- 1Facultad de Ciencias del Mary Recursos Biológicos, Universidad de Antofagasta, Antofagasta, Chile
- 2Grupo de Investigación en Sistemas Marinos y Costeros, Corporación Académica Ambiental, Sede Ciencias del Mar, Universidad de Antioquia, Turbo, Colombia
- 3Corporation Center of Excellence in Marine Sciences (CEMarin), Bogotá, Colombia
Despite their importance as a larval source for the aquaculture industry in several countries, natural stocks of pectinids have been heavily impacted by a variety of natural and anthropogenic stressors. Here we studied the key transition from larvae to early recruitment using the largest natural population of the scallop Argopecten purpuratus (Lamarck, 1819) in Chile. We assessed whether thermal anomalies associated to El Niño-La Niña (EN-LN) cycle had a signal in the temperature regime at La Rinconada Marine Reserve and the consequences of this variability for the settlement of competent larvae of A. purpuratus to artificial and natural substrates in this area. For this, we gathered historical data (February 1950–December 2017) regarding satellite-derived thermal anomalies in the El Niño 1 + 2 region and in situ measurements of sea surface and bottom temperature in the study area. Moreover, we sampled the larval abundance of A. purpuratus in the water column and the settled spat on artificial collectors and on red algae Rhodymenia spp. – their natural settlement surface – between April 1996 and January 2000. Thermal anomalies associated to EN-LN cycle were strongly related to interannual changes in winter and summer temperature in Antofagasta Bay, with a clearer signal in winter than summer temperature. The increased reproductive activity during September–April seemingly foster the observed pulses in the larval abundance of A. purpuratus, as expected in a semi-enclosed system. In contrast to previous studies, the larval abundance did not show clear responses to the local EN-LN-related shifts in temperature regimes. Larval settlement of A. purpuratus in artificial collectors increased through the study period while that on Rhodymenia spp. showed a contrasting, decreasing trend. At the same time, the abundance of Rhodymenia spp. dropped continuously. All these temporal trends and our statistical analysis implied that, whether EN-LN-driven thermal shifts had an effect on the larval settlement of A. purpuratus, it is masked by the strong and continuous reduction in the availability of Rhodymenia spp. as a settlement surface. This reduction may have consequences for the stability of natural stocks of A. purpuratus in Chile.
Introduction
Pectinids stand among the most important fishery resources in several countries, but dramatic reductions of natural stocks have led to tight regulations aiming to preserve source populations that ensure sustainable fisheries and larval supply for the aquaculture industry (Dickie, 1995; Paulet et al., 1997). The scallop Argopecten purpuratus (Lamarck, 1819), distributed between northern Peru and central Chile, has long been exploited by small-scale fishermen, leading to the reduction or collapse of several local populations. The high demand of this scallop has prompted the development of aquaculture operations, which for the most part depend on scallop larvae from natural populations. For this, mesh bags are suspended in the water column to collect larvae that are then transported to culture areas and grown to marketable size. However, scallops populations are also affected by El Niño-Southern Oscillation (ENSO), a quasi-periodic variation in winds and sea surface temperatures (SSTs) over the tropical eastern Pacific Ocean that modify climate patterns in the tropics and subtropics. This interannual environmental variability overlaps with human exploitation in the Humboldt Current System, either bringing local collapses or population blooms in coastal species, depending on their biogeographic affinities (Riascos et al., 2009; Carstensen et al., 2010). In the early 1980s, annual landings in northern Chile (23°S–29°S) hardly reached 500 MT, but these figures drastically changed to ∼5,000 MT in 1984 (Avendaño and Cantillánez, 1996). A warm El Niño episode in 1982–1983 caused population blooms in several populations in Peru and Chile, turning small-scale fisheries into ephemeral multimillion-dollar business (Wolff et al., 2007). Landings in this region rapidly returned to low levels (1,410 MT in 1985 and 492 MT in 1986), which promoted the permanent closure of this fishery and regulatory efforts to protect natural stocks (Avendaño and Cantillánez, 1996; Stotz, 2000).
La Rinconada, located in the innermost area of Antofagasta Bay in northern Chile supports the largest natural population of A. purpuratus in the country. To secure the protection of this important species, the Chilean government declared in 1997 this area as its first marine reserve (Avendaño and Cantillánez, 2016). The reserve aimed to protect natural stocks of A. purpuratus and to maintain the larval supply for neighbor populations and aquaculture facilities that started operations soon after the establishment of the reserve. Despite these efforts, recent evaluations indicate a sustained decline in the abundance, mean size and spawning biomass, most likely reflecting illegal exploitation (Avendaño and Cantillánez, 2016). This decline and the concomitant increase of scallop aquaculture in Peru have seriously affected this business in Chile.
The monitoring program established as part of the implementation of the marine reserve revealed that A. purpuratus consistently display intense spawning activity between September and April, with increases in larval abundance associated to this period of gamete release (Cantillánez et al., 2005). Despite this, there is considerable stochasticity in A. purpuratus recruitment, hinting on the diverse and interacting nature of factors affecting the transition between D-veliger larvae (∼110 μm) and settled larvae (∼244 μm). Wolff (1988) suggested that a combination of high temperature, food availability and oxygen saturation are key conditions for fast larval development to successful metamorphosis, settlement and pre-recruit growth. This in turn would mean less exposure time to filter feeders and currents, which would cause larval drift from the parent bed areas.
In Chile, studies on the ecology of early life stages of A. purpuratus are lacking, but larval settlement in artificial collectors differ between El Niño and La Niña episode (Cantillánez et al., 2007). As, e.g., larval growth, duration and mortality are temperature dependent-factors for pectinids species (Davies et al., 2015; Nicolle et al., 2016) and temperature regimes in La Rinconada may respond to El Niño and La Niña episodes, it is key to assess how temperature may affect the transition between larval release and subsequent settlement in natural surfaces. Settlement of scallop larvae is not random process; an array of species-specific organic (filamentous red algae and hydroids) or inorganic surfaces (gravel, pebbles, mollusk shells) seem to facilitate settlement and provide refuge for settlers. In La Rinconada, the red algae Rhodymenia spp. seems to play an equivalent role for the settlement of A. purpuratus (Avendaño et al., 2008); therefore, changes in the abundance of these red algae were included as part of the monitoring program.
Under normal conditions, the population dynamics of most coastal species in the Humboldt Current System is controlled by mesoscale oceanographic processes, including upwelling events, frontal systems and eddies that influences variation in recruit supply and upwelling shadows – a high temperature region within coastal upwelling – in bay systems that influences larval retention (Thiel et al., 2007). However, these oceanographic processes could be highly modified during the warm El Niño and cold La Niña phases of ENSO. Hence, a critical question is whether ENSO – a large oceanographic process in the tropical eastern Pacific – affects local climatic regimes and how these local changes influences early life stages of coastal species.
In this context, our study aims to (i) evaluate the potential relationship between the El Niño-La Niña cycle and the thermal variability in La Rinconada Marine Reserve and (ii) assess the consequences of this variability for the settlement of competent larvae of A. purpuratus to natural (Rhodymenia spp.) and artificial substrates (moored collectors) and for the stability of natural stocks.
Materials and Methods
Study Area and in situ Thermal Variability
The study was performed at La Rinconada Marine Reserve (23° 28′28 ″S, 70° 30′35″W; hereafter La Rinconada), located 20 km north of Antofagasta, Chile (Figure 1) with samplings between April 1996 and January 2000, with breaks in November 1996, June–August and December 1997, July and September–December 1998, January and September 1999 due to budget cuts.
