- 1NOAA Fisheries, Office of Science and Technology, Cooperative Oxford Laboratory, Oxford, MD, United States
- 2NOAA Fisheries, Northwest Fisheries Science Center, Seattle, WA, United States
- 3NOAA Fisheries, West Coast Regional Office, Seattle, WA, United States
- 4NOAA Fisheries, Southeast Regional Office, Gulf Branch, Baton Rouge, LA, United States
- 5NOAA Fisheries, Alaska Fisheries Science Center, Seattle, WA, United States
- 6NOAA Fisheries, Northeast Fisheries Science Center, Woods Hole, MA, United States
- 7NOAA Fisheries, Pacific Islands Fisheries Science Center, Honolulu, HI, United States
- 8NOAA Fisheries, Southwest Fisheries Science Center, Monterey, CA, United States
Worldwide fisheries management has been undergoing a paradigm shift from a single-species approach to ecosystem approaches. In the United States, NOAA has adopted a policy statement and Road Map to guide the development and implementation of ecosystem-based fisheries management (EBFM). NOAA’s EBFM policy supports addressing the ecosystem interconnections to help maintain resilient and productive ecosystems, even as they respond to climate, habitat, ecological, and social and economic changes. Managing natural marine resources while taking into account their interactions with their environment and our human interactions with our resources and environment requires the support of ecosystem science, modeling, and analysis. Implementing EBFM will require using existing mandates and approaches that fit regional management structures and cultures. The primary mandate for managing marine fisheries in the United States is the Magnuson-Stevens Fishery Conservation and Management Act. Many tenets of the Act align well with the EBFM policy, however, incorporating ecosystem analysis and models into fisheries management processes has faced procedural challenges in many jurisdictions. In this paper, we review example cases where scientists have had success in using ecosystem analysis and modeling to inform management priorities, and identify practices that help bring new ecosystem science information into existing policy processes. A key to these successes is regular communication and collaborative discourse among modelers, stakeholders, and resource managers to tailor models and ensure they addressed the management needs as directly as possible.
Introduction
Worldwide thinking on fisheries management priorities has been moving away from the mid-20th century paradigm of fishing down our fish stocks with the expectation that we can achieve maximum sustainable yield from all stocks in all ecosystems simultaneously (Larkin, 1996; Link, 2018). The United Nations Convention on Biological Diversity, and the Food and Agriculture Organization (FAO), have created opportunities and principles for nations to individually and cooperatively develop ecosystem approaches to natural resource management, including for fisheries management Prins and Henne, 1998; Garcia et al., 2003; UN Fisheries, and Agriculture Organization [UNFAO], 2003; Un Fisheries, and Agriculture Organization [UNFAO], 2009). The FAO defines the ecosystem approach to fisheries as striving “to balance diverse societal objectives, by taking into account the knowledge and uncertainties about biotic, abiotic and human components of ecosystems and their interactions and applying an integrated approach to fisheries within ecologically meaningful boundaries” (UN Fisheries, and Agriculture Organization [UNFAO], 2003).
The National Oceanic and Atmospheric Administration (NOAA), the United States federal agency responsible for marine fisheries management, adopted an ecosystem-based fisheries management (EBFM) Policy and Road Map in 2016 to articulate the agency’s goals for EBFM and practical steps to implementing those goals (NOAA, 2016). The EBFM Policy defines EBFM similarly to the FAO’s definition for the ecosystem approach to fisheries: “a systematic approach to fisheries management in a geographically specified area that contributes to the resilience and sustainability of the ecosystem; recognizes the physical, biological, economic, and social interactions among the affected fishery-related components of the ecosystem, including humans; and seeks to optimize benefits among a diverse set of societal goals” (NOAA, 2016). Regardless of the particular definition of an ecosystem approach to fisheries or EBFM, managing natural marine resources while taking into account their interactions with their environment and our human interactions with our resources and environment requires the support of ecosystem science.
In the United States, our formal shift toward EBFM began in 1996 with amendments to the nation’s marine fisheries management law, the Magnuson-Stevens Fishery Conservation and Management Act [MSA], 2010. Revisions to our national fisheries science and management priorities included: prohibiting overfishing and recovering overfished stocks, protecting essential fish habitat requiring minimizing bycatch, monitoring and managing the fishing gears permitted for use in marine waters, and a public planning process on exploring and expanding the application of ecosystem principles in fishery conservation and management [Pub. L. 104-297 (1996), Ecosystem Principles Advisory Panel [EPAP], 1998; MacPherson, 2001; deReynier, 2014]. Other United States laws that intersect with the MSA, and that affect the policy processes where ecosystem models may be beneficial include the Endangered Species Act (1973), which guides the recovery of threatened and endangered species; the Marine Mammal Protection Act of (1972), which prohibits the directed take and requires minimizing the incidental take of marine mammals; the Coastal Zone Management Act (1972), which coordinates coastal zone planning between U.S. states, territories, and the federal government, and the Coral Reef Conservation Act (2000). This broad array of federal laws, which still does not include all of the federal laws that address marine resources, intersect with similarly intricate laws from smaller regional jurisdictions within the United States (Crowder et al., 2006). Confusion among scientists about how best to interact with the policy processes associated with these laws is understandable and is likely a factor in the slow progress toward using ecosystem science to guide major policy progress beyond context-setting or improvements to individual species management.
This paper reviews example cases where scientists have had success in using ecosystem analysis and modeling to inform management priorities and stakeholder activities, and identifies practices that help bring new ecosystem science information into existing policy processes. We define successful use of ecosystem models in management processes in two ways: (1) management process success, such as the first time use of ecosystem modeling in a management process, where that modeling helped managers gain insights into interactions within their ecosystems; (2) resource outcome success, where the use of ecosystem models in a management process is expected to improve the health or status of particular fish stocks or habitats. Ecosystem modeling is relatively new to United States fisheries management processes and changes within natural systems are often difficult to monitor and detect; therefore, it may be some years before we can fully assess the success of our work as it may affect the overall health of marine ecosystems. While the case studies presented here are examples from United States marine resource management, the policy issues considered share priorities with fisheries conservation and management practices worldwide. Two of the case studies address management challenges related to setting fisheries harvest levels in changing ecosystems. Two additional case studies address estuarine and marine habitat conservation, and the final case study concerns bycatch minimization.
Case Studies of Ecosystem Models in Practice
The United States has been using the integrated ecosystem assessment (IEA) framework (Levin et al., 2008, 2009) to develop collaborative scientific assessment and policy planning for managing marine resources and habitats. Both the IEA framework and the EBFM Policy include steps toward achieving EBFM that emphasize collaboration and consultation between scientists, policy-makers, stakeholders, and the public. Among the most critical research tools in the United States EBFM effort have been ecosystem models, which can assimilate diverse streams of information and support simulation tests of retrospective or future scenarios, scaled to the ecosystem or management issue in question (Latour et al., 2003; Pikitch et al., 2004; Townsend et al., 2008; Espinoza-Tenorio et al., 2012). Using ecosystem models in support of natural resource management requires not only the careful consideration and analysis of key ecosystem interactions, but also an understanding of where and how policy processes provide opportunities for considering the outputs of those models. Each large marine ecosystem, each nation, and regional governing bodies within nations, will have varying policy processes with varying needs and opportunities for using ecosystem models. Recognizing and working within the practical constraints of those policy processes will improve the use and uptake of ecosystem models in natural resource management (Cormier et al., 2017).
While the term “ecosystem model” has specific meaning in some marine science disciplines, we define the term as a wide range of modeling and analysis tools that are used to support the implementation of EBFM. These tools include conceptual models and related analytical approaches (Harvey et al., 2016) and a variety of biophysical, multispecies, food-web and end-to-end ecosystem models (further described in Plagányi, 2007; Townsend et al., 2008). This range covers models and analysis that consider only a few external factors influencing a single fish stock to a more holistic set of factors (e.g., climate, currents, biogeochemistry, fisheries, human dimensions; Rose et al., 2010; Fulton et al., 2011) influencing multiple, interacting fish stocks. While ecosystem models vary in terms of complexity, software platforms, scale, and scope, ecosystem modelers often adopt similar approaches (“best practices”) to developing models designed to address a marine ecosystem management issue (Townsend et al., 2008; Un Fisheries, and Agriculture Organization [UNFAO], 2008). For example, the five case studies presented herein are at different stages of development, but each case generally follows five steps: identifying the problem and related management process; conferring among scientists, managers and stakeholders; review of initial model results; incorporating additional information; and exploring management actions (Figure 1). The narratives below illustrate the flow of these steps in greater detail.
Atlantic Herring Management Strategy Evaluation
This Atlantic herring case study discusses a process (Figure 2) that integrated information and analyses for several species and fisheries occurring off the Northeastern United States. Participants in this process worked through the New England Fishery Management Council (NEFMC), one of eight United States regional fishery management councils authorized under the MSA to provide advice to the United States government on fisheries management and regulations for activities within the United States exclusive economic zone. This case study provides an example of a novel fisheries management question explored through a traditional policymaking process.
Forage fish are ecologically important links between lower trophic level production and economically and socially important top predators. In many ecosystems, there are also commercially important fisheries targeting forage fish. There has been considerable interest in balancing the direct harvest of forage fish (a provisioning service for humans) with the supporting ecosystem services that they provide (e.g., Cury et al., 2011; Pikitch et al., 2012; Essington et al., 2015; Hilborn et al., 2017). In 2016, the NEFMC initiated a management strategy evaluation (MSE) to develop a harvest control rule for Atlantic herring, Clupea harengus, that considered herring’s ecological role as forage (Deroba et al., 2019; Feeney et al., 2019). The harvest control rule needed to meet all MSA requirements, in addition to considering herring’s role as forage for commercially and recreationally important fishes and for protected predators such as seabirds and marine mammals (Overholtz and Link, 2007). Among other fisheries, herring harvests contribute to the success of the Maine lobster, Homarus americanus, fishery, which uses herring as bait (Ryan et al., 2010; NMFS, 2016).
