- 1CORDIO East Africa, Global Coral Reef Monitoring Network, Marine Biodiversity Observation Network, Global Ocean Observing System Biology and Ecosystems Panel, Mombasa, Kenya
- 2Department of Biological and Environmental Sciences, Qatar University, Doha, Qatar
- 3Cooperative Institute of Marine and Atmospheric Sciences (CIMAS) and National Oceanic and Atmospheric Administration (NOAA), Miami, FL, United States
- 4Intergovernmental Oceanographic Commission of UNESCO, Intergovernmental Oceanographic Commission (IOC) Project Office for International Oceanographic Data and Information Exchange (IODE), Oostende, Belgium
- 5Commonwealth Scientific and Industrial Research Organisation, Oceans and Atmosphere, Hobart, TAS, Australia
- 6Institute for Marine and Antarctic Studies, University of Tasmania, Hobart, TAS, Australia
- 7National Oceanic and Atmospheric Administration (NOAA), La Jolla, CA, United States
- 8Pacific Islands Fisheries Science Center, U.S. National Oceanic and Atmospheric Administration, Honolulu, HI, United States
- 9National University of Singapore, Singapore, Singapore
- 10Environmental Science Department, Broward College, Gainesville, FL, United States
- 11Biosciences, College of Life and Environmental Sciences, University of Exeter, Exeter, United Kingdom
- 12Australian Institute of Marine Science, Perth, WA, Australia
- 13CORDIO East Africa, Global Coral Reef Monitoring Network (Western Indian Ocean), Mombasa, Kenya
- 14Environmental Moorings International, Inc., Key Largo, FL, United States
- 15Atlantic Oceanographic and Meteorological Laboratory, National Oceanic and Atmospheric Administration, Miami, FL, United States
- 16Reef Check Foundation, Marina Del Rey, CA, United States
- 17Caribbean Community Climate Change Center, Belmopan, Belize
- 18Japan Wildlife Research Center, Tokya, Japan
- 19Applied Research Laboratory, University of Hawaii at Mānoa, Honolulu, HI, United States
- 20Departamento de Estudios Ambientales, Universidad Simón Bolívar, Caracas, Venezuela
- 21Institute for Marine Remote Sensing/IMaRS, University of South Florida, St. Petersburg, FL, United States
- 22CORDIO East Africa, Mombasa, Kenya
- 23National Parks Board, Singapore, Singapore
- 24Socioeconomic Monitoring for Coastal Management for the Pacific Islands (SOCMON/SEM-Pasifika), NOAA, and Joint Institute of Marine and Atmospheric Research, University of Hawaii, Honolulu, HI, United States
Coral reefs are exceptionally biodiverse and human dependence on their ecosystem services is high. Reefs experience significant direct and indirect anthropogenic pressures, and provide a sensitive indicator of coastal ocean health, climate change, and ocean acidification, with associated implications for society. Monitoring coral reef status and trends is essential to better inform science, management and policy, but the projected collapse of reef systems within a few decades makes the provision of accurate and actionable monitoring data urgent. The Global Coral Reef Monitoring Network has been the foundation for global reporting on coral reefs for two decades, and is entering into a new phase with improved operational and data standards incorporating the Essential Ocean Variables (EOVs) (www.goosocean.org/eov) and Framework for Ocean Observing developed by the Global Ocean Observing System. Three EOVs provide a robust description of reef health: hard coral cover and composition, macro-algal canopy cover, and fish diversity and abundance. A data quality model based on comprehensive metadata has been designed to facilitate maximum global coverage of coral reef data, and tangible steps to track capacity building. Improved monitoring of events such as mass bleaching and disease outbreaks, citizen science, and socio-economic monitoring have the potential to greatly improve the relevance of monitoring to managers and stakeholders, and to address the complex and multi- dimensional interactions between reefs and people. A new generation of autonomous vehicles (underwater, surface, and aerial) and satellites are set to revolutionize and vastly expand our understanding of coral reefs. Promising approaches include Structure from Motion image processing, and acoustic techniques. Across all systems, curation of data in linked and open online databases, with an open data culture to maximize benefits from data integration, and empowering users to take action, are priorities. Action in the next decade will be essential to mitigate the impacts on coral reefs from warming temperatures, through local management and informing national and international obligations, particularly in the context of the Sustainable Development Goals, climate action, and the role of coral reefs as a global indicator. Mobilizing data to help drive the needed behavior change is a top priority for coral reef observing systems.
Introduction
The Importance of Coral Reefs
Coral reefs are exceptionally biodiverse and productive (Snelgrove et al., 2016). They are the most species-rich marine ecosystem, and play a role in the life cycle of one quarter of all marine fish species (Burke et al., 2008; Knowlton et al., 2010). Flanking tropical coastlines and islands, they play a unique role in the lives of coastal and island peoples, communities, and countries, and are an immensely valuable asset from local to national levels (Burke et al., 2011; Wilkinson et al., 2016; Beck et al., 2018). Their productivity supports the livelihoods and food security of more than half a billion people in more than 100 countries, and they are a trillion-dollar economic asset (Hoegh-Guldberg, 2015). The growing global awareness of the value of coral reefs is reflected in citations (Table 1) and their importance in global policy and assessment circles (Table 2).
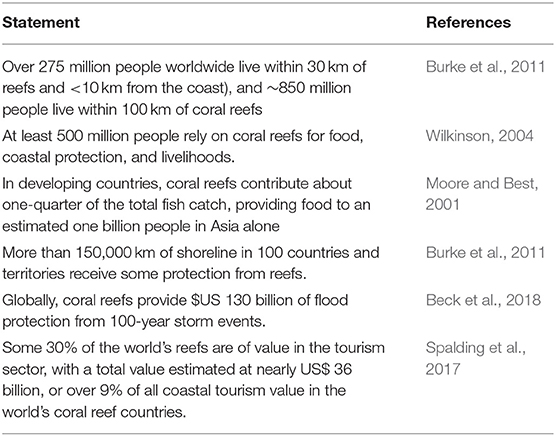
Table 1. Values of coral reef ecosystem services quantified in the literature, from a global perspective.
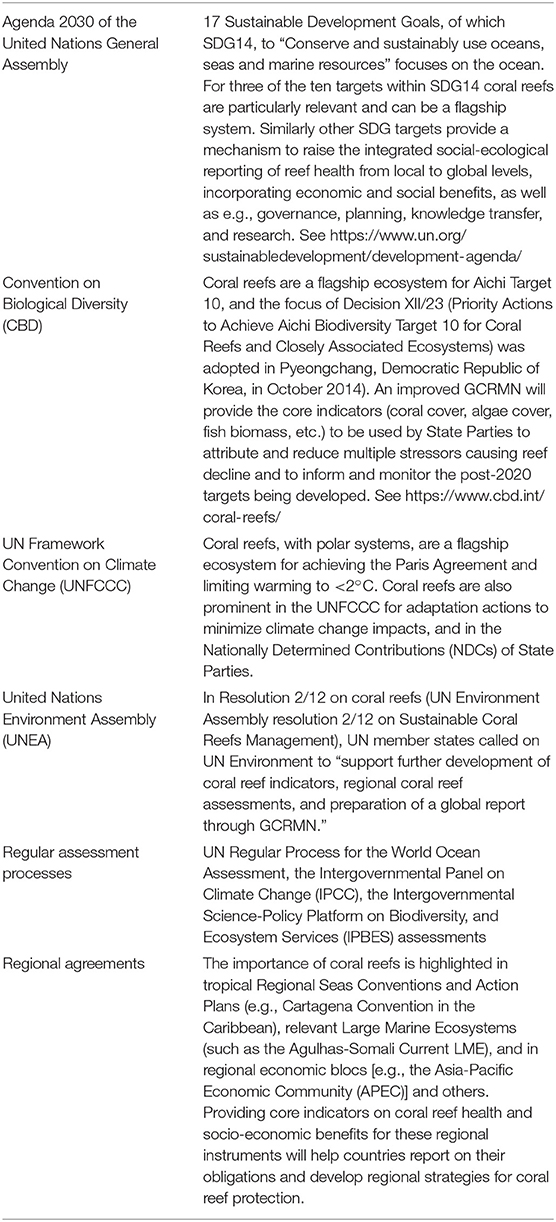
Table 2. Global and regional instruments for which coral reefs are an important and headline ecosystem.
