- 1Department of Plant Sciences, Faculty of Biosciences, Norwegian University of Life Sciences, Ås, Norway
- 2Department of Animal and Aquacultural Sciences, Faculty of Biosciences, Norwegian University of Life Sciences, Ås, Norway
Cultivated kelps and other macroalgae have great potential in future provision of food, feed, bioenergy, fertilizer, and raw material for a range of chemical products including pharmaceuticals, food and feed additives, and cosmetics. Only a few species are currently cultivated, almost exclusively in Asia. There is a range of species that could be utilized in different parts of the world, providing that protocols for reproduction, propagation, and cultivation are developed. Domestication of species involves selection of traits that are desirable in cultivation and in the utilization of the harvested biomass. Genetic improvement of cultivated species through recombination of alleles and selection (breeding) has ensured high productivity and product quality in both agri- and aquaculture and will likely do so for macroalgae cultivation and use as well. According to the published literature, genetic improvement of kelps in Asia has so far largely relied on utilization of heterosis expressed in certain combinations of parental material, sometimes species hybrids. Here, we explore and evaluate the various methods that could be used in kelp breeding and propose an initial simple and low-cost breeding strategy based on recurrent mixed hybridization and phenotypic selection within local populations. We also discuss the genetic diversity in wild populations, and how this diversity can be protected against genetic pollution, either by breeding and cultivating local populations, or by developing cultivars that are not able to establish in, or hybridize with, wild populations.
Introduction
Cultivated marine macroalgae can become an important source of human food in the future (Duarte et al., 2009). Unlike terrestrial crops, macroalgae do not compete for arable land, fertilizer and freshwater resources (Charrier et al., 2017). They also provide a number of ecosystem services such as carbon sequestration and nutrient absorption (Buschmann et al., 2017; Duarte et al., 2017; Kim et al., 2017). Macroalgae are utilized as healthy food and feed (Fleurence et al., 2012; Øverland et al., 2018), and as a raw material for pharmaceutical, cosmetic, bioenergy, and chemical industries (Holdt and Kraan, 2011; Hafting et al., 2015; Milledge and Harvey, 2016; Fernand et al., 2017).
The global annual production of macroalgae is steadily increasing and amounted to 31.2 million tons (fresh weight) in 2016 (FAO, 2018). Of this, only 3.5% was harvested from natural populations, while the remaining 96.5% was produced in aquaculture, making up 27% of the worlds’ total aquaculture production. Almost all of this took place in China, Indonesia, and other Asian countries (47.9, 38.7, and 12.8% of the global production in 2016, respectively), mainly for human food and food additives. The global aquacultural production of macroalgae has more than doubled over the past 20 years (FAO, 2018), and the global potential has been suggested to be 1000–100,000 million tons (Lehahn et al., 2016), but the dominant practice outside Asia is still to harvest natural stocks (Buschmann et al., 2017).
The European supply of macroalgal biomass was 230,000 tons (fresh weight) in 2015. Of this, 65% originated from Norway, while France, Ireland, Iceland, and the Russian Federation accounted for 33% (Camia et al., 2018). In Norway, brown algae were traditionally used for feed and as an agricultural fertilizer, but currently, alginate production based on harvested biomass is by far the most important sector of the macroalgae industry (Meland and Rebours, 2012; Skjermo et al., 2014; Stévant et al., 2017). The harvest of non-cultivated brown macroalgae, mainly Laminaria hyperborea, but also Ascophyllum nodosum, amounted to 169,410 tonnes in 2018 (Stévant et al., 2017; Directorate of Fisheries, 2019). Olafsen et al. (2012) suggested that, through aquaculture, the annual production of macroalgae could be increased up to 20 million tons by 2050. Since 2014 there has been a burst in the number of granted permits for cultivation, the main species being Saccharina latissima and to some extent Alaria esculenta (Stévant et al., 2017; Directorate of Fisheries, 2019). The cultivation potential of S. latissima along the Norwegian coast was recently estimated to be 150–200 tons per hectare and year (Broch et al., 2019).
Algae is a polyphyletic group of diverse organisms (Keeling et al., 2005), which can be divided into micro- and macroalgae, according to size. At present, there are approximately 200 species of macroalgae of commercial use worldwide, of which about 10 genera are intensively cultivated, such as members of the brown algae, e.g., Saccharina and Undaria, red algae, e.g., Porphyra, Pyropia, Eucheuma/Kappaphycus, and Gracilaria, and green algae, e.g., Monostroma and Enteromorpha/Ulva (Lüning and Pang, 2003; Kim et al., 2017). “Kelp” is a common name for large brown algae. The use of this term is often restricted to genera in the order Laminariales (e.g., Alaria, Ecklonia, Laminaria, Macrocystis, Nereocystis, Saccharina, Undaria), although some species in the order Fucales are regarded as kelps by the general public, e.g., in the genera Durvillaea and Sargassum (Fraser, 2012). “Nori” includes several red algae used for human food, especially Porphyra sensu lato, a taxonomically complex group which includes the genera Porphyra and Pyropia (Lim et al., 2017). Other important groups of red algae are known by the main product obtained from their biomass, as goes for the main producers of agar, the so-called “agarophytes” (which include the genera Agarophyton, Gracilaria, Gracilariopsis, and Gelidium), and the main carrageenan producers or “carrageenophytes” (which include the genera Kappaphycus and Eucheuma) (Santelices and Doty, 1989; Hayashi et al., 2017).
Macroalgae are important primary producers in marine ecosystems, providing capture and cycling of energy, carbon, and nutrients. In addition, these species create underwater forests that are habitats for numerous fish, invertebrates, and other species (Smale et al., 2013; Teagle et al., 2017; Filbee-Dexter et al., 2019). For these reasons, overharvesting natural populations has major ecological implications. Further exploitation of macroalgal resources must rely on cultivation, an industry which provides ecosystem services but also poses some environmental risks (Campbell et al., 2019). The productivity of marine aquaculture has the potential to increase dramatically by the use of genetically improved populations, as exemplified by the success of salmon breeding (Gjedrem et al., 2012). The use of genetically superior material of macroalgal species has been key to the success of cultivation in Asia, where cultivars based on a limited number of parental genotypes account for a large proportion of the production (Robinson et al., 2013; Charrier et al., 2017). The corresponding limited genetic diversity provides homogenous crops but can also increase the vulnerability to disease outbreaks and environmental changes (Valero et al., 2017). An issue that has received attention, particularly in the last decade, is the potential for “genetic pollution” of wild populations that can occur if farmed individuals from aquaculture escape and hybridize with individuals in wild populations. Such introgression has been documented for salmon and for species of wrasse (cleaner fish) in Norway (Glover et al., 2013; Jansson et al., 2017; Faust et al., 2018). Consequences of introgressions are largely unknown, but may involve altered ecosystem function of the species (and potential effects of this on other species), or erosion of the genetic diversity in natural populations (and ultimately of the natural gene bank that could be utilized in future breeding).
Hwang et al. (2019) recently described the status of macroalgae aquaculture, range of cultivated species, and the progress that has been made through breeding in Korea, Japan, and China. Robinson et al. (2013) reviewed the status of genetic improvement of macroalgae in general and considered and compared three general options for future genetic improvement: Selective breeding based on recurrent cross-hybridizations and selection, line breeding based on recurrent self-hybridization and selection, and genetic modification. Our objectives are to provide an updated review of the scientific literature on genetic improvement of kelp cultivars for commercial production, and to compare and evaluate different breeding strategies in light of the prospects for increased production and utilization, as well as the need to protect the wild kelp populations. We first describe life cycles and species hybridization among kelps and review the literature describing breeding methods that have been employed in Asia so far. We then discuss the potential for genetic pollution of wild kelp populations and how this could be avoided, before we evaluate different options for breeding of kelp species in the future.
Kelp Life Cycles
The life cycle, including both sexual and asexual reproduction, and our ability to control it, is central for cultivation of any organism. It is also central in any effort to improve the organism so that it performs optimally from a human point of view with regard to yield, quality, etc. Macroalgae life cycles can be rather complex and have been described in detail along with environmental and genetic control mechanisms and ecological or evolutionary perspectives in several previous reviews (Brawley and Johnson, 1992; Schiel and Foster, 2006; Liu et al., 2017; Heesch et al., 2019).
Species in the order Laminariales have a biphasic life cycle with an obligate cycling between separate and free-living diploid sporophytes and haploid gametophytes (Figure 1A). Adult sporophytes are monoecious, that is, each individual produces both male and female haploid zoospores. The zoospores develop into free-living, microscopic gametophytes that produce haploid gametes (eggs and sperm). Eggs are extruded from oogonia of female gametophytes, but remain attached, and release pheromones that attract motile sperms from male gametophytes for fertilization. The zygote grows from the female gametophyte, attaches to a substrate, and develops to become a multicellular sporophyte.
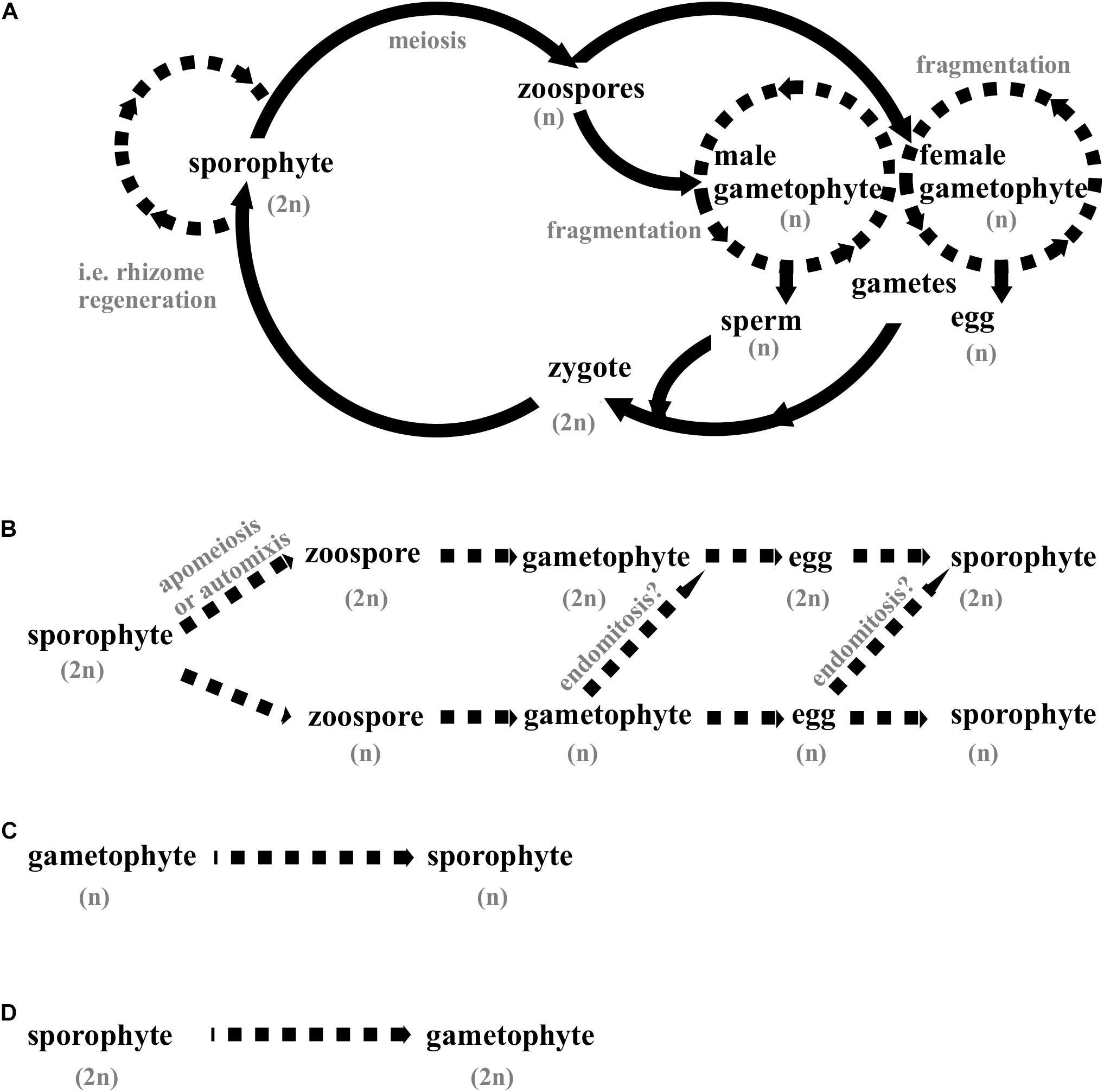
Figure 1. Life cycles are of Laminarialean kelps. The possibilities for asexual reproduction are displayed with discontinued lines. n; haploid stage, 2n; diploid stage. (A) The diplohaplontic life cycle of species in the order Laminariales involves two independent and free-living states, which may look very different from each other: Macroscopic sporophytes that produce zoospores, and microscopic male and female gametophytes that produce gametes. Self-fertilization is common. Some species have vegetative reproduction of the sporophyte through rhizome expansion and outgrowth of additional blades. Under certain conditions, gametophytes can grow continuously and be vegetatively propagated through fragmentation. (B) Parthenogenesis, development of sporophytes from unfertilized female gametes. Diploidy can be generated through apomeiosis (replacement of meiosis with mitosis), automixis (two of the four nuclear products of meiosis fuse), or endomitosis (endoreduplication, spontane chromosome doubling). (C) Apogamy, development of sporophytes from somatic cells of gametophytes. (D) Apospory, development of gametophytes from somatic cells of sporophytes.