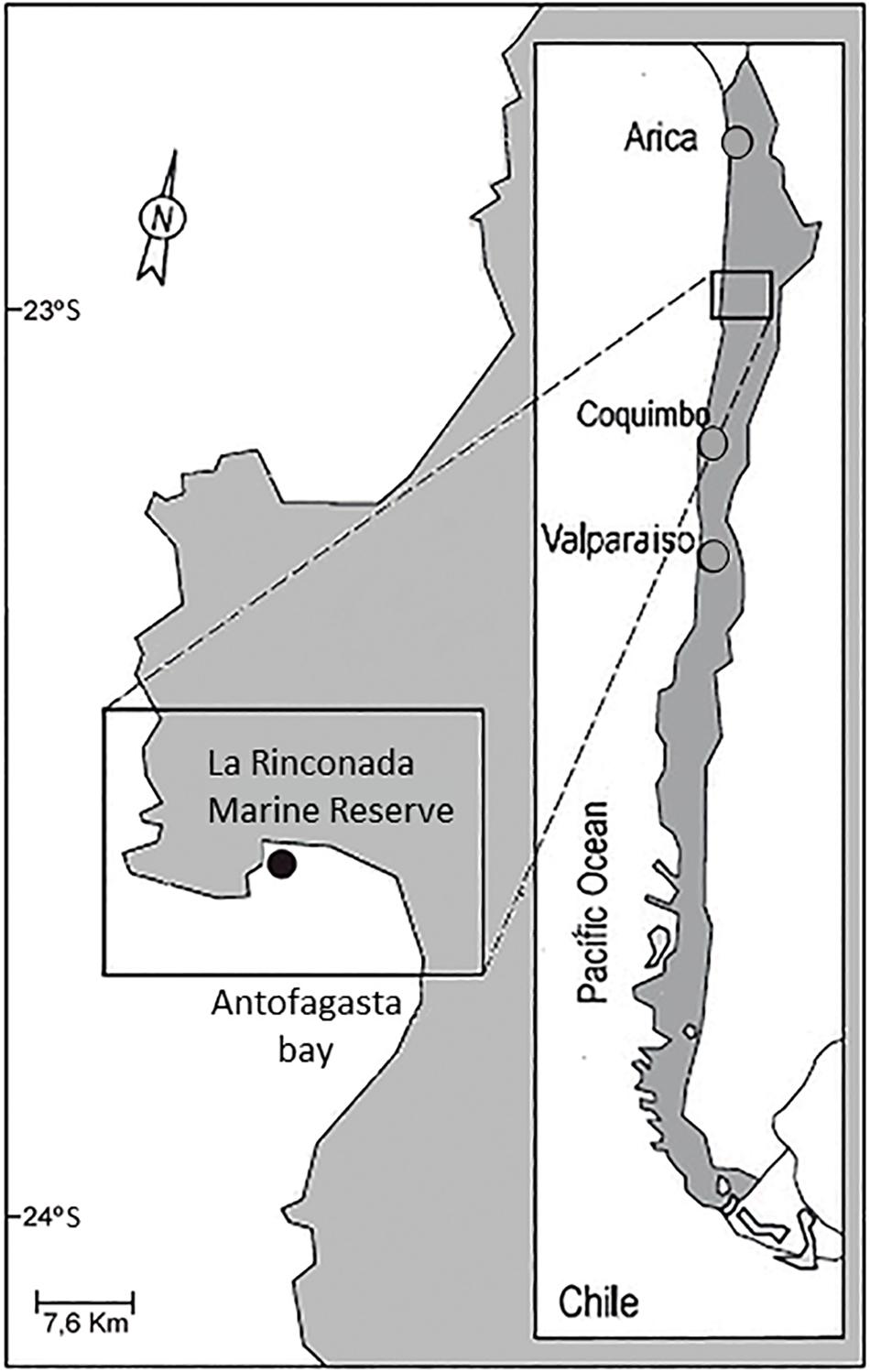
Figure 1. Map of the Antofagasta Bay (northern Chile) and the sampling station (black dot) in La Rinconada Marine Reserve.
La Rinconada represents a retention area for particulate material and larvae, owing to the unique oceanographic characteristics of the Antofagasta Bay: it is located between two active upwelling centers in the mouth, which creates an “upwelling-shadow” with an almost constant sea water temperature frontal oceanographic structure crossing the mouth. As a result, upwelled waters are retained inside the bay long enough for temperatures to be elevated by several degrees through surface heating (Castilla et al., 2002; Ashton et al., 2008; Avendaño and Cantillánez, 2008). The dynamics of shallow water mass in the bay indicates a central retention area, dominated by a gyre with reduced speed by inertial effect (Figure 1), Escribano and Hidalgo (2001). This small area (∼46 ha; 15–17 m water depth) has long been known and monitored for settlement of A. purpuratus on the seaweed Rhodymenia (Avendaño and Cantillánez, 2016) and was thus chosen as the sampling area for this study.
In October 1997 a Minilog 8-BIT (Vemco, Model TR) was installed at 16 m water depth to register bottom temperature every 6 h in the sampling area until the end of the study (January 2000).
Assessing Relationships Between ENSO and Local Thermal Variability
Historical data (February 1950–December 2017) of the el Niño-coastal index (Takahashi et al., 2014) were taken as a relevant descriptor of regional interannual anomalies of SST related to ENSO. The index represent a 3-month moving average of monthly anomalies of SST for the period 1981–2010 at the El Niño 1 + 2 Region, close to the western coast of South America, taken from the ERSST v3b dataset provided by the NOAA (United States). El Niño-La Niña episodes are classified according to the values of the index as: warm (extraordinary, strong, moderate, weak) and cold (strong, moderate, mild), respectively (see Takahashi et al., 2014 for details). In turn, to describe the local thermal variability, we used historical data (the same time range) on the monthly mean SST (°C) from a fixed station off Antofagasta (23°39′S; 70°25′W) provided by the Servicio Hidrográfico y Oceanográfico de la Armada de Chile. Monthly mean SST in this dataset was calculated from daily measurements taken from a fixed station (23°39′S; 70°25′W) by hand (1950–1998) and using automatic temperature sensors (1998 to date). As SST in Antofagasta Bay display a clear seasonal signal, with higher temperatures during January–March (Austral summer) and lower temperatures (Austral winter) during June–August, we tested how the ENSO cycle affect summer SST and winter SST in Antofagasta Bay. For this, a simple regression model (least square fit) was used to fit the relationship between El Niño-Coastal Index (ENCI) and summer (January–March) and winter (June–August) SST in the study area. The significance of the relationship (the regression slope) was tested by an analysis of variance. The relationship between SST (obtained by SHOA) versus bottom (in situ) temperature in La Rinconada was assessed using a non-parametric regression called LOESS (locally estimated scatterplot smoothing; Trexler and Travis, 1993), because conditions for traditional regression analyses were not satisfied. We used the standard package R 3.5.0 (R Core Team, 2018) to fit the LOESS regression.
Larval Sampling
Plankton samples were collected monthly from May through October (the period of low larval abundance) and every 10–15 days from November to April (the period of high larval abundance) from the central retention area described above and shown in Figure 1. Each sample was collected on the whole 15–17 m-depth water column, using a 53-μm-mesh HYDRO-BIOS plankton net (No. 438 005; mouth diameter = 25 cm) to perform the vertical plankton hauls. Each sample was preserved in 70% ethylic alcohol and transported to the laboratory, homogenized and split in 10 parts using a Folsom’s plankton sample divider. Two aliquots were used to count larvae under a dissecting microscope and ocular micrometer and the average was extrapolated to the whole sample. Larval abundance (ind m–3) was estimated from the radius (m) of the net aperture, the haul length and from the total volume of filtered seawater.
