- 1Department of Geosciences and Geography, University of Helsinki, Helsinki, Finland
- 2Tvärminne Zoological Station, University of Helsinki, Hanko, Finland
- 3Baltic Sea Centre, Stockholm University, Stockholm, Sweden
The global biodiversity loss has raised interest in the different facets of diversity, and the importance of diversity for ecosystem functions has been recognized. However, our knowledge on seasonal and inter-annual variation in the composition and diversity of communities is still poor. Here, we investigated the seasonal and inter-annual changes in taxonomic and functional community composition and diversity of benthic diatoms in a coastal habitat of the northern Baltic Sea, where seasonal and inter-annual variation of climate is pronounced. We found that the taxonomic and functional alpha diversity remained stable at seasonal and inter-annual level despite strong changes in community composition. However, alpha diversity decreased during an exceptionally warm winter possibly due to disturbances induced by the lack of ice. This may suggest that climate warming and consequently limited ice cover will affect the diversity of benthic communities.
Introduction
The current global biodiversity crisis threatens all ecosystems and has increased interest in studying the diversity of different organisms on different scales. A great amount of research has resolved patterns of diversity and its effects on different aspects of ecosystem functioning and services, such as productivity (e.g., Smith, 2007; Cadotte et al., 2009) and nutrient cycling (e.g., Smetacek, 1999; Spilling et al., 2018). Some of these studies have presented snapshot results across spatial gradients (e.g., Virta et al., 2019) while others have included temporal variation (e.g., Morin et al., 2014), but most of them have neglected the strong seasonal and inter-annual variation that occurs across most parts of the globe (but see Hillebrand et al., 2010). Ignoring the seasonal and inter-annual changes in diversity undermines our ability to generalize biodiversity patterns, compromises inferences gained from monitoring programs and jeopardizes conservation efforts of valuable ecosystems.
The seasonal cycle of biotic communities is usually characterized by changes in taxonomic community composition induced by temporary species gain during favorable growing season and temporary species loss during more unfavorable conditions (Hobson and McQuoid, 1997). This results in seasonal changes in the functions of the community. However, this pattern can be altered by strong dispersal abilities and a large regional species pool that allow the replacement of temporarily transient species (Zobel, 1997), and thus, maintain steadily high local taxonomic diversity. Temporal changes in the taxonomy also affect the functional composition and diversity of the communities, which are often more effective than taxonomy in showing the relationship between diversity and ecosystem functioning (Chapin et al., 2000). Furthermore, high taxonomic diversity supports functional redundancy (i.e., complementarity of species traits), which allows ecosystem stability throughout the year and also provides resilience against environmental change (Naeem et al., 2012). However, the temporal variation in functional diversity of communities in different ecosystems and habitats is still poorly known.
Diatoms are one of the most important groups of microorganisms in all aquatic ecosystems and account for as much as 20% of the total primary production on Earth (Nelson et al., 1995; Falkowski et al., 1998; Field et al., 1998). Different studies have agreed that the diversity of benthic diatom communities affects ecosystem productivity, although consensus on the direction of this effect has not been found. For example, Virta et al. (2019) showed that the diversity, especially functional alpha diversity, of benthic diatoms promotes ecosystem productivity, whereas Forster et al. (2006) found a negative relationship between diatom diversity and ecosystem productivity, which may be due to the dominance of a few highly productive species. However, considering the importance, high abundance and enormous diversity of benthic diatoms, it is surprising how little attention the temporal variation in their diversity has received.
To reduce these knowledge gaps, we present a study on the seasonal and inter-annual variation of the taxonomic and functional composition and diversity of benthic diatom communities. We conducted the study in the northern part of the Baltic Sea. The predominant climate zone in this area is a warm-summer humid continental climate with strong variations in air and water temperatures. Moreover, our sampling site was located in a generally shallow coastal archipelago area, which results in high seasonal and inter-annual variability in water temperature and annually occurring ice cover. Thus, biotic communities here experience strong temporal changes and are, thus, ideal for seasonal and inter-annual studies. Our specific research question and hypothesis was: Does seasonal and inter-annual climatic variation affect the taxonomic and functional composition and alpha diversity of benthic diatom communities? We hypothesized that the composition and diversity of communities would change significantly between seasons and climatically different years (Gilbert et al., 2010). We also predicted that we would find the lowest diversity during winter because of harsh conditions (Oberbeckmann et al., 2014).
Materials and Methods
Study Area and Sampling
The temporal sampling site was located in a small, sheltered and shallow (<2 m) bay on the Finnish coast of the Baltic Sea, at the Hanko-peninsula (Figure 1). The bottom of the bay is muddy, with cobble-sized stones close to shores. Due to the sheltered location, waves do not roll the stones, which form, thus, stable substrata for benthic diatoms. Climate in this area is a mixture of continental and oceanic climates, with strong seasonal and inter-annual variations. The average annual air temperature is ca. 6.8°C, and ice cover forms annually (Finnish Meteorological Institute, 2019). Water in the area is brackish with a salinity of 5–7. Thus, biotic communities are a mixture of brackish and freshwater species.
