- 1National Marine Environmental Monitoring Center, Ministry of Ecology and Environment, Dalian, China
- 2Department of Applied Mathematics/Research Centre for Experimental Marine Biology and Biotechnology, University of the Basque Country, Bilbao, Spain
- 3Key Laboratory of Marine Ecosystem and Biogeochemistry, State Oceanic Administration and Second Institute of Oceanography, Ministry of Natural Resources, Hangzhou, China
Polychlorinated biphenyls (PCBs) and organochlorine pesticides (OCPs), including hexachlorocyclohexanes (HCHs) and dichlorodiphenyltrichloroethanes (DDTs), were studied in the surface sediments of Qingduizi Bay (Yellow Sea, China). The goal was to identify whether their distribution and levels can be influenced by aquaculture activities in ponds. The total concentration of PCBs in mariculture ponds (MP) and the outer bay (OB) ranged from 0.61 ng g–1 to 2.10 ng g–1 dry weight (dw) and from 0.79 to 2.41 ng g–1 dw, respectively. The concentrations of hexachlorocyclohexanes (HCHs) and dichlorodiphenyltrichloroethanes (DDTs), ranged from 0.03 to 0.18 ng g–1 dw and from 0.24 to 31.14 ng g–1 dw, respectively. The levels of PCBs were significantly different between MP and OB, whereas no significant differences of DDTs and HCHs were detected between the two areas. Among the PCB congeners, tri-PCB and tetra-PCB were dominant, implying a historical input and atmospheric deposition for PCBs. The isomeric ratios of the compositions implicated mixture sources of new and historical inputs for HCHs. However, DDT-containing feed and pesticides in aquaculture activities could be the primary source of newly inputted DDTs. A parallel ecological risk assessment analysis showed the impact of DDTs on marine organisms. In conclusion, aquaculture in ponds influences the occurrence and distribution of persistent halogenated compounds in coastal areas, causing potential ecological impacts. This result should be a baseline for the management of aquaculture ponds regarding these compounds.
Introduction
Coastal ecosystems are the environments most vulnerable to pollution due to anthropogenic stresses worldwide (Ricciardi and Atkinson, 2004). Large amounts of contamination, including persistent organic pollutants (POPs), nutrients and heavy metals, are introduced into estuarine and coastal systems because of rapid increases in agricultural, industrial and domestic activities, which are causing a series of toxicological impacts on marine species worldwide (Islam and Tanaka, 2004). POPs are widely used in agricultural and industrial processes and have attracted considerable interest because of their ubiquity, persistence, bio-accumulation, and biotoxicity (Sharma et al., 2014).
Polychlorinated biphenyls (PCBs), comprising 10 homologs and 209 congeners with various numbers and positions of chlorine atoms, are commonly used as a heat transfer medium in capacitors and transformers and are also perfect additives in industrial materials, such as dyed paper, plastics and paints, because of their high thermoresistance and low electrical conductivity (Ishikawa et al., 2007; Yu et al., 2014). Organochlorine pesticides (OCPs) encompass a wide range of chemicals, including hexachlorocyclohexanes (HCHs), dichlorodiphenyltrichloroethanes (DDTs), dieldrin, endrin, and aldrin. They have also been widely used for pest management in agriculture and aquaculture because of their high efficiency and low cost (Wu et al., 2015). More than 10,000 tons of PCBs, 0.4 million tons of DDTs, and 4.5 million tons of HCHs were used in China from the 1950s to 1980s owing to the population explosion and the increasing requirements for food (Wei et al., 2007; Bao et al., 2012). Though, in China, the manufacture and agricultural usage of most PCBs and OCPs were banned in 1974 and 1983, respectively (Wang et al., 2005), these organic chemicals can still be routinely detected in various environmental compartments (i.e., in air, water, soil, sediments, and organisms) (Adeleye et al., 2016; Li et al., 2017; Zhao et al., 2018). Due to the slow degradation of PCBs and OCPs, the residues and their catabolites would persist in the ecosystem for a long time (Yu et al., 2014; Wu et al., 2015). Therefore, these pollutants in environmental mediums could provide valuable records and represent potential ecological risks that should be seriously considered. However, currently, there are few available reports focusing on the levels and risk assessments of PCBs and OCPs in riverine bays.
Organochlorine pesticides and PCB residues tend to attach to suspended particulates and end up in the sediment by deposition and sedimentation because of their refractory and hydrophobic characteristics in the marine environment (Miglioranza et al., 2004; Zimmerman et al., 2004). Thus, sediments act as an important reservoir for OCP and PCB contaminations and as an efficient indicator for monitoring the pollution levels of the benthic system (Okay et al., 2009; Barhoumi et al., 2014; Yang et al., 2018). The absorbed organic pollutants can also be released and resuspended in seawater via biological and physicochemical processes, which can result in secondary pollution and can even threaten associated biota and ecosystems through bio-accumulation and bio-amplification (Gui et al., 2014; Tsygankov et al., 2015). Therefore, the investigation of PCBs and OCPs in surface sediments can provide a valuable record of contamination in an aquatic environment.
With the increasing demand for animal protein worldwide, the marine aquaculture industry in China has rapidly developed, and its aquatic products are acknowledged as high-quality protein (Cao et al., 2015). However, over-intensive aquaculture activity may lead to serious marine pollution due to the unreasonable use of pesticides and feeds (Primavera, 2006). Qingduizi Bay is a well-known and important pond culture zone and is among the few estuarine bays along the northern Yellow Sea. It receives runoff inputs from three rivers, making identification of the pollution sources difficult. Thus, understanding the sources and ecological impacts of xenobiotics in typical coastal areas, especially in dense mariculture areas, is crucial to protect and maintain habitat quality and ecosystem health (Barbier et al., 2011). Investigations of PCBs and OCPs in estuarine and costal environments have been conducted worldwide (Lebeuf and Nunes, 2005; Wang et al., 2010; Yuan et al., 2015; Montuori et al., 2016). However, studies focusing on the PCBs and OCPs in Qingduizi Bay are limited. The primary objectives of this study are to (i) perform a comparative study concerning the occurrence and distribution of PCBs and OCPs in the surface sediments of mariculture ponds and the adjacent outer areas in a bay; (ii) clarify whether the increasing aquaculture activities cause a fresh input of the pollutants; and (iii) assess the potential ecotoxicological risks of PCBs and OCPs in sediments for benthic organisms. The obtained data could provide important baseline information for environmental monitoring and could be helpful in policy-making on sustainable development in dense mariculture areas.
Materials and Methods
Study Areas
Qingduizi Bay (39°41′59′′–39°49′31′′ N, 123°11′41′′–123°26′06′′ E) is an important estuarine bay in the northern Yellow Sea of China, receiving runoff inputs from the Inna, Huli and Diyin rivers, with an average flow of 7.9 × 108 m3 yr–1, and forms the present complex deltaic system, which covers a tidal flat area of 130 km2 (Editorial Board of China Bay Survey, 1991). It is a semi-enclosed bay and is among the most developed bays for mariculture in China. It is exposed to stress from anthropogenic activity, including dockyards, sea farming along the coastline, and sewage discharge from pond culture (Wu et al., 2011). The main cultured species include Fenneropenaeus chinensis, Rhopilema esculentum, Meretrix meretrix, and Apostichopus japonicus. Sea reclamation had been carried out, with the expanded farming areas ranging from 5958 to 8356 hm2 between 1990 and 2013, representing about 53% of the total area of Qingduizi Bay (Miao et al., 2014; Liu et al., 2018). The pond culture areas cover approximately 5384 hm2 by 2013 (Yin, 2013). Substantial amounts of organic pollutants (e.g., residual feeds and pesticides) generated from aquaculture ponds are discharged into the seawater (Wang et al., 2019).
Field Sampling and Sample Preparation
The study area was divided into two parts: one is a mariculture pond (MP), which is occupied by a number of aquaculture ponds, and the other is the outer bay (OB), where no mariculture activities occur. Five and four sections perpendicular to the bay mouth were set in MP and OB, respectively (Figure 1). A total of 30 sampling sites (14 in MP and 16 in OB) were investigated in August 2012. For each site, the undisturbed surface sediments were gently collected using a stainless-steel grab sampler. The uppermost 5 cm of sediment was accurately collected and mixed, and then placed in pre-cleaned glass jars that had been prewashed with n-hexane and distilled water. The samples were initially stored in a freezer until delivery to the laboratory and kept at −20°C for further analysis. All samples were freeze-dried under conditions of −52°C and <20 Pa with a vacuum freeze dryer (FD-1C-50, Beijing Biocool Instrument, Co., Ltd., Beijing, China) for 36 h to a constant weight. Then, the samples were sieved through a 100-mesh sieve, homogenized, and stored at 4°C for further analysis.
