- 1Negaunee Integrative Research Center, Field Museum of Natural History, Chicago, IL, United States
- 2Committee on Evolutionary Biology, The University of Chicago, Chicago, IL, United States
- 3School of Biological Sciences, Washington State University, Pullman, WA, United States
Deep-sea wood-boring xylophagaid bivalves are thought to ingest only wood and to use nitrogen fixed by their symbiotic microbes. Reconsidering this assumption, we tested whether δ13C and δ15N isotopic values of ten species in four xylophagaid genera collected between 18 m and 4626 m depth suggest that some may use different trophic strategies. Isotopic signatures of six species were entirely consistent with predicted xylophagy, but four species, three members of the Xylophaga dorsalis clade and Abditoconus heterosiphon, had δ15N signatures over 3.7‰, significantly higher than the value predicted for nitrogen fixed by bacteria. These species may supplement freshly fixed nitrogen with an alternate source, such as particulate organic material. Although the animals’ reduced palps and the lack of microbes in the gut of laboratory-maintained specimens were cited as arguing against filter feeding, the animals may filter feed opportunistically. A. heterosiphon was unique in having δ13C signatures more negative than the wood into which they bored, inconsistent with cellulose consumption, and δ15N values higher than predicted for nitrogen fixed by bacteria. We suggest that greater trophic diversity may exist among xylophagaids than has been expected. Ecological studies of wood-fall communities cannot assume that the entire community is sustained only by wood-bound energy. We attribute variation in δ13C signatures among 42 specimens of X. s.l. zierenbergi from six collections, five at the same depth, to variation in the type of wood bored. Investigators beginning food web studies should sample xylophagaids and wood itself; if the deployment is large, repeated samples of the wood, closely matched to xylophagaids, may be necessarily to fully define the substrate’s stable isotopic signal.
Introduction
Wood-boring bivalves of the Xylophagaidae are thought to bore into, ingest and digest sunken wood (Distel and Roberts, 1997); in doing so they are thought to sustain diverse communities associated with wood that has sunken to the deep-sea floor (Turner, 1973). The importance of the bivalves in making the wood’s energy and nutrients accessible to other animals has lately been questioned; bacteria may play a larger role than has been assumed (e.g. Kalenitchenko et al., 2018). This may be symptomatic of how little we know about xylophagaids; the composition of their diet has been poorly documented, but wood is widely accepted to be their main food source.
Anatomy has contributed to the belief that xylophagaids are strict xylophages. Palp size may predict filter feeding ability in teredinids (Saraswathy and Nair, 1971), the sister taxon of the xylophagaids (Distel et al., 2011). Xylophaga dorsalis has reduced palps and gills that lack strong sorting mechanisms (Purchon, 1941), features seemingly indicative of reduced filter feeding ability. However, xylophagaids that bored into the gutta-percha cover of transatlantic cables (Web, 1900) would have had to filter feed (Purchon, 1941), as would those that bored into plastics and vinyl (Muraoka, 1967). Those that were recovered from plastics were, however, half the size of those recovered from wood deployed on the same rack at 722 m for 13.4 months at about 5°C (Muraoka, 1967). These data suggest that, while possible, life without wood is less than ideal. Ansel and Nair (1969) contended, without citing supporting data, that like their relatives the boring pholadids, xylophagaids use wood for support and protection rather than for food.
More recent, data-based arguments for the animals’ strict reliance on xylophagy include that individuals of Xylophaga washingtona that had been held in wood in seawater tanks had few microorganisms among the wood shavings in their gut (Distel and Roberts, 1997). The gills of the two xylophagaid species (X. washingtona and Xylophaga s.l. atlantica) examined supported dense endosymbiotic bacteria, thought to help digest wood as they do in teredinids (Sabbadin et al., 2018). Teredinids appear able to survive by xylophagy, but studies using radio-labeled substrates and δ13C and δ15N isotopic analyses find some teredinids, e.g. Lyrodus pedicellatus, Teredo navalis, Bankia carinata, filter feed at least opportunistically (Pechenik et al., 1979; Gallager et al., 1981; Paalvast and van der Velde, 2013; Charles et al., 2018). Karande et al. (1968), cited by Pechenik et al. (1979) stated that T. fucifera required phytoplankton to grow and reproduce.
Of five studies of xylophagaid δ13C and δ15N stable isotopic signatures (Nishimoto et al., 2009; Bernardino et al., 2010; Yamanaka et al., 2015; Gaudron et al., 2016; Zapata-Hernández et al., 2016), only Gaudron et al. (2016) postulated xylophagaid filter feeding. Dwarf males of Xylophaga s.l. atlantica removed from wood that had been deployed at 2279 m depth near hydrothermal vents on the Mid-Atlantic Ridge had enriched δ13C and δ15N isotopic values. In this dense population, dwarf males were concluded to filter feed on fecal matter and other by-products of large, autonomously boring females.
In terms of δ13C isotopic values, strictly xylophagous xylophagaids are predicted to be 1–2‰ enriched, that is more positive, than the wood they ate, and that the fecal chimneys of members of the X. dorsalis clade would be depleted, or more negative than wood. Because the bivalves digest cellulose (Purchon, 1941; Nishimoto et al., 2009), their fecal chimneys would be composed mainly of lignin. The δ13C signature of cellulose is enriched relative to that of lignin (Loader et al., 2003). If they ingested sulfide-oxidizing bacteria, their δ13C isotopic values may be depleted relative to the wood. Wood, however, is less than ideal for stable isotopic studies. Even within an individual tree, wood can have a surprisingly large range of δ13C signatures (e.g. Leavitt and Long, 1986; Schleser et al., 2015). Weather, age, heartwood versus sapwood, water stress, even sunlight exposure all reportedly affect wood’s δ13C signature by over 2‰ (e.g. Bert et al., 1997; Korol et al., 1999; Loader et al., 2003; Taylor et al., 2007).
In terms of δ15N isotopic values, strictly xylophagous xylophagaids are predicted to have mean δ15N values from −2 to + 3‰. The extremely low nitrogen concentrations in wood, 0.08–0.2% (Filipiak, 2018), force xylophagous animals to get supplemental nitrogen. To do so, xylophagaids are thought to rely on bacteria symbiotic in their gills (Distel and Roberts, 1997). In addition to their physical presence, two bacterial species from the gills of X. dorsalis show a 95% genetic similarity to bacteria in the gills of teredinids that are known to fix nitrogen; other bacterial species that are more distantly affiliated with teredinid symbionts occur in other members of X. dorsalis (Fagervold et al., 2014). Stable isotopic signatures of nitrogen fixed by bacteria are between −2 and 0‰ (Somes et al., 2010). Ingestion of symbionts or recycling of nitrogen (Ferrier-Pagés and Leal, 2019) could expand the range of δ15N values in xylophages to −2 to + 3‰, assuming conventional trophic enrichment of 3.4 ± 1.1‰ (Minagawa and Wada, 1984). Values exceeding 3‰ suggest use of a different nitrogen source, such as Particulate Organic Material (POM).
To test whether xylophagaids rely exclusively on xylophagy and nitrogen-fixing bacteria, we apply δ13C and δ15N stable isotopic analysis to 10 xylophagaid species from four clades collected from 18 to 4626 m depths. If a species δ13C value is 1–2‰ enriched relative to the wood it bored and its δ15N is between −2 and 3‰, it will be viewed as xylotrophic, the null hypothesis. Species that use POM would be revealed by enriched δ15N values; species with depleted δ13C values compared to the wood it bored might ingest sulfide-oxidizing bacteria. We also report analyses of six collections of one species. With stable isotopic studies of xylophagaids so rare, and often with no or unreliable species identifications, we hope to provide a framework for future studies of these animals.
Materials and Methods
Specimens and Wood Analyzed
Seventy-one specimens from ten localities deposited in Field Museum of Natural History (FMNH) collection and one specimen from TePapa National Museum of New Zealand (NMNZ) collection are the basis of this study (Table 1). Of these, 42 are members of Xylophaga s.l. zierenbergi removed from six deployments. The specimens differed in their source, whether from wood randomly encountered on the seafloor (i.e. “wild” wood), or wood experimentally deployed on or above the sediment and in their preservation histories. Fixation in formalin followed by storage in 70% ethanol or preservation and storage in 95% ethanol were the alternative means of preservation.