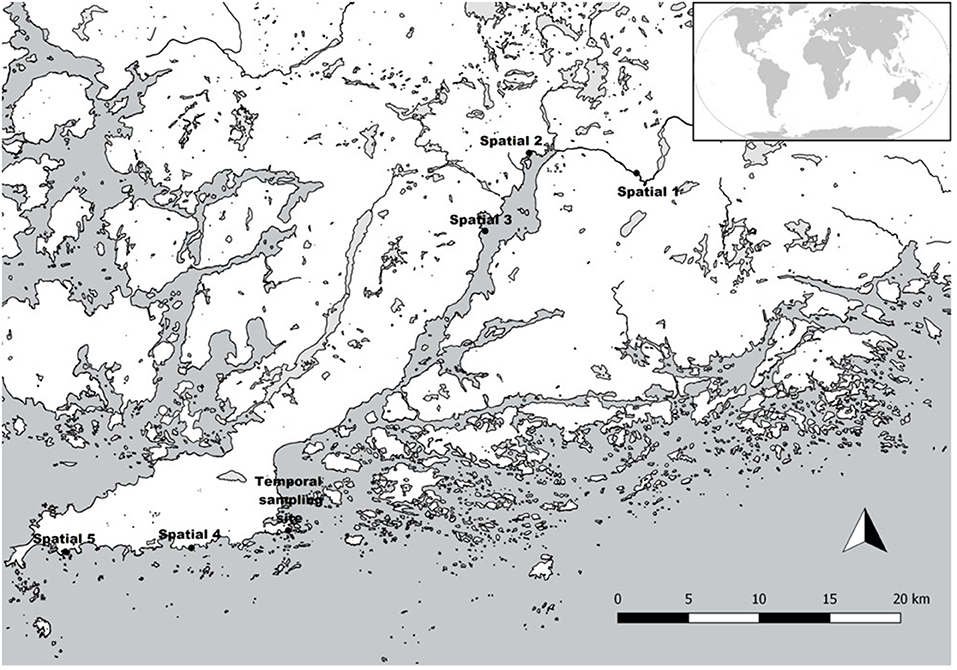
Figure 1. Our temporal sampling site and five spatio-temporal sites along the Hanko Peninsula in southern Finland, Baltic Sea. Map layers derived from HELCOM (2019), European Environment Agency (2019), and SYKE (2019).
To provide more generality and context to the results from our intensively sampled site, we also collected spatio-temporal samples from five sites at three occasions (see below), hence 15 spatio-temporal samples altogether, and compared the temporal changes in diversity between these sites and our temporally intensive sampling site. These five sites were located at a distance of ca. 60 kilometers from the temporally intensive sampling site (Figure 1), and represent a gradient from a freshwater river Karjaanjoki, through its estuary Pojo Bay, to the brackish archipelago area at the coast of the Hanko-peninsula. Thus, biotic communities at these sites represent highly different environmental conditions in terms of salinity, nutrients, and exposure to wave action.
We conducted temporal sampling at two-week-intervals over a ca. two-year-period, 20 April 2017–29 May 2019, covering altogether a period of 111 weeks (Supplementary Table 1). We collected a total of 56 samples from natural stones following the recommendations of Kelly et al. (1998). We randomly selected 10 cobble-sized stones from depths of 20–50 cm, and avoided using the same stones again for at least eight following weeks, because of the required re-colonization time of benthic microalgae (Hillebrand and Sommer, 2000). We collected the biofilm by brushing the surfaces of stones with a toothbrush (25 cm2/stone) and pooled the accumulated suspension into a composite sample. After sampling, we stored the samples in cold (+4°C) and dark conditions until further analyses. Our spatial samples for validation purposes were collected three times, June 2017, September 2017, and May 2019, following the same procedure.
Laboratory Analyses, Trait Analyses, and Temperature Calculations
We boiled the diatom samples in hydrogen peroxide (30% H2O2) to remove organic material, and mounted cleaned diatoms on slides using Naphrax (Brunel Microscopes Ltd., United Kingdom). We identified the diatoms (500 valves/sample) with a phase contrast light microscope with a 1,000× magnification to the lowest possible taxonomic level (typically species level). The identification followed Krammer and Lange-Bertalot (1986, 1988, 1991a,b), Snoeijs (1993), Snoeijs and Vilbaste (1994), Snoeijs and Potapova (1995), and Snoeijs and Kasperovicienè (1996). After identification, we transformed species counts into relative abundances.
To account for functional composition and diversity of diatom communities, we divided species to traits that are robust indicators of ecological behavior (Hodapp et al., 2016). We categorized species according to different classifications: size (biovolume classes: small <1,000 μm/large > 1,000 μm), mobility (mobile/non-mobile), type of attachment [adnate/pedunculate (which was further divided to pad-attached/stalk-attached)/non-attached], colonization (colonial/non-colonial), guilds (low-profile/high-profile/motile/planktonic) (Rimet and Bouchez, 2012), and nitrogen-fixing abilities (nitrogen-fixer/non-nitrogen-fixer) (Passy, 2017). Each species was categorized according to all six classifications, which resulted in numerous possible combinations for classifying a certain species. For example, one of the common species, Bacillaria paxillifera (O.F. Müller) T.Marsson, was classified as large/mobile/non-attached/non-colonial/motile/non-nitrogen-fixer. As the measure of trait composition, we used the combination of traits of all the species present in the community. For the identification of traits, we used above mentioned species and trait literature, and Snoeijs et al. (2002) and Diatoms of North America (2019).
We derived average seasonal air temperatures of the study area from a weather station located in close proximity of our temporal sampling site, at Tvärminne Zoological Station (Finnish Meteorological Institute, 2019). Average seasonal water temperatures were derived from permanent loggers located close to the sampling site (Monicoast, 2019). These loggers by Finnish Meteorological Institute and later by Tvärminne Zoological Station have measured water temperature since the beginning of 1900.
Statistical Analyses
We divided our data into seasons according to thermal seasons (Finnish Meteorological Institute, 2019). Thermal seasons are characteristic to each location and each year. They are categorized as follows: thermal summer begins when daily average temperature rises permanently above +10°C, thermal fall when average daily temperature decreases below +10°C, thermal winter when average daily temperature decreases below 0°C, and thermal spring when average daily temperature rises permanently above 0°C.
To test if the diatom communities are significantly different between adjacent seasons and years, we ran analysis of similarities (ANOSIM), with Bray-Curtis distance (Clarke, 1993). ANOSIM is a distribution-free analog of one-way ANOVA, where values range between−1 and 1, 1 indicating total dissimilarity between groups and −1 greater dissimilarities within groups than between groups.
To illustrate season-specific patterns in taxonomic and functional community composition, we used Nonmetric Multidimensional Scaling (NMDS) with Bray-Curtis distance, and three dimensions for taxonomic composition and two dimensions for functional composition. We also calculated a centroid for the samples of each sampling season. We ran NMDS with the R packages vegan (Oksanen, 2019) and ggplot2 (Wickham, 2019).
