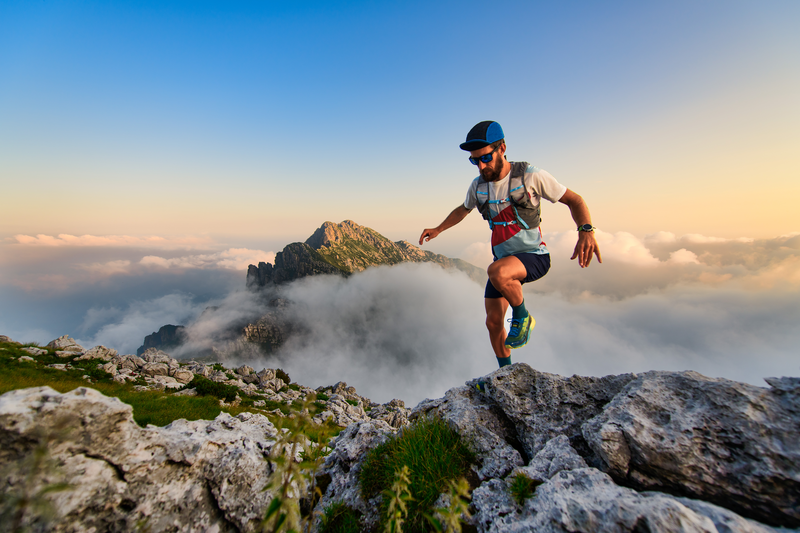
94% of researchers rate our articles as excellent or good
Learn more about the work of our research integrity team to safeguard the quality of each article we publish.
Find out more
BRIEF RESEARCH REPORT article
Front. Mar. Sci. , 20 February 2020
Sec. Marine Megafauna
Volume 7 - 2020 | https://doi.org/10.3389/fmars.2020.00087
Natural or human-induced environmental changes can modify the structure of ecological communities and thus alter food web interactions. After the collapse of hake stocks (Merluccius hubbsi) provoked by fisheries over the Patagonian shelf in 1997 profound changes have taken place in the community, including long-term dietary shifts in some marine vertebrate predators. Stable carbon (δ13C) and nitrogen (δ15N) isotope ratios in bone collagen of subadult and adult male South American fur seals (Arctocephalus australis) were measured for the period 1976–2017 to investigate if the changes occurred in the marine community from northern and central Patagonia affected the diet of this predator. Suess corrected δ13C values and δ15N values in bone collagen of fur seals did not change significantly over the study period. According to this, male fur seals have remained as pelagic foragers without changing their trophic position nor switching their main preys in the Patagonian food web over the last four decades. This long-term stability in the diet of fur seals contrasts with dietary changes reported for benthic foragers in the region. Although long-term diet studies are restricted to a few marine predators in the region, current evidence suggests that benthic-demersal foragers were more prone to dietary shifts than pelagic ones after perturbations that occurred in the marine community of northern and central Patagonia.
Heavy perturbations, either natural or anthropogenic, can modify the structure of ecological communities and thus alter food web interactions. The South Atlantic Ocean has a long history of large-scale removal of predators as a consequence of whaling, sealing and fishing. Populations of southern right whales Eubalaena australis, southern elephant seals Mirounga leonina, South American fur seals Arctocephalus australis, South American sea lions Otaria flavescens, and tope sharks Galeorhinus galeus, among other marine predators, were reduced from the Patagonian waters between end-18th and mid-20th centuries (Mateo, 2015; Würsig et al., 2018). This reduction in the biomass of large marine vertebrates is thought to be the main cause of changes in the regional food web structure, as modern food webs are longer, less redundant and less overlapping than ancient ones (Saporiti et al., 2014).
The Patagonian Shelf Large Marine Ecosystem is one of the most productive and complex marine regions in the Southern Hemisphere (Heileman, 2009). Approximately bounded by 41° and 48° S, the marine food web of northern and central Patagonia is organized around the anchovy Engraulis anchoita, hake Merluccius hubbsi and shortfin squid Illex argentinus (Angelescu and Prenski, 1987). These three core species support a large number of high trophic level predators (Croxall and Wood, 2002; Favero and Silva Rodríguez, 2005; Koen-Alonso and Yodzis, 2005, and references therein; Romero et al., 2012), and hake and shortfin squid also support intensive fishing (Brunetti et al., 1998; Bertolotti et al., 2001). On the other hand, the anchovy fishery is very underdeveloped in Patagonian waters and the main fishing grounds have been concentrated traditionally off Buenos Aires province (Hansen, 2000). In addition, there is an important fishery targeting the red shrimp Pleoticus muelleri (Figure 1A).
Figure 1. (A) Fishery landings of short-fin squid, hake, anchovy and red shrimp off Argentine coast (Sánchez et al., 2012; Navarro et al., 2014, 2019; MPyT-SA, 2020). The dashed vertical line in 1997 indicates when the hake fishery collapsed. Temporal trend in the stable isotope ratios in bone collagen of subadult and adult male South American fur seals from northern and central Patagonia: (B) raw δ13C values (empty pink circles) and the expected change in δ13C in the ocean caused by anthropogenic activity, namely the Oceanic Suess effect (empty orange squares); (C) Suess corrected δ13C values; and (D) δ15N values. The shaded gray bands surrounding the estimated trends in (C,D) are approximate 95% across-the-function confidence intervals.
Over the Patagonian shelf, far more than 70% of hake catches have been extracted historically south of 41° S (MPyT-SA, 2020). Since 1960s, the landings of hake by the Argentine fleet increased steadily, peaking in the mid 1990s and resulting on the overfishing of regional stocks (Bertolotti et al., 2001). On the other hand, fishing for red shrimp occurs mainly in coastal waters off northern and central Patagonia, between 43° and 46° S (Bertuche et al., 2000). This shrimp fishery generates high revenues and is yielding record catches in recent years, with 254,905.9 tonnes landed in 2018 (MPyT-SA, 2020). Hake smaller than 30 cm and anchovies are largely discarded by the shrimp fishery (Crespo et al., 1997; Gandini et al., 1999), as well as large amounts of lobster krill Munida gregaria (Varisco and Vinuesa, 2007). For the hake fishery operating south of the 41° S parallel, hake discards during the period 1986–1997 increased from 15,563 tonnes (1986) to 104,592 tonnes (1997), whereas these figures decreased and varied between 6,000 and 22,000 tonnes during the period 1998–2004 (Góngora et al., 2012). For its part, hake discards by the shrimp fishery varied between 17,195 and 46,452 tonnes during the period 1998–2004 (Góngora et al., 2012). Additionally, it is estimated that around 310,000 tonnes per year of several species of bony fishes and elasmobranchs were returned dead to the ecosystem as part of the by-catch of the hake bottom-trawl and shrimp fisheries in the region during the mid-1990s (LAMAMA, unpublished data).