Long-term monitoring and integrated ecosystem observations of coral reefs can provide critical data that help coastal residents and coastal and marine managing authorities understand the health of the reefs, their dependence on their health and well-being, and the economic and social consequences of reef decline. Thus, the societal need and justification for coral reef monitoring at local levels are high, and requires thoughtful design of integrated monitoring and observing systems to address local social-ecological dynamics, key threats, and socio-economic dimensions. At the same time, the ability of aggregate data from local up to the global level is equally important, to feed into the scale of governance processes that can influence global trajectories of long term sustainability of coral reefs (Table 2).
Vulnerability of Coral Reefs to Local to Global Threats
Coral reefs are under significant direct pressure from human activities in the form of fishing, pollution, recreation, transport and coastal development, and are especially vulnerable to the global threats of ocean warming and acidification (Burke et al., 2011; Hughes et al., 2017). These result in coral stress and mortality, shifts in ecological interactions (including through loss of consumer functional groups) favoring algae and faster-growing invertebrates over hard corals, and at the limit a collapse of coral reef ecosystem functions and shift to other community types not dominated by corals (Hughes et al., 2017). Just in relation to climate change, recent analyses indicate that most coral reefs will not survive the next 3–5 decades unless the most ambitious climate mitigation targets are met (van Hooidonk et al., 2016; Beyer et al., 2018; IPCC, 2018), or they can ecologically adapt. Transition of coral reef habitats to new states (Hughes et al., 2017), whether dominated by different coral taxa, other benthic invertebrates or algae, will be a reality for most coral reef locations globally, posing a novel challenge for designing monitoring systems to accurately track this change.
Because of their value and vulnerability, coral reefs are a sensitive indicator system for global change, and therefore have a prominent role as a flagship socio-ecological system to document the state of nature and its importance for societal well-being, and sustainable development. Their flagship role is highlighted by two recent United Nations global assessment reports, the IPCC report on 1.5°C warming (IPCC, 2018) and the IPBES Global Assessment (IPBES, 2019) (please see Table S1 for a list of all acronyms used).
Global Targets and Societal Relevance of Coral Reefs
Societal relevance of coral reefs is indicated by their importance in Convention and other intergovernmental texts (see Miloslavich et al., 2016). The Convention on Biological Diversity (CBD) Aichi Biodiversity Target 10 states that “By 2015, the multiple anthropogenic pressures on coral reefs, and other vulnerable ecosystems impacted by climate change or ocean acidification are minimized, so as to maintain their integrity and functioning.” It was expected that focusing the international community on taking action toward this target would also facilitate achieving Aichi Biodiversity Target 6 (i.e., that by 2020 all fish and invertebrate stocks and aquatic plants would be managed and harvested sustainably), and Aichi Target 11 (i.e., focusing on establishing effective protection for at least 10 percent of coastal and marine areas). Clearly, the global community is far behind in achieving these Targets for coral reef ecosystems. The U.N. Sustainable Development Goals (SDG) of Agenda 2030 have now garnered significant attention, including SDG 14 focusing on achieving specific targets on marine ecosystem management, conservation and development. Global assessments such as those by the Intergovernmental Platform on Biodiversity and Ecosystem Services (IPBES, 2019), the Intergovernmental Panel on Climate Change (IPCC, 2018), and the UN World Ocean Assessment (WOA, 2016) all highlight coral reefs as an indicator system partly due to their vulnerability, but also the availability of data.
Global Observing Systems
The increasing need for integrated, interdisciplinary data combined with increasing capability for generating, managing and using global datasets, has promoted the growth of international processes supporting global monitoring of terrestrial, atmospheric, and ocean systems. The importance of these data is both to respond to currently identified scientific, management, and policy needs for sustained observation (e.g., CBD Aichi targets and SDG Indicators) and to identify and support future improvements to global indicators that can increase the potential for evidence-based decision making at all levels of governance.
Working groups under both the Global Ocean Observing System (GOOS, a platform of the United Nations Education, Scientific and Cultural Organization's Intergovernmental Oceanographic Commission, UNESCO-IOC; Miloslavich et al., 2018a) and the Marine Biodiversity Observation Network (MBON) of the Group on Earth Observations Biodiversity Observation Network (GEO BON) (Muller-Karger et al., 2018b) are charged with supporting and maturing global observing systems for the oceans and marine biodiversity, respectively. Building on earlier leadership in developing Essential Ocean Variables (EOVs) for the physical and biogeochemical parameters, GOOS established a new panel in 2015 to extend EOVs to the biological and ecosystem components of the ocean. The Biology and Ecosystems Panel identified a set of biological EOVs and is working on strengthening and developing coordinated observing networks around each of these (Miloslavich et al., 2018a) in response to identified global needs. Coral cover and composition, and zooplankton abundance and diversity, are the two most advanced of the 10 EOVs first identified in 2016, both having standardized observational records extending in some places over decades, and recognition by both the scientific and management communities. MBON was established to develop a global community of practice for the collection, curation, analysis, and communication of marine biodiversity data. This requires coordination and collaboration between countries, organizations and individuals involved in the Group on Earth Observations (GEO), and many other organizations. MBON emphasizes objective knowledge of changes in marine life and ecology, and promotes the integration of regional datasets through global systems such as the Ocean Biogeographic Information System (OBIS).
Hard coral cover and composition was identified as one of the leading biological EOVs (see specification sheet at: www.goosocean.org/eov), partly due to the two decades of coordinated coral reef monitoring already undertaken by the Global Coral Reef Monitoring Network (GCRMN), a programme of the International Coral Reef Initiative (e.g., Wilkinson, 2000, 2008). This Community White Paper outlines the status and near future of regional to global scale networks of observing systems for coral reefs. Some emerging technologies hold promise and are also mentioned, though given the breadth of research and effort on coral reefs it is impossible to cover all relevant observing systems.
Current State of Monitoring—in situ Observations
Update Since OceanObs'09 (I-CREOS)
The Community White Paper for Coral Reef Ecosystems for OceanObs'09 (Brainard et al., 2011) described approaches being used and emerging technologies at the time for acquiring integrated and interdisciplinary biological, physical, and geochemical observations using nested combinations of: visual surveys, moored instrument arrays, spatial hydrographic and water quality surveys, satellite remote sensing, and hydrodynamic and ecosystem modeling that was collectively referred to as the International Network of Coral Reef Ecosystem Observing Systems (I-CREOS).
While many portions of I-CREOS have been diligently implemented, these integrated efforts have advanced more rapidly in wealthy developed nations. Moving forward, there is an increasingly important emerging role for MBON and GOOS to provide a framework that more effectively realizes I-CREOS and incorporation of its various elements in all nations with coral reefs. Complementary to the EOV approach of GOOS, MBON focuses on the Essential Biological Variables (EBV) approach of GEOBON (Pereira et al., 2013; Muller-Karger et al., 2018a,b) and both recognize the essential role of capacity development and technology transfer to establishing a global monitoring system (Bax et al., 2018). To fully realize the I-CREOS vision is a continuing goal for the coming decade.
Essential Variables for Coral Reef Monitoring
The state of a coral reef is most simply indicated by the variable “hard coral cover,” which has been the standard variable for reporting coral reef health (see Wilkinson, 2000, 2002, 2004, 2008; Bruno and Selig, 2007; Tittensor et al., 2014). However, while a single indicator provides a useful and communicable message, it is not sufficient to understand nuances of reef health (Díaz-Pérez et al., 2016), such as about biological diversity, the composition and properties of the coral community, recovery potential, and functional, trophic, biogeochemical, and physical processes concurrently interacting to support coral reef ecosystem health and the myriad ecosystem services that people benefit from. Current practice recognizes that function and taxonomic discrimination among corals (e.g., recruitment, stress resistance), information on the algal community (particularly turf, fleshy, and coralline algae) and fish abundance, biomass, and trophic roles are key variables to understand the health of a reef, and are priorities for monitoring (Smith et al., 2016). In addition, contextual information is necessary. Such information may include spatially and temporally-varying environmental conditions and processes, uses and benefits for people, place-based social and cultural practices, and stewardship and governance of reef resources.
The biological and ecological EOVs are an initial step to capture this complexity, with coral and macroalgal cover and composition and fish abundance and distribution as three of the ten priority biological EOVs (Table 3). But variance in methods and approaches to monitoring algae and fish makes aggregating data more challenging than for coral cover (Jackson et al., 2014; Obura et al., 2017), thus they are at a lower level of readiness or maturity (UNESCO, 2012) in the context of global observing and reporting. Incorporating even more nuances on coral population structure and processes, varying environmental conditions and social and economic indicators involves even greater complexity.