To compare the taxonomic and functional alpha diversity in diatom communities between seasons, we used Shannon diversity index with logarithm base of b = 2 (Shannon and Weaver, 1962), which is a commonly used measure of diversity. Due to its sensitivity to rare species (Nagendra, 2002), it is a suitable index for studies concerning diatom communities, where the majority of species is usually rare. Although species richness is the most commonly used measure of diversity, we decided not to include it as a parameter in this study. This decision was due to the large variation in species richness between samples and the fixed amount (500) of frustules identified per sample, which may have underestimated the number of species in speciose samples.
To test the significance of differences in taxonomic and functional diversity between seasons, we first computed one-way ANOVA test with season as a categorical factor. Then, we calculated pairwise significances between diversities of all seasons with Tukey Honest Significant Differences. We considered adjusted p < 0.05 to denote significant differences.
To validate the results of our temporal samples, we compared the degree of temporal beta diversity of our temporal sampling site with five other sites, which were sampled three times, using temporal beta diversity indices (TBI) (Winegardner et al., 2017). Our aim was to see whether the direction of change in diversity at our temporal site coincides with other five sites and, thus, to make our results more general. TBI is based on the Podani family of beta diversity and computes total temporal beta diversity as well as different components (species loss or species gain) of that beta diversity by forming pairs observed at time 1 (T1) and time 2 (T2). It computes species loss (bj) from T1 to T2 with the equation: bj = (y1j–y2j) if y1j > y2j, and species gain (cj) from T1 to T2 with the equation: cj = (y2j–y1j) if y2j > y1j. We ran the TBI for taxonomic temporal beta diversity with binary data using Bray-Curtis dissimilarities. The significances were computed using a parametric paired t-test with 9999 permutations. We ran the TBI with the R package adespatial (Dray et al., 2019).
All statistical analyses were conducted using R version 3.6.1 (R Development Core Team, 2019).
Results
Total species richness in our temporal samples was 272, and species richness per sample varied between 31 and 70 (Supplementary Table 1). In general, all traits were present in all the samples, except planktonic guild, which was absent from nine samples and the nitrogen-fixing trait that was absent from 11 samples. Species abundances at sampling sites are presented in Supplementary Table 2, and trait classifications for all the species in Supplementary Table 3.
Thermal summer at our temporal site began on 19 May in 2017, 8 May in 2018 and 16 May in 2019, thermal fall on 6 October in 2017 and 24 September in 2018, thermal winter on 10 January in 2017–2018 (hereafter referred to as winter 2018) and 13 December in 2018–2019 (hereafter referred to as winter 2019), and thermal spring on 4 April in 2018 and 14 March in 2019 (Finnish Meteorological Institute, 2019). ANOSIM showed that the diatom communities were taxonomically and functionally different between most of the seasons (Table 1). Communities were also significantly different between the first and the second sampling year.
