbor porpoises that were bycaught or stranded dead or alive on the Dutch coast. The mycotic infections of eleven of 18 cases were confirmed to be aspergillosis, either by fungal culture (n = 9), or by PCR (n = 2). Similar to changes described in other mammalian species, the associated lesions of aspergillosis consisted of varying numbers of neutrophils and macrophages, and tissue necrosis. This is typical tissue reaction to infection with Aspergillus spp., including A. fumigatus, making the harbor porpoise no different from other mammalian hosts. The most commonly affected organ system was the lower respiratory tract. This is in contrast to terrestrial mammals (except human beings), where other organ systems are targeted, and symptoms described (summarized in Seyedmousavi et al., 2015). Presumably this difference is a direct consequence of the specific anatomy and physiology of cetaceans. They lack nasal turbinates and thus cannot filter particles, which therefore more easily enter the deeper respiratory tract (St. Leger et al., 2009; Cozzi et al., 2017). In addition, the manner of respiration allows the deposition of particles (including pathogens) deep into the pulmonary tissue, thus making cetaceans more sensitive for significant and fatal pulmonary disease (St. Leger et al., 2009).
Most of the animals with respiratory tract fungal disease had additionally pulmonary parasites. Pulmonary parasitism, however, is very common in the study population, especially for juveniles and adults (ten Doeschate et al., 2017). Therefore, the presence and severity of parasites was considered to have no significant effect on the development of the fungal disease. Interestingly, the animal with obstructive bronchitis (#18) had no macroscopic or histologic evidence of pulmonary parasites. It remains speculation whether the presence or absence of pulmonary parasites has an influence on the character of the pathological changes, as this was observed in only one animal.
Encephalitis and otitis media as presentations of a fungal infection are usually considered to be consequences of vascular dissemination originating from pulmonary foci (Terio et al., 2018; van Elk et al., 2019). However, of the seven cases with encephalitis and/or otitis media, only one animal (#1) additionally had pulmonary fungal disease. It cannot be excluded with absolute certainty that fungal elements were missed during post-mortem investigation, however, as said elsewhere (van Elk et al., 2019) macroscopic and microscopic changes associated to aspergillosis are usually easily recognized. Therefore other possible explanations include clearance of pulmonary lesions or another port d‘entrée for the fungal spores (see below). Pathological changes were present in the brain and AS, either alone or in combination. Lesions were considered to be too advanced to give a reliable indication about their temporal relation.
It is assumed that the three cases with severe Aspergillus-induced otitis (#1, #13, and #15) suffered of unilateral hearing loss as a direct consequence. The inner ear of harbor porpoises carry expanded accessory tympanoperiotic air sinuses called pterygoid sacs (Racicot and Berta, 2013). These are homologous to guttural pouches in horses (Cozzi et al., 2017) representing air-filled extensions of the Eustachian tube that have openings into both the middle ear and oropharynx (Couetil and Hawkins, 2013). It has been suggested that air may be transferred from the lungs via the pharynx to the pterygoid sinuses while diving (Cozzi et al., 2017). That connection increases the probability of infection of the middle ear through the pterygoid sinuses, similar to the guttural pouch mycosis in horses. The most common route of infection is through inhalation of aerolized conidia from the neuston layer of the sea during surfacing, in areas with elevated presence of the fungus, since conspecific infection is thought to be rare (Dagleish et al., 2008), or from open cutaneous lesions. An additional partial paralysis of the blowhole musculature was observed in one of our cases (#15, Supplementary Video and Figure 4), that suffered from A. fumigatus infection which involved all components of the ear, and including the area of the exit of the cranial nerves at the skull base (Cozzi et al., 2017). The facial, hypoglossal, glossopharyngeal, vagal, and accessory cranial nerves exit the skull base in proximity to the middle ear (Cozzi et al., 2017). This could suggest that clinical signs such as those seen in horses could also occur in porpoises. The blowhole musculature is innervated by the facial nerves and is important for e.g. nasal vocalization and breathing motions (Huggenberger et al., 2009; Oelschläger et al., 2010). Dysfunction of the blowhole could lead to predisposition to aspiration pneumonia and dysfunction of the inner ear might have been responsible for insufficiency in foraging, communication and navigation of this animal, and thus could explain the live stranding. The importance of the assessment of the AS is increasingly stated (Morell et al., 2017; Wohlsein et al., 2019) and should be incorporated routinely in all post-mortem examinations.