Spat Collection in Artificial Collectors
To evaluate changes in the settlement of the competent scallop larvae, three replicated artificial 3D Japanese collectors were installed 1–3 m off the bottom in the central retention area described above. The collector unit derives from a Japanese device made up of a plastic mesh bag (mesh size: 0.5 × 1 mm) filled with Netlon netting (10 mm mesh size). Three replicated collectors were deployed with 10 m distance between them and fastened at 1–3 m off the bottom, on ropes suspended from immersed buoys and anchored by a 125-kg concrete weight. Previous experimental work demonstrated that the efficiency of spat collection increased in this position off the bottom (Avendaño and Cantillánez, 1992). Collectors were monthly removed, placed into labeled plastic bags, and immediately replaced by SCUBA divers. In the laboratory, all post larvae and juvenile scallops were washed from individual collectors and the content was screened on a 180-μm sieve to retain any recently settled post-larvae. The collected spat was then mixed and split in 10 parts using a Folsom’s plankton sample divider. Two aliquots were used to count spat under a dissecting microscope and ocular micrometer. The number of post larvae per collector was obtained by multiplying the average obtained in the two subsamples by 10.
Spat Collection of A. purpurtus on Rhodymenia spp.
To assess the importance of natural settlement substrates, the abundance of Rhodymenia spp. and the settlement of A. purpuratus were also monitored in the central retention area described above. For this, the monthly mean algal mass (g m–2) was obtained by collecting algae using three replicate quadrats (0.25 m–2). Each quadrate was haphazardly placed at least 10 m apart from each other. Samples were stored in 180 μm mesh bags and transported to the laboratory. Thereafter samples were carefully washed in a 180 μm sieve to recover all the spat. Thereafter algae were weighted using an analytical scale (0.1 g precision), and the number of post larvae (spat m–2) in each sample was determined following the same procedure described above.
Factors Influencing Larval Settlement
We used linear and non-linear models using least square fitting to assess the influence of the available larval pool (larvae m–3), the SST (°C) and the ENCI on the larval settlement of A. purpuratus in either moored artificial collectors (spat per collector) or in mats of Rhodymenia spp. (spat m–2). Additionally, the relationship between larval settlement density on Rhodymenia spp. (spat m–2) and the algal mass was assessed in order to evaluate if changes in settlement density were dependent on the abundance of the algae. These analyses were performed using Statistica V10 (Statsoft, Inc., 1984–2011).
Results
The SST in Antofagasta Bay shows a consistent seasonal pattern (Figure 2), with high summer temperatures averaging 20.4°C in January and 19.3°C in March and low winter temperatures averaging 15.6°C in June and 15.2°C in August. The ENCI was positively correlated with summer and winter SST in the bay, with a much better fit for winter temperature (Figure 3). The higher values for summer and winter SST registered in the bay since 1950 coincided with extraordinary and strong El Niño episodes. In turn, lower summer SST have been registered under neutral conditions and lower winter SST were observed during weak La Niña episodes. Clearly, a strong interannual variability not explained by ENCI is observed in summer SST, as opposed to winter SST. Figure 4 shows that the normal seasonal variation in SST (∼14–20°C, see Figure 2) had little influence on the rather stable bottom temperature. However, anomalous high SST (20–22°C) observed during El Nino 1997-1008 had strong effects on the bottom temperature.
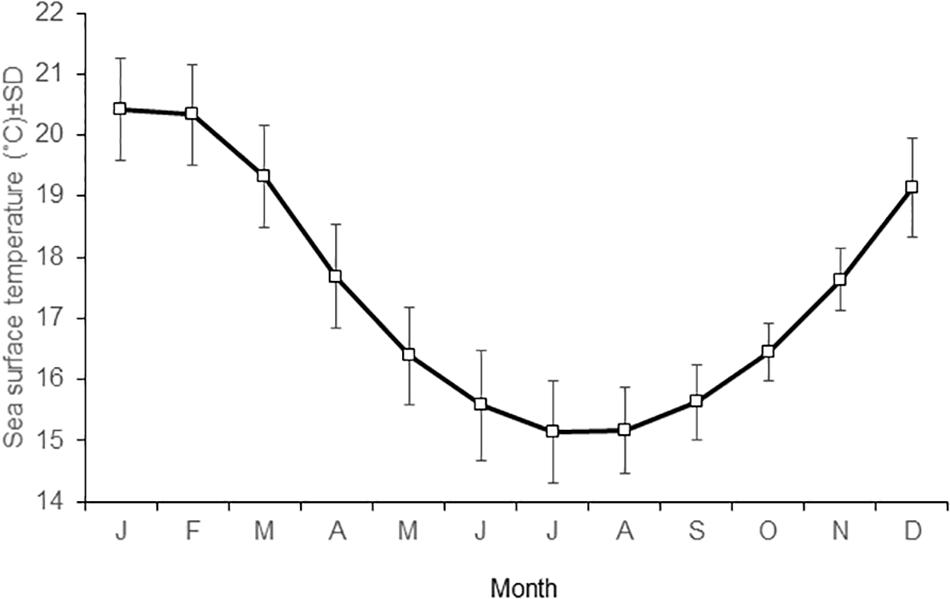
Figure 2. Seasonal variability in mean sea surface temperature in Antofagasta Bay. Monthly values represent the mean value (and standard deviation) for the period 1946–2017.
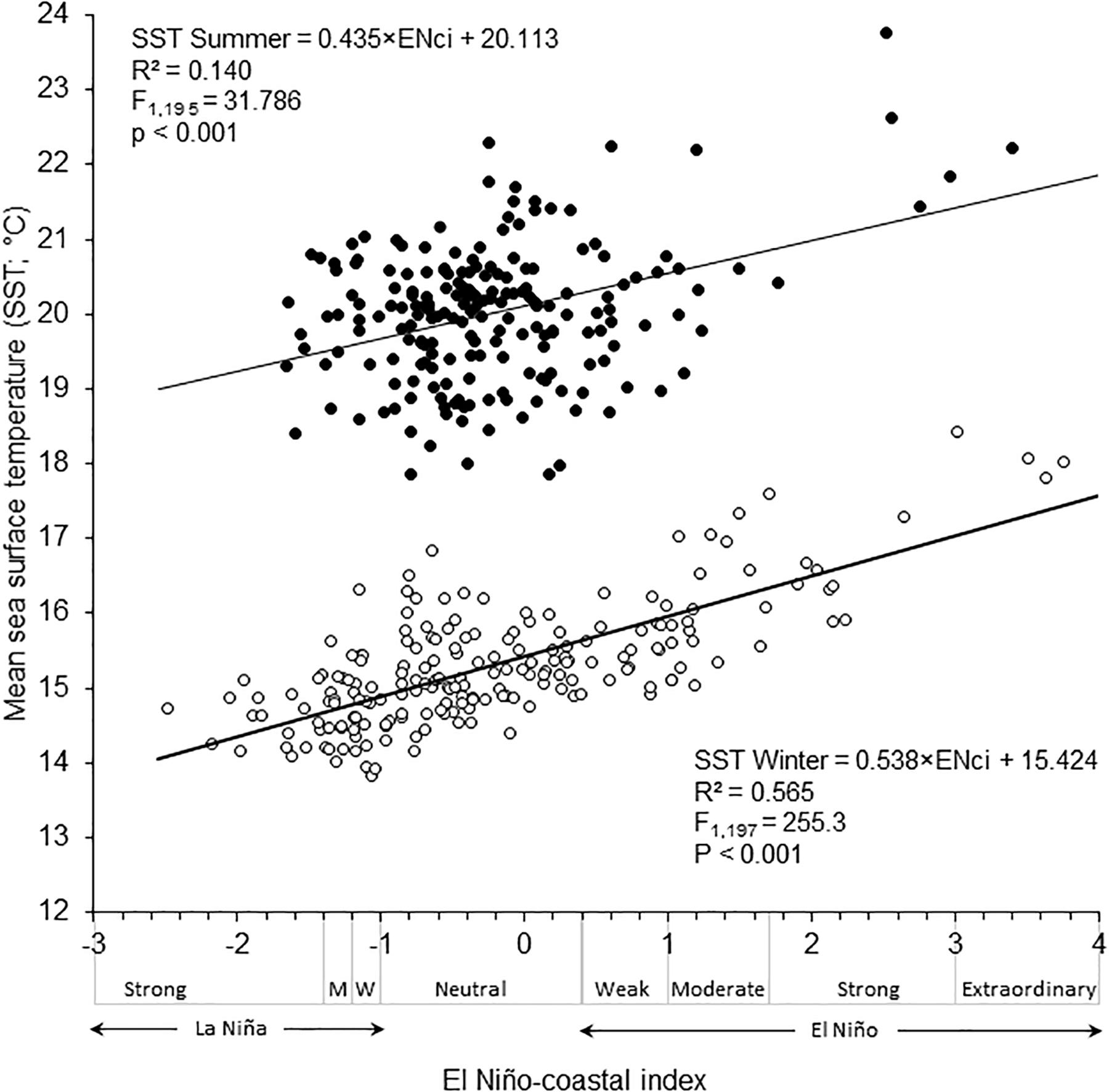
Figure 3. The relationship between El Niño-La Niña episodes, as described by El Niño-Coastal Index, and summer and winter SST in Antofagasta Bay between February 1950 and December 2017. Classification of el Niño-La Niña episodes according to Takahashi et al. (2014).