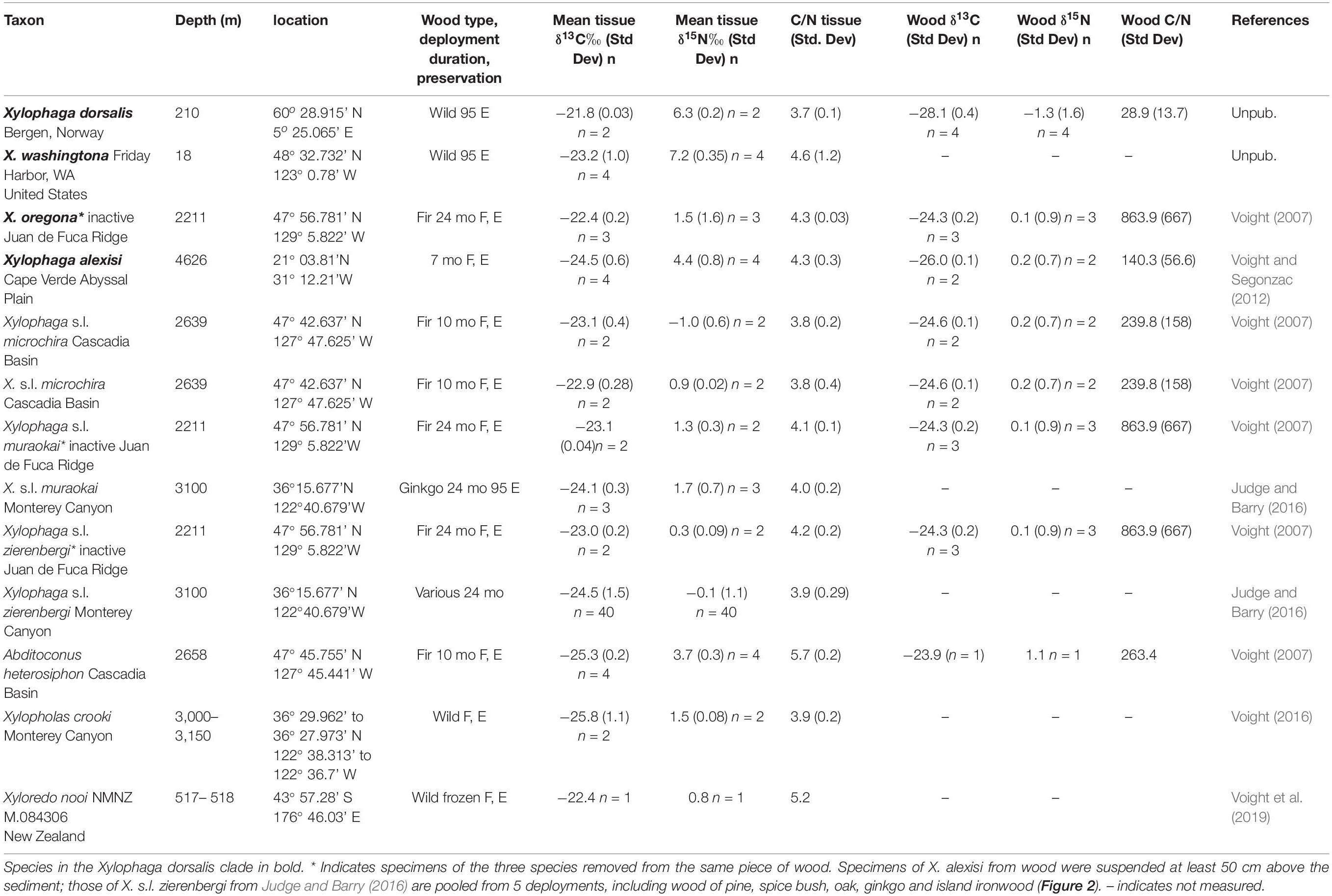
Table 1. The taxon, depth, locality, wood type, deployment duration (if applicable), preservation (F, E formalin to ethanol; 95 E 95% ethanol), mean tissue δ13C value (standard deviation) and number of specimens, mean tissue δ15N value (standard deviation) and number of specimens, mean tissue C/N ratio (standard deviation), mean wood δ13C value (standard deviation), mean wood δ15N value (Standard deviation), wood C/N ratio (standard deviation) and the source of these specimens.
We must address two taxonomic issues. First, the genus Xylophaga is paraphyletic (Voight et al., 2019). The type species of the genus, X. dorsalis, forms a clade with several species included here (X. washingtona, X. oregona, X. alexisi). The other species named in the genus do not share a recent common ancestor with the name-bearing clade. To highlight their independent origin, despite the shared genus name, we apply “s.l.” to their names. Second, when citing literature reports, we used the species names assigned in the original paper, except for Zapata-Hernández et al. (2016). They applied the name of the North Atlantic species, X. dorsalis, to their Chilean specimens; we use instead the name X. globosa, erected for a species from Valparaiso, Chile, about 8° north of the collection site.
Where available, the stable isotopic values of the wood into which the animals bored were determined. Wood’s very low nitrogen concentration, roughly 0.1% that in insect bodies (Filipiak and Weiner, 2014), not only forces xylophages to find an alternate nitrogen source, it made accurately determining its δ15N signature extremely difficult in our laboratory. The nitrogen values we report from wood must be considered with caution. POM from our collection localities are not available. Globally, average deep-sea heterotrophic animals have isotopic values ranging from 10–13‰ for δ15N and −17 to −21‰ for δ13C, depending on latitude (reviewed in Parzanini et al., 2019). POM data from the literature show elevated values compared with nitrogen fixed by bacteria. Examples are: tropical Atlantic (POM 300 m depth 6–8‰, Montoya et al., 2002), North Sea and Coastal Norway (benthic POM average 6‰, Silberberger et al., 2019), Northeast Pacific (surface POM average 8‰, Altabet et al., 1999).
Specimen Preparation
Wood, bivalves and fecal chimneys were sampled with a sterile scalpel; the samples were air-dried or placed in 95% ethanol then air-dried. Samples (0.3–0.7 mg dry weight) were rinsed in distilled water, dried, then packaged in tin capsules for mass spectrometry, and analyzed using a Costech (Valencia, CA, United States) elemental analyzer interfaced with a continuous flow Micromass Isoprime isotope ratio mass spectrometer (EA-IRMS) for 13C/12C and 15N/14N ratios. Measurements are reported in δ notation [per mil (‰)] and ovalbumin was used as a routine standard. Precision for δ13C and δ15N was ± 0.2‰ and ± 0.4‰ (S.D. of 10 replicate standards), respectively.
We analyzed the siphon, gills, mantle and cecum of specimens of X. dorsalis individually to test for organ-specific biases. The wood-filled cecum had a δ13C isotopic value reflecting its contents, being 2‰ depleted compared to the muscle and gills which at −21.7‰ and −22.0‰ were indistinguishable given our precision. Gills had the lowest δ15N value at 4.3‰, the cecum was intermediate at 5.1‰ and the muscle was 6.1‰. Complete data are reported in Supplementary Table S1. We opted to use the siphons for the remaining analyses due to their ease of accessibility and assumed comparability.
Data Analyses
We compared the mean δ13C isotopic signatures of seven bivalve species to those of subsamples of the wood into which they had bored; wood samples were lacking for the other taxa. For X. dorsalis and X. oregona, we also compared the δ13C and δ15N isotopic signatures of the chimneys to those of tissue and wood. To examine variation with depth, we plotted the mean δ15N values of each species versus the species’ collection depth, pooling the five Monterey Canyon collections of X. s.l. zierenbergi. We calculated the correlation coefficient in Excel©, both with and without the relatively shallow species.
Small within-species sample sizes limited the ability of statistical tests to assess the significance of differences. We calculated 95% confidence intervals of the mean nitrogen isotopic values of each species in Excel©, after pooling species from multiple sites. Resultant intervals that did not overlap are thus significantly different at p < 0.05 (Zar, 1999).
We compared the δ13C isotopic values and the δ15N isotopic values of our chemically preserved specimens to isotopic values from literature accounts that were based on frozen tissues, using the rank sum test calculated by hand.
Because multiple variables impact each sample, we performed multiple tests in R 3.6.1 (R Core Team, 2019), as described here. Using clade, depth, δ13C, wood type, taxon, and preservation method, we assessed variable importance for determining δ15N using Random Forests in the package “ranger” (Wright and Ziegler, 2017), manipulating our data using the packages “data.table” (Dowle and Srinivasan, 2019) and “tidyverse” (Wickham, 2017) with reference to the Random Forest pipeline of Johnston et al. (2019). Data were visualized using “ggplot2” (Wickham, 2016). To assure that phylogeny was not overly biasing our data, we compared the nitrogen isotopic values for species that were included in a phylogenetic analysis (Voight et al., 2019) using a phylogenetically corrected ANOVA in the R package “phytools” (Revell, 2012). The phylogenetic tree was pruned to include only species represented in the isotopic analysis; each species then had one tip randomly selected to provide a phylogenetic distance for the test. Lastly, non-parametric Kruskal-Wallis tests were performed using the R function “kruskal.test” to determine significant differences between different variable combinations. Notably, we used this to assess how chemical preservation affected the stable isotopic signatures independent of species membership. Complete code for the analyses is presented in Supplementary Information.