Reagents and Standards
All the standards and chemicals used in the study were purchased from Chem Service (West Chester, PA, United States). The standard solutions for PCBs contain 27 homolog compositions, including PCB8, PCB18, PCB28, PCB52, PCB44, PCB66, PCB101, PCB77, PCB81, PCB123, PCB118, PCB114, PCB153, PCB105, PCB138, PCB126, PCB187, PCB128, PCB167, PCB156, PCB169, PCB180, PCB157, PCB170, PCB189, PCB195, and PCB206. The standard solutions for OCPs contain α-, β-, γ- and δ-HCH, heptachlor, heptachlor epoxide, aldrin, dieldrin, endrin, endrin aldehyde, p,p′-dichlorodiphenyltrichloroethane (p,p′-DDT), p,p′-dichlorodiphenyldichloroethane (p,p′-DDD), p,p′-dichlorodiphenyldichloroethylene (p,p′-DDE), methoxychlor, α-chlordane, γ-chlordane, endosulfan I, and endosulfan II. All standard solutions were diluted using appropriate volumes of isooctane.
Analysis of PCBs and OCPs
The extraction procedures for PCBs and OCPs in freeze-dried sediment samples were carried out using the 33 mL stainless-steel vessels of a Dionex ASE 200 accelerated solvent extractor system (Dionex GmbH, Idstein, Germany) following the description of Richter et al. (1996). In brief, exactly 5.0 g of single sample was homogenized with a 1.0 g mixture of 2:1 Florisil/Al2O3 and 10 μL of surrogate standards (13C-PCB 28, 13C-PCB 111, and 13C-PCB178) in each vessel. Extraction was performed using hexane/dichloromethane in a 1:1 ratio under 100°C and 10.3 × 106 Pa. Two static cycles (each cycle maintained for 5 min) were performed for extraction. At the end, the extraction cell was flushed with solvent (60% of the cell volume) and purged with nitrogen (100 s). The fraction was evaporated to 2 mL using a rotary evaporator. Then, 1.0 g activated copper was added for the clean-up. The extracts were transferred to a chromatographic column packed with silica gel (4.0 g), alumina-N (6.0 g) and sodium sulfate (1.0 g). The column was eluted with 80 mL of n-hexane/dichloromethane (v:v, 3:2). The eluates were evaporated to 1.0 mL, then to 100.0 μL under a gentle stream of nitrogen at 50°C, and transferred for analysis. 10.0 μL of the internal standard (13C-PCB141) solution was spiked before the instrumental analysis.
GC–MS/MS was used for PCB and OCP analysis. A 7890A GC (Agilent, United States) equipped with a 30 m × 0.25 mm × 0.25 μm HT8 column (SGE, United States) coupled to a 7000B MS (Agilent, United States) operated in EI + SRM was used. Injection was pulsed splitless at 280°C with helium as carrier gas at 1.0 mL/min. The GC temperature program was 60°C (1 min hold), then 40°C/min to 120°C, and finally 5°C/min to 300°C, held for 3 min. The concentrations of target elements were quantified by a six-point calibration curve and were corrected by surrogates.
Physio-Chemical Parameters of the Sediments
Six bulk physio-chemical parameters, including the percentage of total carbon (TC), total nitrogen (TN), total organic carbon (TOC), organic matter (OM), mud fractions (silt–clay) and ratios of TC and TN (C/N) of sediments, were estimated from the composite sub-sample in each site. For TOC, the samples were pretreated with 0.1 mol L–1 HCl solution for 18 h to remove inorganic carbon. Then the acidified sediments were rinsed with deionized water and freeze-dried before analysis. The sedimental TN, TC, and TOC were determined using a Vario Macro CHN element analyzer (Elementar Analysensysteme GmbH, Hanau, Germany). C/N values were calculated using TN and TC ratios. OM was determined through loss on ignition (24 h at 450°C) according to the method described by Craft et al. (1991). The proportions of mud (silt–clay fractions, <0.63 μm) were determined by sieving the dried samples following the method described by Soares et al. (1999).
Quality Control and Quality Assurance
Procedure blanks, solvent blanks, spiked samples with standards, and duplicate samples were applied to analyze the cross contaminations and check the quality of chemical compounds. The losses involved during the analysis were also compensated by comparing surrogate standards. The target compounds were not detected in the method blanks. The average surrogate recoveries were 82 ± 9, 86 ± 12, and 92 ± 11% for 13C-PCB 28, 13C-PCB 111, and 13C-PCB178, respectively. The limit of detection (LOD) of individual PCB and OCP compounds has been listed in Supplementary Material. The relative standard deviations of repeatability and reproducibility were < 5%, which showed the acceptable repeatability and reproducibility of the measurement.
Potential Risk Assessments
To evaluate the adverse ecological effects caused by PCBs and OCPs, two commonly used sediment quality guidelines (SQGs) were applied. These compared the concentrations of PCBs and OCPs, namely, the effects range-low (ERL) and effects range-median (ERM) value guidelines from the United States Environmental Protection Agency (Usepa, 1998) and the threshold effects level (TEL) and probable effects level (PEL) guidelines from the Canadian Council of Ministers of the Environment (Ccme, 2002). The ERL was derived from the low 10th percentile of the effect data for the organic contaminants, and the ERM was derived from the median of the effect data (Long et al., 1995, 1998). The ERL and ERM values separated the concentrations of particular chemicals into three ranges to represent adverse biological effects: “rarely,” “occasionally,” and “frequently observed.” Both the TEL and PEL guidelines are based on the benthic community metrics and toxicity test results. The TEL was derived by calculating the geometric mean of the 15th percentile concentrations of the toxic effects dataset and the median of the no-effect dataset, while the PEL was calculated as the geometric mean of the 50th percentile of the effect dataset and the 85th percentile of the no-effect dataset (Long et al., 1998). The TEL and PEL values separated concentrations of specified chemicals into “no-effect range,” “possible effect range,” and “probable effect range.”
Statistical Analysis
ArcGIS 10.0 was used to reflect the sampling locations and spatial distribution of the sedimental indicators, PCBs and OCPs across the study area. The Kriging interpolation method was applied to predict the unknown attribute values at certain sites and to describe the distribution pattern of six sedimental indicators. Pearson correlation analysis was conducted with R statistical software (R Core Team, 2016) using the “ggcorrplot” package to estimate the relationships between organic pollutants and sedimental indicators. Principal component analysis (PCA) using IBM SPSS Statistics 19.0, was applied to the correlation matrix with Varimax rotation and Kaiser Normalization for POP concentrations in the sediments to identify the possible sources of organic pollutants.
Results and Discussion
Spatial Variation of Sedimental Indicators
Due to the complex nature of organic matter in aquatic sediments, sedimental physio-chemical parameters, such as TOC, particle size fraction and Corg/N, are effective and widely used for determining the different signatures and transport routes of OM sources (e.g., Bröder et al., 2016; Koziorowska et al., 2016). Mud contents (silt–clay; <0.063 mm) showed a decreased trend from the nearshore to the offshore, with percentages ranging from 0.25 to 0.86% (Figure 2E). TOC contents ranged between 0.20 and 1.09% with a median value of 0.59%. High levels generally occurred in the mouths of the Huli River and Diyin River (Figure 2A). Higher levels of TOC and mud contents are generally detected in the mouths of rivers, indicating fresh terrigenous inputs linked to increased development (via land reclamation and construction activities) in this catchment. OMs exhibited an increased trend from the nearshore toward the offshore, with percentages ranging from 1.97 to 7.87% (Figure 2B). In contrast to OM, TC, TN and Corg/N of the sediments showed similar distribution trends, with high levels measured in the southeast of OB. The levels varied from 0.04 to 4.72% (with an average value of 1.27%), from 0.17 to 0.57% (with an average value of 0.28%), and from 0.90 to 5.57% (with an average value of 2.31%) for TC, TN, and Corg/N, respectively (Figures 2C–F). The Corg/N ratio generally provided valuable information concerning the origin of the OM. Typical Corg/N values derived from fresh marine biogenic OM range from 4 and 10, whereas OM from terrestrial sources has a ratio of higher than 20 due to the content of peptide material (Meyers, 1994). The low Corg/N values in the present study suggest a greater marine contribution than the terrestrial source. However, one should be cautious with such an interpretation since a preferential utilization of C and bacterial immobilization of N must be considered. Diagenetic processes and complicated transport routes should also be considered because of the special geographical environment (riverine bay) and intense human activities (mariculture).