To understand the interests of many and varied stakeholders with diverse and potentially conflicting objectives, the NEFMC implemented a transparent and inclusive process to select an ecosystem-based harvest control rule and analyze its ecological and economic effects. The MSE was bounded by open stakeholder workshops in recognition of both MSE best practice and MSA requirements for public processes (Feeney et al., 2019). MSE models and analyses were tailored to the specific objectives and performance metrics outlined at the first stakeholder workshop, and were constrained by the management timeline to provide results by early 2017, less than a year after the planning process began (Deroba et al., 2019). This constraint motivated the use of existing models or newly developed models that were relatively simple but still adherent to best practices for multispecies management (Plagányi et al., 2014; Collie et al., 2016; Punt et al., 2016b). Following Plagányi and Butterworth (2012), a previously developed Atlantic herring population model was linked to simple deterministic delay difference models for three predators: bluefin tuna Thunnus thynnus, spiny dogfish Squalus acanthias, and common tern Sterna hirundo.
The MSE process prioritized use of data specific to the Northeast United States continental shelf ecosystem (e.g., Overholtz et al., 2000; Overholtz and Link, 2007; Link et al., 2008; Logan et al., 2015). Multispecies model parameters also drew from regional studies, such as information on herring-bluefin tuna relationships (Golet et al., 2015). Stakeholders helped fill important gaps: some workshop participants worked at seabird refuges and contributed essential data on common tern colony size, fledgling production, and fledgling diet (Deroba et al., 2019; Feeney et al., 2019). In contrast, the relationship between herring and spiny dogfish had to be hypothesized based on trend analysis rather than a clear mechanism, and a lack of existing information prevented the development of delay-difference models for any marine mammal predators of herring (Deroba et al., 2019). Economic analyses were linked to herring population model outputs and limited to performance of the herring fishery (Deroba et al., 2019).
An initial outcome of the MSE process was that several classes of control rules that performed poorly for predators, herring, and the herring fishery were eliminated by consensus at the second stakeholder workshop in December 2016. This left thousands of potential control rules with acceptable predator performance to be further narrowed based on performance for the herring fishery and the herring stock. After many follow-up questions regarding the performance of individual control rules, the NEFMC narrowed the list to ten alternatives for further consideration and National Environmental Policy Act analysis (Feeney et al., 2019). Later, in September 2018, the NEFMC selected a final herring harvest control rule, which took into consideration the role of herring as forage and the outcomes for predator populations, setting aside a portion of the available catch to explicitly account for the important role of Atlantic herring as forage within the ecosystem (New England Fishery Management Council [NEFMC], 2018).
Conducting a multispecies MSE within an existing United States fishery management council process had no precedent, and therefore no formal structure. NEFMC members and staff, NOAA fisheries scientists and policy analysts shaped the MSE collaboratively to ensure that the stakeholder workshops and resulting analyses would be useful in NEFMC decision making. Communication between the managers, analysts and interested stakeholders took place throughout the process (Feeney et al., 2019). During the process, an external expert committee reviewed the MSE, endorsed it as best available science for NEFMC decision making, and suggested possible improvements for future iterations (Feeney et al., 2019). Although no schedule for revisiting control rule performance has been set, standard practice is to evaluate management procedures based on MSEs at approximately 5-year intervals (Plagányi et al., 2007; Rademeyer et al., 2007; Punt et al., 2016a). Other outcomes of this process include improved understanding of both the MSE process and multispecies interactions in the New England region (Feeney et al., 2019). The MSE process succeeded in introducing a wider range of ecological information into the larger fishery management council process, and supported strategic decision making based on simple multispecies modeling approaches. Overall, the NEFMC balanced multiple objectives in refining herring management, but it stated that it selected its control rule “to explicitly account for the important role of Atlantic herring as forage within the ecosystem” (New England Fishery Management Council [NEFMC], 2018). Considering ecological objectives is a critical first step toward the routine use of ecosystem analysis and modeling in fishery management. In the future, addressing societal benefits across a wider range of predators, ecological feedbacks, and fishery interactions would allow us to more fully evaluate harvest control rules.
Gulf of Alaska Pacific Cod Harvest
This Gulf of Alaska Pacific cod harvest case study (Figure 3) discusses how the North Pacific Fishery Management Council (NPFMC) addressed a surprising environmental shift that challenged their customary assessment and harvest rule setting process (Figure 4; Barbeaux et al., unpublished). Although the policymaking process is similar to that discussed in the Atlantic herring case study, the management question in this case study was driven by forces external to that process.
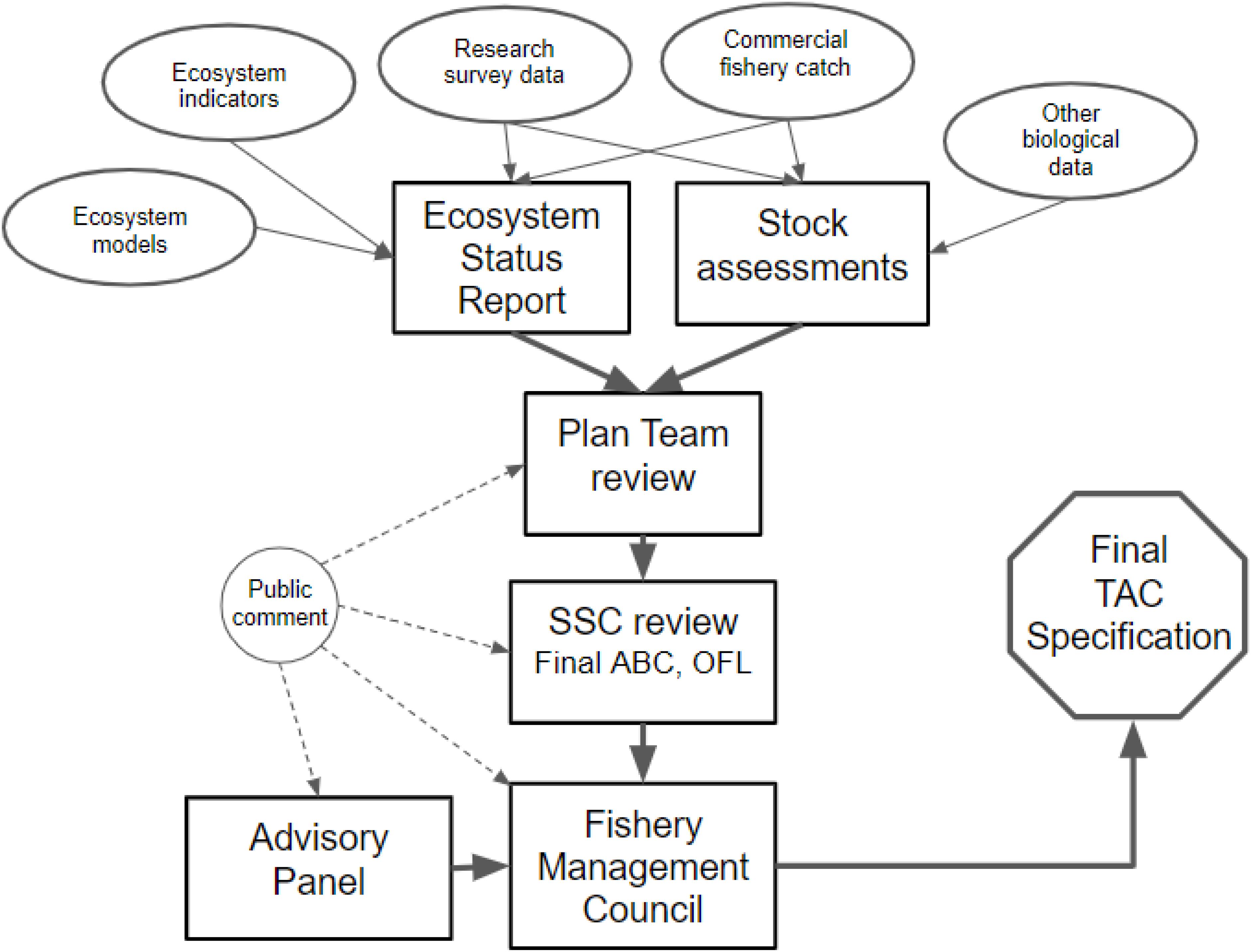
Figure 4. Summary of annual groundfish federal management cycle in Alaska, from assessments through council review and final catch quotas. ABC, allowable biological catch; OFL, overfished level; TAC, total allowable catch (i.e., catch quota).
Alaska supports the largest federally managed fisheries in the United States, with landings of groundfish—such as Pacific cod Gadus macrocephalus, walleye pollock Gadus chalcogrammus, sablefish Anoplopoma fimbria, and various flatfish—that totaled 2.3 million metric tons in 2016 (Fissel et al., 2017). Under the MSA, the NPFMC establishes annual catch limits based on recommended allowable biological catch (ABC) from, in most cases, age-structured stock assessment models. NOAA’s Alaska Fisheries Science Center supports EBFM by directly incorporating ecosystem-informed parameters into a few stock assessments (Marshall et al., 2018), and by presenting additional ecosystem information in tandem with individual stock assessments (Zador et al., 2017a). This coordinated process allows for ecosystem information, which is a synthesis of myriad data sources and model outputs, to be used to support any proposed reduction from the maximum ABC recommended by an individual stock assessment.
In 2017, the abundance estimates of Pacific cod, as observed in the biennial Gulf of Alaska bottom trawl survey of groundfish stocks, dropped by 71% from the previous survey in 2015 (Barbeaux et al., 2018). This resulted in an 80% reduction in the stock assessment-derived ABC from the previous year and a 77% reduction of what had been expected from previous assessments. The drastic change in the final catch limit that was set for 2018 represented a major impact on the Gulf of Alaska fisheries that target Pacific cod as well as towns such as Kodiak, Alaska, where Pacific cod play a vital role in the local economy in this rural, fishing-dependent island community (Himes-Cornell et al., 2013).
The Gulf of Alaska experienced an unprecedented marine heatwave from 2014 to 2016, which caused persistent and widespread sea surface temperature increases of 1–2°C and extensive ecological responses (Bond et al., 2015; Di Lorenzo and Mantua, 2016; Zador and Yasumiishi, 2017). The NPFMC had been informed of the changes in the ecosystem during the heatwave (Figure 3), but Pacific cod was the first managed stock to show a steep decline that could be explained in part due to the heatwave (Zador et al., 2017a; Barbeaux et al., 2018). Collaboration among the stock assessment author and ecosystem scientists resulted in: (1) an explanation of the Pacific cod decline due to heatwave-related increased adult mortality and lack of recruitment in the stock assessment (Barbeaux et al., 2018), and (2) an ecosystem model-based assessment of Pacific cod bioenergetics and diet limitations within the context of trophic-level wide negative impacts of the marine heatwave in the ecosystem assessment (Zador and Yasumiishi, 2017).