Results
The δ13C values for the 72 individual xylophagaids analyzed ranged from −26.6 to −21.5‰; their δ15N values ranged from −2.3 to 7.7‰ (Figure 1 and Table 1). Most individuals and species analyzed had δ13C values enriched 1.2 to 1.7‰ over the wood into which they bored (Table 1); their δ15N isotopic values were generally between −2 and 2‰ (Figure 1 and Table 1). Only in Abditoconus heterosiphon were δ13C values depleted relative to the wood they bored, being 1.4‰ more negative. Three members of the X. dorsalis clade (X. washingtona, X. dorsalis, X. alexisi) and A. heterosiphon had both mean δ15N values (Table 1) and 95% confidence intervals >3.0 (Table 2); they thus significantly exceeded the value predicted for freshly fixed nitrogen. The confidence intervals of X. dorsalis and X. washingtona did not overlap those of any other species (Table 2); their δ15N values are thus significantly enriched (p < 0.05) relative to those of every other taxon we analyzed.
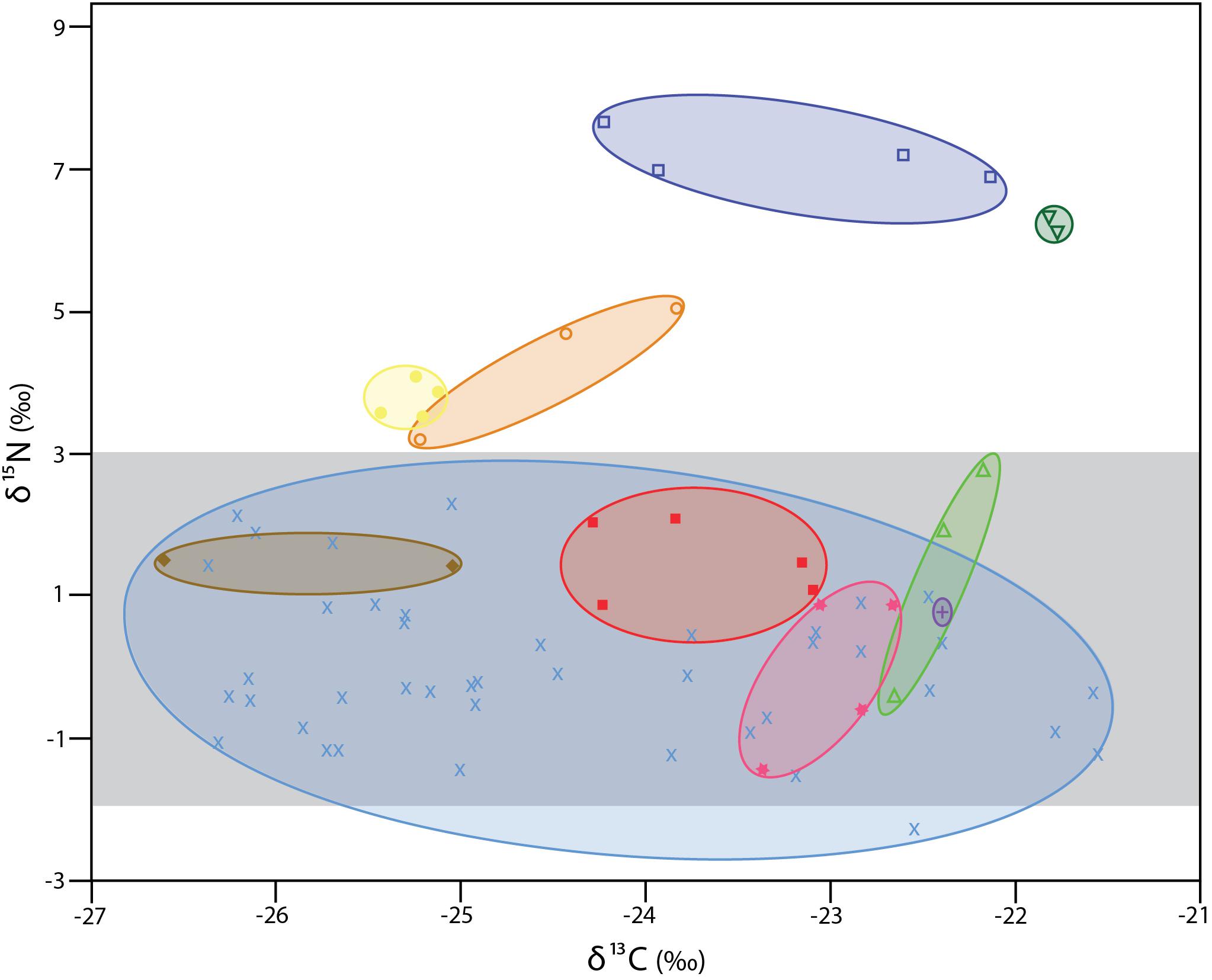
Figure 1. δ13C and δ15N values for specimens analyzed here. Ovals unite species. All members of the X. dorsalis clade are represented by open symbols. Dark blue open squares X. washingtona; dark green open, upside-down triangles X. dorsalis; orange open circles X. alexisi; green open triangles X. oregona. Yellow solid circles A. heterosiphon; brown solid diamonds Xylopholas crooki; red solid squares Xylophaga s.l. muraokai; pink solid stars Xylophaga s.l. microchira; purple plus sign Xyloredo nooi; blue crosses Xylophaga s.l. zierenbergi from six pooled collections (for details see Table 1). The area highlighted in light gray is that consistent with nitrogen fixed by bacteria (–2 to + 3).
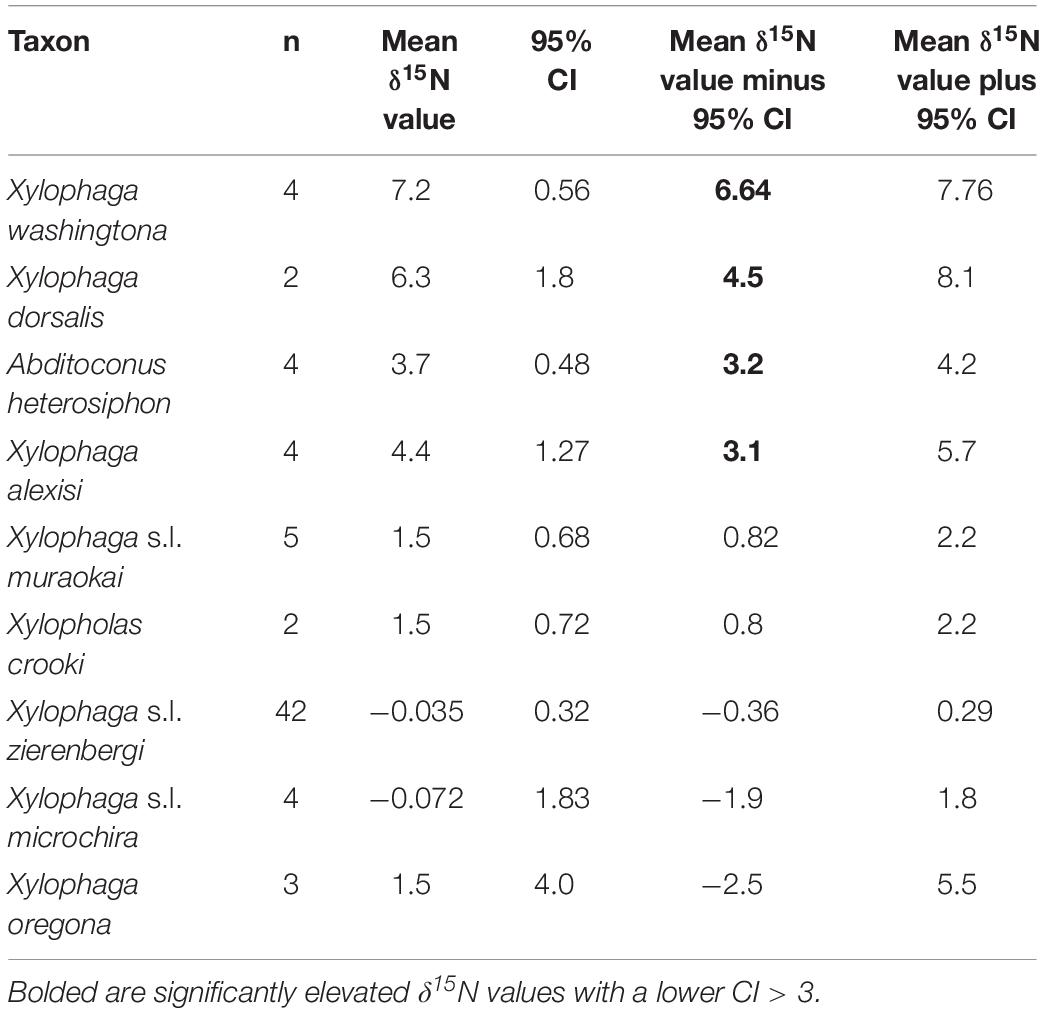
Table 2. Reported are the number of samples, the mean δ15N value, 95% confidence interval of the mean (CI) and the lower and upper 95% confidence intervals for each of the nine species represented by more than one specimen treated here; specimens of X. s.l. zierenbergi, X. s.l. muraokai and X. s.l. microchira from two areas were pooled.