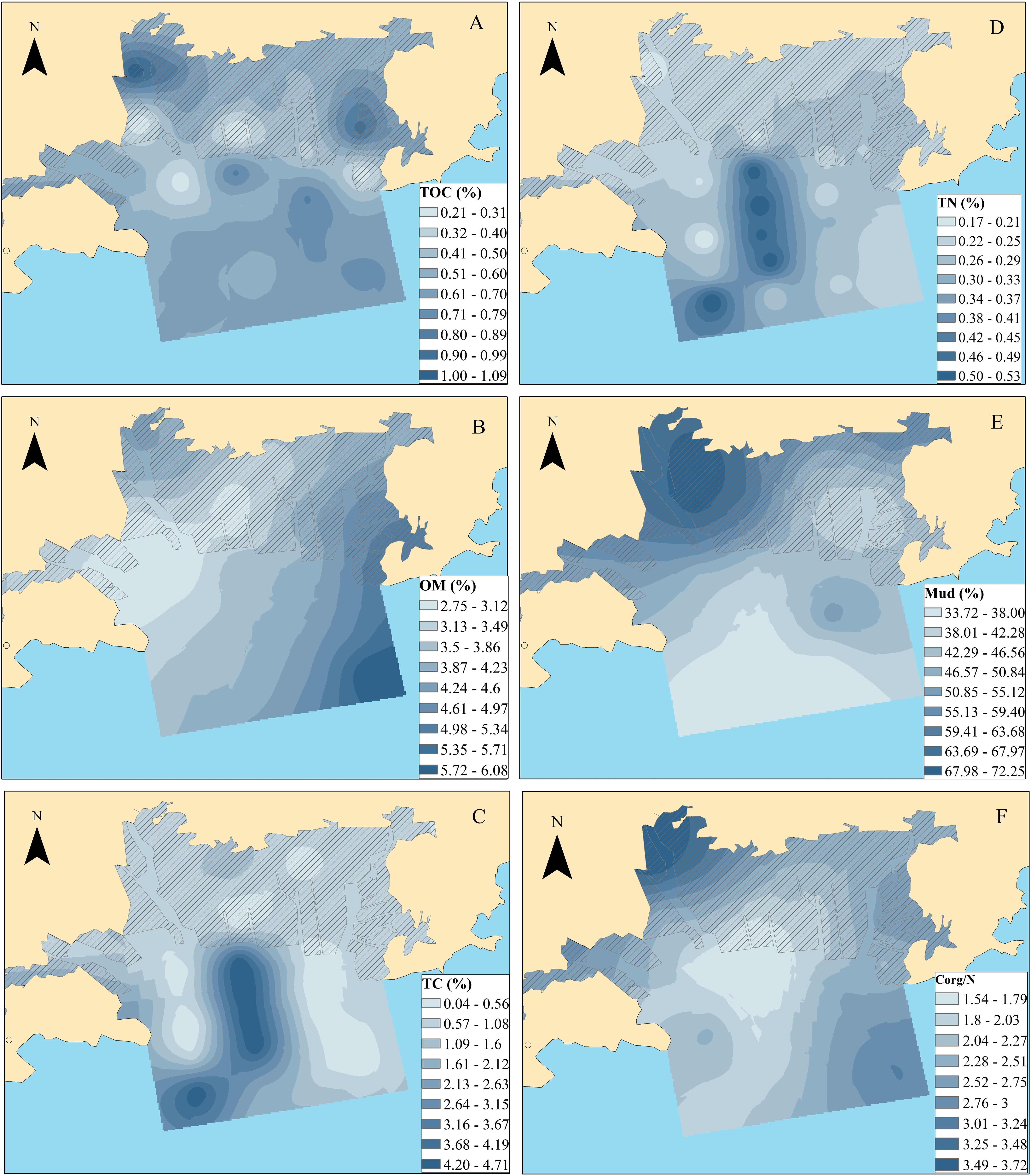
Figure 2. Bulk physio-chemical parameters in the sediments of Qingduizi Bay [(A) TOC; (B) OM; (C) TC; (D) TN; (E) mud fractions (silt + clay); (F) Corg/N].
Occurrence of PCBs
The spatial distribution and levels of ΣPCBs (the sum of the 27 congeners of PCBs) in the sediments of Qingduizi Bay are shown in Figure 3A. PCBs were detected in all the sediment samples, suggesting ubiquitous contamination of these compounds in the aquatic environment of Qingduizi Bay. The residual values of Σ27PCBs ranged from 0.61 to 2.10 ng g–1 dw (mean: 1.11 ng g–1 dw) in MP and from 0.79 to 2.41 ng g–1 dw (mean: 1.57 ng g–1 dw) in OB (Figure 3B). Higher levels of PCBs were detected in OB (p = 0.004).
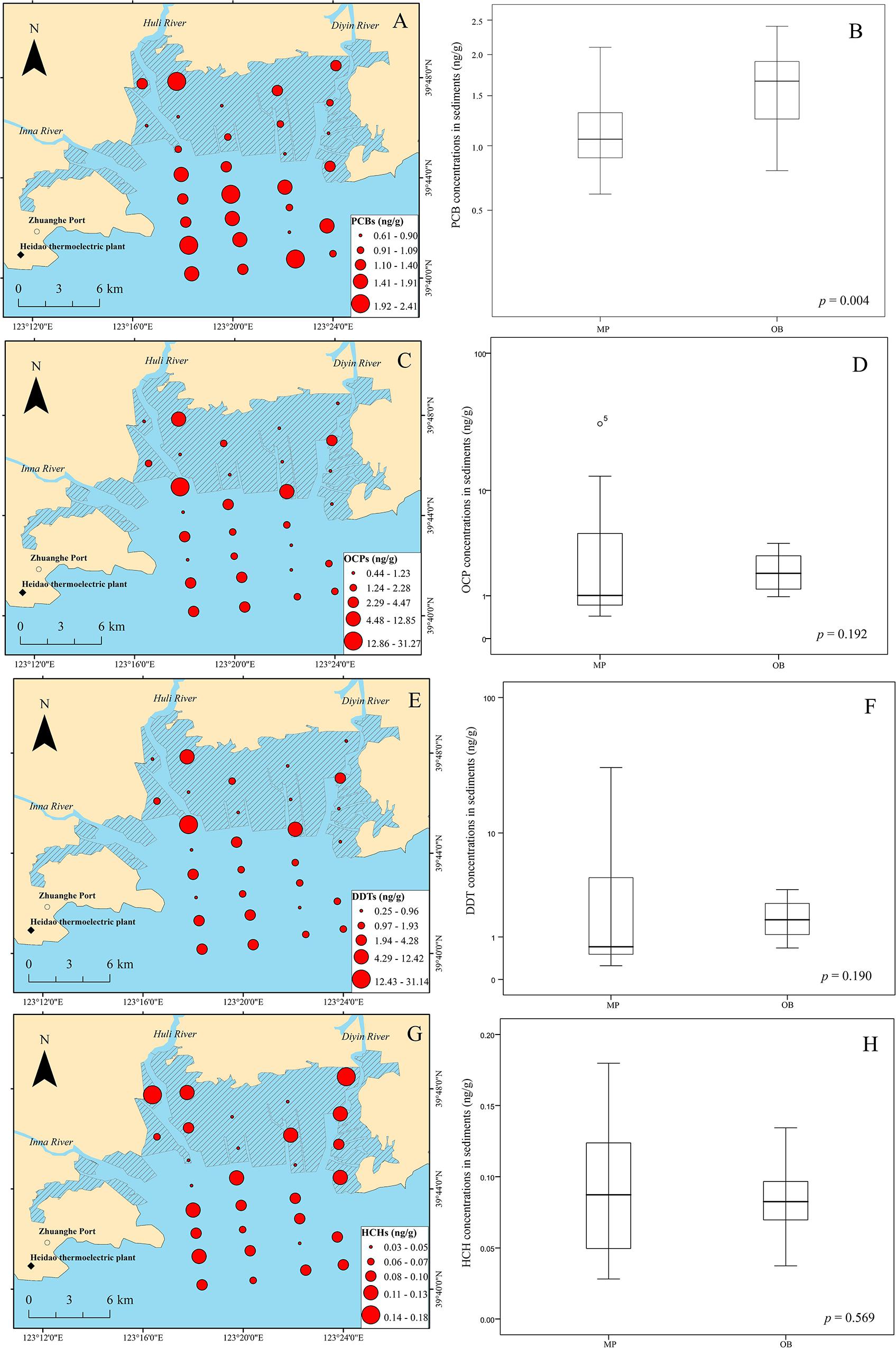
Figure 3. Spatial distribution of PCBs (A), OCPs (C), DDTs (E) and HCHs (G), and boxplots for PCBs (B), OCPs (D), DDTs (F) and HCHs (H) in the surface sediments of Qingduizi Bay.
Previous studies have reported the PCB levels in surface sediments from other bays and estuaries around the world (Table 1). The PCB levels in Qingduizi Bay were generally far below those of more developed industrial estuaries and bays in China, such as Shantou Bay (Shi et al., 2016), three typical bays (Yueqing Bay, Xiangshan Bay, and Sanmen Bay) in the east China Sea (Yang et al., 2011), the Yangtze Estuary (Gao et al., 2013) and the Shuangtaizi Estuary (Yuan et al., 2015); and in other areas of the world, such as Casco Bay in the United States (Kennicutt et al., 1994), Rhone Prodelta in France (Tolosa et al., 1995) and Bizerte lagoon in Tunisia (Barhoumi et al., 2014), yet comparable to the PCB levels in the Daliao Estuary (China) (Men et al., 2014) and Guaratuba Bay (Brazil) (Combi et al., 2013).
The homolog profiles of PCB congeners in Qingduizi Bay are presented in Figure 4. Overall, MP and OB showed similar PCB congener compositions in the surface sediment. Light chlorinated congeners, such as tri-PCB and tetra-PCB, were the most abundant congeners in all sampling sites, accounting for 61.5–81.4% (mean: 71.6%) of the total PCBs. As semi-volatile persistent pollutants, the solubility and volatility of PCBs tend to decrease as the number of chlorine atoms increases. Light chlorinated congeners easily volatilize in air and accumulate in the sediment by atmospheric deposition, whereas highly chlorinated congeners are poorly diffused (Carroll et al., 1994). Consequently, high levels of light PCBs were detected in the sediments. The homolog profiles in the present study were consistent with those from other studies of the coastal regions (Liu et al., 2003; Lebeuf and Nunes, 2005; Barhoumi et al., 2014).
