- 1Department of Marine Biology, Institute of Marine Sciences and Management, Istanbul University, Istanbul, Turkey
- 2Central Fisheries Research Institute, Yomra, Turkey
- 3Mersea Marine Conservation Consulting, Fethiye, Turkey
The Mediterranean Sea is classified as a “data-poor” region in fisheries due to its low number of assessed stocks given its biodiversity and number of exploited species. In this study, the CMSY method was applied to assess the status and exploitation levels of 54 commercial fish and invertebrate stocks belonging to 34 species fished by Turkish fleets in the Eastern Mediterranean (Levantine) and Black Seas, by using catch data and resilience indices. Most of these marine taxa currently lack formal stock assessments. The CMSY method uses a surplus production model (SPM), based on official catch statistics and an abundance index derived from scientific surveys. The SPM estimates maximum sustainable yield (MSY), fishing mortality (F), biomass (B), fishing mortality to achieve sustainable catches (Fmsy), and the biomass to support sustainable catches (Bmsy). Our results show the estimated biomass values for 94% of the stocks were lower than the required amount to support sustainable fisheries (Bmsy). Of the 54 stocks, 85% of them can be deemed as overfished; two stocks were not subject to overfishing (Sardina pilchardus and Trachurus mediterraneus in the Marmara Sea) while only one stock (Sprattus sprattus in the Black Sea) is healthy and capable of producing MSY. Annual values of the stock status indicators, F/Fmsy and B/Bmsy, had opposing trends in all regions, suggesting higher stock biomasses could only be achieved if fishing mortality is drastically reduced. Recovery times and levels were then explored under four varying F/Fmsy scenarios. Under the best-case scenario (i.e., F = 0.5Fmsy), over 60% of the stocks could be rebuilt by 2032. By contrast, if normal fishing practices continue as usual, all stocks will soon be depleted [if not already] (F = 0.95Fmsy), whose recover may be impossible at later dates. The results of this study are supported by previous regional assessments confirming the overexploitation of Turkish fisheries is driving the near-total collapse of these marine wild fisheries. Hence, the need to urgently rebuild Turkey’s marine fisheries ought to be prioritized to ensure their future viability.
Introduction
Fisheries can effectively be managed if there is sufficient scientific understanding of the current biomasses and trends of fish stocks. However, because most stocks lack robust assessments, practical advice for them, and hence management capabilities, are impaired. The main element of management frameworks in the adoption of adequate multi-annual management plans (MAPs) and fishery standards according to the Marine Stewardship Council (MSC) is stock assessment (Aranda et al., 2019). However, stock assessments are understood to be costly and so they are not usually set as a priority for less-developed nations (Cope and Punt, 2009). Thus, for the majority of stocks, only time-series of landings data exists and some biological information on harvested species, such as their fecundity, length-at-maturity, growth rates (Froese and Pauly, 2019); this dearth of knowledge has compromised the ability to manage them.
The proportion of stocks that are routinely and regularly assessed is greater in the Northeast Atlantic (Cardinale et al., 2013) than in the Mediterranean and Black Seas (Lleonart, 2015; Stergiou et al., 2016). This disparity is mainly due to the multi-species nature of fisheries in the Mediterranean (Lleonart and Maynou, 2003), and the more advanced fisheries management capacities of Northern European countries. Consequently, the Mediterranean and Black Seas are generally classified as “data-poor regions,” stemming from a combination of very few empirically assessed stocks and the little landings and biological data available (Pilling et al., 2008). However, the situation has improved slightly since 2010, as more stocks began to undergo assessments (Colloca et al., 2013), but the quality of these data must still be critically improved upon, especially for the “extremely data-poor” Eastern Mediterranean (Tsikliras et al., 2015).
Data-poor regions require a different approach to stock assessment and fisheries management that deviates from classical age-based models, by incorporating novel techniques designed to produce fisheries reference points when biological data are limited and catch/biomass time series are short or incomplete (Froese et al., 2017). The “Surplus Production Model” (SPM) demands the least amount of input data compared to other models for estimating Maximum Sustainable Yield [MSY] (Winker et al., 2018). Although SPM has its limitations, such as not incorporating the size or age structure of a stock’s population (Wang et al., 2014), the model does provide key stock status indicators (i.e., current biomass and fishing mortality) and related fisheries reference points (Bmsy and Fmsy) enabling the formulation of species’ stock assessments in data-limited regions (Punt and Szuwalski, 2012; Froese et al., 2017). Recent improvements to the SPM such as its implementation of Bayesian inferential statistics (McAllister et al., 2001) now provide a realistic estimation of stock biomass relative to carrying capacity (B/k). Establishing fisheries reference points and stock status indicators (F/Fmsy and B/Bmsy) can promote effective management toward achieving sustainability. The main objective of both the EU Common Fisheries Policy (CFP) and the General Fisheries Commission for the Mediterranean (GFCM) is to manage fish stocks so they attain MSY by 2020, at the latest, to deliver the highest possible long-term catches. Additionally, the EU’s “Marine Strategy Framework Directive” (MSFD, 2008) main criteria for assigning “Good Environmental Status” (GES) for fisheries is that fishing mortality (F) does not exceed the level (Fmsy) producing MSY, Spawning Stock Biomass (SSB) is consistent with the level associated with MSY, and the proportion of adult fish is high enough to ensure stock renewability. Since Turkey is now improving its capacities and initiatives in line with this directive, stock sustainability is indeed a vital short-term goal.
Background on the Development and Changes in the Turkish Fisheries
Turkey is one of the strongest fishing countries in the Mediterranean (Figure 1A), mostly owing to its anchovy catch from the Black Sea (Figure 1B). The main controls currently implemented as management measures in Turkey include the following (Resmî Gazete, 2016, Sayı: 29800 #2016/35): (i) Temporal industrial fishing bans in the Black and Marmara Seas from April 15 to September 1, and a spatial trawling ban in the Marmara Sea and the Bosphorus and Dardanelles Straits (Turkish Strait System-TSS); (ii) Minimum Landing Size (MLS) regulations for most commercial taxa; (iii) Vessel licensing restrictions; (iv) Minimum mesh size regulations; and (v) Protected species (i.e., dolphins [Delphinidae], monk seals [Phocidae], sponges [Porifera], sturgeons [Acipenseridae], grouper [Epinephelinae], and several sharks and rays [Elasmobranchii]). Additionally, catch quotas were previously implemented for the striped Venus clam (Chamelea gallina) in the Black Sea and for the bluefin tuna (Thunnus thynnus) in the Mediterranean. The bluefin tuna quota is still operational, along with a recent regulation for swordfish Xiphias gladius, both of which are mandated by the International Commission for the Conservation of Atlantic Tunas (ICCAT).
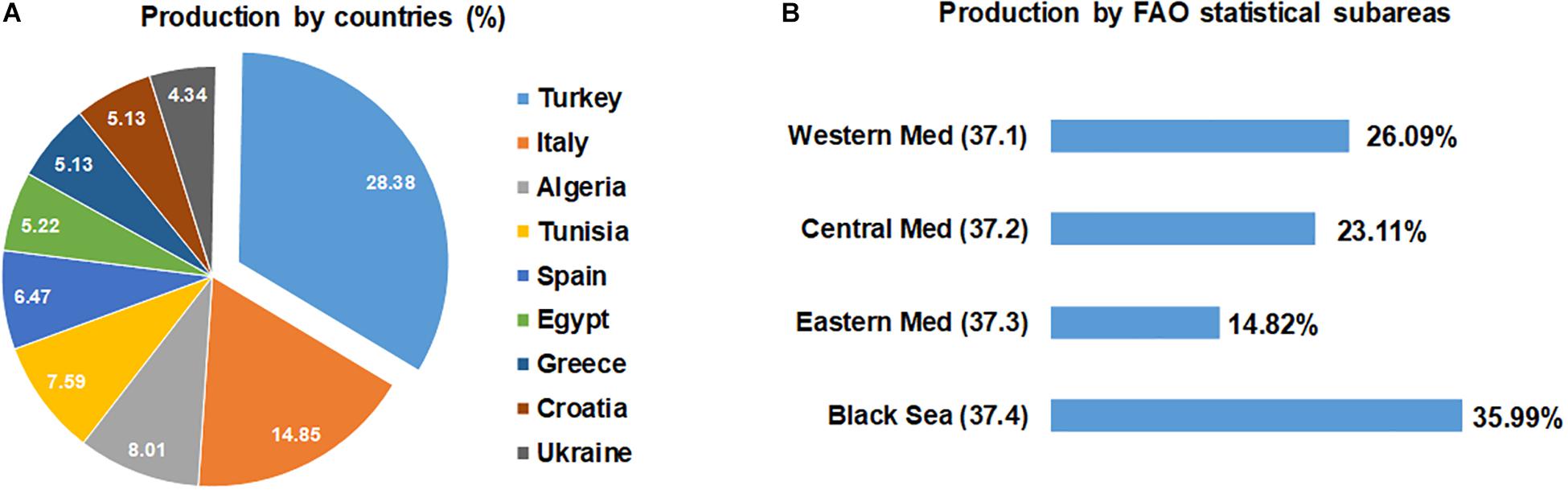
Figure 1. (A) Top ranked fishing nations in the Mediterranean and Black Seas and (B) portion of catches taken from each subregion in 2017 (Food and Agriculture Organization [FAO], 2018).