Our ANOVAs failed to find any significant differences with respect to δ13C and δ15N after phylogeny was taken into account. Different iterations result in different p values, but we found these values to be ca. p ≈ 0.3 and p ≈ 0.2, respectively.
Preservation Artifact?
Differences in isotopic values among species do not appear to be due to preservation. X. dorsalis and X. washingtona were preserved in 95% ethanol, as were all members of X. s.l. zierenbergi from Monterey Canyon (Figure 1). Xylophaga alexisi and A. heterosiphon, the other species with elevated δ15N values, were fixed in formalin and were stored in 70% ethanol, as were the Endeavour specimens of X. s.l. zierenbergi, X. s.l. muraokai and X. s.l. microchira with typically xylophagous δ15N values (Figure 1). The results are also robust to the duration of preservation. The four outstanding species include X. dorsalis, collected 1 year before the analysis, and X. alexisi collected 27 years before the analysis. These are the extremes among the specimens considered.
Abditoconus heterosiphon had δ13C values depleted relative to the wood they bored and enriched δ15N values. The specimens were collected and preserved on the same cruise, using the same chemicals, as were those of Xylophaga s.l. microchira, which had isotopic signatures entirely consistent with strict xylotrophy (Tables 1, 2). Kruskal-Wallis tests found significant effects of preservation type (p = 0.005), but the formalin-fixed, ethanol-stored group contained many individuals of the X. dorsalis clade and A. heterosiphon (11 of 21) while the ethanol-preserved specimens included a comparative few of these (6 of 51).
The δ13C values of the 40 specimens of Xylophaga s.l. zierenbergi removed from different types of wood by Judge and Barry (2016) and likely preserved in the same batch of 95% ethanol exceeded the isotopic δ13C range of the other 32 specimens considered, regardless of different chemicals used to preserve the latter (Figure 1).
The specimens analyzed that were preserved by different chemical treatments (Table 1) did not significantly differ in either δ13C or δ15N isotopic values from specimens reported in the literature that had been frozen (noted in Table 3) prior to isotopic analyses (for δ13C isotopic values W = 0.514; p > 0.05; for δ15N isotopic values W = 0.543; p > 0.05).
Our Random Forest analyses found the most important variable associated with δ15N signatures was clade membership, followed by depth, δ13C value, wood type, taxon and lastly preservation. Given that preservation type covaried strongly with species, evidence for preservation type affecting our results appears to be lacking. Our phylogenetically corrected ANOVAs of Xyloredo nooi, Xylophaga dorsalis, X. washingtona, X. s.l. zierenbergi, X. s.l. muraokai, A. heterosiphon, and Xylopholas crooki failed to find significant differences in δ15N or δ13C values between clades after phylogenetic correction in multiple repeated randomized samplings of the phylogenetic tree. These groups did not statistically differ from one another after accounting for shared evolutionary history.
Xylophaga dorsalis Clade
In addition to the three members of the X. dorsalis clade with significantly elevated δ15N values (Table 2), the fourth member of the clade considered, X. oregona, had a mean δ15N value of 1.5 ± 1.6‰, among the highest of the remaining species considered (Table 1), with the largest standard deviations (Table 1) and much larger confidence intervals (Table 2).
Contrary to our predictions for strict xylophagy, the δ13C values of the fecal chimneys of X. dorsalis (mean = −26.9 ± 1.1‰; n = 8) were intermediate between those of wood (−28.1 ± 0.4‰ n = 5) and siphon tissue (−21.8 ± 0.03‰; n = 2) (Supplementary Table S3). Consistent with our predictions, the tissues (mean δ13C −22.4 ± 0.2‰; n = 3) of X. oregona were enriched relative to the wood (−24.3 ± 0.2‰; n = 3) and the chimneys were depleted (mean δ13C −25.5 ± 0.6‰; n = 5) (Supplementary Table S3).
Relationship of Depth to Stable Nitrogen Isotopic Signature
The species’ collection depths were not significantly correlated with their mean δ15N values (r = 0.394; n = 13; p > 0.05). Removing the extreme shallow-water (<500 m) species (X. dorsalis and X. washingtona) and recalculating did not discover a significant relationship (r = 0.467; n = 11; p > 0.10). Kruskal-Wallis tests found depth to be significantly linked to δ15N (p < < 0.05) when individuals rather than species means were considered.
Within Species Variation
The δ13C isotopic values of six collections of 42 specimens of Xylophaga s.l. zierenbergi ranged from -26.4 to −21.5‰, exceeding the combined range of all other specimens considered (Figure 1). The most depleted δ13C isotopic values of X. s.l. zierenbergi were from those removed from bark-covered logs of Pinus pinea and Quercus agrifolia (mean −25.7‰). The borers in the P. pinea deployment also included seven of the eleven most enriched in δ15N values (mean 1.6 ± 0.6‰) of the 42 specimens of X. s.l. zierenbergi. Even with these outstanding specimens, the δ15N isotopic values of this species ranged from −2.3 to 2.3‰, within the predicted range of freshly fixed nitrogen (Supplementary Table S2). The δ13C values of X. s.l. zierenbergi from a single ginkgo log spanned 4.0‰ (Figure 2). The δ13C values of specimens from deployed spicebush sticks ranged from near −25 to −22.5‰; they also showed a comparatively wide range of δ15N values (−2.25 to 1.0‰).
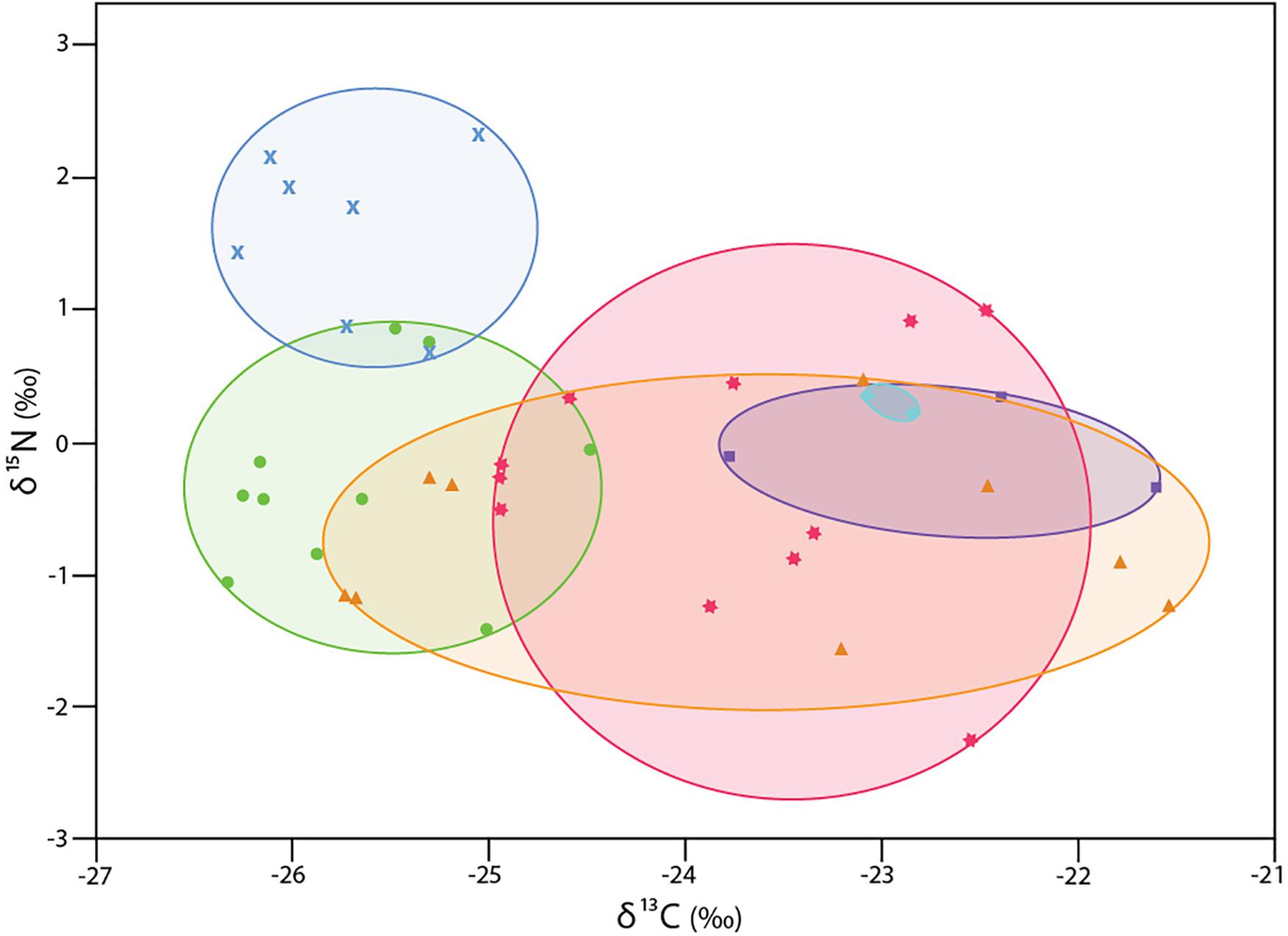
Figure 2. δ13C and δ15N values for 42 specimens of Xylophaga s.l. zierenbergi from six deployments. wood bundles (WB) except aqua diamonds: from Douglas fir on Endeavour Segment, Juan de Fuca Ridge at 2211 m depth (Voight, 2007); all others are from deployments near Monterey Canyon at 3100 m depth (Judge and Barry, 2016): blue crosses bark-covered pine log (WB17); green circles bark-covered oak log (WB23); orange triangles gingko logs (WB26); pink stars spicebush sticks (WB19); purple squares island ironwood (WB32).