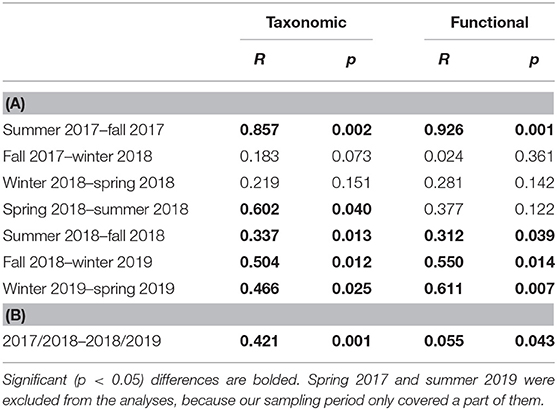
Table 1. Results of the ANOSIM to analyze the differences in taxonomic and functional community composition between (A) seasons and (B) years.
In the NMDS, compositions during most of the individual seasons formed clear clusters (Figure 2). These clusters showed cyclic annual variation in taxonomic and functional community composition. Taxonomic composition cycles showed clear differences between years, whereas functional composition cycles remained more similar between years.
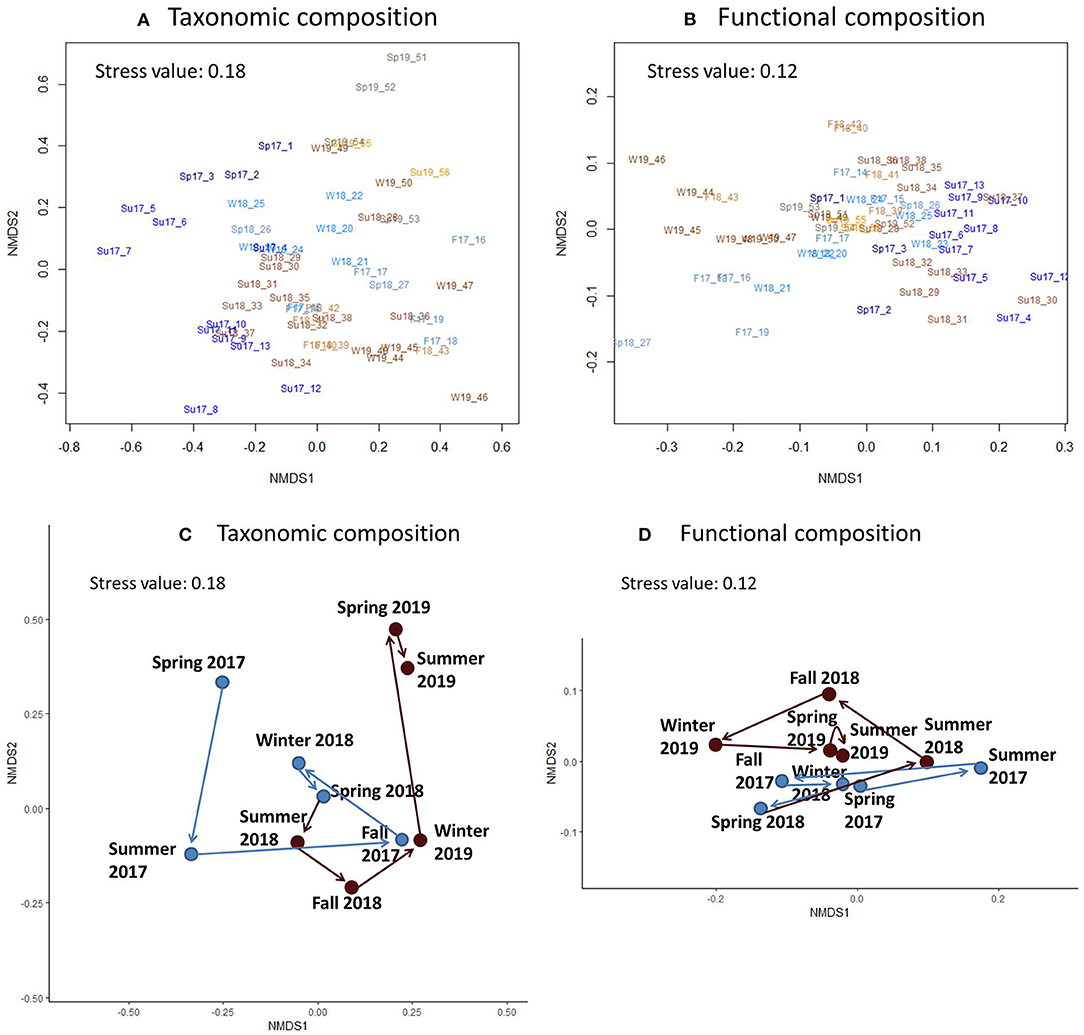
Figure 2. Illustrations for Nonmetric Multidimensional Scaling (NMDS) for community composition. (A,C) Illustrate taxonomic composition, and (B,D) functional composition. (A,B) Illustrate all the sampling times. (C,D) Illustrate centroids calculated for the samples of each season, with arrows showing the directions of annual cycles in community composition. (C,D) Blue circles and arrows illustrate the cycle in community composition during the first sampling year, and the burgundy circles and arrows the cycle during the second sampling year. (A,B) Sp, spring; Su, summer; F, fall; W, winter; 17, year 2017; 18, year 2018; 19, year 2019. Numbers after the sampling year refer to the temporal order of samples.
Despite considerable seasonal variation in air temperature (average seasonal temperature −4.0°C to 16.6°C) and water temperature (average seasonal temperature 0.6°C to 15.1°C), average seasonal diversity in our samples remained stable throughout the sampling period (Table 2A). Seasonal taxonomic diversity (Shannon diversity index) varied between 1.209 and 1.358, and seasonal functional diversity (Shannon diversity index) between 2.932 and 3.272. Season also appeared as a significant factor for both the taxonomic and functional diversity in the ANOVA (Table 2B). Taxonomic diversity was highest during winter 2018, which was a cold winter (average air temperature −4.0°C and solid ice cover at the sampling site for five consecutive sampling times), and lowest during winter 2019, which was a warm winter (average air temperature −1.5°C and solid ice cover at the sampling site for only two consecutive sampling times). Tukey multiple pairwise comparisons confirmed that the diversity of diatom communities was significantly different during the warm winter 2019 than during other seasons (Table 2C).
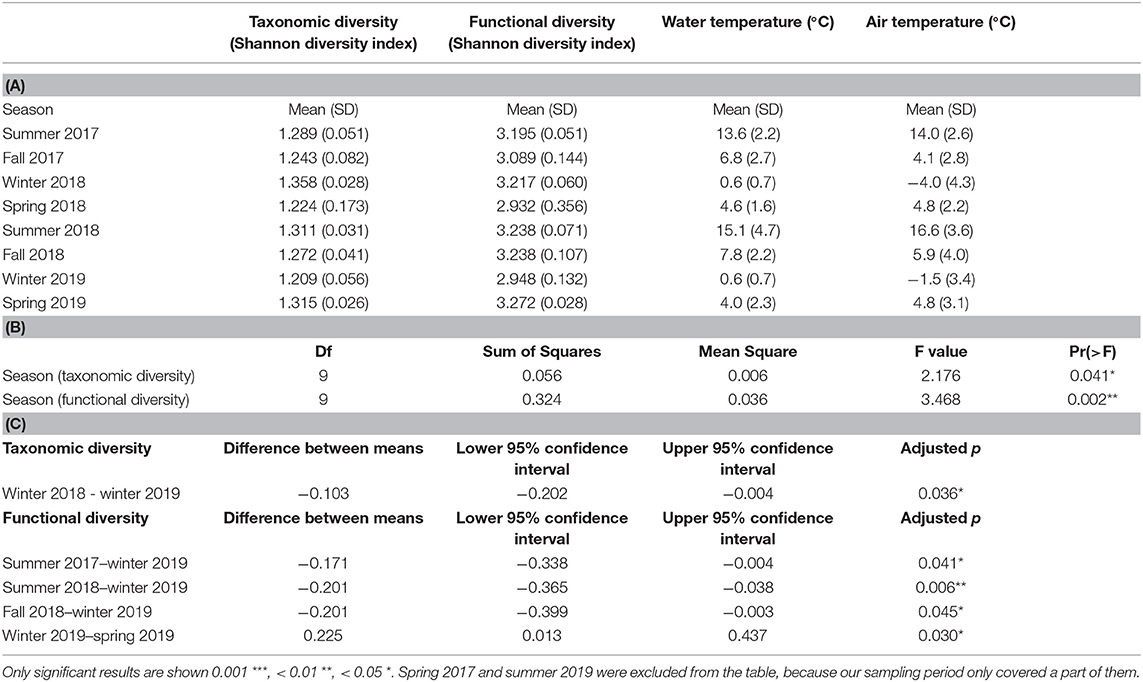
Table 2. (A) Mean and standard deviation for taxonomic and functional diversity (Shannon diversity index), water temperature and air temperature during seasons; (B) Results of the one-way ANOVA test to analyze the significance of the season for taxonomic and functional diversity; (C) Results of the Tukey Honest Significant Differences test to analyze pairwise differences between diversities of all seasons.