From the time when the trawl survey data were available through the setting of the final Pacific cod catch limit (∼3 months), ecosystem and stock assessment scientists, fisheries managers, and industry stakeholders communicated frequently about findings under development (Barbeaux et al., unpublished). Communication occurred among all three groups during formal processes such as management and industry meetings and informally through direct communication, with the end result that the final, drastic cut to the catch limit was accepted without controversy, demonstrating success in the management process. The NPFMC has a longer familiarity with ecosystem models and information than many of the fishery management councils; that familiarity ultimately supported their swift response to challenges for a particular fish stock within an ecosystem perturbed by climate variability. The ecosystem science-based explanations for the Pacific cod decline were integral to building trust among stakeholders in the management decisions. Growing recognition of the importance of communication and transparency for successful EBFM has provided further impetus to formalize the incorporation of ecosystem science into fisheries management processes. This challenge is being met with the development of a suite of climate-informed ecosystem models, a fishery ecosystem plan that incorporates conceptual and quantitative models, and development of risk tables within stock assessments that include quantification of ecosystem concerns external to the assessment models (Barbeaux et al., unpublished).
Coastal Louisiana Restoration
For this case study, we move beyond the fishery management council process to habitat-based processes that support United States natural resource management priorities for estuaries and coastal zones (Figure 5). The MSA requires characterizing and protecting essential fish habitat, and the Coastal Zone Management Act (1972) requires the United States federal government to work with U.S. states and territories to support regional approaches to preserving and protecting the nation’s coasts. A priority for fisheries ecosystem planning under the EBFM Road Map is: “Facilitating the participation of external federal, state (including territories), and tribal partners in the EBFM process by assessing the cumulative effects of human activities on marine ecosystems to help partners minimize the effects of non-fishing activities on trust living marine resources and habitats.” This coastal Louisiana restoration case study explores the use of ecosystem models in an emerging and novel cross-mandate policymaking process.
The U.S. state of Louisiana, with much of its ecology shaped by the Mississippi River and its coastline on the Gulf of Mexico, is experiencing substantial losses of coastal land due to channelization of the outflowing Mississippi River and due to land subsidence. Multiple federal and state authorities have interests in and mandates related to freshwater marshes and coastal habitat restoration and protection in the Mississippi River Delta. Louisiana’s Coastal Protection and Restoration Authority (CPRA) serves as a central authority for the state’s coastal management entities. CPRA coordinates state coastal habitat restoration and cooperation with federal authorities on hurricane response. CPRA’s Louisiana Coastal Master Plan is intended in part to guide the construction of large-scale sediment diversion projects to partially redirect the flow of the Mississippi River and provide sediments to rebuild depleted marsh habitat (Coastal Protection and Restoration Authority of Louisiana [CPRA], 2017). Ecosystem models have been essential for linking together the complex dynamics and currencies that span the interdependent terrestrial, aquatic, marine and social-economic components of this system.
Federal agencies working on and with sediment diversion projects in Louisiana have interests in the engineering aspects of these projects under the United States Army Corps of Engineers (USACE), in natural resource management aspects under the United States Fish and Wildlife Service and NOAA, and in hazardous weather monitoring and response under NOAA. Together, these agencies developed a USACE feasibility study on the potential effects of a suite of sediment diversion projects, and a NOAA plan for ecosystem science and modeling of the proposed diversion projects. To develop its feasibility study, the USACE formed a project development team of federal and state agencies with habitat and fish and wildlife authorities in the region (U.S. Army Corps of Engineers [USACE], 2012). Scientific work in support of the team crossed disciplines to evaluate the potential effectiveness of a suite of diversion projects at rebuilding marshes and the potential consequences of habitat changes on living marine resources and the coastal and marine ecosystem.
Coastal Protection and Restoration Authority and USACE used hydrological and ecological models to coordinate the analysis and planning process for sediment diversion projects. The Delft-3D hydrological model was used to predict the flow of sediment, salt, and other water quality components with and without the operation of the proposed diversion projects (Meselhe et al., 2015). The Delft-3D model allowed agencies to evaluate the effectiveness of diversions for rebuilding marsh habitat, and the influence on water quality factors like salinity. Outputs and ancillary products from the Delft-3D model were used as inputs for two ecosystem models, Ecopath with Ecosim and Ecospace, and the Comprehensive Aquatic Systems Model (Expert Panel, 2014; de Mutsert et al., 2017). These ecosystem models were used to evaluate the effect of diversion operations on the biomass and distribution of key fishery species.
Modelers for the hydrological and ecological models regularly consulted with the multi-agency project development team during the model development processes. These interactions with agency stakeholders helped modelers to understand expectations for the analysis and to get access to needed data. Conversely, agency stakeholders were able to understand the capabilities and limitations of the models and analysis. One key limitation for the ecological models was the lack of long time series of data on important ecological groups, a common challenge in ecological modeling (de Mutsert et al., 2017).
This integrated policy-making scientific modeling process was the first of its kind for the ecosystem-scale projects proposed for the region. Development and review of models was somewhat ad hoc; although the process was successful enough to serve as a framework for planned future analyses of two of the sediment diversion projects (e.g., the Mid-Barataria and Mid-Breton sediment diversion projects). CPRA commissioned an expert panel to provide independent review of the models and analyses, and presented results to the agency stakeholders.
The initial multiple-model evaluation of all of the sediment diversion projects in the region allowed CPRA to proceed with a sediment diversion project in the Barataria Basin of Louisiana (Coastal Protection and Restoration Authority of Louisiana [CPRA], 2014). Stakeholders in the Barataria Bay area have asked for more precise estimates of how the diversion operations would affect the biomass and distribution of key living marine resources as well as the ecosystem structure. Modelers on the project have emphasized the difficulty of making long-term projections about complex ecosystems. To account for and adapt to these concerns, scientists and natural resource managers in the region are investigating adaptive management approaches to ensure that system monitoring and modeling is ready for the eventual implementation of sediment diversion projects. Ecosystem models are being used at the next stage of restoration planning for a diversion in Barataria Bay that will characterize food web dynamics under current conditions. In addition, these models are being used to guide development of a monitoring and adaptive management plan for the restoration process. It is too soon to evaluate resource outcome success for this process because management actions are still under review and have not yet been implemented.
Hawai’i Coral Reefs
Like the Louisiana case study, this Hawai’i case study focuses on marine habitat (Figure 6). In the United States, coral reefs are protected under a variety of laws, including MSA and CZMA discussed above, as well as the Coral Reef Conservation Act (2000). This case study was initiated through the regional Integrated Ecosystem Assessment (IEA) process (PIFSC, 2016). By bringing together scientists, policy makers and an engaged community, and the overall desire to reverse the declines in both coral cover and fish biomass, there was an interest in exploring various management regulations that could mitigate or reverse the downward trend in natural resources. Additionally, in 2016, the governor of Hawai’i pledged to “effectively manage” 30% of the marine areas along Hawai’i’s coastline by 2030. However, defining “effectively” is left up to the managers of the Hawai’i Division of Aquatic Resources (DAR) through a multi-year spatial planning exercise. This case study looks at using ecosystem models to consider issues that cross federal, state, and local mandates and processes.
In Hawai’i, coral reef ecosystems are degrading in many regions due to land-based pollution, fishing, coastal development and other local stressors combined with the devastating 2015 coral mortality from ocean warming (Friedlander et al., 2008; Couch et al., 2014; Bahr et al., 2017). Though coral reefs can recover over decades, climate models project that coral bleaching related mortality may occur annually within the next 20–25 years (van Hooidonk et al., 2016). Changes in marine resource management are needed to improve recovery of ecosystem structure and services. The majority of Hawai’i’s reefs are within state waters, however, under the Coral Reef Conservation Act, and specified in the EBFM road map, NOAA works with jurisdictions to support coral reef conservation and management. Hawai’i’s coral reef management embraces an ecosystem-based approach to management to guarantee that ecosystem services such as fishing and a resilient ecosystem structure are maintained or improved.
Two recent efforts to support local decision making have included the development of ecosystem models. In both cases, the local managers identified the management scenarios for model simulation. One effort was led by the University of Hawai’i at Mānoa (UH) and involved Pacific Islands Fisheries Science Center (PIFSC) scientists in developing the model, while the second effort resulted from PIFSC discussions through its IEA process. In both cases, scientists proposed to develop a model, and alternative management strategies were identified in consultation with DAR. The ecosystem modeling platform used by UH was HIReefSim (Hawai’i Reef dynamics Simulator) for the islands of Maui, Moloka’I, and Lana’i (Weijerman et al., 2018b). HIReefSim details dynamics of five benthic groups (three algal and two coral groups) and two fish groups (herbivorous and piscivorous fish) and is based on gridded (500 × 500 m) base maps of initial conditions and main stressors, such as climate change (leading to coral mortality), land-based sources of pollution and fishing (Melbourne-Thomas et al., 2011). Selection of this model was based on its compatibility with the DAR effort of selecting areas (grid cells) using MARXAN (Ball et al., 2009) and the fact that HIReefSim can simulate land-sea dynamics. The idea being that results from MARXAN identified areas where management would be warranted and HIReefSim could evaluate the tradeoffs of alternative management options that include land based and marine based scenarios. The modeling software used in the IEA effort was Ecopath with Ecosim (EwE; Polovina, 1984; Christensen and Walters, 2004) for Puako Bay on the West Coast of Hawai’i Island (Weijerman et al., 2018a). Selection of EwE was based on its focus on just one bay and the ability to include all fish species and gear restriction as management options.