Following the collapse of hake catches in 1997, an extensive year-round fishing closure, the Patagonian Closed Area, was created to protect juveniles and spawners of the species. After a decade of protection, the structure of the demersal fish assemblage changed in this offshore marine protected area (Alemany et al., 2013). Among the main changes, Alemany et al. (2013) detected a greater abundance of hake and other demersal fish species, increasing hake juvenile size, and a higher proportion of juveniles aged 2+ inside the protected area. In parallel, several high trophic level predator populations began to recover during the 20th century after the implementation of various legal protections (Croxall and Wood, 2002; Schiavini et al., 2005; Crespo et al., 2012, 2015; Ferrari et al., 2013; Raya Rey et al., 2014; Grandi et al., 2015; Baylis et al., 2019).
Massive extraction of marine fauna biomass seems to have produced heavy perturbations in the regional ecosystem. For instance, the harvesting of South American sea lions that took place between 1930 and 1960, together with the removal of large quantities of hake biomass by fisheries during mid-1990s, are thought to have caused a severe reorganization of the whole marine ecosystem in northern and central Patagonia (Koen-Alonso and Yodzis, 2005). In addition, there is evidence of long-term changes in the diet of some large marine vertebrates as a result of fisheries activities, such as in spiny dogfish Squalus acanthias (Koen-Alonso et al., 2002), yellownose skates Zearaja chilensis (Buren, 2004), South American sea lions (Drago et al., 2009), and black-browed albatrosses Thalassarche melanophris (Mariano-Jelicich et al., 2017).
The South American fur seal A. australis (von Zimmermann, 1783) is a high trophic level predator in the ecosystems of the southwestern South Atlantic and the southeastern South Pacific oceans. This predator is considered a generalist feeder (Franco-Trecu et al., 2014), foraging upon a wide variety of prey (Naya et al., 2002; Crespo et al., 2008; Oliveira et al., 2008; Baylis et al., 2014). Individuals of the species forage over both the continental shelf and the shelf-break (Dassis et al., 2012; Mandiola et al., 2015; Baylis et al., 2018). The existence of some degree of competition for shared resources between fur seals and fisheries has been suggested through all its distribution range (Naya et al., 2002; Szteren et al., 2004; Crespo et al., 2008; Vargas Hernández, 2012; Baylis et al., 2014, 2018; Vales et al., 2015). In northern and central Patagonian waters, male fur seals forage mainly on shortfin squid, anchovy, juvenile hake and decapod crustaceans (red shrimp and lobster krill) (Vales et al., 2015). With the exception of lobster krill, all these prey species are of commercial interest (Figure 1A). Shortfin squid and hake consumed by fur seals seem to be smaller than those exploited by fisheries (LAMAMA, unpublished data), although this result can be biased if fur seals break-up larger individuals and consume only some parts. In any case, the use of the same resource with different sizes (or differences in space and time) does not necessarily mitigate the intensity of the interaction (Harwood, 1987). Therefore, some degree of interaction between fisheries and fur seals is expected, although it is unknown how this might affected the long-term diet of this predator in the region.
Stable isotope analysis has become a valuable tool for the study of marine mammal ecology, allowing the reconstruction of dietary changes in some species through the analysis of specimens deposited in museum and scientific collections (Newsome et al., 2010). Stable isotope ratios of N and C are based on a predictable relation between the isotopic composition of a consumer and its diet (DeNiro and Epstein, 1978, 1981). For aquatic organisms, δ15N values can provide data on trophic level (Minagawa and Wada, 1984; Post, 2002), while δ13C values can reveal information on feeding locations, including the relative use of benthic vs. pelagic and coastal vs. oceanic prey (Hobson et al., 1994; France, 1995). This general pattern also applies for the Patagonian marine ecosystem, where benthic prey are more enriched in both 15N and 13C than pelagic ones (Forero et al., 2004; Ciancio et al., 2008; Drago et al., 2009; Vales et al., 2015). By using stable isotope ratios in bone collagen, it is possible to study the long-term trends in dietary patterns of vertebrates. Bone collagen has a slow turnover rate and integrates the stable isotope ratios over several years in marine mammals (Hirons et al., 2001; Riofrío-Lazo and Aurioles-Gamboa, 2013), allowing the comparison of the isotope ratios of many individuals over long periods of time (Schoeninger and DeNiro, 1984; Lee-Thorp et al., 1989; Hirons et al., 2001; Dalerum and Angerbjörn, 2005). Therefore, any change in the diet of the fur seal population over a long timescale should be reflected in the δ15N and δ13C values of their bone tissues.
This study uses stable carbon and nitrogen isotope ratios in bone collagen of South American fur seals to investigate if the reorganization of the marine community in Patagonian waters (Koen-Alonso and Yodzis, 2005; Saporiti et al., 2014) affected the diet of this predator over the last four decades. More precisely, we test the hypothesis that decreasing availability of hake resulted in a decrease in the δ15N and δ13C values of the bone tissues of fur seals, because of increased consumption of anchovies and squids (preys depleted in 15N and 13C).
Sixty-two skulls of male South American fur seals were sampled from individuals collected between 1976 and 2017 along the Atlantic coast of northern and central Patagonia (between 41° and 46° S). However, it is important to mention that samples are not distributed evenly over time. Two samples correspond to the years 1976 and 1977, some are scattered between the years 1990 and 1995, and the remaining correspond to the more recent years (i.e., 1996–2017). Specimens are deposited at the osteological collection of the Marine Mammal Laboratory (CESIMAR, CONICET). For this study, only male individuals older than 5 years (sub-adult and adult age classes) were considered, this is because juvenile males have a different diet than the subsequent age classes (Vales et al., 2015). Individual ages were estimated from counts of growth layer groups (GLGs) in the dentine and/or in the cementum of teeth assuming that one GLG is deposited per year (Schiavini et al., 1992; Crespo et al., 1994; Molina-Schiller and Pinedo, 2004). In order to prevent damage to the skulls, a small fragment of maxillo-turbinal bone from the nasal cavity was sampled (Drago et al., 2009), and then stored dry until isotope analysis.
The analysis of stomach contents, scats and C and N stable isotope ratios point to squids (I. argentinus and Doryteuthis gahi), anchovies (E. anchoita), juvenile hakes (M. hubbsi), and decapod crustaceans (P. muelleri and M. gregaria) as the main prey in the diet of fur seals in northern and central Patagonia (Vales et al., 2015). Accordingly, these prey species were sampled from January 2006 to April 2010 from the study region for isotope analysis. White dorsal muscle was sampled for fish, muscle for shrimp, and mantle for squid; whereas in the case of lobster krill (M. gregaria) the whole body was sampled due to its small size. All prey samples were stored in a freezer at −20°C until analysis. Fish, cephalopod, crustacean, and some of the isotope values in bone of fur seals have been published in previous papers (Drago et al., 2009; Vales et al., 2015) (Tables 1, 2).
Table 1. Stable isotope ratios of carbon (δ13C) and nitrogen (δ15N), and the C:N mass ratio (mean ± SD) of the main prey of South American fur seals.
Table 2. Stable isotope ratios (δ15N, raw δ13C, and Suess corrected δ13C) in bone collagen of subadult and adult male South American fur seals dead stranded during the period 1976–2017 in northern and central Patagonia.