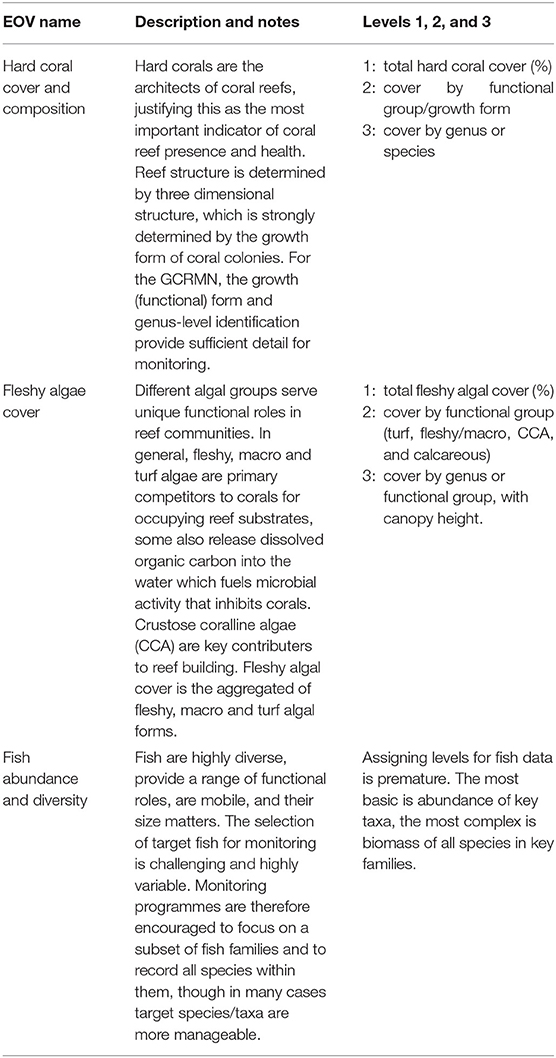
Table 3. Essential Ocean Variables (EOVs) important for monitoring and reporting coral reef health, and levels proposed to assist in assessing data quality by the Global Coral Reef Monitoring Network (GCRMN).
Observer-Based Monitoring of Coral Reefs—The GCRMN
The GCRMN has been the de facto global observing system for coral reefs since 1997 with the publication of the first global status of reefs report (Wilkinson, 2000) that was motivated by the 1st global coral bleaching event of 1997–98. Since then, a range of global, regional, and thematic reports and methods manuals have been published, and have been the backbone of coral reef information used in global reporting for conventions such as the CBD (e.g., Secretariat of the Convention on Biological Diversity, 2014; Tittensor et al., 2014) and global assessments (e.g., WOA, 2016).
In the last 3–5 years the need for renewal and modernization of the GCRMN has been identified, which is being undertaken applying lessons and standards from GOOS and GEOBON, to expand the scope of monitoring to integrate socioeconomic and biophysical elements. The key elements of re-design include:
• applying the principles of the Framework for Ocean Observations (UNESCO, 2012, p. 7), in particular the identification of three core features for an observing system: (a) the justification, or goals, for the observing system in relation to societal priorities; (b) the processes (monitoring elements), methods, and teams used to collect the data needed to address the requirements; and (c) the outputs (data and information products) that inform decisions based on the societal priorities.
• applying the Drivers Pressures Status Impact Responses (DPSIR) model used in many convention processes (Patrício et al., 2016; Miloslavich et al., 2018a), adapted to incorporate more holistic concepts of ecosystem-based management, socio-ecological systems, and human well-being (Kittinger et al., 2012; Kelble et al., 2013; Fletcher et al., 2014; Wongbusarakum et al., 2014), to focus on priority societal needs and interactions for monitoring to deliver the knowledge needed for management;
• adopting the Essential Ocean Variable (EOV)/Essential Biodiversity Variable (EBV) frameworks to identify the priority variables for understanding and reporting on the health of coral reefs, and mechanisms to improve and expand their delivery (Bax et al., 2018; Miloslavich et al., 2018a; Muller-Karger et al., 2018b);
These elements of design are illustrated in Figure 1, and inform a new Implementation and Governance Plan for the GCRMN to be adopted by the ICRI General Meeting in December 2018 (ICRI, 2018) and endorsed by GOOS and MBON, to define a common global strategy for coral reef monitoring and reporting.
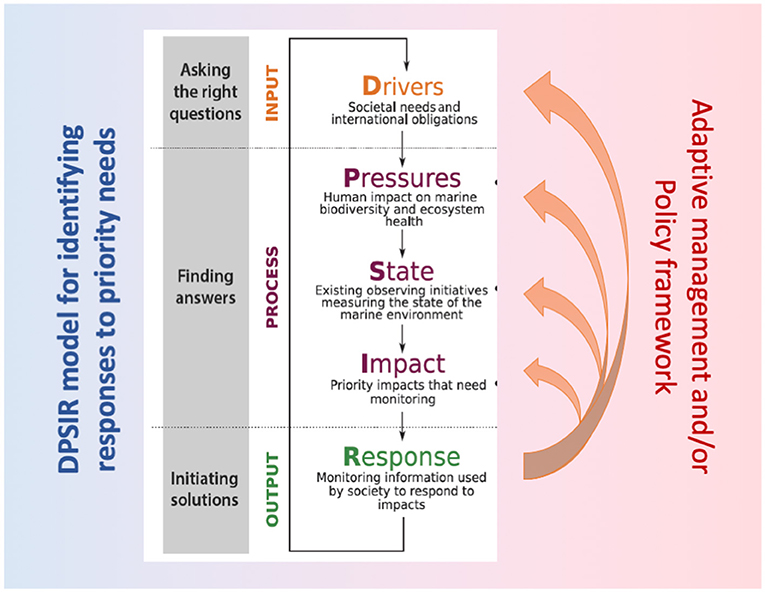
Figure 1. Integrated monitoring for the GCRMN, illustrating the primary areas of focus for monitoring (variables that measure reef state, key pressures and impacts), sandwiching these in a DPSIR (Driver Pressure State Impact Response) framework. The DPSIR framework explicitly asserts key societal questions and drivers so monitoring outputs inform necessary responses in an adaptive management framework. Sources: UNESCO (2012), UNEP/SPAW (2017).
GCRMN Network Structures
Regional networks and their participants form the core of the GCRMN, and provide the foundation for GCRMN activities, bringing together a large number of collaborators across a broad geographic scope. The current set of GCRMN regions (Figure 2) has evolved over the last decade to reflect working relationships among countries and institutions. The UN Environment Regional Seas programmes provide the primary institutional mechanism for coordination or facilitation of many GCRMN regions, with operating procedures tailored to the regional context. For example, the Caribbean network has a coordinator, hosted at the Caribbean Environment Programme's Regional Activity Centre for Specially Protected Areas and Wildlife, whereas the Western Indian Ocean network falls under a Task Force, established by the Nairobi Convention.
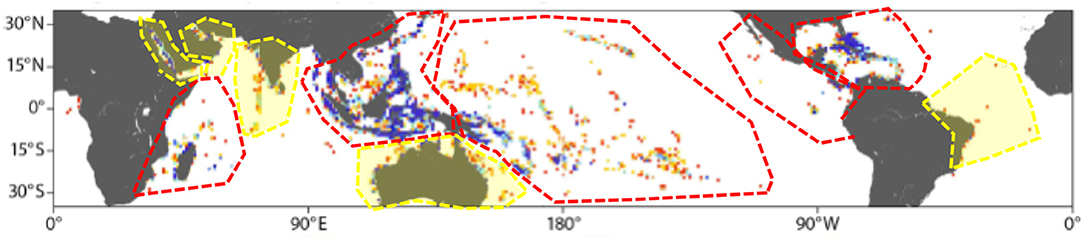
Figure 2. GCRMN regions illustrated with approximate boundaries. Regions with red shading have completed or in advanced stages of reporting by the end of 2018 (Caribbean, Western Indian Ocean, Pacific, East Asian seas, and the Eastern Tropical Pacific). Regions in yellow, reporting during 2019.
Within the regional networks, coral reef monitoring programmes may be grouped in national institutions or networks, and be of varied types: e.g., protected area monitoring programmes, project- or research-based monitoring programmes, non-specialist volunteer, and community monitoring programmes, etc. Frequently, national networks form the primary basis for facilitating data submissions from contributors, and thence to the regional networks. Both national and regional networks may undertake training and awareness raising, to sustain or support development of monitoring programmes and data collection, and build support among stakeholders.