The concept of “Fishing down marine food webs” (Pauly et al., 1998) is clearly exemplified in Turkey. There, fishers first depleted the most profitable stocks before moving on to the next lesser- valued stocks, until left with smaller and less economically valuable taxa. Prior to the development of industrial fisheries in the 1970s, fishers could catch as much swordfish, bluefin tuna, Atlantic mackerel, Atlantic chub mackerel, horse mackerels, and bluefish as they could carry (Ulman and Pauly, 2016). However, in 1980, the Turkish economy was transformed, from a semi-state controlled one to a free market economy. This new economy sought rapid development, and, to encourage this, provided bank credit, subsidies and custom duty exemptions to fishers to quickly develop this sector, which propelled it toward unplanned and uncontrolled growth fueling too much fishing effort (Knudsen et al., 2010). The large marine pelagic predators were first removed from the Black and Marmara Seas in the early 1970s (Oğuz, 2017) due to their lucrative value. This was followed by the collapse of demersals in the 1980s namely stingrays, elasmobranchs, flatfish, turbot, and red mullet (Knudsen et al., 2010) when bottom trawling developed in the 1980s, backed by state funding (Can, 2013). Following the collapse of most demersal populations in both seas, bottom trawling became non-operational in the mid to late 2000s due to the ensuing demersal fish scarcity from depleted stocks, so many boats switched their main harvesting methods to mid-water trawling for pelagic species.
These drastic declines of elasmobranchs and other large predatory fish should alone be a priority concern for management, because large predators are essential for exerting healthy top–down control ecosystem functioning (Myers and Worm, 2003; Steneck, 2012). The benthic food web in the Black Sea littoral area has been heavily disrupted by the considerable loss of its largest predators (i.e., cartilaginous fish; Squalus acanthias, Raja clavata, Scophthalmus maximus), due both to overfishing and high discards; with these top–down controls gone, a noticeable response (Frid et al., 1999) was a greater abundance of crustaceans (especially Liocarcinus depurator) in the absence of their demersal predators (Zengin et al., 2014). A benthic non-indigenous species (NIS) sea snail native to the Pacific Northwest, the Rapa whelk Rapana venosa, first appeared in the Black Sea in 1946, spreading throughout the basin over the next decade—due to its high abundance and demand in other countries, Turkey has been exporting this species into Asia since the 1980s, in sizeable amounts (Turkish Statistical Institute [TUIK], 2018). It has since become a dominant predatory species in the marine food web (Zengin et al., 2014), in both the Black and Marmara Seas. Other Black Sea top predators include three cetacean species: the Black Sea harbor porpoise (Phocoena phocoena relicta), bottlenose dolphin (Tursiops truncatus), and short-beaked common dolphin (Delphinus delphis), but unfortunately, turbot fisheries using trammel netting commonly results in cetacean by-catch (Birkun and Krivokhizhin, 2011; Tonay, 2016). For this reason, their populations are likely declining, especially that of P. phocoena relicta, the dominant Western Black Sea cetacean (Birkun and Frantzis, 2008).
The Levantine Sea also suffered major predator losses, but not as severely as in the Black and Marmara Seas probably due to its larger size and openness. The marine biodiversity of the Eastern Mediterranean has become more tropical since the opening of the Suez Canal in 1869, and its more recent enlargement in 2015, which serves as a migration corridor for NIS of Indo-Pacific origin (European Environment Agency [EEA], 2012). A NIS is considered invasive when it causes either ecological or economical damage or poses a threat to human health. A few invasive species are problematic for fishers, but none more so than the silver-cheeked toadfish Lagocephalus sceleratus, an extremely toxic pufferfish species that has already threatened human life in the Eastern Mediterranean (Kosker et al., 2016) via its neurotoxin tetrodotoxin (TTX), which is 1000 times more poisonous to humans than cyanide (Lago et al., 2015). This pufferfish has since become a dominant species through much of Turkey’s south, bringing additional stress to the already marginalized small-scale fishers, particularly by damaging fishing nets, consuming captured fish within nets, and swallowing many longline fishing hooks (Ünal and Göncüoğlu, 2012). Another NIS whose abundance is also increasing in Turkey’s southeast is the yellowspotted pufferfish Torquigener flavimaculosus. However, not all NIS are pest species, and some even provide economic benefits to fishers, such as Randall’s threadfin bream Nemipterus randalli, the rabbitfish (Siganus spp.), the brushtooth lizardfish Saurida undosquamis, the goldband goatfish Upeneus moluccensis, the redcoat (squirrelfish) Sargocentron rubrum, and the lionfish (Pterois miles).
Study Aims
Here we present a first comprehensive stock assessment for Turkey’s major and/or important commercial fisheries from the Black Sea, Marmara Sea, and Northeastern Levantine Sea. To do this, we applied the new “CMSY” method (Froese et al., 2017) to 50 years of reported catch data, to better understand the current plight of the stocks and the fishing pressure exerted upon them, to provide management advice and realistic future scenarios aimed at rebuilding these fisheries. The “CMSY” method is a low-cost assessment approach incorporating the “surplus production” stock assessment model, requiring only a long time-series of catch data (Froese et al., 2017). Specifically, this study aimed to: (i) Estimate total current stock biomass (B) and fishing mortality (F) in relation to MSY levels; (ii) Estimate the total potential stock biomass (Bmsy) and the fishing mortality (Fmsy) required to produce MSY; (iii) Calculate the differences between current biomasses and potential biomasses (i.e., recovery ability); and, finally, (iv) Present recovery options for managers by estimating the time needed for stocks’ restoration under different fishing mortality scenarios.
Materials and Methods
The Dataset
A total of 54 commercial fish and invertebrate stocks belonging to 34 species were analyzed for respective stock status by using a 50 years’ time-series of archived national catch data. Turkey began spatially reporting its catches from five distinct marine sub-regions in 1967: Western Black Sea, Eastern Black Sea, Marmara Sea, Aegean Sea, and Levantine Sea (Eastern Mediterranean portion); so this was the initial year used in our study. Underreporting is still serious problem in catch statistics globally. Although the uncertainty levels associated with the Turkish catch data were admittedly higher in the past (50 to 70 years ago), recently this has been somewhat improved upon (see Ulman et al., 2016a,b,c). Nevertheless, national data (from 1967 to 2017) were chosen as the source data to demonstrate how this stock assessment technique could be specifically applied to marine catch data. Catches for the Western and Eastern Black Sea were combined to represent catches from the Black Sea. The Aegean Sea was excluded from this analysis, since its commercial catches are more of an artisanal nature owing to its very narrow continental shelf, and the reporting of industrial catches from there are better represented than small-scale catches (Ulman et al., 2013). Therefore, the Turkish Exclusive Economic Zone (EEZ) in the Black Sea, Marmara Sea and the Levantine Sea constituted the three marine bodies investigated in this study. Stocks were chosen based on either their historical or current commercial influence and/or their hypothesized role in the trophic ecosystem, by relying on expert knowledge. All analyses were done using the R statistical program (v3.6.1; R Core Team, 2014).
Stocks’ Status
The CMSY model (Froese et al., 2017) is an open-source stock assessment model for data-limited stocks in fisheries that produces reference points, such as biomass trends, and MSY, based on only catch and species resilience data. Using a time-series of catches can be a very useful indicator of stock status as declining catches generally represent stock reductions, so long as mortality is not decreasing over time, and migration is not a factor (ICES, 2018). Particularly, CMSY-BSM is a Bayesian model that uses a Markov Chain Monte Carlo approach based on the Schaefer SPM (Schaefer, 1954). Species resilience is defined as the “measure of a species’ ability to absorb changes in variable states, driving influences and parameters, and still persist” (Holling, 1973). Resilience ranges are assigned by combining several population parameters, such as maximum population growth (rmax), von Bertalanffy growth rate (K), fecundity (total egg production), age of maturity (tm), and maximum age (tmax). The maximum population growth rate (rmax) is defined as the “intrinsic rate of population increase, equal to per capita birth minus death rate,” which is closely related to carrying capacity (k), the latter corresponding to when “a population’s per capita growth rate gets diminutively smaller as the population size approaches its maximum limit imposed by the limited resources of the environment” (Rees, 1996). Below is the dynamic formula of the Schaefer model using a Bayesian approach for the best estimate of biomass:
where B is the biomass (metric tons), C is the catch (metric tons), r is the intrinsic rate of population growth, k is the carrying capacity, and t is the time (years).
For a given species, CMSY requires prior information to be specified for resilience and productivity at the beginning and the end of the catch time-series. Priors are the most important part of this model; in particular, the “resilience prior r” (later represented as r) must be estimated by experts for this CMSY analysis. It should be noted that the reliability of stock assessment results is completely dependent upon setting a proper prior range for r. If only catches are known, a prior r is derived from life-history traits and stock assessment records, while a prior range for k can be derived from the maximum catch. Here, prior estimates for r were taken from the “Estimates based on models” section found on summary pages for species in FishBase1 and SeaLifeBase2 (Table 1).