The process of developing the model was ad hoc. Models were developed by PIFSC scientists in collaboration with scientists from UH, the United States federal Environmental Protection Agency, Gulf Ecology Division, Oregon State University for HIReefSim, and with staff from The Nature Conservancy and DAR for EwE. In both cases, the developed models were used as examples of how ecosystem models can be used as decision support tools in the face of climate change by quantifying socio-ecological tradeoffs of alternative fisheries and land-based management policies. UH is in constant dialog with DAR to discuss the usefulness of HIReefSim as a management-support tool for their spatial planning. The EwE model was developed in collaboration with DAR to ensure that the simulated policy regulations were relevant for actual implementation. Results showed that the “only line fishing” scenario in combination with a reduction in nutrients and sediments generated the most balanced trade-off between marine resource users and ecosystem resilience. In both cases, results were presented to DAR and were well received. Upon request from DAR, HIReefSim is now being parameterized for Kaneohe Bay on the windward coast of Oahu and there is also interest for developing HIReefSim for other areas. The results of the EwE model were presented at a regional IEA symposium to the public, scientists, non-governmental organizations, fishers and managers. DAR has since requested similar model development for other geographic areas, but potential further development awaits funding.
From a management process perspective, ecosystem modeling and analysis was used for strategic management decisions, i.e., to get insight in the socio-ecological tradeoffs in alternative marine and land-based management strategies. Interest in the use of ecosystem models to evaluate potential changes in ecosystem structure attributable to changes in water quality (e.g., temperature, nutrients) and fish biomass (e.g. herbivorous fishes) is present. Models output have highlighted potential impacts to various stakeholders (tourist, fishers) and the overall ecosystem structure and resilience. At this stage, resource outcome success cannot be evaluated as the DAR spatial planning exercise is still ongoing, and the state of Hawai’i has not yet decided what constitutes “effective” management. However, DAR’s positive reception of the regional ecosystem models, provides a useful example of how to incorporate ecosystem models into the decision making process and will facilitate the ongoing discussion between UH, PIFSC and DAR about “effective” coastline management into the future.
Dynamic Ocean Management in the California Current Ecosystem
For this final case study, we return to the fishery management council process to look at bycatch minimization off the United States West Coast (Figure 7). Dynamic ocean management in the California Current Ecosystem combines multiple species distribution models to emulate a simple ecosystem model, providing nowcasts for potential bycatch issues in fisheries for highly migratory species. NOAA scientists worked with the West Coast Regional Office, Pacific Fishery Management Council (PFMC) and West Coast fishermen to make both the model and its inclusion into management processes and the fishery itself more practical for on-the-water use. Bycatch in these fisheries includes species protected under the Endangered Species Act and Marine Mammal Protection Act, laws with different and sometimes competing priorities from the MSA. The participating scientists, managers, and stakeholders had to use the fishery management council process to simultaneously meet these different priorities to come up with solutions that also worked for the fishing fleets subject to these laws.
Federal fishery management policies in the MSA are implemented through regional fishery management plans, which guide tactical decision-making for the management of individual stocks and species groups. Decisions such as harvest limits and allocations are often made annually, and decisions on where and when fishing can occur may range from short-term (seasonal) schedules to long-term designations of areas opened or closed to particular gear types. These scales and time frames for management are practical, given the time, effort and data required for conducting stock assessments, technical and public review, evaluating alternatives, and developing final decisions. In the United States, this process has been successful in maintaining sustainable fishing pressure on target species, rebuilding populations of overfished species, and reducing bycatch of protected species. However, the resulting plans can constrain opportunities for the fishing industry if the management action is conservative and overly lacking in flexibility for adjustments in space and time (Maxwell et al., 2015; Dunn et al., 2016). In addition, anomalous years can result in protected species shifting out of their normal habitats, where protections may be in place, and into unprotected waters, leading to crises such as mass entanglements of North Atlantic right whale (Eubalaena glacialis) in fishing gear during 2017 (Meyer-Gutbrod and Greene, 2018).
One solution is “dynamic ocean management,” which employs species data and distribution models to provide fishermen with real-time spatial estimates or short-term forecasts of fishing conditions and bycatch risks (Hobday et al., 2014; Maxwell et al., 2015). Many dynamic ocean management tools employ species distribution models to track changes in ocean conditions and estimate the probabilities of encountering each target and bycatch species in a given area, which are then combined using individual risk weightings to produce a single product (Hazen et al., 2018; Welch et al., 2019a). Providing output from these models thus allows managers and fishing vessels to assess fishing opportunities and risks of protected species bycatch at much finer spatiotemporal scales than large closures implemented over long time periods.
The EcoCast tool1 is one example of a dynamic ocean management tool from the United States West Coast (Hazen et al., 2018). The California drift gillnet fishery targets swordfish (Xiphias gladius) and secondarily mako sharks (Isurus oxyrinchus) and thresher sharks (Alopias vulpinus) under the PFMC highly migratory species fishery management plan (Eguchi et al., 2017; Mason et al., 2019). Multiple management measures have coincided with the decline of fishery effort over the past 20 years (Mason et al., 2019). In particular, bycatch of protected species continues to constrain the fishery such that swordfish harvest in United States waters is well below maximum sustainable yield. In 2001, a drift gillnet fishery closure was implemented from August to November in a 552,000-km2 area that encompassed 22 different bycatch events of federally endangered leatherback turtle (Dermochelys coriacea) (66 FR 44549, August 24, 2001; Eguchi et al., 2017). This closure severely limited drift gillnet fishing opportunity (Hazen et al., 2018; Mason et al., 2019). EcoCast was developed to provide a dynamic approach to test and improve on the static closed area, by providing nowcasts of target species catch (swordfish), protected species bycatch [leatherback turtle, California sea lion (Zalophus californianus)], and fish bycatch [blue shark (Prionace glauca)] (Hazen et al., 2018). In all scenarios, managers and fishers themselves face tradeoffs among catch and bycatch in where to fish; thus EcoCast implements a weighting scheme that reflects management priorities when coming up with estimates of catch and bycatch risk (Hazen et al., 2018). These estimates are produced daily, and additional analyses have been added to assess uncertainty caused by missing ocean data (e.g., poor satellite coverage; Welch et al., 2019a, b). At the request of fishermen and managers, EcoCast is being updated to use high-resolution ocean model output (daily and 10 km; Brodie et al., 2018) instead of remotely sensed data (daily and 25 km), and to incorporate new species such as protected cetaceans. Most recently, EcoCast was presented to swordfish fishermen with the hope of ultimately improving the utility of EcoCast with on the water validation.
The National Oceanic and Atmospheric Administration scientists first presented EcoCast to the PFMC in 2014 and 2015, after National Aeronautics and Space Administration had funded the project but before work had begun. This was done to alert the PFMC of the tool and to get feedback on the framework and approach. Draft models and data integration approaches were presented to PFMC advisory bodies for technical review in the fall of 2016. EcoCast was also presented twice to the fishing community during development to incorporate their feedback, and has been presented three more times to fishermen seeking fishing permits. As an example of the value of stakeholder feedback, one feature under development was a smart phone application for uploading opportunistic sightings data and to distribute model results, but many of the fishermen did not use smart phones, which lowered the value of the proposed feature. The EcoCast team thus developed two web-based alternatives available by smartphone or computer: a map product2 that can be downloaded with the limited bandwidth available at sea; and an explorer tool3 to explore risk weightings and how changing weightings affect the map product. The final product and tool was presented on the PFMC floor in November of 2017, going live at the same time for the 2017 fishing season. Researchers are currently working on exploring the utility of EcoCast for additional gear types, assessing the efficacy of the existing spatiotemporal closures, and identifying other fisheries where a similar dynamic modeling approach may be beneficial.
While EcoCast has been successfully explained to and shared with managers and the public, management of allowable fishing gears and locations for highly migratory species fisheries within the United States West Coast exclusive economic zone has been in flux for several years. EcoCast use will likely remain experimental in the near-term, although with benefits to fisheries and protected species. While this case study illustrates successful development of an ecosystem model and tools appropriate to fisheries management, it also shows that management processes often face challenges to using ecological models that are not at all associated with the quality or utility of the models themselves.
Discussion
Each of these case studies provides an example of using some level of ecosystem modeling to advance EBFM. The Dynamic Ocean Management example used relatively simple analysis of simple spatial and gear interactions among species to improve options for minimizing bycatch in a particular fishery. The Coastal Louisiana and Hawai’i case studies used complex food-web models. The Atlantic Herring example used simplified food web interactions and economic models. The Gulf of Alaska case focused on environmental drivers and bioenergetic models.
In these examples, ecosystem modeling and analysis was used to support ecosystem-based fisheries management decision-making. The Alaska, Atlantic, and California examples, illustrate the use of ecosystem modeling in direct ecosystem-based fisheries management decisions. That is climate, habitat, ecological, or human dimensions information was quantified and used to adjust how at least one fishery was managed or to allow flexibility in how the fishery was executed. In the Hawai’i example, discussions on how to best implement EBFM to effectively manage 30% of the coastline are still on-going but these are informed by the modeling efforts. Although the Coastal Louisiana example was less focused on direct fishery management and more focused on habitat management, identifying and understanding essential fish habitat is an important component of the MSA’s vision for fisheries management and habitats are a relevant unit of analysis to help operationalize EBFM (Marshak and Brown, 2017). For the habitat-oriented models (Hawai’i and Louisiana), a broader set of stakeholders and agencies were involved, and a less well-defined decision-making process (i.e., not a structured fisheries management council approach) was in place, so the efforts were more geared toward heuristic understanding of the focal systems and strategic management of habitats for supporting a broad set of fish stocks.
In these examples, participating scientists used best practices for model development and implementation. Over the past decade scientists and administrators at NOAA Fisheries Service have regularly convened a National Ecosystem Modeling Workshop (NEMoW) in support of living-marine resource management and to more formally review, evaluate, and support the ecosystem modeling efforts of NOAA Fisheries. The general objectives of the NEMoWs are: (1) to address broad questions of national interest for applied ecosystem modeling for living marine resource management, (2) to provide a forum for ecosystem modelers and scientists in the agency to network and share information on ecosystem modeling advancements and best practices, and (3) to provide a vehicle to advance ecosystem modeling within NOAA Fisheries as it meets its mandates and obligations. Scientists involved in these workshops have generated recommendations and best practices for ecosystem modeling and analysis and have documented them in a series of technical memoranda (Townsend et al., 2008, 2014, 2017; Link et al., 2010). The list of recommendations and best practices from these reports is extensive. A few major recommendations that have been discussed in multiple workshops include:
(1) Develop and maintain ecosystem modeling capacity and infrastructure, because anticipating management needs can help to ensure utility and relevance of modeling efforts.