Maxillo-turbinal bone and prey samples were dried in a stove at 60°C for 36–48 h and ground to a fine powder with a mortar and pestle. Lipids were extracted from samples through sequential washes with a chloroform/methanol (2:1) solution (Folch et al., 1957). Since bone samples contain a high concentration of inorganic carbon that may add undesirable variability to δ13C (Lorrain et al., 2003), they were treated by soaking for 24 h in 0.5 N hydrochloric acid (HCl) to decarbonize them (Newsome et al., 2006). Given that HCl treatment may affect δ15N (Bunn et al., 1995), each sample was divided into 2 subsamples: one was used for 13C analysis after decarbonizing and the other one was used for 15N analysis without decarbonizing.
Approximately 0.5–1 mg of dried bone and 0.3 mg of prey tissues were weighed into tin cups. Then, samples were combusted at 900°C, and analyzed in a continuous flow isotope ratio mass spectrometer. Samples were analyzed at the Centres Científic i Tecnològics of the University of Barcelona (CCiT-UB), the Instituto de Geocronología y Geología Isotópica (INGEIS-CONICET/UBA), and the University of New Mexico Center for Stable Isotopes (UNM-CSI). In all cases, international isotope secondary standards given by the International Atomic Energy Agency (IAEA) were used for calibration at a precision equal to or less than 0.3 ‰ for nitrogen and 0.2 ‰ for carbon. Stable isotope values of paired bone samples (n = 5) analyzed at the CCiT-UB and the INGEIS-CONICET/UBA laboratories proved to be not statistically significant (Vales et al., 2015). On the other hand, we did not have paired samples to test potential biases among the isotope values reported by these two laboratories and the UNM-CSI, thus we had to rely on the assumption that measurements conducted at these three labs are comparable. Stable isotope abundance is expressed in standard δ notation relative to carbonate Pee Dee Belemnite and atmospheric nitrogen. Carbon to nitrogen (C:N) atomic ratio was used as proxy for data quality (e.g., conservation status of the isotopic ratio in bone collagen and adequate lipid extraction; DeNiro, 1985; Newsome et al., 2010).
During the last three centuries, the accelerating release of anthropogenic CO2 to the atmosphere due to fossil fuel burning and deforestation has been decreasing rapidly the content of 13C in atmospheric CO2, and this is called the Suess effect (Keeling, 1979). In turn, CO2 is exchanged between air and water and the isotope values of atmospheric CO2 and aqueous CO2 equilibrate. In this way, the global oceanic 13C Suess effect follows the atmospheric 13C Suess effect record, although with a roughly decadal lag due to the long equilibration time-scale of 13C (Gruber et al., 1999; Körtzinger et al., 2003; Quay et al., 2003). In turn, as 13C-depleted oceanic aqueous CO2 increases, phytoplankton fractionation increases, and thus phytoplankton δ13C decreases through time (Mizutani and Wada, 1982; Laws et al., 1995). Because of this, the Suess effect needs to be accounted when interpreting temporal changes in δ13C in the aquatic environment (Verburg, 2007). Accordingly, raw δ13C values of the skeletal material of fur seals were corrected applying the equation developed by Verburg (2007) for correcting the Suess effect in organic matter from lacustrine environments. However, this equation was modified in the present study to consider the 10 years lag existing for isotopic equilibration between atmospheric CO2 and oceanic aqueous CO2:
where Y is the year when the fur seal died and was found beach stranded. The result of the equation, which is expressed in per mille units (‰), is added to the raw δ13C data. All Suess corrected δ13C values were referenced to the year 2008, as this is the average year for the prey-sampling period (2006–2010) (Figure 1B).
Generalized additive mixed models (GAMMs) were used to test temporal trends in the δ15N and Suess corrected δ13C values of skull bone samples between 1990 and 2017 years. By using GAMMs, it is possible to observe the tendency of stable isotope ratios to change over time without defining a particular curve order a priori, while also accounting for temporal correlation (Wood, 2017; Simpson, 2018). GAMMs were fitted using a Gaussian distribution with an identity link function in the Mixed GAM Computation Vehicle (mgcv version 1.8–28) package with the restricted maximum likelihood (REML) smoothing method (Wood, 2017) in R 3.6.0 (R Development Core Team, 2019). Models for δ15N and Suess corrected δ13C data were fitted using basis dimensions (k) of 20 and 5, respectively, after checking via the gam.check() function in mgcv that these basis functions were sufficiently large to capture the wiggliness in the data (i.e., p-value > 0.05). To account for residual temporal autocorrelation, a continuous-time first-order autoregressive [CAR(1)] process was introduced to models by selecting the corCAR1() function in the correlation argument of the gamm() function. The time series were constructed by using the weighted average of the isotopic values per year and by computing the normalization of these values, as explained by Wood (2017). Model assumptions were verified by observing the plots provided by the gam.check() function (e.g., plotting residuals versus fitted values, etc.).
The carbon and nitrogen isotope ratios of fur seal tissues were compared to those of its main prey in a δ13C-δ15N biplot through the application of trophic discrimination factors (TDFs) to consumer isotopic values. Estimated TDFs from diet to bone reported for adult male fur seals were used (δ15N = +5.1 ‰; δ13C = +3.6 ‰; Vales et al., 2014).
The δ15N and Suess corrected δ13C values of skull bone ranged from +17.5 to +22.8 ‰ (mean = + 20.6 ‰, SD = 0.9) and from −14.7 to −12.6 ‰ (mean = −13.6 ‰, SD = 0.5), respectively (Table 2). Carbon to nitrogen (C:N) atomic ratio ranged from 3.2 to 3.6 (mean = 3.3, SD = 0.1), falling within the expected range (from 2.9 to 3.6) for unaltered bone samples (DeNiro, 1985).
No temporal trend was found in the stable isotope ratios of subadult and adult male fur seals during the period 1990–2017 [δ15N: effective degrees of freedom (edf) = 1, F = 0.008, p-value = 0.929, N = 23; Suess corrected δ13C: edf = 1, F = 0.329, p-value = 0.572, N = 23] (Figures 1C,D). After correcting the δ15N and the Suess corrected δ13C values for diet-to-bone discrimination, the bivariated signal of most subadult and adult male fur seals lay within a polygon formed by shortfin squid, anchovy, Patagonian squid, juvenile hakes, and decapod crustaceans (red shrimp and lobster krill) (Figure 2).
Figure 2. δ13C–δ15N biplot showing the bivariated stable isotope ratios of subadult and adult male South American fur seals during the last 4 decades, after correcting for trophic discrimination factors (TDFs), and their main prey (mean ± SD) in the region of northern and central Patagonia.