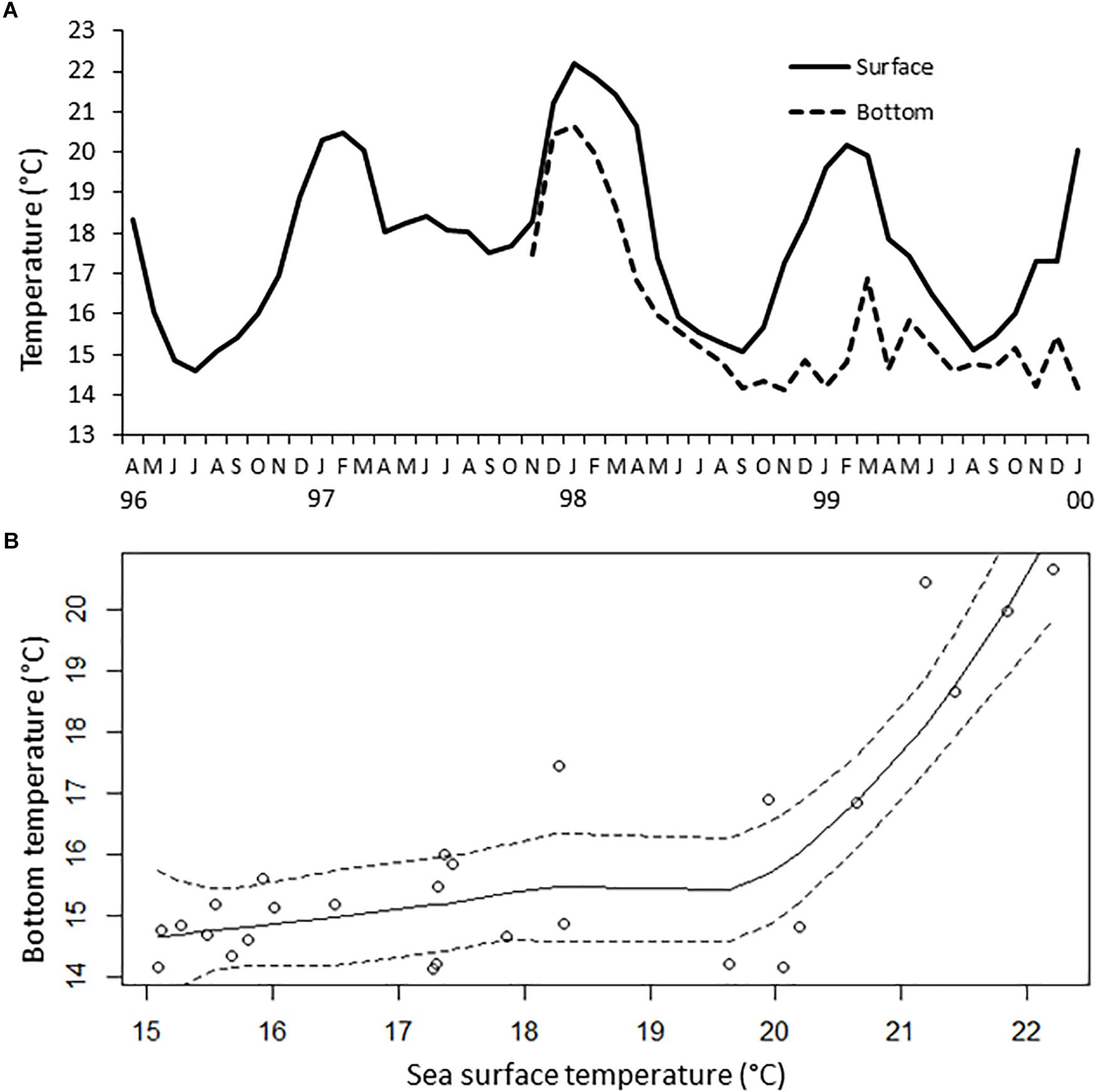
Figure 4. Thermal variability of sea surface and bottom temperature in Antofagasta Bay. (A) Time series of sea surface temperature in a meteorological station in Antofagasta Bay and bottom temperature (16 m) in the sampling area at La Rinconada Marine Reserve (see Figure 1); (B) LOESS (Locally estimated scatterplot smoothing) regression of the relationship between sea surface temperature and bottom temperature in the sampling area (spam argument: 2/3; dashed lines represent 95% confidence intervals).
The larval abundance of A. purpuratus in the water column (Figure 5A) generally increased each year after the reproductive period (September–April), but it showed a strong interannual variability; there was a strong pulse at the end of the study period but the observed interannual variability was not related to EN-LN episodes. In turn, the settlement of A. purpuratus in artificial collectors generally increased through time but also displayed seasonal pulses that generally coincided with pulses of larval abundance (Figure 5B), with no evident responses to EN-LN episodes. In contrast, the larval settlement of A. purpuratus on Rhodymenia spp., as well as the abundance of this seaweed, decreased through time (Figure 5C). Moreover, there were not consistent seasonal changes in larval settlement on its natural settlement surface that could be related to seasonal or ENSO-related pulses of larval abundance.