Laminarialean kelp species have a variety of asexual reproduction mechanisms. Some species can propagate vegetatively through the expansion of the rhizome (holdfast, base) and outgrowth of additional blades (Demes and Graham, 2011; Murúa et al., 2017), or vegetative growth and fragmentation of gametophytes (Destombes and Oppliger, 2011, see also section “Manipulation of Life Cycles”). These processes are regulated by environmental conditions. Several species can produce sporophytes through parthenogenesis, where sporophytes are produced directly from non-fertilized female gametes (Nakahara and Nakamura, 1973; Fang, 1984; Wu and Lin, 1987; Lewis et al., 1993; Ar Gall et al., 1996; Liptack and Druehl, 2000; Druehl et al., 2005; Oppliger et al., 2007; 2014; Westermeier et al., 2007; Shan et al., 2013; Müller et al., 2019). Parthenogenic sporophytes can either be haploid or diploid. Diploidy can be generated through apomeiosis (replacement of meiosis with mitosis) or automixis (where two of the four nuclear products of meiosis fuse) during zoospore formation, or through endomitosis (endoreduplication, spontane chromosome doubling) (Oppliger et al., 2014; Müller et al., 2016) (Figure 1B). Both haploid and diploid parthenogenic sporophytes can have normal morphology and produce spores that give rise to fertile female gametophytes, creating a female life cycle. Other peculiar modes of reproduction that can occur in Laminariales include apogamy, where a haploid sporophyte can emerge directly from a somatic cell of a gametophyte (Nakahara and Nakamura, 1973; Fang, 1984; Wu and Lin, 1987; Müller et al., 2019) (Figure 1C), and apospory, where a diploid gametophyte can develop from vegetative cells of a diploid sporophyte without any meiosis or spore formation (Nakahara and Nakamura, 1973; Lewis et al., 1993) (Figure 1D). Self-fertilization appears to be possible in most studied species of Laminariales (Wu and Lin, 1987; Brawley and Johnson, 1992; Kraan et al., 2000; Camus et al., 2018). Some kelp species are annual or biennial, but most are perennial (Schiel and Foster, 2006).
Species Hybridization Among Kelps
The distinction between related kelp species is not always clear, and in some cases, what is defined as two or more species may actually be locally adapted and genetically different populations of the same species, or simply the result of phenotypic plasticity (Kilar et al., 1992; Demes et al., 2009; Fraser et al., 2009). Species hybrids in the Laminariales have been observed in nature, and under controlled conditions, it is possible to create viable offspring from a number of species hybridizations (Chapman, 1974; Lüning et al., 1978; Sangonsuga and Neushul, 1978; Bolton et al., 1983; Lewis et al., 1986; tom Dieck, 1992; tom Dieck and de Oliveira, 1993; Liptack and Druehl, 2000; Druehl et al., 2005; Westermeier et al., 2007; Martins et al., 2019; Müller et al., 2019). As described further in Section “Hybrid Breeding and Selection Among Crosses (HB/SC),” several kelp cultivars bred in China and Korea are species hybrids. Interestingly, sporophyte morphology of some species hybrids is similar to the female parent, indicating that genetic control of morphology is determined by genes that are linked to sex-determining genes, or that are present in chloroplast or mitochondrial DNA, maternally inherited with the cytoplasm (Patwary and van der Meer, 1992; Druehl et al., 2005). In some cases, the direction of the cross also affects the performance in aquaculture, e.g., in the cross between Undaria pinnatifida and Undaria peterseniana, where vigorous F1 sporophytes expressing strong heterosis in biomass are only produced when U. pinnatifida is used as the female (Hwang et al., 2012, 2014).
Development of Kelp Cultivars in the Past
In China, kelp cultivars have been developed since the 1950s (Fang et al., 1962a, b, Fang and Jiang, 1963; Cheng, 1969; van der Meer, 1983; Wu and Lin, 1987). Several Laminarialean kelp cultivars with good characteristics for aquaculture have been produced in China and South Korea (Table 1). So far, selection has focused mostly on biomass and size, although traits like high chemical composition (IOIMF, 1976; Zhang et al., 2007), stress tolerance (Li et al., 2008a; Zhang et al., 2011; Liu et al., 2014), and late maturity (Liu et al., 2014; Hwang et al., 2017) have also been targeted.
Manipulation of Life Cycles
Initially, propagation of sporophytes for transplantation to different installations in the sea was based on zoospore collection from a large number of parental plants (Cheng, 1969; Zhang et al., 2011; Liu et al., 2014). This is still the common practice in many parts of the world, but in East-Asia techniques to vegetatively propagate gametophyte filaments has now been employed for several decades in order to select, maintain, and propagate superior material (Valero et al., 2017; Hwang et al., 2019). Gametophyte filaments of a number of species, e.g., S. latissima, Saccharina japonica, Laminaria digitata, Laminaria hyperborea, Macrocystis pyrifera, and U. pinnatifida, can grow vegetatively in culture at low photon flux density of around 10–30 μmol photons m–2 s–1. Usually only red light, which is photosynthetically active, is used. To keep the gametophytes vegetative, it is important that they are not exposed to violet/blue light (380–500 nm) as this induces production of male and female gametes (Lüning and Dring, 1975; Cosson and Gayral, 1979; Xu et al., 2005). Gametogenesis is also regulated by temperature, the optimum depending on the origin of the genotype (Lüning, 1980), and on nutrient conditions (Ratcliff et al., 2017). Once a culture is established, the gametophytes can be maintained for long periods and multiplied by periodical mechanical disruption of the filaments and renewal of the growth medium. The introduction of vegetatively propagated gametophyte cultures in East-Asia made it possible to screen the performance of offspring and then go back to desired parental gametophyte cultures in order to initiate large-scale propagation of material for cultivation (Pang et al., 1997; Li et al., 1999). This could be repeated using the same stock of gametophytes every year allowing for the annual production of sporophytes of superior F1 hybrid cultivars. Today, protocols for induction of zoospore formation in sporophyte blades, spore collection, vegetative gametophyte culture, and induction of gametogenesis have been developed for several species (e.g., Pang and Lüning, 2004; Wu et al., 2004; Westermeier et al., 2006; Zhang et al., 2008; Xu et al., 2009; Forbord et al., 2012, 2018; Ratcliff et al., 2017; Bartsch, 2018). A method of clonal propagation through regeneration of sporophytes from a diploid cell-filament suspension of L. digitata has also been described (Asensi et al., 2001).
Hybrid Breeding and Selection Among Crosses (HB/SC)
According to available publications in English, mainly from the last decade, development of most Chinese Saccharina cultivars and some Chinese and Korean Undaria cultivars were based on hybridization of contrasting gametophyte cultures, utilizing a considerable amount of heterosis (hybrid vigor) (Figure 2). Hybridizations were made between material of the same species, sometimes originating from different geographical regions (Li et al., 2016a, b; Zhao et al., 2016), sometimes not (Niwa and Kobiyama, 2019), or between different species of the same genus (Li et al., 2007, 2008a; Zhang et al., 2007, 2011, 2016; Hwang et al., 2012, 2014). Gametophyte culture combinations with high heterosis were selected as parents, and in many cases, the first generation was used directly as a commercial hybrid cultivar (Li et al., 2007, 2016b; Hwang et al., 2012, 2014; Zhao et al., 2016). Most publications are not clear on the number of crosses tested and on whether the gametophyte cultures were resulting from one individual gametophyte, many gametophytes from the same individual sporophyte, or from several sporophytes. Those studies that compared selected Saccharina F1 hybrid populations with the two parents or parental populations reported strong heterosis effects (deviation from the means of the parents) on blade length (17–53%), width (7–71%), thickness (2–32%), fresh weight (45–150%), and dry weight (36–67%), as well as yield (biomass per area, 57–69%) (Li et al., 2007, 2008a, 2016a, 2016b, Hwang et al., 2012). F1 hybrid cultivars were generally documented to have a high level of homogeneity, which is desired by the growers.
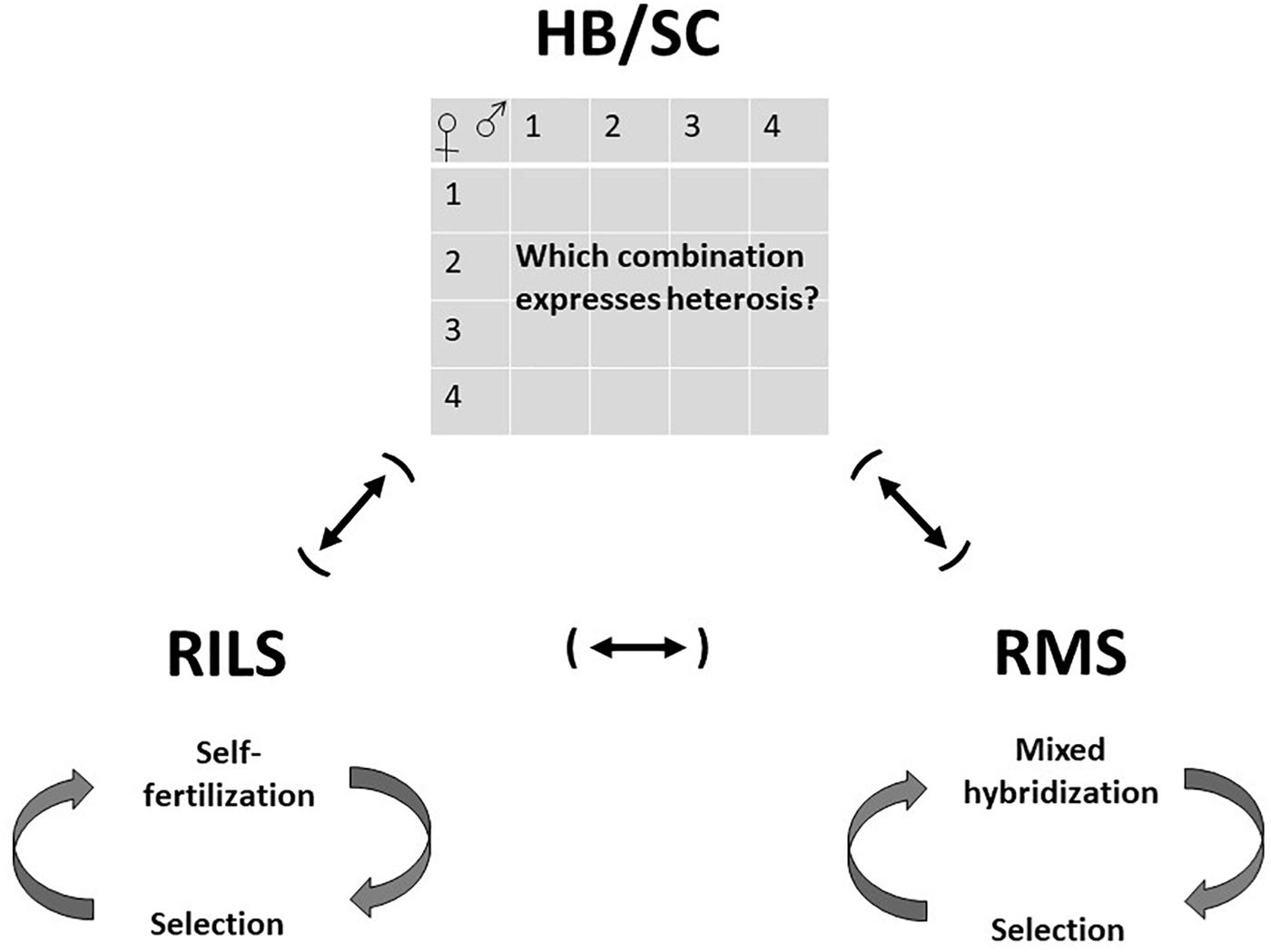
Figure 2. Simplified illustration of breeding schemes employed in cultivar development of Laminarialean kelp species. HB/SC; hybrid breeding/selected crosses. The cultivar is the F1 offspring of a planned cross between divergent parents, resulting in considerable heterosis. Hybrids can be the result of interspecific hybridization or crosses within species (e.g., between individuals with different geographic origin or complementary traits). Selection of individual parents is often made on the basis of heterosis, that is the performance of F1 offspring from pair crosses between single gametophyte clones. RILS; recurrent inbred line selection. Desirable individuals are self-fertilized, the best offspring selected, and the cycle repeated several times. RMS; recurrent mixed hybridization and mass selection. Desirable individuals are selected from a population (without progeny testing) and subjected to mixed hybridization, and the cycle repeated several times. This type of selection exploits additive genetic variance and a large part of the genetic diversity in the population can be maintained. Both RILS and RMS can be combined with HB/SC in any order.
Recurrent Inbred Line Selection (RILS)
Several cultivars have been developed by selection and self-hybridization for 3–15 generations (IOIMF, 1976; Zhang et al., 2007, 2016; Li et al., 2016a) (Figure 2). In some cases, the starting point for inbreeding was a few superior sporophytes, in other cases, it was the offspring of a limited number of pair crosses with high heterosis. Zhang et al. (2007) found maximum values for the selected traits in F3–F4, with a breeding progress of 19% (blade fresh weight) and 44% (blade dry weight) relative to the F1 hybrid. The breeding progress during inbreeding reported by Li et al. (2016a) was limited.
Recurrent Mixed Hybridization and Mass Selection (RMS)
An alternative to the line breeding described above has been to select the offspring of a limited number of gametophyte pair crosses with high heterosis and then subject these to recurrent mixed hybridization and selection for desirable traits (Zhang et al., 2011; Shan et al., 2016) (Figure 2). This type of recurrent selection was also used to introgress desirable traits from S. latissima into S. japonica (Zhang et al., 2018). This method has been reported to result in 30–40% higher individual fresh weights after four to six generations of selection (Zhang et al., 2011, 2018).