In all the cases of mycotic infections we encountered microscopic presentation of fungal elements in form of septated hyphae with acute angle branching (except for #14), placing aspergillosis high in the list of differential diagnosis. Identification of Aspergillus spp. is indicative during histopathologic examination when invasive, 45°-branched, septate filaments are observed within characteristic (granulomatous) lesions (Desoubeaux et al., 2017). However, infections with Scedosporium spp. Fusarium spp. and several other fungal species have similar histopathological appearance (Guarner and Brandt, 2011). Therefore, definite diagnosis requires the presence of the typical hyphae in tandem with coniodiophores. In animal tissue, Aspergillus usually develops without producing conidial heads (Seyedmousavi et al., 2015) limiting the characterization to Aspergillus –like hyphae. In our study aspergillosis was established in 9/18 cases following consistent microscopic hyphae with positive culture and in 2/18 cases following consistent microscopic hyphae with positive PCR. In the remaining 7 cases the aspergillus-like hyphae were morphologically similar to the positive cases but culture and PCR were negative and therefore we cannot confirm infection with Aspergillus spp.
The prevalence of fungal disease in the free-living harbor porpoise population, bycaught in Dutch waters or stranded on the Dutch coast, was 2.4%. This is lower than the prevalence recently reported by van Elk et al. (2019), who diagnosed aspergillosis in nine of 61 (14%) harbor porpoises that stranded alive on the Dutch, German, and Belgian coasts. The fundamental difference with the animals of that study was that these were rehabilitated following live stranding. The time between initial stranding and death was not presented. In birds, rehabilitation has been associated with increased risk to develop aspergillosis, most likely due to the microenvironment and/or the diseased state of animals in rehabilitation (Burco et al., 2014). It is possible that similar factors play a role in disease development of mycotic infections in captive harbor porpoises, highlighting that caution should be taken when extrapolating such findings to free-living animals. Other studies reporting prevalence of aspergillosis in cetaceans are scarce. In a worldwide survey including both captive and wild marine mammals, aspergillosis accounted for 36% of all the fungal infections (Reidarson et al., 1998). On the Atlantic and Pacific coast, this prevalence was significantly lower with aspergillosis accounting for 0,5% of the fungal infections (Fenton et al., 2017). Two surveys investigating causes of death in harbor porpoises of the North Sea and a retrospective study in a population of bottlenose dolphins from the US marine mammal program had no records of aspergillosis (Siebert et al., 2001; Jauniaux et al., 2002, Venn-Watson et al., 2012). In a study in the United Kingdom on pulmonary pathology of harbor porpoises, a prevalence of 4% of mycotic infections was reported, without further typing of mycotic species given (Jepson et al., 2000). It is unclear what might have caused the difference in prevalence between these studies. Mycotic infections were diagnosed most frequently in juvenile male harbor porpoises in our study, and mainly in animals stranded in the Dutch Delta area (Zeeland), but it should be noted that this reflects stranding numbers in Netherlands (IJsseldijk and ten Doeschate, 2019).