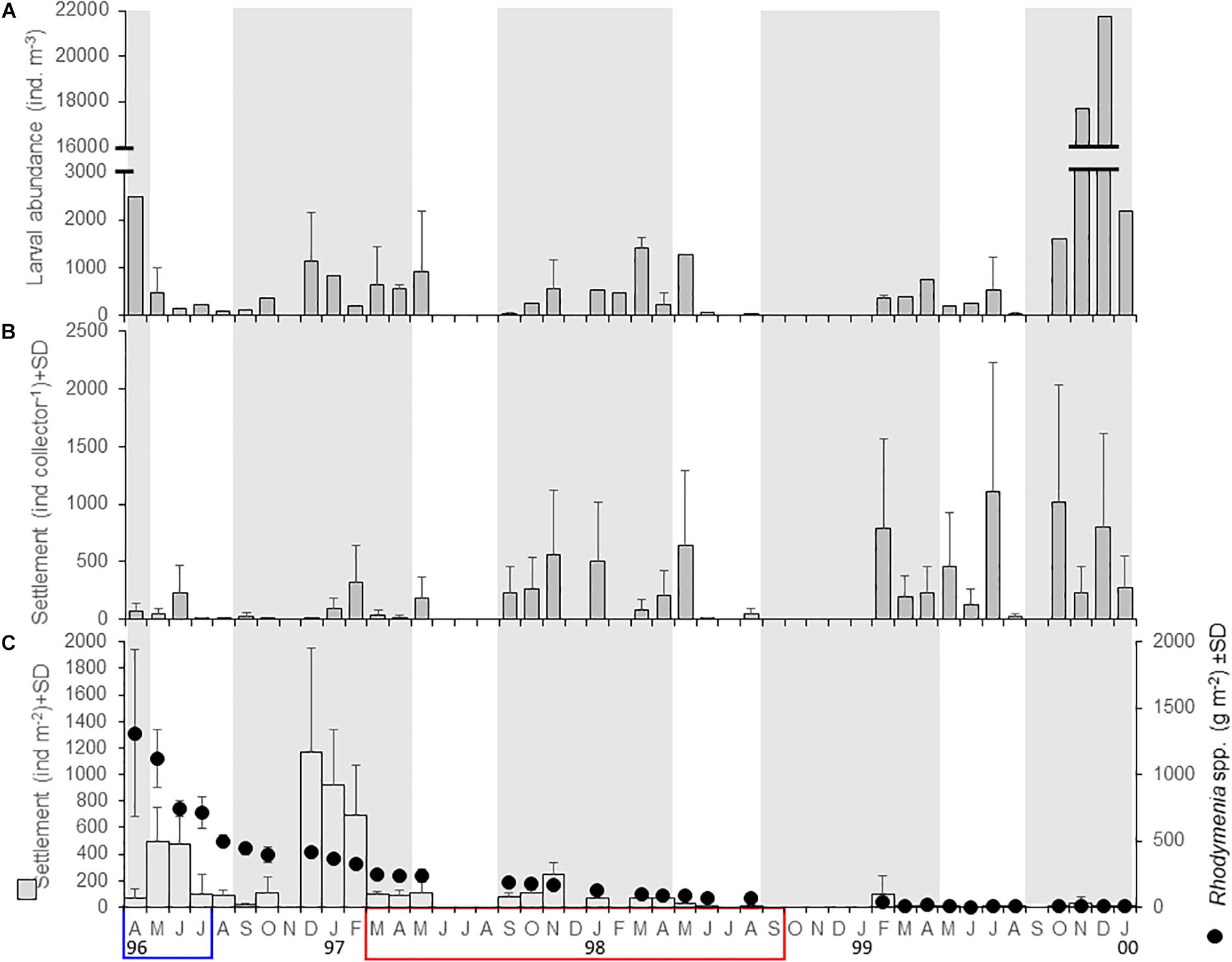
Figure 5. Temporal variability of population processes during the larval stage and settlement of Argopecten purpuratus in La Rinconada Marine Reserve between April 1996 and January 2000. (A) Abundance of larvae in the water column; (B) larval settlement in artificial collectors, and (C) larval settlement in the seaweed Rhodymenia spp. Blue and red panels indicate the occurrence of La Niña and El Niño episodes, respectively, according to El Niño Coastal Index (see Figure 3). Gray panels in the background denote periods of spawning activity after Cantillánez et al. (2005).
Although the relationships were weak, the larval settlement of A. purpuratus in artificial collectors (Figure 6A) was significantly related to the larval pool available in the water column and to the thermal variability in the study area, but not to the thermal forcing related to ENSO episodes (Figure 6A). In turn, none of those factors showed a significant effect on the settlement of the scallops in their natural settlement surface (Figure 6B); only the abundance of Rhodymenia spp. in the sandy bottom of La Rinconada showed a significant association to settlement success.
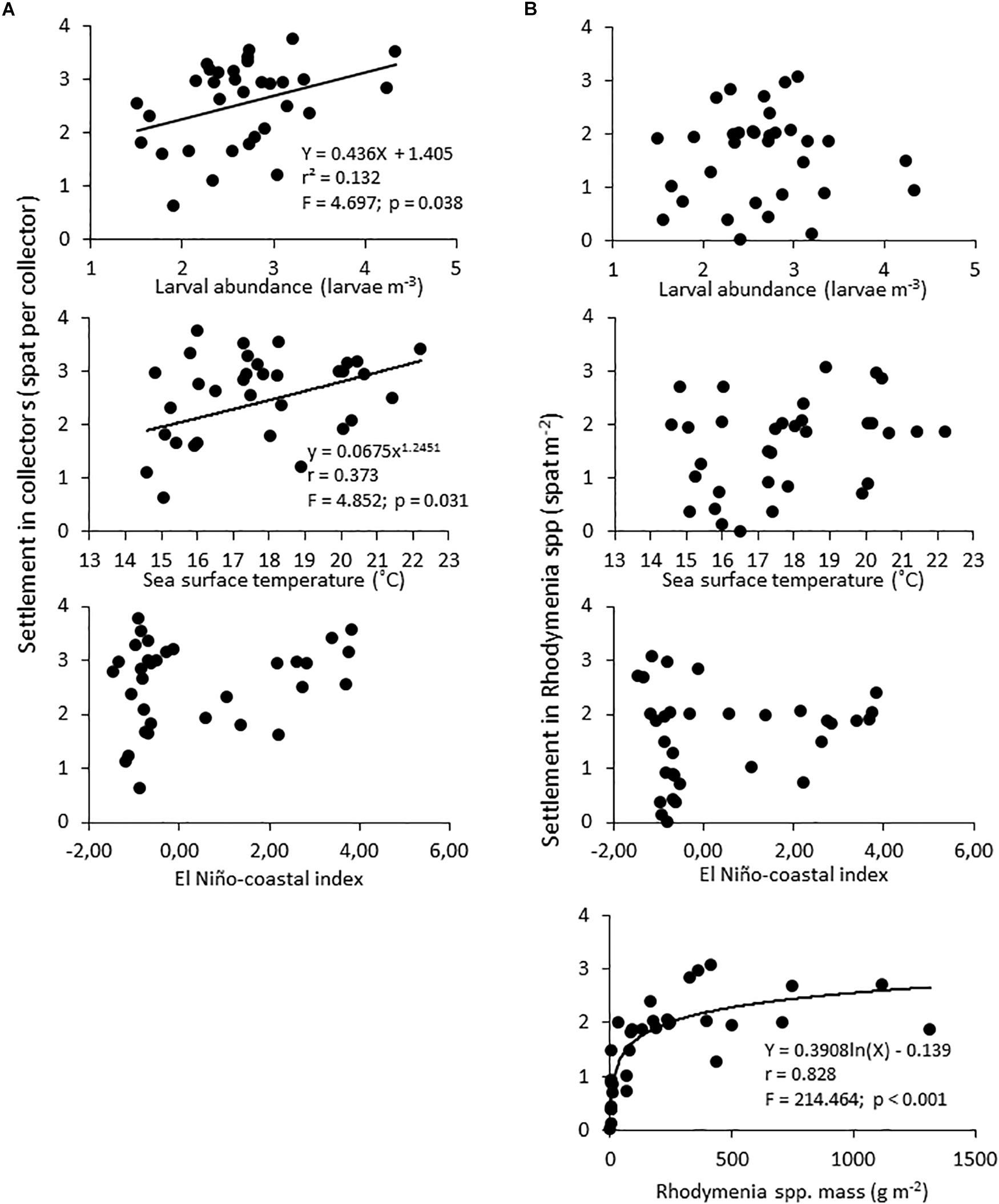
Figure 6. Bivariate scatterplots and model fittings assessing factors affecting the larval settlement of A. purpuratus in artificial collectors (A) and in Rhodymenia spp. (B). Data on larval settlement were transformed (Log X + 1) to meet the assumption of the models.