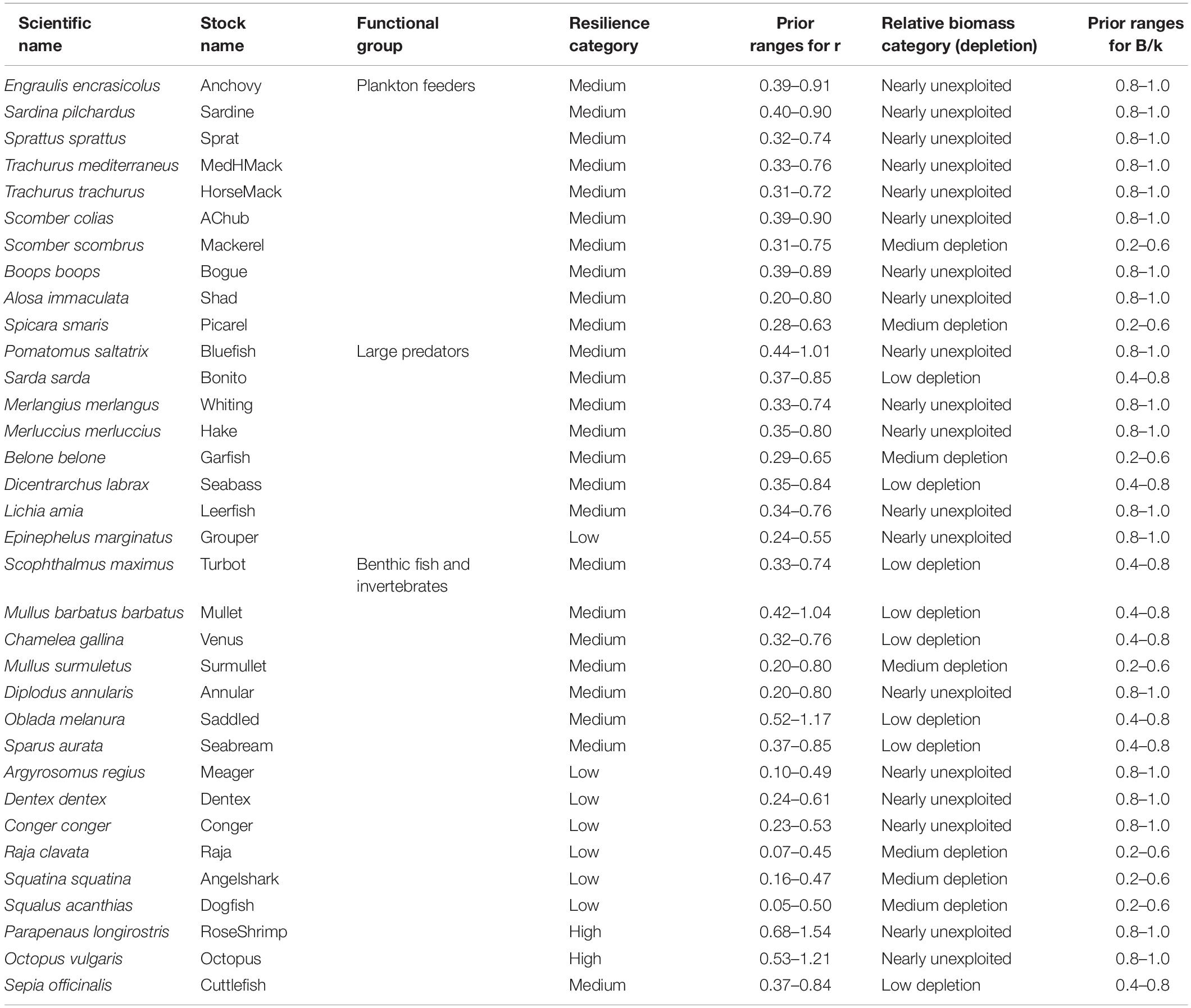
Table 1. Scientific names, functional groups, and resilience categories from FishBase (and from SeaLifeBase for invertebrates) with prior ranges as input parameters for r (intrinsic rate of population), based on classification of resilience and for relative biomass (B/k) depending on the depletion status.
Probable ranges for the maximum intrinsic rate of population increase (r) and for the unexploited population size or carrying capacity (k) are filtered with a Monte Carlo approach, to detect “viable” r–k pairs. Their empirical relation is formulated this way:
where M is the rate of natural mortality, K is the von Bertalanffy growth parameter, tgen is the generation time, and tmax is the maximum age (Froese et al., 2017). The CMSY method incorporates a routine for estimating wide (uniform) priors for k (Froese et al., 2017):
where klow and khigh are the lower and upper bounds of the prior range of k; Cmax is the recorded maximum catch in the time series; rlow and rhigh are respectively the lower and upper bounds of the range of r values that the CMSY method explores.
Prior ranges for Bt/k (beginning and end of catch time-series) were derived from expert knowledge. Using a Monte Carlo approach, all r–k combinations that are compatible with the life history traits (r, M, K), the catches (Ct), and the expert knowledge (Bt/k) were identified. Therefore, we followed generic rules established by Froese et al. (2017), for setting the biomass priors based on general available knowledge of the fisheries. Rules for the initial prior biomass range assumed a high initial biomass (0.5–0.9 k) if the time-series of catch data started before 1960, when most fisheries were recovering after World War II (Pauly et al., 2002; Froese et al., 2017; Supplementary Material), and medium (0.2–0.6 k) biomass after 1960. For the final biomass, its range was set up using the last catch relative to maximum catch. Final biomass range is set to high if this ratio was over 0.7 and set to low if it was lower than 0.3. Since there is no proper stock assessment research or CPUE data for Turkish stocks available for use, an automatic selection of low, medium, or high prior biomass ranges on the simple default rules described above were used for our CMSY analyses.
In the Schaefer model, 0.5 rmax = Fmsy and 0.5 k = Bmsy (Ricker, 1975). Fmsy is the maximum rate of fishing mortality (i.e., the proportion of caught fish) that eventually ensues, usually over a very long time frame, in a population size of Bmsy (the resulting stock biomass from fishing at Fmsy). The outputs of the analyses produce proxies for MSY, key reference points such as fishing mortality that can produce MSY (Fmsy), biomass that can produce MSY (Bmsy), and indicators such as relative stock size (B/Bmsy) and fishing mortality (F/Fmsy) to better understand current stock trends and status. We also present cumulative results for biomass and MSY, and median fishing mortality by main functional groups (benthic fish and invertebrates, small pelagics, benthopelagics, and large predators) according to sea location.
These criteria were used for evaluating stock status: severely depleted (B < 0.2Bmsy), critical condition (B < 0.2Bmsy, F > Fmsy), exploited outside safe biological limits (B < 0.5Bmsy), subject to overfishing (F > Fmsy), recovering (B < Bmsy, F ≤ Fmsy), and healthy (B > Bmsy, F ≤ Fmsy) (Table 2). Overall, 16 stocks were assessed from the Black Sea, 17 stocks from the Marmara Sea, and 21 stocks from the Levantine Sea.
Expected Time to Rebuild Overfished Stocks
In addition to the Fmsy estimation for each stock, recovery options were also considered according to stock biomass (projected for 2018) under four fishing mortality scenarios for 15 year (until 2032) (Table 3). The time needed to rebuild stocks so that they are at Bmsy was calculated using outputs of the CMSY model. Thus, the recovery time needed to reach the biomass level (Bmsy) capable of producing MSY, is a function of depletion and remaining fishing mortality (Fmsy – F), and it was calculated for F equal to 0.5 Fmsy for every stock according to the this formula:
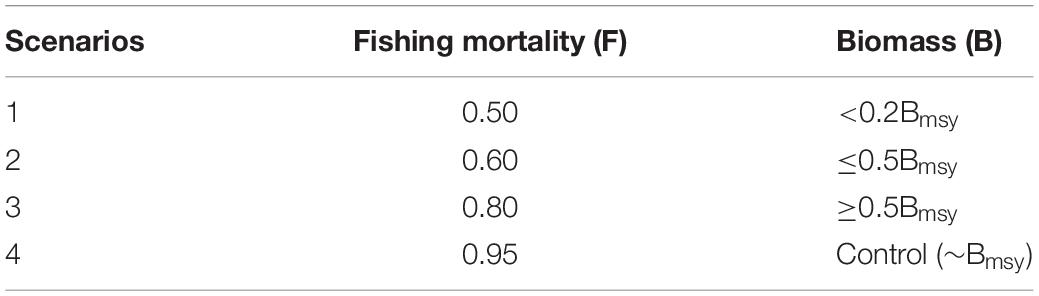
Table 3. Four scenarios using different fishing mortality ratios according to exploitation level of stocks.
For a given stock, its potential catch was calculated as the difference between the current catch and the catch at MSY. The final value was conservatively reduced by 10% to account for stocks that cannot reach MSY at the same time.
The CMSY model output showed that, in 2017, of the 54 assessed stocks, 29 stocks (54%) were in critical condition and/or being severely depleted (B < 0.2Bmsy), yet all are still being fished (Figure 2). Another 17 stocks (31%) were exploited outside safe biological limits (0.2Bmsy < B < 0.5Bmsy), while five stocks (9%) are over 0.5 Bmsy and two stocks (Sardina pilchardus and Trachurus mediterraneus from the Marmara Sea) are not overfished and close to a sustainable level (F ≤ Fmsy and B < Bmsy), with just one stock of the 54 seemingly sustainable (Sprattus sprattus from Black Sea) (Figure 2 and Table 4). Overall, 46 stocks (85%) were overfished. Catches in 2017 were below the maximum sustainable yield (C/MSY < 1) for 52 stocks—the Marmara Sea sardine being very close to MSY—and above the MSY for the remaining two stocks (Atlantic mackerel and striped Venus clam). We provide plotted outputs of the CMSY models for each stock assessment and its associated management information in the Supplementary Material.
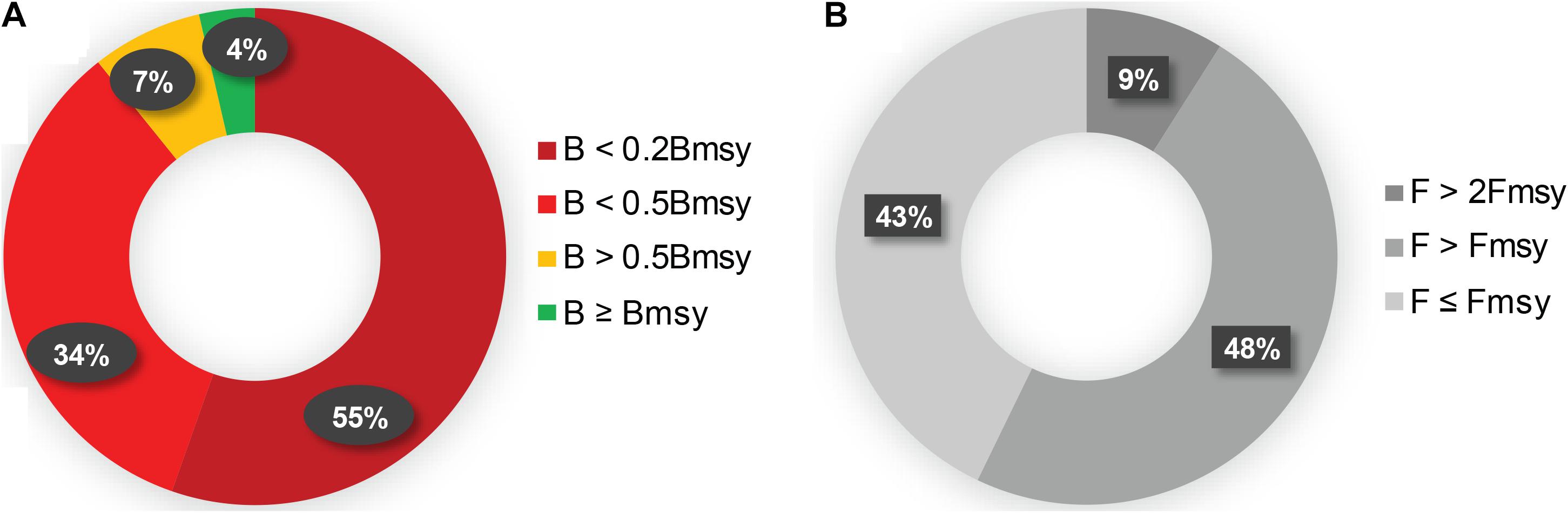
Figure 2. Status of assessed stocks ranked by their (A) current biomass levels relative to different Bmsy levels, for which the dark red area indicates stocks under critical condition and/or severely depleted, the red area the overfished stocks, the yellow area the recovering stocks, and the green area with healthy stocks; and (B) fishing mortality over three different Fmsy levels.