In human beings, aspergillosis was once considered to be restricted to immunosuppressed individuals, but this association is no longer valid as cases have also been described in immunocompetent people (Seyedmousavi et al., 2015). The role of immunosuppression in the pathogenesis of aspergillosis in other mammals remains a point of discussion, and for harbor porpoises will remain uncertain as this involves the assessment of all factors that could result in immunosuppression. Aspergillosis in free-living cetaceans has often been reported in co-infection with morbillivirus (e.g., Domingo et al., 1992). In our animals, there was no indication for morbillivirus induced lesions and in general, morbillivirus is an uncommon pathogen in harbor porpoises (Kennedy et al., 1991; De Swart et al., 1995, Müller et al., 2000), however, this was not further assessed in our study and we therefore cannot fully rule out viral coinfections. There may be multiple other causes that could explain immunosuppression in harbor porpoises from the southern North Sea, which were also not further assessed in this study, and that do not necessarily affect the numbers of macrophages or polymorphonuclear leukocytes. These are the main cell populations responsible for the clearance of Aspergillus infections, and impairment of their function reduces their ability to contain the disease (Su et al., 2019). Malnutrition, other viral diseases, increased parasitic load, chemical pollutants and heavy metals are usually incriminated to induce immunosuppression, along with any kind of increased physiologic stress: all of which have previously been noted to affect porpoises in the North Sea (Jepson et al., 1999; Bennett et al., 2001, Murphy et al., 2015, van Beurden et al., 2015b, 2017). Burdens of chemical pollutants and heavy metals are found to exceed threshold levels for negative effects in at least a proportion of porpoises from the North Sea (Murphy et al., 2015; Jepson et al., 2016). Although decreased nutritional condition was present in some of the studied animals (Table 1), this was not consistently found. This suggests that, at least for some animals, the development of the lesions was rather quick and disruptive. Environmental degradation is thought to be related to lowering of cetacean population immune response, following disturbance of the balance between populations and pathogens (Van Bressem et al., 2009). This poses the harbor porpoises inhabiting the coastal North Sea, with their habitats altered by anthropogenic factors more severely than those of pelagic cetaceans, at higher risk of a range of infectious diseases, including those of fungal origin. Mycoses may therefore become more common in coastal cetacean species, and along with the rise of anthropogenic activities.
Data Availability Statement
All datasets generated for this study are included in the article/Supplementary Material.
Ethics Statement
Ethical review and approval was not required for the animal study because all animals in our study were found dead, died naturally or were euthanized based on welfare grounds and none of the animals were killed for the purpose of this study. Staff of the Division of Pathology of Utrecht University (UU) declare to have no role in the decision upon the fate of live stranded harbor porpoises in Netherlands. The SOS Dolfijn Foundation deals with live strandings under their permit number Wnb/OPV/2018/008.
Author Contributions
AK, LI, and AG contributed conception and design of the study. EE collected data from live stranded animals. LI, MK, and AG conducted the post-mortem investigations or reassessed previous records. DW conducted the diagnostic imaging. EB, JB, and PV conducted the microbiology. AK organized the database. AK, LI, and AG drafted the manuscript. DW, EB, JB, and PV wrote sections of the manuscript. All authors participated in revising of the manuscript. LI processed co-author comments. All authors gave final approval and agreed to be accountable for all aspects of work to ensure integrity and accuracy.
Funding
Post-mortem research on harbor porpoises in Netherlands is commissioned by the Dutch Ministry of Agriculture, Nature and Food Quality (2008–2015 under project reference number 140000353; 2016–2019 under project reference number WOT-04-009-045). No specific funding was acquired for this study.
Conflict of Interest
The authors declare that the research was conducted in the absence of any commercial or financial relationships that could be construed as a potential conflict of interest.
Acknowledgments
We would like to acknowledge the help of Jolanda Meerbeek and Annemarie van den Berg for transportation of the cases following euthanasia. In addition, we sincerely thank the stranding network volunteers and organizations for reporting and retrieving deceased harbor porpoises for our research. The investigations of the animals included in this study were conducted with the help of students and staff of the Division of Pathology of UU, for which we specifically acknowledge Lineke Begeman, Natashja Buijs, Marianthi Ioannidis, and Liliane Solé. In addition, we thank the staff at the Department of Clinical Sciences of UU, specifically Stefanie Veraa, for their assistance with the diagnostic imaging (MRI) conducted for this study. We also thank two reviewers for their helpful comments and suggestions on a previous manuscript version.