(2) Apply iterative communication with managers and stakeholders; get them accustomed to seeing ecosystem models and analysis to build credibility.
(3) Ensure periodic review (informal: colleagues, stakeholders, managers) throughout model building, to avoid rejection of model at a late formal review stages when problems (model structure, mismatch of objectives, etc.) could be caught earlier.
(4) Use multiple models to address uncertainty in model structure and major ecosystem drivers - apply a range of multiple-model analytical or quantitative approaches appropriate for the type of question, the types of uncertainty, and amount of data available.
(5) Implement an MSE framework for providing and ecosystem context for living marine resource management and for exploring uncertainty.
Many of these best practices have been suggested elsewhere (e.g., Un Fisheries, and Agriculture Organization [UNFAO], 2008). Most of these best practices and recommendations have been implemented by ecosystem modelers and scientists in the United States, and as illustrated in these example cases, this has led to progress in advancing EBFM in the United States. A longstanding ecosystem modeling capacity and infrastructure for Alaska and the Northeast, enabled science centers to respond to requests by management councils to address their ecosystem concerns in a timely fashion. In those cases, prior regular, iterative communications between councils and ecosystem scientists had helped the scientists to build credibility in their analytical products and facilitated the use and further development of products for EBFM decision-making. In all of these examples, periodic informal review was used to help guide tool development and ensure a level of utility to managers and stakeholders. In the Dynamic Ocean Management case, regular stakeholder review was especially important for honing the tools and ensuring their utility. In the Coastal Louisiana and Hawai’i examples, a multiple model approach was implemented to allow exploration of the influence of drivers and stressors on focal ecosystems. This laid the groundwork for future ecosystem modeling and analysis to inform management actions. The Atlantic Herring case illustrates the use of MSE as an approach for providing ecosystem context to fisheries management and enabling managers to make an ecosystem-based decision.
Collaborative Processes for Ecosystem Model Use in EBFM
At the heart of each case study is dedicated collaboration between scientists, managers, and stakeholders from early in the process of ecosystem model development and use. As many authors have concluded, long-term commitment to collaboration is essential to successful EBFM because it clarifies objectives, promotes participation and information exchange, facilitates identification of tradeoffs, and ultimately builds trust and investment among parties (Peterman, 2004; Caddy and Seijo, 2005; Levin et al., 2009; Link et al., 2012). The collaborative process generalized in Figure 1 and customized in different case studies provides real-world illustration of the efforts involved and the benefits derived.
The first step of the common collaborative framework, identifying the policy problem through an existing resource management process (Figure 1), is an essential starting point that has often proven difficult to achieve, perhaps because the initial question scientists ask (“What are your ecosystem objectives?”) can be very abstract to managers and stakeholders in the absence of more specific context. Contemporary fisheries management has many very specific objectives articulated in legislation such as MSA and further honed in regional fishery management plans. Ecosystem objectives are not spelled out as clearly in legislation, and fishery management councils have only recently begun to adopt fishery ecosystem plans (FEPs) (Pew Charitable Trust Issue Brief, 2015). However, the case studies here are founded in specific policy questions that are anchored in existing resource management processes in which the managers and stakeholders are deeply invested (Figure 1). This is likely a better starting point from which to build and tailor ecosystem models than “what are your ecosystem objectives?”
The next two steps in Figure 1 involve conferring early with managers and stakeholders to ensure that scientists have an appropriate understanding of the policy problem and the capabilities of different ecosystem models and products; and to frequently reconvene to ensure that all parties continue to correctly understand and characterize management needs and model results. Specifying and reaffirming such details is of great importance for several reasons. First, defining key details up front (e.g., appropriate spatial scales, temporal scales, currencies of model outputs, platforms of product delivery) improves the likelihood that model products will meet the expectations of managers and stakeholders. This should support more efficient implementation, and reduce the need for time-consuming, expensive revisions of models late in the process. Second, the initial dialog puts an emphasis on building models that are tailored to the specific management problem, rather than trying to redefine the problem so that it can be tested in an existing model with no major adjustments to the model structure. Tailoring the model to the problem is an essential best practice of ecosystem modeling). Third, dialog enables managers and stakeholders to become more familiar with the assumptions, strengths and weaknesses of a modeling framework. This transparency increases trust among participants; it also encourages managers and stakeholders to contribute their wealth of accumulated knowledge, which can help to identify specific mechanisms that a model should include, or to highlight information that can assist in parameter development or filling of gaps (Miller et al., 2010). The MSE workshop process described in the Atlantic herring case study (Deroba et al., 2019; Feeney et al., 2019) is an excellent illustration of how this emphasis on ongoing dialog reaps benefits throughout the course of ecosystem model development and implementation.
The fourth step is to assess additional scientific information and analyses needed to address the problem. This step emphasizes the need for the process to maintain sufficient flexibility to adapt as understanding of a management problem evolves or the nature of the problem changes. Several of the case studies above arose from events that required an urgency in response (e.g., rapid coastal erosion in Louisiana; sudden decline in Gulf of Alaska Pacific cod; bycatch of endangered leatherback turtles), and such events tend to incite considerable attention and research effort, which can generate important new information that can hone ecosystem-level management objectives and add valuable functionality to ecosystem models. This step can also further build trust and transparency among scientists, managers and the public, such as in the Atlantic herring case where stakeholders were able to provide data that filled modeling gaps. This step accentuates the value of having relatively simple, readily adaptable models as part of a model ensemble (e.g., Plagányi et al., 2014; Collie et al., 2016; Punt et al., 2016a). One additional example of a relatively simple, adaptable approach is qualitative network modeling, for instance used in the Gulf of Alaska (Zador et al., 2017b) and in the California Current IEA (Harvey et al., 2016). In these applications, qualitative modeling has proven useful for incorporating stakeholder and management input, understanding linkages from ecology to human communities, and modeling portions of the ecosystem that are not well sampled (i.e., “data-poor”). This step of addressing scientific analyses and data needs should also be valuable if one assumes that the problem is going to require long-term, adaptive management: building in a process for onboarding new information, from researchers, stakeholders, and local ecological knowledge (Ainsworth, 2011; Beaudreau and Levin, 2014) into ecosystem models should improve the value and efficiency of model-based decision support.
The final step in Figure 1 is to explore the possible management actions that can lead to better, even if not perfect, outcomes under existing legal mandates and policy processes. This step is at the heart of MSE, as outlined in the Atlantic herring case study, and is also evident in the outcomes of the Gulf of Alaska cod and Louisiana sediment diversion case studies; the other two case studies have not reached the stage of full management implementation yet but the collaborative relationships are in place. This step further ensures a participatory process, in that the possible management actions and outcomes are best defined by the managers who will implement the actions and the stakeholders who will be affected by them.
Conclusion
This manuscript was intended as a broad, brief review to illustrate the use of ecosystem modeling and analysis to advance EBFM in the United States. A more focused review on specific aspects of modeling and EBFM could provide more detailed, actionable steps for making progress. This review highlights the processes and broad steps needed for continued progress toward EBFM and provides some evidence that best practices, when implemented, provide positive results.
To implement EBFM, a broad suite of tools beyond single species/stock population dynamics models are needed. These examples emphasize the need for models that incorporate a range of biophysical factors influencing stocks and interactions among fish stocks. They also illustrate a need to incorporate social and ecological factors. Most significantly, these case studies emphasize the need for ecosystem modelers to have or find access to policy-making processes. Where clear, established processes exist, like fishery management council processes, ecosystem modelers are introducing new science tools to those processes. Where policy-making processes have to be first designed by participants, modelers may face practical challenges in figuring out who to consult with and how their work might influence decisions.
A broad view of fisheries management is also necessary. Within the traditional single species/stock management process, there are limited controls (harvest regulations) to influence the status of a stock. However, other mandates enable some additional regulation of other external factors that influence stocks (e.g., habitat regulations). Moreover, the application of simple ecosystem models enables fisheries management (e.g., managing bycatch) on a finer time scale and with more agility than the conventional fisheries management processes. Ecosystem modeling can help make the connections between these external factors, their control, and stocks.
Communication among modelers is needed. Modelers focused on a particular ecosystem can benefit from regular interactions with modelers in other systems. Regular organized communication among model groups facilitates development, revision, and implementation of methodologies as well as developing and implementing best practices of model use.
Communication between modelers, managers, and stakeholders is essential. These examples demonstrated that early and frequent communication among these groups expedited model implementation and management decision-making. The cases highlighted that continued communication with stakeholders enabled model refinement and ensured their utility. While this level of communication has been beneficial, further iterative and systematic communication would also benefit scientists, managers, and stakeholders. Doing so would move modelers responding to urgent and critical events to providing strategic and tactical advice for holistic planning and operational use. One forum for this type of communication in the fisheries realm is annual Ecosystem Status Reports delivered to United States fishery managers through the IEA process (Zador and Yasumiishi, 2017; Gaichas et al., 2019; Harvey et al., 2019). Repeated communication allows modelers to transition from responding to urgent and critical events, to providing strategic advice for operational use. This can include holistic risk assessments (Gaichas et al., 2018; Samhouri et al., 2019) and planning for climate change effects, for instance for the California drift gillnet fishery considered by EcoCast (Smith et al., unpublished). This type of risk assessment can lead to further ecosystem modeling and analysis. Risk assessment is the first step in Mid-Atlantic Fishery Management Council’s ecosystem process that also includes social-ecological conceptual modeling, potentially leading to MSE addressing social-ecosystem linkages (Gaichas et al., 2016).
Science in support of EBFM has evolved and grown over time, and relies on multidisciplinary models to illustrate and weigh the tradeoffs we make in managing natural marine resources. Ecosystem models can inform the IEA process and support EBFM efforts by: (1) synthesizing available data to help us understand and assess system dynamics, (2) testing scenarios of the risk to key species of top-down or bottom-up mediated stressors, (3) testing scenarios of the effectiveness and tradeoffs of management strategy alternatives at meeting the various requirements of a nation’s or region’s natural resource management laws and policies. Ecosystem modelers themselves can support EBFM efforts by reaching out to managers and stakeholders for a better understanding of management process challenges and possibilities.