Under the GCRMN's new guidelines, the regional level is reinforced as the priority scale for reporting (Figure 2). Three regions have completed coral reef status reports—the Caribbean (Jackson et al., 2014), the Western Indian Ocean (Obura et al., 2017), and the Pacific (Moritz et al., 2018)—and two are in progress—East Asian seas (Box 1) and the Eastern Tropical Pacific. A target for the GCRMN is to compile a global report for 2020 to respond to Aichi Target and SDG requirements, which will require activation of the remaining regions, updates to the older regional reports, and a global data compilation.
Box 1. Case study: Decadal trend assessment of coral reefs in the East Asia Region.
The greatest regional coverage of coral reefs occurs within the countries of Northeast and Southeast Asia, collectively known as East Asia, and includes much of the reefs within the biodiverse Coral Triangle ecoregion (Figure 3). Countries within the region continue to be highly dependent on coral reef resources for the livelihoods of their people, which have consequently put sustained pressures on them. The situation is exacerbated by increasing uncertainties arising from global climatic events that put greater stress on coral reefs.
In response, the East Asian regional coral reef monitoring network has identified an urgent need to take stock of the current status of coral reefs, assess decadal trends, establish mechanisms for regular assessments within the region, and to build the capacity of early-career scientists. This effort comprises one of the ongoing GCRMN regional processes for data compilation and reporting. With the support of the Japanese government, a 4-year, multi-phased project to undertake a comprehensive regional analysis of coral reef monitoring data was initiated in 2017. The project is led by a task force consisting of two coordinators and seven meta-analysis experts. Annual workshops are organized to maintain the network and share progress and results.
Given the volume and variety of coral reef data that exist across monitoring teams, a phased approach was adopted, starting with a pilot analysis from 2017 to 2018 covering monitoring data from seven countries and states (Figure 3) to ensure robustness of the analysis routines, data consistency, as well as longer-term scalability for regular assessments. Once analyses routines are finalized, complete analysis of data from all 14 countries and states represented in the region will commence in 2019, with a goal to complete the draft regional report in 2020. Through this regional analysis effort, the network hopes to identify pathways that can lead to the development of management options to safeguard and improve coral reef condition within the region.
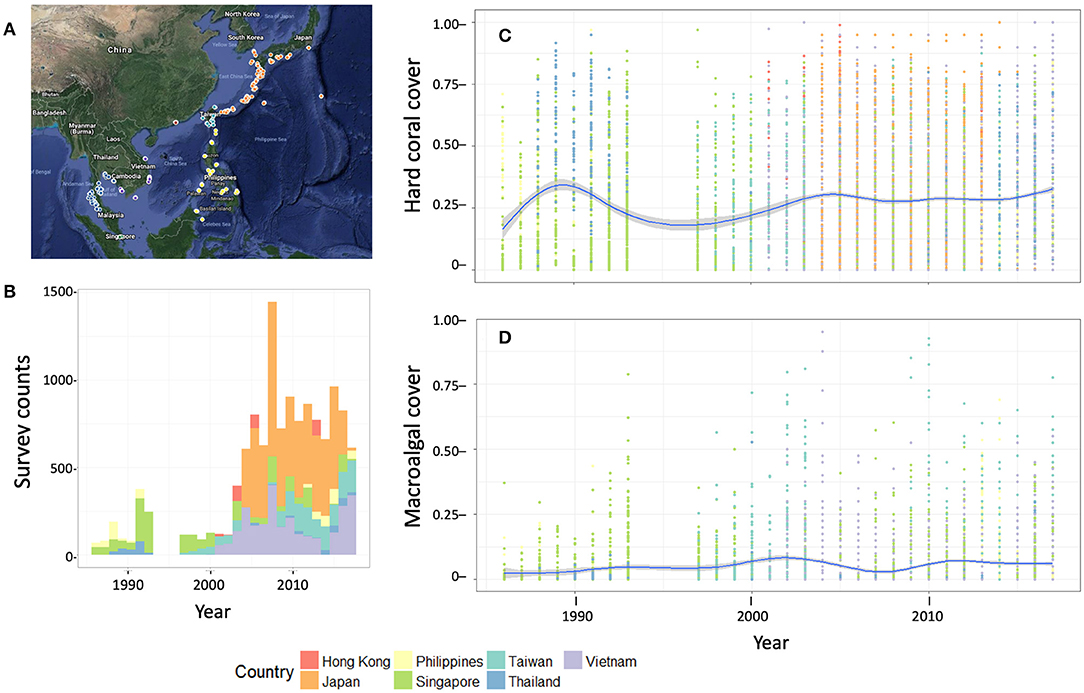
Figure 3. Preliminary results of the GCRMN East Asian region's pilot analysis of data from 7 countries. (A) Survey sites represented in the dataset, (B) number of sites by country, and (C) hard coral and (D) macroalgal cover by year (mean and 95% confidence interval of the mean, and individual survey points).
Revitalizing the GCRMN
Greater precision and consistency in how data are measured and reported is a priority focus for the GCRMN in coming years. The GCRMN is adopting the Essential Variable approach [section Update Since OceanObs'09 (I-CREOS)] and a data quality model (Table 3) that enables (a) effective submission of minimum data required to monitor and assess reef health (hard coral cover and composition), and (b) procedures for strengthening and extending the data to additional key variables (e.g., algae and fish), and improving the resolution and 'quality' of each variable. The data quality model scores three levels of data, from minimum requirements (Level 1) to maximum (Level 3), providing specific guidance on how to improve data quality from level 1 to 3, and incentivizing monitoring teams to improve quality. The key method for ensuring data quality is the preparation and submission of appropriate metadata (GCRMN, 2018a,b) that includes information on sites and sampling, variable precision and replication, and any data processing or transformations applied subsequently. This approach enables two objectives: first, to maximize submission of data meeting a minimum from all parts of the globe, and second, provide a pathway for capacity building to improve data quality to as high levels as possible.
GCRMN accepts data from of a variety of observing platforms, including national, local and institutional monitoring programmes, participatory monitoring by communities, divers, and the interested public; from researchers and scientific cruises; and other in situ measurements that meet methodological and quality criteria. The essential variable/data quality approach facilitates blending data from these different types of sources. Adoption of new platforms is further facilitated by the focus on essential variables and data quality, rather than methodology. A key tension exists, however, between the need for common standards to enable aggregation of data to larger scales, and location-specific priorities and needs for monitoring. EOVs provide a minimum set of observations for sustained monitoring supporting regional and global comparison and increasing influence of the measurements on relevant decision makers. Additional local and regional data will often be needed for improved interpretation and long-term management at those levels. Local monitoring programmes will have to deal with balancing these needs against the resources they have available, and the GCRMN will address this by making its requirements supportive of local capacity building and revenue generation, by increasing the value of data through its sharing.
Human Dimensions Monitoring in the GCRMN
Socio-economic aspects of people and coral reefs, both as beneficiaries and as drivers of changes in reef state, have been a focus for the GCRMN, with the first manual published in 2001 (Bunce et al., 2000; Bunce and Pomeroy, 2003), followed by regional socioeconomic monitoring (SocMon and later SEM-Pasifika) guidelines available in multiple languages. Implemented in nearly 40 countries at more than 80 sites globally, SocMon/SEM-Pasifika has built the capacity of hundreds of fisheries and Marine Protected Area (MPA) managers, field staff, government departments, Community-Based Organizations (CBOs) and Non-Government Organizations NGOs.
The most recent guidance update (UNEP/SPAW, 2017) has identified a narrower set of variables to forge greater consistency among monitoring programmes, focusing on the key sectors tourism, fisheries, agriculture (large scale) and industry, human population, and governance. Concurrently, integrating socio-economic and biophysical data is necessary to improve the usefulness of monitoring data for holistic ecosystem-based management, particularly to promote adaptive management (Wongbusarakum and Heenan, 2019) in diverse settings from community-based to government-led settings. Achieving this integration is facilitated by a new approach that turns the impacts focus of DPSIR approaches to a more holistic perspective of both positive and negative interations between people and nature, in the Drivers, Pressures, State, Ecosystem Services and Response (DPSER) model (Kelble et al., 2013; Fletcher et al., 2014, 2015) and a greater focus on ecosystem-based management, socio-ecological systems, and human well-being (Kittinger et al., 2012; Wongbusarakum et al., 2014) in coral reef management.