Discussion
The stable isotopic signatures of six of ten xylophagaid species we considered are entirely consistent with predicted stable isotopic signatures of strict xylophages; they eat the wood that they bore and use nitrogen fixed by bacteria. The other four species (X. dorsalis, X. washingtona, X. alexisi and A. heterosiphon) have δ15N values significantly enriched compared to those we predict characterize nitrogen fixed by bacteria; they are the focus of our discussion. Although supporting data are required, we offer a hypothesis of filter feeding.
Clade Membership and Preservation Effects
Members of the X. dorsalis clade, X. dorsalis, X. washingtona, and X. alexisi have mean δ15N values from 4.4 to 6.5‰; these significantly exceed those predicted for bacterial nitrogen fixation. Comparable data for members of this clade have been reported previously: Bernardino et al. (2010) plotted three individuals of X. washingtona as having an average δ15N signature near 4.9‰; Zapata-Hernández et al. (2016) reported X. globosa, assigned to this clade due to its morphology (Turner, 1955), to have δ15N values of 9 ± 5.1‰. These data and the fact that most of these specimens were collected from shallow, likely more productive depths suggest that these xylophagaids may filter feed. The X. dorsalis clade has a significantly shallower distribution than do other members of the family (Voight, 2008).
One member of the clade, Xylophaga alexisi, violates the depth generality. From 4626 m depth in the Cape Verde Abyssal Plain, it has a mean δ15N value of 4.4‰, despite the predicted scarcity of plankton at the abyssal collection depths. A simultaneously deployed near-bottom sediment trap, however, documented that the deployment re-suspended abyssal sediments (Khripounoff et al., 1998); perhaps these xylophagaids fed on these particles. Deep-sea nitrogen particulates may have comparatively high δ15N signatures (Sigman and Casciotti, 2001). Globally, deep-sea heterotrophic animals have average δ15N isotopic values from 10 to 13‰ depending on latitude (reviewed in Parzanini et al., 2019). Montoya et al. (2002) found POM δ15N increases rapidly to 6–8‰ in the first 300 m in the tropical Atlantic. A small amount of abyssal sediment may enrich 15N values to a greater degree than would be expected at shallow depths.
Limited behavioral data may support the hypothesis of filter feeding in the X. dorsalis clade. These taxa share an otherwise unique excurrent siphon that is truncated relative to the incurrent siphon. The incurrent siphon can extend over a cm beyond the wood (Purchon, 1941) into the water column, as in situ photos show (Bernardino et al., 2010, Figure 2b; Romano et al., 2014, Figure 2). Siphonal extension was thought to ensure access to well-oxygenated water, away from dense populations with the numerous fecal chimneys in the wood. Siphonal extension could, however, also facilitate filter feeding. As dense populations bore the wood, some individuals might secure more resources from the water column than from the remaining wood. Perhaps the extremely high variation in stable isotopic δ15N signatures we document in individuals of X. oregona from an unusually dense population is indicative of such a scenario.
An alternate explanation for the enriched δ15N signatures of the X. dorsalis clade is vertical transmission of a clade-specific symbiont that used another source of nitrogen. Available data, however, argue against vertical transmission of symbionts. Pyrosequencing revealed that two symbionts from three individuals of X. dorsalis (as sp. A) share 95% genetic similarity to those known from shallow-water teredinids (Fagervold et al., 2014). This supports environmental acquisition of symbionts rather than vertical transmission.
The fourth species identified here as an outlier, A. heterosiphon, is the only species to have a mean δ13C value (−25.3 ± 0.15‰) more depleted than is the wood (−23.9‰) it bored, and apparently consumed. Its mean δ15N signature of 3.7‰ is also significantly enriched compared to predictions for nitrogen fixed by bacteria. This species has complete siphons which are covered by a periostracal cone (Voight, 2007), seemingly eliminating the possibility of filter feeding. Perhaps this species exploits wood-fall associated sulfide-oxidizing bacteria (e.g. Kalenitchenko et al., 2018). Its δ13C values are depleted relative to those of X. s.l. microchira that were collected during the same cruise from the same type of wood deployed at the same time and preserved in the same way with the same chemicals.
Different chemical preservatives minimally affect the stable isotopic signatures discussed here. The comparatively high variation in δ13C values in wood that is likely to be reflected in the δ13C values of strictly xylophagous bivalves (Figure 2), and the fact that stable nitrogen isotopic values are typically more robust to preservatives than are δ13C values (Kaehler and Pakhomov, 2001; Ogawa et al., 2001; Sarakinos et al., 2002; Syväranta et al., 2008, 2011; Rennie et al., 2012; González-Bergonzoni et al., 2015), likely contributed to this result.
The Effects of Wood
The wood substrate into which the animals bore also appears to affect their δ13C values. Specimens of Xylophaga zierenbergi removed from bark-covered pine and oak logs have distinctly depleted δ13C values compared to conspecifics from the wood of spice bush, island ironwood, and Douglas fir. Those from logs of P. pinea, identified by Judge and Barry (2016), also have relatively enriched δ15N values. Why these seemingly anomalous values are present remains open to conjecture and may offer an attractive area for future research. The δ13C value of P. pinea reported in the literature is relatively depleted, −26.1 and −27‰ (Battipaglia et al., 2010; Sarris et al., 2013), but specimens of X. s.l. zierenbergi from both pine and the oak have relatively depleted δ13C values. Perhaps notably, both the pine and oak logs were bark-covered; the bark may affect the wood, or its microbial community.
The δ15N values of five of seven specimens from P. pinea are enriched relative to conspecifics, although they remain within our predicted range for nitrogen fixed by bacteria. Specimens removed from spice bush wood show more variation in the δ15N values than do those from other substrates (Figure 2). Wood itself cannot be responsible for enriching the δ15N values as its nitrogen content is too low to do so. Other factors such as oxygen levels possibly mediated by the bark covering could have influenced these results. Could such factors also have resulted in the enriched δ15N values of the three members of the X. dorsalis clade? Given that the dramatically and consistently enriched δ15N values of three species of that clade derive from wild wood falls and artificial deployments in the North Atlantic and the North Pacific from depths of 18 to 4626 m, it seems unlikely but proof is lacking. In addition, the δ13C values of the X. dorsalis clade are generally not depleted, being directly comparable to most specimens of X. s.l. zierenbergi and to predictions for strict xylophagy.
Xylophagy: A Flawed Conclusion?