Our TBI analysis showed that temporal beta diversity was non-significant at the temporal sampling site and four of the spatio-temporal sites from June 2017 to September 2017, and from September 2017 to May 2019 (Table 3). The only significant temporal beta diversity occurred at the spatio-temporal sampling site 4, where the change was significant and negative from June 2017 to September 2017.
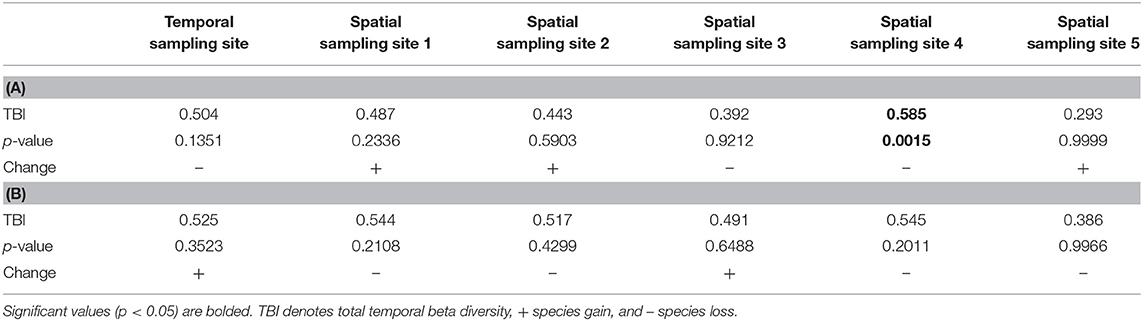
Table 3. Temporal beta diversity according to temporal beta diversity indices (TBI) at our temporal sampling site and five spatial sites between (A) June 2017 and September 2017, and (B) September 2017 and May 2019.
Discussion
We studied the seasonal and inter-annual variation in the taxonomic and functional structure and diversity of littoral benthic diatom communities in the Baltic Sea, where seasons and years are climatically highly variable. Despite the importance of benthic communities for ecosystem functioning and services, seasonal and inter-annual changes are often overlooked, which compromises the effectiveness of monitoring and conservation efforts of these valuable ecosystems.
We collected samples at two-week-intervals for two consecutive years, from spring 2017 to summer 2019. There was considerable variation in air and water temperatures between seasons and years, year 2017–2018 featuring a cool summer and a cold winter, whereas 2018–2019 featuring the warmest ever recorded summer (Monicoast, 2019) and a mild winter. Due to the northern and continental/marine location of our sampling area, such strong seasonal and annual changes in climate are typical. Along with the climatic temperature changes, other factors that have previously been shown to affect diatom communities in the Baltic Sea and elsewhere, such as nutrient concentrations (Svensson et al., 2014), daytime length and light intensity (Yang and Flower, 2012), also vary seasonally in this area and may have had an impact on the communities. In contrast, salinity, which often is the strongest driver of benthic diatom communities (Ulanova et al., 2009), remains highly similar throughout the year and was, thus, not likely to affect the communities in our study.
As we expected, this variation in environment led to highly variable taxonomic composition of communities between seasons and years, and also highly variable functional composition between seasons. Similar results of strong temporal variation have been found with different organisms in different ecosystems, such as soil microbes (Waldrop and Firestone, 2006), bacterioplankton (Van der Gucht et al., 2001), and fishes (Lazzari et al., 1999). However, our results disagree with several previous studies in the Baltic Sea, which have shown temporally stable community composition of benthic diatoms (Sabbe, 1993; Vilbaste et al., 2000). However, the duration of these studies has been remarkably shorter than ours, and they have been conducted on sedimentary soft bottoms, which may provide a more stable environment for the microphytobenthic organisms than stones.
However, the functional composition showed only low variation between years, and the alpha diversity of communities remained fairly stable between seasons and years. Such stable diversity patterns were discovered not only on our temporal sampling site but also on our five spatial sites, where we collected three samples during different seasons to validate our temporal results. This disagrees with studies conducted in other ecosystems, such as deep sea (Ramalho et al., 2014) and pelagic waters (Sabanci and Koray, 2011), but is in line with other benthic diatom studies in the Baltic Sea (Sabbe, 1993; Vilbaste et al., 2000). Reasons for the temporally stable diversity may include effective dispersal and large regional species pool, and consequent high functional redundancy of benthic microalgal communities. In other words, changing environmental conditions, such as water temperature, nutrients and light intensity, led to the disappearance of some species, but the seed bank, i.e., locally occurring resting stages of some species, the effective dispersal, and large regional species pool allowed them to be replaced by species that were favored by the new predominant conditions (Zobel, 1997). Hence, the communities were able to preserve high taxonomic diversity, which supported functional redundancy, i.e., complementarity of species traits that allows ecosystem stability throughout the year and also provides resilience against environmental change (Naeem et al., 2012).
Despite the similar result of temporally stable diversity in our study and other studies on benthic diatoms in the Baltic Sea, the diversity measured with Shannon's diversity index was remarkably lower in our study than in other studies in the same area. Our seasonal taxonomic mean diversity varied between 1.2 and 1.4, and seasonal functional mean diversity between 2.9 and 3.3, whereas, e.g., Vilbaste et al. (2000) found values of 2.8–5.2 for the taxonomic diversity of benthic diatom communities in the Gulf of Riga, Baltic Sea. Low diversity in our communities is astonishing, considering the fairly highly species richness, but we speculate that the low diversity is due to the dominance of a few species and, thus, low evenness of the communities.