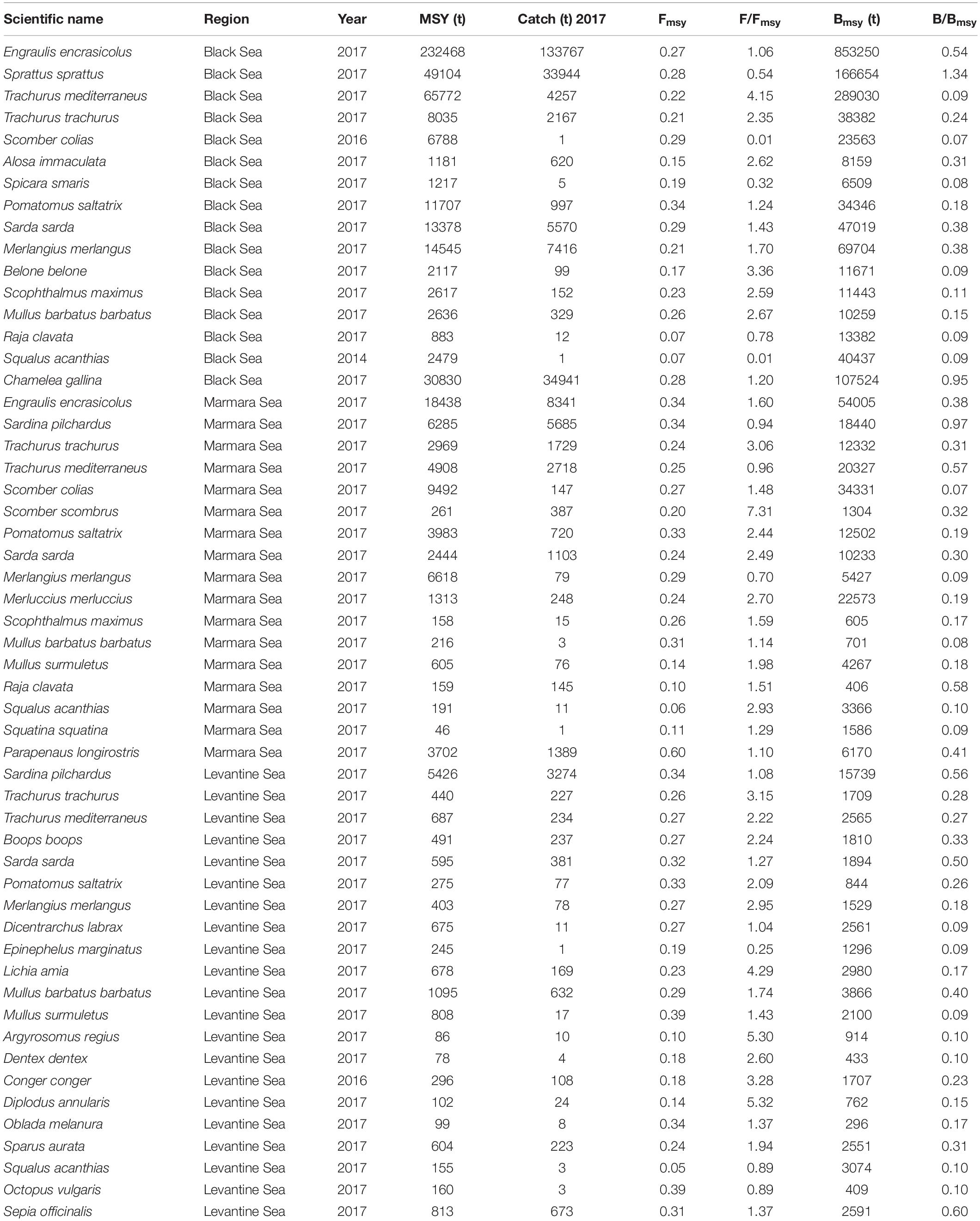
Table 4. CMSY outputs for MSY, Bmsy, Fmsy, and r with stock indicators B/Bmsy and F/Fmsy for stocks in Turkish seas from 2017.
Outputs of CMSY analyses by main functional groups (benthic fish and invertebrates, large predators, pelagic plankton feeders) are presented as summed catches, median biomass relative to the level that can produce the MSY (B/Bmsy), and median fishing mortality to the MSY level (F/Fmsy) for stocks according to seas (Black Sea, Marmara Sea, Levantine Sea) (Figure 3). Lastly, fishing mortality (F/Fmsy) and biomass state (B/Bmsy), respectively, are presented for each assessed stock by Turkish sea (Figure 4).
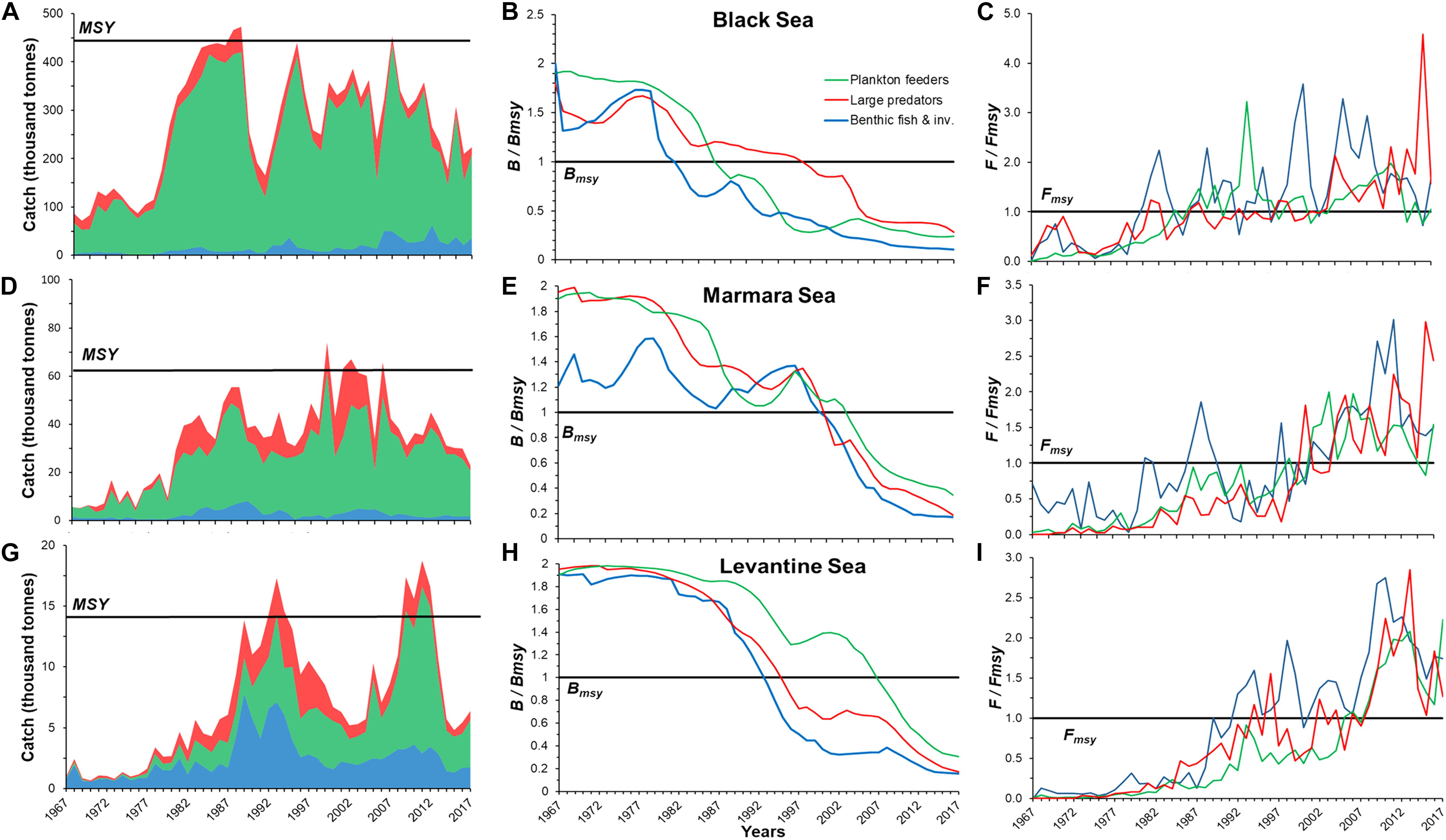
Figure 3. Time-series of stock status by functional group (red: large predators, green: pelagic plankton feeders, blue: benthic species) for the Black Sea, Marmara Sea, and Levantine Sea, from 1967 to 2017. Summed catch (A,D,G), median biomass relative to the level that can produce the MSY (B/Bmsy; B,E,H), and median fishing mortality (C,F,I) relative to the MSY level (F/Fmsy) is shown respectively for 17 stocks in the Black Sea (A–C); 17 stocks in the Marmara Sea (D–F), and 21 stocks in the Levantine Sea (G–I). The black lines indicate the summed MSY, sustainable stock biomass at Bmsy level, and fishing mortality at Fmsy level, respectively, going from left to right.