The present study reveals no major changes in the trophic ecology of subadult and adult male fur seals in Patagonian waters during at least the past three decades (period 1990–2017), as Oceanic Suess corrected carbon and nitrogen isotope ratios in bone collagen were remarkably stable during this period. Moreover, isotopic values from two individuals collected in 1976 and 1977 suggest that the long-term stability in the diet may have extended further in time. Unfortunately, the gap existing in our isotopic dataset over the period 1978–1989 prevents us from modeling the data beyond the 1990s and assessing this last hypothesis. Nevertheless, in spite of the uneven distribution of the data over time, the 1990–2017 dataset allow us to test potential shifts in the diet of fur seals around 1997 due to the hake stock reduction provoked by the fishery. As a result, the isotope ratios in bone of fur seals, which are expected to reflect the diet over the last years of life of individuals, did not evidence significant changes in the temporal trend. Such temporal stability in the diet of fur seals at first seem surprising, as profound changes have taken place in the marine community of northern and central Patagonia (Koen-Alonso and Yodzis, 2005).
The long-term reconstruction of predator diets through stable isotope analysis requires, in parallel, to account for changes over time in the stable isotope baseline of the ecosystem. This is because any change affecting the stable isotope ratios in primary producers is expected to be transferred upwards in the food web and be reflected in top consumers (Hirons et al., 2001). Unfortunately, δ15N and δ13C baseline time series are not available for Patagonian waters and hence natural environmental changes potentially affecting the isotope signature at the base of the food web over time cannot be considered. On the other hand, the Oceanic Suess effect could be corrected for δ13C values in bone collagen of fur seals by using the equation developed by Verburg (2007) for δ13C values in atmospheric CO2 for the period 1700–2000. This equation was modified in the present study to account for the 10 years lag existing for isotopic equilibration between atmospheric CO2 and oceanic aqueous CO2 (Gruber et al., 1999; Körtzinger et al., 2003; Quay et al., 2003). However, this adjustment in the equation produced no relevant changes in the results, as differences between paired atmospheric and oceanic Suess corrected δ13C values were in the order of 0.004–0.007 ‰. In addition, it is important to note that the dataset presented here spans beyond the year 2000. Nevertheless, δ13C values obtained from the Verburg’s equation during the first two decades of the 21st century agree with the more recent atmospheric δ13C records that extend to the year 2015 (Graven et al., 2017). Therefore, we understand that the Verburg’s equation is a reliable method for correcting the carbon dataset. Using this equation, we estimated the overall Suess effect from 1976 to 2017 in 1.16 ‰. This figure is consistent with the reported by Graven et al. (2017), although is several times higher than the estimation developed by Hilton et al. (2006) to account for the Suess effect in marine predators during the same period (0.11 ‰). Because of this, Suess corrected δ13C values reported in this study for fur seals are not directly comparable with values from other studies using the Hilton’s equation (e.g., Quillfeldt et al., 2010; Mariano-Jelicich et al., 2017).
After accounting for the Oceanic Suess effect, δ13C values in bone collagen of male fur seals showed no temporal trend. This implies that the decreasing trend observed in raw δ13C values reflects the input of anthropogenic CO2 in the marine aquatic environment of the region over time, rather than a dietary shift in fur seals. Likewise, δ15N values did not exhibit any temporal shifts throughout the study period. According to this, male fur seals from northern Patagonia have remained at the same trophic position and have not switched their main prey species over the last four decades. However, this does not mean that the diet of fur seals has not changed at all, because different proportions of assimilated prey may result into similar average stable isotope ratios in the tissues of the predator. Nevertheless, major changes in the relative contribution of pelagic and demersal prey to the diet of fur seals over the Patagonian shelf in the period studied can be ruled out.
Likewise, male fur seals inhabiting the neighbor ecosystem of the Río de la Plata estuary and surroundings had steady δ13C and δ15N values during the period 1994–2011, despite the varying abundance of demersal-benthic prey induced by fisheries in the region (Vales et al., 2014). Nonetheless, such stability throughout time in the stable isotope ratios of fur seals from the Río de la Plata and adjoining waters does not hold when the carbon and nitrogen isotope data set span a longer period of time and shifts in the isotopic baseline are considered. Baseline corrected carbon and nitrogen stable isotope ratios were found to increase significantly in male and female fur seals in the Río de la Plata from 1944 to 2012, probably as a consequence of the increased consumption of high trophic level demersal prey over time (Drago et al., 2017). On the other hand, demersal sciaenid fishes do not abound in the Patagonian waters and the fur seals rely upon other trophic resources available (see below). This may explain in part the different responses of fur seal populations over time between the Río de la Plata and the Patagonian ecosystems.
According to the δ13C–δ15N biplot, the main prey targeted by male fur seals over the last four decades were pelagic squid, small pelagic fishes and decapod crustaceans (Vales et al., 2015; this study). Most of these trophic resources are shared between fisheries and fur seals in the Patagonian region (see section “Introduction”). Nevertheless, the collapse of hake stocks caused by fisheries development seems to have not affected the diet of male fur seals, despite juvenile hakes being one of the main prey of this predator (Vales et al., 2015).
In any case, the long-term stability in the trophic ecology recorded in fur seals from Patagonia is remarkable, as other predators have undergone marked dietary shifts in recent decades. For instance, mature and large individuals of the spiny dogfish are predators feeding mostly of demersal and benthic species (Koen-Alonso et al., 2002). Diet studies on these sharks over the period 1984–1998 indicated a switch from hake as the main prey in 1984–1985 and 1994 to shortfin squids in 1998 (Koen-Alonso et al., 2002). Such a dietary shift was related to the major decrease of the hake population in 1997 due to overfishing and the increase of squid abundance between 1994 and 1999 (Koen-Alonso et al., 2002). In a similar way, yellownose skates that are predators with demersal and benthic feeding habits, switched their diet between 1996–1998 and 2000–2001 periods. The main changes involved a delay in the size at which these skates shift from a carcinophagous diet to a piscivorous diet, and the increase in the consumption of invertebrates to the detriment of fish relevance in diet (Koen-Alonso et al., 2001; Buren et al., 2003; Buren, 2004). For its part, South American sea lions exhibit differences in diet between the sexes, with males feeding mainly on benthic resources, and females consuming more pelagic prey than adult males throughout the year (Drago et al., 2009, 2010). During the period 1974–2002, sea lions revealed a decreasing trend in δ13C values, which was interpreted as a dietary switch from benthic to pelagic prey (Drago et al., 2009). Such a change in diet was less marked in adult females than in adult males, as females had a more pelagic diet through all the study period. In this case, the dietary shift was triggered by the reduction of the hake biomass resulting from overfishing, massive discard of pelagic fish and the sea lion population recovery in the region (Drago et al., 2009). In short, dietary shifts seem to have been more profound in adult male sea lions, less marked in adult female sea lions, and absent in subadult and adult male fur seals; whereas yellownose skates, and mature and large spiny dogfishes, also exhibited some degree of dietary change through time. According to these, we suggest that predators relying on benthic and demersal resources were more prone to suffer dietary shifts than those depending on pelagic prey after the changes induced by fisheries activity in the marine community of northern and central Patagonia. Nevertheless, more long-term diet studies are needed in a greater variety of high trophic level predators to contrast this hypothesis.
All datasets generated for this study are included in the article/supplementary material.