Although the diversity of communities remained temporally fairly stable throughout our 2-year sampling period, we found the highest diversity of all seasons during the cold winter 2018 and the lowest diversity during the mild winter 2019. Taxonomic and functional diversity during the warm winter 2019 differed also significantly from several other seasons. Furthermore, the functional compositions of communities were different between winters, the cold winter 2018 featuring proportionally large amounts of large and high-growing species (traits: large-sized, pedunculate, pad-attached, colonial, and high-profile) and the warm winter 2019 small and mobile species (traits: small-sized, mobile, non-colonial, and motile). This finding of different response of small and large diatom species to environmental conditions is in agreement with Busse and Snoeijs (2002), who showed that small species were mostly affected by exposure to wave action and large species by salinity.
We speculate that the difference between winters in our study was due to differences in the duration of ice cover. Solid ice cover at the sampling site lasted for ca. 10 weeks during winter 2018 but only ca. 4 weeks during winter 2019. Microalgal studies in winter have concentrated on pelagic and sea-ice algae (e.g., Edgar et al., 2016; Enberg et al., 2018), which prevents comparing our results, but we speculate that low water temperature that always occurs during winter and wind-induced waves due to the lack of ice are cumulative stressors (Morin et al., 2015) that make species more vulnerable during ice-free winters. Thus, ice cover seems to be needed to protect high benthic diversity in winter. However, we only compared two consecutive years, and for broader conclusions on the effects of ice-cover or climate in general, studies covering longer time series are needed.
Conclusions
We showed that the diversity of benthic diatom communities is seasonally and inter-annually highly stable despite strong climatic variation and consequent changes in community composition. However, diversity seems to decrease during exceptionally warm winters possibly due to disturbances induced by the lack of ice. This may suggest that climate warming with smaller extent and shorter duration of ice cover will affect the diversity of benthic communities.
Data Availability Statement
The raw data supporting the conclusions of this article will be made available by the authors, without undue reservation, to any qualified researcher.
Author Contributions
LV, JS, and AN designed the study. LV conducted the field work, identified species, defined traits, conducted statistical analyses, and drafted the manuscript. All authors commented on the manuscript.
Funding
This work was supported by Walter and Andrée de Nottbeck Foundation (LV) and the Academy of Finland (Project ID 294853 to AN).
Conflict of Interest
The authors declare that the research was conducted in the absence of any commercial or financial relationships that could be construed as a potential conflict of interest.
Acknowledgments
We thank the staff at the Tvärminne Zoological Station: Tapio Rautalin and Jostein Solbakken for valuable help during winter sampling, and Noora Haavisto and Joanna Norkko for providing the climatic data.
Supplementary Material
The Supplementary Material for this article can be found online at: https://www.frontiersin.org/articles/10.3389/fmars.2020.00088/full#supplementary-material
Supplementary Table 1. Sampling details.
Supplementary Table 2. Species abundance table.
Supplementary Table 3. Trait table.
References
Busse, S., and Snoeijs, P. (2002). Gradient responses of diatom communities in the Bothnian Bay, Northern Baltic Sea. Nova Hedwigia 74, 501–525. doi: 10.1127/0029-5035/2002/0074-0501
Cadotte, M. W., Cavender-Bares, J., Tilman, D., and Oakley, T. H. (2009). Using phylogenetic, functional and trait diversity to understand patterns of plant community productivity. PLoS ONE 4:e5e5695. doi: 10.1371/journal.pone.0005695
Chapin, F. S., Zavaleta, E. S., Eviner, V. T., Naylor, R. L., Vitousek, P. M., Reynolds, H. L., et al. (2000). Consequences of changing biodiversity. Nature 405, 234–242. doi: 10.1038/35012241
Clarke, K. R. (1993). Non-parametric multivariate analyses of changes in community structure. Aust. J. Ecol. 18, 117–143. doi: 10.1111/j.1442-9993.1993.tb00438.x
Diatoms of North America (2019). Available online at: https://diatoms.org/ (accessed October 22, 2019).
Dray, S., Bauman, D., Blanchet, G., Borcard, D., Clappe, S., Guenard, G., et al. (2019). Package “adespatial”. Available online at: https://cran.r-project.org/web/packages/adespatial/adespatial.pdf (accessed October 15, 2019).
Edgar, R. E., Morris, P. F., Rozmarynowycz, M. J., D'souza, N. A., Moniruzzaman, M., Bourbonniere, R. A., et al. (2016). Adaptations to phytoautotrophy associated with seasonal ice cover in a large lake revealed by metatranscriptome analysis of a winter diatom bloom. J. Great Lakes Res. 42, 1007–1015. doi: 10.1016/j.jglr.2016.07.025
Enberg, S., Majaneva, M., Autio, R., Blomster, J., and Rintala, J. M. (2018). Phases of microalgal succession in sea ice and the water column in the Baltic Sea from autumn to spring. Mar. Ecol. Prog. Ser. 599, 19–34. doi: 10.3354/meps12645
European Environment Agency (2019). Available online at: https://www.eea.europa.eu/data-and-maps (accessed October 28, 2019).
Falkowski, P. G., Barber, R. T., and Smetacek, V. (1998). Biogeochemical controls and feedbacks on ocean primary production. Science 281, 200–206. doi: 10.1126/science.281.5374.200
Field, C. B., Behrenfeld, M. J., Randerson, J. T., and Falkowski, P. (1998). Primary production of the biosphere: integrating terrestrial and oceanic components. Science 281, 237–240. doi: 10.1126/science.281.5374.237
Finnish Meteorological Institute (2019). Available online at: https://en.ilmatieteenlaitos.fi/ (accessed October 22, 2019).
Forster, R. M., Creach, V., Sabbe, K., Vyverman, W., and Stal, L. J. (2006). Biodiversity-ecosystem function relationship in microphytobenthic diatoms of the Westerschelde estuary. Mar. Ecol. Prog. Ser. 311, 191–201. doi: 10.3354/meps311191
Gilbert, J. A., Field, D., Swift, P., Thomas, S., Cummings, D., Temperton, B., et al. (2010). The taxonomic and functional diversity of microbes at a temperate coastal site: a ‘multi-omic' study of seasonal and diel temporal variation. PLoS ONE 5:e1e15545. doi: 10.1371/journal.pone.0015545
HELCOM (2019). Available onlione at: http://helcom.fi/baltic-sea-trends/data-maps (accessed October, 28 2019).
