- 1National Marine Science Centre, Southern Cross University, Coffs Harbour, NSW, Australia
- 2Australian Institute of Marine Science, Townsville, QLD, Australia
The tegulid gastropod, Rochia nilotica is harvested in small-scale fisheries throughout Southeast Asia and the Pacific Islands, many of which were created from inter-country translocations. This species is found on structurally complex shallow reefs, but its specific habitat requirements have not yet been quantified in order to maximize the success of future translocations and help future-proof the fishery in changing ocean conditions. At 28 sites around Samoa, where the species was introduced in the early 2000s, we measured a suite of habitat variables along transects in which R. nilotica were counted and the shell sizes measured in a parallel study. Boosted regression tree analyses revealed that R. nilotica were most abundant at reef locations that were shallow, with fairly consistent depth, had high coverage of branching coral, low cover of erect macroalgae, low wave exposure and high surface complexity. Smaller individuals were associated with wide reef flats and high cover of branching coral, whereas larger animals occurred in deeper water with high surface complexity. Multivariate analyses showed this species to be a habitat generalist, sharing much of its niche with an endemic herbivorous gastropod, Tectus pyramis. Future stocking programs should focus on sites with habitats optimal for both adults and juveniles. R. nilotica populations are likely to be especially affected by broadscale stressors that result in declines in live coral cover and substratum complexity and increasing coverage of macroalgae on coral reefs.
Introduction
Coral reefs are naturally highly complex and provide habitat for a diverse suite of marine species (Spalding et al., 2001; Hoegh-Guldberg et al., 2007). Important habitat characteristics may include: substratum type, cover of benthic biota, reef surface complexity, wave exposure, and depth (McCormick, 1994; Graham and Nash, 2013; Komyakova et al., 2013). These habitat features are likely to influence key ecological processes such as recruitment, competition, foraging and predation (Luckhurst and Luckhurst, 1978; McCormick, 1994; Graham and Nash, 2013). In order to understand population patterns, we therefore require a clear understanding of the habitat requirements of animals in relation to potential biophysical factors. Such knowledge can reveal habitat traits driving distributional patterns of marine invertebrates and, importantly, enhance the success of stock restoration projects by identifying optimal deployment locations (Bell et al., 2005).
Coral reefs are increasingly under threat from global climate change through rises in sea temperature, ocean acidification, coral bleaching, and an increased frequency and severity of storm events (Hoegh-Guldberg et al., 2007; Ham, 2018; Hughes et al., 2018). These stressors and other disturbances such as coral-eating starfish, fishing pressure, and pollution threaten coral reefs through the reduction in reef surface complexity, loss of live coral cover, and phase-shifts to macroalgal dominated systems (Hoegh-Guldberg et al., 2007; Fabricius et al., 2013; Hughes et al., 2018). Changes in coral-reef habitats can have serious consequences for substrate-dependent species, including altering distribution patterns and species interactions, often leading to reductions in population abundance (Przeslawski et al., 2008; Bozec et al., 2015; Hughes et al., 2018).
Subsistence and commercial fisheries often form the primary basis for economic development, food security, and coastal livelihoods in Pacific Islands (Wright and Hill, 1993; Bell et al., 2013; Gillett and Tauati, 2018). The most socio-economically important invertebrate resources are sea cucumbers, giant clams, lobsters, octopus, and gastropods (Gillett and Tauati, 2018). In efforts to maintain or enhance stocks, certain invertebrates have been translocated to reefs throughout the Pacific Islands (Bell et al., 2005). Broodstock of two marine snails, Rochia nilotica (trochus) and Turbo marmoratus (green snail) have been translocated on numerous occasions to localities beyond their natural distributions, with the intention of creating new populations of self-replenishing stocks (Purcell and Cheng, 2010; Gillett and Tauati, 2018). Considerable research effort is usually required to maximize the success and cost-effectiveness of such programs, often necessitating lengthy studies of optimal conditions (Bell et al., 2005). However, for many fisheries, including for R. nilotica, these conditions are often poorly defined.
The herbivorous gastropod, R. nilotica (formerly Trochus niloticus, then Tectus niloticus) (WoRMS, 2020) is one of the largest members of the order Trochida, with a basal shell width (BSW) of up to 150 mm (Smith, 1987; Nash, 1993; Figure 1). R. nilotica grows at an estimate of 25 mm per year in the first 3 years and 15 mm per year thereafter (Heslinga, 1981; Smith, 1987). R. nilotica reach sexual maturity between 50 and 90 mm BSW and can live up to 10–15 years (Nash, 1993).
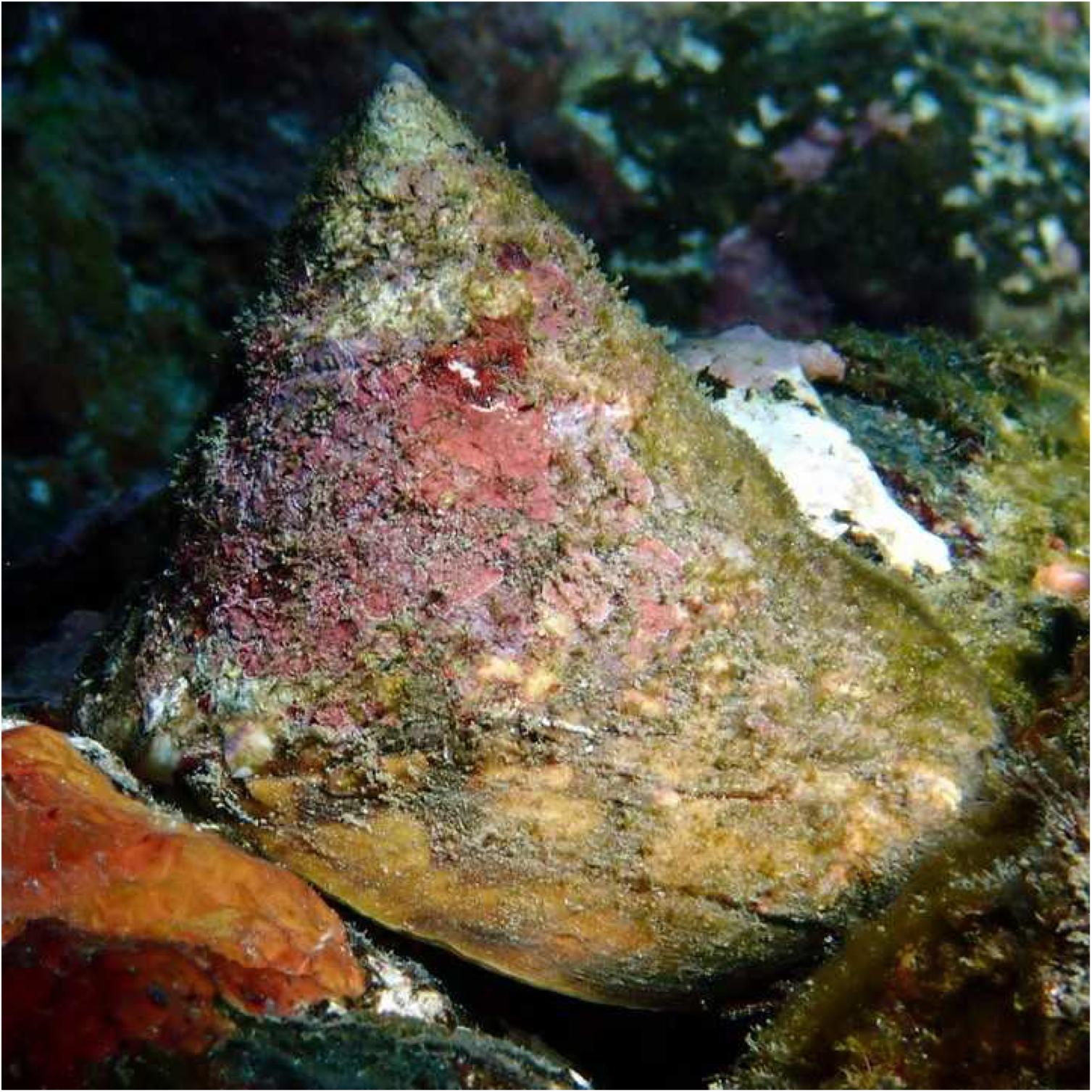
Figure 1. Rochia nilotica on the reef in Samoa (illustrated specimen ∼90 mm BSW) (Photo: Steven Purcell).