Author Contributions
All authors listed have made a substantial, direct and intellectual contribution to the work, and approved it for publication.
Conflict of Interest
The authors declare that the research was conducted in the absence of any commercial or financial relationships that could be construed as a potential conflict of interest.
Acknowledgments
We would like to thank the NOAA Fisheries Science Board for supporting the NEMoW meetings that enabled modelers to exchange ideas and interact with managers in a less formal environment as we developed approaches for using models to address management needs. As the authors have noted the value of clear communications and collaborative work with stakeholders and managers, we would like to acknowledge partners who collaborated in the progress toward EBFM highlighted in these example cases. Atlantic Herring Management Strategy Evaluation: Jonathan Deroba and Min-Yang Lee, NOAA Fisheries NEFSC; Rachel Feeney and Dierdre Boelke, NEFMC; Brian Irwin, USGS and University of Georgia, all participants in the NEFMC herring MSE stakeholder process. Gulf of Alaska Pacific Cod Harvest: Steven J. Barbeaux, Kirstin K. Holsman, and Ellen Yasumiishi, NOAA Fisheries AFSC. Coastal Louisiana Restoration: Cherie Price, United States Army Corps of Engineers; Dr. Kim De Mutsert and Dr. Kristy Lewis, George Mason University; Dr. Shaye Sable, Dynamic Solutions, Inc.; Dr. Ehab Meselhe, The Water Institute of the Gulf; Project development team comprised of employees from Coastal Protection and Restoration Authority, NOAA, USFWS, National Resources and Conservation Service, Louisiana Department of Wildlife and Fisheries, Louisiana Department of Natural Resources, and United States Army Corps of Engineers. Hawai’i Coral Reefs: William Walsh, DAR-Kona; Dwayne Minton, TNC; Jamison Gove, Ivor Williams, and Jeff Polovina PIFSC; Lindsay Veazey and Kirsten Oleson, University of Hawaii – Manoa; Brian Neilson, Anne Chung, DAR. Dynamic Ocean Management in the California Current Ecosystem: Amber Rhodes, Rachael Wadsworth, Tina Fahy, and Heidi Taylor, WCR. Heather Welch and Stephanie Brodie – University of California Santa Cruz.
Footnotes
- ^ https://coastwatch.pfeg.noaa.gov/ecocast/
- ^ https://coastwatch.pfeg.noaa.gov/ecocast/map_product.html
- ^ https://coastwatch.pfeg.noaa.gov/ecocast/explorer.html
References
Ainsworth, C. H. (2011). Quantifying species abundance trends in the Northern Gulf of California using local ecological knowledge. Mar. Coast. Fish. 3. 1, 190–218. doi: 10.1080/19425120.2010.549047
Bahr, K. D., Rodgers, K. S., and Jokiel, P. L. (2017). Impact of three bleaching events on the reef resiliency of Kāne‘ohe Bay, Hawai‘i. Front. Mar. Sci. 4:398. doi: 10.3389/fmars.2017.00398
Ball, I. R., Possingham, H. P., and Watts, M. (2009). “Marxan and relatives: software for spatial conservation prioritisation,” in Spatial Conservation Prioritisation: Quantitative Methods and Computational Tools, eds A. Moilanen, K. A. Wilson, and H. P. Possingham (Oxford: Oxford University Press), 184–195.
Barbeaux, S., Aydin, K., Fissel, B., Holsman, K. L., Palsson, W., Shotwell, K., et al. (2018). “Chapter 2: assessment of the Pacific cod stock in the Gulf of Alaska,” in Stock Assessment and Fishery Evaluation Report. North Pacific Fishery Management Council, (NOAA: Silver Spring, MD).
Beaudreau, A. H., and Levin, P. S. (2014). Advancing the use of local ecological knowledge for assessing data-poor species in coastal ecosystems. Ecol. Appl. 24. 2, 244–256. doi: 10.1890/13-0817.1
Bond, N. A., Cronin, M. F., Freeland, H., and Mantua, N. (2015). Causes and impacts of the 2014 warm anomaly in the NE Pacific. Geophys. Res. Lett. 42, 3414–3420. doi: 10.1002/2015gl063306
Brodie, S., Jacox, M. G., Bograd, S. J., Welch, H., Dewar, H., Scales, K. L., et al. (2018). Integrating dynamic subsurface habitat metrics into species distribution models. Front. Mar. Sci. 5:219. doi: 10.3389/fmars.2018.00219
Caddy, J. F., and Seijo, J. C. (2005). This is more difficult than we thought! The responsibility of scientists, managers and stakeholders to mitigate the unsustainability of marine fisheries. Philos. Trans. R. Soc. Lond. B Biol. Sci. 360, 59–75. doi: 10.1098/rstb.2004.1567
Christensen, V., and Walters, C. J. (2004). Ecopath with Ecosim: methods, capabilities and limitations. Ecol. Model. 172, 109–139. doi: 10.1016/j.ecolmodel.2003.09.003
Coastal Protection and Restoration Authority of Louisiana [CPRA] (2014). Mid-Barataria Sediment Diversion Alternative 1, Base Design Report 30% Basis of Design. Available at: http://coastal.la.gov/wp-content/uploads/2016/07/MBSD_Alt_1_Base_Design_Report_30_July_2014.pdf (accessed May 9, 2019).
Coastal Protection and Restoration Authority of Louisiana [CPRA] (2017). Louisiana’s Comprehensive Master Plan for a Sustainable Coast. Available at: http://coastal.la.gov/wp-content/uploads/2017/04/2017-Coastal-Master-Plan_Web-Single-Page_CFinal-with-Effective-Date-06092017.pdf (accessed May 9, 2019).
Collie, J. S., Botsford, L. W., Hastings, A., Kaplan, I. C., Largier, J. L., Livingston, P. A., et al. (2016). Ecosystem models for fisheries management: finding the sweet spot. Fish Fish 17, 101–125. doi: 10.1111/faf.12093
Coral Reef Conservation Act (2000). Coral Reef Conservation Act of 2000. Pub. L 106–562. 114 Stat 2800. doi: 10.1111/faf.12093
Cormier, R., Kelble, C., Anderson, M., Allen, J., Grehan, A., and Gregersen, Ó (2017). Moving from ecosystem-based policy objectives to operational implementation of ecosystem-based management measures. ICES J. Ma. Sci. 74, 406–413. doi: 10.1093/icesjms/fsw181
Couch, C., Most, R., Wiggins, C., Minton, D., Conclin, E., Sziklay, J., et al. (2014). Understanding the Consequences of Land-Based Pollutants on Coral Health in South Kohala. Final Report. NOAA Coral Reef Conservation Program Award # NA11NOS4820006. NOAA: Silver Spring, MD. doi: 10.1093/icesjms/fsw181
Crowder, L. B., Osherenko, G., Young, O. R., Airamé, S., Norse, E. A., Baron, N., et al. (2006). Resolving mismatches in U.S. ocean governance. Science 313, 617–618. doi: 10.1126/science.1129706
Cury, P. M., Boyd, I. L., Bonhommeau, S., Anker-Nilssen, T., Crawford, R. J. M., Furness, R. W., et al. (2011). Global seabird response to forage fish depletion–one-third for the birds. Science 334, 1703–1706. doi: 10.1126/science.1212928
de Mutsert, K., Lewis, K., Milroy, S., Buszowski, J., and Steenbeek, J. (2017). Using ecosystem modeling to evaluate trade-offs in coastal management: effects of large-scale river diversions on fish and fisheries. Ecol. Modell. 360, 14–26. doi: 10.1016/j.ecolmodel.2017.06.029
deReynier, Y. L. (2014). U.S. fishery management councils as ecosystem-based management policy takers and policymakers. Coast. Manag. 42, 512–530. doi: 10.1080/08920753.2014.964678
Deroba, J. J., Gaichas, S. K., Lee, M.-Y., Feeney, R. G., Boelke, D. V., and Irwin, B. J. (2019). The dream and the reality: meeting decision-making time frames while incorporating ecosystem and economic models into management strategy evaluation. Can. J. Fish. Aquat. Sci 76, doi: 10.1139/cjfas-2018-0128
Di Lorenzo, E., and Mantua, N. (2016). Multi-year persistence of the 2014/15 North Pacific marine heatwave. Nat. Clim. Chang 6:1042. doi: 10.1038/nclimate3082
Dunn, D. C., Maxwell, S. M., Boustany, A. M., and Halpin, P. N. (2016). Dynamic ocean management increases the efficiency and efficacy of fisheries management. Proc. Natl. Acad. Sci. U.S.A. 113, 668–673. doi: 10.1073/pnas.1513626113
Ecosystem Principles Advisory Panel [EPAP] (1998). Ecosystem-Based Fishery Management: A Report to Congress. Mumbai: BHL.
Eguchi, T., Benson, S. R., Foley, D. G., and Forney, K. A. (2017). Predicting overlap between drift gillnet fishing and leatherback turtle habitat in the California current ecosystem. Fish. Oceanogr. 26, 17–33. doi: 10.1111/fog.12181
Endangered Species Act, (1973). Endangered Species Act. Pub. L 93–205. 87 Stat 884. doi: 10.1111/fog.12181
Espinoza-Tenorio, A., Wolff, M., Taylor, M. H., and Espejel, I. (2012). What model suits ecosystem-based fisheries management? A plea for a structured modeling process. Rev. Fish. Biol. Fish. 22, 81–94. doi: 10.1007/s11160-011-9224-8
Essington, T. E., Moriarty, P. E., Froehlich, H. E., Hodgson, E. E., Koehn, L. E., Oken, K. L., et al. (2015). Fishing amplifies forage fish population collapses. PNAS 112, 6648–6652. doi: 10.1073/pnas.1422020112
Expert Panel (2014). Expert Panel on Diversion Planning and Implementation. Available at: https://thewaterinstitute.org/reports/diversion-panel-report-3 (accessed May 9, 2019).
