- 1Department of Earth, Environmental and Physical Sciences, University of Siena, Siena, Italy
- 2Department of Veterinary Science, University of Pisa, Pisa, Italy
- 3Interuniversity Consortium for Marine Biology and Applied Ecology “G. Bacci”, Livorno, Italy
The aim of this study was to evaluate a set of ecotoxicity biotests on three marine model species exposed to elutriates of marine sediments inoculated with the biodegradable plastic Mater-Bi or with cellulose. The sediments were incubated at 28°C and tested after 6 months, when clear signs of degradation were visible in the exposed samples, and after 12 months, when the samples had completely disappeared. The model organisms selected for the study were the unicellular algae Dunaliella tertiolecta, the sea urchin (Paracentrotus lividus) and sea bass (Dicentrarchus labrax) juveniles. The unicellular algae and sea urchins were used to determine the toxicological endpoint of growth inhibition and embryotoxicity, respectively, and the sea bass juveniles were tested to evaluate sublethal effect endpoints using lipid peroxidation and genotoxicity biomarkers. Elutriates of sediment inoculated with Mater-Bi for 6 and 12 months showed an absence of toxic effects in all of the model organisms exposed in this study. The Mater-Bi degradation process did not generate or transfer into the elutriates toxic substances that could cause alterations in the growth of D. tertiolecta, in the P. lividus embryo-toxicity assay or in the sensitive biomarker responses of the fish D. labrax. The tested species are considered to be representatives of different levels of the marine trophic chain. The results obtained in this study suggest that the ecotoxicological approach applied may be suitable for investigating the environmental impact of the degradation of bioplastics in marine sediments.
Introduction
Biodegradable plastics are mainly used in the packaging sector, for carrier bags, tableware, and similar products (e.g., waste bags, coffee pods). Biodegradable packaging and products are designed to be collected together with bio-waste (kitchen, food, garden waste) and recovered as compost for soil fertilization by means of organic recycling (e.g., composting). Biodegradable plastics are also used in products for agriculture (e.g., mulch films for weed control), where biodegradation takes place directly in the soil after use. The use of bio-based and biodegradable (bioplastic) polymers has been proposed as a possible solution to the increasing problem of plastic waste, as substitutes for non-biodegradable polymers when other forms of recovery are not feasible. Suitability for composting and biodegradability in soil are determined with multi-tier testing schemes and acceptability criteria defined by international standards (EN, 2000, 2018).
The next frontier in the characterization of biodegradable plastics is the behavior in marine environments, following the growing concern about the pollution of water bodies with non-biodegradable plastics.
The awareness of the global problem of plastics in the seas and oceans has been growing since the first discovery of large aggregations of floating plastic debris (which cover an area as large as central Europe) in the North Pacific Ocean (Moore et al., 2001; Moore, 2003), in the North Atlantic Ocean (Law et al., 2010) and in enclosed and semi-enclosed seas, such as the Mediterranean (Colton et al., 1974; Barnes et al., 2009; Browne et al., 2010).
Tosin et al. (2012) developed three test methods to characterize the degradation of plastic in marine environments. Recently, Eich et al. (2015) investigated the early formation of biofilms on plastic shopping bags and its effects on the degradation of the plastic. Pauli et al. (2017) analyzed the marine fouling communities on plastic, studying the composition of their macroscopic community, their primary production and the degradation of polymer. In the study they compared conventional polyethylene and a biodegradable starch-based plastic blend, in coastal benthic and pelagic habitats in the Mediterranean Sea.
Considering biodegradability as a solution to littering is mistaken, because natural environments cannot deal with irresponsible littering even of fully biodegradable products. However, biodegradable materials could be used in specific applications where traditional non-biodegradable plastics are used with a high risk of littering. For example, aquaculture facilities could disperse non-biodegradable plastic gear in the sea, and replacing it with biodegradable materials could have a mitigating effect.
As mentioned above, biodegradation can now be measured thanks to the development of specific test methods developed and standardized by the ISO (ISO, 2016, 2017) and the ASTM (ASTM, 2015). However, to evaluate the ecotoxic impact of substances that may be released into the surrounding environment during biodegradation, an additional level of testing is necessary. Even if the release of ecotoxic substances is unlikely, considering the very high mineralization threshold levels required by the testing schemes applied for biodegradable plastics, the absence of negative effects is required by all the testing protocols developed, as an additional form of reassurance.
In order to detect ecotoxicity effects, all the testing protocols developed require that the plastic material be exposed to a very high dose of the matrix of interest (e.g., compost, soil, or seawater/marine sediments) and be left to biodegrade. After a selected time, ecotoxicity biotests are applied to the exposed matrix, as well as to a control sample that has not been exposed to the plastic material, and to a control sample that has been exposed to a GRAS (Generally Recognized As Safe) substance, such as cellulose. Absence of statistically significant difference between exposed and control matrices must be found (Sforzini et al., 2016).
In this contest, the main aim of this study was to evaluate a set of ecotoxicity biotests on three marine model species (that belong to different trophic levels) that have been exposed to elutriates of marine sediments inoculated with bioplastics. The product chosen for the testing was a commercially produced bag made with a biodegradable material produced by Novamont S.p.A. (Italy).
The model organisms selected for the study were the unicellular algae Dunaliella tertiolecta, the sea urchin (Paracentrotus lividus) and sea bass (Dicentrarchus labrax) juveniles. The unicellular alga e and sea urchins were used to determine the toxicological endpoint of growth inhibition and embryotoxicity, respectively, and the sea bass juveniles were tested to evaluate sublethal effect endpoints using lipid peroxidation (LPO) and genotoxicity biomarkers.
Since microalgae are positioned at the lower levels of trophic webs, the inhibition of growth bioassays is commonly used in ecotoxicity test batteries, because of the ecological relevance of these organisms (Prata et al., 2019). However, limited literature on the effects of plastic leachates on microalgae is available (Prata et al., 2019). Sea urchins are a useful indicator species for environmental contamination due to the fact that their gametes and larval stages are very sensitive to various classes of contaminants in complex environmental matrices, such as estuarine/marine waters and sediments (Pagano et al., 1993; Kobayashi and Okamura, 2005; Novelli et al., 2006; Rodríguez-Romero et al., 2016) including trace elements (Fernández and Beiras, 2001; Xu et al., 2011; Manzo et al., 2013) and plastic debris (Martínez-Gómez et al., 2017; Botterell et al., 2019).
The evaluation of toxicological effects associated with the presence of plastic can be made in bioindicator organisms, such as fish, using biomarkers. In this study we selected a biomarker of oxidative stress (LPO) and a biomarker of genotoxic effects (ENA assay).