R. nilotica is found on coral reefs with suitable habitat for all life-history stages (Pakoa et al., 2010; Dumas et al., 2017). The larval phase is characteristically short (3–7 days) and larvae are believed to settle close to natal sites (Smith, 1987; Lee et al., 2001). The juveniles tend to be found mostly on intertidal reef flats with high cover of consolidated coral rubble and turfing algae and high reef surface complexity (Castell, 1997; Colquhoun, 2001; Crowe et al., 2002). Adults tend to inhabit consolidated, rocky forereef zones (Long et al., 1993; Purcell et al., 2009; Dumas et al., 2013), typically in depths shallower than 10 m (Nash, 1993; Rees et al., 2003). Optimal habitat features for adults are believed to be a high percentage of coral cover, low sand cover, abundant turfing algae, and a complex reef surface, although habitat associations are spatially variable (Colquhoun, 2001; Purcell et al., 2009; Ceccarelli et al., 2011).
Rochia nilotica is a non-selective herbivore, feeding on turfing, and encrusting algae (Nash, 1993). Potential competitors for food resources could include Trochus maculatus, Tectus pyramis, Turbo chrysostomus, and Turbo argyrostomus (Gosliner et al., 1996; Tiitii and Aiafi, 2016). The native T. pyramis is harvested by subsistence fishers in Samoa and fishing pressure has previously led to diminished stocks (Taule’alo, 1993).
With an aim to enhance resources for subsistence fishers, R. nilotica was first introduced to a far-eastern site in Samoa in 1990 (Gillett, 1993; Lober et al., 2003). A total 118 individuals were translocated to Samoa from Fiji and an unknown number translocated from Tonga, however, F1 populations were not established (Gillett, 1993; Tiitii and Aiafi, 2016). Broodstock were further introduced from Vanuatu and Fiji in 2003 and 2004, where ∼360 individuals were distributed at three sites across two operations (Lober et al., 2003; Tiitii and Aiafi, 2016). In 2004, 500 locally cultured juveniles were stocked at additional sites (Lober et al., 2003; Tiitii and Aiafi, 2016). These stocking events led to populations being established around both of the main islands, Upolu and Savai’i (Tiitii and Aiafi, 2016). Socioeconomic surveys of fishers in 2018 suggested that the populations became well established only in the past 4–10 years, depending on the location (Purcell et al., unpublished data).
The primary aim of this study was to determine the associations of R. nilotica densities and shell sizes with a range of biophysical habitat features. To ensure our surveys found R. nilotica within ample abundances for future analysis, we aimed to assess the most suitable habitat reported in the primary literature. Such data can inform decisions about the best sites for future stocking in the Indo-Pacific and aid in forecasting future shifts in fisheries productivity and ecosystem services of this important species in rapidly changing oceans. A previous study by Dumas et al. (2013) investigated “the influence of small-scale (101 m) habitat structure” and the related distribution of R. nilotica and examined sediment type and substratum coverage. Dumas et al. (2017) similarly examined associations of R. nilotica with sediment type and substratum coverage. We make significant advances on that approach by examining larger-scale habitat variables and incorporating physical variables (wave exposure, reef width from shore, reef topography, substratum complexity), leading to a more comprehensive evaluation of habitat associations to guide future stocking of this key species. Our broad sampling scale (28 sites across 350 km of coastline in Samoa) permits inferences to a broad range of reef types and conditions which, together with our modeling approach using boosted regression trees (BRTs), offers a template for future research on habitat associations of other marine taxa.
Our secondary objective was to provide insights into the partial niche breadth of R. nilotica and identify potential niche overlap with other common, herbivorous gastropods. Such information forms a basis for debate about the potential ecological consequences of translocations. Reefs on the northern coast of Upolu island in Samoa have also been partly degraded by pollution, sedimentation and nutrient inputs (Ziegler et al., 2018). Across many reef sites in Samoa, the coverage of live coral has declined recently, attributed to coral bleaching events associated with thermal stress from climate change, crown-of-thorns starfish, and cyclones (Samuelu and Sapatu, 2009; Ziegler et al., 2018). We discuss our findings about habitat relationships of R. nilotica in the context of impacts of such major stressors on reef habitats. The approach we use to examine habitat associations to infer about vulnerability of this species to changing habitats is widely used throughout other studies. This study has broad significance, not just for R. nilotica as an important resource in Samoa and throughout the Pacific, but also as it may provide insights into considerations about habitats for stock restoration and enhancement of other marine invertebrates.
Materials and Methods
Study Site
Samoa is an island country in the central Pacific, between latitudes 13°S and 14°S and longitudes 171°W and 173°W (BOM and CSIRO, 2011). Samoa’s volcanic geology provides coastlines of old lava flows which support >10,000 km2 of narrow fringing coral reef (Taule’alo, 1993; MNRE, 2013). The reefs are mostly characterized by shallow lagoon and patch reef extending out to the high energy reef crest and steep reef slope (MAF, 1997; Spalding et al., 2001), however, specific geospatial data on reef types across the Samoan coastline were unavailable.
Coral bleaching, tropical storms, cyclones, and flooding have led to recent loss of live coral across reefs in Samoa (MNRE, 2010, 2013; Ziegler et al., 2018). Indeed, many reefs are currently dominated by algal-covered dead coral, indicating relatively recent coral mortality, some of which may be related to mass bleaching events between 2014 and 2017 (Berthe et al., 2016; Ziegler et al., 2018). While coral cover in Samoa was moderately high in the early 2000s (Samuelu and Sapatu, 2009; MNRE, 2010) cover has now declined to <1% on some reefs (Berthe et al., 2016; Ziegler et al., 2018).
Study Design
Habitat assessments were conducted concurrently with a broader population assessment (Purcell and Ceccarelli, unpublished data). Underwater visual censuses and habitat surveys were conducted at 28 coral reef sites surrounding the two main islands (Upolu and Savai’i, Figure 2) from January to March 2018. The sites were chosen on the boat, before entering the water to conduct surveys, in a haphazard fashion in order to avoid surveyor bias and to enable a broad range of reef sites to be surveyed. Each site extended 200–300 m across the reef front with sites spaced at least 5 km apart. Habitat assessments were conducted on independent (>10 m apart), replicated (n = 4–8), 50 × 2 m transects, positioned haphazardly on the reef crest and reef slope at depths between 1 and 10 m. A total of 155 transects across 28 sites were surveyed, with 81 transects on Upolu and 74 on Savai’i. Transects shallower than 5 m were surveyed on snorkel and deeper transects were surveyed using SCUBA. Apart from the site of Lano on Savai’i, which was on the reef flat behind the crest due to reasons of field safety, the other sites were situated on the reef crest and upper reef slope habitats. This habitat has been shown to be the most important, by far, for populations of R. nilotica in Samoa and elsewhere in the Pacific (Purcell et al., 2009; Tiitii and Aiafi, 2016), and so was the focus of the population assessment. This strategy to focus on reef fronts maximized the chance of finding R. nilotica at sites, if they existed there. The reef sites covered a wide range of conditions, including orientation to predominant winds, varying extent of wave exposure, and distance from shore. Our data collected along transects also show that the sites covered a broad spectrum of habitat conditions including depth, coral cover, substratum complexity, and transect-scale topography. Hence, while being focused on reef front habitats, the array of site features and habitat conditions offers a broad capacity for extrapolating the results beyond these sites.
Population Assessments
All R. nilotica found during our field searches were counted; this involved carefully inspecting under ledges, in holes and crevices. The maximum BSW of the first 16 individuals was measured to the nearest mm in situ, which provided ample replication for calculating average shell sizes and satisfied time constraints. To assess the potential niche overlap with other common gastropods, the number of T. maculatus, T. pyramis, T. chrysostomus, and T. argyrostomus were also counted on each transect. The latter two were pooled due to low replication and to overcome difficulty in identification in the field.
Habitat Assessments
Habitat assessments involved measuring reef surface complexity, benthic cover, depth, topographic complexity, densities of Echinostrephus sp. (a small boring sea urchin), reef distance from shore, and site exposure. Reef surface complexity was measured using the “chain and tape” method at three predetermined random points on each transect and averaged to provide an index of rugosity (Luckhurst and Luckhurst, 1978).