Discussion
Our results clearly show a strong interannual signal of EN-LN cycle, a regional-scale oceanographic process, on the rather consistent seasonal SST variability in Antofagasta Bay. The observed differences in the strength of this signal between summer and winter seasons can be partially explained by the lower number of strong La Niña episodes (ENCI < −1.4) occurring in summer in comparison with those during winter. But there is objectively more thermal variability in summer than in winter, if neutral or weak and mild El Niño and La Niña episodes are compared. This is more likely related to the particular oceanography of Antofagasta Bay, whereby water retention greatly magnifies the thermal seasonality in comparison with surrounding areas (Piñones et al., 2007). During summer, the pulsing increment of upwelling strength may bring even colder waters than those during winter, which can enter the bay by relaxation of thermal fronts forming upwelling shadows in coastal bays (Marín et al., 2003; Piñones et al., 2007). The good fit observed between bottom and surface temperature suggest that the described signal of EN-LN cycle also affects shallow bottom water in Antofagasta Bay. Notably, according to ENCI, the period 1999–2000 was considered neutral, as opposed to Oceanic Niño Index (based in thermal anomalies in the El Niño 3.4 region, central Pacific Ocean) that classified this period as La Niña conditions1.
As expected in a semi-enclosed system, the larval abundance was temporally coupled to the seasonally increased reproductive activity reported by Cantillánez et al. (2005) between September and April. However, a strong increment of larval abundance between October 1999 and January 2000 was the main feature in the variability of larval abundance. This was likely related to the establishment of La Rinconada Marine Reserve in 1997, that implied the increased abundance of large (sell length > 90 mm), sexually mature scallops (Cantillánez et al., 2007). Populations of A. purpuratus typically display outbreaks during exceptionally strong El Niño episodes, resulting from faster recovery between spawning events, increased larval production, increased settlement of larvae and increased survival rate and somatic production of juveniles (Illanes et al., 1985; Wolff, 1987; Cantillánez et al., 2005; Wolff et al., 2007). These changes seemingly herald responses of a relict of tropical Miocene fauna that evolved in the cold Humboldt Current System but retained warm water characteristics because recurrent El Niño episodes (Wolff, 1987; Uribe et al., 2012; Riascos et al., 2017). Therefore, it is surprising that larval abundance of A. purpuratus increased only slightly during El Niño 1997–1998 (Figure 5A). This can be explained by the fact that changes in gonado-somatic indices were not necessarily related to gamete release but also with atresia and oocyte lysis during this period. Moreover, within-day changes in temperature, which may be stronger than seasonal variations and important triggers of spawning episodes, were more common under non-El Niño periods (see Cantillánez et al., 2007).
Larval settlement of A. purpuratus in artificial collectors increased through time while that on Rhodymenia spp. showed a contrasting, decreasing trend. As artificial collectors are designed to mimic natural settling surfaces, the clear drop in the abundance of Rhodymenia spp. seems the factor driving this shifting temporal pattern. Therefore, our results suggest that the intra-annual dynamics in the scallop‘s larval settlement in artificial surfaces was largely influenced by the typical pulses of larval release determined by the reproductive cycle in the study area (Cantillánez et al., 2005). It is worth noting, however, that larval settlement did not increase in artificial or natural surfaces during the huge increase in larval abundance in the water column observed between November and December 1999, hinting on the strong influence of stochastic processes on larval settlement of scallops documented by Wolff (1988). Our results show that changes in temperature and the larval abundance of A. purpuratus in the water column were significant predictors of subsequent settlement in artificial collectors, but not of settlement in Rhodymenia spp. This, and the fact that those factors have been portrayed in the literature as determinants of larval settlement of A. purpuratus (Illanes et al., 1985; Wolff, 1988) suggest either additional influencing factors or increased stochasticity associated to larval settlement in the red algae. In turn, larval settlement in artificial or natural substrates did not show a significant relation to LN and EN thermal anomalies, despite clear thermal shifts related to this cycle in the study area. Instead, the strong and systematic reduction in the abundance of Rhodymenia spp. (from ∼1,300 to ∼7 g m–2) was the most important predictor of changes in larval settlement. Taylor et al. (2008) reported that the abundance of macroalgae, including Rhodymenia spp. decreased in response to warmer EN conditions in Independence Bay (Peru). However, the algal abundance was decreasing rapidly already in April 1996 under LN conditions and kept decreased during and after EN, hence we could not claim an effect of LN or EN episodes.
Owing to the observed shift in the larval settlement between artificial and natural surfaces, the question arises whether density of settled larvae in each surface changed through time, as a consequence of the reduction in the abundance of the macroalgae. To assess this, we plotted the number of larvae per gram of netlon rigid mesh or Rhodymenia spp. (Figure 7) and show that while the density of larvae settled on the artificial surface significantly increased as a function of time, the density on the natural surface did not. This suggests that intraspecific or interspecific interactions limits the settlement density in the red algae (as reported by Cragg, 2006 for the Anctarctic scallop) and precludes the increase in density as a mechanism to compensate the loss of natural settlement surfaces. Alternatively, this may indicate that other substrates may be substituting the red algae as a settlement surface.
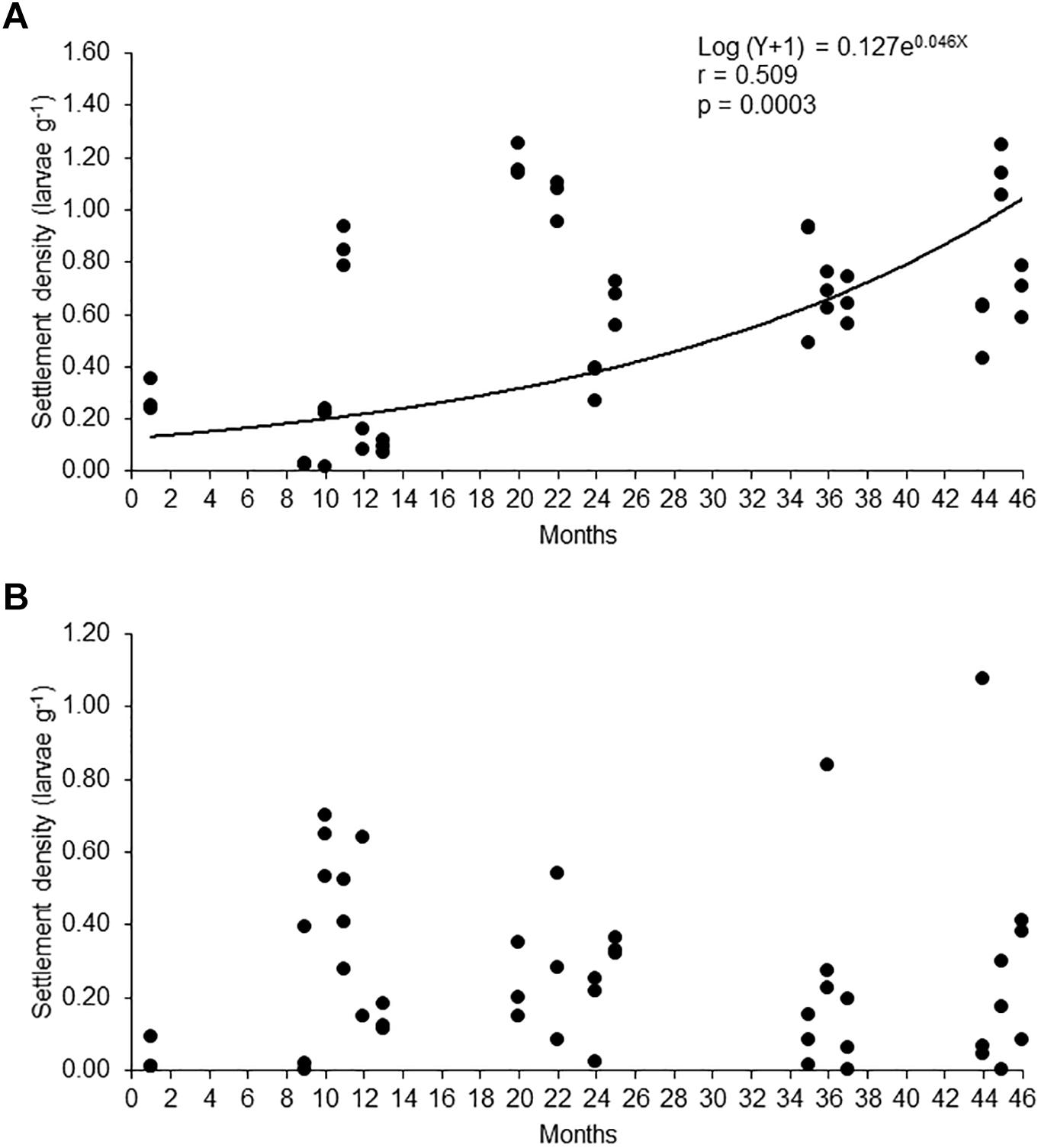
Figure 7. Comparison of the relationship of the log-transformed settlement density (log X + 1) in (A) artificial collectors and (B) in Rhodymenia spp. during months of high larval abundance (November–April) through the study period. Statistics associated with model fitting are given.