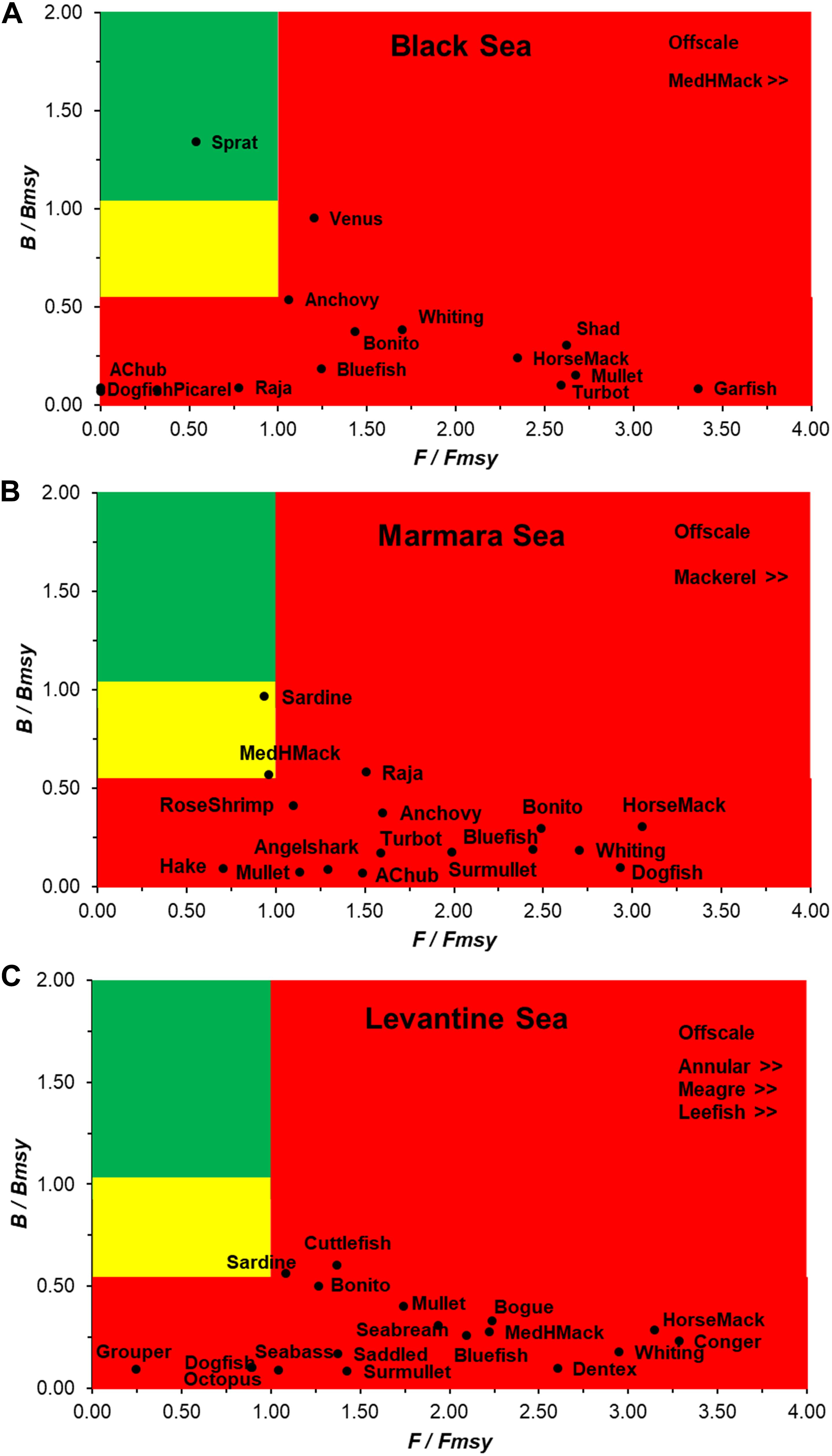
Figure 4. Pressure plots showing fishing mortality (F/Fmsy) and relative stock size (B/Bmsy) for the assessed stocks in the (A) Black Sea, (B) Marmara Sea, and (C) Levantine Sea for 2017. The red area indicates overfished stocks (including those outside of safe biological limits, in critical condition, and severely depleted). The yellow area indicates recovering stocks and the green area shows healthy stocks. Scientific names of the fish were presented in Table 1.
Black Sea
The 16 Black Sea stocks analyzed consisted of 13 teleosts, two elasmobranches, and one invertebrate. Functionally, they were grouped as follows: seven plankton feeders, four large predators, and five benthic fish and invertebrates. Biomasses of all these stocks have gradually decreased since the 1980s, and, from about 1990, all stocks can be deemed unhealthy, with the exception of sprat that only became a target species in the late 1980s. Beginning in the 1980s, fishing mortality for all 16 stocks exceeded the level of mortality needed to produce MSY, and fishing mortality continued to increase over time. In 2017, 15 stocks (93.8%) were subject to ongoing overfishing of which nine (55%) were fished outside their safe biological limits (Figures 3A–C, 4A and Table 4).
Marmara Sea
Of the 17 stocks analyzed for the Sea of Marmara, 13 were teleosts, plus three elasmobranches and one invertebrate. Their functional grouping was as follows: six plankton feeders, four large predators, and seven benthic fish and invertebrates. Two stocks (sardine and Mediterranean horse mackerel) still retain a moderate stock status, but because each is being fished at maximum mortality (F = Fmsy), this leaves little prey for their predators. Ten stocks were subject to ongoing overfishing and 14 stocks were fished outside safe biological limits (85%), of which nine are in critical condition. After 2005, fishing mortality was at a much higher, dangerous level than what is needed to produce MSY, and biomass values were critically low for all stocks, as confirmed by declining catches (Figures 3D–F, 4B and Table 4).
Levantine Sea
For the Levantine Sea, the 21 stocks analyzed were composed of 18 teleosts, one elasmobranch, and two invertebrates. The dominant functional group was benthic fish and invertebrates with 11 stocks, followed by six large predators and four plankton feeders. Of the 17 stocks (90%) exploited outside safe biological limits, 10 of them are in critical condition. Since the mid-1990s, biomasses and catches for these three functional groups continued to decrease while fishing mortality increased (Figures 3G–I, 4C and Table 4).
Future Scenarios
Future fishing scenarios were projected for four different fishing mortality reduction possibilities (50, 40, 20, and 5% of the stocks’ respective Fmsy), according to the estimated biomass of the latest year that stocks could reach the biomass necessary to produce MSY (Figure 5A) and by reduction in catch necessary for catches to attain an MSY (Figure 5B). For the stocks capable of recovery, these scenarios show that if fishing mortality was reduced to 0.5Fmsy, 40% of the 54 stocks could reach the Bmsy level within 10 years, and 60% of them within 15 years. For the 0.6Fmsy scenario, half of the stocks can fully recover by 2032. If fishing mortality is set to 0.8Fmsy, within the next 15 years, 30% of stocks can reach the Bmsy level, for which catch values come closer to MSY levels than under any other options examined. However, a lowering of fishing mortality by only 5% (i.e., the 0.95 Fmsy values) would leave stocks overfished and unable to rebuild, while their catches remain similar to present values.
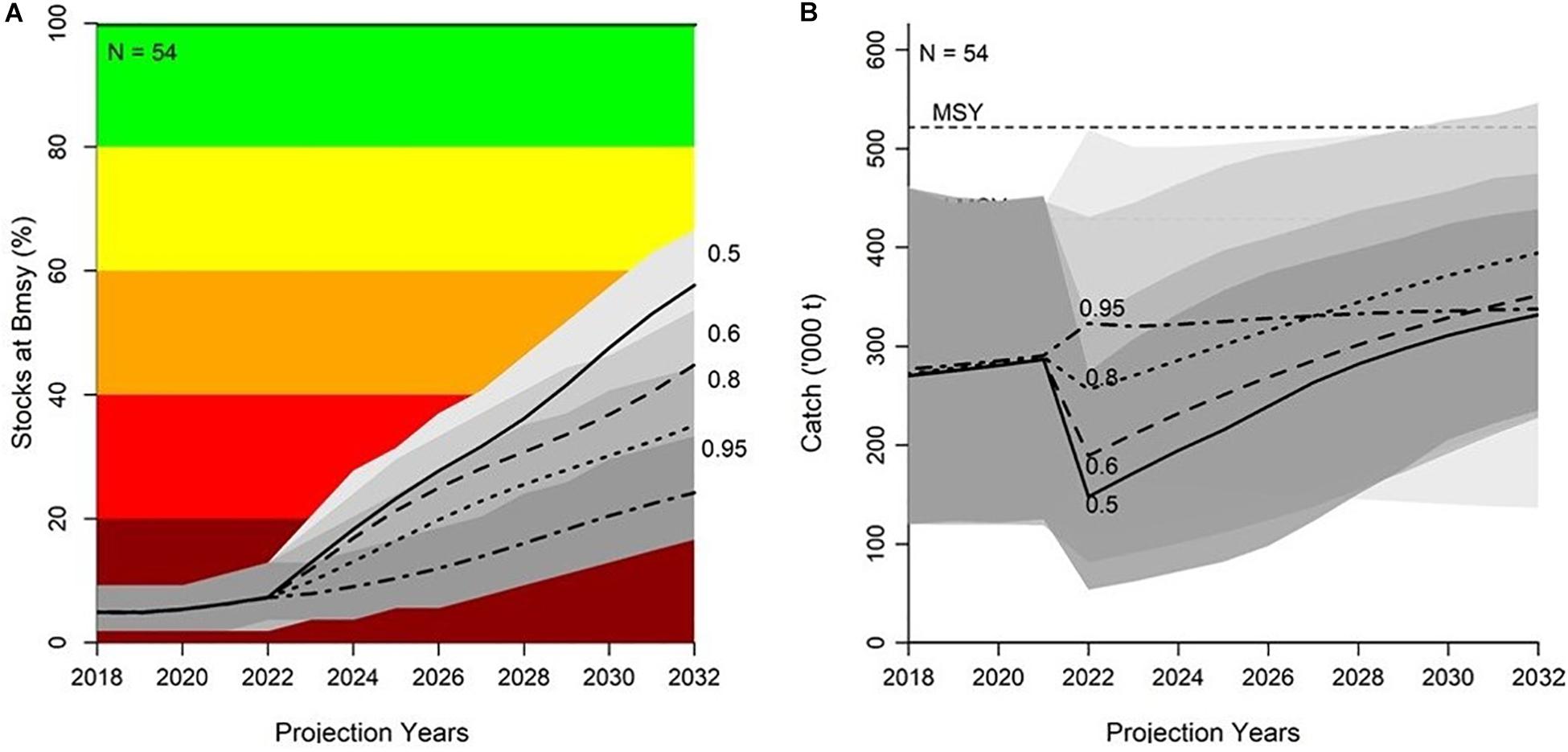
Figure 5. Future scenarios for all of Turkeys’ 54 commercial stocks assessed in this study, showing the (A) predicted percentage of stocks reaching the Bmsy level, and (B) predicted cumulative catch under four different fishing mortality scenarios, ranging from 0.5 to 0.95 Fmsy. Gray areas indicate the approximate 95% CI for each scenario.