Coral Point Count with Excel extensions (CPCe) (Kohler and Gill, 2006) software was used to quantify the cover of benthic organisms and substratum types from eight randomly allocated replicate photographs taken at a fixed distance from the substrate (0.7 m) with a 0.57-m2 (92 cm × 62 cm) field-of-view. A total of 1240 photos was imported into CPCe and the software overlaid each photo with 20 randomly distributed points. Habitat underlying each point was allocated to one of the following categories: turf algae, crustose coralline algae, unconsolidated benthos, consolidated benthos, macroalgae, invertebrates, dead coral, other coral, encrusting coral, branching coral, and plate coral (Table 1). The number of Echinostrephus sp. on each photo was counted and averaged to provide a density across each transect.
Depth was measured using a digital depth gauge at eight predetermined random points and at the start and end of each transect. Tidal records were obtained using WS Tides software and depth measurements were corrected to zero tidal datum. Topographic complexity was obtained by calculating the standard deviation of the depth measurements on each transect as a summary metric of reef topography at the transect scale. The distance from the shore to the reef crest (reef distance) was determined as the average of measurements from three points on each transect using Google Earth. Exposure to high energy over the reef crest was assessed at each site using a rank system defined by the sites’ physical surrounding, wave exposure, surge potential, and orientation to dominant winds and currents (Table 2). Five Samoan fishery officers independently scored each site, assigning an exposure rank between 1 and 5, and an average rank score was calculated across officers for each site.
Statistical Analyses
The strength of the association between each environmental variable and the density and size of R. nilotica was estimated via boosted regression tree (BRT) analyses (De’ath, 2007; Elith et al., 2008; Ridgeway, 2018). Analyses of size relationships used the average size of R. nilotica on each transect and excluded transects lacking R. nilotica. Each BRT was run against a Gaussian distribution and included 1000 trees with an interaction depth of 4, bag ratio of 0.5 and shrinkage of 0.01. Overfitting was controlled with the use of three cross-validation folds (Ridgeway, 2018). The relative influence of each covariate was estimated as the proportion of all tree splits involving the focal covariate. The relative influence associated with a covariate was considered substantial if it exceeded 100/p where p is the number of covariates. Partial dependency plots, in which predictions were plotted against single covariates, were used to explore the nature of associations. All partial dependencies were considered non-monotonic and there were no correlations between predictor variables. Ninety-five percent confidence intervals on partial effects and relative influences were provided by bootstrapping the BRTs 100 times (each with a random subset of the original data). All BRTs were performed using the gbr package in R (Ridgeway, 2018).
To compare partial niches among the target species, differences in their associations with habitat and environmental variables were explored using analysis of similarity (ANOSIM) and similarity percentage (SIMPER) routines in PRIMER v7 using the density of each species (Clarke and Gorley, 2015). Square-root and log transformations of the environmental variables were conducted to improve normality where appropriate. The transformed dataset was standardized, and a resemblance matrix was formulated based on Bray–Curtis similarities. ANOSIM was performed using a one-way unordered design and Spearman rank correlation. SIMPER was performed on the Bray–Curtis resemblance matrix of predictor variables using a one-way design and 50% similarity cut-off.
Results
Habitat Characteristics
Habitat variables differed considerably among sites, allowing for relationships with R. nilotica to be examined over a broad spectrum of conditions (Supplementary Table 1). The average depth of the reef across our sites was 3.3 ± 2.2 m, within a range of 0.3–11.4 m. The topographic complexity (i.e., the variability of depth at the transect scale) ranged from 0.1 to 2.6 m and was on average 0.6 ± 0.3 m. The variability in topographic complexity reflects some reefs with prominent spur and groove formations, however, we found that most of the reefs had fairly consistent depths across the reef front. Reefs were comprised of consolidated dead coral and rocky pavement forming a matrix of holes, crevasses, and ledges, the reef surfaces were typically complex with an average rugosity of 1.5 ± 0.8. The reef distance, a measure of the lagoon and reef flat between the shore and reef crest was highly variable, ranging from 0.1 to 2.2 km. Reef crests were considerably exposed with an average rank of 3 across all sites. Site exposure ranged from sheltered sites in a bay to fully exposed sites such as those across steep rocky shores exposed to dominant winds and swells. Turfing algae and crustose coralline algae dominated benthic cover with average values of 29 ± 12% and 39 ± 16%, respectively. The cover of algae was highly variable, ranging from 6–60% for turf algae and 0–80% for crustose coralline algae. The cover of live coral was generally low ranging from 0–46% and averaging 11 ± 10%, with only 5 out of 28 sites having average live coral cover over 20%. The dominant growth forms of live coral were encrusting and branching coral.
R. nilotica Density
In the transects examined in this study, a total of 433 R. nilotica were found on 64 out of the 155 transects at 18 of the 28 sites. The BRT modeling found that the best predictors for high densities of R. nilotica were shallow depths (0–1.5 m), high coverage of branching coral (>10%), low transect-scale topographic complexity (standard deviation in depth along transects <0.3), low site exposure, high reef surface complexity (surface contour distance >1.5 times greater than linear distance) and low cover of erect macroalgae (<3%) (Figure 3).
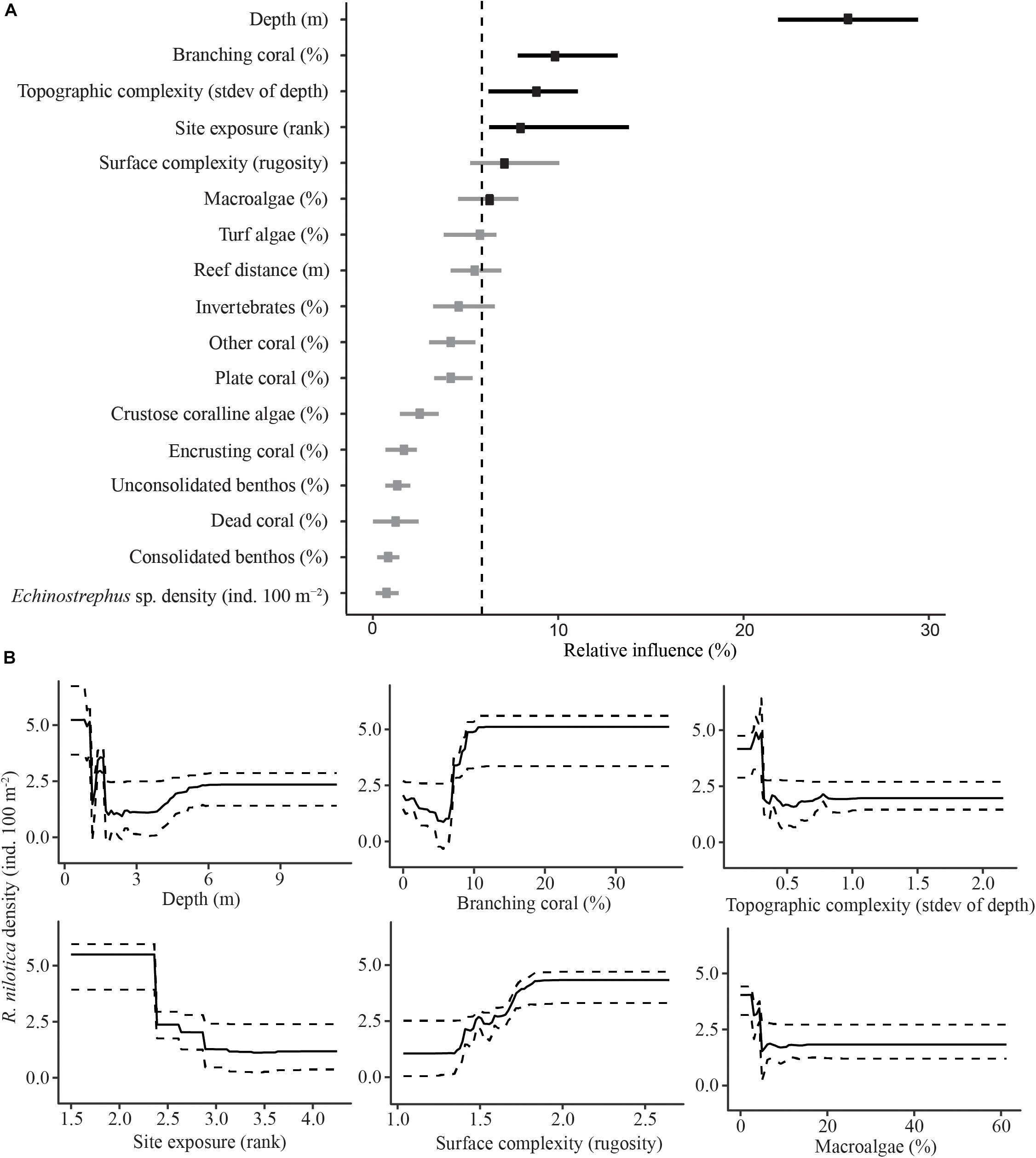
Figure 3. Boosted Regression Tree relative influence plot for predictor variables of R. nilotica density (A). Dots represent means for each predictor variable and bars represent upper and lower 95% quantiles. The vertical dashed line represents the significance threshold (100/17), variables exceeding the threshold are in black and those that do not are gray. Partial effects for significant predictor variables against R. nilotica density (B). Solid lines represent means for each predictor variable and dashed lines represent upper and lower 95% quantiles.