The implicit view that all xylophagaids are strict xylophages has uncertain origins. Purchon (1941) reported reduced palps in X. dorsalis and the poor sorting potential of their gills (Distel and Roberts, 1997). Reduced palps do characterize bivalves that host chemosynthetic bacteria on their gills (Roeselers and Newton, 2012), but gill endosymbionts do not preclude filter feeding. Bivalves of Lucinoma borealis and mussels of Bathymodiolus are among the chemosynthetic bivalves documented to feed by both means; the lucinids are estimated to obtain about half of their carbon through filter feeding (Dando et al., 1986; Page et al., 1990). Reduced palps appear to poorly predict filter feeding in wood borers, despite statements to the contrary (Saraswathy and Nair, 1971). In T. navalis, the paired dorsal palps are inconspicuous and the ventral ones are reduced to slightly raised ciliated patches (Lazier, 1924). Saraswathy and Nair (1971) cited Lazier’s (1924) report but omitted his mention that the palps’ function is “retained in Teredo” (p. 458), although whether they effectively controlled the plankton stream was doubtful (Lazier, 1924). Isotopic data indicate that T. navalis secures most of its carbon through filter feeding (Paalvast and van der Velde, 2013). The stable isotopic signatures of B. carinata, another teredinid with small palps (Saraswathy and Nair, 1971 as B. indica, synonymy following Turner, 1966), indicate that it relies entirely on xylophagy (Charles et al., 2018). The small palps of X. dorsalis may limit sorting, but captive individuals regularly expel pseudofeces through the incurrent siphon (JRV oight unpub data), which may indicate they exert some selection.
The scarcity of alternative food sources in the deep sea was cited by Distel and Roberts (1997) as limiting xylophagaid filter feeding. However, the species they examined had been collected at 60 and 80–100 m depth. Distel and Roberts (1997) reported few phytoplankton or microorganisms in the gut of the two species of Xylophaga they examined, but the animals had been maintained in “seawater tanks,” potentially without a source of plankton, for up to 2 weeks before their examination; whether they examined the cecum, which has few microbes in teredinids, or the intestine, which hosts many more microbes in teredinids (Betcher et al., 2012), was not stated.
The nitrogen isotopic data cited as supporting the hypothesis of filter feeding can be subject to interpretation. A case in point is the elevated δ15N signature of dwarf males of Xylophaga s.l. atlantica that was interpreted as evidence of filter feeding on fecal matter and by-products of boring in a dense population (Gaudron et al., 2016). An alternate explanation for these data is that they might reflect dwarf males parasitizing the autonomously boring female to which they attach. A review of parasitic interactions (Thieltges et al., 2019) found that parasites rarely exhibit a standard trophic shift of δ15N 3.4‰. The trophic shift in δ15N values seen in host-parasite interactions is often much lower, averaging 1.7‰; the nature of the parasitic interaction is likely to strongly impact that value. How the dwarf males access female resources remains conjectural; in this clade, dwarf males attach to the dorsal shell (Voight et al., 2019) rather than to the tissues of the autonomous borers as in other clades, such as Feaya dostwous (Voight, 2016). The high mean δ15N values (4.6 ± 0.5‰) of the autonomously boring females X. s.l. atlantica may reflect the influence of nearby hydrothermal fluid flow (Gaudron et al., 2016).
In a study that included sulfur isotopic data of the bivalves and wood, and amino acid δ15N analyses, Yamanaka et al. (2015) report xylophagy by individuals of Xyloredo teramachii in deployments made at four depths. Yamanaka et al. (2015) used compound-specific isotopic analyses and patterns observed in marine nitrogen-fixing cyanobacteria, and terrestrial plants (Chikaraishi et al., 2009) to conclude that the bivalves were entirely xylophagous. Although their standard deviations overlapped, the mean δ15N values of the bivalves at each depth are significantly and negatively correlated with depth (r = −0.972; n = 4; p < 0.05). A significant correlation would be expected if the animals were to opportunistically filter feed in shallower, more productive waters.
The intraspecific, and intra-deployment, variation in δ13C signatures among specimens of Xylophaga s.l. zierenbergi may warn investigators seeking to use stable isotopic analyses of xylophagaids in food web studies. The points representing X. s.l. zierenbergi from gingko generally form two clusters, perhaps indicative differences between the heartwood and sapwood exposed on that log (J. Judge, unpub data). The origin of each xylophagaid might strongly influence its stable isotopic signatures. Multiple xylophagaids to be analyzed should be sampled from different parts of the wood, and matched with wood samples from the same specific area, to test if intra-wood differences affect the results. Such within-wood differences are consistent with the observed 4‰ range in δ13C values of a single species in a single deployment.
The entrenched idea of strict xylophagy and the few comparable data available from other xylophagaids may have led to elevated stable isotopic signatures being discounted. Additional study of correctly identified taxa, collection and analysis of samples of particulate organic matter and sediment associated with the bored wood-fall are required to fully test the hypothesis that mixotrophy exists in xylophagaids. Although filter feeding may be opportunistic and not necessarily occur in every habitat or in every taxon, assuming that wood is the sole source of energy available to wood-fall communities may be fallacious. The amount of unknown variation in the composition of wood-fall communities (McClain and Barry, 2014; Judge and Barry, 2016) argues that assumptions should be re-examined.
Data Availability Statement
All datasets generated for this study are included in the article/Supplementary Material.
Author Contributions
JV: conceptualization, funding acquisition, resources, visualization, writing original draft preparation, review, editing, and revision. JC: statistical analyses, editing, and revision. RL: conceptualization, data curation, formal analysis, investigation, project administration, resources, validation, and writing – review and editing.
Funding
Funding was provided by the Robert A. Pritzker Center for Meteoritics and Polar Studies established by a grant from the Tawani Foundation.
Conflict of Interest
The authors declare that the research was conducted in the absence of any commercial or financial relationships that could be construed as a potential conflict of interest.
Acknowledgments
We thank University of Bergen Marine Station; Captain and crew of the R/V HANS BRATTSTROM. J. Judge who donated specimens of X. s.l. zierenbergi to Field Museum; C. R. Smith and K. Halanych provided specimens of X. washingtona; B. Marshall allowed sampling of Xyloredo nooi from his collection. Lisa Kanellos executed the plots.
Supplementary Material
The Supplementary Material for this article can be found online at: https://www.frontiersin.org/articles/10.3389/fmars.2020.00050/full#supplementary-material
References
Altabet, M. A., Pilskaln, C., Thunell, R., Pride, C., Sigman, D., Chavez, F., et al. (1999). The nitrogen isotope biogeochemistry of sinking particles from the margin of the eastern North Pacific. Deep Sea Res. Part I 46, 655–679. doi: 10.1016/s0967-0637(98)00084-3
Ansel, A. D., and Nair, N. B. (1969). The mechanisms of boring in Martesia striata Linne (Bivalvia: Pholadidae) and Xylophaga dorsalis Turton (Bivalvia: Xylophaginidae). Proc. R. Soc. Lond. B 174, 123–133. doi: 10.1098/rspb.1969.0084
Battipaglia, G., Marzaioli, F., Lubritto, C., Altieri, S., Strumia, S., Cherubini, P., et al. (2010). Traffic pollution affects tree-ring width and isotopic composition of Pinus pinea. Sci. Total Environ. 408, 586–593. doi: 10.1016/j.scitotenv.2009.09.036
Bernardino, A. F., Smith, C. R., Baco, A., Altamira, I., and Sumida, P. Y. G. (2010). Macrofaunal succession in sediments around kelp and wood falls in the deep NE Pacific and community overlap with other reducing habitats. Deep Sea Res. I 57, 708–723. doi: 10.1016/j.dsr.2010.03.004
Bert, D., Leavitt, S. W., and Dupouey, J.-L. (1997). Variations of wood δ13C and water-use efficiency of Abies alba during the last century. Ecology 78, 1588–1596. doi: 10.1890/0012-9658(1997)078
Betcher, M. A., Fung, J. M., Han, A. W., O’Connor, R., Seronay, R., Concepcion, G. P., et al. (2012). Microbial distribution and abundance in the digestive system of five shipworm species (Bivalvia: Teredinidae). PLoS One 7:e45309. doi: 10.1371/journal.pone.0045309
Charles, F., Sauriau, P.-G., Aubert, F., Lebreton, B., Lantoine, F., and Riera, P. (2018). Sources partitioning in the diet of the shipworm Bankia carinata (J.E. Gray, 1827): an experimental study based on stable isotopes. Mar. Environ. Res. 142, 208–213. doi: 10.1016/j.marenvres.2018.10.009
Chikaraishi, Y., Ogawa, N. O., Kashiyama, Y., Takano, Y., Suga, H., Tomitani, A., et al. (2009). Determination of aquatic food-web structure based on compound-specific nitrogen isotopic composition of amino acids. Limnol. Oceanogr. 7, 740–750. doi: 10.1016/j.jchromb.2016.09.004
R Core Team (2019). R: A Language and Environment for Statistical Computing. Vienna: R Foundation for Statistical Computing.