Supplementary Material
The Supplementary Material for this article can be found online at: https://www.frontiersin.org/articles/10.3389/fmars.2020.00344/full#supplementary-material
References
Abdo, W., Kawachi, T., Sakai, H., Fukushi, H., Kano, R., Shibahara, T., et al. (2012). Disseminated mycosis in a killer whale (Orcinus orca). J. Vet. Diagn. Investig. 24, 211–218. doi: 10.1177/1040638711416969
Balajee, S. A., Baddley, J. W., Peterson, S. W., Nickle, D., Varga, J., Boey, A., et al. (2009). Aspergillus alabamensis, a new clinically relevant species in the section Terrei. Eukaryot. Cell 8, 713–722. doi: 10.1128/EC.00272-08
Bennett, P. M., Jepson, P. D., Law, R. J., Jones, B. R., Kuiken, T., Baker, J. R., et al. (2001). Exposure to heavy metals and infectious disease mortality in harbour porpoises from England and Wales. Environ. Pollut. 112, 33–40. doi: 10.1016/s0269-7491(00)00105-6
Bossart, G. D. (2011). Marine mammals as sentinel species for oceans and human health. Vet. Pathol. 48, 676–690. doi: 10.1177/0300985810388525
Burco, J. D., Massey, J. G., Byrne, B. A., Tell, L., Clemons, K. V., and Ziccardi, M. H. (2014). Monitoring of fungal loads in seabird rehabilitation centers with comparisons to natural seabird environments in northern California. J. Zoo Wildlife Med. 45, 29–40. doi: 10.1638/2012-0051R1.1
Cassle, S. E., Landrau-Giovannetti, N., Farina, L. L., Leone, A., Wellehan, J. F. Jr., Stacy, N. I., et al. (2016). Coinfection by Cetacean morbillivirus and Aspergillus fumigatus in a juvenile bottlenose dolphin (Tursiops truncatus) in the Gulf of Mexico. J. Vet. Diagn. Investig. 28, 729–734. doi: 10.1177/1040638716664761
Couetil, L., and Hawkins, J. F. (2013). Respiratory Diseases of the Horse: A Problem-Oriented Approach to Diagnosis and Management. Boca Raton, FL: CRC Press.
Cozzi, B., Huggenberger, S., and Oelschläger, H. A. (2017). Anatomy of Dolphins: Insights into Body Structure and Function. Cambridge, MA: Academic Press.
Dagleish, M. P., Foster, G., Howie, F. E., Reid, R. J., and Barley, J. (2008). Fatal mycotic encephalitis caused by Aspergillus fumigatus in a northern bottlenose whale (Hyperoodon ampullatus). Vet. Rec. 163, 602–604. doi: 10.1136/vr.163.20.602
Dagleish, M. P., Patterson, I. A. P., Foster, G., Reid, R. J., Linton, C., and Buxton, D. (2006). Intracranial granuloma caused by asporogenic Aspergillus fumigatus in a harbour porpoise (Phocoena phocoena). Vet. Rec. 159:458. doi: 10.1136/vr.159.14.458
De Swart, R., Harder, T., Ross, P., Vos, H., and Osterhaus, A. (1995). Morbilliviruses and morbillivirus diseases of marine mammals. Infect. Agents Dis. 4, 125–130.
Desoubeaux, G., Le-Bert, C., Fravel, V., and Cray, C. (2017). Evaluation of a genus-specific ELISA and a commercial Aspergillus Western blot IgGR immunoblot kit for the diagnosis of aspergillosis in common bottlenose dolphins (Tursiops truncatus). Med. Mycol. 57, 847–856. doi: 10.1093/mmy/myx114
Domingo, M., Visa, J., Pumarola, M., Marco, A. J., Ferrer, L., Rabanal, R., et al. (1992). Pathologic and immunocytochemical studies of morbillivirus infection in striped dolphins (Stenella coeruleoalba). Vet. Pathol. 29, 1–10. doi: 10.1177/030098589202900101
Fenton, H., Daoust, P. Y., Forzán, M. J., Vanderstichel, R. V., Ford, J. K., Spaven, L., et al. (2017). Causes of mortality of harbor porpoises Phocoena phocoena along the Atlantic and Pacific coasts of Canada. Dis. Aquat. Organ. 122, 171–183. doi: 10.3354/dao03080
Grattarola, C., Giorda, F., Iulini, B., Pautasso, A., Ballardini, M., Zoppi, S., et al. (2018). Occlusive mycotic tracheobronchitis and systemic Alphaherpesvirus coinfection in a free-living striped dolphin Stenella coeruleoalba in Italy. Dis. Aquat. Organ. 127, 137–144. doi: 10.3354/dao03190
Groch, K. R., Díaz-Delgado, J., Sacristán, C., Oliveira, D. E., Souza, G., Sánchez-Sarmiento, A. M., et al. (2018). Pulmonary and systemic fungal infections in an Atlantic spotted dolphin and a Bryde’s whale, Brazil. Dis. Aquat. Organ. 128, 73–79. doi: 10.3354/dao03207
Guarner, J., and Brandt, M. E. (2011). Histopathologic diagnosis of fungal infections in the 21st century. Clin. Microbiol. Rev. 24, 247–280. doi: 10.1128/CMR.00053-10
Hammond, P. S., Berggren, P., Benke, H., Borchers, D. L., Collet, A., Heide-Jørgensen, M. P., et al. (2002). Abundance of harbour porpoise and other cetaceans in the North Sea and adjacent waters. J. Appl. Ecol. 39, 361–376.