R. nilotica Size Distributions
Shell diameter of the 295 R. nilotica found along transects averaged 87 ± 19 mm; range: 35–136 mm. The BRT model suggests that R. nilotica shells were larger in places with greater depth (>2.5 m) and where the coverage of other sessile invertebrates was high (>4%) and, to a lesser extent, where the reef surface was reasonably complex (Figure 4). The modeling also indicates that smaller R. nilotica were found at shallow depths (0–2.5 m), and where the reef flat was wider than ∼1 km between shore and the reef crest, where there was at least ∼8% coverage of branching coral and reasonable (>1.5) surface complexity (Figure 4).
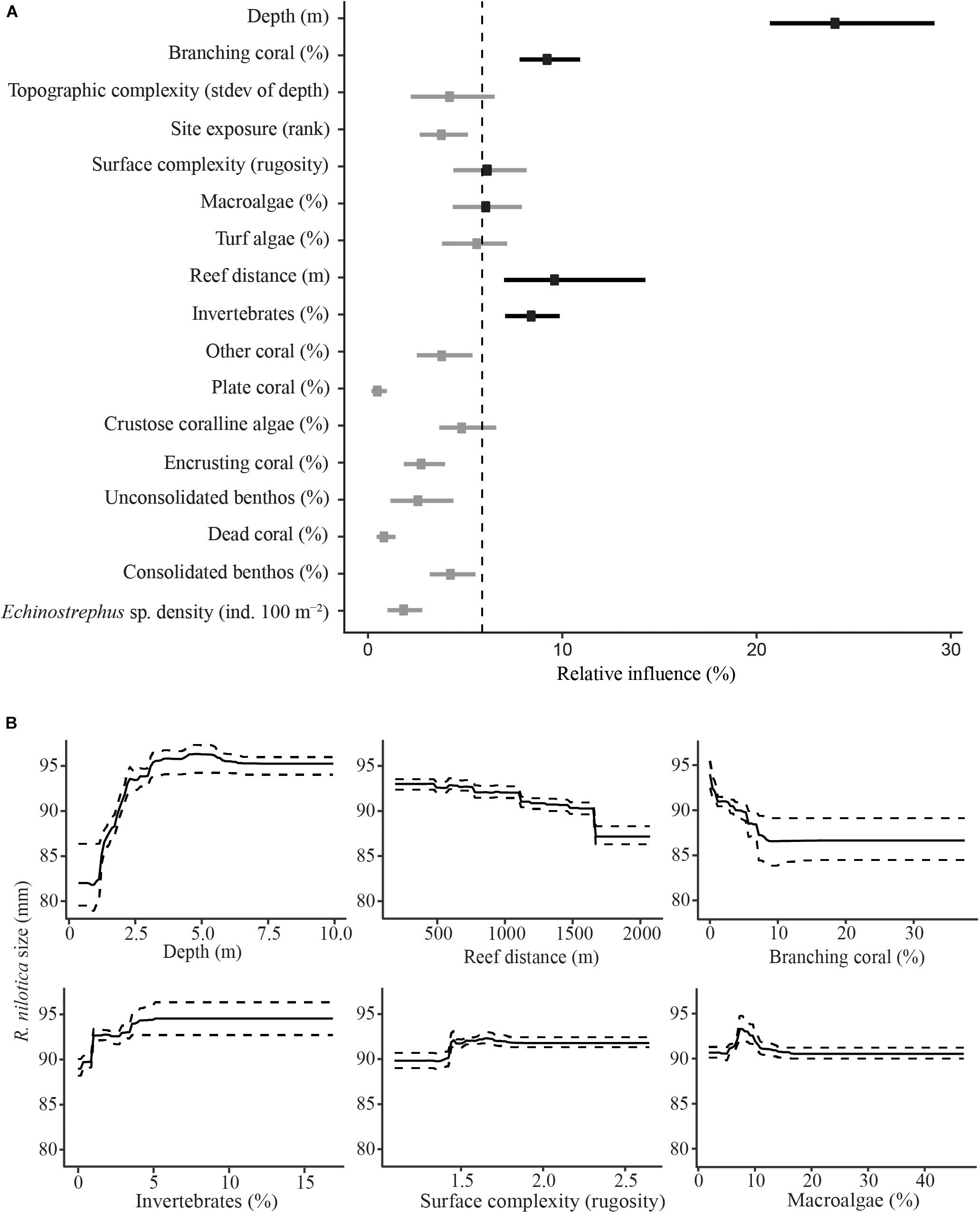
Figure 4. Boosted Regression Tree relative influence plot for predictor variables of R. nilotica size (A). Dots represent means for each predictor variable and bars represent upper and lower 95% quantiles. The vertical dashed line represents the significance threshold (100/17), variables exceeding the threshold are in black and those that do not are gray. Partial effects for significant predictor variables against R. nilotica size (B). Solid lines represent means for each predictor variable and dashed lines represent upper and lower 95% quantiles.
Niche Breadth and Overlap
We found a total of 69 T. maculatus on 42 transects, 25 T. pyramis on 20 transects and 16 T. chrysostomus/T. argyrostomus on 14 transects. There was a significant segregation in habitat niche among the studied species (ANOSIM: R = 0.03, p = 0.003). ANOSIM revealed that R. nilotica share some of the same habitat as the native T. pyramis but occupy different habitat to T. maculatus and T. chrysostomus/T. argyrostomus (Table 3).
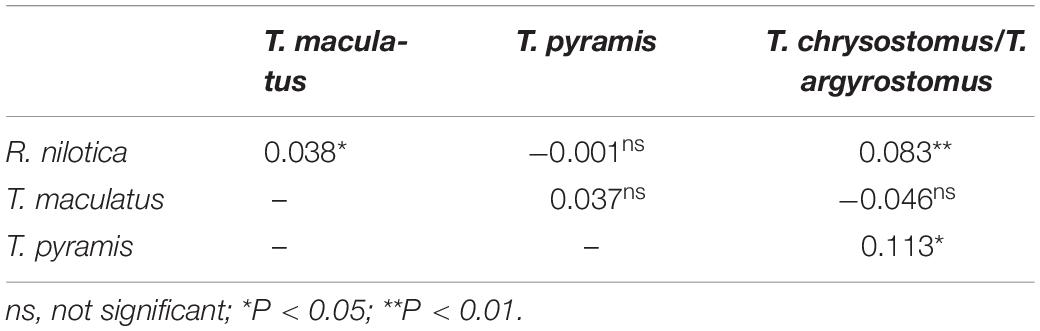
Table 3. Analysis of Similarity test (ANOSIM), pairwise R values and statistical significance levels for habitat variables relating to the density of different gastropod species.
Each gastropod species had a wide niche, displaying generalist habitat requirements (Table 4). The SIMPER routine found that site exposure was a key variable affecting the density of all studied species and depth was important for three species (including R. nilotica) (Table 4). The topshell gastropods (Trochida) were strongly associated with reef areas that had a high degree of rugosity (fine-scale substratum complexity), but not the Turbo species, whilst larger-scale topographic complexity (at the transect scale) was important for all species other than R. nilotica. Densities of R. nilotica were greatest at sites where the reef crest was far from shore, allowing for an extensive reef flat habitat, but this habitat feature did not appear to be associated closely with any other studied species.