Dando, P. R., Southward, A. J., and Southward, E. C. (1986). Chemoautotrophic symbionts in the gills of the bivalve mollusc Lucinoma borealis and the sediment chemistry of its habitat. Proc. R. Soc. Lond. B 227, 227–247. doi: 10.1098/rspb.1986.0021
Distel, D. L., Amin, M., Burgoyne, A., Linton, E., Mamangkey, G., Morrill, W., et al. (2011). Molecular phylogeny of Pholadoidea Lamarck, 1809 supports a single origin for xylotrophy (wood feeding) and xylotrophic bacterial endosymbiosis in Bivalvia. Mol. Phylogenet. Evol. 61, 245–254. doi: 10.1016/j.ympev.2011.05.019
Distel, D. L., and Roberts, S. J. (1997). Bacterial endosymbionts in the gills of the deep-sea wood-boring bivalves Xylophaga atlantica and Xylophaga washingtona. Biol. Bull. 192, 253–261. doi: 10.2307/1542719
Dowle, M., and Srinivasan, A. (2019). Data.Table: Extension of ‘Data.Frame’. R package version 1.12.6.
Fagervold, S. K., Romano, C., Kalenitchenko, D., Borowski, C., Nunes-Jorge, A., Martin, D., et al. (2014). Microbial communities in sunken wood are structured by wood-boring bivalves and location in a submarine canyon. PLoS One 9:e96248. doi: 10.1371/journal.pone.0096248
Ferrier-Pagés, C., and Leal, M. (2019). Stable isotopes as tracers of trophic interactions in marine mutualistic symbioses. Ecol. Evol. 9, 723–740. doi: 10.1002/ece3.4712
Filipiak, M. (2018). “Nutrient dynamics in decomposing dead wood in the context of wood eater requirements: the ecological stoichiometry of saproxylophagous insects,” in Saproxylic Insects. Zoological Monographs, Vol. 1, ed. M. Ulyshen, (Cham: Springer), 429–469. doi: 10.1007/978-3-319-75937-1_13
Filipiak, M., and Weiner, J. (2014). How to make a beetle out of wood: multi-elemental stoichiometry of wood decay, xylophagy and fungivory. PLoS One 9:e115104. doi: 10.1371/journal.pone.0115104
Gallager, S. M., Turner, R. D., and Berg, C. J. Jr. (1981). Physiological aspects of wood consumption, growth, and reproduction in the shipworm Lyrodus pedicellatus Quatrefages (Bivalvia: Teredinidae). J. Exp. Mar. Biol. Ecol. 52, 63–77. doi: 10.1016/0022-0981(81)90171-4
Gaudron, S. M., Haga, T., Wang, H., Laming, S. R., and Duperron, S. (2016). Plasticity in reproduction and nutrition in wood-boring bivalves (Xylophaga atlantica) from the Mid-Atlantic Ridge. Mar. Biol. 163:213. doi: 10.1007/s00227-016-2988-6
González-Bergonzoni, I., Vidal, N., Wang, B., Ning, D., Liu, Z., Jeppesen, E., et al. (2015). General validation of formalin-preserved fish samples in food web studies using stable isotopes. Methods Ecol. Evol. 6, 307–314. doi: 10.1111/2041-210x.12313
Johnston, A., Hochcahka, W. M., Strimas-Mackey, M. E., Ruiz Gutierrez, V., Robinson, O. J., Miller, E. T., et al. (2019). Best practices for making reliable inferences from citizen science data: a case study using eBird to estimate species distributions. bioRxiv [Preprint]. doi: 10.1101/574392
Judge, J., and Barry, J. P. (2016). Macroinvertebrate community assembly on deep-sea wood falls in Monterey Bay is strongly influenced by wood type. Ecology 97, 3031–3043. doi: 10.1002/ecy.1546
Kaehler, S., and Pakhomov, E. A. (2001). Effects of storage and preservation on the δ13C and δ15N signatures of selected marine organisms. Mar. Ecol. Prog. Ser. 219, 299–304. doi: 10.3354/meps219299
Kalenitchenko, D., Péru, E., Pereira, L. C., Petetin, C., Galand, P. E., and LeBris, N. (2018). The early conversion of deep-sea wood falls into chemosynthetic hotspots revealed by in situ monitoring. Sci. Rep. 8:907. doi: 10.1038/s41598-017-17463-2
Karande, A. A., Balasubramanian, K., and Prema, S. (1968). “Development of a laboratory method for bioassay of candidate toxins against teredid wood-borers,” in Proceedings of the Symposium on Molluscs, Part 111, (Mandapam: Marine Biological Association of India), 736–745.
Khripounoff, A., Vangriesheim, A., and Crassous, P. (1998). Vertical and temporal variations of particle fluxes in the deep tropical Atlantic. Deep Sea Res. 45, 193–216. doi: 10.1016/s0967-0637(97)00104-0
Korol, R. L., Kirschbaum, M. U. F., Farquhar, G. D., and Jeffreys, M. (1999). Effects of water status and soil fertility on the C-isotope signature in Pinus radiata. Tree Physiol. 19, 551–562. doi: 10.1093/treephys/19.9.551
Lazier, E. L. (1924). Morphology of the Digestive Tract of Teredo navalis, Vol. 22. Berkeley, CA: University of California Publications in Zoology, 455–474. doi: 10.1093/treephys/19.9.551
Leavitt, S. W., and Long, A. (1986). Stable-carbon isotope variability in tree foliage and wood. Ecology 67, 1002–1010. doi: 10.2307/1939823
Loader, N. J., Robertson, I., and McCarroll, D. (2003). Comparison of stable carbon isotope ratios in the whole wood, cellulose and lignin of oak tree-rings. Palaeogeogr. Palaeoclimatol. Palaeoecol. 196, 395–407. doi: 10.1016/s0031-0182(03)00466-8
McClain, C., and Barry, J. (2014). Beta-diversity on deep-sea wood falls reflects gradients in energy availability. Biol. Lett. 10:20140129. doi: 10.1098/rsbl.2014.0129
Minagawa, M., and Wada, E. (1984). Stepwise enrichment of 15N along food chains: further evidence and the relation between δ15N and animal age. Geochim. Cosmochim. Acta 48, 1135–1140. doi: 10.1016/0016-7037(84)90204-7
Montoya, J. P., Carpenter, E. J., and Capone, D. G. (2002). Nitrogen fixation and nitrogen isotope abundances in zooplankton of the oligotrophic North Atlantic. Limnol. Oceanogr. 47, 1617–1628. doi: 10.1371/journal.pone.0131258
Muraoka, J. (1967). Deep-ocean biodeterioration of materials – Part VI. One year at 2370 feet. Naval Facilities Engineering Command. Port Hueneme, CA: U.S. Naval Civil Engineering Lab, 1–57.
Nishimoto, A., Mito, S., and Shirayama, Y. (2009). Organic carbon and nitrogen source of sunken wood communities on continental shelves around Japan inferred from stable isotope ratios. Deep Sea Res. II 56, 1683–1688. doi: 10.1016/j.dsr2.2009.05.032
Ogawa, N. O., Koitabashi, T., Oda, H., Nakamura, T., Ohkouchi, N., and Wada, E. (2001). Fluctuations of nitrogen isotope ratio of gobiid fish (Isaza) specimens and sediments in Lake Biwa. Japan, during the 20th century. Limn. Oceanogr. 46, 1228–1236. doi: 10.4319/lo.2001.46.5.1228
Paalvast, P., and van der Velde, G. (2013). What is the main food source of the shipworm (Teredo navalis)? A stable isotope approach. J. Sea Res. 80, 58–60. doi: 10.1016/j.seares.2013.03.003
Page, H. M., Fisher, C. R., and Childress, J. J. (1990). Role of filter-feeding in the nutritional biology of a deep-sea mussel with methanotrophic symbionts. Mar. Biol. 104, 251–257. doi: 10.1007/bf01313266
Parzanini, C., Parrish, C. C., Hamel, J.-F., and Mercier, A. (2019). Reviews and syntheses: insights into deep-sea food webs and global environmental gradients revealed by stable isotope (δ15N, δ13C) and fatty acid trophic biomarkers. Biogeosciences 16, 2837–2856. doi: 10.5194/bg-16-2837-2019
Pechenik, J. A., Perron, E. F., and Turner, R. D. (1979). The role of phytoplankton in the diets of adult and larval shipworms, Lyrodus pedicellatus (Bivalvia: Teredinidae). Estuar. Coasts 2, 58–60.