Discussion
This first large-scale stock assessment of Turkish marine fisheries has revealed the impacts on them arising from overexploitation. Fishing mortality has resulted in unsustainable and rapidly declining stocks, which will inevitably cause further stock collapses without prompt management interventions. Of the 54 stocks assessed here, 85% are clearly overexploited (B < 0.5Bmsy), with only Black Sea sprat sustainably fished, and Marmara Sea Mediterranean horse mackerel and sardine near overfishing levels. It must be stressed that sprat is not consumed as a fish item but instead processed into fishmeal to support aquaculture and used in fish oil products (Ceyhan and Emir, 2015). Wild fish are a cheaper, much healthier alternative to farmed fish not requiring the additional culturing input costs, such as feed and placement, and so they should be prioritized over aquaculture fisheries. For the majority of the Turkish commercial fisheries, their stocks need to rebuild to levels near to or equal to their respective MSY. Our investigation of future scenarios shows that rebuilding the fisheries is indeed possible and it can be achieved through a reduction of effort and mortality to levels that allow stocks to reach their MSY (Merino et al., 2012; Froese et al., 2018).
Our results confirm other similar studies conducted in the Mediterranean and the Black Seas. That body of work shows that declines in catches and stock biomasses are a general theme in the region (Merino et al., 2015; Tsikliras et al., 2015; GFCM, 2016; Guillen et al., 2016; Scientific Technical Economic Committee for Fisheries [STECF], 2017; Food and Agriculture Organization [FAO], 2018), with the consensus being that stocks are exploited outside of safe biological limits (Osio et al., 2015). Further, after analyzing all completed stock assessments for the Mediterranean, Colloca et al. (2013) concluded that over 90% of those stocks are overexploited.
The future scenario outputs presented here suggest that, to rebuild Turkey’s fisheries, a 50% reduction in mortality is the fastest way, in that over half of the 54 stocks could recover within 15 years. A 40% (0.6Fmsy) reduction would support the full recovery of half of the overexploited stocks by the year 2032. However, a 20% reduction enables less than half of the stocks to recover, but it would increase catches and revenue within 15 years. Conversely, if fishing continues as normal (F = 0.95Fmsy), stocks may not be able to recover later. Firstly, a clear management objective should be identified for which path to pursue rebuilding these stocks. In addition to mortality reductions, regulations on size selectivity are also needed (Colloca et al., 2013). Stocks currently at critical biomass levels may have much slower recovery rates (Neubauer et al., 2013), since a recovery will also depend on life-history strategies and trophic interactions between species (Hutchings and Reynolds, 2004; Audzijonyte and Kuparinen, 2016), especially for slow-growing and less-fecund species (Hutchings, 2000). Across the Mediterranean, young and juvenile fish, mostly ages 1 and 2 years, comprise the dominant portion of catch compositions because of low size selectivity by fishing gears (Colloca et al., 2013). To help improve the standing stock biomass, size regulation for the conservation of large adult females (Birkeland and Dayton, 2005; Froese et al., 2016) and juveniles, fishing restriction on spawning seasons and ensuring protection of nursery habitats should be used as additional measures to improve recruitment opportunities (Caddy, 2015). In Turkey, most commercial taxa (68% according to the 2016–2020 regulations) already have minimum landing size (MLS) regulations, and strengthening the effectiveness of such MLS measures would be quite easy, namely by improving awareness, controls, and fines (Froese et al., 2018).
Regulating fishing mortality to MSY levels is a true investment for the ecosystem and economy (Sumaila et al., 2012); additionally, species-specific MLS regulations must be effective to support the rebuilding of stocks (Froese et al., 2018). Beddington et al. (2007) noted that the definition of successful management is a combination of biological, economic, social, and political objectives. Aside from the obvious ecological benefits regarding the structure and function of marine ecosystems (Murawski, 2000), the economic benefits of exploiting the Mediterranean fish and invertebrate stocks at or near MSY within an ‘Ecosystem Approach to Fisheries’ (EAF) framework—proper fishing selectivity, restrictions on fishing gear, fishing season, and fishing areas—are enormous (Sumaila et al., 2012; Colloca et al., 2013). These would guarantee revenues and long-term profitability (Ye et al., 2012; Sumaila et al., 2016), with a view to job security as the long-term goal (Beddington et al., 2007) for the sector. Rebuilding the global fisheries is a costly process, estimated at ca. $200 billion, because it may include subsidies, buyback programs, and alternative employment training for fishers, but once stocks reach their MSY levels, profits for the global fishing fleet could increase by ca. $50 billion per year (Sumaila et al., 2016).
Challenges in Assessing Stocks
While we agree that MSY is a less sophisticated model than, for example, the yield-per-recruit model of Beverton and Holt (1957), the MSY concept was nevertheless chosen as the framework for fisheries management by the CFP. Accordingly, we relied on it to make a first evaluation in Turkey for its future fisheries within the framework of data-limited stocks. Note that our results actually confirm several MSY criticisms: while most managers still believe that fishing at F = Fmsy is the best option, our results demonstrate that fishing at that level provides the least desirable outcome. Thus, here we proposed fisheries reference points and indicators derived from the SPM for the MSY concept, which provides assessments for highly fluctuating and high-biomass small pelagics, and also for mixed-fisheries in the Mediterranean, which is a pertinent issue due to their biological and ecological traits. We acknowledge there are limits to production models for providing realistic results if high variability is present in stock productivity or catchability have occurred during the time-series under analysis. As a species-specific example, the disappearance of European hake at the end of the 1990s, due to overfishing, is associated with competition from alien species (mainly lizardfish) and changes in hydrographic conditions in the Levantine Sea (Gücü and Bingel, 2011). As a very unique environment, the Black Sea is an important example of a severe marine ecosystem regime shift, initiated by overfishing that started in the 1950s with the removal of top predators, continued with trophic cascades and eutrophication until the 1980s, followed by an alien ctenophore Mnemiopsis leidyi invasion in the 1990s (Daskalov et al., 2007). The Black Sea anchovy fishery is regarded as a very good example of a fishery whose catch is greatly affected by high volatility (Gücü et al., 2017; Libralato et al., 2019). It should also be stressed that fluctuations of small pelagics such as anchovy are more likely to be heavily influenced by climatic variability than by exploitation per se (Salihoğlu et al., 2017; Tsikliras et al., 2018). Despite the current lack of operational state indicators for understanding pelagic fish assemblages, the MSFD clearly requires considering the state of the biotic community, the environmental status of pelagic habitats, and the functioning of pelagic components of the food web (Shephard et al., 2014). Another important point: the multi-fleet, multi-species (MF-MS) characteristics of demersal fisheries in the Mediterranean present challenges to achieving MSY for single species under the EAF (Beddington et al., 2007). For example, one species can be targeted by one gear type yet be considered as bycatch by others; or achieving an objective for one species may preclude attaining the objective for another. The fact remains that there is intense competition for resources by nations, sectors, and multi-fleets in the Mediterranean. Colloca et al. (2013) have stated that sudden changes in fishing selectivity and use of Fmsy can be difficult. Hence, the MSY objective alone is insufficient for rebuilding without implementing other supporting measures, such as increasing the size of first capture and better incorporation of the ‘Ecosystem Approach to Fisheries.’
Conclusion
Following from this first assessment of 54 data-poor fish and invertebrate stocks exploited by Turkish fleets, we warn that if current levels of overexploitation continue unabated, only the sprat stock will remain a viable fishery in the near future. If swift action is not taken soon, many of these overexploited stocks may become too depleted to ever recover. The Turkish fisheries are plagued with excessively high fishing mortality that depletes standing stocks far below the EU and GFCM targets for sustainable fisheries and Good Environmental Status. Based on these results, to rebuild most of the remaining commercial stocks within 15 years, we suggest that fishing mortality must first be reduced to the Fmsy level, accompanied by the second proposed 40%-effort reduction as the best option. Concerning the other proposed options, the 20%-effort reduction would maximize catches over a much longer time frame, and the 50%-effort reduction would achieve sustainability goals in a minimal time frame. But the reduction of fishing mortality should be complemented with other effective management measures, especially improving MLS effectiveness and incorporation of the EAF. These mortality reduction recommendations may appear severe, but the ongoing drastic decline of marine fisheries is not a trivial matter, justifying the necessity of radical policy and intervention.