Occurrence of OCPs
The distribution of OCPs generally shows a different pattern to that of PCBs in Qingduizi Bay (Figure 3C). The total concentrations of the OCPs vary from 0.44 to 31.27 ng g–1 dw (mean: 3.38 ng g–1 dw) with an average detection frequency of 93.3%. No significant difference was found in the concentrations of OCPs between OB and MP (p = 0.192) (Figure 3D). The proportions of OCP compositions in sediment samples greatly varied: heptachlor, heptachlor epoxide, and γ-chlordane were absent; and α-chlordane, methoxychlor, endosulfan II, cyclodiene pesticides, and their metabolites, including aldrin, dieldrin, endrin and endrin aldehyde, were very low or negligible. Nevertheless, DDTs and their metabolites DDXs (e.g., p,p′-DDD, p,p′-DDE) collectively contributed 54.1–99.6%, and HCHs (α-HCH, β-HCH, γ-HCH, and δ-HCH) collectively contributed 0.1–25.0% of the total OCPs. As abundant and commonly used OCPs, DDTs, and HCHs were detected in all surface sediments of Qingduizi Bay.
The concentrations of DDTs varied from 0.25 to 31.14 ng g–1 dw (mean: 3.13 ng g–1 dw), and the highest level of DDTs was found at site P5 located in the western MP near the mouth of the Inna River (Figure 3E). Overall, the DDT levels showed no significant differences between MP and OB (p = 0.190) (Figure 3F). Among the DDT compounds, p,p′-DDT was the predominant isomer (0.12–29.56 ng g–1 dw), accounting for 33.0–94.6% of all DDTs, whereas p,p′-DDD (0.06–1.91 ng g–1 dw) and p,p′-DDE (0.06–0.22 ng g–1 dw) were present in minimal amounts. When compared with other important bays and estuaries around the world, DDT concentrations in the surface sediments of Qingduizi Bay showed a range of variability. The DDT levels in this study were greater than those of most other Chinese bays and estuaries (Shantou Bay, Shuangtaizi Bay, the Daliao Estuary, the Yangtze Estuary, and other bays in the Eastern Sea) and Guaratuba Bay of Brazil, but were similar to those measured in Bizerte lagoon (Tunisia) and Casco Bay (United States). However, the ranges were more limited than those detected in developed coastal areas, such as the Rhone Prodelta in France (Table 1).
The levels of HCHs showed lower variability among sites (p = 0.569) (Figure 3H). Previous studies reported that 11,400 t of lindane (γ-HCH) were manufactured and used between 1991 and 2000, even after the prohibition on the usage and production of technical HCHs in 1983 (Li et al., 2001). The highest level of HCHs was found at site P1, located in the northwestern MP near the mouth of the Huli River with a concentration of 3.13 ng g–1 dw (Figure 3G). High levels of HCHs with elevated β-HCH fraction were observed in the mouths of the Huli River (P1) and Diyin River (P11 and P12), indicating the input of technical HCH residues to rivers from local agriculture runoff and aquaculture drug usage. The HCH levels in the surface sediment at Qingduizi Bay were similar to those at Casco Bay (United States) but were much lower than those in other estuaries and coastal sediments (Table 1).
Qualitative Analysis for PCBs Sources
The sections located in the western part of the bay showed higher levels of PCBs than that located in the eastern part of the bay, suggesting a possible presence of point sources in the western coast of Qingduizi Bay. The distribution patterns of PCBs could be based on various factors, such as distance from the source(s), hydrodynamic factors and the origin of the OM or clay swelling (Zhou et al., 2012). In the present study, the main point sources of the PCBs could be the Zhuanghe Port and Zhuanghe power plant, which are located on the westernmost shore of Qingduizi Bay. Inputs can probably be attributed to the exchange fluids in the transformers and capacitors from the power plant, as well as the paint additives of ships from the port. Nevertheless, the concentration of PCBs in P3 of MP (2.10 ng g–1 dw) was abnormally higher than those from other MP sites. P3 is speculated to be located near the mouth of Huli River, suggesting direct PCB inputs from the river runoff.
Theoretically, the distributions and levels of hydrophobic pollutants are influenced by the physico-chemical characteristics of sediments. Therefore, it is feasible to analyze the relationship between pollutants and sedimental characteristics to identify the potential sources and occurrences of pollution in estuaries or bays (Wu et al., 2015; Yang et al., 2015). PCBs are mainly derived from waste incineration, accidental fires, equipment/utility usage (e.g., old transformers and capacitors), and atmospheric deposition (Alonso-Hernandez et al., 2014). In the present study, Pearson’s correlation coefficients were used to identify correlations among six sedimental physicochemical parameters and PCB homolog compositions (Figure 5). The results showed that all PCB compounds had weak correlations with physicochemical parameters, indicating that PCBs are more likely to come from atmospheric deposition than surface runoff.
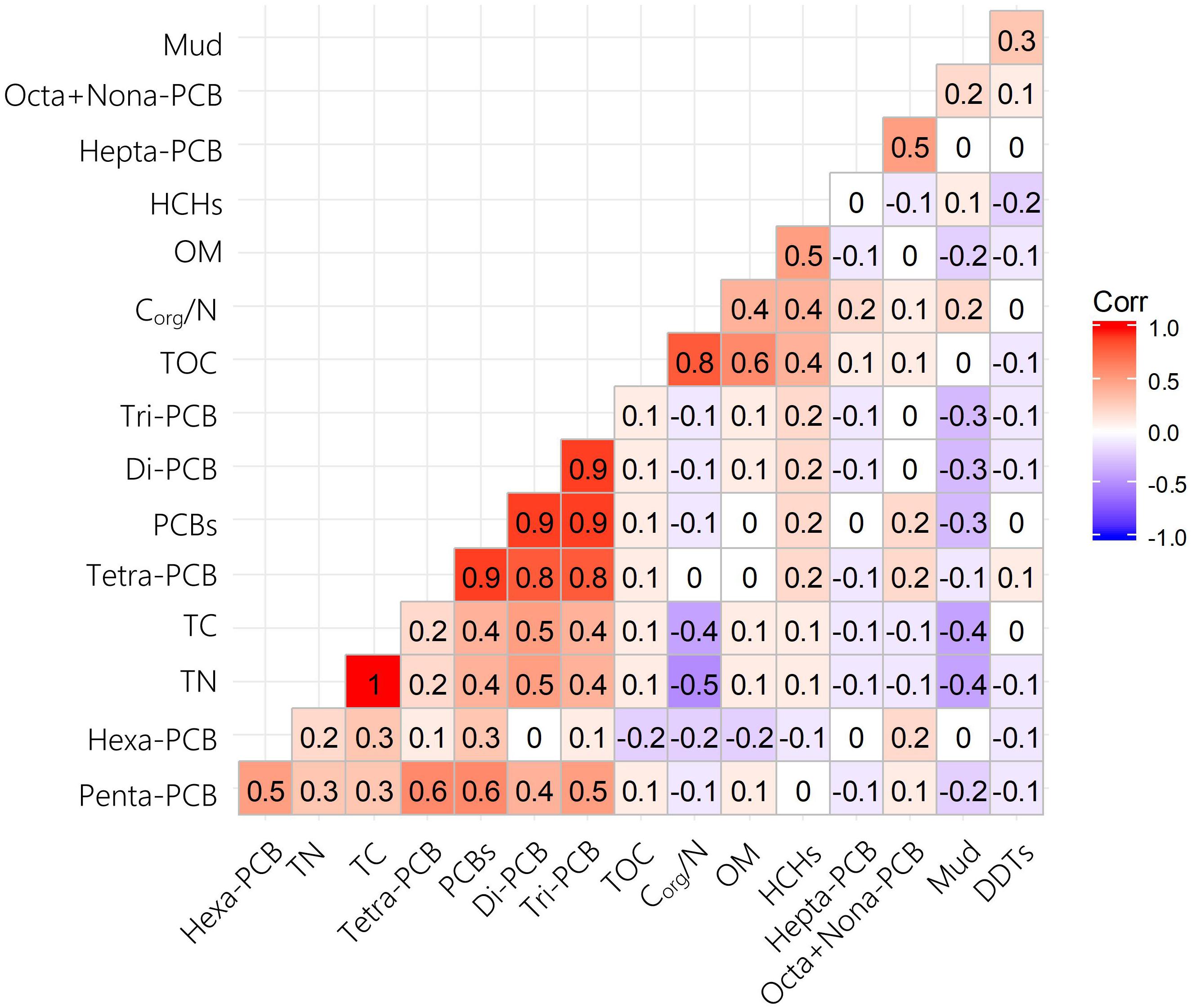
Figure 5. Pearson correlations of PCB homolog compositions and physicochemical characteristics of sediments in Qingduizi Bay.