The restricted genetic variation resulting from all the breeding schemes that we have described so far, all using a low number of parents, limits adaptability and further genetic improvement. In order to overcome these disadvantages, Liu et al. (2014) developed a cultivar based only on additive gene effects and not on heterosis. Hundred and fifty individuals with large size and other desired morphological characteristics were collected at many locations. Mixed hybridization followed by selection for blade length and width over five generations resulted in an 88% increase in blade length, 21% increase in blade width, and 71% increase in individual fresh weight relative to the average of the parents.
Invasiveness, Introduction of Non-Native Genotypes and Gene Flow Into Local Populations
Some marine macroalgae and other species used in aquaculture can be invasive and have strong ecosystem impact when spread to new geographical areas where they were not formerly present (Naylor et al., 2001; Williams and Smith, 2007; Davidson et al., 2015; Thomsen et al., 2016). A well-known example of an invasive kelp species is U. pinnatifida, which originates from northeast Asia, where it is also cultivated. This species has been both intentionally and non-intentionally spread and is now invasive in a large number of coastal areas across the globe (Fletcher and Farrell, 1999; Casas et al., 2004; Pickering et al., 2007; Russell et al., 2008; Miller and Engle, 2009; Thompson and Schiel, 2012; Dellatorre et al., 2014; Minchin and Nunn, 2014; South et al., 2017).
A more subtle form of invasion can occur when new genetic variation is introduced to a natural population through the spread of competitive genotypes and/or through hybridization and gene flow. This has the potential to either increase or reduce the overall genetic variation within a species, and in the latter case, degrade an important resource for future development of cultivars. Permanent incorporation of genes from one population into another (introgression) is widely documented in terrestrial agriculture, where alleles from cultivated crops have become incorporated in populations of wild relatives that the crop species can hybridize with (Ellstrand et al., 2013). There is considerable concern regarding genetic pollution of wild populations from the aquaculture industry (Barbier et al., 2019). Currently, existing and draft policies in Europe typically forbid the use of non-native species and restrict the use of non-native genotypes in macroalgae aquaculture (Loureiro et al., 2015; Barbier et al., 2019). Barbier et al. (2019) recommended that only local populations from native species or cultivars/strains selected from crosses between local genetic variants should be cultivated until the population dynamics and population genetics are better understood for each cultivated species. However, they also stated that data enabling a definition of a local population are still missing for most species.
The dynamics of invasion or introgression depends on factors that affect spread, hybridization, and fitness in the natural environment (e.g., type and longevity of propagules, patterns of propagule dispersal, relative population sizes, and physiological tolerances). In terrestrial plants, which, like macroalgae, are sessile and ectothermic organisms, strong patterns of local adaptation are often found along with local variations in the environment. Gene flow opposes local adaptation and can lead to maladaptation. On the other hand, selection also opposes gene flow, so the outcome depends on the relative strength of these two forces acting on the genetic make-up of populations (McKay et al., 2005).
There is limited understanding of population dynamics of macroalgae (reviewed by Schiel and Foster, 2006), and it is therefore difficult to predict and evaluate the risk of invasions and introgressions. The main natural dispersal mechanism for Laminarialean kelps is passive transport of microscopic stages of the life cycle with sea currents. These species release enormous quantities of microscopic zoospores that are viable for only a few days at the most (Schiel and Foster, 2006; Grant, 2016). Due to the limited viability, the spread of zoospores is usually rather limited (Fletcher and Callow, 1992; Billot et al., 2003; Reed et al., 2004; Grant, 2016; Itou et al., 2019), although observations and theoretical considerations suggest that dispersal in some cases can occur over distances of several kilometers (Gaylord et al., 2006; Grant, 2016). The microscopic gametophytes may live longer, but there is very little information on their contribution to dispersal (Schiel and Foster, 2006).
Recently, a number of population genetic analyses using molecular marker data have been conducted in order to describe the geographical pattern of genetic variation in kelp species. For example, based on microsatellite data, it has been shown that there is statistically significant genetic differentiation among both European and North-American populations of S. latissima, at least at distances above around 100 km (Guzinski et al., 2016; Nielsen et al., 2016; Paulino et al., 2016; Breton et al., 2018; Evankow et al., 2018; Luttikhuizen et al., 2018; Mooney et al., 2018). In spite of the genetic differentiation between populations, analysis of molecular variance indicated that most of the genetic variation (>90%) was found within populations rather than between populations (Breton et al., 2018; Mooney et al., 2018). The genetic structuring appears to be affected both by spread via ocean currents (Mooney et al., 2018) and by environmental variation and local adaptation (Nielsen et al., 2016). Similar studies of M. pyrifera populations along stretches of the American Pacific coast also identified several distinct genetic groups and suggested that both ocean currents and local adaptation to different environments can be important for this grouping (Alberto et al., 2011; Johansson et al., 2015; Camus et al., 2018).
The many examples of introductions of macroalgal species to new areas show that escaping individuals from cultivated populations are often able to survive and reproduce under various conditions in the wild. It has been suggested that the higher growth rate of cultivated individuals may provide a selective advantage when they spread to the natural environment (Williams and Smith, 2007). However, it might also be that individuals of natural populations have a selective advantage when it comes to other traits that may be more important in the natural environment than under cultivation. Genetic studies comparing farmed populations with nearby wild populations suggest that, in spite of the wild populations being a result of domestication of farmed populations, there is limited gene flow between them (Guzinski et al., 2018; Shan et al., 2018, 2019; Li et al., 2019).
Options to Develop Cultivars That Do Not Invade Natural Populations
Application of the precautionary principle regarding the use of non-native macroalgae leaves two main options for the breeding of genetically improved material for cultivation in the open sea: (1) develop local populations for local use, or (2) develop improved material that is not able to cross-hybridize with natural populations, and which could be cultivated without having to take the origin of the material into consideration. For option (1), questions regarding “how local” and “how different” the developed cultivars are, arise. More information on population genetics and population dynamics at species and regional levels is needed to give clearer answers to these questions (Barbier et al., 2019). Regarding option (2), there are several possible strategies that could be used to achieve sterile sporophytes that will not hybridize with natural populations, outlined below. Moreover, in many macroalgae, a trade-off between growth and reproductive development has been observed, i.e., sterile individuals grow substantially larger than fertile individuals (Guillemin et al., 2014; Liu et al., 2017). Thus, the use of sterile sporophytes in cultivation can have the positive side effect of increased productivity. Sterile cultivars would also have the effect that cultivators must buy new genetic material each year, providing an enlarged market for commercial breeding companies.
Sterility may be obtained in several ways, e.g., through polyploidization and/or species hybridization. Polyploidization has been used in the breeding of a range of terrestrial crops because polyploids are often superior to their diploid counterparts, particularly in size (Sattler et al., 2016). Polyploids, particularly triploids, as well as interspecific hybrids, often have reduced fertility due to problems with chromosome pairing during meiosis. Therefore, triploidy has been utilized in several fruit crops (e.g., watermelon, citrus, and banana species) to produce seedless fruits (Wang et al., 2016). Triploid plants can be produced when a diploid gamete hybridizes with a haploid one. This can occur if unreduced gametes form in a diploid plant and hybridize with haploid gametes, and it can occur in crosses between tetraploid and diploid plants (with diploid and haploid gametes, respectively) of the same or related species. Unreduced gametes can form spontaneously and can occur more frequently under environmental stress (Mason et al., 2011; Oppliger et al., 2014), and in species hybrids (Zhang et al., 2010). Several species in Laminariales can produce diploid gametophytes through apospory and possibly through endoreduplication (see section “Kelp Life Cycles” and Figure 1D). A better understanding of these processes and how they are environmentally and genetically regulated may allow the development of methods to obtain diploid gametes. Chromosome doubling can also be achieved artificially by treatment with chemicals that inhibit spindle formation and nuclear and cell division (e.g., colchicine, oryzaline, trifluralin) (Dhooghe et al., 2011). Moreover, triploid macroalgae, as well as hybrids between species that cannot hybridize by natural means, may be obtained by protoplast fusion (somatic hybridization), followed by plant regeneration (Reddy et al., 2008; Charrier et al., 2015). For recurrent selection-based development of triploid cultivars, it would be necessary to conduct the genetic improvement before inducing chromosome doubling, while subsequent propagation of the material prior to cultivation would have to be carried out through some form of asexual reproduction. The most obvious way to do this would be vegetative propagation of gametophyte cultures (one diploid and one haploid). After propagation, triploid sporophytes for cultivation could be generated by controlled induction of fertilization.
Like in higher plants, the transition to reproductive development in macroalgae is controlled by temperature and light factors, in interaction with genes (Liu et al., 2017; Martins et al., 2017; de Bettignies et al., 2018). As more is becoming known about this process in kelp species, it may become possible to select for (or engineer) alleles that will prevent reproductive development under the prevailing conditions or desynchronize the timing of reproduction in cultivated vs. wild populations. For example, alleles present in Southern European populations may confer different requirements than what can be fulfilled under Northern European conditions, and vice versa, and such alleles could be selected or engineered in a breeding program. Several molecular strategies have been developed in order to prevent transgenic terrestrial crops from hybridizing with natural populations of the same or related species (Ding et al., 2014), and these could also be employed in kelp breeding. Such strategies include, for instance, tissue-specific (in terrestrial crops: pollen, seed) expression of toxin-producing genes, and repression of genes with an essential function in reproductive organs. These strategies therefore necessitate in-depth knowledge of tissue-specific promoters or molecular regulation of organ development in the species in question. Our understanding of the genetic and molecular mechanisms underlying life cycle control in macroalgae is limited, but gradually increasing, particularly for the model species Ectocarpus siliculosus (Liu et al., 2017).
Evaluation of Options for Breeding of Laminarialean Kelp Species
Breeding Goals and Phenotyping
Breeding aims at changing the genetic make-up of a population in order to improve its traits in relation to the intended usage of the population. The first step in the design of a breeding program is to decide for the breeding goal—which trait(s) to improve. Traits expressed at the gametophyte stage are of importance for the maintenance of the genetic material and for the propagation and production of young sporophytes to be deployed in the production installations at sea. Examples of relevant traits are rate and stability of vegetative growth in culture, and fertility when transferred to conditions that induce sexual development. When it comes to the sporophyte stage, biomass (or yield) is an important trait, and it is encouraging that large improvements in kelp biomass have been made through breeding in Asia. Depending on the intended use, the concentration of certain compounds (protein, certain carbohydrates, and metal ions), or other qualities (taste, texture, morphology, color), are also of interest, as are both developmental traits and resistance mechanisms to various abiotic (e.g., temperature, light, waves, salinity) and biotic stresses (e.g., epiphytes, pathogens, herbivores), which in the end affect both yield and quality.
There are surely some biological trade-offs between traits, e.g., between growth on one hand and resistance to biotic or abiotic stress on the other hand, e.g., as described by Jormalainen and Honkanen (2004). Moreover, the level of genotype by environment interactions is often high in sessile ectothermic organisms. This is highly relevant for the breeding of cultivars, as the robustness, that is, the ability of a cultivar to perform well across a large geographical area and across year-to-year variation in conditions at each location, will benefit the breeding industry as well as the kelp producer. Robustness is also of increasing importance in the face of the unpredictable and variable future climate.
Phenotyping kelp cultivated in the sea can be more practically demanding than phenotyping crops in a field, particularly for traits that are recorded some time before harvest. Development of phenotyping protocols using young sporophytes cultivated under controlled conditions could allow for cheaper phenotyping and several generations per year. This would increase the breeding efficiency, provided that there is no or very limited genotype by environment interaction for the characterized traits. Also, development of high-throughput methods to assess chemical composition of the biomass could have a big impact. Chemical analysis is laborious and expensive, and could, after the development of prediction models for a range of chemical constituents, be replaced by efficient near-infrared spectroscopy (NIRS) or other spectroscopy techniques. Such techniques are currently used routinely in quality assessments of crop products, also for breeding purposes (e.g., Osborne, 2006; Fakayode et al., 2019).
Breeding Strategies
Breeding capitalizes on variation—the availability of different alleles of the genes. Breeding is largely about creating a pool of alleles, recombining these through meiosis and hybridization, and selecting among the generated variation. There are different ways of structuring the recombination, e.g., through hybridization between distinct genetic groups, self-fertilization and creation of inbred lines, and mixed hybridization in a population, like in the HB/SC, recurrent inbred line selection (RILS), and RMS cases described in Section “Development of Kelp Cultivars in the Past.” With RILS and RMS, the desired variation is selected over many generations, gradually accumulating favorable alleles and allele combinations, and improving the traits of interest (see section “Recurrent Selection and Accumulation of Favorable Alleles”). Hybridization of genetically distinct material can both increase variation on which selection can be applied and make it possible to exploit the positive effects on traits that can result from heterozygosity (see section “Heterosis and Utilization of Heterozygosity”).
Fundamental to the design of a breeding program is the reproductive system of the species, both with regard to the breeding process and with regard to the maintenance of material and propagation prior to cultivation. These features affect the design of breeding programs for these species. Because kelps, like many higher plants, have the capacity for vegetative propagation and self-fertilization (see section “Kelp Life Cycles”), kelp breeding can utilize the broad range of breeding methods that are employed in plant breeding (Acquaah, 2012), but that are not all relevant for breeding of mammals or fish. The presence of a free-living haploid gametophytic stage in Laminariales opens up additional possibilities for efficient DNA-based selection (see section “Marker-Assisted and Genome-Enabled Selection Methods”).