Contextual variation increases from physical/chemical to biological to social systems. This is reflected by the initial focus of GOOS and global climate monitoring systems on physical and chemical variables in the late 1990s, followed by the current focus on biological variables some 20 years later (Miloslavich et al., 2018a). A similarly cohesive set of social and economic indicators relevant to the human dimensions of coral reefs across multiple contexts globally has yet to emerge. Within the GCRMN, the next decade will include work on the emergence of integrated social and economic variables that are relevant to, and measurable at ecosystem scales aligned with biophysical monitoring, to become standard practice across an increasing proportion of monitoring programmes. As with the tension across scales in biophysical monitoring, SocMon/SEM-Pasifika will continue to serve the needs of site-based management by taking into consideration local needs and resources, and useful place-based indicators.
Event/Response Monitoring
Several major threats to coral reefs occur in short episodic events, and at larger spatial scales than can be addressed by regular monitoring. Event-based monitoring is necessary to track these, by narrowing the variables for collection to extend the scale and replication of samples at more frequent intervals and/or larger spatial scales. Coral bleaching is the most significant such event globally, but other significant events at varied scales include crown of thorns sea star outbreaks, tsunami or cyclone damage, coastal flooding, and disease outbreaks. EOVs remain relevant to rapid response and again provide an opportunity to build understanding of the scale of the event beyond the local, however, modified methods may be required.
Coral Bleaching
Global-scale coral bleaching risk alerts have been generated for the past 20-years based on satellite observations of sea surface temperature (SST), through detection and mapping of high positive SST anomalies relative to long-term averages. Bleaching alert products were developed by the National Oceanic and Atmospheric Administration (NOAA) Coral Reef Watch Program (http://coralreefwatch.noaa.gov/satellite/; Liu et al., 2014). Other regional forecast models of coral stress due to heat, extreme cold winter anomalies, and coral disease have been implemented, such as in Australia (Maynard et al., 2011). These models, combined with volunteer-based ground-truth monitoring networks (e.g., Gudka and Obura, 2017), help generate awareness of the threat of coral bleaching, and build interest and capacity in management responses to mitigate them and promote resilience and recovery to the events (Marshall and Schuttenberg, 2006).
A typical major bleaching event occurs over 2–4 months, and monitoring one effectively requires different approaches at its start, during the event, and afterwards to track long term impact. The basic setup for monitoring coral bleaching is outlined in Table 4. Data collected in stages 1 and 2 need to provide an estimate of the proportion of corals bleached and/or dead, which can be done at low levels of resolution by volunteers with basic training and experience. Typically, once an event is underway at stage 3, improved data quality that becomes more quantitative helps to target management and policy responses more effectively.
Small Unmanned Aerial Systems (sUAS) have been used to effectively assess spatial distributions of coral bleaching on shallow-water patch reefs in Kaneohe Bay, Oahu. sUAS were able to collect imagery and create orthomosaics with ~1 cm resolution at a rate of 2000 m2/min vs. 10 m2/min using in situ video surveys; the most efficient survey methods as detailed in Jokiel et al. (2015) and Levy et al. (2018). With the ability to resolve individual coral colonies from the aerial imagery, methods to compare colony health metrics from in situ and high-resolution aerial images of the same coral colonies over time are under development. Among other questions, this effort will help understand the tradeoff between in situ millimeter resolution imagery and centimeter resolution aerial imagery, and pose ideas for reef survey protocols that efficiently utilize both methodologies for shallow patch or fringing reef environments.
Coral Diseases
While a normal component of all ecosystems, disease outbreaks can occur when environmental conditions change disrupting the usual pathogen-host dynamics (Daszak et al., 2000; Dobson and Foufopoulos, 2001). Coral disease outbreaks are increasing in impact and frequency worldwide (Harvell et al., 2007) exacerbated by degraded coastal waters and climate change (Harvell et al., 1999; Maynard et al., 2015). The most severe disease outbreak ever recorded has spread throughout most of the Florida reef tract and has been ongoing since 2014 (Precht et al., 2016; Walton et al., 2018).
The need for standardized disease response protocols has been recognized and resulted in the recent creation of multiple resources for biologists (Aeby et al., 2008; Raymundo et al., 2008; Woodley et al., 2008; Beeden et al., 2012). An important component of disease assessment is the use of standardized nomenclature to describe coral lesions in the field. Assigning a specific name to a disease lesion in-situ is difficult because there is limited information on coral disease etiologies, ecologies and pathogen specificities. For example, “white syndromes” are tissue loss diseases that have different underlying etiologies, and ecologies, even though the gross lesions (i.e., lesion observed underwater) may look similar (Work et al., 2012; Bourne et al., 2014). Until more is known about the etiology and ecology of coral diseases, researchers are advised to report diseases by host genera affected and lesion type (tissue loss, growth anomaly or discoloration; Work and Aeby, 2006; Woodley et al., 2008).
Improved monitoring of diseases allows development of predictive models that give options to resource managers in mitigating future outbreaks (Aeby et al., 2008; Woodley et al., 2008; Beeden et al., 2012). The development of a Disease Response Plan is a critical component of managing disease, with notable examples from Hawaii (https://dlnr.hawaii.gov/reefresponse/rapid-response-contingency-plan-rrcp/) and the Great Barrier Reef (http://elibrary.gbrmpa.gov.au/jspui/handle/11017/2809). Disease outbreaks of unprecedented severity and spatial extent, are likely in the future, and effective management will depend on biologists and reef managers proactively developing the capacity to mitigate, assess, monitor, and respond to disease outbreaks.
Citizen Science and Coral Reef Monitoring
Citizen science is growing rapidly (www.citizenscience.org/). The vast extent of coral reefs, in both remote locations and populated areas with few resources for science-based monitoring, means that citizen science opportunities are abundant. Many programmes have arisen to monitor reef conditions in local areas, such as with local communities or diver-volunteers. These contributions scale from programs with limited entry of expert-volunteers (e.g., Reef Life Survey, Stuart-Smith et al., 2017) to large communities of recreational diver-volunteers (e.g., Reef Check, Hodgson, 1999; Hodgson et al., 2016). Some opportunities for training in coral reef monitoring have been identified in Miloslavich et al. (2018b).
In 1997, Reef Check carried out the first global survey of coral reefs that documented the extent of the coral reef crisis (Hodgson, 1999). Since then, the method (Hodgson et al., 2016) has served as the community-based monitoring component of GCRMN and Reef Check data have been used in GCRMN reports and dozens of scientific publications (reefcheck.org/publications). Reef Check pioneered the use of a set of about 30 regional and global “indicator organisms” to track major human impact on coral reefs as well as ecological and socioeconomic changes. Over 100 scientists participated in the design and testing of Reef Check and there is an online database of 20 years of standardized monitoring data from reefs in over 100 countries/territories (data.reefcheck.us). Participants undergo a rigorous 3-day certified training course before they are allowed to contribute data. More than 50% of Reef Check data have been collected by professional scientists at universities and research institutions. The data have been used at all management levels (local, national, regional, and global).
Reef Life Survey (RLS) is a citizen-science program on shallow rocky and coral reefs, based on selective recruitment and intensive training of committed volunteer SCUBA divers (Edgar and Stuart-Smith, 2014), producing data indistinguishable from those collected by professional scientists. Since 2008 more than 250 divers have contributed 12,000 surveys at more than 3,300 sites in 54 countries. The RLS survey methods are globally standardized, and consistent with methods used in long term scientific programs such as the Smithsonian MarineGEO program in the Americas (https://marinegeo.si.edu/), Eastern Tropical Pacific Collaboration (Edgar et al., 2011), and the Australian Temperate Reef Collaboration (Edgar and Barrett, 2012). Target taxa include all fishes and larger mobile fauna, mobile invertebrates and cryptic fishes, and photoquadrats of substrate cover. Data collection occurs through three primary mechanisms: (1) annual targeted monitoring of reef sites at dispersed locations in temperate and tropical waters (as used to support Australia's State of Environment report, Stuart-Smith et al., 2017), (2) targeted voyages to poorly surveyed locations, and (3) ad hoc data collection by divers in their local waters and when on vacation. RLS methods are used for training scientists and potential citizen scientists in many areas around the globe.
An application of citizen science to address the challenges of extensive and remote coral reef areas is in Hawaii, where it is difficult for resource managers to detect the early onset of coral bleaching, disease, Crown-of-Thorns, and invasive species outbreaks. To address this need, the citizen science program, the Eyes of the Reef Network (EOR) (eorhawaii.org) was developed. It trains reef users to recognize and report coral reef health conditions. EOR shares reports with the State of Hawai'i Division of Aquatic Resources and provides the critical first stage of Hawaii's Rapid Response Contingency Plan (https://dlnr.hawaii.gov/reefresponse/rapid-response-contingency-plan-rrcp/). EOR network members played a critical role during the 2015 bleaching event by submitting 100s of reports helping to document the severity and extent of coral bleaching on Hawaii's reefs.