No manipulation of living animals occurred in this study, as bone samples come from fur seals found dead on beaches, and prey samples were obtained from fishery discards. Research authorizations and collection of dead and stranded fur seals were approved by the Argentine Government through the “Secretaría de Turismo” and the “Dirección de Fauna y Flora Silvestre” from the Chubut Province, the “Dirección de Areas Protegidas” from the Río Negro Province, and the “Administración de Parques Naturales” to work in the marine protected area “Parque interjurisdiccional Marino Costero Patagonia Austral.”
DV, LC, and EC conceived and designed the experiments. DV, NG, RL, and EC conducted the fieldwork. DV and RL processed the samples for stable isotope analysis. DV processed the data, performed the statistical analysis and wrote the manuscript with contributions from all authors. DV and LC analyzed the data. All authors gave final approval for publication.
The authors declare that the research was conducted in the absence of any commercial or financial relationships that could be construed as a potential conflict of interest.
This research was funded by the Fundación BBVA (BIOCON 08 – 194/09 2009-2011), the Agencia Nacional de Promoción Científica y Tecnológica (PICT N° 2008-2110 and 2015-2063), the Ministerio de Ciencia, Tecnología e Innovación Productiva – National Research Council of Argentina (CONICET; PIP 11220150100085CO), the Society for Marine Mammalogy Small Grants in Aid of Research (2011–2012), and PADI Foundation (App # 40457). DV would like to thank the CONICET for supporting this research article through a Postdoc Fellowship.
We would like to thank to Florencia Grandi (CESIMAR, CONICET) for her collaboration as reader in the process of age determination, Fabiana Saporiti (University of Barcelona) and Laura Silva (CENPAT, CONICET) for their assistance at lab with sample processing, and Lucas Bandieri (IPCSH, CONICET) for his assistance in R programing and figures design. We would also like to thank the two reviewers for their valuable comments and suggestions that helped greatly to improve the manuscript.
Alemany, D., Iribarne, O. O., and Acha, E. M. (2013). Effects of a large-scale and offshore marine protected area on the demersal fish assemblage in the Southwest Atlantic. ICES J. Mar. Sci. 70, 123–134. doi: 10.1093/icesjms/fss166
Angelescu, V., and Prenski, L. B. (1987). Ecología Trófica de la Merluza Común del Mar Argentino (Merlucciidae. Merluccius hubbsi). Parte 2. Dinámica de la Alimentación Analizada Sobre la Base de las Condiciones Ambientales, la Estructura y las Evaluaciones de Los Efectivos en su Área de Distribución. Mar del Plata: Instituto Nacional de Investigación y Desarrollo Pesquero (INIDEP).
Baylis, A. M., Arnould, J. P., and Staniland, I. J. (2014). Diet of South American fur seals at the Falkland Islands. Mar. Mamm Sci. 30, 1210–1219. doi: 10.1111/mms.12090
Baylis, A. M., Orben, R. A., Arkhipkin, A. A., Barton, J., Brownell, R. L., Staniland, I. J., et al. (2019). Re-evaluating the population size of South American fur seals and conservation implications. Aquat. Conserv. Mar. Freshw. Ecosyst. 29, 1988–1995. doi: 10.1002/aqc.3194
Baylis, A. M., Tierney, M., Staniland, I. J., and Brickle, P. (2018). Habitat use of adult male South American fur seals and a preliminary assessment of spatial overlap with trawl fisheries in the South Atlantic. Mamm. Biol. 93, 76–81. doi: 10.1016/j.mambio.2018.07.007
Bertolotti, M. I., Verazay, G. A., Errazti, E., Pagani, A. N., and Buono, J. J. (2001). “Flota pesquera Argentina. Evolución durante el periodo 1960-1998, con una actualización al 2000,” in El Mar Argentino y sus Recursos Pesqueros. Tomo III: Evolución de la Flota Pesquera Argentina, Artes de Pesca y Dispositivos Selectivos, ed. E. Boschi (Mar del Plata: Publicaciones Especiales INIDEP), 9–53.
Bertuche, D., Fischbach, C., Roux, A., Fernández, M., and Piñero, R. (2000). “Langostino (Pleoticus muelleri),” in Síntesis del Estado de las Pesquerías Marítimas Argentinas y de la Cuenca del Plata. Años 1997–1998, Con Una Actualización de 1999, eds S. I. Bezzi, R. Akselman, and E. Boschi (Mar del Plata: Publicaciones Especiales INIDEP), 179–190.
Brunetti, N. E., Ivanovic, M. L., and Elena, B. (1998). “Calamares omastréfidos (Cephalopoda. Ommastrephidae),” in El Mar Argentino y sus Recursos Pesqueros. Tomo II: Los moluscos de Interés Pesquero. Cultivos y Estrategias Reproductivas de Bivalvos y Equinoideos, ed. E. Boschi (Mar del Plata: Publicaciones Especiales INIDEP), 37–68.
Bunn, S. E., Loneragan, N. R., and Kempster, M. A. (1995). Effects of acid washing on stable isotope ratios of C and N in penaeid shrimp and seagrass: implications for food-web studies using multiple stable isotopes. Limnol. Oceanogr. 40, 622–625. doi: 10.4319/lo.1995.40.3.0622
Buren, A. D. (2004). Dieta de la Raya Picuda, Dipturus Chilensis, en Aguas del Norte y Centro de Patagonia Durante el Período 2000—2001. Degree dissertation, Universidad Nacional de la Patagonia San Juan Bosco – Sede Puerto Madryn, Puerto Madryn.
Buren, A. D., Koen-Alonso, M., Pedraza, S. N., Crespo, E. A., García, N. A. (2003). “Cambios en la dieta de la raya picuda (Dipturus flavirostris) en dos períodos de los últimos diez años,” in Abstract Book V Jornadas Nacionales de Ciencias del Mar, 8th-12th December 2003, eds Boschi, E. E., Bremec, C. S., Cousseau, M. B., Elías, R., and Roux, A. M.(Mar del Plata: Universidad Nacional de Mar del Plata / INIDEP).
Ciancio, J. E., Pascual, M. A., Botto, F., Frere, E., and Iribarne, O. (2008). Trophic relationships of exotic anadromous salmonids in the southern Patagonian Shelf as inferred from stable isotopes. Limnol. Oceanogr. 53, 788–798. doi: 10.2307/40006459
Crespo, E. A., García, N. A., Dans, S., and Pedraza, S. N. (2008). “Mamíferos marinos. Arctocephalus australis,” in Atlas de Sensibilidad Ambiental de la Costa y el Mar Argentino (Proyecto ARG 02/018 Conservación de la Diversidad Biológica y Prevención de la Contaminación Marina en Patagonia), ed. D. Boltovskoy (Buenos Aires: Secretaría de Ambiente y Desarrollo Sustentable de la Nación), 1–9.
Crespo, E. A., Oliva, D., Dans, S. L., and Sepúlveda, M. (2012). Current Situation of the South American sea Lion Along the Distribution Range. Valparaíso: Universidad de Valparaíso Press.