Marine organisms are commonly exposed to various environmental stressors and oxidative stress is a crucial part of the stress response (Vinagre et al., 2012). Oxidative stress may be defined as a disturbed balance between the production of reactive oxygen species (ROS) and an organism’s ability to deal with them (Halliwell and Gutteridge, 2015). Increased reactive species production or reduced antioxidant defense can damage lipid components, proteins and DNA. Some ROS can start the self-propagating process of LPO, in which a peroxyl radical is formed when a ROS reacts sufficiently to a hydrogen atom from an intact lipid (Halliwell and Gutteridge, 2015). The reaction of ROS with lipids is one of the prevalent mechanisms of cell damage (Halliwell and Gutteridge, 2015). Progressive damage of biomolecules triggers an inflammatory response, cell and tissue damage, and induces degenerative changes, which can result in cell senescence and death, organ dysfunction and loss of, or reduced organism performance (Costantini, 2014). We selected this biomarker, as some studies have reported that the exposure of marine fish and invertebrates to non-biodegradable plastic resulted in oxidative stress (Paul-Pont et al., 2016; Alomar et al., 2017), and the subsequent induction of LPO (Avio et al., 2015; Barboza et al., 2018) and genotoxic damage (Avio et al., 2015; Martins et al., 2016).
Several techniques have been designed to detect the genotoxic damage caused by a wide range of substances. The technique most used to determine chromosomal clastogenesis, by analyzing nuclear aberrations, is the micronucleus test (MN) in mammalian cells. MN assay was extended to non-mammalian organisms that have nucleated erythrocytes, leading to the development of the erythrocytic nuclear abnormality (ENA) test (Bolognesi and Cirillo, 2014; Çavaş and Ergene-Gözükara, 2005). Avio et al. (2015) reported a statistically significant increase in the frequency of micronuclei, and number of nuclear anomalies in mussels (Mytilus galloprovincialis) that had been treated with microplastics of polystyrene and polyethylene.
Materials and Methods
Test Material
The test material was compostable produce bags that are commercially available in the Italian market for selecting and bagging fruit and vegetables. These bags were made of the Mater-Bi grade HF03V1, a biodegradable and compostable plastic material produced by Novamont S.p.A. This plastic material can be recovered by organic recycling, i.e., it is biodegradable and disintegrable under composting conditions, in accordance with the European standard EN (2000). The plastic material is made of starch, biodegradable polyesters and a natural plasticizer.
The bags were powdered by a process of cryogenic grinding, with liquid nitrogen. Pieces of the film (40 mm × 40 mm) were used to monitor the progress of the physical deterioration (disintegration).
Reference Material
The reference material was pure microcrystalline cellulose (Merck), in powder form.
Pieces of cellulose filter paper (40 mm × 40 mm) (Whatman n.42) were also used to monitor the progress of the physical deterioration (disintegration).
Inoculum
The inoculum was a sandy sediment sampled at Punta Ala (Grosseto, Italy). The sediment was selected for its low content of heavy metals and polycyclic aromatic hydrocarbons.
Exposure of Materials to Inoculum
The sediment was filtered using a coarse/fast filter paper to eliminate excess seawater. The wet sediment thus prepared had a pH of 8.27, a water content of 20.74% and volatile solids of 1.70%. Samples (2500 g each) of wet sediment were placed in six reactors i.e., glass tanks of 2.6-litre capacity (22 cm in diameter). Test and reference materials were supplemented in the form of particles to the reactors as indicated in Table 1. The sediments and the particles were carefully blended, using a spatula, to obtain a homogeneous layer in the reactor. Samples of Mater-Bi films and of cellulose filter paper were also buried in the sediment of each reactor, in order to monitor the physical deterioration of the sample, i.e., disintegration.
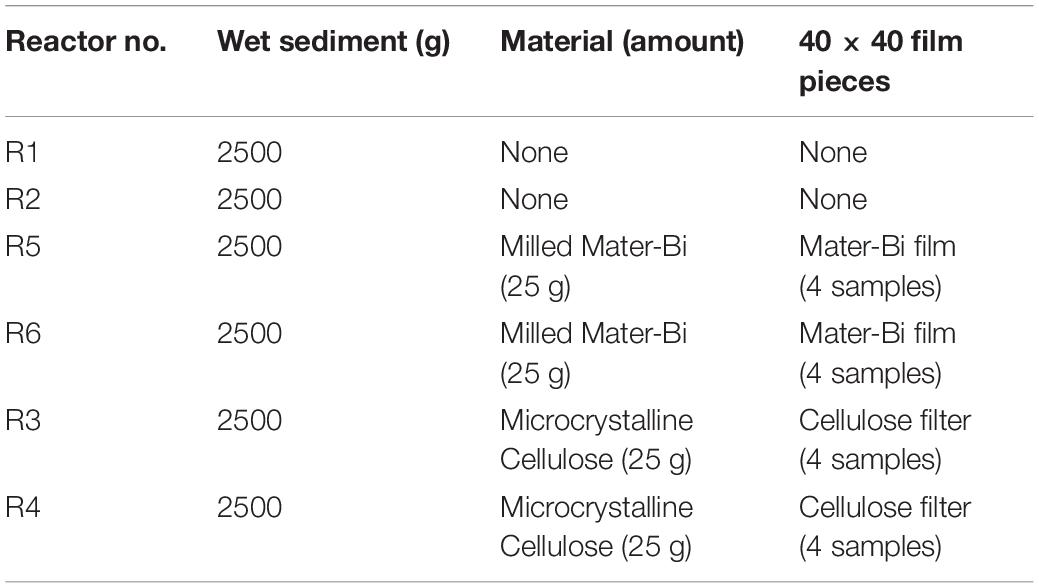
Table 1. Test set-up: sediment elutriates after 6 months (R1 = control, R3 = biodegradable plastics, R5 = cellulose) and after 12 months (R2 = control, R4 biodegradable plastics, R6 = cellulose).
The reactors were then closed with a lid with two holes to ensure gas exchange, and incubated in the dark at 28 ± 2°C. Periodically the reactors/crystallizers were weighed and, if necessary, the initial masses were restored using distilled water.
A salt solution (0.2 g/Kg KH2PO4; 0.1g/Kg MgSO4; 0.6 g/Kg NaNO3; 0.6 g/Kg NH4Cl) and 2 g/Kg of CH3COONa were added to each reactor after 3 months of incubation and 2 g/Kg of Nutrient Broth after 7 months, in order to provide the isolated mesocosm with potentially missing nutrients and reduce the duration of the experiment.
After 6 and 12 months, one replica for each treatment was analyzed in ecotoxicity tests.
Elutriate Preparation
Elutriates were obtained by diluting each marine sediments, including the native sediment (R0), with synthetic marine water (Figure 1). A volume of three liters of filtered synthetic marine water was added to 1 Kg of sediments (dry weight) in a five-liter glass jar (dilution 1:4 w/v). The samples were stirred for 60 m and allowed to settle for 24 h at 4°C. The resulting liquid phase was filtered to obtain a clear solution. The seven types of elutriates obtained were analyzed applying a set of bioassays to algae, crustaceans and fish.