Principal component analysis was also applied to analyze the potential sources of PCBs. Kaiser–Meyer–Olkin and Bartlett tests yielded 0.63 and 122.66 (df = 105, p < 0.01), respectively, indicating that this method is effective for source apportionment. Two principal components (PCs) were successfully extracted, which revealed the potential sources of PCBs in Qingduizi Bay and explained 67.27% of the total variance (Figure 6). PC1 accounted for 44.68% of the total variance and was mostly composed of light chlorinated congeners, including Di-PCB, Tri-PCB, Tetra-PCB and Penta-PCB, representing a non-point source deposition (e.g., atmospheric deposition and surface runoff). By contrast, PC2 accounted for 22.59%, and was composed of chlorinated congeners, such as Hepta-PCB, Octa-PCB, Nona-PCB and Hexa-PCB, indicating the point source deposition. The results were consistent with the conclusion of the Pearson’s correlation analysis. Due to their characteristics of volatility, light chlorinated congeners volatilize easily to the atmosphere after being released. They are effectively adsorbed by suspended particulate matter and are finally deposited in sediments (Offenberg and Baker, 2002). Therefore, the conclusions of correlation analysis and PCA added credence to the view that light chlorinated congeners are derived from atmospheric deposition. As for highly chlorinated congeners, the existence of a single major source in the bay is possibly related to the point source (e.g., intense use of heat transfer and hydraulic fluids and solvent extenders in ship painting) (Hong et al., 2005; Erickson and Kaley, 2011).
Qualitative Analysis for DDT and HCH Sources
Dichlorodiphenyltrichloroethanes and technical HCH applications for agriculture purposes have been banned since 1983, whereas DDT continued to be used as a synthetic intermediate for the production of dicofol, which was not banned in China until 2018 (Guo et al., 2009; Grung et al., 2015; Yu et al., 2019). Several molecular ratios of selected OCP compounds, such as the abundance ratio of α-HCH/γ-HCH, (DDE + DDD)/DDT and DDE/DDD, were employed to understand their sources and fate in the environment.
Technical HCH contains 60–70% of α-HCH, 5–12% of β-HCH, 10–12% of γ-HCH and 6–10% of δ-HCH (Iwata et al., 1993), with a ratio of α/γ-HCH between 4 and 7 for technical compounds and nearly 0 for pure lindane, and the ratio would increase during the degradation process in the environment (Syed et al., 2014). The ratios of α/γ-HCH in the sediment of Qingduizi Bay varied from 0.88 to 4.29, while the ratios in 93% of sampling sites were below 4, implying that HCH residuals in sediments are mainly derived from the recent lindane usage and historical HCH residuals (Figure 7A). The percentage of β-HCH was used to identify the source of historical usage of HCHs, because the beta isomers are relatively resistant to microbial degradation (Law et al., 2001). The average compositions of β-HCH measured in the sediments ranged from 37 to 77% of the total HCHs, with an average of 48%, implying the mixture contained new and historical inputs of HCHs.
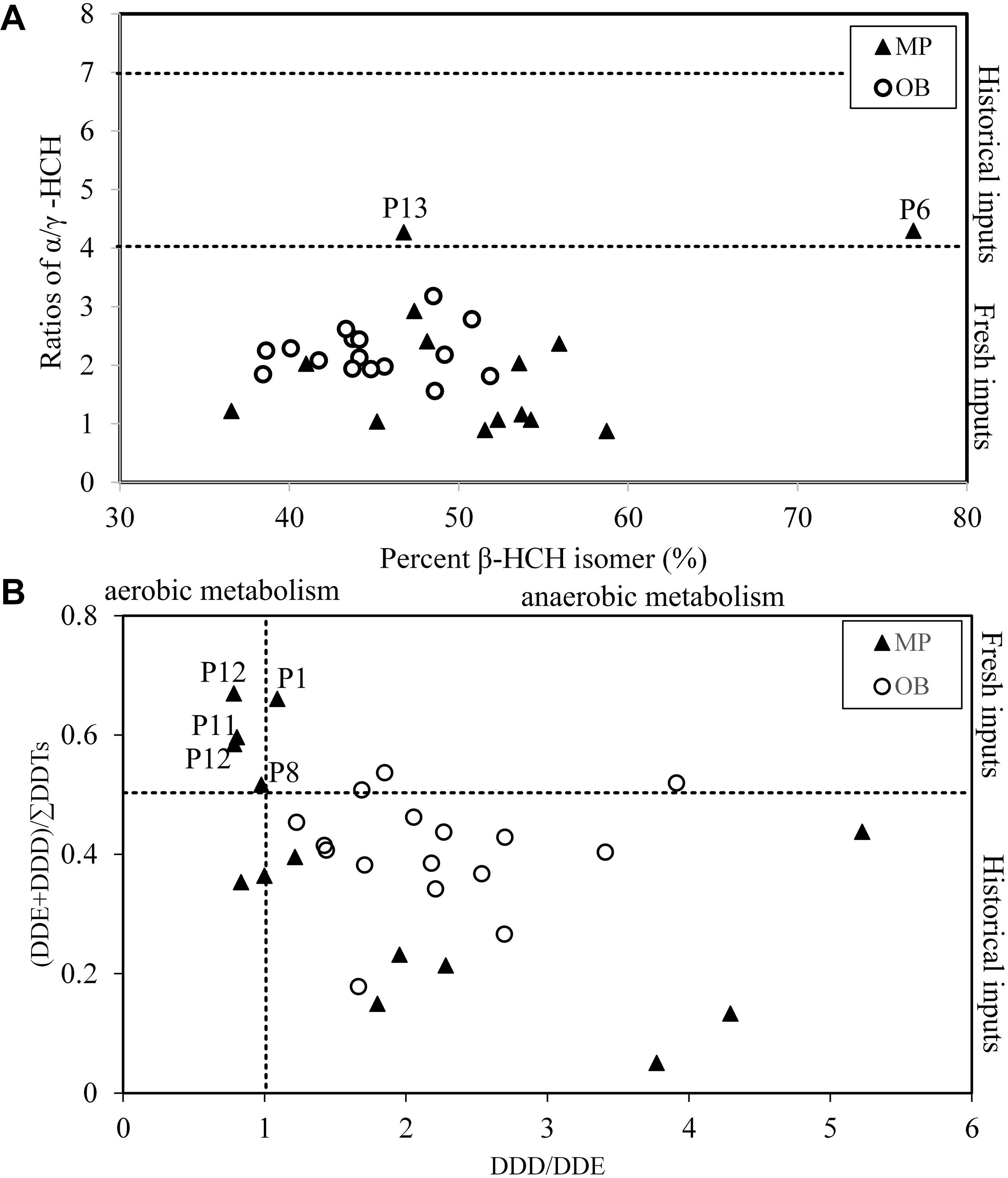
Figure 7. Cross plots for the isomeric ratios of: (A) β-HCH percentage vs. (B) α/γ-HCH and DDD/DDE vs. (DDE + DDD)/ΣDDTs in different samples from Qingduizi Bay.
Generally, p,p′-DDT will be dechlorinated into p,p′-DDE under aerobic conditions and into p,p′-DDD under anaerobic conditions by microbial degradation (Hitch and Day, 1992). DDT sources in the sediments of Qingduizi Bay were identified by plotting p,p′-DDD/DDE against p,p′-(DDE + DDD)/ΣDDT (Figure 7B). Generally, the ratio of p,p′-(DDE + DDD)/ΣDDT < 0.5 indicates a fresh input because most DDTs have not started degrading yet (Hitch and Day, 1992). In the present study, 73% of the sampling sites were characterized by p,p′-(DDE + DDD)/ΣDDT ratios of <0.5, reflecting that new inputs of DDTs were predominant. Specifically, the ratios in several MP sites were less than 0.2, indicating a recent usage of DDTs in aquaculture. However, the ratios in five sites (P1, P4, P8, P9, and P11) were higher than 0.5, implying historical terrestrial usage of DDTs since these sites were located near the mouths of rivers. The ratios of p,p′-DDD/DDE in all sites of OB and most sites of MP were greater than 1, indicating the occurrence of a strong anaerobic transformation of DDT in the sediments of Qingduizi Bay.
Pesticide usage in agriculture and aquaculture is commonly known as one of the primary contributors to persistent halogenated compounds (PHCs) (Yu et al., 2011). Thus, PHCs that originate from mariculture should not be ignored, especially in intensive pond culture areas. The marine pollution derived from the rapid development of mariculture has elicited worldwide concern. Previous studies have frequently detected POPs, especially DDTs, in fishery feed (e.g., compound feed and trash fish) (Hites et al., 2004; Minh et al., 2006). The total areas of marine pond culture have been found to reach approximately 265,513 hectares, and more than 1.2 × 105 metric tons of unused feed were directly discharged into the sea without treatment (Cao et al., 2007). Therefore, pond culture activities could be a main contributor of DDTs in the coastal environment and organisms, as further confirmed by Yu et al. (2011).