Recurrent Selection and Accumulation of Favorable Alleles
In naturally self-fertilizing crops (e.g., wheat), and also in crops that are naturally open-pollinating but can self (e.g., maize), producing inbred lines through recurrent selfing over a few generations generates almost fully homozygous lines (RILS). Selection among lines during or after this inbreeding process is an important means of sorting out undesired alleles and allele combinations and fixing and accumulating the desired ones. Selected inbred lines can be used as cultivars (e.g., wheat) or as parents of hybrid cultivars (e.g., maize), and they can enter a new cycle of recombination, inbreeding, and selection. In certain crops, e.g., perennial forage grasses and legumes, selfing is not possible due to self-incompatibility systems, and it is also not possible in mammals, birds, and fish, where each individual has one sex. These organisms must be crossed, either by mixed hybridization (as in RMS) or in a more structured way, and through recurrent selection and crossing, desired alleles and allele combinations can gradually accumulate. The improvement in a trait that can be made per year (genetic gain) in both these recurrent selection systems (RILS and RMS), is the product of the genetic variance for the trait of interest, the selection intensity, and the accuracy of the estimated breeding values on which selection is based, divided by the number of years it takes to go through one cycle of the breeding program (Falconer and McKay, 1996). The breeding value of an individual can be estimated on the basis of its own phenotype, the phenotypes of its relatives, or on genetically correlated traits. Recently, DNA information has become more common to utilize in prediction of breeding values (see section “Marker-Assisted and Genome-Enabled Selection Methods”).
Heterosis and Utilization of Heterozygosity
Heterosis (hybrid vigor) can be defined as the deviation of the offspring trait value from the average trait value of its parents or parental populations. There are many mechanisms behind heterosis defined in this broad manner (Kaeppler, 2012)—it is largely the result of increased heterozygosity (e.g., due to fewer homozygous recessive loci with negative effect, i.e., dominance), but it can also result from, e.g., new favorable combinations of alleles at different loci showing interaction (epistasis) or additive effects. Heterosis is more prominent the more genetically different the parental material is, because different alleles or different allele frequencies in the parental material will result in a high level of heterozygosity in the hybrid offspring. Heterosis gradually disappears over the generations following the initial hybrid cross, as the average heterozygosity decreases. Positive effects of heterozygosity can therefore be utilized as a last step in breeding programs, e.g., as a hybrid between recurrently inbred and selected lines (created through RILS), or a hybrid population of two separately recurrently selected populations (created through RMS). The expression of heterosis in these hybrids depends not only on the level of heterozygosity, but also to some extent on combining ability, i.e., favorable allele combinations.
Design of Breeding Programs for Laminarialean Kelp Species
Asian breeding of Laminarialean kelps so far (see section “Development of Kelp Cultivars in the Past”) was mainly based on hybridization of genetically different material and selection of specific crosses where the offspring had a much better performance than the parents (HB/SC). This was sometimes followed by RILS, i.e., by first identifying a cross between two gametophyte families expressing high heterosis, and then inbreeding and selection within the resulting population over a few generations in order to accumulate favorable alleles and allele combinations. Although most of the genetic improvement was achieved in the initial cross, subsequent RILS led to some additional genetic gain in breeding of Saccharina (Zhang et al., 2007; Li et al., 2016a). As the parent sporophytes of the crossed gametophyte cultures were not available, the true heterosis was not measured in the Asian studies; instead the hybrids were compared to the populations that the parents were originating from, or to control varieties. Moreover, the variation in heterosis among different parental combinations was frequently not reported. Nevertheless, there are several indications that the Asian kelp breeding so far has capitalized on both heterozygosity and on combining ability. Li et al. (2008b) found that the level of heterosis in yield-related traits in 14 Saccharina species hybrids correlated with the genetic distance between the parent gametophyte cultures (r = 0.70–0.82), suggesting a role of heterozygosity. However, there were also 12 hybrids in the study that either died or were discarded from the study due to low performance. This suggests that hybridization had a negative effect in many of the crosses, probably due to unfavorable allele combinations. The heterosis expressed in Saccharina species hybrid cultivars therefore appears to depend on both heterozygosity and the combining ability of the chosen pair of parental gametophyte cultures. Similarly, in a study of M. pyrifera, Westermeier et al. (2010) also found both positive and negative effects of hybridization.
A natural extension of the kelp breeding work conducted so far could be to create hybrid populations by crossing inbred lines that have been continuously selected for desired traits (see section “Design of Breeding Programs for Laminarialean Kelp Species”). Phenotypic characterization of offspring of an initial reciprocal diallel cross could be used to identify the gametophyte families (originating from one sporophyte) with the highest additive breeding values, and also the family combinations expressing the highest heterosis. These could then be inbred in order to fix the alleles with positive additive effects. Subsequent hybridization of such lines could add heterosis effects to a final hybrid for commercial production. Similar strategies have been very successful in some other organisms, e.g., maize (Andorf et al., 2019), and could be explored for kelps. The haploid or double haploid parthenosporophytes that can form spontaneously in Laminariales can potentially become very useful in breeding based on RILS, because they are like instantly inbred lines made in just one generation. Their use could therefore shorten the number of years of each cycle, and greatly increase the genetic gain per year. It may be possible to develop methods to create double haploid sporophytes from gametophyte cultures more efficiently, e.g., by using chemicals like colchicine. Moreover, there is an increasing understanding of how parthenogenesis in Ectocarpales is regulated and may be controlled (Bothwell et al., 2010; Coelho et al., 2011; Arun et al., 2013; Han et al., 2014), which may facilitate utilization of parthenogenesis in breeding of kelp cultivars in the future.
Cultivars that are based on HB/SC and RILS consist of genetically similar individuals—this homogeneity will be advantageous for management of the crop and utilization of the biomass but reduces the robustness of the crop in the face of variable conditions, and increases the risk of disease epidemics, necessitating the constant development of new cultivars. Moreover, RILS requires production and maintenance of a large number of inbred lines that need to be screened for performance, and HB/SC requires the labor-intensive production and maintenance of a large number of gametophyte cultures, which need to be screened for heterosis prior to selection. Gametophyte cultures require regular renewal of growing medium, can become contaminated, and grow very slowly, and the fewer the number of individual gametophytes that are used as a basis, the longer time it takes to produce enough biomass of each culture. However, the use of a large number of single-spore or sibling cultures allows for phenotyping relevant traits at the gametophyte stage and subsequent selection based on this. The parental gametophyte cultures of a hybrid cultivar must be maintained and amplified for a new round of hybridization each year. Preservation of gametophyte cultures is obviously less straightforward than storage of seeds of higher plants, but methods of long-term storage including cryopreservation are being developed (Taylor and Fletcher, 1999; Barrento et al., 2016).
An alternative to HB/SC and RILS is simple recurrent mixed hybridization and mass selection [RMS, see section “Recurrent Mixed Hybridization and Mass Selection (RMS)”]. With this method, the labor-intensive and time-consuming establishment and maintenance of large numbers of gametophyte cultures, controlled pair-crosses, and inbred lines can be avoided. The possibility to select genotypes which express desirable traits at the gametophyte stage is lost, but there will be some natural selection for, e.g., growth rate and fertility, occurring in the mixed culture. RMS has given significant short-term genetic gains in the same range as HB/SC and RILS (Zhang et al., 2011; Liu et al., 2014; Shan et al., 2016). It would likely be easy to carry out and very cost-efficient in the initial phases of breeding a new species. RMS exploits additive genetic variation but could be combined with steps that exploit positive effects of heterozygosity and combining ability (see section “Design of Breeding Programs for Laminarialean Kelp Species”). After selecting for additive gene effects with RMS, a diallel cross could be set up to identify specific combinations of gametophyte cultures (individual haploid genotypes or families originating from one sporophyte) expressing heterosis, which could subsequently be used for production of plants for cultivation. Alternatively, if an initial diallel cross at population level reveals that the offspring of certain combinations of populations express considerable heterosis on average, RMS could first be carried out in these populations separately to accumulate alleles with additive gene effects, then the best individuals in each population could be crossed to also exploit heterozygosity. This strategy is commonly used in various species like pig and poultry (Bourdon, 2013).
With RMS, it is, in most outcrossing species, necessary to balance the genetic gain with maintenance of sufficient genetic variation to avoid inbreeding depression and to make future progress possible. This can be achieved by controlling and restricting the rate of inbreeding per cycle in the breeding program. In outcrossing species, recessive alleles causing inbreeding depression can then be eliminated by natural selection (Meuwissen and Woolliams, 1994). However, selfing appears to be common among kelp species, and there is little knowledge on the degree of inbreeding depression in the various species. Obtaining more knowledge on this before designing a breeding program would be an advantage.
Marker-Assisted and Genome-Enabled Selection Methods
At present, S. japonica is the only kelp species whose genome has been sequenced (Ye et al., 2015; Liu et al., 2019). Transcriptomic and genomic studies have provided some insight into carbohydrate and halogen metabolism in S. japonica (Liang et al., 2014; Ye et al., 2015; Chi et al., 2018). Genetic maps have been constructed and quantitative trait loci (QTLs) identified for morphological traits related to yield in S. japonica and Saccharina longissima, and for sex determination in U. pinnatifida (Liu et al., 2009, 2011; Shan et al., 2015; Zhang et al., 2015). A few other brown algae has been sequenced up to now: E. siliculosus (Cock et al., 2010), Cladosiphon okamuranus (Nishitsuji et al., 2016), Nemacystus decipiens (Nishitsuji et al., 2019), Ectocarpus subulatus (Dittami et al., 2020). E. siliculosus has become a model for brown algae, the genome sequence forming the basis for a large number of studies of brown algae biology and evolution. E. siliculosus is a filamentous algae belonging to Ectocarpales, a sister order of Laminariales (Silberfeld et al., 2010), and knowledge gained from studies of this species is therefore also of some relevance for species in Laminariales. There is considerable similarity (∼80%) between homologous genes across S. japonica, L. digitata, M. pyrifera, and E. siliculosus (Konotchick et al., 2013; Ye et al., 2015; Liu et al., 2019), but the level of synteny (collinearity of gene or marker order along the chromosomes) between S. japonica and E. siliculosus appears to be low (Liu et al., 2019). Genetic maps and identified QTLs facilitate development of marker-assisted selection (MAS), where individuals are selected on the basis of their genotype of specific DNA markers genetically linked to genes conferring a trait of interest. This can be useful for known genes or loci explaining a large part of the genetic variation. Once developed, MAS assays can reduce costs in kelp breeding programs because it could be applied to gametophyte cultures before hybridization or selfing, or to very young sporophytes, allowing unwanted individuals to be discarded early in the process. Genomic selection (GS, Meuwissen et al., 2001) is a more advanced way of utilizing DNA marker information in breeding. Information on high-density single nucleotide polymorphism (SNP) markers across the whole genome can be combined with phenotype information to develop genomic prediction models for the desired phenotypes. Thus, the breeding values of individuals in a breeding program can be calculated on the basis of SNP information obtained by sequencing. Although the prediction models must be regularly updated during the breeding program in order to be accurate, GS can reduce the need for costly phenotyping and allows for screening of gametophyte cultures or very young sporophytes cultivated under controlled conditions. This can increase the genetic gain per year by (1) shortening the length of each cycle, (2) reducing the amount of resources allocated to phenotyping, allowing for more individuals to be screened and higher selection intensity to be applied (particularly relevant for traits that are costly to characterize), and (3) improving the accuracy of estimated breeding values, mainly because the actual genetic relationship between individuals can be estimated and replace expected genetic relationships.
Genetic Manipulation of Individuals
The use of random mutagenesis, transgenesis, and precise gene editing in algae was recently reviewed by Gan and Maggs (2017). Protocols for protoplast isolation, culture, and regeneration into plants exist for many species including kelps (Reddy et al., 2008). Random mutations can be induced by physical or chemical mutagens or occur spontaneously and be revealed as somaclonal variation in protoplast cultures and during other natural or manipulated mechanisms of regeneration, such as parthenogenesis and tissue culture (Charrier et al., 2015). Heritable genetic modification through transgenesis, insertion of gene constructs into random locations in the genome, is possible for a number of algae, including S. japonica and U. pinnatifida (Qin et al., 2012; Gan and Maggs, 2017). There are, however, still needs for optimization of, e.g., promoters, codon usage, and systems for selecting transformed cells (Lin and Qin, 2014; Mikami, 2014). Initial attempts of targeted gene editing have only been reported from a few microalgae so far (Mikami, 2014; Gan and Maggs, 2017). As our understanding of functional genomics in kelp species develops, gene editing has the potential to facilitate introduction of specific traits that are controlled by one or a few genes.
Conclusion
Macroalgae cultivation, a growing industry that could supply the human population with sustainable production of raw materials for food, feed, and a range of other products, would benefit greatly from the development of genetically improved macroalgae for cultivation. In non-domesticated kelp species, it should initially be possible to obtain considerable genetic improvement of desirable traits in a cultivation and utilization context, using a simple and cost-efficient breeding strategy based on mixed hybridization and simple phenotypic mass selection (RMS). Such a strategy would exploit additive genetic variation and could be employed by kelp growers, using local populations, e.g., from one fjord, as a starting point. The resulting improved populations would not contain new alleles, only changed frequencies of alleles. Concomitant with this first phase of breeding, relevant phenotypic data and high-density SNP data could be collected and analyzed in order to gain knowledge on the heritability and genetic variance of traits, genetic correlation between traits, inbreeding depression and the potential for genetic gain. RILS and utilization of heterosis (heterozygosity effects and combining ability) through hybrid breeding and selection among crosses (HB/SC) could well achieve comparable or larger genetic gain than RMS, at least in the short term. These strategies should be explored, but are more labor-intensive and also require laboratory and controlled environment facilities in order to manage and screen a large number of gametophyte cultures, crosses, and inbred lines. There are also several possible combined strategies that can utilize both additive genetic variation and heterosis. These could be utilized as breeding progresses and genetic information accumulates. A broader genetic base including populations from a larger geographic area covering a range of environmental conditions and genetic diversity would increase the potential for genetic gain. However, use of non-local genetic material may require development of technology to produce sporophytes that are non-reproductive in cultivation, or in some other way prevent hybridization with natural populations. Methodology to manipulate developmental processes in the life cycle, development of sophisticated phenotyping technologies, functional understanding of gene action, development of gene editing protocols, and implementation of DNA-based selection methods, would help to improve breeding efficiency and genetic gains as well as the introduction of novel traits in the future.