Huggenberger, S., Rauschmann, M. A., Vogl, T. J., and Oelschläger, H. H. (2009). Functional morphology of the nasal complex in the harbor porpoise (Phocoena phocoena L.). Anatom. Rec. 292, 902–920. doi: 10.1002/ar.20854
IJsseldijk, L. L., Brownlow, A. C., and Mazzariol, S. (eds) (2019). Best Practice on Cetacean Post Mortem Investigation and Tissue Sampling. ACCOBAMS/ASCOBANS document. doi: 10.31219/osf.io/zh4ra
IJsseldijk, L. L., and ten Doeschate, M. T. I. (2019). Analysis of stranding data on harbour porpoises along the North Sea for a better understanding of the population structure of the Department of Pathobiology. (Utrecht: Utrecht University) pp 37.
Jauniaux, T., Petitjean, D., Brenez, C., Borrens, M., Brosens, L., Haelters, J., et al. (2002). Post-mortem findings and causes of death of harbour porpoises (Phocoena phocoena) stranded from 1990 to 2000 along the coastlines of Belgium and Northern France. J. Comp. Pathol. 126, 243–253. doi: 10.1053/jcpa.2001.0547
Jepson, P. D., Baker, J. R., Kuiken, T., Simpson, V. R., Kennedy, S., and Bennett, P. M. (2000). Pulmonary pathology of harbour porpoises (Phocoena phocoena) stranded in England and Wales between 1990 and 1996. Vet. Rec. 146, 721–728. doi: 10.1136/vr.146.25.721
Jepson, P. D., Bennett, P. M., Allchin, C. R., Law, R. J., Kuiken, T., Baker, J. R., et al. (1999). Investigating potential associations between chronic exposure to polychlorinated biphenyls and infectious disease mortality in harbour porpoises from England and Wales. Sci. Total Environ. 243, 339–348. doi: 10.1016/s0048-9697(99)00417-9
Jepson, P. D., Deaville, R., Barber, J. L., Aguilar, À, Borrell, A., Murphy, S., et al. (2016). PCB pollution continues to impact populations of orcas and other dolphins in European waters. Sci. Rep. 6:18573. doi: 10.1038/srep18573
Kennedy, S., Smyth, J. A., Cush, P. F., McAliskey, M., McCullough, S. J., and Rima, B. K. (1991). Histopathologic and immunocytochemical studies of distemper in harbor porpoises. Vet. Pathol. 28, 1–7. doi: 10.1177/030098589102800101
Lamoth, F. (2016). Aspergillus fumigatus-related species in clinical practice. Front. Microbiol. 7:683. doi: 10.3389/fmicb.2016.00683
Lass-Flörl, C., Griff, K., Mayr, A., Petzer, A., Gastl, G., Bonatti, H., et al. (2005). Epidemiology and outcome of infections due to Aspergillus terreus: 10-year single centre experience. Br. J. Haematol. 131, 201–207. doi: 10.1111/j.1365-2141.2005.05763.x
Morell, M., Lehnert, K., IJsseldijk, L. L., Raverty, S. A., Wohlsein, P., Gröne, A., et al. (2017). Parasites in the inner ear of harbour porpoise: cases from the North and Baltic Seas. Dis. Aquat. Organ. 127, 57–63. doi: 10.3354/dao03178
Müller, G., Siebert, U., Wünschmann, A., Artelt, A., and Baumgärtner, W. (2000). Immunohistological and serological investigation of morbillivirus infection in harbour porpoises (Phocoena phocoena) from the German Baltic and North Sea. Vet. Microbiol. 75, 17–25. doi: 10.1016/s0378-1135(00)00209-1
Murphy, S., Barber, J. L., Learmonth, J. A., Read, F. L., Deaville, R., Perkins, M. W., et al. (2015). Reproductive failure in UK harbour porpoises Phocoena phocoena: legacy of pollutant exposure? PLoS One 10:e0131085. doi: 10.1371/journal.pone.0131085