Feeney, R. G., Boelke, D. V., Deroba, J. J., Gaichas, S. K., Lee, M.-Y., and Irwin, B. J. (2019). Integrating management strategy evaluation into fisheries management: advancing best practices for stakeholder inclusion based on an MSE for Northeast US Atlantic herring. Can. J. Fish. Aquatic Sci. 76, 1103–1111. doi: 10.1139/cjfas-2018-0125
Fissel, B., Dalton, M., Garber-Yonts, B., Haynie, A., Kasperski, S., Lee, J., et al. (2017). “Economic status of the groundfish fisheries off Alaska, 2016,” in Stock Assessment and Fishery Evaluation Report, (Anchorage, AK: North Pacific Fishery Management Council).
Friedlander, A. M., Aeby, G., Brainard, R. E., Brown, E. K., Chaston, K., Clark, A., et al. (2008). “The state of coral reef ecosystems of the main Hawaiian Islands,” in The State of Coral Reef Ecosystems of the United States and Pacific freely associated States: 2008. NOAA Technical Memorandum NOS NCCOS 73, eds J. E. Waddell, and A. M. Clarke (Silver Spring, MD: NOAA/NCCOS Center for Coastal Monitoring and Assessment’s Biogeography Team), 222–269.
Fulton, E. A., Link, J. S., and Kaplan, I. C. (2011). Lessons in modelling and management of marine ecosystems: the Atlantis experience. Fish Fish. 12, 171–188. doi: 10.1111/j.1467-2979.2011.00412.x
Gaichas, S., Hardison, S., Large, S., and Lucey, S. (eds) (2019). State of the Ecosystem 2019: New England. Woods Hole, MA: NOAA NEFSC.
Gaichas, S., Seagraves, R., Coakley, J., DePiper, G., Guida, V., Hare, J., et al. (2016). A Framework for incorporating species, fleet, habitat, and climate interactions into fishery management. Front. Mar. Sci. 3:105. doi: 10.3389/fmars.2016.00105
Gaichas, S. K., DePiper, G. S., Seagraves, R. J., Muffley, B. W., Sabo, M., Colburn, L. L., et al. (2018). Implementing ecosystem approaches to fishery management: risk assessment in the US Mid-Atlantic. Front. Mar. Sci. 5:442. doi: 10.3389/fmars.2018.00442
Garcia, S. M., Zerbi, A., Aliaume, C., Do Chi, T., and Lasserre, G. (2003). The Ecosystem Approach to Fisheries. issues, terminology, principles, institutional foundations, implementation and outlook. FAO Fisheries Technical Paper. No. 443. Rome: FAO.
Golet, W., Record, N., Lehuta, S., Lutcavage, M., Galuardi, B., Cooper, A., et al. (2015). The paradox of the pelagics: why bluefin tuna can go hungry in a sea of plenty. Mar. Ecol. Prog. Ser. 527, 181–192. doi: 10.3354/meps11260
Harvey, C., Garfield, T., Williams, G., and Tolimieri, N. (eds) (2019). California Current Integrated Ecosystem Assessment (CCIEA) California Current Ecosystem Status Report 2019. Portland, OR: Pacific Fishery Management Council.
Harvey, C., Reum, J. C. P., Poe, M. R., Williams, G. D., and Kim, S. J. (2016). Using conceptual models and qualitative network models to advance integrative assessments of marine ecosystems. Coast. Manag. 44, 486–503. doi: 10.1080/08920753.2016.1208881
Hazen, E. L., Scales, K. L., Maxwell, S. M., Briscoe, D. K., Welch, H., Bograd, S. J., et al. (2018). A dynamic ocean management tool to reduce bycatch and support sustainable fisheries. Sci. Adv. 4:eaar3001. doi: 10.1126/sciadv.aar3001
Hilborn, R., Amoroso, R. O., Bogazzi, E., Jensen, O. P., Parma, A. M., Szuwalski, C., et al. (2017). When does fishing forage species affect their predators? Fish. Res. 191, 211–221. doi: 10.1016/j.fishres.2017.01.008
Himes-Cornell, A., Hoelting, K., Maguire, C., Munger-Little, L., Lee, J., Felthoven, C., et al. (2013). Community profiles for North Pacific fisheries - Alaska. Silver Spring, MD: NOAA.
Hobday, A. J., Maxwell, S. M., Forgie, J., McDonald, J., Darby, M., Seto, K., et al. (2014). Dynamic ocean management: integrating scientific and technological capacity with law, policy, and management. Stanford Environ. Law J. 33, 125–165.
Larkin, P. A. (1996). Concepts and issues in marine ecosystem management. Rev. Fish Biol. Fish. 6, 139–164.
Latour, R. J., Brush, M. J., and Bonzek, C. F. (2003). Toward ecosystem-based fisheries management: strategies for multispecies modeling and associated data requirements. Fisheries 28, 10–22. doi: 10.1577/1548-8446(2003)28%5B10:tefm%5D2.0.co;2
Levin, P. S., Fogarty, M. J., Matlock, G. C., and Ernst, M. (2008). Integrated ecosystem assessments. NOAA Tech Memo NMFS-NWFSC-92. Available at: https://swfsc.noaa.gov/publications/FED/01272.pdf (accessed May 9, 2019).
Levin, P. S., Fogarty, M. J., Murawski, S. A., and Fluharty, D. (2009). Integrated ecosystem assessments: developing the scientific basis for ecosystem-based management of the ocean. PLoS Biol. 7:e1000014. doi: 10.1371/journal.pbio.1000014
Link, J. (2018). System-level optimal yield: increased value, less risk, improved stability, and better fisheries. Can. J. Fish. Aquat. Sci. 75, 1–16. doi: 10.1139/cjfas-2017-0250
Link, J., Ihde, T., Townsend, H., Osgood, K., Schirripa, M., Kobayashi, D., et al. (2010). “U.S. department of commerce, national oceanic and atmospheric administration, national marine fisheries service,” in Report of the 2nd National Ecosystem Modeling Workshop (NEMoW II), Bridging the Credibility Gap - Dealing with Uncertainty in Ecosystem Models, (Silver Spring, MD: NOAA Fisher).
Link, J., Overholtz, W., O’Reilly, J., Green, J., Dow, D., Palka, D., et al. (2008). The Northeast U.S. continental shelf energy modeling and analysis exercise (emax): ecological network model development and basic ecosystem metrics. J. Mar. Syst. 74, 453–474. doi: 10.1016/j.jmarsys.2008.03.007
Link, J. S., Ihde, T., Harvey, C., Gaichas, S., Field, J., Brodziak, J., et al. (2012). Dealing with uncertainty in ecosystem models: the paradox of use for living marine resource management. Prog. Oceanogr. 102, 102–114. doi: 10.1016/j.pocean.2012.03.008
Logan, J. M., Golet, W. J., and Lutcavage, M. E. (2015). Diet and condition of Atlantic bluefin tuna (Thunnus thynnus) in the gulf of maine, 2004–2008. Environ. Biol. Fish. 98, 1411–1430. doi: 10.1007/s10641-014-0368-y
MacPherson, M. (2001). Integrating ecosystem management approaches into federal fishery management through the magnuson-stevens fishery conservation and management act. Ocean Coast. Law J. 6, 1–32.
Magnuson-Stevens Fishery Conservation and Management Act [MSA] (2010). Magnuson-Stevens Fishery Conservation and Management Act. Silver Spring, MD: NOAA Fisher.
Marshak, A. R., and Brown, S. K. (2017). Habitat science is an essential element of ecosystem-based fisheries management. Fisheries 42:300. doi: 10.1016/B978-0-12-800287-2.00002-0
Marshall, K. N., Koehn, L. E., Levin, P. S., Essington, T. E., and Jensen, O. P. (2018). Inclusion of ecosystem information in US fish stock assessments suggests progress toward ecosystem-based fisheries management. ICES J. Mar. Sci. 76, 1–9. doi: 10.1093/icesjms/fsy152
Mason, J. G., Hazen, E. L., Bograd, S. J., Dewar, H., and Crowder, L. B. (2019). Community-level effects of spatial management in the California drift gillnet fishery. Fish. Res. 214, 175–182. doi: 10.1016/j.fishres.2019.02.010
Maxwell, S. M., Hazen, E. L., Lewison, R. L., Dunn, D. C., Bailey, H., Bograd, S. J., et al. (2015). Dynamic ocean management: defining and conceptualizing real-time management of the ocean. Mar. Policy 58, 42–50. doi: 10.1016/j.marpol.2015.03.014
Melbourne-Thomas, J., Johnson, C. R., Fung, T., Seymour, R. M., Chérubin, L. M., Arias-González, J. E., et al. (2011). Regional-scale scenario modeling for coral reefs: a decision support tool to inform management of a complex system. Ecol. Appl. 21, 1380–1398. doi: 10.1890/09-1564.1
Meselhe, E., Baustian, M. M., Khadka, A., Jung, H., Allison, M., Duke-Sylvester, S. M., et al. (2015). “Basin wide model development for the Louisiana coastal area mississippi river hydrodynamic and delta management study,” in Prepared for and Funded by the Coastal Protection and Restoration Authority under Task Order 27.1, (Baton Rouge, LA: The Water Institute of the Gulf).
Meyer-Gutbrod, E. L., and Greene, C. H. (2018). Uncertain recovery of the North Atlantic right whale in a changing ocean. Glob. Chang. Biol. 24, 455–464. doi: 10.1111/gcb.13929
Miller, T. J., Blair, J. A., Ihde, T. F., Jones, R. M., Secor, D. H., and Wilberg, M. J. (2010). FishSmart: an innovative role for science in stakeholder centered approaches to fisheries management. Fisheries 35, 424–433. doi: 10.1577/1548-8446-35.9.422
New England Fishery Management Council [NEFMC] (2018). NEFMC-Approves-Atlantic-Herring-Amendment-8_181001_165323.pdf. Available at: https://s3.amazonaws.com/nefmc.org/NEFMC-Approves-Atlantic-Herring-Amendment-8_181001_165323.pdf (accessed November 6, 2018).
NMFS, (2016). Fisheries of the United States, 2015. Available at: https://www.st.nmfs.noaa.gov/Assets/commercial/fus/fus15/documents/FUS2015.pdf (accessed November 6, 2018).