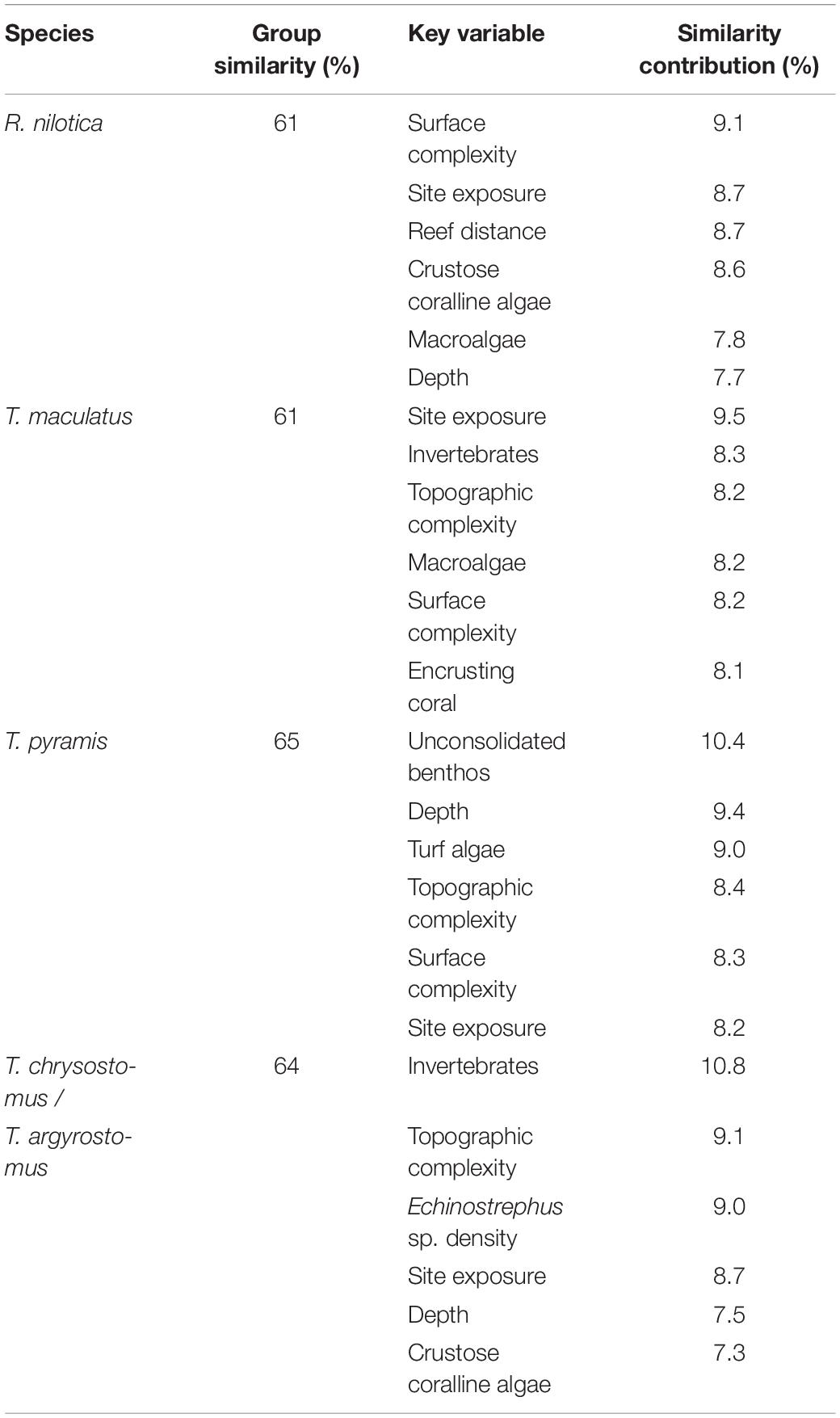
Table 4. Similarity percentage routine (SIMPER) on the habitat variables relating to the density of each gastropod species, displaying the key habitat variables that altogether contributed at least 50% to habitat similarity among replicates within each species.
Discussion
The present study provides a comprehensive approach to examining the relationships between benthic reef invertebrates and a range of biophysical habitat features. This study revealed the strong influence of physical habitat variables not assessed in previous studies, such as depth, site exposure, reef distance, and substratum complexity. We also demonstrate that physical habitat variables need to be measured and analyzed alongside traditional measures of substratum coverage of benthic biota in order to provide a complete evaluation of habitat associations relevant to site selection for future stocking programs. While previous studies have provided assessments of densities and sizes of R. nilotica across different reef types, they have not directly linked populations with specific habitat features (Smith, 1987; Dolorosa et al., 2010; Pakoa et al., 2010). Other studies have assessed R. nilotica habitats using multivariate analyses of sediment type and substratum coverage variables (Dumas et al., 2013, 2017). We demonstrate the advantage of a multivariate approach to view multiple possible predictor variables within the analysis. These analyses provide a sophisticated approach to visualize the relative influence of each predictor variable, whilst holding all other variables constant in their influence. Our framework of measuring both biological and physical features of sites and analysis with BRTs offers a useful template for future studies of habitat associations of other marine taxa.
The most influential habitat features in this study pertained to reef configuration, physical forces, and fine-scale substratum structure at the site. R. nilotica displayed characteristics of a generalist species, it is therefore not surprising that there has been so much success in translocating R. nilotica throughout the Pacific despite reef habitat features varying from one country to another. The clear associations among biophysical variables and the densities and sizes of R. nilotica provides further insight into the reasons for the relative success of introductions and guidelines for stock-enhancement programs. Nevertheless, we found that R. nilotica lives most abundantly at reef sites with features predicted to be strongly modified by climate change and other stressors. Our study identifies key habitat features for future stocking programs yet paints an uncertain future for productive R. nilotica fisheries due to declines of reef-building corals on tropical reefs.
Habitat Associations
Among the focal species (R. nilotica) and the other three recorded species, the prevalent association of depth, surface complexity, site exposure, and coral cover indicates that these can be influential characteristics driving the distributions of herbivorous gastropods on coral reefs. Highest densities of R. nilotica should be expected on shallow reefs with a relatively consistent depth across the reef front and complex surface habitat, corroborating earlier speculation in the literature (Smith, 1987; Rees et al., 2003; Purcell et al., 2009; Dolorosa et al., 2010). R. nilotica have previously been found to inhabit the higher-energy reef crest in their highest abundance with declining numbers onto the outer reef slope into deeper waters (Ceccarelli et al., 2011). The present study found an apparent gradation in the distribution of different-sized individuals, with smaller animals having higher abundance in shallow depths, especially on the reef crest. Larger individuals were more commonly found in deeper waters on the outer reef slope, which supports the long-held hypothesis of ontogenetic migration to deeper waters (Nash, 1993; Castell, 1997).
The topshells were positively associated with reef surface complexity and this may be owing to their cryptic nature of requiring physical refugia (Gosliner et al., 1996; Dolorosa et al., 2010; Soekendarsi, 2018). The surface structural complexity of the reef is considered as an important factor dictating the distribution of marine invertebrates including R. nilotica (Ceccarelli et al., 2011; Dumas et al., 2013; Fabricius et al., 2013). The most suitable habitat for promoting high densities of R. nilotica are those with a high degree of structural complexity. High structural complexity provides holes and crevices within the reef in which individuals can seek refuge from predation (Komyakova et al., 2013; Bozec et al., 2015; Dumas et al., 2017).
We posit that the narrow fringing reef sites in Samoa might have sparse densities of R. nilotica because they have high wave exposure and lack an extensive reef flat habitat (MAF, 1997; Spalding et al., 2001). In this study, R. nilotica were typically found at sites with moderate-low wave exposure, however, this notion is contested throughout the literature, with dense populations found at wave-exposed sites in some cases (Rees et al., 2003; Pakoa et al., 2010) but not others (Smith, 1987). Our study suggests that the optimal habitat for R. nilotica are those with a moderate degree of wave exposure. The incongruence with our findings might be attributable to different measures of exposure among studies, leading to differing interpretation of results.