Author Contributions
FG and ÅE collected relevant literature, made the figures, and wrote the manuscript. GK and ÅE evaluated the options for breeding of kelps. GK revised and gave critical comments to all parts of the manuscript.
Funding
This research has been partly funded by the Norwegian Research Council (project number 280534—Breed4Kelp2Feed) and Eckbos Legat (project number 120840).
Conflict of Interest
The authors declare that the research was conducted in the absence of any commercial or financial relationships that could be construed as a potential conflict of interest.
References
Acquaah, G. (2012). Principles of Plant Genetics and Breeding, 2nd Edn. Hoboken, NJ: John Wiley and Sons.
Alberto, F., Raimondi, P. T., Reed, D. C., Watson, J. R., Siegel, D. A., Mitarai, S., et al. (2011). Isolation by oceanographic distance explains genetic structure for Macrocystis pyrifera in the Santa Barbara Channel. Mol. Ecol. 20, 2543–2554. doi: 10.1111/j.1365-294X.2011.05117.x
Andorf, C., Beavis, W. D., Huford, M., Smith, S., Suza, W. P., Wang, K., et al. (2019). Technological advances in maize breeding: past, present and future. Theor. Appl. Genet. 132, 817–849. doi: 10.1007/s00122-019-03306-3
Ar Gall, E., Asensi, A., Marie, D., and Kloareg, B. (1996). Parthenogenesis and apospory in the Laminariales: a flow cytometry analysis. Eur. J. Phycol. 31, 369–380. doi: 10.1080/09670269600651601
Arun, A., Peters, N. T., Scornet, D., Peters, A. F., Cock, J. M., and Coelho, S. M. (2013). Non-cell autonomous regulation of life cycle transitions in the model brown alga Ectocarpus. New Phytol. 197, 503–510. doi: 10.1111/nph.12007
Asensi, A., Ar Gall, E., Marie, D., Billot, C., Dion, P., and Kloareg, B. (2001). Clonal propagation of Laminaria digitata (Phaeophyceae) sporophytes through a diploid cell-filament suspension. J. Phycol. 37, 411–417. doi: 10.1046/j.1529-8817.2001.037003411.x
Barbier, M., Charrier, B., Araujo, R., Holdt, S. L., Jacquemin, B., and Rebours, C. (2019). PEGASUS - PHYCOMORPH European Guidelines for a Sustainable Aquaculture of Seaweeds, COST Action FA1406, eds M. Barbier and B. Charrier (Roscoff: Station biologique de Roscoff). doi: 10.21411/2c3w-yc73
Barrento, S., Camus, C., Sousa-Pinto, I., and Buschmann, A. H. (2016). Germplasm banking of the giant kelp: our biological insurance in a changing environment. Algal Res. 13, 134–140. doi: 10.1016/j.algal.2015.11.024
Bartsch, I. (2018). “Derivation of clonal stock cultures and hybridization of kelps,” in Protocols for Macroalgae Research eds B. Charrier, T. Wichard, and C. Reddy (New York, NY: CRC Press), 496.
Billot, C., Engel, C. R., Rousvoal, S., Kloareg, B., and Valero, M. (2003). Current patterns, habitat discontinuities and population genetic structure: the case of the kelp Laminaria digitata in the English Channel. Mar. Ecol. Prog. Ser. 253, 111–121. doi: 10.3354/meps253111
Bolton, J. J., Germann, I., and Lüning, K. (1983). Hybridization between Atlantic and Pacific representatives of the Simplices section of Laminaria (Phaeophyta). Phycologia 22, 133–140. doi: 10.2216/i0031-8884-22-2-133.1
Bothwell, J. H., Marie, D., Peters, A. F., Cock, J. M., and Coelho, S. M. (2010). Role of endoreduplication and apomeiosis during parthenogenetic reproduction in the model brown alga Ectocarpus. New Phytol. 188, 111–121. doi: 10.1111/j.1469-8137.2010.03357.x
Brawley, S. H., and Johnson, L. E. (1992). Gametogenesis, gametes and zygotes: an ecological perspective on sexual reproduction in the algae. Br. Phycol. J. 27, 233–252. doi: 10.1080/00071619200650241
Breton, T. S., Nettleton, J. C., O’Connell, B., and Bertocci, M. (2018). Fine-scale population genetic structure of sugar kelp, Saccharina latissima (Laminariales, Phaeophyceae), in eastern Maine, USA. Phycologia 57, 32–40. doi: 10.2216/17-72.1
Broch, O. J., Alver, M. O., Bekkby, T., Gundersen, H., Forbord, S., Handå, A., et al. (2019). The kelp cultivation potential in coastal and offshore regions of Norway. Front. Mar. Sci. 5:529. doi: 10.3389/fmars.2018.00529
Buschmann, A. H., Camus, C., Infante, J., Neori, A., Israel, Á, Hernández-González, M. C., et al. (2017). Seaweed production: overview of the global state of exploitation, farming and emerging research activity. Eur. J. Phycol. 52, 391–406. doi: 10.1080/09670262.2017.1365175
Camia, A., Robert, N., Jonsson, R., Pillis, R., García-Condado, S., López-Lozano, R., et al. (2018). Biomass Production, Supply, Uses and Flows in the European Union. First Results from an Integrated Assessment, EUR 28993 EN. Luxembourg: Publications Office of the European Union.
Campbell, I., Macleod, A., Sahlmann, C., Neves, L., Funderud, J., Øverland, M., et al. (2019). The environmental risks associated with the development of seaweed farming in Europe - prioritizing key knowledge gaps. Front. Mar. Sci. 6:107. doi: 10.3389/fmars.2019.00107
Camus, C., Faugeron, S., and Buschmann, A. H. (2018). Assessment of genetic and phenotypic diversity of the giant kelp, Macrocystis pyrifera, to support breeding programs. Algal Res. 30, 101–112. doi: 10.1016/j.algal.2018.01.004
Casas, G., Scrosati, R., and Piriz, M. L. (2004). The invasive kelp Undaria pinnatifida (Phaeophyceae, Laminariales) reduces native seaweed diversity in Nuevo Gulf (Patagonia, Argentina). Biol. Invasions 6, 411–416. doi: 10.1023/b:binv.0000041555.29305.41
Chapman, A. R. O. (1974). The genetic basis of morphological differentiation in some Laminaria populations. Mar. Biol. 24, 85–91. doi: 10.1007/bf00402851
Charrier, B., Abreu, M. H., Araujo, R., Bruhn, A., Coates, J. C., De Clerck, O., et al. (2017). Furthering knowledge of seaweed growth and development to facilitate sustainable aquaculture. New Phytol. 216, 967–975. doi: 10.1111/nph.14728
Charrier, B., Rolland, E., Gupta, V., and Reddy, C. R. (2015). Production of genetically and developmentally modified seaweeds: exploiting the potential of artificial selection techniques. Front. Plant Sci. 6:127. doi: 10.3389/fpls.2015.00127
Cheng, T.-H. (1969). Production of kelp – A major aspect of China’s exploitation of the sea. Econ. Bot. 23, 215–236. doi: 10.1007/bf02860454
Chi, S., Liu, T., Wang, X., Wang, R., Wang, S., Wang, G., et al. (2018). Functional genomics analysis reveals the biosynthesis pathways of important cellular components (alginate and fucoidan) of Saccharina. Curr. Genet. 64, 259–273. doi: 10.1007/s00294-017-0733-4
Cock, J. M., Sterck, L., Rouze, P., Scornet, D., Allen, A. E., Amoutzias, G., et al. (2010). The Ectocarpus genome and the independent evolution of multicellularity in brown algae. Nature 465, 617–621.
Coelho, S. M., Godfroy, O., Arun, A., Le Corguillé, G., Peters, A. F., and Cock, J. M. (2011). OUROBOROS is a master regulator of the gametophyte to sporophyte life cycle transition in the brown alga Ectocarpus. Proc. Natl. Acad. Sci. U.S.A. 108, 11518–11523. doi: 10.1073/pnas.1102274108
Cosson, J., and Gayral, P. (1979). Optimal conditions for growth and fertility of Laminaria digitata (Phaeophyceae) gametophytes. Proc. Int. Seaweed Symp. 9, 59–65.
Davidson, A. D., Campbell, M. L., Hewitt, C. L., and Schaffelke, B. (2015). Assessing the impacts of nonindigenous marine macroalgae: an update of current knowledge. Bot. Mar. 58, 55–79. doi: 10.1515/bot-2014-0079
de Bettignies, T., Wernberg, T., and Gurgel, C. F. D. (2018). Exploring the influence of temperature on aspects of the reproductive phenology of temperate seaweeds. Front. Mar. Sci. 5:218. doi: 10.3389/fmars.2018.00218
Dellatorre, F. G., Amoroso, R., Saravia, J., and Orensanz, J. M. (2014). Rapid expansion and potential range of the invasive kelp Undaria pinnatifida in the Southwest Atlantic. Aquat. Invasions 9, 467–478. doi: 10.3391/ai
Demes, K. W., and Graham, M. H. (2011). Abiotic regulation of investment in sexual versus vegetative reproduction in the clonal kelp Laminaria sinclairii (Laminariales, Phaeophyceae). J. Phycol. 47, 463–470. doi: 10.1111/j.1529-8817.2011.00981.x
Demes, K. W., Graham, M. H., and Suskiewicz, T. S. (2009). Phenotypic plasticity reconciles incongruous molecular and morphological taxonomies: the giant kelp, Macrocystis (Laminariales, Phaeophyceae), is a monospecific genus (note). J. Phycol. 45, 1266–1269. doi: 10.1111/j.1529-8817.2009.00752.x
Destombes, C., and Oppliger, L. V. (2011). Male gametophyte fragmentation in Laminaria digitata: a life history strategy to enhance reproductive success. Cah. Biol. Mar. 52, 385–394.
Dhooghe, E., Van Laere, K., Eeckhaut, T., Leus, L., and Van Huylenbroeck, J. (2011). Mitotic chromosome doubling of plant tissues in vitro. Plant Cell Tissue Organ Cult. 104, 359–373. doi: 10.1007/s11240-010-9786-5
Ding, J., Duan, H., Deng, Z., Zhao, D., Yi, G., McAvoy, R., et al. (2014). Molecular strategies for addressing gene flow problems and their potential applications in abiotic stress tolerant transgenic plants. Crit. Rev. Plant Sci. 33, 190–204. doi: 10.1080/07352689.2014.870414
Directorate of Fisheries (2019). Economic and Biological Figures from Norwegian Fisheries – 2018. Bergen: Directorate of Fisheries, 38.
Dittami, S. M., Corre, E., Brillet, L., Lipinska, A. P., Pontoizeau, N., Aite, M., et al. (2020). The genome of Ectocarpus subulatus – A highly stress-tolerant brown alga. Mar. Genomics 11:100740. doi: 10.1016/j.margen.2020.100740.
Druehl, L. D., Collins, J. D., Lane, C. E., and Saunders, G. W. (2005). An evaluation of methods used to assess intergeneric hybridization in kelp using Pacific Laminariales (Phaeophyceae). J. Phycol. 41, 250–262. doi: 10.1111/j.1529-8817.2005.04143.x
Duarte, C. M., Holmer, M., Olsen, Y., Soto, D., Marbá, N., Guiu, J., et al. (2009). Will the oceans help feed humanity? Bioscience 59, 967–976. doi: 10.1525/bio.2009.59.11.8
Duarte, C. M., Wu, J., Xiao, X., Bruhn, A., and Krause-Jensen, D. (2017). Can seaweed farming play a role in climate change mitigation and adaptation? Front. Mar. Sci. 4:100. doi: 10.3389/fmars.2017.00100
Ellstrand, N. C., Meirmans, P., Rong, J., Bartsch, D., Ghosh, A., de Jong, T. J., et al. (2013). Introgression of crop alleles into wild or weedy populations. Annu. Rev. Ecol. Evol. Syst. 44, 325–345. doi: 10.1146/annurev-ecolsys-110512-135840
Evankow, A., Christie, H., Hancke, K., Brysting, A. K., Junge, C., Fredriksen, S., et al. (2018). Genetic heterogeneity of two bioeconomically important kelp species along the Norwegian coast. Conserv. Genet. 20, 615–628. doi: 10.1007/s10592-019-01162-8
Fakayode, S. O., Baker, G. A., Bwambok, D. K., Bhawawet, N., Elzey, B., Siraj, N., et al. (2019). Molecular (Raman, NIR, and FTIR) spectroscopy and multivariate analysis in consumable products analysis1. Appl. Spectrosc. Rev. 144:324. doi: 10.1080/05704928.2019.1631176
Falconer, D. S., and McKay, T. F. C. (1996). Introduction to Quantitative Genetics, 4th Edn. London: Pearson Education Limited.
Fang, T. C. (1984). Some genetic features revealed from culturing the haploid cells of kelps. Hydrobiologia 11, 317–318. doi: 10.1007/bf00027693
Fang, T. C., and Jiang, B. Y. (1963). Inheritance of frond length in Laminaria japonica Aresch. Oceanol. Limnol. Sin. 5, 172–182.
Fang, T. C., Wu, C. Y., Jiang, Y., Li, T. T., and Ren, G. Z. (1962a). The breeding of a new breed of haidai (Laminaria japonica Aresch.) and its preliminary genetic analysis. Acta Bot. Sin. 10, 197–209.
Fang, T. C., Wu, C. Y., and Li, C. Z. (1962b). Increased adaptability to high temperature of gametophytes and sporelings of the Haiqing No. 1 breed of Laminaria japonica Aresch. Oceanol. Limnol. Sin. 4, 29–37.