Purchon, R. D. (1941). On the biology and relationships of the lamellibranch Xylophaga dorsalis (Turton). J. Mar. Biol. Assoc. U. K. 45, 1–39. doi: 10.1017/s0025315400014259
Rennie, M. D., Ozersky, T., and Evans, D. O. (2012). Effects of formalin preservation on invertebrate stable isotope values over decadal time scales. Can. J. Zool. 90, 1320–1327. doi: 10.1139/z2012-101
Revell, L. J. (2012). Phytools: an R package for phylogenetic comparative biology (and other things). Methods Ecol. Evol. 3, 217–223. doi: 10.1111/j.2041-210X.2011.00169.x
Roeselers, G., and Newton, I. L. G. (2012). On the evolutionary ecology of symbioses between chemosynthetic bacteria and bivalves. Appl. Microbiol. Biotechnol. 94, 1–10. doi: 10.1007/s00253-011-3819-9
Romano, C., Pérez-Portela, R., and Martin, D. (2014). Morphological and genetic diversity of the wood-boring Xylophaga (Mollusca, Bivalvia): new species and records from deep-sea Iberian canyons. Plos One 9:e102887. doi: 10.1371/journal.pone.0102887
Sabbadin, F., Pesante, G., Elias, L., Besser, K., Li, Y., Steele-King, C., et al. (2018). Uncovering the molecular mechanisms of lignocellulose digestion in shipworms. Biotechnol. Biofuels 11:59. doi: 10.1186/s13068-018-1058-3
Sarakinos, H. C., Johnson, M. L., and Zanden, M. J. V. (2002). A synthesis of tissue-preservation effects on carbon and nitrogen stable isotope signatures. Can. J. Zool. 80, 381–387. doi: 10.1002/rcm.5049
Saraswathy, M., and Nair, N. B. (1971). XIV.—Observations on the structure of the shipworms, Nausitora hedleyi, Teredo furcifera and Teredora princesae (Bivalvia: Teredinidae). Trans. R. Soc. Edinb. 68, 507–566. doi: 10.1017/s0080456800014861
Sarris, D., Siegwolf, R., and Körner, C. (2013). Inter- and intra-annual stable carbon and oxygen isotope signals in response to drought in Mediterranean pines. Agric. For. Meteorol. 168, 59–68. doi: 10.1016/j.agrformet.2012.08.007
Schleser, G. H., Anhuf, D., Helle, G., and Vos, H. (2015). A remarkable relationship of the stable carbon isotopic compositions of wood and cellulose in tree-rings of the tropical species Cariniana micrantha (Ducke) from Brazil. Chem. Geol. 401, 59–66. doi: 10.1016/j.chemgeo.2015.02.014
Sigman, D. M., and Casciotti, K. L. (2001). “Nitrogen isotopes in the ocean,” in Encyclopedia of Ocean Sciences, eds J. Steele, S. Thorpe, and K. Turekian, (Oxford: Academic Press), 4139–4152.
Silberberger, M. J., Renaud, P. E., Buhl−Mortensen, L., Ellingsen, I. H., and Reiss, H. (2019). Spatial patterns in sub-Arctic benthos: multiscale analysis reveals structural differences between community components. Ecol. Monogr. 89:e01325. doi: 10.1002/ecm.1325
Somes, C. J., Schmittner, A., and Altabet, M. A. (2010). Nitrogen isotope simulations show the importance of atmospheric iron deposition for nitrogen fixation across the Pacific ocean. Geophys. Res. Lett. 37:L23605. doi: 10.1029/2010GL044537
Syväranta, J., Martino, A., Kopp, D., Céréghino, R., and Santoul, F. (2011). Freezing and chemical preservatives alter the stable isotope values of carbon and nitrogen of the Asiatic clam (Corbicula fluminea). Hydrobiologia 658, 383–388. doi: 10.1007/s10750-010-0512-4
Syväranta, J., Vesala, S., Rask, M., Ruuhijärvi, J., and Jones, R. I. (2008). Evaluating the utility of stable isotope analyses of archived freshwater sample material. Hydrobiologia 600, 121–130. doi: 10.1007/s10750-007-9181-3
Taylor, A. M., Brooks, J. R., Lachenbruch, B., and Morrell, J. J. (2007). Radial patterns of carbon isotopes in the xylem extractives and cellulose of Douglas-fir. Tree Physiol. 27, 921–927. doi: 10.1093/treephys/27.6.921
Thieltges, D. W., Goedknegt, M. A., O’Dwyer, K., Senior, A. M., and Kamiya, T. (2019). Parasites and stable isotopes: a comparative analysis of isotopic discrimination in parasitic trophic interactions. Oikos 128, 1329–1339. doi: 10.1111/oik.06086We
Turner, R. D. (1955). The family Pholadidae in the western Atlantic and the eastern Pacific II. Martesiinae, Jouannetinae and Xylophaginae. Johnsonia 3, 65–160.
Turner, R. D. (1966). A Survey and Illustrated Catalogue of the Teredinidae. Cambridge, MA: Museum Comparative Zoology.
Turner, R. D. (1973). Wood-boring bivalves, opportunistic species in the deep sea. Science 180, 1377–1379. doi: 10.1126/science.180.4093.1377
Voight, J. R. (2007). Experimental deep-sea deployments reveal diverse Northeast Pacific wood-boring bivalves of Xylophagainae (Myoida: Pholadidae). J. Mollus. Stud. 73, 377–391. doi: 10.1093/mollus/eym034
Voight, J. R. (2008). Deep-sea wood-boring bivalves of Xylophaga (Myoida: Pholadidae) on the continental shelf. J. Mar. Biol. Assoc. U. K. 88, 1467–1472.
Voight, J. R. (2016). New insights on Xylopholas (Mollusca: Xylophagaidae): diversity, growth and reproduction. Am. Malacol. Bull. 34, 138–146. doi: 10.4003/006.034.0210
Voight, J. R., Marshall, B. A., Judge, J., Halanych, K. M., Li, Y., Bernardino, A. F., et al. (2019). Life in wood: preliminary phylogeny of deep-sea wood-boring bivalves (Xylophagaidae), with descriptions of three new genera and one new species. J. Mollus. Stud. 85, 232–243. doi: 10.1093/mollus/eyz003
Voight, J. R., and Segonzac, M. (2012). At the bottom of the deep blue sea: a new wood-boring bivalve (Mollusca, Pholadidae, Xylophaga) from the Cape Verde Abyssal Plain (subtropical Atlantic). Zoosystema 34, 171–180. doi: 10.5252/z2012n1a8
Wright, M., and Ziegler, A. (2017). Ranger: a fast implementation of random forests for high dimensional data in C++ and R. J. Stat. Softw.77, 1–7. doi: 10.18637/jss.v077.i01
Yamanaka, T., Shimamura, S., Chikaraishi, Y., Haga, T., and Fujiwara, Y. (2015). Re-evaluation of nutrient sources for deep-sea wood-boring bivalves using the isotopic composition of bulk C, N, S, and amino acid nitrogen. Mar. Ecol. Prog. Ser. 540, 157–165. doi: 10.3354/meps11510
Zapata-Hernández, G., Sellanes, J., Thiel, M., Henríquez, C., Hernández, S., Fernández, J. C. C., et al. (2016). Community structure and trophic ecology of megabenthic fauna from the deep basins in the interior sea of Chiloé, Chile (41–43° S). Cont. Shelf Res. 130, 47–67. doi: 10.1016/j.csr.2016.10.002
Keywords: carbon isotope, nitrogen isotope, Xylophaga, Abditoconus, Xyloredo
Citation: Voight JR, Cooper JC and Lee RW (2020) Stable Isotopic Evidence of Mixotrophy in Xylophagaids, Deep-Sea Wood-Boring Bivalves. Front. Mar. Sci. 7:50. doi: 10.3389/fmars.2020.00050
Received: 26 July 2019; Accepted: 27 January 2020;
Published: 14 February 2020.
Edited by:
Toshi Nagata, The University of Tokyo, JapanReviewed by:
Sylvie Gaudron, Sorbonne Université, FranceChiara Romano, Center for Advanced Studies of Blanes (CSIC), Spain
Onishi Yuji, Kyoto University, Japan
Copyright © 2020 Voight, Cooper and Lee. This is an open-access article distributed under the terms of the Creative Commons Attribution License (CC BY). The use, distribution or reproduction in other forums is permitted, provided the original author(s) and the copyright owner(s) are credited and that the original publication in this journal is cited, in accordance with accepted academic practice. No use, distribution or reproduction is permitted which does not comply with these terms.
*Correspondence: Janet R. Voight, anZvaWdodEBmaWVsZG11c2V1bS5vcmc=