The present analyses predict that R. nilotica populations establish better on reefs at greater distance from shore (∼1 km between shore and reef crest). We found that juvenile R. nilotica associated with extensive reef flats and the requirement of the reef flat as a nursery habitat is well documented (Nash, 1993; Castell, 1997; Purcell and Cheng, 2010). Certain sites might possess suitable reef crest and forereef slope habitats but lack a wide reef flat needed for settlement and development of juveniles (Nash, 1993; Castell, 1997), such as across the south-eastern coastline of Savai’i in Samoa. The most suitable reefs for R. nilotica populations to establish are those which possess the reef types that are optimal for both the juvenile and adult, within close proximity. This may help to explain why R. nilotica were sparse or absent at certain sites that otherwise have reef crests and fore-reef slopes that appear to be excellent habitat for adult populations.
Our findings are congruent with previous studies indicating that a moderate cover of live coral is influential in typifying higher densities of marine invertebrates (Pakoa et al., 2010; Dumas et al., 2013, 2017). Apparently R. nilotica settle and establish on healthy coral reefs, therefore the conservation values of these healthy reefs are important for the ongoing success of the fishery. This study further supports previous studies that R. nilotica are mostly found on coral reefs and do not favor areas with an abundance of erect macroalgae (Long et al., 1993). The cover of branching coral appears to play an important role for the size distribution of R. nilotica, whereby smaller individuals associated with higher cover of branching coral. Thus, we should expect to find higher abundance of smaller R. nilotica on reefs that have >10% cover of live branching coral. In previous studies, juvenile R. nilotica have been associated with rocky substrata and lagoon reefs with ample coral rubble, however, the cover of live coral in these studies was often quite low and patchy (Castell, 1997; Colquhoun, 2001; Pakoa et al., 2010). Extensive cover of branching coral may be favorable as settlement sites for protection; however, these relationships have not been widely explored for marine gastropods.
Future Invertebrate Translocations in the Indo-Pacific
There have been limited negative environmental or ecological consequences reported from previous R. nilotica introductions (Fao, 1992; Bell et al., 2005). Coral reefs throughout the Pacific have supported exotic invertebrate introductions and are believed to have capacity to support a wide range of herbivorous grazers (Fao, 1992; Bell et al., 2005; Vunisea et al., 2008). Our results suggest that all four gastropod species are generalists and can occupy a wide range of habitat conditions. Within the forereef habitat zones studied here, R. nilotica shared similar niche requirements with T. pyramis and it is likely that this is due to their taxonomic and biological similarities. Dumas et al. (2013) also examined habitats of T. pyramis and concluded that it and R. nilotica “share very similar ecological niches,” although their study did not show differences in habitat occupancy of the two species. We are cautious in our interpretation of niche of the other gastropod species due to their low replication in this instance. In this study we were unable to study all potential habitats, therefore limiting our interpretations of niche breadth. Our data types and multivariate analyses have been used to examine niche breadth in a wide range of other marine species, so this is not a novel approach (Dumas et al., 2013; Purcell et al., 2014; Jankowski et al., 2015; Bennice et al., 2018), such information forms a basis for debate about the potential ecological consequences of translocations. We are also cautious about inferring any competition based on overlap in habitat associations, since it may be that food and refugia might not be limiting in many cases. Whilst T. pyramis numbers in this study were fairly low, previous surveys indicated that T. pyramis populations were fairly patchy and moderately abundant prior to R. nilotica introductions (Vunisea et al., 2008).
Future studies for marine invertebrate habitat should consider a multivariate approach, including a range of biophysical characteristics such as depth, availability of juvenile habitat, reef exposure, substratum complexity and substratum cover of benthic biota. Our findings offer additional guidance for future stocking efforts of R. nilotica, by identifying areas where translocations might be sub-optimal. Sites should have a moderate cover of live coral (>10%) and a good cover of turfing algae (>40%) to provide food supply for grazing invertebrates. The reef flat should be extensive and highly structurally complex to provide holes and crevices for gastropods to seek refuge (Colquhoun, 2001; Fabricius et al., 2013; Dumas et al., 2017). Our results strongly suggest that the failure of initial translocation at some sites is most likely related to their high wave exposure, relatively low coral cover, and low surface complexity. Two of the three initial seeding sites (to which R. nilotica adults were first transplanted) appear to have habitats unsuitable for fostering abundant populations of R. nilotica in the long term. While these sites have evidently provided suitable conditions for adults to survive and spawn, they are likely to have been sub-optimal for substantive colonization by their progeny. For fisheries, these findings infer that higher productivity can be expected where both juvenile and adult habitats are present (Smith, 1987).
Reef Degradation and Invertebrate Fisheries Productivity
At many sites around both Upolu and Savai’i islands, dead coral skeletons dominated the seascape. Few sites appear to have been spared by recent impacts of broadscale stressors and retained a moderate cover of live hard coral (5 of 28 sites had greater than 20% live hard coral cover; Supplementary Table 1). The turfing and fleshy encrusting algae covering the coral skeletons has offered an abundant food supply to herbivores such as R. nilotica. The proliferation of this introduced topshell might be helping to maintaining top-down control over these smaller algal types, thus contributing to coral recovery (Francis et al., 2019). However, the indirect effects of coral loss on this gastropod species should be considered.
The predicted indirect impacts of climate change and other broadscale stressors on reef fishes are well reported (Roessig et al., 2004; Munday et al., 2008; Pratchett et al., 2008) but few reports provide evidence for susceptibility of reef invertebrates, many of which are vital for coastal livelihoods and food security (Przeslawski et al., 2008). The degree of climate change stressors as well as other anthropogenic threats could impact corals and, in turn, affect the habitats to which R. nilotica closely associate. Hard corals, especially branching and plate growth types, enhance the surface complexity of reef substrata (Alvarez-Filip et al., 2009; Graham and Nash, 2013), but with the decline of these reef builders the complexity of coral reefs is also predicted to decline (Graham et al., 2006; Fabricius et al., 2013; Bozec et al., 2015). Our results indicate that R. nilotica need high surface complexity to enable dense populations, implying that fisheries of this topshell are likely to be strongly affected by broadscale stressors that threaten reef-building corals.
The impacts of declining coverage of hard corals can shift benthic community dominance towards fleshy macroalgae on Indo-Pacific reefs (Diaz-Pulido et al., 2009; Graham et al., 2015), although effects might not be universal across algal types (Anthony et al., 2011; Bender-Champ et al., 2017). Our analyses illustrate that the highest densities of R. nilotica occurred where macroalgae cover was particularly low (<5% cover). These findings suggest that a growing prevalence of fleshy macroalgae on reefs through impacts of climate change and other broadscale stressors (Hughes et al., 2018), or coastal eutrophication, will further disfavor R. nilotica populations and fishery productivity.
Through the habitat associations of R. nilotica, this study furnishes evidence to link expected impacts of coral degradation to the productivity of invertebrate fisheries. R. nilotica have shown to closely associate with habitat characteristics that may be predicted to change such as, high structural complexity, live coral cover and algal phase shifts, this can be used to infer their vulnerability to broad-scale change to reef habitats that can occur from climate change. The R. nilotica fishery in Samoa has been praised as a success story of Australian foreign aid and coastal communities are gaining a livelihood benefit from the fishery. Paradoxically, our findings highlight the precarious future of this fishery because the habitat features favored by R. nilotica are threatened by climate change and other broadscale stressors.
Data AVailability Statement
The datasets generated for this study are available on request to the corresponding author.
Author Contributions
KS and SP conceived the research and conducted the fieldwork. All authors analyzed the data and wrote the manuscript.
Funding
This research was funded by the Australian Centre for International Agricultural Research, project FIS/2016/128. Southern Cross University provided a postgraduate grant to further support research costs. KS received financial support from the Ralph Barclay Braun Memorial Scholarship.
Conflict of Interest
The authors declare that the research was conducted in the absence of any commercial or financial relationships that could be construed as a potential conflict of interest.
Acknowledgments
Thanks to Dr. Robert Clarke of Plymouth Marine Laboratory for assistance with statistical analyses in PRIMER v7 and the Ministry of Fisheries and Agriculture Samoa for providing boating and survey equipment. Special thanks to Justin Aiafi, Moso Lesa, and Atapana Tony, of the Inshore Fisheries Division, Samoa, for their support in the field.