FAO (2018). The State of World Fisheries and Aquaculture 2018: Meeting the sustainable Development Goals. Rome: FAO, 210.
Faust, E., Halvorsen, K. T., Andersen, P., Knutsen, H., and André, C. (2018). Cleaner fish escape salmon farms and hybridize with local wrasse populations. R. Soc. Open Sci. 5:171752. doi: 10.1098/rsos.171752
Fernand, F., Israel, A., Skjermo, J., Wichard, T., Timmermans, K. R., and Golberg, A. (2017). Offshore macroalgae biomass for bioenergy production: environmental aspects, technological achievements and challenges. Renew. Sustain. Energy Rev. 75, 35–45. doi: 10.1016/j.rser.2016.10.046
Filbee-Dexter, K., Wernberg, T., Fredriksen, S., Magnus Norderhaug, K., and Foldager Pedersen, M. (2019). Arctic kelp forests: diversity, resilience and future. Glob. Planet. Change 172, 1–14. doi: 10.1016/j.gloplacha.2018.09.005
Fletcher, R. L., and Callow, M. E. (1992). The settlement, attachment and establishment of marine algal spores. Br. Phycol. J. 27, 303–329. doi: 10.1080/00071619200650281
Fletcher, R. L., and Farrell, P. (1999). Introduced brown algae in the North East Atlantic, with particular respect to Undaria pinnatifida (Harvey) Suringar. Helgol. Meeresunters. 52, 259–275. doi: 10.1007/bf02908901
Fleurence, J., Morancais, M., Dumay, J., Turpin, V., Munier, M., Garcia-Buneo, N., et al. (2012). What are the prospects for using seaweeds in human nutrition and for marine animals raised through aquaculture? Trends Food Sci. Technol. 27, 57–61. doi: 10.1016/j.tifs.2012.03.004
Forbord, S., Skjermo, J., Arff, J., Handå, A., Reitan, K. I., Bjerregaard, R., et al. (2012). Development of Saccharina latissima (Phaeophyceae) kelp hatcheries with year-round production of zoospores and juvenile sporophytes on culture ropes for kelp aquaculture. J. Appl. Phycol. 24, 393–399. doi: 10.1007/s10811-011-9784-y
Forbord, S., Steinhovden, K. B., Rød, K. K., Handå, A., and Skjermo, J. (2018). “Cultivation protocol for Saccharina latissima,” in Protocols for Macroalgae Research, eds B. Charrier, T. Wichard, and C. Reddy (New York, NY: CRC Press), 496.
Fraser, C. I. (2012). Is bull-kelp kelp? The role of common names in science. N. Z. J. Mar. Freshw. Res. 46, 279–284. doi: 10.1080/00288330.2011.621130
Fraser, C. I., Hay, C. H., Spencer, H. G., and Waters, J. M. (2009). Genetic and morphological analyses of the southern bull kelp Durvillaea antarctica (Phaeophyceae: Durvillaeales) in New Zealand reveal cryptic species. J. Phycol. 45, 436–443. doi: 10.1111/j.1529-8817.2009.00658.x
Gan, S. Y., and Maggs, C. A. (2017). Random mutagenesis and precise gene editing technologies: applications in algal crop improvement and functional genomics. Eur. J. Phycol. 52, 466–481. doi: 10.1080/09670262.2017.1358827
Gaylord, B., Reed, D. C., Raimondi, P. T., and Washburn, L. (2006). Macroalgal spore dispersal in coastal environments: mechanistic insights revealed by theory and experiment. Ecol. Monogr. 76, 481–502. doi: 10.1890/0012-9615(2006)076%5B0481:msdice%5D2.0.co;2
Gjedrem, T., Robinson, N., and Rye, M. (2012). The importance of selective breeding in aquaculture to meet future demands for animal protein: a review. Aquaculture 350–353, 117–129. doi: 10.1016/j.aquaculture.2012.04.008
Glover, K. A., Pertoldi, C., Besnier, F., Wennevik, V., Kent, M., and Skaala, Ø. (2013). Atlantic salmon populations invaded by farmed escapees: quantifying genetic introgression with a Bayesian approach and SNPs. BMC Genet. 14:74. doi: 10.1186/1471-2156-14-74
Grant, W. S. (2016). “Paradigm shifts in the phylogeographic analysis of seaweeds,” in Seaweed Phylogeography, eds Z.-M. Hu and C. Fraser (Dordrecht: Springer), 23–62.
Guillemin, M. L., Valenzuela, P., Gaitán-Espitia, J. D., and Destombe, C. (2014). Evidence of reproductive cost in the triphasic life history of the red alga Gracilaria chilensis (Gracilariales. Rhodophyta). J. Appl. Phycol. 26, 569–575. doi: 10.1007/s10811-013-0072-x
Guzinski, J., Ballenghien, M., Daguin-Thiébaut, C., Lévêque, L., and Viard, F. (2018). Population genomics of the introduced and cultivated Pacific kelp Undaria pinnatifida: marinas—not farms—drive regional connectivity and establishment in natural rocky reefs. Evol. Appl. 11, 1582–1597. doi: 10.1111/eva.12647
Guzinski, J., Mauger, S., Cock, J. M., and Valero, M. (2016). Characterization of newly developed expressed sequence tag-derived microsatellite markers revealed low genetic diversity within and low connectivity between European Saccharina latissima populations. J. Appl. Phycol. 28, 3057–3070. doi: 10.1007/s10811-016-0806-7
Hafting, J. T., Craigie, J. S., Stengel, D. B., Loureiro, R. R., Buschmann, A. H., Yarish, C., et al. (2015). Prospects and challenges for industrial production of seaweed bioactives. J. Phycol. 51, 821–837. doi: 10.1111/jpy.12326
Han, J. W., Klochkova, T. A., Shim, J., Nagasato, C., Motomura, T., and Kim, G. H. (2014). Identification of three proteins involved in fertilization and parthenogenetic development of a brown alga, Scytosiphon lomentaria. Planta 240, 1253–1267. doi: 10.1007/s00425-014-2148-5
Hayashi, L., Reis, R. P., dos Santos, A. A., Castelar, B., Robledo, D., de Vega, B. G., et al. (2017). “The cultivation of Kappaphycus and Eucheuma in tropical and sub-tropical waters,” in Tropical Seaweed Farming Trends, Problems and Opportunities Focus on Kappaphycus and Eucheuma of Commerce. Developments in Applied Phycology, Vol. 9, eds A. Q. Hurtado, A. T. Critchley, and I. C. Neish (Cham: Springer), 55–90. doi: 10.1007/978-3-319-63498-2_4
Heesch, S., Serrano-Serrano, M., Luthrunger, R., Peters, A. F., Destombe, C., Cock, M., et al. (2019). Evolution of life cycles and reproductive traits: insights from the brown algae. bioRxiv doi: 10.1101/530477
Holdt, S. L., and Kraan, S. (2011). Bioactive compounds in seaweed: functional food applications and legislation. J. Appl. Phycol. 23, 543–597. doi: 10.1007/s10811-010-9632-5
Hwang, E. K., Gong, Y. G., and Park, C. S. (2012). Cultivation of a hybrid of free-living gametophytes between Undariopsis peterseniana and Undaria pinnatifida: morphological aspects and cultivation period. J. Appl. Phycol. 24, 401–408. doi: 10.1007/s10811-011-9727-7
Hwang, E. K., Ha, D. S., and Park, C. S. (2017). Strain selection and initiation timing influence the cultivation period of Saccharina japonica and their impact on the abalone feed industry in Korea. J. Appl. Phycol. 29, 2297–2305. doi: 10.1007/s10811-017-1179-2
Hwang, E. K., Hwang, I. K., Park, E. J., Gong, Y. G., and Park, C. S. (2014). Development and cultivation of F2 hybrid between Undariopsis peterseniana and Undaria pinnatifida for abalone feed and commercial mariculture in Korea. J. Appl. Phycol. 26, 747–752. doi: 10.1007/s10811-013-0164-7
Hwang, E. K., Yotsukura, N., Pang, S. J., Su, L., and Shan, T. F. (2019). Seaweed breeding programs and progress in eastern Asian countries. Phycologia 58, 484–495. doi: 10.1080/00318884.2019.1639436
IOIMF (Section of Seaweed Genetics and Breeding, Institute of Oceanology, Section of seaweed cultivation, Institute of Marine Fisheries) (1976). The breeding of new varieties of Haidai (Laminaria japonica) with high production and high iodine content. Sci. Sin. 19, 243–252.
Itou, T., Kanno, M., Suyama, Y., Inaba, K., and Aoki, M. N. (2019). Opening the black box: microspatial patterns of zoospore dispersal, parentage, and selfing in the kelp Ecklonia cava as revealed by microsatellite markers. J. Appl. Phycol. 31, 3283–3294. doi: 10.1007/s10811-019-01790-0
Jansson, E., Quintela, M., Dahle, G., Albretsen, J., Knutsen, H., Andrè, K., et al. (2017). Genetic analysis of goldsinny wrasse reveals evolutionary insights into population connectivity and potential evidence of inadvertent translocation via aquaculture. ICES J. Mar. Sci. 74, 2135–2147. doi: 10.1093/icesjms/fsx046
Johansson, M. L., Alberto, F., Reed, D. C., Raimondi, P. T., Coelho, N. C., and Young, M. A. (2015). Seascape drivers of Macrocystis pyrifera population genetic structure in the northeast Pacific. Mol. Ecol. 24, 4866–4885. doi: 10.1111/mec.13371
Jormalainen, V., and Honkanen, T. (2004). Variation in natural selection for growth and phlorotannins in the brown alga Fucus vesiculosus. J. Evol. Biol. 17, 807–820. doi: 10.1111/j.1420-9101.2004.00715.x
Kaeppler, S. (2012). Heterosis: many genes, many mechanisms - end the search for an undiscovered unifying theory. Int. Sch. Res. Netw. ISRN Bot. 2012:682824. doi: 10.5402/2012/682824
Keeling, P. J., Burger, G., Durnford, D. G., Lang, B. F., Lee, R. W., Pearlman, R. E., et al. (2005). The tree of eukaryotes. Trends Ecol. Evol. 20, 670–676.
Kilar, J. A., Hanisak, M. D., and Yoshida, T. (1992). “On the expression of phenotypic variability: why is Sargassum so taxonomically difficult?,” in Taxonomy of Economic Seaweeds: With Reference to Some Pacific and Western Atlantic species, Vol. 3, ed. I. A. Abbott (San Diego, CA: California Sea Grant College), 95–117.
Kim, J. K., Yarish, C., Hwang, E. K., Park, M., and Kim, Y. (2017). Seaweed aquaculture: cultivation technologies, challenges and its ecosystem services. Algae 32, 1–13. doi: 10.4490/algae.2017.32.3.3
Konotchick, T., Dupont, C. L., Valas, R. E., Badger, J. H., and Allen, A. E. (2013). Transcriptomic analysis of metabolic function in the giant kelp, Macrocystis pyrifera, across depth and season. New Phytol. 198, 398–407. doi: 10.1111/nph.12160
Kraan, S., Tramullas, A. V., and Guiry, M. D. (2000). The edible brown seaweed Alaria esculenta (Phaeophyceae, Laminariales): hybridization, growth and genetic comparisons of six Irish populations. J. Appl. Phycol. 12, 577–583.
Lehahn, Y., Ingle, K. N., and Golberg, A. (2016). Global potential of offshore and shallow waters macroalgal biorefineries to provide for food, chemicals and energy: feasibility and sustainability. Algal Res. 17, 150–160. doi: 10.1016/j.algal.2016.03.031
Lewis, R. J., Jiang, B. Y., Neushul, M., and Fei, X. G. (1993). Haploid parthenogenetic sporophytes of Laminaria japonica (Phaeophyceae). J. Phycol. 29, 363–369. doi: 10.1111/j.0022-3646.1993.00363
Lewis, R. J., Neushul, M., and Harger, B. W. W. (1986). Interspecific hybridization of the species of Macrocystis in California. Aquaculture 57, 203–210. doi: 10.1016/0044-8486(86)90198-5
Li, D., Zhou, Z., Liu, H., and Wu, C. (1999). “A new method of Laminaria japonica strain selection and sporeling raising by the use of gametophyte clones,” in Sixteenth International Seaweed Symposium, Developments in Hydrobiology, Vol. 137, eds J. M. Kain, M. T. Brown, and M. Lahaye (Dordrecht: Springer), doi: 10.1007/978-94-011-4449-0_57
Li, Q., Shan, T., Wang, X., and Pang, S. (2019). Evaluation of the genetic relationship between the farmed populations on a typical kelp farm and the adjacent subtidal spontaneous population of Undaria pinnatifida (Phaeophyceae, Laminariales) in China. J. Appl. Phycol. doi: 10.1007/s10811-019-01917-3
Li, X. J., Cong, Y. Z., Yang, G. P., Shi, Y. Y., Qu, S. C., Li, Z. L., et al. (2007). Trait evaluation and trial cultivation of Dongfang no. 2, the hybrid of a male gametophyte clone of Laminaria longissima (Laminariales, Phaeophyta) and a female one of L. japonica. J. Appl. Phycol. 19, 139–151. doi: 10.1007/s10811-006-9120-0
Li, X., Liu, J. L., Cong, Y. Z., Qu, S. C., Zhang, Z. Z., Dai, H. L., et al. (2008a). Breeding and trial cultivation of Dongfang no.3, a hybrid of Laminaria gametophyte clones with a more than intraspecific less than interspecific relationship. Aquaculture 280, 76–80. doi: 10.1016/j.aquaculture.2008.05.005
Li, X., Yang, G., Shi, Y., Cong, Y., Che, S., Qu, S., et al. (2008b). Prediction of the heterosis of Laminaria hybrids with the genetic distance between their parental gametophyte clones. J. Appl. Phycol. 20, 1097–1102. doi: 10.1007/s10811-008-9321-9
Li, X. J., Zhang, Z., Qu, S., Liang, G., Sun, J., Zhao, N., et al. (2016a). Improving seedless kelp (Saccharina japonica) during its domestication by hybridizing gametophytes and seedling-raising from sporophytes. Sci. Rep. 6:21255. doi: 10.1038/srep21255
Li, X. J., Zhang, Z., Qu, S., Liang, G., Zhao, N., Sun, J., et al. (2016b). Breeding of an intraspecific kelp hybrid Dongfang no. 6 (Saccharina japonica, Phaeophyceae, Laminariales) for suitable processing products and evaluation of its culture performance. J. Appl. Phycol. 28, 439–447.