Risk Assessment of PCBs and OCPs in the Sediment of Qingduizi Bay
Exogenic organic pollutants tend to accumulate in fatty tissue and are toxic to aquatic organisms because they are lipophilic (Zhu et al., 2015). The evaluation of potential ecotoxicological risks of POPs in estuarine and coastal areas has raised substantial concerns (Tolosa et al., 1995; Yuan et al., 2017). Ecological risk assessment is a useful tool to analyze and evaluate the adverse ecological effects caused by environmental contaminants. However, no specific guidelines are currently available for POPs in sediments in China. Based on the SQGs established by the United States Environmental Protection Agency (Usepa, 1998) and Canada (Ccme, 2002), the potential risks of PCBs and OCPs in the sediments from Qingduizi Bay were assessed (Table 2).
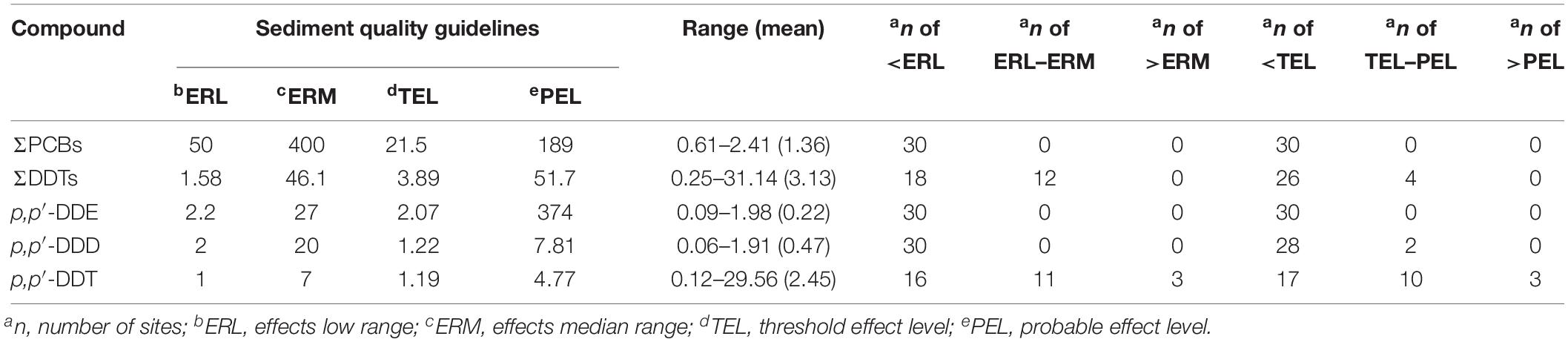
Table 2. Concentrations of PCB and OCP in surface sediments from Qingduizi Bay (ng g–1 dw) and toxicity guidelines.
The present data and the guidelines were compared. The concentrations of PCBs and p,p′-DDE were all below the ERL and TEL values, suggesting that adverse biological effects would rarely occur for those compounds. As for p,p′-DDD, its concentrations in most sites (93.3% of total sites) were below TEL, indicating rather low ecotoxicity to aquatic organisms. Among all organic pollutants, p,p′-DDT and ΣDDTs were the most serious pollutants, and their levels in site 11 and site 12 respectively were above the ERL values. Even the levels of p,p′-DDT in three samples of MP were higher than ERM and PEL values, suggesting that adverse effects of p,p′-DDT and ΣDDTs were expected to occur in Qingduizi Bay.
Conclusion
Comprehensive surveys and experimental analyses of PCBs and OCPs in the sediments of Qingduizi Bay, a typical developing pond culture bay located in the northernmost part of the Yellow Sea of China, were conducted in this study. The results showed significant occurrences and levels of PCBs between MP and OB, whereas there were no significant differences of OCPs between MP and OB. Overall, PCBs and OCPs in the sediments have low to moderate levels without consideration of HCHs and DDTs. The inputs of PCBs could be attributed to the extensive historical application in adjacent areas, such as power plants and ship ports, whereas HCHs, which could be preserved in agricultural soils released via major river runoffs into the sea, could result mainly from historical applications. Nevertheless, DDTs showed recent usage and fresh inputs from aquaculture activities due to the high concentrations of p,p′-DDT in MP. Intensive mariculture activities could influence the levels and distributions of organic contaminants, especially for compounds contained in feed and pesticides. In addition, concerns should be raised regarding DDTs because the levels of several sites were above the SQGs and may cause ecotoxicological risks to marine organisms.
Author Contributions
XlY, XtY, and WH conceptualized and executed the project. AZ, CG, and HZ collected the sediment samples. XL, XlY, MS, and LQ performed the experiments and conducted the laboratory analyses. XlY, LW, and GB performed the statistical analysis, interpretation of data, and the manuscript writing. XtY, WH, and GB edited and critically reviewed the final version of the manuscript which all the authors approved before submission.
Funding
This study was funded by the National Natural Science Foundation of China (Grant No. 41106115) and National Marine Public Welfare Research Projects (Grant Nos. 201305043 and 201105006).
Conflict of Interest
The authors declare that the research was conducted in the absence of any commercial or financial relationships that could be construed as a potential conflict of interest.
Acknowledgments
The authors express their gratitude to all the volunteers who participated in the field and laboratory work.
Supplementary Material
The Supplementary Material for this article can be found online at: https://www.frontiersin.org/articles/10.3389/fmars.2019.00602/full#supplementary-material
References
Adeleye, A. O., Jin, H., Di, Y., Li, D., Chen, J., and Ye, Y. (2016). Distribution and ecological risk of organic pollutants in the sediments and seafood of Yangtze estuary and hangzhou bay. East China Sea. Sci. Total Environ. 541, 1540–1548. doi: 10.1016/j.scitotenv.2015.09.124
Alonso-Hernandez, C., Mesa-Albernas, M., and Tolosa, I. (2014). Organochlorine pesticides (OCPs) and polychlorinated biphenyls (PCBs) in sediments from the gulf of batabanó. Cuba. Chemosphere 94, 36–41. doi: 10.1016/j.marpolbul.2012.09.008
Bao, L.-J., Maruya, K. A., Snyder, S. A., and Zeng, E. Y. (2012). China’s water pollution by persistent organic pollutants. Environ. Pollut. 163, 100–108. doi: 10.1016/j.envpol.2011.12.022
Barbier, E. B., Hacker, S. D., Kennedy, C., Koch, E. W., Stier, A. C., and Silliman, B. R. (2011). The value of estuarine and coastal ecosystem services. Ecol. Monogr. 81, 169–193.
Barhoumi, B., LeMenach, K., Dévier, M.-H., Hammami, B., Ameur, W. B., Hassine, S. B., et al. (2014). Distribution and ecological risk of polychlorinated biphenyls (PCBs) and organochlorine pesticides (OCPs) in surface sediments from the Bizerte lagoon, Tunisia. Environ. Sci. Pollut. Res. 21, 6290–6302. doi: 10.1007/s11356-013-1709-7
Bröder, L., Tesi, T., Salvadó, J. A., Semiletov, I. P., Dudarev, O. V., and Gustafsson, Ö (2016). Fate of terrigenous organic matter across the laptev sea from the mouth of the lena river to the deep sea of the arctic interior. Biogeosciences 13, 5003–5019. doi: 10.5194/bg-13-5003-2016
Cao, L., Naylor, R., Henriksson, P., Leadbitter, D., Metian, M., Troell, M., et al. (2015). China’s aquaculture and the world’s wild fisheries. Science 347, 133–135.
Cao, L., Wang, W., Yang, Y., Yang, C., Yuan, Z., Xiong, S., et al. (2007). Environmental impact of aquaculture and countermeasures to aquaculture pollution in China. Environ. Sci. Pollut. Res. 14, 452–462. doi: 10.1065/espr2007.05.426
Carroll, K. M., Harkness, M. R., Bracco, A. A., and Balcarcel, R. R. (1994). Application of a permeant/polymer diffusional model to the desorption of polychlorinated biphenyls from Hudson River sediments. Environ. Sci. Technol. 28, 253–258. doi: 10.1021/es00051a011
Ccme. (2002). Canadian Sediment Quality Guidelines for the Protection of Aquatic Life. Winnipeg: CCME.
Combi, T., Taniguchi, S., Figueira, R. C. L., Mahiques, M. M. D., and Martins, C. C. (2013). Spatial distribution and historical input of polychlorinated biphenyls (PCBs) and organochlorine pesticides (OCPs) in sediments from a subtropical estuary (Guaratuba Bay. SW Atlantic). Mar. Pollut. Bull. 70, 247–252. doi: 10.1016/j.marpolbul.2013.02.022
Craft, C., Seneca, E., and Broome, S. (1991). Loss on ignition and kjeldahl digestion for estimating organic carbon and total nitrogen in estuarine marsh soils: calibration with dry combustion. Estuaries 14, 175–179.
Erickson, M. D., and Kaley, R. G. (2011). Applications of polychlorinated biphenyls. Environ. Sci. Pollut. Res. 18, 135–151.