Algae and Crustacean Bioassay
Assay of the Inhibition of D. tertiolecta Growth
The inhibition of the growth of D. tertiolecta was evaluated in accordance with the protocol described in ISO (2016) procedures, with slight modifications. The D. tertiolecta strain CCAP 19/27 was purchased from the reference center CCAP (Culture Collection of Algae and Protozoa—Scottish Association for Marine Science/SAMS Research Services Ltd). The D. tertiolecta was cultured in an F/2 medium [artificial seawater (ASW) supplemented with a salt mix, a trace element mix, and a vitamin mix, in accordance with (Guillard et al., 1975)]. Late logarithmic phase algae were inoculated in 25 mL of fresh medium (50 mL conical flasks) to an initial concentration of 104 cells/mL and were grown at 20 ± 2°C, under cool white fluorescent continuous light of 7000 l×, with slow shaking for 72 h. The experiments were carried out in triplicate. The F/2 medium acted as a control. The eluates were tested at eluate maximum concentration (100%, no dilution, three replicates); where samples differed significantly from controls by more than 20% (Dunnett’s test), a full test was performed. Potassium dichromate was used as the reference toxicant. The endpoint was the inhibition of growth (no. of cells/ml) at the end of 72 h. The cells were counted using a Scepter 2.0 Handheld Automated Cell Counter (Millipore Corporation, Billerica, MA, United States).
P. lividus Embryo-Toxicity Assay
Adult P. lividus individuals were collected from MPA Secche della Meloria (Livorno, Italy). The embryo toxicity test was carried out following the EPA method (Chapman et al., 1995). The spawning of eggs and sperm was induced in the sea urchins by injecting 1 mL of 0.5 M KCl through the perioral membrane. The eggs were collected by placing each spawning female separately in a different 250 mL beaker with ASW, and “dry” sperm was collected from each male using an automatic pipette and stored in a sterile tube placed on ice. After a quality check of the gametes, three male and three female gametes were pooled and filtered through nylon plankton mesh (∅ = 200 μm for eggs and 50 μm for sperm). The egg suspension (stock solution) was diluted, to obtain the final concentration of 250–300 eggs/mL. Fertilization was carried out by adding 1 mL of pooled-sperm (1:1000 in ASW), to the egg suspension and by incubating it at 18°C for 20 min. A volume of the egg suspension corresponding to 250–300 fertilized eggs was treated with 10 mL of test solution, starting with the whole eluate and then another four 1:1 serial dilutions (three replicates/dilution). The eggs were then incubated at 18°C, for 48 h. After this period, 100 μl of 40% buffered formalin was added to each vessel and the developmental abnormalities were determined in each replicate by directly observing 100 randomly chosen individuals. Copper nitrate was used as a reference toxicant. For each treatment scheduled, 100 plutei were scored for the frequencies of normal larvae, according to their symmetry, shape, and size and malformed/unformed larvae, following EPA guidelines (Chapman et al., 1995); the sample scores were corrected for control response using Abbott’s formula.
Dicentrarchus Labrax Sub Lethal End Points
Experimental Design
Sea bass juveniles of about 10 cm in length and 6 g in weight were exposed to five different sediment elutriates obtained from reactor contents after 6 and 12 months of degradation (Table 2). Each experimental tank contained 10 fish, which were exposed to 25% v/v of elutriate, and 1 control tank contained synthetic seawater. The fish were fed every 7 days with commercial, certified chemical residue-free fish food, and kept under natural photoperiod.
After 2 weeks the animals were sacrificed and a drop of blood was smeared on a glass slide to measure the ENA assay. The gills were sampled and stored in liquid nitrogen for LPO analysis.
The experiment was carried out in accordance with Directive 2010/63/EU on the protection of animals used for scientific purposes.
Erythrocytic Nuclear Abnormalities Assay
Two blood smears per sample were prepared. The slides obtained were fixed in methanol or ethanol for 10 min, and then stained with Giemsa (5%) for 30 min. The erythrocytic nuclear abnormalities (ENA) were scored in 1000 mature erythrocytes per sample, in accordance with the procedures of Maier and Schmid (1976); Carrasco et al. (1990) and Smith (1990), adapted by Pacheco and Santos (1997). In accordance with these authors, the nuclear lesions were scored into one of the following categories: micronuclei, lobed nuclei, segmented nuclei and kidney-shaped nuclei. The results were expressed as the ENA frequency, the mean value (‰) of each abnormality and the sum of all the lesions observed.
Lipid Peroxidation
The LPO was determined in the gills, following the procedure of Ohkawa et al. (1979) and Bird and Draper (1984). A total of 10 μl of 4% butylated hydroxytoluene (BHT) in methanol were added to and mixed homogeneously with 150 μl of plasma. Then, 1 ml of 12% trichloroacetic acid (TCA) in aqueous solution, 0.75 ml of Tris–HCl (60 mM, pH 7.4 and 0.1 mM DTPA) and 1 ml of 0.73% 2-thiobarbituric acid (TBA) were added to 100 μl of the mixture and mixed well. The mixture was incubated at 100°C for 1 h and then cooled on ice. Then, each sample was centrifuged at 13,400 g, for 5 min. The absorbance of the supernatant was measured at 535 nm in a spectrophotometer (Agilent Cary UV 60) and the rate of LPO expressed as nmol of thiobarbituric acid reactive substances (TBARS) formed/mg protein (ε = 1.56 × 105 M−1 cm−1).
Results and Discussion
Bioplastic Biodegradation
The biodegradable plastics were incubated with the sediments for one year. The biodegradation process was indirectly monitored following the level of disintegration of film samples (test material) and a paper filter (reference material) buried in the sediment. After 6 months a reactor in each series (R1, R3, R5) was discontinued, the film/paper samples were withdrawn and the degree of disintegration was qualitatively assessed. The sediments were subjected to extraction and the elutriates were tested for ecotoxicity. The remaining reactors were incubated for another 6 months. After 12 months the film/paper samples had completely disappeared, indicating the biodegradation process had further advanced. The remaining sediments (R2,R4, R6) were extracted and the elutriates were tested. The disintegration levels are shown in Figure 2 and Table 2.
Inhibition of D. tertiolecta Growth
All tested elutriates showed an absence of effects, such as the inhibition of D. tertiolecta growth (Table 3). It could be hypothesized either that the compounds resulting from the degradation of the Mater-Bi and cellulose were not harmful to D. tertiolecta, or that they were not sufficiently concentrated in the elutriate. There are no published studies concerning exposure of microalgae to elutriates of sediment inoculated with plastics to compare with. A study by Sjollema et al. (2016), investigated the effects of polystyrene particles on microalgal photosynthesis and growth: microalgal growth was negatively affected by uncharged polystyrene particles at high concentrations, with effects increasing with decreasing particle size.
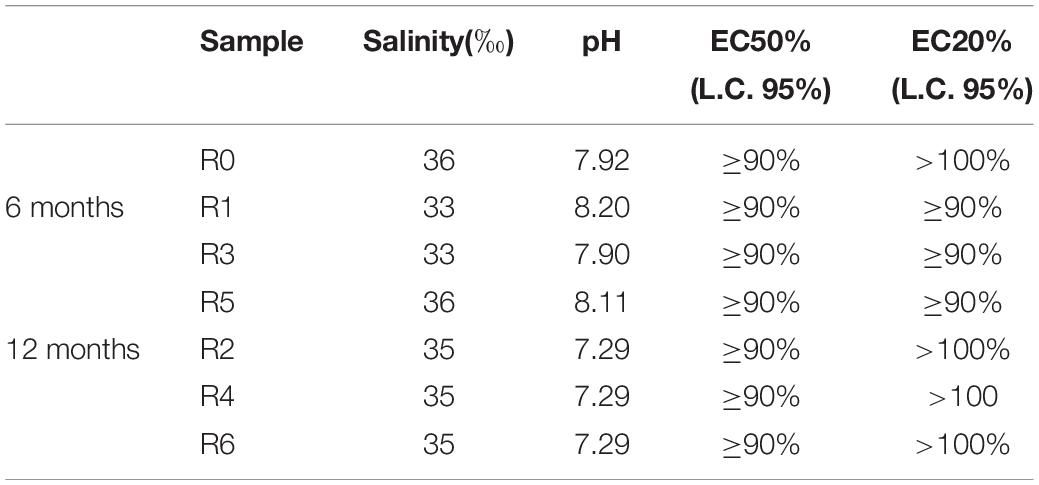
Table 3. Algal growth inhibition test on D. tertiolecta with different sediment elutriates zero-time (R0), after 6 months (R1 = control, R3 = biodegradable plastics, R5 = cellulose, control) and after 12 months (R2 = control, R4 biodegradable plastics, R6 = cellulose).