Liang, X., Wang, X., Chi, S., Wu, S., Sun, Jg, et al. (2014). Analysis of Saccharina japonica transcriptome using the high-throughput DNA sequencing technique and its vanadium-dependent haloperoxidase gene. Acta Oceanol. Sin. 33, 27–36. doi: 10.1007/s13131-014-0438-1
Lim, P.-E., Yang, L.-E., Tan, J., Maggs, C. A., and Brodie, J. (2017). Advancing the taxonomy of economically important red seaweeds (Rhodophyta). Eur. J. Phycol. 52, 438–451. doi: 10.1080/09670262.2017.1365174
Lin, H., and Qin, S. (2014). Tipping points in seaweed genetic engineering: scaling up opportunities in the next decade. Mar. Drugs 12, 3025–3045. doi: 10.3390/md12053025
Liptack, M., and Druehl, L. (2000). Molecular evidence for an interfamilial laminarialean cross. Eur. J. Phycol. 35, 135–142. doi: 10.1080/09670260010001735721
Liu, F., Wang, X., Liu, J., Fu, W., Duan, D., and Yang, Y. (2009). Genetic mapping of tha Laminaria japonica (Laminarales, Phaeophyta) using amplified fragment length polymorphism markers. J. Phycol. 45, 1228–1233. doi: 10.1111/j.1529-8817.2009.00729.x
Liu, F., Yao, J., Wang, X., Hu, Z., and Duan, D. (2011). Identification of SCAR marker linking to longer frond length of Saccharina japonica (Laminariales, Phaeophyta) using bulked-segregant analysis. J. Appl. Phycol. 23, 709–713. doi: 10.1007/s10811-010-9567-x
Liu, F. L., Sun, X. T., Wang, F. J., Wang, W. J., Liang, Z. R., Lin, Z., et al. (2014). Breeding, economic traits evaluation, and commercial cultivation of a new Saccharina variety “Huangguan No. 1”. Aquac. Int. 22, 1665–1675. doi: 10.1007/s10499-014-9772-8
Liu, T., Wang, X., Wang, G., Jia, S., Liu, G., Shan, G., et al. (2019). Evolution of complex thallus alga: genome sequencing of Saccharina japonica. Front. Genet. 10:378. doi: 10.3389/fgene.2019.00378
Liu, X., Bogaert, K., Engelen, A. H., Leliaert, F., Roleda, M. Y., and De Clerck, O. (2017). Seaweed reproductive biology: environmental and genetic controls. Bot. Mar. 60, 89–108.
Loureiro, R., Gachon, C. M. M., and Rebours, C. (2015). Seaweed cultivation: potential and challenges of crop domestication at an unprecedented pace. New Phytol. 206, 489–492. doi: 10.1111/nph.13278
Lüning, K. (1980). Critical levels of light and temperature regulating gametogenesis of three Laminaria species. J. Phycol. 16, 1–15. doi: 10.1111/j.1529-8817.1980.tb02992.x
Lüning, K., Chapman, A. R. O., and Mann, K. H. (1978). Crossing experiments in the non-digitate complex of Laminaria from both sides of the Atlantic. Phycologia 17, 293–298. doi: 10.2216/i0031-8884-17-3-293.1
Lüning, K., and Dring, M. J. (1975). Reproduction, growth and photosynthesis of gametophytes of Laminaria saccharina grown in blue and red light. Mar. Biol. 29, 195–200. doi: 10.1007/bf00391846
Lüning, K., and Pang, S. (2003). Mass cultivation of seaweeds: current aspects and approaches. J. Appl. Phycol. 15, 115–119. doi: 10.1023/a:1023807503255
Luttikhuizen, P. C., van den Heuvel, F. H. M., Rebours, C., Witte, H. J., van Bleijswijk, J. D. L., and Timmermans, K. (2018). Strong population structure but no equilibrium yet: genetic connectivity and phylogeography in the kelp Saccharina latissima (Laminariales. Phaeophyta). Ecol. Evol. 8, 4265–4277. doi: 10.1002/ece3.3968
Martins, N., Pearson, G. A., Gouveia, L., Tavares, A. I., Serrão, E. A., and Bartsch, I. (2019). Hybrid vigour for thermal tolerance in hybrids between the allopatric kelps Laminaria digitata and L. pallida (Laminariales, Phaeophyceae) with contrasting thermal affinities. Eur. J. Phycol. 54, 548–561. doi: 10.1080/09670262.2019.1613571
Martins, N., Tanttu, H., Pearson, G. A., Serrao, E. A., and Bartsch, I. (2017). Interactions of daylength, temperature and nutrients affect thresholds for life stage transitions in the kelp Laminaria digitata (Phaeophyceae). Bot. Mar. 60, 109–121.
Mason, A. S., Nelson, M. N., Yan, G., and Cowling, W. A. (2011). Production of viable male unreduced gametes in Brassica interspecific hybrids is genotype specific and stimulated by cold temperatures. BMC Plant Biol. 11:103. doi: 10.1186/1471-2229-11-103
McKay, J. K., Christian, C. E., Harrison, S., and Rice, K. J. (2005). ≪ How local is local?≫ - a review of practical and conceptual issues in the genetics of restoration. Restor. Ecol. 13, 432–440. doi: 10.1111/j.1526-100x.2005.00058.x
Meland, M., and Rebours, C. (2012). Short description of the Norwegian seaweed industry. Bioforsk FOKUS 7, 275–277.
Meuwissen, T. H. E., Hayes, B. J., and Goddard, M. E. (2001). Prediction of total genetic value using genome-wide dense marker maps. Genetics 157, 1819–1829.
Meuwissen, T. H. E., and Woolliams, J. A. (1994). Maximizing genetic response in breeding schemes of dairy cattle with constraints on variance of response. J. Dairy Sci. 77, 1905–1916. doi: 10.3168/jds.s0022-0302(94)77133-2
Mikami, K. (2014). A technical breakthrough close at hand: feasible approaches toward establishing a gene-targeting genetic transformation system in seaweeds. Front. Plant Sci. 5:498. doi: 10.3389/fpls.2014.00498
Milledge, J. J., and Harvey, P. J. (2016). Potential process ‘hurdles’ in the use of macroalgae as feedstock for biofuel production in the British Isles. J. Chem. Technol. Biotechnol. 91, 2221–2234. doi: 10.1002/jctb.5003
Miller, K. A., and Engle, J. M. (2009). “The natural history of Undaria pinnatifida and Sargassum filicinum at the California channel islands: non-native seaweeds with different invasion styles,” in Proceedings of the 7th California Islands Symposium, eds C. C. Damiani and D. K. Garcelon (Arcata, CA: Institute for Wildlife Studies), 131–140.
Minchin, D., and Nunn, J. (2014). The invasive brown alga Undaria pinnatifida (Harvey) Suringar, 1873 (Laminariales, Alariaceae), spreads nothwards in Europe. Bioinvasions Rec. 3, 57–63. doi: 10.3391/bir.2014.3.2.01
Mooney, K. M., Beatty, G. E., Elsäßer, B., Follis, E. S., Kregting, L., O’Connor, N. E., et al. (2018). Hierarchical structuring of genetic variation at differing geographic scales in the cultivated sugar kelp Saccharina latissima. Mar. Environ. Res. 142, 108–115. doi: 10.1016/j.marenvres.2018.09.029
Müller, D. G., Maier, I., Marie, D., and Westermeier, R. (2016). Nuclear DNA level and life cycle of kelps: evidence for sex-specific ployteny in Macrocystis (Laminariales, Phaeophyceae). J. Phycol. 52, 157–160. doi: 10.1111/jpy.12380
Müller, D. G., Murúa, P., and Westermeier, R. (2019). Reproductive strategies of Lessonia berteroana (Laminariales, Phaeophyceae) gametophytes from Chile: apogamy, parthenogenesis and cross-fertility with L. spicata. J. Appl. Phycol. 31, 1475–1481. doi: 10.1007/s10811-018-1625-9
Murúa, P., Müller, D. G., Patiño, D. J., and Westermeier, R. (2017). Giant kelp vegetative propagation: adventitious holdfast elements rejuvenate senescent individuals of the Macrocystis pyrifera “integrifolia” ectomorph. J. Phycol. 53, 230–234. doi: 10.1111/jpy.12493
Nakahara, H., and Nakamura, Y. (1973). Parthenogenesis, apogamy and apospory in Alaria crassifolia (Laminariales). Mar. Biol. 18, 327–332.
Naylor, R. L., Williams, S. L., and Strong, D. R. (2001). Aquaculture - a gateway for exotic species. Science 294, 1655–1656. doi: 10.1126/science.1064875
Nielsen, M. M., Paulino, C., Neiva, J., Krause-Jensen, D., Bruhn, A., and Serrao, E. A. (2016). Genetic diversity of Saccharina latissima (Phaeophyceae) along a salinity gradient in the North Sea – Baltic Sea transition zone. J. Phycol. 52, 523–531. doi: 10.1111/jpy.12428
Nishitsuji, K., Arimoto, A., Higa, Y., Mekaru, M., Kawamitsu, M., Satoh, N., et al. (2019). Draft genome of the brown alga, Nemacystus decipiens, Onna-1 strain: fusion of genes involved in the sulfated fucan biosynthesis pathway. Sci. Rep. 9:4607.
Nishitsuji, K., Arimoto, A., Iwai, K., Sudo, Y., Hisata, K., Fujie, M., et al. (2016). A draft genome of the brown alga, Cladosiphon okamuranus, S-strain: a platform for future studies of ‘mozuku’ biology. DNA Res. 23, 561–570. doi: 10.1093/dnares/dsw039
Niwa, K., and Kobiyama, A. (2019). Development of a new cultivar with high yield and high-temperature tolerance by crossbreeding of Undaria pinnatifida (Laminariales, Phaeophyta). Aquaculture 506, 30–34. doi: 10.1016/j.aquaculture.2019.03.002
Olafsen, T., Winther, U., Olsen, Y., and Skjermo, J. (2012). Verdiskaping Basert på Produktive hav i 2050. Available at: https://www.sintef.no/globalassets/upload/fiskeri_og_havbruk/publikasjoner/verdiskaping-basert-pa-produktive-hav-i-2050.pdf
Oppliger, L. V., Correa, J. A., and Peters, A. F. (2007). Parthenogenesis in the brown algae Lessonia nigrescens (Laminariales, Phaeophyceae) from Chile. J. Phycol. 43, 1295–1301. doi: 10.1111/j.1529-8817.2007.00408.x
Oppliger, L. V., von Dassow, P., Bouchemousse, S., Robuchon, M., Valero, M., Correa, J. A., et al. (2014). Alteration of sexual reproduction and genetic diversity in the kelp species Laminaria digitata at the Southern limit of its range. PLoS One 9:e102518. doi: 10.1371/journal.pone.0102518
Osborne, B. G. (2006). Applications of near infrared spectroscopy in quality screening of early-generation material in cereal breeding programmes. J. Near Infrared Spectrosc. 14, 93–101. doi: 10.1255/jnirs.595
Øverland, M., Mydland, L. T., and Skrede, A. (2018). Marine macroalgae as sources of protein and bioactive compounds in feed for monogastric animals. J. Sci. Food Agric. 99, 13–24. doi: 10.1002/jsfa.9143
Pang, S., and Lüning, K. (2004). Breaking seasonal limitation: year-round sporogenesis in the brown alga Laminaria saccharina by blocking the transport of putative sporulation inhibitors. Aquaculture 240, 531–541. doi: 10.1016/j.aquaculture.2004.06.034
Pang, S. J., Hu, X. Y., Wu, C. Y., Hirosawa, A., and Ohno, M. (1997). Intraspecific crossing of Undaria pinnatifida (Harv.) - A possible time-saving way of strain selection. Chin. J. Oceanol. Limnol. 15, 227–235. doi: 10.1007/bf02850878
Patwary, M. U., and van der Meer, J. P. (1992). Genetics and breeding of cultivated seaweeds. Algae 7, 281–318.