Supplementary Material
The Supplementary Material for this article can be found online at: https://www.frontiersin.org/articles/10.3389/fmars.2020.00223/full#supplementary-material
References
Alvarez-Filip, L., Dulvy, N. K., Gill, J. A., Cote, I. M., and Watkinson, A. R. (2009). Flattening of caribbean coral reefs: region-wide declines in architectural complexity. P. Roy. Soc. B Biol. Sci. 276, 3019–3025. doi: 10.1098/rspb.2009.0339
Anthony, K. R. N., Maynard, J. A., Diaz-Pulido, G., Mumby, P. J., Marshall, P. A., Cao, L., et al. (2011). Ocean acidification and warming will lower coral reef resilience. Global Change Biol. 17, 1798–1808. doi: 10.1111/j.1365-2486.2010.02364.x
Bell, J. D., Ganachaud, A., Gehrke, P. C., Griffiths, S. P., Hobday, A. J., Hoegh-Guldberg, O., et al. (2013). Mixed responses of tropical Pacific fisheries and aquaculture to climate change. Nat. Clim. Change 3, 591–599. doi: 10.1038/nclimate1838
Bell, J. D., Rothlisberg, P. C., Munro, J. L., Loneragan, N. R., Nash, W. J., Ward, R. D., et al. (2005). Restocking and stock enhancement of marine invertebrate fisheries. Adv. Mar. Ecol. 49, xi–374.
Bender-Champ, D., Diaz-Pulido, G., and Dove, S. (2017). Effects of elevated nutrients and CO2 emission scenarios on three coral reef macroalgae. Harmful Algae 65, 40–51. doi: 10.1016/j.hal.2017.04.004
Bennice, C., Rayburn, A., Brooks, W. R., and Hanlon, R. (2018). Fine-scale habitat partitioning facilitates sympatry between two octopus species in a shallow Florida lagoon. Mar. Ecol. Prog. Ser 609, 151–161. doi: 10.3354/meps12845
Berthe, C., Chancerelle, Y., Lecchini, D., and Hedouin, L. (2016). First report of a dramatic loss of living coral on the north coast of Western Samoa. Life and Environment 66, 155–157.
BOM and CSIRO (2011). Climate change in the Pacific: scientific assessment and new research. Volume 2: Country Reports. Melbourne, VIC: Australian Bureau of Meteorology.
Bozec, Y., Alvarez-Filip, L., and Mumby, P. J. (2015). The dynamics of architectural complexity on coral reefs under climate change. Global Change Biol. 21, 223–235. doi: 10.1111/gcb.12698
Castell, L. L. (1997). Population studies of juvenile Trochus niloticus on a reef flat on the north-eastern Queensland coast. Australia. Mar. Freshwater Res. 48, 211–217. doi: 10.1071/MF96002
Ceccarelli, D. M., Berger, M., Kospartov, M. C., Richards, Z. T., and Birrell, C. L. (2011). Population trends of remote invertebrate resources in a marine reserve: trochus and holothurians at Ashmore Reef. Pac. Conserv. Biol. 17, 123–140. doi: 10.1071/PC110132
Clarke, K. R., and Gorley, R. N. (2015). PRIMER v7: User Manual/Tutorial. United Kingdom: PRIMER-E Ltd.
Colquhoun, J. R. (2001). Habitat preferences of juvenile trochus in Western Australia: implications for stock enhancement and assessment. SPC Trochus Inf. Bull. 7, 14–20.
Crowe, T. P., Lee, C. L., McGuinness, K. A., Amos, M. J., Dangeubun, J., Dwiono, S. A. P., et al. (2002). Experimental evaluation of the use of hatchery-reared juveniles to enhance stocks of the topshell Trochus niloticus in Australia. Indonesia and Vanuatu. Aquaculture 206, 175–197. doi: 10.1016/S0044-8486(01)00676-7
De’ath, G. (2007). Boosted trees for ecological modelling and prediction. Ecology 88, 243–251. doi: 10.1890/0012-9658(2007)88
Diaz-Pulido, G., McCook, L. J., Dove, S., Berkelmans, R., Roff, G., Kline, I., et al. (2009). Doom and boom on a resiliant reeef: Climate change, algal overgrowth and coral recovery. PLoS One 4:e5239. doi: 10.1371/journal.pone.0005239
Dolorosa, R. G., Songco, A. M., Calderon, V., Magbanua, R., and Matillano, J. A. (2010). Population structure and abundance of Trochus niloticus in Tubbataha Reefs National Park, Palawan, Philippines with notes on poaching effects. SPC Trochus Inf. Bull. 15, 17–23.
Dumas, P., Ham, J., Kaku, R., William, A., Kaltavara, J., Gereva, S., et al. (2017). Tectus (trochus) niloticus search for suitable habitats can cause equivocal benefits of protection in village-based marine reserves. PLoS One 12:e0176922. doi: 10.1371/journal.pone.0176922
Dumas, P., Jimenez, H., Reignon, C., Wantiez, L., and Adjeroud, M. (2013). Small-scale habitat structure modulates the effects of no-take marine reserves for coral reef macroinvertebrates. PloS One 8:e58998. doi: 10.1371/journal.pone.0058998
Elith, J., Leathwick, J. R., and Hastie, T. (2008). A working guide to boosted regression trees. J. Anim. Ecol. 77, 802–813. doi: 10.1111/j.1365-2656.2008.01390.x
Fabricius, K. E., De’ath, G., Noonan, S., and Uthicke, S. (2013). Ecological effects of ocean acidification and habitat complexity on reef-associated macroinvertebrate communities. P. Roy. Soc. B-Biol. Sci 281, 20132479. doi: 10.1098/rspb.2013.2479
Fao, B. (1992). Information on trochus fisheries in the South Pacific. SPC Trochus Inf. Bull. 1, 12–15.
Francis, F. T., Filbee-Dexter, K., Yan, H. F., and Côté, I. M. (2019). Invertebrate herbivores: Overlooked allies in the recovery of degraded coral reefs? Glob. Ecol. Conserv 17, e00593. doi: 10.1016/j.gecco.2019.e00593
Gillett, R., and Tauati, I. (2018). Fisheries of the Pacific Islands. Regional and National Information. Apia, Samoa: FAO.
Gosliner, T. M., Behrens, D. W., and Williams, G. C. (1996). Coral Reef Animals of the Indo-Pacific. Animal Life From Africa to Hawaii Exclusive of the Vertebrates. California: Sea Challengers.
Graham, N. A. J., Jennings, S., MacNeil, M. A., Mouillot, D., and Wilson, S. K. (2015). Predicting climate-driven regime shifts versus rebound potential in coral reefs. Nature 518, 94–97. doi: 10.1038/nature14140
Graham, N. A. J., and Nash, K. L. (2013). The importance of structural complexity in coral reef ecosystems. Coral Reefs 32, 315–326. doi: 10.1007/s00338-012-0984-y
Graham, N. A. J., Wilson, S. K., Jennings, S., Polunin, N. V. C., Bijoux, J. P., and Robinson, J. (2006). Dynamic fragility of oceanic coral reef ecosystems. P. Natl. Acad. Sci. USA 103, 8425–8429. doi: 10.1073/pnas.0600693103
Ham, Y. (2018). El Niño events set to intensify. Nature 564, 192–193. doi: 10.1038/d41586-018-07638-w
Heslinga, G. A. (1981). “Growth and maturity of Trochus niloticus in the laboratory,” in Proceedings of the fourth international coral reef symposium, (Manila).