NOAA, (2016). Ecosystem Based Fisheries Management Policy of the National Marine Fisheries Service, Policy 01-120. Silver Spring, MD: NOAA.
Overholtz, W. J., and Link, J. S. (2007). Consumption impacts by marine mammals, fish, and seabirds on the Gulf of Maine–Georges Bank Atlantic herring (Clupea harengus) complex during the years 1977–2002. ICES J. Mar. Sci. 64, 83–96.
Overholtz, W. J., Link, J. S., and Suslowicz, L. E. (2000). Consumption of important pelagic fish and squid by predatory fish in the Northeastern USA shelf ecosystem with some fishery comparisons. ICES J. Mar. Sci. 57, 1147–1159. doi: 10.1006/jmsc.2000.0802
Peterman, R. M. (2004). Possible solutions to some challenges facing fisheries scientists and managers. ICES J. Mar. Sci. 61, 1331–1343. doi: 10.1016/j.icesjms.2004.08.017
Pew Charitable Trust Issue Brief (2015). Fishery Ecosystem Plans Big-Picture Thinking for Healthy Ecosystems and Coastal Communities. Available at: https://www.pewtrusts.org/en/research-and-analysis/issue-briefs/2015/03/fishery-ecosystem-plans (accessed May 9, 2019).
PIFSC (2016). West Hawai’i Integrated Ecosystem Assessment: Ecosystem Trends and Status Report. Wasp Blvd, HI: NOAA.
Pikitch, E. K., Rountos, K. J., Essington, T. E., Santora, C., Pauly, D., Watson, R., et al. (2012). The global contribution of forage fish to marine fisheries and ecosystems. Fish Fish. 15, 43–64. doi: 10.1111/faf.12004
Pikitch, E. K., Santora, C., Babcock, E. A., Bakun, A., Bonfil, R., Conover, D. O., et al. (2004). Ecosystem-based fishery management. Science 35, 346–347. doi: 10.1126/science.1098222
Plagányi, ÉE. (2007). Models for an Ecosystem Approach to Fisheries. FAO Fisheries Technical Paper. No. 477. Rome: FAO.
Plagányi, ÉE., and Butterworth, D. S. (2012). The Scotia Sea krill fishery and its possible impacts on dependent predators: modeling localized depletion of prey. Ecol. Appl. 22, 748–761. doi: 10.1890/11-0441.1
Plagányi, ÉE., Punt, A. E., Hillary, R., Morello, E. B., Thébaud, O., Hutton, T., et al. (2014). Multispecies fisheries management and conservation: tactical applications using models of intermediate complexity. Fish Fish 15, 1–22. doi: 10.1111/j.1467-2979.2012.00488.x
Plagányi, ÉE., Rademeyer, R. A., Butterworth, D. S., Cunningham, C. L., and Johnston, S. J. (2007). Making management procedures operational—innovations implemented in South Africa. ICES J. Mar. Sci. 64, 626–632. doi: 10.1093/icesjms/fsm043
Polovina, J. J. (1984). Model of a coral reef ecosystem. Coral Reefs 3, 1–11. doi: 10.1007/bf00306135
Prins, H. H. T., and Henne, G. (1998). CBD-Workshop on the Ecosystem Approach, Lilongwe, Malawi, 26-28 January 1998. Wageningen: Wageningen Agricultural University.
Punt, A. E., Butterworth, D. S., de Moor, C. L., De Oliveira, J. A. A., and Haddon, M. (2016a). Management strategy evaluation: best practices. Fish Fish. 17, 303–334. doi: 10.1111/faf.12104
Punt, A. E., MacCall, A. D., Essington, T. E., Francis, T. B., Hurtado-Ferro, F., Johnson, K. F., et al. (2016b). Exploring the implications of the harvest control rule for Pacific sardine, accounting for predator dynamics: a MICE model. Ecol. Mod. 337, 79–95. doi: 10.1016/j.ecolmodel.2016.06.004
Rademeyer, R. A., Plagányi, ÉE., and Butterworth, D. S. (2007). Tips and tricks in designing management procedures. ICES J. Mar. Sci. 64, 618–625. doi: 10.1093/icesjms/fsm050
Rose, K. A., Allen, J. I., Artioli, Y., Barange, M., Blackford, J., Carlotti, F., et al. (2010). End-to-end models for the analysis of marine ecosystems: challenges, issues, and next steps. Mar. Coast. Fish.: Dyn. Manag. Ecosyst. Sci. 2, 115–130. doi: 10.1577/c09-059.1
Ryan, R. W., Holland, D. S., and Herrera, G. E. (2010). Bioeconomic equilibrium in a bait-constrained fishery. Mar. Resour. Econ. 25, 281–293. doi: 10.5950/0738-1360-25.3.281
Samhouri, J. F., Ramanujam, E., Bizzarro, J. J., Carter, H., Sayce, K., and Shen, S. (2019). An ecosystem-based risk assessment for California fisheries co-developed by scientists, managers, and stakeholders. Biol. Conserv. 231, 103–121. doi: 10.1016/j.biocon.2018.12.027
Townsend, H., Aydin, K., Holsman, K., Harvey, C., Kaplan, I., Hazen, E., et al. (2017). “Ecosystem models to evaluate inevitable trade-offs,” in Report of the 4th National Ecosystem Modeling Workshop (NEMoW 4), (Silver Spring, MD: NOAA).
Townsend, H. M., Harvey, C., Aydin, K. Y., Gamble, R., Grüss, A., Levin, P. S., et al. (2014). “Mingling models for marine resource management – multiple model inference,” in Report of the 3rd National Ecosystem Modeling Workshop (NEMoW 3), (Silver Spring, MD: NOAA).
Townsend, H. M., Link, J. S., Osgood, K. E., Gedamke, T., Watters, G. M., Polovina, J. J., et al. (2008). “National marine fisheries service,” in Report of the National Ecosystem Modeling Workshop (NEMoW), (Silver Spring, MD: NOAA).
U.S. Army Corps of Engineers [USACE] (2012). Louisiana Coastal Area Mississippi River Delta Management Study St. Charles, Jefferson, Lafourche, Plaquemines Parish, Louisiana Feasibility Report. Washington, D.C: USACE.
UN Fisheries, and Agriculture Organization [UNFAO] (2003). Fisheries mAnagement. The Ecosystem Approach to Fisheries, FAO Technical Guidelines for Responsible Fisheries. Rome: UNFAO.
Un Fisheries, and Agriculture Organization [UNFAO] (2008). Fisheries Management. 2. The Ecosystem Approach to Fisheries. 2.1 Best Practices in Ecosystem Modelling for Informing an Ecosystem Approach to Fisheries. FAO Fisheries Technical Guidelines for Responsible Fisheries. Rome.: UNFAO.
Un Fisheries, and Agriculture Organization [UNFAO] (2009). Fisheries Management. 2. The Ecosystem Approach to Fisheries. 2.2 Human Dimensions of the Ecosystem Approach to Fisheries FAO Technical Guidelines for Responsible Fisheries. Rome: UNFAO.
van Hooidonk, R. J., van Maynard, J., Tamelander, J., Gove, J. M., Ahmadia, G., Raymundo, L., et al. (2016). Local-scale projections of coral reef futures and implications of the paris agreement. Sci. Rep. 6:396666. doi: 10.1038/srep39666
Weijerman, M., Gove, J. M., Williams, I. D., Walsh, W. J., Minton, D., and Polovina, J. J. (2018a). Evaluating management strategies to optimise coral reef ecosystem services. J. Appl. Ecol. 55, 1823–1833. doi: 10.1111/1365-2664.13105
Weijerman, M., Veazey, L., Yee, S., Vaché, K., Delevaux, J. M. S., Donovan, M. K., et al. (2018b). Managing local stressors for coral reef condition and ecosystem services delivery under climate scenarios. Front. Mar. Sci. 5:425. doi: 10.3389/fmars.2018.00425
Welch, H., Brodie, S., Jacox, M. G., Bograd, S. J., and Hazen, E. L. (2019a). Comparing decision support tools for multi-species dynamic management. Conserv. Biol. doi: 10.1111/cobi.13417
Welch, H., Hazen, E. L., Bograd, S. J., Jacox, M. G., Brodie, S., Robinson, D., et al. (2019b). Practical considerations for operationalizing dynamic management tools. J. Appl. Ecol. 56, 459–469. doi: 10.1111/1365-2664.13281
Zador, S., and Yasumiishi, E. (2017). “Ecosystem considerations 2017: status of the Gulf of Alaska marine ecosystem,” in Stock Assessment and Fishery Evaluation Report, North Pacific Fishery Management Council, 605 W 4th Ave, Suite 306, (Anchorage, AK).
Zador, S. G., Gaichas, S. K., Kasperski, S., Ward, C. L., Blake, R. E., Ban, N. C., et al. (2017a). Linking ecosystem processes to communities of practice through commercially fished species in the Gulf of Alaska. ICES J. Mar. Sci. 74, 2024–2033. doi: 10.1093/icesjms/fsx054
Keywords: ecosystem-based fisheries management, ecosystem modeling, fisheries science, fisheries management, natural resource management
Citation: Townsend H, Harvey CJ, deReynier Y, Davis D, Zador SG, Gaichas S, Weijerman M, Hazen EL and Kaplan IC (2019) Progress on Implementing Ecosystem-Based Fisheries Management in the United States Through the Use of Ecosystem Models and Analysis. Front. Mar. Sci. 6:641. doi: 10.3389/fmars.2019.00641
Received: 15 May 2019; Accepted: 30 September 2019;
Published: 29 October 2019.
Edited by:
Fiorenza Micheli, Stanford University, United StatesReviewed by:
Marjo Kristiina Vierros, United Nations University, JapanAndrew M. Fischer, University of Tasmania, Australia
Copyright © 2019 Townsend, Harvey, deReynier, Davis, Zador, Gaichas, Weijerman, Hazen and Kaplan. This is an open-access article distributed under the terms of the Creative Commons Attribution License (CC BY). The use, distribution or reproduction in other forums is permitted, provided the original author(s) and the copyright owner(s) are credited and that the original publication in this journal is cited, in accordance with accepted academic practice. No use, distribution or reproduction is permitted which does not comply with these terms.
*Correspondence: Howard Townsend, Howard.Townsend@noaa.gov