Automating Data Collection and Analysis
The vast majority of biological observations of coral reefs have been collected and analyzed by human observers. A key challenge, particularly in monitoring for long-term changes, is the inherent subjectivity and lack of consistent repeatability of human observers. Underwater, human observers are also physiologically limited in the duration and depth of their observations. Because of this, and the inherent heterogeneity, size, and in some cases, remoteness of coral reefs, routine reef monitoring at biologically relevant timescales is time and cost intensive.
However, when developing new technologies, it is important to remember the technology is not the end-goal but a tool to provide scientists with the ability to collect information safer, faster, and with greater accuracy and/or quantity. As such, the introduction of new technologies into coral reef science, while necessary to continue increasing our understanding of coral reef dynamics, must not interfere with current data collection methodologies, but rather enhance or supplement current methods, while these new technologies are vetted. Over time, it is possible that new techniques supercede previous methods after a rigorous vetting processes. The emerging focus on EOVs (see section Essential Variables for Coral Reef Monitoring) facilitates this process, by focusing on the data, rather than the methods or platforms.
Autonomous High Resolution Data Collection Systems
Unmanned systems have the potential to be cost effective, persistent reef monitoring tools that are capable of collecting information about a number of important reef monitoring variables including coral species diversity, composition, and contextual data on environmental conditions. Integrating manned in situ, and unmanned in situ and aerial survey techniques is an area of active research and development, to provide comprehensive monitoring support for remote shallow water reef environments. Current work attempts to integrate multi-domain unmanned systems with a variety of environmental and physical sensors to collect fine-scale data, enhancing our understanding of environmental stressor impacts on coastal coral reef health. Unmanned systems can be tasked with collecting physical data such as imagery, and bathymetry data, in addition to environmental data such as oxygen, temperature, chlorophyll, turbidity, and salinity (Grasmueck et al., 2006; Chirayath and Earle, 2016; Koparan et al., 2018; Levy et al., 2018; Monk et al., 2018).
A wide range of unmanned systems are under development (Figure 4). The majority are larger Autonomous Underwater Vehicles (AUVs), which are designed for open ocean sampling such as Wave Gliders and Remote Environmental Monitoring UnitS (REMUS). These platforms are capable of traveling large distances and carry large payloads, but are not designed to operate in shallow water environments. Smaller Remotely Operated Vehicles (ROVs) are capable of maneuvering in shallow water environments, carrying cameras and/or other small payloads, but current technological limitations on autonomous underwater guidance and navigation controls limit operations that require precision navigation to tethers, limiting range. Autonomous Surface Vehicles (ASVs) are able to access shallow environments and operate autonomously due to access to GPS data. These vehicles are effective at collecting imagery and bathymetry information but cannot easily sample into the water column. AUVs may also be applied to multiple tasks, such as impacts of invasive species (Ling et al., 2016), changes in coral reef structural complexity (Ferrari et al., 2016), population trends in demersal fishes (Seiler et al., 2012), and assessing the diversity in reef communities (James et al., 2017).
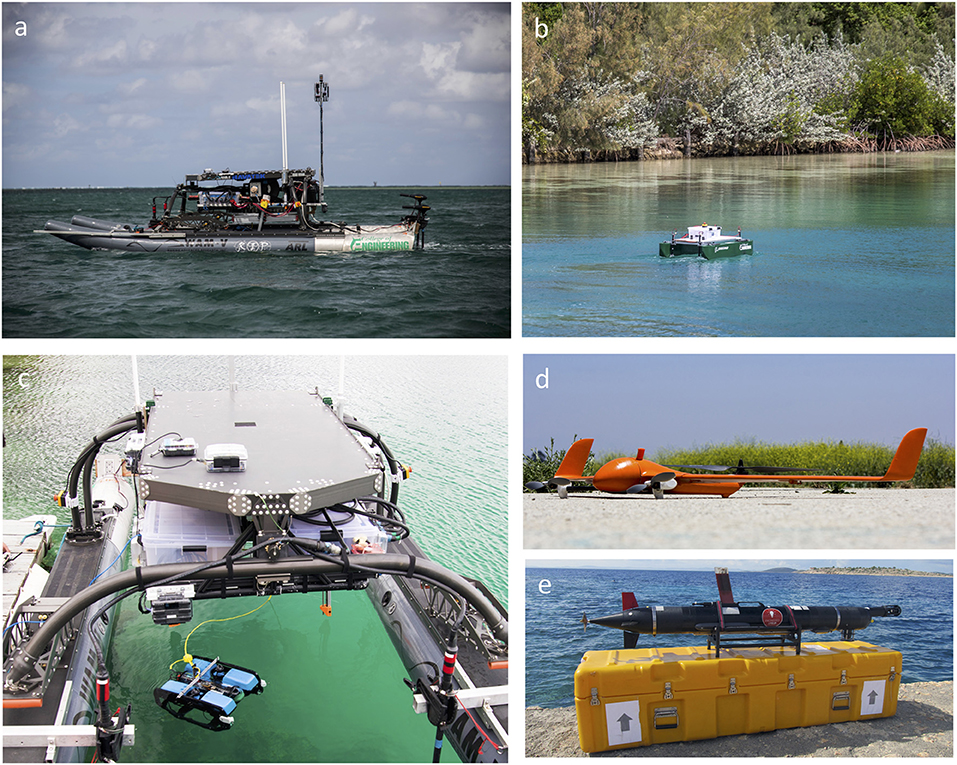
Figure 4. Autonomous Surface Vehicles (ASV's) can range from commercial products (a) (Marine Advanced Research Inc's Wave Adaptive Modular—Vessel (WAM-V), to custom built platforms (b) (UH Manoa Engineering “Mr. UH”). (c) Blue Robotic ROV tethered to the WAM-V extends the range of ROV capabilities. Unmanned Aerial Systems (UAS) such as the Flightwave Edge (d) have collected aerial data on open ocean currents, coral reefs, and marine debris. Autonomous Underwater Vehicles (AUVs) such as the LAUV (e) designed by The Faculdada de Engenharia de Universidade do Porto can collect environmental and physical data in open ocean and shallow water environments.
Small Unmanned Aerial Systems (sUAS) are capable of collecting high resolution imagery over large areas, and can carry small sensor packages for collecting discrete water quality data. However, regardless of flight endurance, these systems must refuel and are restricted to relatively lightweight (~5 kg) payloads. As such, there is significant interest in developing heterogeneous teams of unmanned systems, combining capabilities from different types of systems to conduct persistent sampling of the marine environment (Ferreira et al., 2017). Leveraging commercial off-the-shelf vehicles, which can be modified and integrated with other hardware and software, is an effective method to keep costs of unmanned systems reasonable while still providing custom capabilities.
Stationary imaging systems are also under development and with high potential for expanded deployment. Baited Remote Underwater Video (BRUVs) are becoming a standard tool for monitoring spatial and temporal changes in fish communities, including stereo applications for benthic fish and their habitat structure (Langlois et al., 2018), and for pelagic fish (Bouchet et al., 2018).
Automated Image Capture and Analysis
Building on the historical dominance of in situ observer-based techniques in coral reef monitoring (section Observer-Based Monitoring of Coral Reefs—the GCRMN), the development of methods to capture images that can be analyzed visually, first by observers onshore and unrestricted by diving constraints, and now autonomously by software, has been an area of rapid development. Recent focus has been on systems that collect images autonomously but are navigated underwater by a diver, often on a scooter for propulsion to cover larger areas (e.g., the XL Catlin Seaview Survey http://catlinseaviewsurvey.com/), as well as tethered (ROVs) and fully autonomous vehicles carrying cameras.
Automated image analysis using rules-based or statistical (Artificial Intelligence/Convolutional Neural Network, or CNN) methods significantly increase the speed of analysis (Beijbom et al., 2015). Rules-based image analysis techniques use thresholds of known image properties such as pixel value to determine the color or shape of a specific type of object or substrate. Issues with rules based analysis occur when objects in need of identification occur outside these thresholds. Statistics-based image analysis uses training sets to “learn” an object or substrate of interest. While these techniques can be powerful and efficient, developing a training set is very time intensive, and adjusting variables inside the “black box” of a CNN is difficult due to the traceability of information through the CNN workflow. These automated image analysis methods are already to the point where they assess coral cover as well as and more consistently than human observers Williams et al. (2019).