We are likely nearing the last opportunity to rebuild the Turkish marine fisheries, considering how many stocks of them are in critical condition, but many fishers will require assistance in the transition out of this sector into another for employment. It also must be stressed that the future scenario predictions presented here are likely overly optimistic considering the other anthropogenic stressors involved, such as the use of destructive fishing techniques (i.e., bottom trawling), by-catch, eutrophication, pollution, invasive species, habitat loss, sea warming, and heavy urbanization, none of which were accounted for in our modeled scenarios. Now that the current and future alarming status of Turkey’s marine fisheries have been clearly presented, this opportunity should be seized upon to rebuild them for long-term viability, so that wild fish persist into the foreseeable future, valued as protein, biodiversity, biotic resistance to stressors, and an integral part of Turkish culture.
Data Availability Statement
All datasets generated for this study are included in the article/Supplementary Material.
Author Contributions
ND, MZ, and AU collected and organized the data and wrote the manuscript. ND analyzed the data.
Conflict of Interest
The authors declare that the research was conducted in the absence of any commercial or financial relationships that could be construed as a potential conflict of interest.
Acknowledgments
ND appreciates methodological advice provided by Rainer Froese.
Supplementary Material
The Supplementary Material for this article can be found online at: https://www.frontiersin.org/articles/10.3389/fmars.2020.00103/full#supplementary-material
Footnotes
References
Aranda, M., Ulrich, C., Le Gallic, B., Borges, L., Metz, S., Prellezo, R., et al. (2019). Researchfor PECH Committee – EU Fisheries Policy – Latest Developments and Future Challenges. Brussels: European Parliament.
Audzijonyte, A., and Kuparinen, A. (2016). The role of life histories and trophic interactions in population recovery. Conserv. Biol. 30, 734–743. doi: 10.1111/cobi.12651
Beddington, J. R., Agnew, D. J., and Clark, C. W. (2007). Current problems in the management of marine fisheries. Science 316, 1713–1716. doi: 10.1126/science.1137362
Beverton, R. J. H., and Holt, S. J. (1957). On the Dynamics of Exploited Fish Populations. London: FAO.
Birkeland, C., and Dayton, P. K. (2005). The importance in fishery management of leaving the big ones. Trends Ecol. Evol. 20, 356–358. doi: 10.1016/j.tree.2005.03.015
Birkun, A. Jr., and Frantzis, A. (2008). Phocoena phocoena ssp.Relicta. In: IUCN 2012. IUCN Red List of Threatened Species. Version 2012.2.
Birkun, A. Jr., and Krivokhizhin, S. (2011). Cetacean by-Catch Levels in the Northern Black Sea: Results of Onboard Monitoring Programme. Report of the 2nd Transversal Working Group on by-catch, Antalya, Turkey, 7–9 December 2011. Roma, RM: General Fisheries Commission for the Mediterranean).
Caddy, J. F. (2015). Criteria for sustainable fisheries on juveniles illustrated for Mediterranean hake: control the juvenile harvest, and safeguard spawning refugia to rebuild population fecundity. Sci. Mar. 79, 287–299. doi: 10.3989/scimar.04230.06A
Cardinale, M., Dorner, H., Abella, A., Andersen, J. L., Casey, J., Doring, R., et al. (2013). Rebuilding EU fish stocks and fisheries, a process under way? Mar. Policy 39, 43–52. doi: 10.1016/j.marpol.2012.10.002
Ceyhan, V., and Emir, M. (2015). Structural and economic analysis of Turkish fishmeal and fish oil industry. Turk. J. Fish. Aquat. Sc. 15, 835–844.
Colloca, F., Cardinale, M., Maynou, F., Giannoulaki, M., Scarcella, G., Jenko, K., et al. (2013). Rebuilding Mediterranean fisheries: a new paradigm for ecological sustainability. Fish Fish. 14, 89–109. doi: 10.1111/j.1467-2979.2011.00453.x
Cope, J. M., and Punt, A. E. (2009). Length-based reference points for data-limited situations: applications and restrictions. Mar. Coast. Fish. 1, 169–186. doi: 10.1577/C08-025.1
Daskalov, G. M., Grishin, A. N., Rodionov, S., and Mihneva, V. (2007). Trophic cascades triggered by overfishing reveal possible mechanisms of ecosystem regime shifts. Proc. Natl. Acad. Sci. U.S.A. 104, 10518–10523. doi: 10.1073/pnas.0701100104
European Environment Agency [EEA] (2012). The Impacts Of Invasive Alien Species in Europe. Technical Report (16). Luxembourg: EEA.
Food and Agriculture Organization [FAO] (2018). The State of Mediterranean, and Black Sea Fisheries. Rome: FAO.
Frid, C. L. J., Hansson, S., Ragnarsson, S. A., Rijnsdorp, A., and Steingrimsson, S. A. (1999). Changing levels of predation on benthos as a result of exploitation of fish populations. Ambio 28, 578–582.
Froese, R., Coro, G., Kleisner, K., and Demirel, N. (2016). Revisiting safe biological limits in fisheries. Fish Fish. 17, 193–209. doi: 10.1111/faf.12102
Froese, R., Demirel, N., Coro, G., Kleisner, K. M., and Winker, H. (2017). Estimating fisheries reference points from catch and resilience. Fish Fish. 18, 506–526. doi: 10.1111/faf.12190
Froese, R., Winker, H., Coro, G., Demirel, N., Tsikliras, A., Dimarchopoulou, D., et al. (2018). Status and rebuilding European Fisheries. Mar. Policy 93, 159–170. doi: 10.1016/j.marpol.2018.04.018
Gücü, A. C., Sakınan, S., Ok, M., Dağtekin, M., Genç, Y., Ak, O., et al. (2017). On Black Sea anchovy and its fishery. Rev. Fish. Sci. Aquac. 25, 230–244. doi: 10.1080/23308249.2016.1276152
Gücü, C., and Bingel, F. (2011). Hake, Merluccius merluccius L., in the northeastern Mediterranean Sea: a case of disappearance. J. Appl. Ichthyol. 27, 1001–1012. doi: 10.1111/j.1439-0426.2011.01765.x
Guillen, J., Santos, A. C., Carpenter, G., Carvalho, N., Casey, J., Lleonart, J., et al. (2016). Sustainability now or later? Estimating the benefits of pathways to maximum sustainable yield for EU Northeast Atlantic fisheries. Mar. Policy 72, 40–47. doi: 10.1016/j.marpol.2016.06.015
Holling, C. S. (1973). Resilience and stability of ecological systems. Annu. Rev. Ecol. Syst. 4, 1–23. doi: 10.1146/annurev.es.04.110173.000245
Hutchings, J. A. (2000). Collapse and recovery of marine fishes. Nature 406, 882–885. doi: 10.1038/35022565
Hutchings, J. A., and Reynolds, J. D. (2004). Marine fish population collapses: consequences for recovery and extinction risk. Bioscience 54, 297–309.
Knudsen, S., Zengin, M., and Koçak, M. H. (2010). Identifying drivers for fishing pressure. A multidisciplinary study of trawl and sea snail fisheries in Samsun, Black Sea coast of Turkey, Ocean. Coast. Manage 53, 252–269. doi: 10.1016/j.ocecoaman.2010.04.008
Kosker, A., Özoğul, F., Durmuş, M., Uçar, Y., Ayas, D., Regenstein, J., et al. (2016). Tetrodotoxin levels in pufferfish (Lagocephalus sceleratus) caught in the Northeastern Mediterranean Sea. Food. Chem. 210, 332–337. doi: 10.1016/j.foodchem.2016.04.122
Lago, J., Rodríguez, L. P., Blanco, L., Vieites, J. M., and Cabado, A. G. (2015). Tetrodotoxin, an extremely potent marine neurotoxin: distribution, toxicity, origin and therapeutical uses. Mar. Drugs 13, 6384–6406. doi: 10.3390/md13106384
Libralato, S., Colloca, F., Gücü, A. C., Maravelias, C. D., Solidoro, C., and Villasante, S., et al. (eds) (2019). Challenges and Opportunities for the EU Common Fisheries Policy Application in the Mediterranean and Black Sea. Lausanne: Frontiers Media, doi: 10.3389/978-2-88945-684-0
Lleonart, J. (2015). Mediterranean fisheries. stocks, assessments and exploitation status. IEMED Mediterranean yearbook 2015. Strateg. Sec. Econ. Territ. 2015, 276–281.
Lleonart, J., and Maynou, F. (2003). Fish stock assessments in the Mediterranean: state of the art. Sci. Mar. 67, 37–49. doi: 10.3989/scimar.2003.67s137
McAllister, M. K., Pikitch, E. K., and Babcock, E. A. (2001). Using demographic methods to construct Bayesian priors for the intrinsic rate of increase in the Schaefer model andimplications for stock rebuilding. Can. J. Fish. Aquat. Sci. 58, 1871–1890. doi: 10.1139/f01-114
Merino, G., Barange, M., Blanchard, J. L., Harle, J., Holmes, R., Allen, I., et al. (2012). Can marine fisheries and aquaculture meet fish demand from a growing human population in a changing climate? Glob. Environ. Chang. 22, 795–806. doi: 10.1016/j.gloenvcha.2012.03.003
Merino, G., Quetglas, A., Maynou, F., Garau, A., Arrizabalaga, H., Murua, H., et al. (2015). Improving the performance of a Mediterranean demersal fishery toward economic objectives beyond MSY. Fish. Res. 161, 131–144. doi: 10.1016/j.fishres.2014.06.010
MSFD (2008). European Parliament and Council, Directive 2008/56/EC of the European Parliament and of the Council of 17 June 2008 Establishing a Framework Community Action in the Field of Marine Environmental Policy. Panama City, FL: Marine Strategy Framework Directive.