P. lividus Embryo-Toxicity Assay
As shown in Table 4, the time-zero control sediment elutriate showed EC50 values of 45.8%. After six months’ incubation, R3 elutriates (Mater-Bi) showed EC50 of 95.4%, which was substantially higher than the 6 month control and the 6 month cellulose. The Mater-Bi EC50 further increased after 12 months of experiments (EC50 > 100). A similar trend was found for the no effect concentrations EC10 and EC20. In fact, elutriates of Mater-Bi showed very low/absent P. lividus embryo-toxicity, which was lower than the controls and the cellulose. There are no studies in the literature that could allow a comparison with the data obtained in this research.
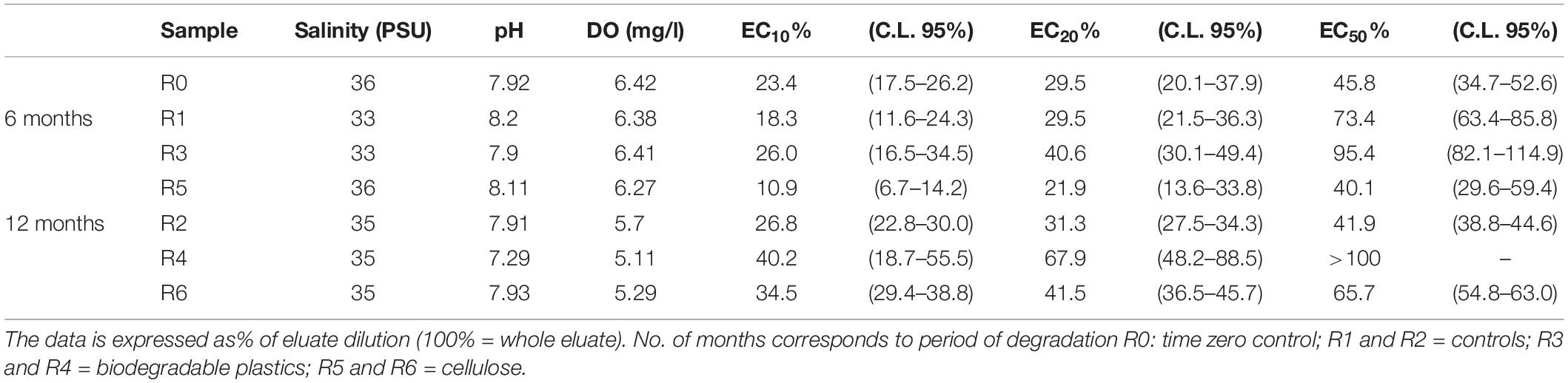
Table 4. P. lividus embryo-toxicity test: Physicochemical parameters of eluates and corresponding EC10, EC20 and EC50 values (C.L. 95%: confidence limit set at 95%).
Biomarkers in D. labrax
Lipid Peroxidation
Figure 3 shows the results of the LPO test carried out on the gills of D. labrax specimens exposed to the different sediment elutriates. The data does not show any statistically significant difference between the various groups (Mann-Whitney U, p < 0.05). The studies carried out by Barboza et al. (2018), which exposed specimens of D. labrax to persistent microplastics, Oliveira et al. (2013), which exposed Pomatoschistus microps to persistent microplastics and pyrene, Alomar et al. (2017), which exposed Mullus surmuletus to persistent microplastics, and Avio et al. (2015), which exposed Mytilus galloprovincialis to persistent microplastics, showed an induction of LPO levels in all treatments, with respect to controls. As there are no studies in the literature that refer to fish exposure to elutriates of sediment inoculated with plastics, the above-mentioned papers are the only ones available for comparison with the present work. Our data does not show any LPO alteration in the treatments with respect to control groups, and in general, the mean values of the byproducts of TBARS are extremely low when compared to the above-mentioned studies. Also, by comparing our data with that published by Cotou et al. (2012) in which the authors exposed specimens of D. labrax to an antifouling agent, obtaining mean TBARS values of 100 nmol mg−1, it is clear that the values found in our study should be considered low. The values recorded for each experimental group in the present study are in line with the control values reported by a study carried out by Duarte et al. (2018) on D. labrax from natural estuary areas.
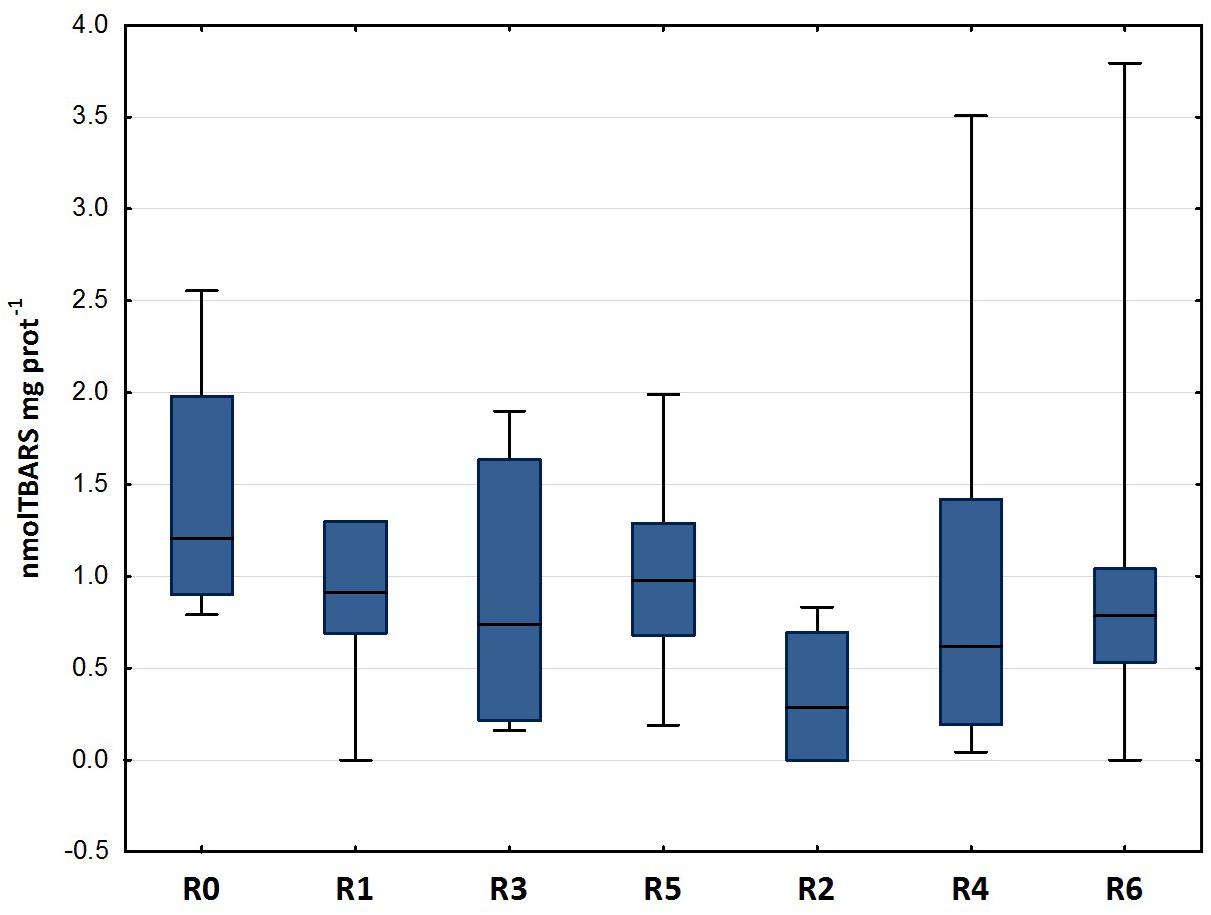
Figure 3. Lipid peroxidation (median, 25–75%, min–max) in D. labrax exposed to sediment eluates at time zero (R0), after 6 months (R1 = control, R3 = biodegradable plastics, R5 = cellulose) and after 12 months (R2 = control, R4 = biodegradable plastics, R6 = cellulose).