Hillebrand, H., Soininen, J., and Snoeijs, P. (2010). Warming leads to higher species turnover in a coastal ecosystem. Glob. Change Biol. 16, 1181–1193. doi: 10.1111/j.1365-2486.2009.02045.x
Hillebrand, H., and Sommer, U. (2000). Diversity of benthic microalgae in response to colonization time and eutrophication. Aquat. Bot. 67, 221–236. doi: 10.1016/S0304-3770(00)00088-7
Hobson, L. A., and McQuoid, M. R. (1997). Temporal variations among planktonic diatom assemblages in a turbulent environment of the southern Strait of Georgia, British Columbia, Canada. Mar. Ecol. Prog. Ser. 150, 263–274. doi: 10.3354/meps150263
Hodapp, D., Hillebrand, H., Blasius, B., and Ryabov, A. B. (2016). Environmental and trait variability constrain community structure and the biodiversity-productivity relationship. Ecology 97, 1463–1474. doi: 10.1890/15-0730.1
Kelly, M. G., Cazaubon, A., Coring, E., Dell'Uomo, A., Ector, L., Goldsmith, B., et al. (1998). Recommendations for the routine sampling of diatoms for water quality assessments in Europe. J. Appl. Phycol. 10, 215–224. doi: 10.1023/A:1008033201227
Krammer, K., and Lange-Bertalot, H. (1986). “Bacillariophyceae 1. Teil: naviculaceae,” in Süßwasserflora von Mitteleuropa, eds H. Ettl, J. Gerloff, H. Heynig and D. Mollenhauer (Stuttgart: Gustav Fischer Verlag), 876.
Krammer, K., and Lange-Bertalot, H. (1988). “Bacillariophyceae 2. Teil: bacillariaceae, epithemiaceae, Surirellaceae,” in Süßwasserflora von Mitteleuropa, eds H. Ettl, J. Gerloff, H. Heynig and D. Mollenhauer (Stuttgart: Gustav Fischer Verlag), 596.
Krammer, K., and Lange-Bertalot, H. (1991a). “Bacillariophyceae 3. Teil: centrales, fragilariaceae, eunotiaceae,” in Süßwasserflora von Mitteleuropa, eds H. Ettl, J. Gerloff, H. Heynig and D. Mollenhauer (Stuttgart: Gustav Fischer Verlag), 576.
Krammer, K., and Lange-Bertalot, H. (1991b). “Bacillariophyceae 4. Teil: achnanthaceae, kritische ergänzungen zu navicula (Lineolatae) und Gomphonema,” in Süßwasserflora von Mitteleuropa, eds H. Ettl, J. Gerloff, H. Heynig and D. Mollenhauer (Stuttgart: Gustav Fischer Verlag), 437.
Lazzari, M. A., Sherman, S., Brown, C. S., King, J., Joule, B. J., Chenoweth, S. B., et al. (1999). Seasonal and annual variations in abundance and species composition of two nearshore fish communities in Maine. Estuaries 22, 636–647. doi: 10.2307/1353051
Monicoast (2019). http://helsinki.fi/monicoast. (accessed October 25, 2019).
Morin, S., Bonet, B., Corcoll, N., Guasch, H., Bottin, M., and Coste, M. (2015). Cumulative stressors trigger incrased vulnerability of diatom communities to additional disturbances. Microb. Ecol. 70, 585–595. doi: 10.1007/s00248-015-0602-y
Morin, X., Fahse, L., de Mazancourt, C., Scherer-Lorenzen, M., and Bugmann, H. (2014). Temporal stability in forest productivity increases with tree diversity due to asynchrony in species dynamics. Ecol. Lett. 17, 1526–1535. doi: 10.1111/ele.12357
Naeem, S., Duffy, J. E., and Zavaleta, E. (2012). The functions of biological diversity in an age of extinction. Science 336, 1401–1406. doi: 10.1126/science.1215855
Nagendra, H. (2002). Opposite trends in response for the Shannon and Simpson indices of landscape diversity. Appl. Geogr. 22, 175–186. doi: 10.1016/S0143-6228(02)00002-4
Nelson, D. M., Treguer, P., Brzezinki, M. A., Leynaert, A., and Queguiner, B. (1995). Production and dissolution of biogenic silica in the ocean – Revised global estimates, comparison with regional data and relationship to biogenic sedimentation. Global Biogeochem. Cy. 9, 359–372. doi: 10.1029/95GB01070
Oberbeckmann, S., Loeder, M. G., Gerdts, G., and Osborn, A. M. (2014). Spatial and seasonal variation in diversity and structure of microbial biofilms on marine plastics in Northern European waters. Fems Microbiol. Ecol. 90, 478–492. doi: 10.1111/1574-6941.12409
Oksanen, J. (2019). Package “vegan”. Available onlone at: https://cran.r-project.org/web/packages/vegan/vegan.pdf (accessed October 22, 2019).
Passy, S. I. (2017). Framework for community functioning: synthesis of stress gradient and resource partitioning concepts. PEERJ 5:e3885. doi: 10.7717/peerj.3885
R Development Core Team (2019). The R Project for Statistical Computing. Retrieved from: http://www.R-project.org
Ramalho, S. P., Adao, H., Kiriakoulakis, K., Wolff, G. A., Vanreusel, A., and Ingels, J. (2014). Temporal and spatial variation in the Nazare Canyon (Western Iberian margin): Inter-annual and canyon heterogeneity effects on meiofauna biomass and diversity. Deep-sea Res. Pt I 83, 102–114. doi: 10.1016/j.dsr.2013.09.010
Rimet, F., and Bouchez, A. (2012). Life-forms, cell-sizes and ecological guilds of diatoms in European rivers. Knowl. Manag. Aquat. Ec. 406:01. doi: 10.1051/kmae/2012018
Sabanci, F. C., and Koray, T. (2011). Annual variation in the diversity, species richness and composition of the phytoplankton assemblages in the Izmir Bay (Eastern Aegean). Turk. J. Fish Aquat. Sci. 11, 303–314. doi: 10.4194/trjfas.2011.0215
Sabbe, K. (1993). Short-term fluctuations in benthic diatom numbers on an intertidal sandflat in the Westerschelde estuary (Zeeland, the Netherlands). Hydrobiologia 269, 275–284. doi: 10.1007/BF00028026
Shannon, C. E., and Weaver, W. (1962). The Mathematical Theory of Communication. Urbana, IL: University of Illinois Press.