There are rapidly developing technologies to both acquire and process 3-dimensional photomosaic imagery using structure-from-motion (SfM) photogrammetry (Burns et al., 2015), using sequentially captured images constructed into a photo-mosaic. The software creates a dense point cloud of common features, and overlays texture over a mesh model derived from the point cloud. The resulting orthomosaic, once georeferenced, can provide high-resolution layer of an entire surveyed area (Stal et al., 2012). This offers the ability to acquire statistically-robust observations about 3-D reef structure, overall coral biomass, and, importantly, key coral vital rates such as growth (Edwards et al., 2017). This technique has been used with both aerial and in situ imagery (Burns et al., 2015; Casella et al., 2016; Levy et al., 2018). Over the coming decade, these technologies will enable tremendous advances in spatial and temporal coverage of coral reef monitoring observations that will be easily transferred to the use of volunteers and citizen-scientists for shallow waters and the use of autonomous vehicles for deeper depths.
Integrating Sensor/Observing Sytems
Remote Sensing
Satellite-based remote sensing enables covering shallow-water tropical coral reefs over a broad range of spatial and temporal scales (meters to global cover and from near-daily to decadal). Satellite images and other data, such as measures of the roughness of the sea surface or sea level can cover large areas, such as hundreds to thousands of square kilometers, quickly and synoptically, frequently, and repeatedly, over long periods of time.
Two major categories of satellite observations of coral reef areas may be defined by the objectives of the application (Eakin et al., 2010; McCarthy et al., 2017). The first category focuses on environmental conditions that affect coral reef organisms, such as variations in temperature, light, waves, and winds. Remote sensing of environmental conditions is critical in the interpretation of changes observed in community structure, coral reef cover, or at the level of habitat. For example, sea surface temperature (SST) satellite observations have been instrumental in the systematic mapping and detection of coral stress related to warm extremes in temperature, which can lead to bleaching, disease, death, changes in biodiversity, and the reduction of coral reef cover (Eakin et al., 2010; Soto et al., 2011; Liu et al., 2014; Vega-Rodriguez et al., 2015). Liu et al. (2014) provide a comprehensive review of the operational global-scale warm SST-related stress assessments published daily by the US National Oceanic and Atmospheric Administration (NOAA) Coral Reef Watch Program. Extreme cold events have also been demonstrated to cause mass mortality of corals in the Florida Keys, USA (Lirman et al., 2011). These programs use a combination of infrared and microwave observations to map SST.
Satellite images collected in the visible part of the electromagnetic spectrum (reflected visible sunlight light) are extremely useful to map water quality conditions. Among some of the products derived from such images are ocean surface spectral reflectance, from which a number of products can be derived, including total suspended sediment concentration (TSS), turbidity, colored dissolved organic matter (CDOM) absorption coefficient, chlorophyll concentration and chlorophyll fluorescence, and water transparency or a measure of the diffuse attenuation coefficient (see summary in Muller-Karger et al., 2013). Microwave and radar observations are also used to generate routine observations of important parameters including wind speed and direction, wave height, and sea surface height including long-term changes in sea level (Muller-Karger et al., 2013). These types of data are also critical to understand connectivity between different reefs located at considerable distances from each other (Soto et al., 2009).
The second category focuses on mapping coral reef habitats at the landscape scale and globally. The technologies available for mapping of coral reef habitats have advanced substantially over the past 30 years see summaries by Andréfouët et al. (2005a; 2005b) and Eakin et al. (2010). Of great interest are data available since the 1970's from a number of satellites that have provided time series of images in the visible part of the spectrum, with spatial resolution of 30 m pixels. Each image covers hundreds of square kilometers, allowing mapping of general outlines of reef areas, sand or bare bottom areas, and seagrasses (see Wabnitz et al., 2008, 2010). Satellite images also allow a unique way to assess water quality around coral reefs (Palandro et al., 2004; Barnes et al., 2015), as well as area-wide estimates of metabolism or calcification (Moses et al., 2009). Landsat-class data since 2000 have been used to derive the Millennium Coral Reef Map, the only global-scale coral reef map (Andréfouët et al., 2006; UNE/WCMC, 2016). Improvements are now possible through incorporation of narrow blue band data from the Operational Land Imager/OLI sensor on Landsat-8, and new data from the European Sentinel-2 series of satellites (e.g., Hedley et al., 2018). The Paul G. Allen Philanthropies' Allen Coral Atlas project plans to achieve global mapping of coral reefs at ≈3 m resolution using the Planet satellites' 4-band sensors by 2020.
The availability of very high spatial-resolution satellite imagery has now brought a revolution in the way that coral reef areas can be mapped and monitored around the world (Mumby and Alasdair Edwards, 2002; Roelfsema and Phinn, 2010; Naidu et al., 2017; Ampou et al., 2018). Palandro et al. (2003a,b) shows the advantages of mapping across a range of spatial scales. These types of data provide unprecedented maps of complex benthic habitat types and bathymetry estimates. When satellite data are combined with airborne imagery and airborne Light Detection And Ranging (LiDAR) data, particularly from unmanned systems (see section Automating Data Collection and Analysis) new ways to assess benthic habitats become available in a way never before possible (e.g., Wirt et al., 2015). Such combined data are now routinely used in identifying and monitoring marine protected areas (Magris et al., 2015). There is much hope that in the not-too-distant future, coral reef Essential Biodiversity Variables (Muller-Karger et al., 2018b) may be collected with high radiometric quality satellite data at high spectral resolution, relatively high spatial resolution, and at high temporal frequency. Such observations, with high resolution in four dimensions, are referred to as H4 satellite observations (see Muller-Karger et al., 2018a). Integrating these data sources with in situ manned observations may provide unprecedented empowerment for managers of coral reef locations to understand the complexity of processes affecting their sites, and be able to respond to these with effective management tools.
CREWS
Fixed buoys for monitoring aerial and subsurface biophysical properties of coral reef waters are monitored in some specialized cases, notably the Coral Reef Early Warning System (CREWS) of the National Oceanic and Atmospheric Administration (NOAA) of the United States. Started in 1997, it combined software and a sensor platform development to automatically detect conditions thought conducive to coral bleaching (Hendee et al., 2008) in a network of stations around the Caribbean and in Saipan, with data relayed by satellite to the Atlantic Oceanographic and Meteorological Laboratory (AOML) in Miami, Florida USA.
The key research questions addressed by a CREWS station are: (1) what are the long term trends of meteorological and oceanographic variables at key national and international coral reef areas? (2) Can data from various sources be integrated in near real time to provide ecological forecasts at coral and coastal ecosystem areas? (3) What are the key environmental correlates related to coral bleaching and other coral ecosystem concerns such as adaptation and resilience (Gramer et al., 2016), disease, spawning, and migrating organisms?
The challenges facing the installation and operation of an in situ meteorological and oceanographic monitoring network are reported in depth in Hendee et al. (2012). Key challenges have included the loss of fixed stations and buoys due to hurricanes, changes in sensor technologies requiring major system upgrades, inadvertent damage to installations by technicians, etc. The installation of CREWS stations requires significant resources and expertise, although the routine maintenance does not, and is currently attended to by local host country stakeholders who can use the data for climate change and other locally-relevant research interests.
Acoustic Techniques
The increasing availability of affordable, user-friendly underwater recorders, and analysis techniques provide opportunities for passive acoustic monitoring of coral reefs (Servick, 2014). Hydrophones capture a range of sounds produced by coral-reef organisms, potentially complementing traditional visual survey methods. Potential benefits of passive acoustic monitoring include (a) quantitative and objective measures of reef health, (b) low demands on labor, logistics and skill; (c) records of rarely-seen cryptic and nocturnal species; and (d) information about diel and monthly variation in reef ecosystems as well as single-time-point measures (Staaterman et al., 2014; Kaplan et al., 2015; Parsons et al., 2016; Lillis and Mooney, 2018).
Acoustic and visual indicators of reef health can be linked across spatial and temporal scales. Sound-pressure level (particularly at frequencies below 2 kHz) can positively correlate with coral cover, invertebrate abundance, and fish diversity (Kennedy et al., 2010; Kaplan et al., 2015, 2018; Nedelec et al., 2015; Freeman and Freeman, 2016). Several other acoustic indices such as rates of invertebrate snapping sounds (Butler et al., 2017) and the acoustic complexity index (Pieretti et al., 2011) may also correlate with visual measures of diversity (Nedelec et al., 2015; Bertucci et al., 2016), although the generality of these trends is not fully established (Staaterman et al., 2017). Further, habitat degradation can alter reef soundscapes; lower-quality reefs with poor management sound quieter than well-protected reefs (Piercy et al., 2014, Figure 5), and cyclone and bleaching events change dramatically the acoustic profile of a reef (Gordon et al., 2018). Soundscape recordings can therefore be used to compare reef health across both space and time. More classical application of active acoustic techniques is in the realm of multibeam acoustic surveys to baseline exploratory surveys, mapping and monitoring reef habitats (Lucieer et al., 2018).