Genotoxicity: Erythrocytic Nuclear Abnormalities
The results of the ENA assay performed on mature erythrocytes of D. labrax exposed to the different elutriates are shown in Figures 4, 5. None of the groups tested differed from the controls in terms of the percentage of mature erythrocytes with nuclear abnormalities (Figure 4). Kidney and lobed nuclei were the most common ENA (Figure 5). Overall, the frequency of intact mature erythrocytes exhibiting nuclear abnormalities of any type ranged between 1.6% (R4) and 13% (highest scores in R3). There were no micronuclei (MN), which are extra-nuclear bodies that contain damaged chromosome fragments and/or whole chromosomes that are not incorporated into the nucleus after cell division (Fenech, 2011; Luzhna et al., 2013) in any of the treatments, except for R1, in which the frequency was 0.2 ‰.
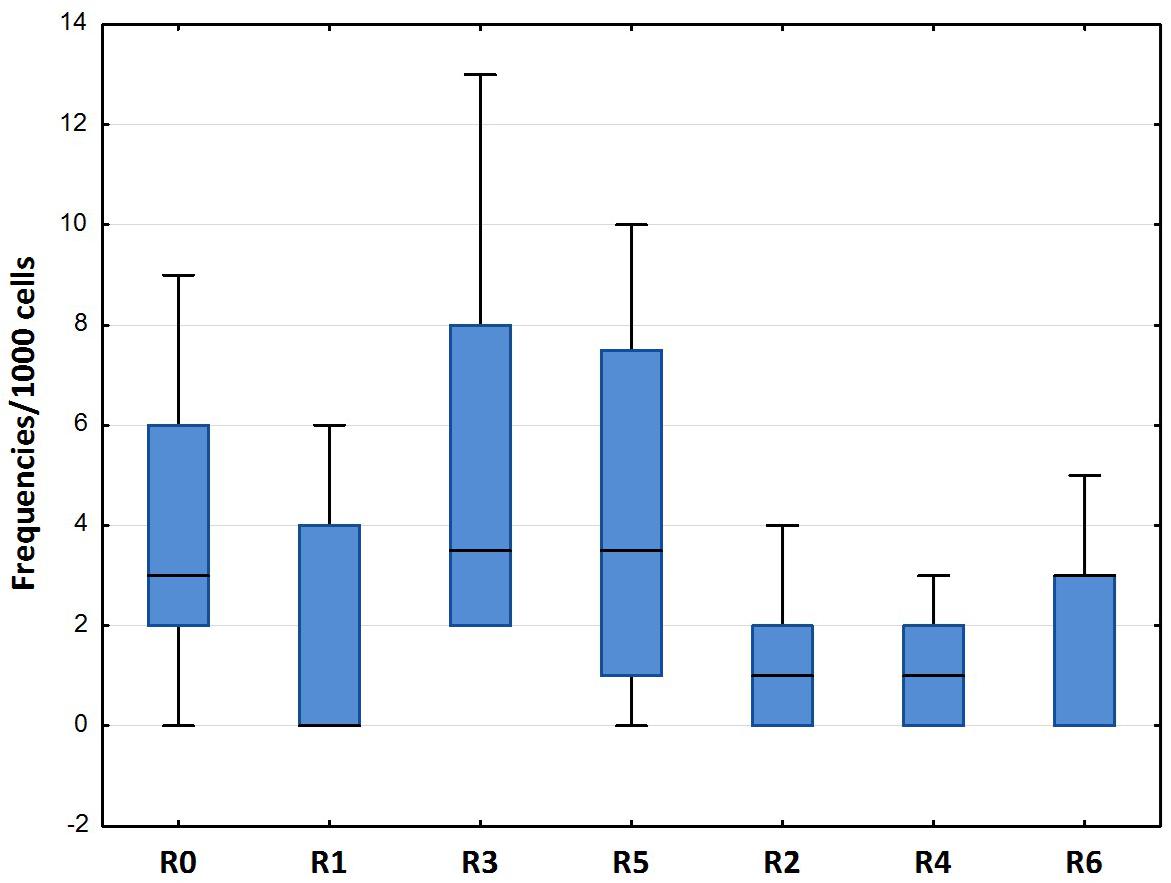
Figure 4. Total erythrocytic nuclear abnormalities (median, 25–75%, min–max) in D. labrax exposed to sediment eluates at time zero (R0), after 6 months (R1 = control, R3 = biodegradable plastics, R5 = cellulose) and after 12 months (R2 = control, R4 = biodegradable plastics, R6 = cellulose).