Rhodymenia spp. have been reported as the preferred settlement surface for A. purpuratus in the study area and elsewhere (Navarro et al., 1991; Avendaño and Cantillánez, 2008). Pectinid larvae generally use living (e.g., coralline algae, hydroids, briozoans, red algae) and non-living structures (e.g., empty shells, pebbles, mooring lines) above the seabed as a primary settling surface, probably to protect themselves from silting on bottom sediments (Orensanz et al., 1991; Harvey et al., 1993; Steller and Cáceres-Martínez, 2009). The importance of these structures for scallop recruitment has led to the suggestion that algal or rhodolith beds may serve as nursery grounds (Thouzeau, 1991) and that when they are not available they may limit scallop population size and stability (Orensanz et al., 1991).
Conclusion
Our study showed a clear relationship between regional thermal anomalies related to EN-LN phases of ENSO and local variability in sea surface and bottom temperature in La Rinconada Marine Reserve, with a stronger signal during the Austral winter. Despite these strong thermal changes, the observed variability in the transition from the larval stage to the subsequent settlement of A. purpuratus was mainly influenced by the continuous reduction in the availability of its preferred settlement surface – the red algae Rhodymenia spp. This reduction may have consequences for the stability of natural stocks of A. purpuratus, which is thought to be mainly affected by ENSO dynamics and misleading management.
Author Contributions
MA and MC obtained funding and conceived the monitoring program. JR conducted the statistical analysis. JR, MA, and MC conceived and wrote the manuscript and revised the final draft.
Funding
This research was supported by the “Fondo Nacional de Desarrollo Regional” (FNDR), Antofagasta, Chile (Grant Numbers 20124810-0 and 20127869-0).
Conflict of Interest
The authors declare that the research was conducted in the absence of any commercial or financial relationships that could be construed as a potential conflict of interest.
Footnotes
References
Ashton, T., Riascos, J. M., and Pacheco, A. (2008). First record of cymatium keenae Beu, 1970 (Mollusca: Ranellidae) from Antofagasta bay, northern chile, in connection with el niño events. Helgol. Mar. Res. 62, S107–S110. doi: 10.1007/s10152-007-0084-5
Avendaño, M., and Cantillánez, M. (1996). Efecto de la pesca clandestina, sobre Argopecten purpuratus (Lamarck, 1819), en el banco de la rinconada. II Región. Cienc. Tec. Mar. 19, 57–65. doi: 10.4067/S0716-078X2001000300012
Avendaño, M., and Cantillánez, M. (1992). Colecta artificial de semilla de A. purpuratus (Lamarck, 1819) en la bahía de mejillones, chile. II.- observaciones sobre niveles óptimos de captación. Estud. Oceanol. 11, 39–43.
Avendaño, M., and Cantillánez, M. (2008). “Aspectos biológicos y poblacionales de Argopecten purpuratus (Lamarck, 1819) en la reserva marina la rinconada: contribución para su manejo,” in Estado Actual del Cultivo y Manejo de Moluscos Bivalvos y su Proyección Futura: Factores que afectan su sustentabilidad en América Latina, eds A. Lovatelli, A. Farías, and I. Uriarte, (Rome: Actas de Pesca de la FAO), 249–266.
Avendaño, M., Cantillánez, M., Le Pennec, M., and Thouzeau, G. (2008). Reproductive and larval cycle of the scallop Argopecten purpuratus (Ostreoida: Pectinidae), during El niño-la niña events and normal condition in Antofagasta, northern chile. Rev. Biol. Trop. 56, 121–132. doi: 10.15517/rbt.v56i1.5512
Avendaño, M., and Cantillánez, M. (2016). Evaluación del desempeño de la primera reserva marina de chile, la rinconada, en el ámbito de la conservación de su especie focal, el ostión del norte Argopecten purpuratus. Rev. Biol. Mar. Oceanogr. 51, 407–420. doi: 10.4067/S0718-19572016000200017
Cantillánez, M., Avendaño, M., Thouzeau, G., and Le Pennec, M. (2005). Reproductive cycle of Argopecten purpuratus (Bivalvia: Pectinidae) in la rinconada marine reserve, (Antofagasta, Chile): response to environmental effects of El niño and la niña. Aquaculture 246, 181–195. doi: 10.1016/j.aquaculture.2004.12.031
Cantillánez, M., Thouzeau, G., and Avendaño, M. (2007). Improving Argopecten purpuratus (Bivalvia: Pectinidae) culture in la rinconada marine reserve (Antofagasta, Chile): results from the study of larval and post-larval stages in relation to environmental forcing. Aquaculture 272, 423–443. doi: 10.1016/j.aquaculture.2007.07.211
Carstensen, D., Riascos, J. M., Heilmayer, O., Arntz, W. E., and Laudien, J. (2010). Recurrent, thermally-induced shifts in species distribution range in the humboldt current upwelling system. Mar. Environ. Res. 70, 293–299. doi: 10.1016/j.marenvres.2010.06.001
Castilla, J. C., Lagos, N. A., Guiñez, R., and Largier, J. (2002). “Embayments and nearshore retention of plankton: the Antofagasta bay and other examples,” in The Oceanography and Ecology of the Near Shore and Bays in Chile. Proceedings of the International Symposium on Linkages and Dynamics of Coastal Systems: Open Coasts and Embayments, eds J. C. Castilla, and J. Largier, (Santiago: Ediciones Universidad Católica de Chile), 179–203.