Hoegh-Guldberg, O., Mumby, P. J., Hooten, A. J., Steneck, R. S., Greenfield, P., Gomez, E., et al. (2007). Coral reefs under rapid climate change and ocean acidification. Science 318, 1737–1742. doi: 10.1126/science.1152509
Hughes, T. P., Kerry, J. T., Baird, A. H., Connolly, S. R., Dietzel, A., Eakin, C. M., et al. (2018). Global warming transforms coral reef assemblages. Nature 556, 492–526. doi: 10.1038/s41586-018-0041-2
Jankowski, M. W., Jones, G. P., and Graham, N. A. J. (2015). Depth gradients in diversity, distribution and habitat specialisation in coral reef fishes: Implications for the depth-refuge hypothesis. Mar. Ecol. Prog. Ser 540, 203–215. doi: 10.3354/meps11523
Kohler, K. E., and Gill, S. M. (2006). Coral Point Count with Excel extensions (CPCe): A visual basic program for the determination of coral and substrate coverage using random point count methodology. Comput. Geosci. 32, 1259–1269. doi: 10.1016/j.cageo.2005.11.009
Komyakova, V., Munday, P. L., and Jones, G. P. (2013). Relative importance of coral cover, habitat complexity and diversity in determining the structure of reef fish communities. PLoS ONE 8:e83178. doi: 10.1371/journal.pone.0083178
Lee, C., Purcell, S. W., and Maguire, G. (2001). Farming Trochus. Aquaculture WA, No. 8. Perth: Department of Fisheries Western Australia.
Lober, M., Solofa, A., and Taua, A. (2003). ACIAR Regional Trochus Project (FIS/2001/085): Samoa node. SPC Trochus Inf. Bull. 10, 4–6.
Long, B. G., Poiner, I. R., and Harris, A. N. M. (1993). Method of estimating the standing stock of Trochus niloticus incorporating Landsat satellite data, with application to the trochus resources of the Bourke Isles. Torres Strait, Australia. Mar. Biol. 115, 587–593. doi: 10.1007/BF00349366
Luckhurst, B. E., and Luckhurst, K. (1978). Analysis of the Influence of Substrate Variables on Coral Reef Fish Communities. Mar. Biol. 49, 317–323. doi: 10.1007/BF00455026
MAF (1997). Trochus Resource of Western Samoa. Workshop on Trochus Resource Assessment, Management and Development. Report and Selected Papers. Noumea, New Caledonia: SPC.
McCormick, M. I. (1994). Comparison of field methods for measuring surface topography and their associations with a tropical reef fish assemblage. Mar. Ecol. Prog. Ser. 112, 87–96. doi: 10.3354/meps112087
MNRE (2010). State of the Environment Report 2006. Apia, Samoa: Ministry of Natural Resources and Environment: Government of Samoa.
MNRE (2013). Samoa’s State of the Environment (SOE) Report 2013. Apia, Samoa: Ministry of Natural Resources and Environment, Government of Samoa.
Munday, P. L., Jones, G. P., Pratchett, M. S., and Williams, A. J. (2008). Climate change and the future for coral reef fishes. Fish Fish. 9, 261–285. doi: 10.1111/j.1467-2979.2008.00281.x
Nash, W. J. (1993). “Trochus,” in Nearshore Marine Resources of the South Pacific, eds A. Wright and L. Hill, (Suva, Fiji: Institute of Pacific Studies), 451–495.
Pakoa, K., Friedman, K., and Damlamian, H. (2010). The status of trochus (Trochus niloticus) in Tongatapu Lagoon, Kingdom of Tonga. SPC Trochus Inf. Bull. 15, 3–16.
Pratchett, M. S., Munday, P. L., Wilson, S. K., Graham, N. A. J., Cinner, J. E., Bellwood, D. R., et al. (2008). Effects of climate-induced coral bleaching on coral-reef fishes – ecological and economic consequences. Oceanog. Mar. Biol 46, 251–296. doi: 10.1201/9781420065756.ch6
Przeslawski, R., Ahyong, S., Byrne, M., Worheides, G., and Hutchings, P. (2008). Beyond corals and fish: the effects of climate change on noncoral benthic invertebrates of tropical reefs. Global Change Biol. 14, 2773–2795. doi: 10.1111/j.1365-2486.2008.01693.x
Purcell, S. W., and Cheng, Y. W. (2010). Experimental restocking and seasonal visibility of a coral reef gastropod assessed by temporal modelling. Aquat. Biol. 9, 227–238. doi: 10.3354/ab00253
Purcell, S. W., Clarke, K. R., Rushworth, K., and Dalton, S. J. (2014). Defining critical habitats of threatened and endemic reef fishes with a multivariate approach. Conservation Biology 28, 1688–1698. doi: 10.1111/cobi.12343
Purcell, S. W., Gossuin, H., and Agudo, N. S. (2009). Status and management of the sea cucumber fishery of La Grande Terre, New Caledonia. Penang: The WorldFish Center.
Rees, M., Colquhoun, J., Smith, L., and Hayward, A. (2003). Surveys of trochus, holothuria, giant clams and the coral communities at Ashmore Reef, Cartier Reef and Mermaid Reef, Northwestern Australia: 2003. Townsville, Australia: The Australian Institute of Marine Science.
Roessig, J. M., Woodley, C. M., Cech, J. J. Jr., and Hansen, L. J. (2004). Effects of global climate change on marine and estuarine fishes and fisheries. Rev. Fish Biol. Fisher. 14, 251–275. doi: 10.1007/s11160-004-6749-0
Samuelu, J. A., and Sapatu, M. (2009). “Status of Coral Reefs in Samoa 2007,” in South-West Pacific Status of Coral Reefs Report, ed. C. Whippy-Morris, (Noumea, New Caledonia: Coral Reef Initiatives for the Pacific), 81–114.
Smith, B. D. (1987). Growth rate, distribution and abundance of the introduced topshell Trochus niloticus linnaeus on Guam. Mariana Islands. B. Mar. Sci. 41, 466–474.
Soekendarsi, E. (2018). The habitat of yellow mouth turban Turbo chrysostomus, Linnaeus, 1758. J. Phys. Conf. Ser 979, 012040. doi: 10.1088/1742-6596/979/1/012040
Spalding, M. D., Ravilious, C., and Green, E. P. (2001). World Atlas of Coral Reefs. Berkeley, USA: University of California Press.
Tiitii, U., and Aiafi, J. (2016). Assessment of Samoa’s Trochus (Tectus niloticus) Fishery: History, Status and Recommendations for Management. Noumea, New Caledonia: SPC.
Vunisea, A., Friedman, K., Ribanataake, A., Kronen, M., Pinca, S., Chapman, L., et al. (2008). Samoa Country Report: Profiles and Results From Survey Work at Manono-Uta, Salelavalu, Vailoa and Vaisala. Noumea, New Caledonia: SPC.
WoRMS. (2020). Rochia nilotica (Linnaeus, 1767). Available online at: World Register of Marine Species at: http://www.marinespecies.org/aphia.php?p=taxdetails&id=1251282 (accessed February 23, 2020).
Wright, A., and Hill, L. (1993). Nearshore marine resources of the South Pacific. Information for fisheries development and management. Suva: Institute of Pacific Studies.
Ziegler, M., Quere, G., Ghiglione, J., Iwankow, G., Barbe, V., Boissin, E., et al. (2018). Status of coral reefs of Upolu (Independant State of Samoa) in the South West Pacific and recommendations to promote resilience and recovery of coral ecosystems. Mar. Pollut. Bull. 129, 392–398. doi: 10.1016/j.marpolbul.2018.02.044
Keywords: habitat association, invertebrate, fishery, climate change, coral reef, species translocation, Rochia nilotica
Citation: Seinor KM, Smith SDA, Logan M and Purcell SW (2020) Biophysical Habitat Features Explain Colonization and Size Distribution of Introduced Trochus (Gastropoda). Front. Mar. Sci. 7:223. doi: 10.3389/fmars.2020.00223
Received: 21 October 2019; Accepted: 23 March 2020;
Published: 15 April 2020.
Edited by:
Susana Carvalho, King Abdullah University of Science and Technology, Saudi ArabiaReviewed by:
Paulo Vasconcelos, Portuguese Institute for the Ocean and Atmosphere (IPMA), PortugalLuís Felipe Skinner, Rio de Janeiro State University, Brazil
Copyright © 2020 Seinor, Smith, Logan and Purcell. This is an open-access article distributed under the terms of the Creative Commons Attribution License (CC BY). The use, distribution or reproduction in other forums is permitted, provided the original author(s) and the copyright owner(s) are credited and that the original publication in this journal is cited, in accordance with accepted academic practice. No use, distribution or reproduction is permitted which does not comply with these terms.
*Correspondence: Kate M. Seinor, kate.seinor@outlook.com; Steven W. Purcell, steven.purcell@scu.edu.au