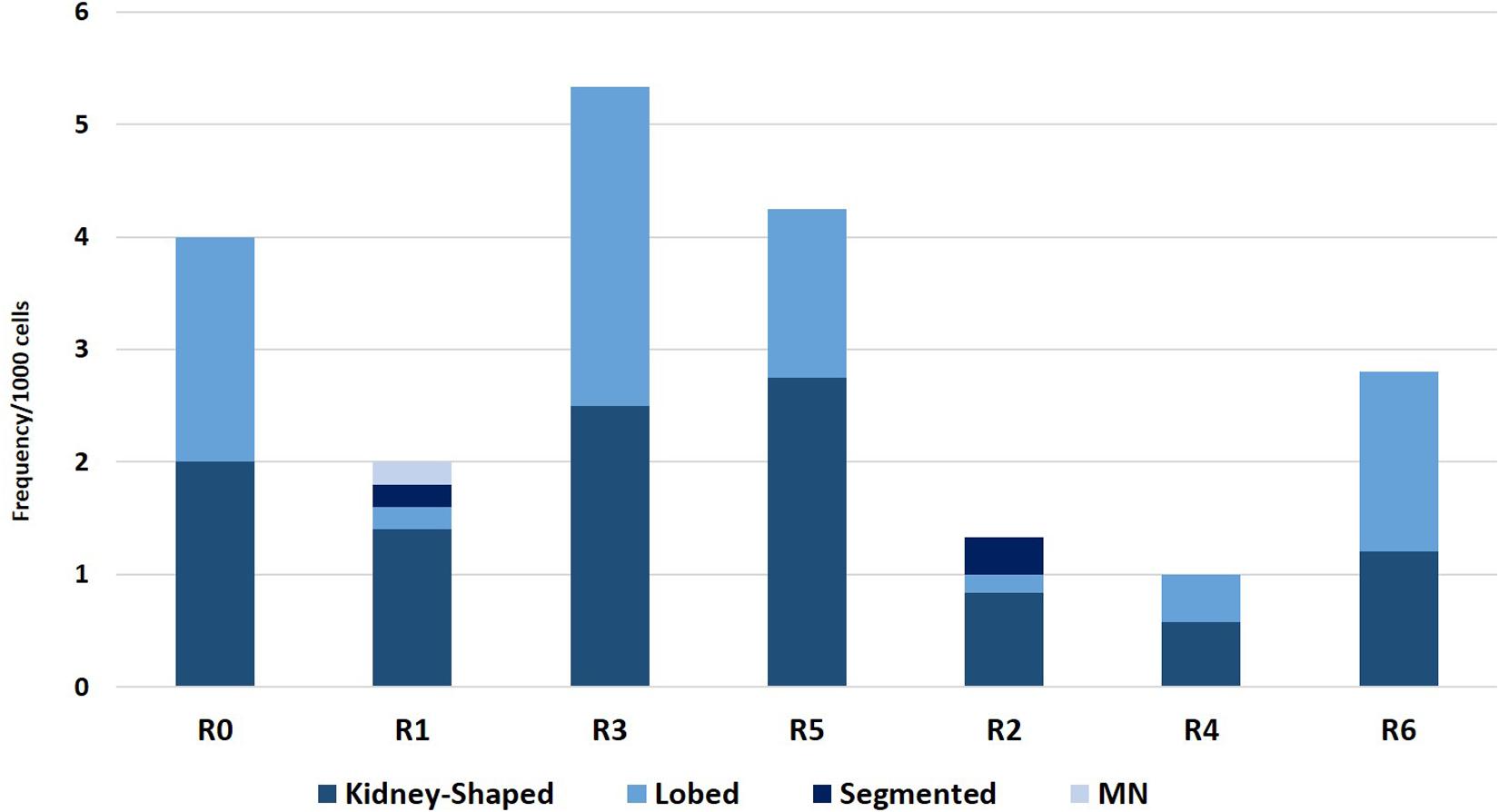
Figure 5. Frequency of erythrocytes with lobed nuclei, micronuclei, kidney shaped nuclei, and segmented nuclei found in D. labrax exposed to the sediment eluates at time zero (R0), after 6 months (R1 = control, R3 = biodegradable plastics, R5 = cellulose) and after 12 months (R2 = control, R4 = biodegradable plastics, R6 = cellulose).
The micronucleus frequencies obtained in this study were lower than the values found in sea bass specimens exposed to nanoparticles of TiO2 and CdCl2 in a study by Nigro et al. (2015), and were in line with the values reported as controls in the same work. Moreover the total nuclear abnormalities and the micronucleus frequencies were lower than in the work of Avio et al. (2015), which shows higher values of nuclear abnormalities in all treatments than in the control, with statistically significant differences in mussels exposed to microplastics and microplastics+pyrene.
A study by Caliani (2009) on sea bass sampled in a reference area in Liguria (Lavagna) and in the port of La Spezia, showed average values of total ENA ranging from 30 to 70‰, which were much higher than those found in this study. The lowest values were found in samples from the area in front of the town of Lavagna, and the highest values were found in the port of La Spezia, following dredging of the port.
Conclusion
Elutriates of marine sediment exposed for 6 and 12 months to Mater-Bi fragments at a 1% (dry weight/wet weight) content showed the absence of the toxic effects examined in all of the model organisms exposed in this study, in both an absolute sense, and after comparison with the controls and with elutriates from sediments exposed to cellulose. The Mater-Bi degradation process did not generate and transfer into the elutriates toxic substances that could cause alterations in the growth of D. tertiolecta, in the P. lividus embryo-toxicity assay, or, in particular, in the sensitive biomarker responses (LPO and ENA assay) in the fish D. labrax. The tested species are considered to be representatives of different levels of the marine trophic chain.
The results obtained in this study suggest that the exposure phase of sediments to plastics and the preparation of elutriates are suitable for the preparation of samples for the investigation of the environmental impact of bioplastic degradation in marine sediments. In particular, we confirm the suitability of the use of a detailed ecotoxicological multi-endpoint protocol for bioplastic degradation in marine sediments, the evaluation of toxicological test in three different trophic level organisms, using acute and chronic endpoints and the use of biomarkers to evaluate the sublethal effects. Further studies will be needed in order to confirm whether this approach is suitable for a standardized testing scheme to be routinely used for the assessment of biodegradable materials.
Data Availability Statement
The datasets generated for this study are available on request to the corresponding author.
Ethics Statement
The animal study was reviewed and approved by Fish husbandry and experimental procedures were conducted within the EU legislation for the protection of animals used for scientific purposes (Directive, 2010/63/EU). The present experiment was authorized by the Ministry of Health (authorization no. 1255/2015-PR).
Author Contributions
TC participated in the definition of the experimental plan, carried out the sampling and set up the experiments. He carried out fish analyzes and statistical processing of the results, participated in the drafting of the manuscript. SC has developed the experimental plan, supervised the laboratory phases, the analysis of the results, and the statistical analysis of the results. She wrote and reviewed the manuscript. IC participated in the preparation of the experiments and carried out the analysis on fish. CP carried out the toxicity tests, elaborated the data, and wrote the results on D. tertiolecta and P. lividus. MF developed the experimental plan, supervised all the various parts of the study and writing and reviewing of the manuscript.
Funding
This study was financially supported by Novamont S.p.A., Italy.
Conflict of Interest
The authors declare that the research was conducted in the absence of any commercial or financial relationships that could be construed as a potential conflict of interest.
References
Alomar, C., Sureda, A., Capó, X., Guijarro, B., Tejada, S., and Deudero, S. (2017). Microplastic ingestion by Mullus surmuletus Linnaeus, 1758 fish and its potential for causing oxidative stress. Environ. Res. 159, 135–142. doi: 10.1016/j.envres.2017.07.043
ASTM (2015). ASTM 7991:2015 Determining Aerobic Biodegradation of Plastics Buried in Sandy Marine Sediment under Controlled Laboratory Conditions. West Conshohocken, PA: ASTM International.