Oelschläger, H. H. A., Ridgway, S. H., and Knauth, M. (2010). Cetacean brain evolution: dwarf sperm whale (Kogia sima) and common dolphin (Delphinus delphis)–an investigation with high-resolution 3D MRI. Brain Behav. Evol. 75, 33–62. doi: 10.1159/000293601
Prahl, S., Jepson, P. D., Sanchez-Hanke, M., Deaville, R., and Siebert, U. (2011). Aspergillosis in the middle ear of a harbour porpoise (Phocoena phocoena): a case report. Mycoses 54, e260–e264. doi: 10.1111/j.1439-0507.2010.01863.x
Racicot, R. A., and Berta, A. (2013). Comparative morphology of porpoise (Cetacea: Phocoenidae) pterygoid sinuses: phylogenetic and functional implications. J. Morphol. 274, 49–62. doi: 10.1002/jmor.20075
Reidarson, T. H., McBain, J., Rinaldi, M., and Dalton, L. (1998). “Diagnosis and treatment of fungal infections in marine mammals,” in Zoo and Wildlife Medicine: Current Therapy, 4th Edn, eds M. Fowler and E. Miller, (Philadelphia: W. B. Saunders Co.), 478–485.
Reidarson, T. H., McBain, J. F., Dalton, L. M., and Rinaldi, M. G. (2001). “Mycotic diseases,” in CRC Handbook of Marine Mammal Medicine, eds L. A. Dierauf and F. M. D. Gulland, (Boca Raton, FL: CRC Press), 337–355.
Schoch, C., Seifert, K. A., Huhndorf, S., Robert, V., Spouge, J. L., Levesque, C. A., et al. (2012). Nuclear ribosomal internal transcribed spacer (ITS) region as a universal DNA barcode marker for Fungi. Proc. Natl. Acad. Sci. U.S.A. 109, 6241–6246. doi: 10.1073/pnas.1117018109
Seibel, H., Beineke, A., and Siebert, U. (2010). Mycotic otitis media in a harbour porpoise (Phocoena phocoena). J. Comp. Pathol. 143, 294–296. doi: 10.1016/j.jcpa.2010.03.002
Seyedmousavi, S., Guillot, J., Arné, P., De Hoog, G. S., Mouton, J. W., Melchers, W. J., et al. (2015). Aspergillus and aspergilloses in wild and domestic animals: a global health concern with parallels to human disease. Med. Mycol. 53, 765–797. doi: 10.1093/mmy/myv067
Siebert, U., Wünschmann, A., Weiss, R., Frank, H., Benke, H., and Frese, K. (2001). Post-mortem findings in harbour porpoises (Phocoena phocoena) from the German North and Baltic Seas. J. Comp. Pathol. 124, 102–114. doi: 10.1053/jcpa.2000.0436
St. Leger, J. S., Begeman, L., Fleetwood, M., Frasca, S. Jr., Garner, M. M., Lair, S., et al. (2009). Comparative pathology of nocardiosis in marine mammals. Vet. Pathol. 46, 299–308. doi: 10.1354/vp.46-2-299
Su, H., Li, C., Wang, Y., Li, Y., Dong, L., Li, L., et al. (2019). Kinetic host defense of the mice infected with Aspergillus Fumigatus. Future Microbiol. 14, 705–716. doi: 10.2217/fmb-2019-0043
ten Doeschate, M. T., IJsseldijk, L. L., Hiemstra, S., De Jong, E. A., Strijkstra, A., Gröne, A., et al. (2017). Quantifying parasite presence in relation to biological parameters of harbour porpoises Phocoena phocoena stranded on the Dutch coast. Dis. Aquat. Organ. 127, 49–56. doi: 10.3354/dao03182
Terio, K. A., McAloose, D., and Leger, J. S. (eds) (2018). Pathology of Wildlife and Zoo Animals. Cambridge, MA: Academic Press.