Cragg, S. M. (2006). “Development, physiology, behaviour, and ecology of scallop larvae,” in Scallops: Biology, ecology, and aquaculture, eds S. E. Shumway, and G. J. Parsons, (Amsterdam: Elsevier), 45–122. doi: 10.1016/s0167-9309(06)80029-3
Davies, K. T., Gentleman, W. C., DiBacco, C., and Johnson, C. L. (2015). Fisheries closed areas strengthen scallop larval settlement and connectivity among closed areas and across international open fishing grounds: a model study. Environ. Manage. 56, 587–602. doi: 10.1007/s00267-015-0526-9
Dickie, L. M. (1995). Fluctuation in abundance of the giant scallop, Placopecten magellanicus (Gmelin) in the digby area of the bay of fundy. J. Fish. Res. Board Can. 12, 797–857. doi: 10.1139/f55-045
Escribano, R., and Hidalgo, P. (2001). Circulación inducida por el viento en bahía de Antofagasta, norte de chile (23° S). Rev. Biol. Mar. Oceanogr. 36, 43–60. doi: 10.4067/S0718-19572001000100005
Harvey, M., Bourget, E., and Miron, G. (1993). Settlement of iceland scallop chlamys islandica spat in response to hydroids and filamentous red algae: field observations and laboratory experiments. Mar. Ecol. Prog. Ser. 99, 283–292. doi: 10.3354/meps099283
Illanes, J. E., Akaboshi, S., and Uribe, E. (1985). Efectos de la temperatura en la reproducción del ostión del norte Argopecten purpuratus en la bahía de tongoy durante el fenómeno el niño. Invest. Pesq. 32, 167–173.
Marín, V. H., Delgado, L. E., and Escribano, R. (2003). Upwelling shadows at mejillones b ay (northern Chilean coast): a remote sensing in situ analysis. Invest. Mar. 31, 47–55.
Navarro, R., Sturla, L., Cordero, L., and Avendaño, M. (1991). in Scallop: Biology, Ecology and Aquaculture, ed. S. Shumway, (Amsterdam: Elsevier Science), 1001–10015.
Nicolle, A., Moitié, R., Ogor, J., Dumas, F., Foveau, A., Foucher, E., et al. (2016). Modelling larval dispersal of pecten maximus in the english channel: a tool for the spatial management of the stocks. ICES J. Mar. Sci. 74, 1812–1825. doi: 10.1093/icesjms/fsw207
Orensanz, J., Parma, A., and Iribarne, O. (1991). “Population dynamics and management of natural stocks,” in Scallop: Biology, Ecology and Aquaculture, ed. S. Shumway, (Amsterdam: Elsevier Science), 625–713.
Paulet, Y. M., Bekhadra, F., Devauchelle, N., Donval, A., and Dorange, G. (1997). Cycles saisonniers, reproduction et qualité des ovocytes chez pecten maximus en rade de brests. Ann. Inst. Oceanogr. 73, 101–112.
Piñones, A., Castilla, J. C., Guiñez, R., and Largier, J. L. (2007). Temperaturas superficiales en sitios cercanos a la costa en la bahía de Antofagasta (Chile) y centros de surgencia adyacentes. Cienc. Mar. 33, 37–48. doi: 10.7773/cm.v33i1.1226
Riascos, J. M., Carstensen, D., Laudien, J., Arntz, W. E., Oliva, M. E., Güntner, A., et al. (2009). Thriving and declining: climate variability shaping life-history and population persistence of Mesodesma donacium in the humboldt upwelling system. Mar. Ecol. Prog. Ser. 385, 151–163. doi: 10.3354/meps08042
Riascos, J. M., Solís, M. A., Pacheco, A. S., and Ballesteros, M. (2017). Breaking out of the comfort zone: el niño-southern oscillation as a driver of trophic flows in a benthic consumer of the humboldt current ecosystem. Proc Biol Sci. 284:20170923. doi: 10.1098/rspb.2017.0923
R Core Team (2018). R: A Language and Environment for Statistical Computing. Vienna: R Foundation for Statistical Computing.
Steller, D. L., and Cáceres-Martínez, C. (2009). Coralline algal rhodoliths enhance larval settlement and early growth of the pacific calico scallop Argopecten ventricosus. Mar. Ecol. Prog. Ser. 396, 49–60. doi: 10.3354/meps0826
Stotz, W. B. (2000). When aquaculture restores and replaces a overfished stock: is the conservation of the species assured? The case of the scallop Argopecten purpuratus (Lamarck, 1819) in northern Chile. Aquac. Int. 8, 237–247. doi: 10.1023/A:100921511
Takahashi, K., Mosquera, K., and Reupo, J. (2014). El Índice Costero El Niño (ICEN): historia y actualización. Boletín Técnico, Instituto Geofísico del Perú 1: 8–9. Available at: http://www.met.igp.gob.pe/datos/icen.txt (accessed January 19, 2018).
Taylor, M. H., Wolff, M., Mendo, J., and Yamashiro, C. (2008). Changes in trophic flow structure of independence bay (Peru) over an ENSO cycle. Prog. Oceanogr. 79, 336–351. doi: 10.1016/j.pocean.2008.10.006
Thiel, M., Macaya, E. C., Acuña, E., Arntz, W. E., Bastias, H., Brokordt, K., et al. (2007). The humboldt current system of northern and central chile: oceanographic processes, ecological interactions and socioeconomic feedback. Oceanogr. Mar. Biol. 45, 195–344. doi: 10.1201/9781420050943.ch6
Thouzeau, G. (1991). Experimental collection of postlarvae of Pecten maximus (l.) and other benthic macrofaunal species in the bay of saint-brieuc, france: I. Settlement patterns and biotic interactions among the species collected. J. Exp. Mar. Biol. Ecol. 148, 159–180. doi: 10.1016/0022-0981(91)90080-G
Trexler, J. C., and Travis, J. (1993). Nontraditional regression analyses. Ecology 74, 1629–1637. doi: 10.2307/1939921
Uribe, R. A., Oliva, M. E., Aguilar, S., Yamashiro, C., and Riascos, J. M. (2012). Latitudinal variation in the reproductive cycle of two bivalves with contrasting biogeographical origin along the humboldt current upwelling ecosystem. Sci. Mar. 76, 713–720. doi: 10.3989/scimar.03480.26D
Wolff, M. (1987). Population dynamics of the peruvian scallop Argopecten purpuratus during the EL NIÑO phenomenon of 1983. Can. J. Fish. Aquat. Sci. 44, 1684–1691. doi: 10.1139/f87-207
Wolff, M. (1988). Spawning and recruitment in the peruvian scallop Argopecten purpuratus. Mar. Ecol. Prog. Ser. 42, 213–217. doi: 10.3354/meps042213
Keywords: Pectinidae, larval settlement, larval ecology, ENSO, Humboldt Current System, marine reserves
Citation: Avendaño M, Cantillánez M and Riascos JM (2019) The Decreasing Availability of Settlement Surfaces Affects the Transition From Larvae to Early Recruitment of the Scallop Argopecten purpuratus Through El Niño and La Niña Episodes. Front. Mar. Sci. 6:630. doi: 10.3389/fmars.2019.00630
Received: 22 November 2018; Accepted: 24 September 2019;
Published: 11 October 2019.
Edited by:
Susana Carvalho, King Abdullah University of Science and Technology, Saudi ArabiaReviewed by:
Yoichi Miyake, The University of Tokyo, JapanSteve P. Geiger, Florida Fish and Wildlife Research Institute, United States
Rodrigo Gaspar Soria, Centro Para el Estudio de Sistemas Marinos (CONICET), Argentina
Copyright © 2019 Avendaño, Cantillánez and Riascos. This is an open-access article distributed under the terms of the Creative Commons Attribution License (CC BY). The use, distribution or reproduction in other forums is permitted, provided the original author(s) and the copyright owner(s) are credited and that the original publication in this journal is cited, in accordance with accepted academic practice. No use, distribution or reproduction is permitted which does not comply with these terms.
*Correspondence: José M. Riascos, jose.riascos@udea.edu.co