Murawski, S. A. (2000). Definitions of overfishing from an ecosystem perspective. ICES J. Mar. Sci. 57, 649–658. doi: 10.1006/jmsc.2000.0738
Myers, R. A. M., and Worm, B. (2003). Rapid worldwide depletion of predatory fish communities. Nature 423, 280–283. doi: 10.1038/nature01610
Neubauer, P., Jensen, O. P., Hutchings, J. A., and Baum, J. K. (2013). Resilience and recovery of overexploited marine populations. Science 340, 347–349. doi: 10.1126/science.1230441
Oğuz, T. (2017). Controls of multiple stressors on the Black Sea fishery. Front. Mar. Sci. 4:110. doi: 10.3389/fmars.2017.00110
Osio, G. C., Orio, A., and Millar, C. P. (2015). Assessing the vulnerability of Mediterranean demersal stocks and predicting exploitation status of un-assessed stocks. Fish. Res. 171, 110–121. doi: 10.1016/j.fishres.2015.02.005
Pauly, D., Christensen, V., Dalsgaard, J., Froese, R., and Torres, F. Jr. (1998). Fishing down marine food webs. Science 279, 860–863. doi: 10.1126/science.279.5352.860
Pauly, D., Christensen, V., Guénette, S., Pitcher, T. J., Sumaila, U. R., Walters, C. J., et al. (2002). Towards sustainability in world fisheries. Nature 418, 689–695. doi: 10.1038/nature01017
Pilling, G. M., Apostolaki, P., Failler, P., Floros, C., Large, P. A., Morales-Nin, B., et al. (2008). “Assessment and management of data-poor fisheries,” in Advances in Fisheries Science: 50 Years on From Beverton and Holt, eds A. Payne, J. Cotter, and T. Potter (Hoboken, NJ: Blackwell Publishing), 280–305. doi: 10.1002/9781444302653.ch12
Punt, A. E., and Szuwalski, C. (2012). How well can FMSY and BMSY be estimated using empirical measures of surplus production? Fish. Res. 134–136, 113–124. doi: 10.1016/j.fishres.2012.08.014
R Core Team (2014). R: A Language and Environment for Statistical Computing. Vienna: R Foundation for Statistical Computing.
Rees, W. E. (1996). Revisiting carrying capacity: area-based indicators of sustainability. Popul. Environ. 17, 195–215. doi: 10.1007/bf02208489
Ricker, W. E. (1975). Computation and interpretation of biological statistics of fish populations. Bull. Fish. Res. Board Can. 191, 1–382.
Salihoğlu, B., Arkın, S. S., Akoğlu, E., and Fach, B. A. (2017). Evolution of future Black Sea fish stocks under changing environmental and climatic conditions. Front. Mar. Sci. 4:339. doi: 10.3389/fmars.2017.00339
Schaefer, M. (1954). Some aspects of the dynamics of populations important to the management of the commercial marine fisheries. Bull. Inter. Am. Trop. Tuna Comm. 1, 27–56. doi: 10.1016/S0065-2881(09)56003-8
Scientific Technical Economic Committee for Fisheries [STECF] (2017). Assessment of Black Sea Stocks (STECF 17-. (14)). Luxembourg: Publications Office of the European Union.
Shephard, S., Rindorf, A., Dickey-Collas, M., Hintzen, N. T., Farnsworth, K., and Reid, D. G. (2014). Assessing the state of pelagic fish communities within an ecosystem approach and the European MARINE STRATEGY FRAMEWORK DIREctive. ICES J. Mar. Sci. 71, 1572–1585. doi: 10.1093/icesjms/fsu005
Steneck, R. S. (2012). Apex predators and trophic cascades in large marine ecosystems: learning from serendipity. Proc. Natl. Acad. Sci. U.S.A. 109, 7953–7954. doi: 10.1073/pnas.1205591109
Stergiou, K. I., Somarakis, S., Triantafyllou, G., Tsiaras, K. P., Giannoulaki, M., Petihakis, G., et al. (2016). Trends in productivity and biomass yields in the Mediterranean large marine ecosystem during climate change. Environ. Dev. 17(Suppl. 1), 57–74. doi: 10.1016/j.envdev.2015.09.001
Sumaila, U. R., Cheung, W., Dyck, A., Gueye, K., Huang, L., Lam, V., et al. (2012). Benefits of rebuilding global marine fisheries outweigh costs. PLoS One 7:e40542. doi: 10.1371/journal.pone.0040542
Sumaila, U. R., Lam, V., Le Manach, F., Swartz, W., and Pauly, D. (2016). Global fisheries subsidies: an updated estimate. Mar. Policy 69, 189–193. doi: 10.1016/j.dib.2019.104706
Resmî Gazete, T. C. (2016). 4/1 Numaralı Ticari Amaçlı Su Ürünleri Avcılığının Düzenlenmesi Hakkında Tebliğ (Tebliğ No: 2016/35). Washington, DC: Mevzuat bilgi sistemi.
Tonay, M. A. (2016). Estimates of cetacean by-catch in the turbot fishery on the Turkish Western Black Sea Coast in 2007 and 2008. J. Mar. Biol. Assoc. U.K. 96, 993–998. doi: 10.1017/S0025315416000060
Tsikliras, A. C., Dinouli, A., Tsiros, V.-Z., and Tsalkou, E. (2015). The Mediterranean and Black Sea fisheries at risk from overexploitation. PLoS One 10:e0121188. doi: 10.1371/journal.pone.0121188
Tsikliras, A. C., Licandro, P., Pardalou, A., McQuinn, I. H., Gröger, J. P., and Alheit, J. (2018). Synchronization of Mediterranean pelagic fish populations with the North Atlantic climate variability. Deep Sea Res. Part II Top. Stud. Oceanogr. 159, 143–151. doi: 10.1016/j.dsr2.2018.07.005
Turkish Statistical Institute [TUIK] (2018). Fishery Statistics 2017. Ankara. Available at: http://www.tuik.gov.tr (accessed September 14, 2018).
Ulman, A., Bekisoglu, S., Zengin, M., Knudsen, S., Unal, V., Matthews, C., et al. (2013). From bonito to anchovy, a reconstruction of Turkey’s marine fish catches (1950-2010). Med. Mar. Sci. 14, 309–342.
Ulman, A., and Pauly, D. (2016). Making history count: the shifting baselines of the Turkish fisheries. Fish. Res. 183, 74–79. doi: 10.1016/j.fishres.2016.05.013
Ulman, A., Unal, V., and Bekisoglu, S. (2016a). “Turkey (Mediterranean),” in Global Atlas of Marine Fisheries: A Critical Appraisal of Catches and Ecosystem Impacts, eds D. Pauly and D. Zeller (Washington, DC: Island Press), 418.
Ulman, A., Unal, V., and Mathews, C. (2016b). “Turkey (Marmara Sea),” in Global Atlas of Marine Fisheries: A Critical Appraisal of Catches and Ecosystem Impacts, eds D. Pauly and D. Zeller (Washington, DC: Island Press), 417.
Ulman, A., Zengin, M., and Knudsen, H. (2016c). “Turkey (Black Sea),” in Global Atlas of Marine Fisheries: A Critical Appraisal of Catches and Ecosystem Impacts, eds D. Pauly and D. Zeller (Washington, DC: Island Press), 416.
Ünal, V., and Göncüoğlu, H. (2012). “Fisheries Management in Turkey,” in The State of the Turkish Fisheries, eds A. Tokaç, A. C. Gücü, and B. Öztürk (Ýstanbul: Turkish Marine Research Foundation).
Wang, S.-P., Maunder, M. N., and Aires-da-Silva, A. (2014). Selectivity’s distortion of the production function and its influence on management advice from surplus production models. Fish. Res. 158, 181–193. doi: 10.1016/j.fishres.2014.01.017
Winker, H., Carvalho, F., and Kapur, M. (2018). JABBA: just another bayesian biomass assessment. Fish. Res. 204, 275–288. doi: 10.1016/j.fishres.2018.03.010
Ye, Y., Cochrane, K., Bianchi, G., Willmann, R., Majkowski, J., Tandstad, M., et al. (2012). Rebuilding global fisheries: the World summit Goal, costs and benefits Fish. Fish 14, 174–185. doi: 10.1111/j.1467-2979.2012.00460.x
Zengin, M., Gümüş, A., Süer, S., Van, A., Özcan Akpınar, İ, and Dağtekin, M. (2014). “Discard trends of bottom trawl fishery along the Samsun Shelf Area of the Turkish Black Sea coast,” in Proceedings of the Abstracts Books of ICES Symposium - Effects of Fishing on Benthic Fauna, Habitat and Ecosystem Function, Tromso.
Keywords: stock status, data-poor stocks, CMSY, recovery, Turkey, fisheries management
Citation: Demirel N, Zengin M and Ulman A (2020) First Large-Scale Eastern Mediterranean and Black Sea Stock Assessment Reveals a Dramatic Decline. Front. Mar. Sci. 7:103. doi: 10.3389/fmars.2020.00103
Received: 03 July 2019; Accepted: 07 February 2020;
Published: 21 February 2020.
Edited by:
Tomaso Fortibuoni, Higher Institute for Environmental Protection and Research (ISPRA), ItalyReviewed by:
Marianna Giannoulaki, Hellenic Centre for Marine Research (HCMR), GreeceEkin Akoglu, Middle East Technical University, Turkey
Copyright © 2020 Demirel, Zengin and Ulman. This is an open-access article distributed under the terms of the Creative Commons Attribution License (CC BY). The use, distribution or reproduction in other forums is permitted, provided the original author(s) and the copyright owner(s) are credited and that the original publication in this journal is cited, in accordance with accepted academic practice. No use, distribution or reproduction is permitted which does not comply with these terms.
*Correspondence: Nazli Demirel, ndemirel@istanbul.edu.tr