Crespo, E. A., Pedraza, S. N., Dans, S. L., Koen-Alonso, M., Reyes, L. M., García, N. A., et al. (1997). Direct and indirect effects of the highseas fisheries on the marine mammal populations in the northern and central Patagonian coast. J. Northw. Atl. Fish. Sci. 22, 189–207. doi: 10.2960/J.v22.a15
Crespo, E. A., Schiavini, A. C., García, N. A., Franco-Trecu, V., Goodall, R. N. P., Rodríguez, D., et al. (2015). Status, population trend and genetic structure of South American fur seals, Arctocephalus australis, in southwestern Atlantic waters. Mar. Mamm. Sci. 31, 866–890. doi: 10.1111/mms.12199
Crespo, E. A., Schiavini, A. C. M., Pérez Macri, G., Reyes, L., and Dans, S. (1994). “Estudios sobre determinación de edad en mamíferos marinos del Atlántico Sudoccidental,” in Anales IV Reunión de Trabajo de Especialistas en Mamíferos Acuáticos de América del Sur, ed. J. A. Oporto (Valdivia: Centro de Investigaciones y Manejo de Mamíferos Marinos), 31–55.
Croxall, J. P., and Wood, A. G. (2002). The importance of the Patagonian Shelf for top predator species breeding at South Georgia. Aquat. Conserv. Mar. Freshw. Ecosyst. 12, 101–118. doi: 10.1002/aqc.480
Dalerum, F., and Angerbjörn, A. (2005). Resolving temporal variation in vertebrate diets using naturally occurring stable isotopes. Oecologia 144, 647–658. doi: 10.1007/s00442-005-0118-0
Dassis, M., Farenga, M., Bastida, R., and Rodríguez, D. (2012). At-sea behavior of South American fur seals: influence of coastal hydrographic conditions and physiological implication. Mamm. Biol. 77, 47–52. doi: 10.1016/j.mambio.2011.07.003
DeNiro, M. J. (1985). Postmortem preservation and alteration of in vivo bone Influence of diet on the distribution of nitrogen isotopes in animals collagen isotope ratios in relation to palaeodietary reconstruction. Nature 317, 806–809. doi: 10.1038/317806a0
DeNiro, M. J., and Epstein, S. (1978). Influence of diet on the distribution of carbon isotopes in animals. Geochim. Cosmochim. Acta 42, 495–506. doi: 10.1016/0016-7037(78)90199-0
DeNiro, M. J., and Epstein, S. (1981). Influence of diet on the distribution of nitrogen isotopes in animals. Geochim. Cosmochim. Acta 45, 341–351. doi: 10.1016/0016-7037(81)90244-1
Drago, M., Cardona, L., Crespo, E. A., García, N., Ameghino, S., and Aguilar, A. (2010). Change in the foraging strategy of female South American sea lions (Carnivora: Pinnipedia) after parturition. Sci. Mar. 74, 589–598. doi: 10.3989/scimar.2010.74n3589
Drago, M., Cardona, L., Franco-Trecu, V., Crespo, E. A., Vales, D. G., Borella, F., et al. (2017). Isotopic niche partitioning between two apex predators over time. J. Anim. Ecol. 86, 766–780. doi: 10.1111/1365-2656.12666
Drago, M., Crespo, E. A., Aguilar, A., Cardona, L., García, N., Dans, S. L., et al. (2009). Historic diet change of the South American sea lion in Patagonia as revealed by isotopic analysis. Mar. Ecol. Prog. Ser. 384, 273–286. doi: 10.3354/meps08017
Favero, M., and Silva Rodríguez, M. P. (2005). Estado actual y conservación de aves pelágicas que utilizan la plataforma continental argentina como área de alimentación. Hornero 20, 95–110.
Ferrari, M. A., Campagna, C., Condit, R., and Lewis, M. N. (2013). The founding of a southern elephant seal colony. Mar. Mamm. Sci. 29, 407–423. doi: 10.1111/j.1748-7692.2012.00585.x
Folch, J., Lees, M., and Stanley, G. H. S. (1957). A simple method for the isolation and purification of total lipides from animal tissues. J. Biol. Chem. 226, 497–509.
Forero, M. G., Bortolotti, G. R., Hobson, K. A., Donazar, J. A., Bertelloti, M., and Blanco, G. (2004). High trophic overlap within the seabird community of Argentinean Patagonia: a multiscale approach. J. Anim. Ecol. 73, 789–801. doi: 10.1111/j.0021-8790.2004.00852.x
France, R. L. (1995). Carbon-13 enrichment in benthic compared to planktonic algae: foodweb implications. Mar. Ecol. Prog. Ser. 124, 307–312. doi: 10.3354/meps124307
Franco-Trecu, V., Aurioles-Gamboa, D., and Inchausti, P. (2014). Individual trophic specialisation and niche segregation explain the contrasting population trends of two sympatric otariids. Mar. Biol. 161, 609–618. doi: 10.1007/s00227-013-2363-9
Gandini, P. A., Frere, E., Pettovello, A. D., and Cedrola, P. V. (1999). Interaction between Magellanic penguins and shrimp fisheries in Patagonia, Argentina. Condor 101, 783–789. doi: 10.2307/1370065
Góngora, M. E., González-Zevallos, D., Pettovello, A., and Mendía, L. (2012). Caracterización de las principales pesquerías del golfo San Jorge Patagonia, Argentina. Lat. Am. J. Aquat. Res. 40, 1–11. doi: 10.3856/vol40-issue1-fulltext-1
Grandi, M. F., Dans, S. L., and Crespo, E. A. (2015). The recovery process of a population is not always the same: the case of Otaria flavescens. Mar. Biol. Res. 11, 225–235. doi: 10.1080/17451000.2014.932912
Graven, H., Allison, C. E., Etheridge, D. M., Hammer, S., Keeling, R. F., Levin, I., et al. (2017). Compiled records of carbon isotopes in atmospheric CO2 for historical simulations in CMIP6. Geosci. Model Dev. 10, 4405–4417. doi: 10.5194/gmd-10-4405-2017
Gruber, N., Keeling, C. D., Bacastow, R. B., Guenther, P. R., Lueker, T. J., Wahlen, M., et al. (1999). Spatiotemporal patterns of carbon-13 in the global surface oceans and the oceanic Suess effect. Glob. Biogeochem. Cycles 13, 307–335. doi: 10.1029/1999GB900019
Hansen, J. E. (2000). “Anchoíta (Engraulis anchoita),” in Síntesis del Estado de Las Pesquerías Marítimas Argentinas y de la Cuenca del Plata. Años 1997–1998, con Una Actualización de 1999, eds S. I. Bezzi, R. Akselman, and E. Boschi (Mar del Plata: Publicaciones Especiales INIDEP), 205–216.
Heileman, S. (2009). “XVI-55 Patagonian Shelf LME,” in The UNEP Large marine Ecosystem Report: A Perspective On Changing Conditions in LMES of the World’s Regional Seas. UNEP Regional Seas Report and Studies No. 182. United Nations Environment Programme, eds K. Sherman and G. Hempel (Nairobi: UNDP), 735–746.