Paulino, C., Neiva, J., Coelho, N. C., Aires, T., Marbà, N., Krause-Jensen, D., et al. (2016). Characterization of 12 polymorphic microsatellite markers in the sugar kelp Saccharina latissima. J. Appl. Phycol. 28, 3071–3074. doi: 10.1007/s10811-016-0811-x
Pickering, T. D., Skelton, P., and Sulu, R. J. (2007). Intentional introductions of commercially harvested alien seaweeds. Bot. Mar. 50, 338–350. doi: 10.1515/BOT.2007.039
Qin, S., Lin, H., and Jiang, P. (2012). Advances in genetic engineering of marine algae. Biotechnol. Adv. 30, 1602–1613. doi: 10.1016/j.biotechadv.2012.05.004
Ratcliff, J. J., Soler-Vila, A., Hanniffy, D., Johnson, M. P., and Edwards, M. D. (2017). Optimisation of kelp (Laminaria digitata) gametophyte growth and gametogenesis: effects of photoperiod and culture media. J. Appl. Phycol. 29, 1957–1966. doi: 10.1007/s10811-017-1070-1
Reddy, C. R. K., Gupta, M. K., Mantri, V. A., and Jha, B. (2008). Seaweed protoplasts: status, biotechnological perspectives and needs. J. Appl. Phycol. 20, 619–632. doi: 10.1007/s10811-007-9237-9
Reed, D. C., Schroeter, S. C., and Raimondi, P. T. (2004). Spore supply and habitat availability as sources of recruitment limitation in the giant kelp Macrocystis pyrifera (Phaeophyceae). J. Phycol. 40, 275–284. doi: 10.1046/j.1529-8817.2004.03119.x
Robinson, N., Winberg, P., and Kirkendale, L. (2013). Genetic improvement of macroalgae: status to date and needs for the future. J. Appl. Phycol. 25, 703–716. doi: 10.1007/s10811-012-9950-x
Russell, L. K., Hepburn, C. D., Hurd, C. L., and Stuart, M. D. (2008). The expanding range of Undaria pinnatifida in southern New Zealand: distribution, dispersal mechanisms and the invasion of wave-exposed environments. Biol. Invasions 10, 103–115. doi: 10.1007/s10530-007-9113-1
Sangonsuga, Y., and Neushul, M. (1978). Hybridization of Macrocystis (Phaeophyta) with other float-bearing kelps. J. Phycol. 14, 214–224. doi: 10.1111/j.1529-8817.1978.tb02451.x
Santelices, B., and Doty, M. S. (1989). A review of Gracilaria farming. Aquaculture 78, 95–133. doi: 10.1016/j.biotechadv.2014.05.002
Sattler, M. C., Carvalho, C. R., and Clarindo, W. R. (2016). The polyploidy and its key role in plant breeding. Planta 243, 281–296. doi: 10.1007/s00425-015-2450-x
Schiel, D. R., and Foster, M. S. (2006). The population biology of large brown seaweeds: ecological consequences of multiphase life histories in dynamic coastal environments. Ann. Rev. Ecol. Evol. Syst. 37, 343–372. doi: 10.1146/annurev.ecolsys.37.091305.110251
Shan, T., Li, Q., Wang, X., Su, L., and Pang, S. (2019). Assessment of the genetic connectivity between farmed populations on a typical kelp farm and adjacent spontaneous populations of Saccharina japonica (Phaeophyceae, Laminariales) in China. Front. Mar. Sci. 6:494. doi: 10.3389/fmars.2019.00494
Shan, T., Pang, S., Li, J., Li, X., and Su, L. (2015). Construction of a high-density genetic map and mapping of a sex-linked locus for the brown alga Undaria pinnatifida (Phaeophyceae) based on large scale marker development by specific length amplified fragment (SLAF) sequencing. BMC Genomics 16:902. doi: 10.1186/s12864-015-2184-y
Shan, T., Pang, S., Wang, X., Li, J., and Su, L. (2018). Assessment of the genetic connectivity between farmed and wild populations of Undaria pinnatifida (Phaeophyceae) in a representative traditional farming region of China by using newly developed microsatellite markers. J. Appl. Phycol. 30, 2707–2714. doi: 10.1007/s10811-018-1449-7
Shan, T. F., Pang, S. J., and Gao, S. Q. (2013). Novel means for variety breeding and sporeling production in the brown seaweed Undaria pinnatifida (Phaeophyceae): crossing female gametophytes from parthenosporophytes with male gametophyte clones. Phycol. Res. 61, 154–161. doi: 10.1111/pre.12014
Shan, T. F., Pang, S. J., Li, J., and Gao, S. Q. (2016). Breeding of an elite cultivar Haibao No. 1 of Undaria pinnatifida (Phaeophyceae) through gametophyte clone crossing and consecutive selection. J. Appl. Phycol. 28, 2419–2426. doi: 10.1007/s10811-015-0748-5
Silberfeld, T., Leigh, J. W., Verbruggen, H., Cruaud, C., de Reviers, B., and Rousseau, F. (2010). A multi-locus time-calibrated phylogeny of the brown algae (Heterokonta, Ochrophyta, Phaeophyceae): investigating the evolutionary nature of the “brown algal crown radiation”. Mol. Phylogenet. Evol. 56, 659–674. doi: 10.1016/j.ympev.2010.04.020Skjermo
Skjermo, J., Aasen, I. M., Arff, J., Broch, O. J., Carvajal, A. K., and Christie, H. C. (2014). A New Norwegian Bioeconomy Based on Cultivation and Processing of Seaweeds: Opportunities and RandD Needs. Trondheim: SINTEF Report No. A25981.
Smale, D. A., Burrows, M. T., Moore, P., O’Connor, N., and Hawkins, S. J. (2013). Threats and knowledge gaps for ecosystem services provided by kelp forests: a northeast Atlantic perspective. Ecol. Evol. 3, 4016–4038. doi: 10.1002/ece3.774
South, P. M., Floer, O., Forrest, B. M., and Thomsen, M. S. (2017). A review of three decades of research on the invasive kelp Undaria pinnatifida in Australasia: an assessment of its success, impacts and status as one of the world’s worst invaders. Mar. Environ. Res. 131, 243–257. doi: 10.1016/j.marenvres.2017.09.015
Stévant, P., Rebours, C., and Chapman, A. (2017). Seaweed aquaculture in Norway: recent industrial developments and future perspectives. Aquac. Int. 25, 1373–1390. doi: 10.1007/s10499-017-0120-7
Taylor, R., and Fletcher, R. L. (1999). Cryopreservation of eukaryotic algae – a review of methodologies. J. Appl. Phycol. 10, 481–501.
Teagle, H., Hawkins, S. J., Moore, P. J., and Smale, D. A. (2017). The role of kelp species as biogenic habitat formers in coastal marine ecosystems. J. Exp. Mar. Biol. Ecol. 492, 81–98. doi: 10.1016/j.jembe.2017.01.017
Thompson, G. A., and Schiel, D. R. (2012). Resistance and facilitation by native algal communities in the invasion success of Undaria pinnatifida. Mar. Ecol. Prog. Ser. 468, 95–105. doi: 10.3354/meps09995
Thomsen, M. S., Wernberg, T., South, P. M., and Schiel, D. R. (2016). “Non-native seaweeds drive changes in marine coastal communities around the world,” in Seaweed Phylogeography, eds Z.-M. Hu and C. Fraser (Dordrecht: Springer), 147–185. doi: 10.1007/978-94-017-7534-2_6
tom Dieck, I. (1992). North Pacific and North Atlantic digitate Laminaria species (Phaeophyta): hybridization experiments and temperature responses. Phycologia 31, 147–163. doi: 10.2216/i0031-8884-31-2-147.1
tom Dieck, I., and de Oliveira, E. C. (1993). The section Digitatae of the genus Laminaria (Phaeophyta) in the northern and southern Atlantic: crossing experiments and temperature responses. Mar. Biol. 115, 151–160. doi: 10.1007/bf00349397
Valero, M., Guillemin, M.-L., Destombe, C., Jacquemin, B., Gachon, C. M. M., Badis, Y., et al. (2017). Perspectives on domestication research for sustainable seaweed aquaculture. Perspect. Phycol. 4, 33–46. doi: 10.1127/pip/2017/0066
van der Meer, J. P. (1983). The domestication of seaweeds. Bioscience 33, 172–176. doi: 10.2307/1309270
Wang, X., Cheng, Z.-M., Zhi, S., and Xu, F. (2016). Breeding triploid plants: a review. Czech J. Genet. Plant Breed. 52, 41–54. doi: 10.17221/151/2015-CJGPB
Westermeier, R., Patiño, D., and Müller, D. G. (2007). Sexual compatibility and hybrid formation between the giant kelp species Macrocystis pyrifera and M. integrifolia (Laminariales, Phaeophyceae) in Chile. J. Appl. Phycol. 19, 215–221. doi: 10.1007/s10811-006-9126-7
Westermeier, R., Patiño, D., Piel, M. I., Maier, I., and Mueller, D. (2006). A new approach to kelp mariculture in Chile: production of free-floating sporophyte seedlings from gametophyte cultures of Lessonia trabeculata and Macrocystis pyrifera. Aquac. Res. 37, 164–171. doi: 10.1111/j.1365-2109.2005.01414.x
Westermeier, R., Patiño, D. J., Müller, H., and Müller, D. G. (2010). Towards domestication of giant kelp (Macrocystis pyrifera) in Chile: selection of haploid parent genotypes, outbreeding, and heterosis. J. Appl. Phycol. 22, 357–361. doi: 10.1007/s10811-009-9466-1
Williams, S. L., and Smith, J. E. (2007). A global review of the distribution, taxonomy, and impacts of introduced seaweeds. Annu. Rev. Ecol. Evol. Syst. 38, 327–359. doi: 10.1146/annurev.ecolsys.38.091206.095543
Wu, C., Li, D., Liu, H., Peng, G., and Liu, J. (2004). Mass culture of Undaria gametophyte clones and their use in sporeling culture. Hydrobiologia 512, 153–156. doi: 10.1023/b:hydr.0000020321.73728.21
Wu, C. Y., and Lin, G. (1987). Progress in the genetics and breeding of economic seaweeds in China. Hydrobiologia 15, 57–61. doi: 10.1007/bf00046105
Xu, B., Zhang, Q. S., Qu, S. C., Cong, Y. Z., and Tang, X. X. (2009). Introduction of a seedling production method using vegetative gametophytes to the commercial farming of Laminaria in China. J. Appl. Phycol. 21, 171–178. doi: 10.1007/s10811-008-9347-z
Xu, Z., Dapeng, L., Hanhua, H., and Tianwei, T. (2005). Growth promotion of vegetative gametophytes of Undaria pinnatifida by blue light. Biotechnol. Lett. 27, 1467–1475. doi: 10.1007/s10529-005-1313-0
Ye, N., Zhang, X., Miao, M., Fan, X., Zheng, Y., Xu, D., et al. (2015). Saccharina genomes provide novel insight into kelp biology. Nat. Commun. 6:6986. doi: 10.1038/ncomms7986
Zhang, J., Liu, T., Bian, D., Zhang, L., Li, X., Liu, D., et al. (2016). Breeding and genetic stability evaluation of the new Saccharina variety “Ailunwan” with high yield. J. Appl. Phycol. 28, 3413–3421. doi: 10.1007/s10811-016-0810-y
Zhang, J., Liu, T., Feng, R., Liu, C., and Chi, S. (2015). Genetic map construction and quantitative trait locus (QTL) detection of six economic traits using an F2 population of the hybrid from Saccharina longissima and Saccharina japonica. PLoS One 10:e0128588. doi: 10.1371/journal.pone.0128588
Zhang, J., Liu, T., Feng, R., Liu, C., Jin, Y., Jin, Z., et al. (2018). Breeding of the new Saccharina variety “Sanhai” with high-yield. Aquaculture 485, 59–65. doi: 10.1016/j.aquaculture.2017.11.015
Zhang, J., Liu, Y., Yu, D., Song, H. Z., Cui, J. J., and Liu, T. (2011). Study on high temperature-resistant and high-yield Laminaria variety “Rongfu”. J. Appl. Phycol. 23, 165–171. doi: 10.1007/s10811-011-9650-y
Zhang, L.-Q., Liu, D.-C., Zheng, Y.-L., Yan, Z.-H., Dai, S.-D. F., Li, Y.-F., et al. (2010). Frequent occurrence of unreduced gametes in Triticum turgidum–Aegilops tauschii hybrids. Euphytica 172, 285–294. doi: 10.1007/s10681-009-0081-7
Zhang, Q. S., Qu, S. C., Cong, Y. Z., Luo, S. J., and Tang, X. X. (2008). High throughput culture and gametogenesis induction of Laminaria japonica gametophyte clones. J. Appl. Phycol. 20, 205–211. doi: 10.1007/s10811-007-9220-5
Zhang, Q. S., Tang, X. X., Cong, Y. Z., Qu, S. C., Luo, S. J., and Yang, G. P. (2007). Breeding of an elite Laminaria variety 90-1 through inter-specific gametophyte crossing. J. Appl. Phycol. 19, 303–311. doi: 10.1007/s10811-006-9137-4
Zhao, X. B., Pang, S. J., Liu, F., Shan, T. F., Li, J., Gao, S. Q., et al. (2016). Intraspecific crossing of Saccharina japonica using distantly related unialgal gametophytes benefits kelp farming by improving blade quality and productivity at Sanggou Bay, China. J. Appl. Phycol. 28, 449–455. doi: 10.1007/s10811-015-0597-2
Keywords: aquaculture, breeding, genetic improvement, genetic pollution, Laminariales, macroalgae, seaweed, variety
Citation: Goecke F, Klemetsdal G and Ergon Å (2020) Cultivar Development of Kelps for Commercial Cultivation—Past Lessons and Future Prospects. Front. Mar. Sci. 8:110. doi: 10.3389/fmars.2020.00110
Received: 02 December 2019; Accepted: 10 February 2020;
Published: 25 February 2020.
Edited by:
Mercedes González-Wangüemert, University of Algarve, PortugalReviewed by:
Tifeng Shan, Institute of Oceanology (CAS), ChinaKhor Waiho, University of Malaysia Terengganu, Malaysia
Copyright © 2020 Goecke, Klemetsdal and Ergon. This is an open-access article distributed under the terms of the Creative Commons Attribution License (CC BY). The use, distribution or reproduction in other forums is permitted, provided the original author(s) and the copyright owner(s) are credited and that the original publication in this journal is cited, in accordance with accepted academic practice. No use, distribution or reproduction is permitted which does not comply with these terms.
*Correspondence: Åshild Ergon, YXNoaWxkLmVyZ29uQG5tYnUubm8=