Avio, C. G., Gorbi, S., Milan, M., Benedetti, M., Fattorini, D., d’Errico, G., et al. (2015). Pollutants bioavailability and toxicological risk from microplastics to marine mussels. Environ. Pollut. 198, 211–222. doi: 10.1016/j.envpol.2014.12.021
Barboza, L. G. A., Vieira, L. R., Branco, V., Figueiredo, N., Carvalho, F., Carvalho, C., et al. (2018). Microplastics cause neurotoxicity, oxidative damage and energy-related changes and interact with the bioaccumulation of mercury in the European seabass, Dicentrarchus labrax (Linnaeus, 1758). Aquat. Toxicol. 195, 49–57. doi: 10.1016/j.aquatox.2017.12.008
Barnes, D. K., Galgani, F., Thompson, R. C., and Barlaz, M. (2009). Accumulation and fragmentation of plastic debris in global environments. Philos. Trans. R. Soc. B Biol. Sci. 364, 1985–1998. doi: 10.1098/rstb.2008.0205
Bird, R. P., and Draper, H. H. (1984). “[35] Comparative studies on different methods of malonaldehyde determination,” in Methods in Enzymology, Vol. Vol 105, ed. L. A. Packer (Orlando, FL: Academic Press), 299–305. doi: 10.1016/s0076-6879(84)05038-2
Bolognesi, C., and Cirillo, S. (2014). Genotoxicity biomarkers in aquatic bioindicators. Curr. Zool. 60, 273–284. doi: 10.1093/czoolo/60.2.273
Botterell, Z. L. R., Beaumont, N., Dorrington, T., Steinke, M., Thompson, R. C., and Lindeque, P. K. (2019). Bioavailability and effects of microplastics on marine zooplankton: a review. Environ. Pollut. 245, 98–110. doi: 10.1016/j.envpol.2018.10.065
Browne, M. A., Galloway, T. S., and Thompson, R. C. (2010). Spatial patterns of plastic debris along estuarine shorelines. Environ. Sci. Technol. 44, 3404–3409. doi: 10.1021/es903784e
Caliani, I. (2009). Messa a punto ed applicazione di Biomarker di genotossicità e biochimici in bioindicatori ittici. Tesi di Dottorato. Università di Siena. (tesi di dottorato). Siena: UIniversità di Siena.
Carrasco, K. R., Tilbury, K. L., and Myers, M. S. (1990). Assessment of the piscine micronucleus test as an in situ biological indicator of chemical contaminant effects. Can. J. Fish. Aquat. Sci. 47, 2123–2136. doi: 10.1139/f90-237
Çavaş, T., and Ergene-Gözükara, S. (2005). Micronucleus test in fish cells: a bioassay for in situ monitoring of genotoxic pollution in the marine environment. Environ. Mol. Mutagen 46, 64–70. doi: 10.1002/em.20130
Chapman, G. A., Denton, D. L., and Lazorchak, J. M. (1995). Short-term methods for estimating the chronic toxicity of effluents and receiving waters to west coast marine and estuarine organisms, 3rd Edn. Washington DC: US EPA, Office of Research and Development, National Exposure Research.
Colton, J. B., Knapp, F. D., and Burns, B. R. (1974). Plastic particles in surface waters of the northwestern Atlantic. Science 185, 491–497. doi: 10.1126/science.185.4150.491
Costantini, D. (2014). Oxidative stress and hormesis in evolutionary ecology and physiology: a marriage between mechanistic and evolutionary approaches. Berlin: Springer, 348.
Cotou, E., Henry, M., Zeri, C., Rigos, G., Torreblanca, A., and Catsiki, V.-A. (2012). Short-term exposure of the European sea bass Dicentrarchus labrax to copper-based antifouling treated nets: Copper bioavailability and biomarkers responses. Chemosphere 89, 1091–1097. doi: 10.1016/j.chemosphere.2012.05.075
Duarte, I. A., Vasconcelos, R. P., França, S., Batista, M. I, Tanner, S., Cabral, H. N., et al. (2018). Short-term variability of fish condition and growth in estuarine and shallow coastal areas. Mar. Environ. Res 134, 130–137. doi: 10.1016/j.marenvres.2018.01.008
Eich, A., Mildenberger, T., Laforsch, C., and Weber, M. (2015). Biofilm and Diatom Succession on Polyethylene (PE) and Biodegradable Plastic Bags in Two Marine Habitats: Early Signs of Degradation in the Pelagic and Benthic Zone? PLoS One 10:e0137201. doi: 10.1371/journal.pone.0137201
EN (2000). EN 13432:2000 Packaging. Requirements for packaging recoverable through composting and biodegradation. Test scheme and evaluation criteria for the final acceptance of packaging.
EN (2018). EN 17033:2018 Plastics. Biodegradable mulch films for use in agriculture and horticulture. Requirements and test methods.
Fenech, M. (2011). Micronuclei and their association with sperm abnormalities, infertility, pregnancy loss, pre-eclampsia and intra-uterine growth restriction in humans. Mutagenesis 26, 63–67. doi: 10.1093/mutage/geq084
Fernández, N., and Beiras, R. (2001). Combined toxicity of dissolved mercury with copper, lead and cadmium on embryogenesis and early larval growth of the Paracentrotus lividus sea-urchin. Ecotoxicology 10, 263–271.
Guillard, R. R., Bold, H. C., and MacEntee, F. J. (1975). Four new unicellular chlorophycean algae from mixohaline habitats. Phycologia 14, 13–24. doi: 10.2216/i0031-8884-14-1-13.1
Halliwell, B., and Gutteridge, J. M. (2015). Free radicals in biology and medicine. Oxford: Oxford University Press.
ISO (2016). BS ISO 19679:2016 Plastics. Determination of aerobic biodegradation of non-floating plastic materials in a seawater/sediment interface. Method by analysis of evolved carbon dioxide. London: BSI, 1–20.
ISO (2017). ISO 18830:2017 Plastics — Determination of aerobic biodegradation of non-floating plastic materials in a seawater/sandy sediment interface — Method by measuring the oxygen demand in closed respirometer. Geneva: ISO.
Kobayashi, N., and Okamura, H. (2005). Effects of heavy metals on sea urchin embryo development. Part 2. Interactive toxic effects of heavy metals in synthetic mine effluents. Chemosphere 61, 1198–1203. doi: 10.1016/j.chemosphere.2005.02.071
Law, K. L., Morét-Ferguson, S., Maximenko, N. A., Proskurowski, G., Peacock, E. E., Hafner, J., et al. (2010). Plastic accumulation in the North Atlantic subtropical gyre. Science 329, 1185–1188. doi: 10.1126/science.1192321
Luzhna, L., Kathiria, P., and Kovalchuk, O. (2013). Micronuclei in genotoxicity assessment: from genetics to epigenetics and beyond. Front. Genet. 4:131. doi: 10.3389/fgene.2013.00131
Maier, P., and Schmid, W. (1976). Ten model mutagens evaluated by the micronucleus test. Mutat. Res. Toxicol. 40, 325–337. doi: 10.1016/0165-1218(76)90031-8
Manzo, S., Miglietta, M. L., Rametta, G., Buono, S., and Di Francia, G. (2013). Embryotoxicity and spermiotoxicity of nanosized ZnO for Mediterranean sea urchin Paracentrotus lividus. J. Hazard. Mater. 254, 1–9. doi: 10.1016/j.jhazmat.2013.03.027
Martínez-Gómez, C., León, V. M., Calles, S., Gomáriz-Olcina, M., and Vethaak, A. D. (2017). The adverse effects of virgin microplastics on the fertilization and larval development of sea urchins. Mar. Environ. Res. 130, 69–76. doi: 10.1016/j.marenvres.2017.06.016
Martins, M., Ferreira, A. M., Costa, M. H., and Costa, P. M. (2016). Comparing the genotoxicity of a potentially carcinogenic and a noncarcinogenic PAH, singly, and in binary combination, on peripheral blood cells of the European sea bass. Environ. Toxicol. 31, 1307–1318. doi: 10.1002/tox.22135
Moore, C. (2003). Trashed: across the Pacific Ocean, plastics, plastics. Everywhere. Natural History 112, 46–51.