van Beurden, S. J., IJsseldijk, L. L., Cremers, H. J. W. M., Gröne, A., Verheije, M. H., and Begeman, L. (2015a). Anisakis spp. induced granulomatous dermatitis in a harbour porpoise Phocoena phocoena and a bottlenose dolphin Tursiops truncatus. Dis. Aquat. Organ. 112, 257–263. doi: 10.3354/dao02818
van Beurden, S. J., IJsseldijk, L. L., Ordonez, S. R., Förster, C., de Vrieze, G., Gröne, A., et al. (2015b). Identification of a novel Gammaherpesvirus associated with (muco) cutaneous lesions in harbour porpoises (Phocoena phocoena). Arch. Virol. 160, 3115–3120. doi: 10.1007/s00705-015-2607-8
van Beurden, S. J., IJsseldijk, L. L., Van de Bildt, M. W., Begeman, L., Wellehan, J. F., Waltzek, T. B., et al. (2017). A novel cetacean Adenovirus in stranded harbour porpoises from the North Sea: detection and molecular characterization. Arch. Virol. 162, 2035–2040. doi: 10.1007/s00705-017-3310-8
Van Bressem, M. F., Raga, J. A., Di Guardo, G., Jepson, P. D., Duignan, P. J., Siebert, U., et al. (2009). Emerging infectious diseases in cetaceans worldwide and the possible role of environmental stressors. Dis. Aquat. Organ. 86, 143–157. doi: 10.3354/dao02101
van Elk, C. E., van de Bildt, M. W., van Run, P. R., Bunskoek, P., Meerbeek, J., Foster, G., et al. (2019). Clinical, pathological, and laboratory diagnoses of diseases of harbour porpoises (Phocoena phocoena), live stranded on the Dutch and adjacent coasts from 2003 to 2016. Vet. Res. 50:88. doi: 10.1186/s13567-019-0706-3
Venn-Watson, S., Daniels, R., and Smith, C. (2012). Thirty year retrospective evaluation of pneumonia in a bottlenose dolphin Tursiops truncatus population. Dis. Aquat. Organ. 99, 237–242. doi: 10.3354/dao02471
White, P. L., Linton, C. J., Perry, M. D., Johnson, E. M., and Barnes, R. A. (2006). The evolution and evaluation of a whole blood polymerase chain reaction assay for the detection of invasive aspergillosis in hematology patients in a routine clinical setting. Clin. Infect. Dis. 42, 479–486. doi: 10.1086/499949
Williamson, E. C., Leeming, J. P., Palmer, H. M., Steward, C. G., Warnock, D., Marks, D. I., et al. (2000). Diagnosis of invasive aspergillosis in bone marrow transplant recipients by polymerase chain reaction. Br. J. Haematol. 108, 132–139. doi: 10.1046/j.1365-2141.2000.01795.x
Keywords: aspergillosis, cetacean, North Sea, histopathology, culture, MRI, otitis, bronchopneumonia
Citation: Kapetanou A, IJsseldijk LL, Willems DS, Broens EM, Everaarts E, Buil JB, Verweij PE, Kik MJL and Gröne A (2020) Mycotic Infections in Free-Ranging Harbor Porpoises (Phocoena phocoena). Front. Mar. Sci. 7:344. doi: 10.3389/fmars.2020.00344
Received: 16 February 2020; Accepted: 23 April 2020;
Published: 25 May 2020.
Edited by:
Stephen Raverty, Animal Health Center, CanadaReviewed by:
Carla Grattarola, Zooprofilattico Sperimentale del Piemonte, ItalyNorbert Van De Velde, National Veterinary Institute, Sweden
Copyright © 2020 Kapetanou, IJsseldijk, Willems, Broens, Everaarts, Buil, Verweij, Kik and Gröne. This is an open-access article distributed under the terms of the Creative Commons Attribution License (CC BY). The use, distribution or reproduction in other forums is permitted, provided the original author(s) and the copyright owner(s) are credited and that the original publication in this journal is cited, in accordance with accepted academic practice. No use, distribution or reproduction is permitted which does not comply with these terms.
*Correspondence: Lonneke L. IJsseldijk, L.L.IJsseldijk@uu.nl
†These authors have contributed equally to this work