Smetacek, V. (1999). Diatoms and the ocean carbon cycle. Protist 150, 25–32. doi: 10.1016/S1434-4610(99)70006-4
Smith, V. H. (2007). Microbial diversity-productivity relationships in aquatic ecosystems. Fems. Microbiol. Ecol. 62, 181–186. doi: 10.1111/j.1574-6941.2007.00381.x
Snoeijs, P. (1993). Intercalibration and Distribution of Diatom Species in the Baltic Sea Volume 1. Uppsala: Opulus Press.
Snoeijs, P., Busse, S., and Potapova, M. (2002). The importance of diatom cell size in community analysis. J. Phycol. 38, 265–272. doi: 10.1046/j.1529-8817.2002.01105.x
Snoeijs, P., and Kasperovicienè, J. (1996). Intercalibration and Distribution of Diatom Species in the Baltic Sea Volume 4. Uppsala: Opulus Press.
Snoeijs, P., and Potapova, M. (1995). Intercalibration and Distribution of Diatom Species in the Baltic Sea Volume 3. Uppsala: Opulus Press.
Snoeijs, P., and Vilbaste, S. (1994). Intercalibration and Distribution of Diatom Species in the Baltic Sea Volume 2. Uppsala: Opulus Press.
Spilling, K., Olli, K., Lehtoranta, J., Kremp, A., Tedesco, L., Tamelander, T., et al. (2018). Shifting diatom-dinoflagellate dominance during spring bloom in the Baltic Sea and its potential effects on biogeochemical cycling. Front. Mar. Sci. 5:327. doi: 10.3389/fmars.2018.00327
Svensson, F., Norberg, J., and Snoeijs, P. (2014). Diatom cell size, coloniality and motility: trade-offs between temperature, salinity and nutrient supply with climate change. PLoS ONE 9:e1e109993. doi: 10.1371/journal.pone.0109993
SYKE (2019). Ladattavat Paikkatietoaineistot. Available online at: https://www.syke.fi/fi-FI/Avoin_tieto/Paikkatietoaineistot (accessed October, 28 2019).
Ulanova, A., Busse, S., and Snoeijs, P. (2009). Coastal diatom-environment relationships in the brackish Baltic Sea. J. Phycol. 45, 54–68. doi: 10.1111/j.1529-8817.2008.00628.x
Van der Gucht, K., Sabbe, K., De Meester, L., Vloemans, N., Zward, G., Gillis, M., et al. (2001). Contrasting bacterioplankton community composition and seasonal dynamics in two neighbouring hypertrophic freshwater lakes. Environ. Microbiol. 3, 680–690. doi: 10.1046/j.1462-2920.2001.00242.x
Vilbaste, S., Sundback, K., Nilsson, C., and Truu, J. (2000). Distribution of benthic diatoms in the littoral zone of the Gulf of Riga, the Baltic Sea. Eur. J. Phycol. 35, 373–385. doi: 10.1080/09670260010001735981
Virta, L., Gammal, J., Järnström, M., Bernard, G., Norkko, J., and Norkko, A. (2019). The diversity of benthic diatoms affects ecosystem productivity in heterogeneous coastal environments. Ecology 100:e02765. doi: 10.1002/ecy.2765
Waldrop, M. P., and Firestone, M. K. (2006). Seasonal dynamics of microbial community composition and function in oak canopy and open grassland soils. Microb. Ecol. 52, 470–479. doi: 10.1007/s00248-006-9100-6
Wickham, H. (2019). Package ‘ggplot2'. Available online at: https://cran.r-project.org/web/packages/ggplot2/ggplot2.pdf (accessed January 14, 2020).
Winegardner, A. K., Legendre, P., Beisner, B. E., and Gregory-Eaves, I. (2017). Diatom diversity patterns over the past c. 150 years across the conterminous United States of America: identifying mechanisms behind beta diversity. Global Ecol. Biogeogr. 26, 1303–1315. doi: 10.1111/geb.12640
Yang, H., and Flower, R. J. (2012). Effects of light and substrate on the benthic diatoms in an oligotrophic lakes: a comparison between natural and artificial substrates. J. Phycol. 48, 1166–1177. doi: 10.1111/j.1529-8817.2012.01201.x
Keywords: seasonal variation, annual variation, community composition, diversity, benthic diatoms, Baltic Sea, microphytobenthos
Citation: Virta L, Soininen J and Norkko A (2020) Stable Seasonal and Annual Alpha Diversity of Benthic Diatom Communities Despite Changing Community Composition. Front. Mar. Sci. 7:88. doi: 10.3389/fmars.2020.00088
Received: 04 December 2019; Accepted: 03 February 2020;
Published: 25 February 2020.
Edited by:
Vona Meleder, Université de Nantes, FranceReviewed by:
Lourenço Soares Ribeiro, Center for Marine and Environmental Sciences (MARE), PortugalDu Guoying, Ocean University of China, China
Copyright © 2020 Virta, Soininen and Norkko. This is an open-access article distributed under the terms of the Creative Commons Attribution License (CC BY). The use, distribution or reproduction in other forums is permitted, provided the original author(s) and the copyright owner(s) are credited and that the original publication in this journal is cited, in accordance with accepted academic practice. No use, distribution or reproduction is permitted which does not comply with these terms.
*Correspondence: Leena Virta, leena.virta@helsinki.fi