Hilton, G. M., Thompson, D. R., Sagar, P. M., Cuthbert, R. J., Cherel, Y., and Bury, S. J. (2006). A stable isotopic investigation into the causes of decline in a sub-Antarctic predator, the rockhopper penguin Eudyptes chrysocome. Glob. Change Biol. 12, 611–625. doi: 10.1111/j.1365-2486.2006.01130.x
Hirons, A. C., Schell, D. M., and Finney, B. P. (2001). Temporal records of δ13C and δ15N in North Pacific pinnipeds: inferences regarding environmental change and diet. Oecologia 129, 591–601. doi: 10.1007/s004420100756
Hobson, K. A., Piatt, J. F., and Pitocchelli, J. (1994). Using stable isotopes to determine seabird trophic relationships. J. Anim. Ecol. 63, 786–798. doi: 10.2307/5256
Keeling, C. D. (1979). The suess effect: 13 Carbon and 14 Carbon interactions. Environ. Int. 2, 229–300. doi: 10.1016/0160-4120(79)90005-9
Koen-Alonso, M., Crespo, E. A., García, N. A., Pedraza, S. N., Mariotti, P. A., and Mora, N. J. (2002). Fishery and ontogenetic driven changes in the diet of the spiny dogfish, Squalus acanthias, in Patagonian waters, Argentina. Environ. Biol. Fishes 63, 193–202. doi: 10.1023/A:1014229432375
Koen-Alonso, M., Crespo, E. A., García, N. A., Pedraza, S. N., Mariotti, P. A., Vera, B. B., et al. (2001). Food habits of Dipturus chilensis (Pisces: Rajidae) off Patagonia, Argentina. ICES J. Mar. Sci. 58, 288–297. doi: 10.1006/jmsc.2000.1010
Koen-Alonso, M., and Yodzis, P. (2005). Multispecies modelling of some components of the marine community of northern and central Patagonia, Argentina. Can. J. Fish. Aquat. Sci. 62, 1490–1512. doi: 10.1139/f05-087
Körtzinger, A., Quay, P. D., and Sonnerup, R. E. (2003). Relationship between anthropogenic CO2 and the 13C suess effect in the North Atlantic Ocean. Glob. Biogeochem. Cycles 17, 5-1–5-20. doi: 10.1029/2001GB001427
Laws, E. A., Popp, B. N., Bidigare, R. R., Kennicutt, M. C., and Macko, S. A. (1995). Dependence of phytoplankton carbon isotopic composition on growth rate and [CO2]aq: theoretical considerations and experimental results. Geochim. Cosmochim. Acta 59, 1131–1138. doi: 10.1016/0016-7037(95)00030-4
Lee-Thorp, J. A., Sealy, J. C., and Van Der Merwe, N. J. (1989). Stable carbon isotope ratio difference between bone collagen and bone apatite, and their relationship to diet. J. Archaeol. Sci. 16, 585–599. doi: 10.1016/0305-4403(89)90024-1
Lorrain, A., Savoye, N., Chauvaud, L., Paulet, Y. M., and Naulet, N. (2003). Decarbonation and preservation method for the analysis of organic C and N contents and stable isotope ratios of low-carbonated suspended particulate material. Anal. Chim. Acta 491, 125–133. doi: 10.1016/S0003-2670(03)00815-8
Mandiola, M. A., Giardino, G. V., Bastida, J., Rodríguez, D. H., and Bastida, R. O. (2015). Marine mammal occurrence in deep waters of the Brazil-Malvinas confluence off Argentina during summer. Mastozool. Neotrop. 22, 397–402.
Mariano-Jelicich, R., Copello, S., Pon, J. P. S., and Favero, M. (2017). Long-term changes in Black-browed albatrosses diet as a result of fisheries expansion: an isotopic approach. Mar. Biol. 164:148. doi: 10.1007/s00227-017-3176-z
Mateo, J. A. (2015). Gringos Que Montaban Olas. Historia de la Pesca Costera en Argentina. Mar del Plata: GESMar.
Minagawa, M., and Wada, E. (1984). Stepwise enrichment of 15N along food chains: further evidence and the relation between δ15N and animal age. Geochim. Cosmochim. Acta 48, 1135–1140. doi: 10.1016/0016-7037(84)90204-7
Mizutani, H., and Wada, E. (1982). Effects of high atmospheric CO2 on δ13C of algae: a possible cause for the average depletion of 13C in Precambrian reduced carbon. Orig. Life Evol. B 12, 377–390. doi: 10.1007/BF00927070
Molina-Schiller, D., and Pinedo, M. C. (2004). Growth layer patterns in Arctocephalus australis canine teeth: evaluation of techniques for age determination. Lat. Am. J. Aquat. Mamm. 3, 107–118. doi: 10.5597/lajam00056
MPyT-SA (2020). Ministerio de Producción y Trabajo Presidencia de la Nación (República Argentina). Available at: https://www.agroindustria.gob.ar/sitio/areas/pesca_maritima/desembarques/ (accessed January 18, 2020).
Navarro, G., Rozycki, V., and Monsalvo, M. (2014). Estadísticas de la Pesca Marina en la Argentina: Evolución de los Desembarques 2008-2013. Buenos Aires: Ministerio de Agricultura, Ganadería y Pesca de la Nación.
Navarro, G., Rozycki, V., and Monsalvo, M. (2019). Estadísticas de la Pesca Marina en la Argentina: Evolución de los Desembarques 2012-2016. Buenos Aires: Secretaría de Gobierno de Agroindustria. Secretaría de Agricultura, Ganadería y Pesca de la Nación.
Naya, D. E., Arim, M., and Vargas, R. (2002). Diet of South American fur seals (Arctocephalus australis) in Isla de Lobos, Uruguay. Mar. Mamm. Sci. 18, 734–745. doi: 10.1111/j.1748-7692.2002.tb01070.x
Newsome, S. D., Clementz, M. T., and Koch, P. L. (2010). Using stable isotope biogeochemistry to study marine mammal ecology. Mar. Mamm. Sci. 26, 509–572. doi: 10.1111/j.1748-7692.2009.00354.x
Newsome, S. D., Koch, P. L., Etnier, M. A., and Aurioles-Gamboa, D. (2006). Using carbon and nitrogen isotope values to investigate maternal strategies in northeast Pacific otariids. Mar. Mamm. Sci. 22, 556–572. doi: 10.1111/j.1748-7692.2006.00043.x
Oliveira, L. R., Ott, P. H., and Malbarba, L. R. (2008). “Ecologia alimentar dos pinípedes do sul do Brasil e uma avaliação de suas interações com atividades pesqueiras,” in Ecologia de Mamíferos, eds N. R. Reis, A. L. Peracchi, and G. A. S. D. Santos (Londrina: Technical Books Editora), 97–116.