Gao, S., Chen, J., Shen, Z., Liu, H., and Chen, Y. (2013). Seasonal and spatial distributions and possible sources of polychlorinated biphenyls in surface sediments of Yangtze Estuary. China. Chemosphere 91, 809–816. doi: 10.1016/j.chemosphere.2013.01.085
Grung, M., Lin, Y., Zhang, H., Steen, A. O., Huang, J., Zhang, G., et al. (2015). Pesticide levels and environmental risk in aquatic environments in China—A review. Environ. Int. 81, 87–97. doi: 10.1016/j.envint.2015.04.013
Gui, D., Yu, R., He, X., Tu, Q., Chen, L., and Wu, Y. (2014). Bioaccumulation and biomagnification of persistent organic pollutants in indo-pacific humpback dolphins (Sousa chinensis) from the Pearl River Estuary. China. Chemosphere 114, 106–113. doi: 10.1016/j.chemosphere.2014.04.028
Guo, Y., Yu, H.-Y., and Zeng, E. Y. (2009). Occurrence, source diagnosis, and biological effect assessment of DDT and its metabolites in various environmental compartments of the Pearl River Delta. South China: a review. Environ. Pollut. 157, 1753–1763. doi: 10.1016/j.envpol.2008.12.026
Hitch, R. K., and Day, H. R. (1992). Unusual persistence of DDT in some western USA soils. Bull. Environ. Contam. Toxicol. 48, 259–264.
Hites, R. A., Foran, J. A., Carpenter, D. O., Hamilton, M. C., Knuth, B. A., and Schwager, S. J. (2004). Global assessment of organic contaminants in farmed salmon. Science 303, 226–229. doi: 10.1126/science.1091447
Hong, S. H., Yim, U. H., Shim, W. J., and Oh, J. R. (2005). Congener-specific survey for polychlorinated biphenlys in sediments of industrialized bays in Korea: regional characteristics and pollution sources. Environ. Sci. Technol. 39, 7380–7388. doi: 10.1021/es050397c
Ishikawa, Y., Noma, Y., Mori, Y., and Sakai, S. I (2007). Congener profiles of PCB and a proposed new set of indicator congeners. Chemosphere 67, 1838–1851. doi: 10.1016/j.chemosphere.2006.05.075
Islam, M. S., and Tanaka, M. (2004). Impacts of pollution on coastal and marine ecosystems including coastal and marine fisheries and approach for management: a review and synthesis. Mar. Pollut. Bull. 48, 624–649. doi: 10.1016/j.marpolbul.2003.12.004
Iwata, H., Tanabe, S., Sakai, N., and Tatsukawa, R. (1993). Distribution of persistent organochlorines in the oceanic air and surface seawater and the role of ocean on their global transport and fate. Environ. Sci. Technol. 27, 1080–1098. doi: 10.1021/es00043a007
Kennicutt, M. C., Wade, T. L., Presley, B. J., Requejo, A. G., Brooks, J. M., and Denoux, G. J. (1994). Sediment contaminants in casco bay, maine: inventories, sources, and potential for biological impact. Environ. Sci. Technol. 28, 1–15. doi: 10.1021/es00050a003
Koziorowska, K., Kuliński, K., and Pempkowiak, J. (2016). Sedimentary organic matter in two Spitsbergen fjords: terrestrial and marine contributions based on carbon and nitrogen contents and stable isotopes composition. Cont. Shelf Res. 113, 38–46. doi: 10.1016/j.csr.2015.11.010
Law, S. A., Diamond, M. L., Helm, P. A., Jantunen, L. M., and Alaee, M. (2001). Factors affecting the occurrence and enantiomeric degradation of hexachlorocyclohexane isomers in northern and temperate aquatic systems. Environ. Toxicol. Chemis. Int. J. 20, 2690–2698. doi: 10.1002/etc.5620201206
Lebeuf, M., and Nunes, T. (2005). PCBs and OCPs in sediment cores from the lower St. lawrence estuary, canada: evidence of fluvial inputs and time lag in delivery to coring sites. Environ. Sci. Technol. 39, 1470–1478. doi: 10.1021/es049051c
Li, Y., Cai, D., Shan, Z., and Zhu, Z. (2001). Gridded usage inventories of technical hexachlorocyclohexane and lindane for China with 1/6 latitude by 1/4 longitude resolution. Arch. Environ. Contam. Toxicol. 41, 261–266. doi: 10.1007/s002440010247
Li, Z., Lin, T., Li, Y., Jiang, Y., and Guo, Z. (2017). Atmospheric deposition and air-sea gas exchange fluxes of DDT and HCH in the Yangtze River Estuary, East China Sea. J. Geophys. Res. Atmos. 122, 7664–7677. doi: 10.1002/2016jd026330
Liu, G., Yuan, X., Guan, C., Luo, H., and Gu, Y. (2018). Influence of reclamation on off-shore macrobenthic community in the qingduizi bay. Mar. Environ. Sci. 663–669.
Liu, M., Cheng, S., Ou, D., Yang, Y., Liu, H., Hou, L., et al. (2008). Organochlorine pesticides in surface sediments and suspended particulate matters from the Yangtze Estuary. China. Environ. Pollut. 156, 168–173. doi: 10.1016/j.envpol.2007.12.015
Liu, M., Yang, Y., Hou, L.-J., Xu, S., Ou, D., Zhang, B., et al. (2003). Chlorinated organic contaminants in surface sediments from the Yangtze Estuary and nearby coastal areas. China. Mar. Pollut. Bull. 46, 672–676. doi: 10.1016/s0025-326x(03)00050-x
Long, E. R., Field, L. J., and MacDonald, D. D. (1998). Predicting toxicity in marine sediments with numerical sediment quality guidelines. Environ. Toxicol. Chemis. Int. J. 17, 714–727. doi: 10.1002/etc.5620170428
Long, E. R., Macdonald, D. D., Smith, S. L., and Calder, F. D. (1995). Incidence of adverse biological effects within ranges of chemical concentrations in marine and estuarine sediments. Environ. Manag. 19, 81–97. doi: 10.1007/bf02472006
Men, B., He, M., Tan, L., and Lin, C. (2014). Distributions of polychlorinated biphenyls in the Daliao River estuary of Liaodong Bay. Bohai Sea (China). Mar. Pollut. Bull. 78, 77–84. doi: 10.1016/j.marpolbul.2013.11.005
Meyers, P. A. (1994). Preservation of elemental and isotopic source identification of sedimentary organic matter. Chem. Geol. 144, 289–302. doi: 10.1016/0009-2541(94)90059-0
Miao, L. J., Yu, Y. H., Guan, C. J., Lin, X., Kang, J., and Wei, Q. F. (2014). Application of opportunity cost method in the determination of marine ecological compensation standard - a case study of Zhuanghe Qingdui bay area. Ocean Dev. Manag. 5, 21–25.
Miglioranza, K. S., de Moreno, J. E., and Moreno, V. C. J. (2004). Organochlorine pesticides sequestered in the aquatic macrophyte Schoenoplectus californicus (CA Meyer) Sojak from a shallow lake in Argentina. Water Res. 38, 1765–1772. doi: 10.1016/j.watres.2004.01.017
Minh, N. H., Minh, T. B., Kajiwara, N., Kunisue, T., Iwata, H., Viet, P. H., et al. (2006). Contamination by polybrominated diphenyl ethers and persistent organochlorines in catfish and feed from Mekong River Delta, Vietnam. Environ. Toxicol. Chem. Int. J. 25, 2700–2708.
Montuori, P., Aurino, S., Garzonio, F., and Triassi, M. (2016). Polychlorinated biphenyls and organochlorine pesticides in Tiber River and Estuary: occurrence, distribution and ecological risk. Sci. Total Environ. 571, 1001–1016. doi: 10.1016/j.scitotenv.2016.07.089
Offenberg, J. H., and Baker, J. E. (2002). Precipitation scavenging of polychlorinated biphenyls and polycyclic aromatic hydrocarbons along an urban to over-water transect. Environ. Sci. Technol. 36, 3763–3771. doi: 10.1021/es025608h
Okay, O., Karacık, B., Başak, S., Henkelmann, B., Bernhöft, S., and Schramm, K.-W. (2009). PCB and PCDD/F in sediments and mussels of the Istanbul strait (Turkey). Chemosphere 76, 159–166. doi: 10.1016/j.chemosphere.2009.03.051
Primavera, J. (2006). Overcoming the impacts of aquaculture on the coastal zone. Ocean Coastal Manag. 49, 531–545. doi: 10.1016/j.ocecoaman.2006.06.018
R Core Team. (2016). R: A Language and Environment for Statistical Computing. R Foundation for Statistical Computing. Vienna: R Core Team
Ricciardi, A., and Atkinson, S. K. (2004). Distinctiveness magnifies the impact of biological invaders in aquatic ecosystems. Ecol. Lett. 7, 781–784. doi: 10.1111/j.1461-0248.2004.00642.x
Richter, B. E., Jones, B. A., Ezzell, J. L., Porter, N. L., Avdalovic, N., and Pohl, C. (1996). Accelerated solvent extraction: a technique for sample preparation. Anal. Chem. 68, 1033–1039. doi: 10.1021/ac9508199
Sharma, B. M., Bharat, G. K., Tayal, S., Nizzetto, L., Čupr, P., and Larssen, T. (2014). Environment and human exposure to persistent organic pollutants (POPs) in India: a systematic review of recent and historical data. Environ. Int. 66, 48–64. doi: 10.1016/j.envint.2014.01.022
Shi, J., Li, P., Li, Y., Liu, W., Zheng, G. J., Xiang, L., et al. (2016). Polychlorinated biphenyls and organochlorine pesticides in surface sediments from Shantou Bay, China: sources, seasonal variations and inventories. Mar. Pollut. Bull. 113, 585–591. doi: 10.1016/j.marpolbul.2016.09.006
Soares, H., Boaventura, R., Machado, A., and Da Silva, J. E. (1999). Sediments as monitors of heavy metal contamination in the Ave river basin (Portugal): multivariate analysis of data. Environ. Pollut. 105, 311–323. doi: 10.1016/s0269-7491(99)00048-2
Syed, J. H., Malik, R. N., Li, J., Chaemfa, C., Zhang, G., and Jones, K. C. (2014). Status, distribution and ecological risk of organochlorines (OCs) in the surface sediments from the Ravi River. Pakistan. Sci. Total Environ. 472, 204–211. doi: 10.1016/j.scitotenv.2013.10.109
Tolosa, I., Bayona, J. M., and Albaiges, J. (1995). Spatial and temporal distribution, fluxes, and budgets of organochlorinated compounds in Northwest Mediterranean sediments. Environ. Sci. Technol. 29, 2519–2527. doi: 10.1021/es00010a010
Tsygankov, V. Y., Boyarova, M. D., and Lukyanova, O. N. (2015). Bioaccumulation of persistent organochlorine pesticides (OCPs) by gray whale and Pacific walrus from the western part of the Bering Sea. Mar. Pollut. Bull. 99, 235–239. doi: 10.1016/j.marpolbul.2015.07.020
Usepa. (1998). Guidelines for Ecological Risk Assessment EPA/630/R-95/002F USEPA. Washington, DC: USEPA.