Moore, C. J., Moore, S. L., Leecaster, M. K., and Weisberg, S. B. (2001). A comparison of plastic and plankton in the North Pacific central gyre. Mar. Pollut. Bull. 42, 1297–1300. doi: 10.1016/s0025-326x(01)00114-x
Nigro, M., Bernardeschi, M., Costagliola, D., Della Torre, C., Frenzilli, G., Guidi, P., et al. (2015). n-TiO2 and CdCl2 co-exposure to titanium dioxide nanoparticles and cadmium: Genomic, DNA and chromosomal damage evaluation in the marine fish European sea bass (Dicentrarchus labrax). Aquat. Toxicol. 168, 72–77. doi: 10.1016/j.aquatox.2015.09.013
Novelli, A. A., Losso, C., Libralato, G., Tagliapietra, D., Pantani, C., and Ghirardini, A. V. (2006). Is the 1: 4 elutriation ratio reliable? Ecotoxicological comparison of four different sediment: water proportions. Ecotoxicol. Environ. Saf. 65, 306–313. doi: 10.1016/j.ecoenv.2005.08.010
Ohkawa, H., Ohishi, N., and Yagi, K. (1979). Assay for lipid peroxides in animal tissues by thiobarbituric acid reaction. Anal. Biochem. 95, 351–358. doi: 10.1016/0003-2697(79)90738-3
Oliveira, M., Ribeiro, A., Hylland, K., and Guilhermino, L. (2013). Single and combined effects of microplastics and pyrene on juveniles (0+ group) of the common goby Pomatoschistus microps (Teleostei, Gobiidae). Ecol. Indic. 34, 641–647. doi: 10.1016/j.ecolind.2013.06.019
Pacheco, M., and Santos, M. A. (1997). Induction of EROD Activity and Genotoxic Effects by Polycyclic Aromatic Hydrocarbons and Resin Acids on the Juvenile Eel (Anguilla anguillaL.). Ecotoxicol. Environ. Saf. 38, 252–259. doi: 10.1006/eesa.1997.1585
Pagano, G., Anselmi, B., Dinnel, P. A., Esposito, A., Guida, M., Iaccarino, M., et al. (1993). Effects on sea urchin fertilization and embryogenesis of water and sediment from two rivers in Campania, Italy. Arch. Environ. Contam. Toxicol. 25, 20–26.
Pauli, N.-C., Petermann, J. S., Lott, C., and Weber, M. (2017). Macrofouling communities and the degradation of plastic bags in the sea: an in situ experiment. R. Soc. Open Sci. 4, 170549. doi: 10.1098/rsos.170549
Paul-Pont, I., Lacroix, C., Fernández, C. G., Hégaret, H., Lambert, C., Le Goïc, N., et al. (2016). Exposure of marine mussels Mytilus spp. to polystyrene microplastics: toxicity and influence on fluoranthene bioaccumulation. Environ. Pollut. 216, 724–737. doi: 10.1016/j.envpol.2016.06.039
Prata, J. C., da Costa, J. P., Lopes, I., Duarte, A. C., and Rocha-Santos, T. (2019). Effects of microplastics on microalgae populations: A critical review. Sci. Total Environ 665, 400–405. doi: 10.1016/j.scitotenv.2019.02.132
Rodríguez-Romero, A., Khosrovyan, A., DelValls, T. A., and Riba, I. (2016). Dredged material characterization and management frameworks: A case study at the port Vilagarcia (NW, Spain). J. Hazard. Mater. 302, 129–136. doi: 10.1016/j.jhazmat.2015.09.034
Sforzini, S., Oliveri, L., Chinaglia, S., and Viarengo, A. (2016). Application of biotests for the determination of soil ecotoxicity after exposure to biodegradable plastics. Front. Environ. Sci. 4:68. doi: 10.3389/fenvs.2016.00068
Sjollema, S. B., Redondo-Hasselerharm, P., Leslie, H. A., Kraak, M. H., and Vethaak, A. D. (2016). Do plastic particles affect microalgal photosynthesis and growth? Aquat. Toxicol. 170, 259–261. doi: 10.1016/j.aquatox.2015.12.002
Smith, I. R. (1990). Erythrocytic micronuclei in wild fish from Lakes Superior and Ontario that have pollution-associated neoplasia. J. Gt. Lakes Res. 16, 139–142. doi: 10.1016/s0380-1330(90)71406-2
Tosin, M., Weber, M., Siotto, M., Lott, C., and Degli-Innocenti, F. (2012). Laboratory test methods to determine the degradation of plastics in marine environmental conditions. Front. Microbiol. 3:225. doi: 10.3389/fmicb.2012.00225
Vinagre, C., Madeira, D., Narciso, L., Cabral, H. N., and Diniz, M. (2012). Effect of temperature on oxidative stress in fish: Lipid peroxidation and catalase activity in the muscle of juvenile seabass, Dicentrarchus labrax. Ecol. Indic. 23, 274–279. doi: 10.1016/j.ecolind.2012.04.009
Keywords: biodegradable plastic, ecotoxicology, sea bass, sea urchin, algae
Citation: Campani T, Casini S, Caliani I, Pretti C and Fossi MC (2020) Ecotoxicological Investigation in Three Model Species Exposed to Elutriates of Marine Sediments Inoculated With Bioplastics. Front. Mar. Sci. 7:229. doi: 10.3389/fmars.2020.00229
Received: 27 January 2020; Accepted: 25 March 2020;
Published: 17 April 2020.
Edited by:
Patrick Reis-Santos, University of Adelaide, AustraliaReviewed by:
Chiara Gambardella, Italian National Research Council, ItalyMaria Violetta Brundo, University of Catania, Italy
Copyright © 2020 Campani, Casini, Caliani, Pretti and Fossi. This is an open-access article distributed under the terms of the Creative Commons Attribution License (CC BY). The use, distribution or reproduction in other forums is permitted, provided the original author(s) and the copyright owner(s) are credited and that the original publication in this journal is cited, in accordance with accepted academic practice. No use, distribution or reproduction is permitted which does not comply with these terms.
*Correspondence: Silvia Casini, U2lsdmlhLmNhc2luaUB1bmlzaS5pdA==