Post, D. M. (2002). Using stable isotopes to estimate trophic position: models, methods, and assumptions. Ecology 83, 703–718. doi: 10.1890/0012-9658(2002)083%5B0703:usitet%5D2.0.co;2
Quay, P., Sonnerup, R., Westby, T., Stutsman, J., and McNichol, A. (2003). Changes in the 13C/12C of dissolved inorganic carbon in the ocean as a tracer of anthropogenic CO2 uptake. Glob. Biogeochem. Cycles 17:1004. doi: 10.1029/2001GB001817
Quillfeldt, P., Masello, J. F., McGill, R. A., Adams, M., and Furness, R. W. (2010). Moving polewards in winter: a recent change in the migratory strategy of a pelagic seabird? Front. Zool. 7:15. doi: 10.1186/1742-9994-7-15
R Development Core Team (2019). R: A Language and Environment for Statistical Computing. Vienna: R Foundation for Statistical Computing.
Raya Rey, A., Rosciano, N., Liljesthröm, M., Samaniego, R. S., and Schiavini, A. (2014). Species-specific population trends detected for penguins, gulls and cormorants over 20 years in sub-Antarctic Fuegian Archipelago. Polar Biol. 37, 1343–1360. doi: 10.1007/s00300-014-1526-6
Riofrío-Lazo, M., and Aurioles-Gamboa, D. (2013). Timing of isotopic integration in marine mammal skull: comparative study between calcified tissues. Rapid Commun. Mass Spectrom. 27, 1076–1082. doi: 10.1002/rcm.6556
Romero, M. A., Dans, S. L., García, N., Svendsen, G. M., González, R., and Crespo, E. A. (2012). Feeding habits of two sympatric dolphin species off North Patagonia, Argentina. Mar. Mamm. Sci. 28, 364–377. doi: 10.1111/j.1748-7692.2011.00477.x
Sánchez, R. P., Navarro, G., and Rozycki, V. (2012). Estadísticas de la Pesca Marina en la Argentina. Evolución de los Desembarques 1898-2010. Buenos Aires: Ministerio de Agricultura, Ganadería y Pesca de la Nación.
Saporiti, F., Bearhop, S., Silva, L., Vales, D. G., Zenteno, L., Crespo, E. A., et al. (2014). Longer and less overlapping food webs in anthropogenically disturbed marine ecosystems: confirmations from the past. PLoS One 9:e103132. doi: 10.1371/journal.pone.0103132
Schiavini, A. C., Lima, M., and Batalles, L. M. (1992). Growth structures of maxillary canines of the southern fur seal (Arctocephalus australis). Mar. Mamm. Sci. 8, 89–93. doi: 10.1111/j.1748-7692.1992.tb00130.x
Schiavini, A. C., Yorio, P., Gandini, P., Raya Rey, A., and Boersma, P. D. (2005). Los pingüinos de las costas argentinas: estado poblacional y conservación. Hornero 20, 5–23.
Schoeninger, M. J., and DeNiro, M. J. (1984). Nitrogen and carbon isotopic composition of bone collagen from marine and terrestrial animals. Geochim. Cosmochim. Acta 48, 625–639. doi: 10.1016/0016-7037(84)90091-7
Simpson, G. L. (2018). Modelling palaeoecological time series using generalized additive models. Front. Ecol. Evol. 6:149. doi: 10.3389/fevo.2018.00149
Szteren, D., Naya, D. E., and Arim, M. (2004). Overlap between pinniped summer diet and artisanal fishery catches in Uruguay. Lat. Am. J. Aquat. Mamm. 3, 119–125. doi: 10.5597/lajam00057
Vales, D. G., Cardona, L., García, N. A., Zenteno, L., and Crespo, E. A. (2015). Ontogenetic dietary changes in male South American fur seals Arctocephalus australis in Patagonia. Mar. Ecol. Prog. Ser. 525, 245–260. doi: 10.3354/meps11214
Vales, D. G., Saporiti, F., Cardona, L., De Oliveira, L. R., Dos Santos, R. A., Secchi, E. R., et al. (2014). Intensive fishing has not forced dietary change in the South American fur seal Arctophoca (=Arctocephalus) australis off Río de la Plata and adjoining areas. Aquat. Conserv. Mar. Freshw. Ecosyst. 24, 745–759. doi: 10.1002/aqc.2397
Vargas Hernández, C. A. (2012). Hábitos Alimentarios del Lobo Fino Austral (Arctocephalus australis) en la Isla Guafo Durante las Temporadas Reproductivas de 2010 y 2012. Degree dissertation, Universidad Austral de Chile, Valdivia.
Varisco, M., and Vinuesa, J. H. (2007). Diet of Munida gregaria (Fabricius, 1793) (Crustacea: Anomura: Galatheidae) in fishing beds of the San Jorge Gulf, Argentina. Rev. Biol. Mar. Oceanogr. 42, 221–229. doi: 10.4067/S0718-19572007000300002
Verburg, P. (2007). The need to correct for the Suess effect in the application of δ13C in sediment of autotrophic Lake Tanganyika, as a productivity proxy in the Anthropocene. J. Paleolimnol. 37, 591–602. doi: 10.1007/s10933-006-9056-z
von Zimmermann, E. A. W. (1783). Geographische Geshichte der Menschen, und der Alge Meiss Verbreiteten vierfEURussigen Thiere, 2nd Edn. Vol. 3 (Leipzig: Wenganschen Buchhandlung), 1778–1783.
Wood, S. N. (2017). Generalized Additive Models: An Introduction with R, 2nd Edn, Boca Raton, FL: C Chapman & Hall/CRC Texts in Statistical Science.
Keywords: high trophic level marine predator, Patagonian shelf, South American fur seals, dietary reconstruction, stable isotope analysis, δ13C, δ15N, collagen
Citation: Vales DG, Cardona L, Loizaga R, García NA and Crespo EA (2020) Long-Term Stability in the Trophic Ecology of a Pelagic Forager Living in a Changing Marine Ecosystem. Front. Mar. Sci. 7:87. doi: 10.3389/fmars.2020.00087
Received: 11 October 2019; Accepted: 03 February 2020;
Published: 20 February 2020.
Edited by:
Lyne Morissette, M – Expertise Marine, CanadaReviewed by:
Rebecca Ruth McIntosh, Phillip Island Nature Parks, AustraliaCopyright © 2020 Vales, Cardona, Loizaga, García and Crespo. This is an open-access article distributed under the terms of the Creative Commons Attribution License (CC BY). The use, distribution or reproduction in other forums is permitted, provided the original author(s) and the copyright owner(s) are credited and that the original publication in this journal is cited, in accordance with accepted academic practice. No use, distribution or reproduction is permitted which does not comply with these terms.
*Correspondence: Damián G. Vales, dmFsZXNAY2VucGF0LWNvbmljZXQuZ29iLmFy
Disclaimer: All claims expressed in this article are solely those of the authors and do not necessarily represent those of their affiliated organizations, or those of the publisher, the editors and the reviewers. Any product that may be evaluated in this article or claim that may be made by its manufacturer is not guaranteed or endorsed by the publisher.
Research integrity at Frontiers
Learn more about the work of our research integrity team to safeguard the quality of each article we publish.