Wang, H., He, M., Lin, C., Quan, X., Guo, W., and Yang, Z. (2007). Monitoring and assessment of persistent organochlorine residues in sediments from the Daliaohe River Watershed. Northeast of China. Environ. Monit. Assess. 133, 231–242. doi: 10.1007/s10661-006-9576-z
Wang, J., Tan, L., Zhang, W., and Lian, Z. (2010). Concentrations and distribution characteristic of PAHs, PCBs and OCPs in the surface sediments of Qingtao coastal area. Environ. Sci. 31, 2713–2722.
Wang, L., Yang, X., Zhang, A., Bidegain, G., Li, R., Na, G., et al. (2019). Distribution patterns and ecological risk of endocrine-disrupting chemicals at Qingduizi Bay (China): a preliminary survey in a developing maricultured bay. Mar. Pollut. Bull. 146, 915–920. doi: 10.1016/j.marpolbul.2019.07.026
Wang, T., Lu, Y., Zhang, H., and Shi, Y. (2005). Contamination of persistent organic pollutants (POPs) and relevant management in China. Environ. Int. 31, 813–821. doi: 10.1016/j.envint.2005.05.043
Wei, D., Kameya, T., and Urano, K. (2007). Environmental management of pesticidal POPs in China: past, present and future. Environ. Int. 33, 894–902. doi: 10.1016/j.envint.2007.04.006
Wu, J., Liu, G., Wang, N., and Li, A. (2011). Water quality and eutrophication in Qingduizi Bay from 2008 to 2009. Fish. Sci. 30, 708–710.
Wu, Q., Leung, J. Y., Yuan, X., Huang, X., Li, H., Huang, Z., et al. (2015). Biological risk, source and pollution history of organochlorine pesticides (OCPs) in the sediment in Nansha mangrove. South China. Mar. Pollut. Bull. 96, 57–64. doi: 10.1016/j.marpolbul.2015.05.047
Yang, H., Xue, B., Jin, L., Zhou, S., and Liu, W. (2011). Polychlorinated biphenyls in surface sediments of Yueqing Bay, Xiangshan Bay, and Sanmen Bay in East China Sea. Chemosphere 83, 137–143. doi: 10.1016/j.chemosphere.2010.12.070
Yang, X., Yuan, X., Zhang, A., Mao, Y., Li, Q., Zong, H., et al. (2015). Spatial distribution and sources of heavy metals and petroleum hydrocarbon in the sand flats of Shuangtaizi Estuary, Bohai Sea of China. Mar. Pollut. Bull. 95, 503–512. doi: 10.1016/j.marpolbul.2015.02.042
Yang, X., Zhang, P., Li, W., Hu, C., Zhang, X., and He, P. (2018). Evaluation of four seagrass species as early warning indicators for nitrogen overloading: Implications for eutrophic evaluation and ecosystem management. Sci. Total Environ. 635, 1132–1143. doi: 10.1016/j.scitotenv.2018.04.227
Yin, J. (2013). The Causation Cognizance and Assessment of Marine Ecological Damage for Coastal Reclamation Aquaculture - a Case Study in Qingduizi Bay [M]. Dalian: Dalian Ocean University.
Yu, H.-Y., Zhang, B.-Z., Giesy, J. P., and Zeng, E. Y. (2011). Persistent halogenated compounds in aquaculture environments of South China: implications for global consumers’ health risk via fish consumption. Environ. Int. 37, 1190–1195. doi: 10.1016/j.envint.2011.04.012
Yu, S., Liu, W., Xu, Y., Zhao, Y., Cai, C., Liu, Y., et al. (2019). Organochlorine pesticides in ambient air from the littoral cities of northern China: spatial distribution, seasonal variation, source apportionment and cancer risk assessment. Sci. Total Environ. 652, 163–176. doi: 10.1016/j.scitotenv.2018.10.230
Yu, Y., Li, Y., Shen, Z., Yang, Z., Mo, L., Kong, Y., et al. (2014). Occurrence and possible sources of organochlorine pesticides (OCPs) and polychlorinated biphenyls (PCBs) along the Chao River. China. Chemosphere 114, 136–143. doi: 10.1016/j.chemosphere.2014.03.095
Yuan, X., Yang, X., Na, G., Zhang, A., Mao, Y., Liu, G., et al. (2015). Polychlorinated biphenyls and organochlorine pesticides in surface sediments from the sand flats of Shuangtaizi Estuary, China: levels, distribution, and possible sources. Environ. Sci. Pollut. Res. 22, 14337–14348. doi: 10.1007/s11356-015-4688-z
Yuan, X., Yang, X., Zhang, A., Ma, X., Gao, H., Na, G., et al. (2017). Distribution, potential sources and ecological risks of two persistent organic pollutants in the intertidal sediment at the Shuangtaizi Estuary. Bohai Sea of China. Mar. Pollut. Bull. 114, 419–427. doi: 10.1016/j.marpolbul.2016.09.058
Zhao, D., Zhang, P., Ge, L., Zheng, G. J., Wang, X., Liu, W., et al. (2018). The legacy of organochlorinated pesticides (OCPs), polycyclic aromatic hydrocarbons (PAHs) and polychlorinated biphenyls (PCBs) in Chinese coastal seawater monitored by semi-permeable membrane devices (SPMDs). Mar. Pollut. Bull. 137, 222–230. doi: 10.1016/j.marpolbul.2018.10.004
Zhou, S.-S., Shao, L.-Y., Yang, H.-Y., Wang, C., and Liu, W.-P. (2012). Residues and sources recognition of polychlorinated biphenyls in surface sediments of Jiaojiang Estuary. East China Sea. Mar. Pollut. Bull. 64, 539–545. doi: 10.1016/j.marpolbul.2011.12.023
Zhu, C., Wang, P., Li, Y., Chen, Z., Li, W., Ssebugere, P., et al. (2015). Bioconcentration and trophic transfer of polychlorinated biphenyls and polychlorinated dibenzo-p-dioxins and dibenzofurans in aquatic animals from an e-waste dismantling area in East China. Environ. Sci. Process. Impacts 17, 693–699. doi: 10.1039/c5em00028a
Keywords: persistent organic pollutants, pond mariculture, sea reclamation, sediment, Northern Yellow Sea, ecological impact
Citation: Yang X, Wang L, Zhang A, Liu X, Bidegain G, Zong H, Guan C, Song M, Qu L, Huang W and Yuan X (2019) Levels, Sources and Potential Risks of Polychlorinated Biphenyls and Organochlorine Pesticides in Sediments of Qingduizi Bay, China: Does Developing Mariculture Matter? Front. Mar. Sci. 6:602. doi: 10.3389/fmars.2019.00602
Received: 28 December 2018; Accepted: 11 September 2019;
Published: 25 September 2019.
Edited by:
Xiaoshou Liu, Ocean University of China, ChinaReviewed by:
Yuxin Sun, South China Sea Institute of Oceanology (CAS), ChinaWenzhe Xu, Tianjin University of Science and Technology, China
Copyright © 2019 Yang, Wang, Zhang, Liu, Bidegain, Zong, Guan, Song, Qu, Huang and Yuan. This is an open-access article distributed under the terms of the Creative Commons Attribution License (CC BY). The use, distribution or reproduction in other forums is permitted, provided the original author(s) and the copyright owner(s) are credited and that the original publication in this journal is cited, in accordance with accepted academic practice. No use, distribution or reproduction is permitted which does not comply with these terms.
*Correspondence: Wei Huang, willhuang@sio.org.cn; Xiutang Yuan, xtyuan@nmemc.org.cn