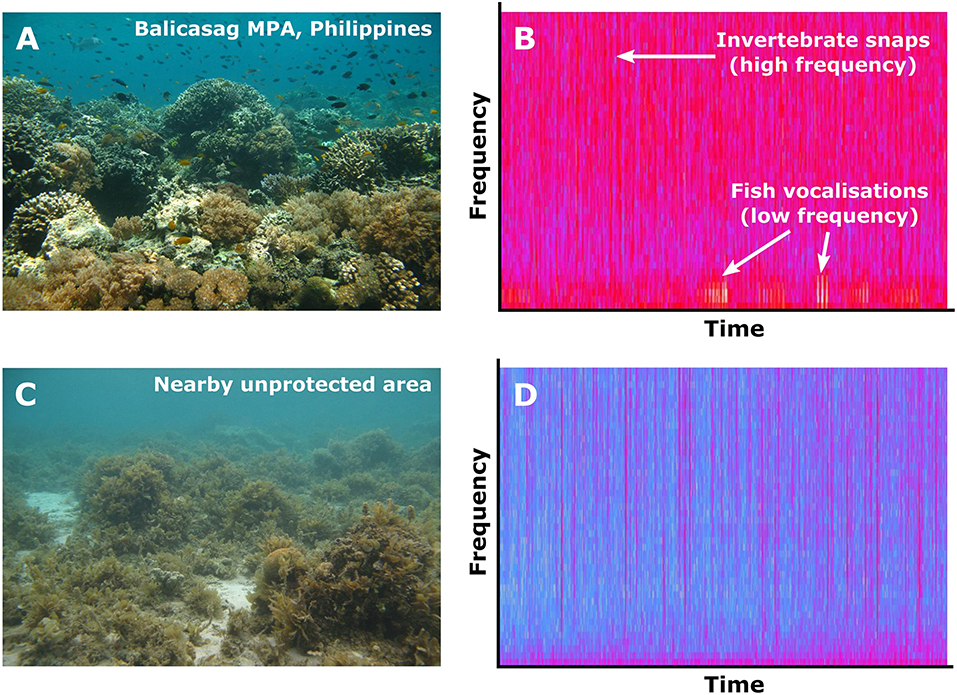
Figure 5. Visual and acoustic comparisons of (A,B) Balicasag Marine Protected Area, a fully-protected reef in Central Philippines, and (C,D) a nearby unprotected, overfished reef. (A,C) Show representative pictures of each habitat. (B,D) Spectrograms showing sound intensity (red colors are high intensity; blue colors are low intensity) at different frequencies (0–7 kHz) over time (4 s). Recordings are explored further in Piercy et al. (2014).
Future challenges for acoustic monitoring include collating global soundscape databases, identifying, and standardizing the most useful analysis techniques, and improving accessibility for practitioners and managers. Sharing and compiling libraries of recordings will increase the resolution of acoustic monitoring programmes, facilitating more meaningful analyses that account for spatial and temporal variation in reef soundscapes (Kaplan et al., 2018). Engaging local stakeholders, ecosystem managers, and citizen science with coordinated and standardized protocols could also greatly increase the scope of surveying efforts. Through increased participation in passive acoustic monitoring, we can add a dimension to our understanding of reef health worldwide.
Ocean Acidification and Coral Reefs
Coral reefs are uniquely vulnerable to ocean acidification (OA) as more acidic conditions erode dead carbonate structures more quickly, and impose greater energetic costs to individual organisms for skeleton formation (Secretariat of the Convention on Biological Diversity, 2014; Hoegh-Guldberg et al., 2017). Equally, impacts of ocean acidification to society are complex though only beginning to be unraveled (IAEA, 2015). The scientific and policy needs for coordinated, worldwide information-gathering on ocean acidification and its ecological impacts, recognized by the United Nations General Assembly (UNGA, 2013), and by many governmental and non-governmental bodies, resulted in the creation of the Global Ocean Acidification Observing Network (GOA-ON) (Newton et al., 2015). GOA-ON includes observing assets across multiple ecosystems, including the open ocean, shelf seas, coasts (including the nearshore and estuaries), and warm and cold-water coral habitats, as well as a variety of observing platforms: ship-based sampling including survey cruises and ships of opportunity; fixed platforms, including moorings and piers; and mobile platforms, including gliders (both profiling and wave) and floats (possibly others, such as animals). Approaches to monitoring and research are interdisciplinary, including carbon chemistry, meteorology, oceanography, biogeochemistry, ecology, and biology.
Basic data collected include temperature, salinity, water depth, oxygen concentration and carbon-system constraint, plus fluorescence, and irradiance were possible. In coral reef habitats, variables corresponding to some specified for the GCRMN are also targeted (e.g., coral and coralline algae cover), with the addition of key processes, including calcification/dissolution and production/respiration ratios. A broader suite of other biological and environmental data is specified for higher level analyses. As with the current dominance of thermal stress and coral bleaching as a primary focus for monitoring programmes, the impacts of OA will be of increasing importance for coral reefs, so incorporating OA-specific protocols in coral reef monitoring programmes will be critical for understanding and preparing for future impacts of OA.
Data Curation and Data Sharing
Despite “hard coral cover and composition” being one of the two most “ready for deployment” EOVs, both GOOS and GCRMN stress the need to strengthen capacity in data and information management. Without enhancing access to coral reef data and ensuring the reliability and provenance to underpin scientific credibility, the science and reporting on the status and trends of global coral reefs will remain limited in their impact. Regional GCRMN datasets (Jackson et al., 2014; Obura et al., 2017; Moritz et al., 2018) are compiled and managed by GCRMN regional coordinators/data leads, in data files and programming code maintained on version-control platforms, such as GitHub (GCRMN, 2018a). In many cases, the original data are still held and archived by institutes and individuals, are often not accessible, and methods and standards differ. The lack of interoperability makes integration and comparison of data difficult and time consuming.
The new GCRMN Implementation Plan (GCRMN, 2018a) puts strong emphasis on improving data management practices, and encourages more open-access and international collaboration. One of the possible options, which is in line with the agreement between GOOS and GEO BON MBON, is to align with and build on the existing efforts of the Ocean Biogeographic Information System (OBIS). OBIS provides an existing global data sharing platform used by nearly 1,000 scientific institutions worldwide and is supported under the intergovernmental framework of UNESCO-IOC. OBIS holds nearly 60 million distribution records of 120,000 marines species and is growing by around 3 million observations per year. An important recent change is that OBIS adopted the Event Core format of DarwinCore and developed the Extended MeasurementorFact Extension, making it possible to document sampling events and link sampling facts and environmental measurements, such as temperature and habitat cover as well as species occurrences, to the event hierarchy. Any biotic measurements (e.g., biomass, abundance, absence/presence, health condition, etc.) are linked to the occurrence records (De Pooter et al. (2017). This change from a species presence system to embrace any type of measurements structured around the sampling event makes OBIS a much more powerful system to support biological and ecosystem monitoring. In 1 year, OBIS already received 8 million measurements and sampling facts.
A truly effective global coral reef health status reporting mechanism will require tools to improve quality control procedures and to ensure data consistency and interoperability, across different regional observing networks. Data need to be collected once and used many times. Online portals will improve easy access to and visualization of data on coral reefs and will support research and modeling of future reef conditions, and reporting on international obligations relevant to coral reefs. This will also require efforts in training people in data management practices, applying international data standards, and quality control procedures. This can be done through both online and on-site training courses such as those organized by regional training centres under IOC's OceanTeacher Global Academy (OTGA, www.oceanteacher.org). The Ocean Best Practices portal (www.oceanbestpractices.org) provides a gateway to a suite of Standard Operating Procedures and best practice documents that researchers can refer to to ensure that their data are collected and archived with appropriate metadata that supports their re-use (Przeslawski et al., 2019).
Incentives for sharing data will have an important influence on the rate of uptake. Acknowledgment and citation of contributors are central elements for recognizing intellectual property, and justifying funding. Funders and infrastructure providers are increasingly requiring that data collected under their programs are made publicly available and that their contribution is recognized. Many global processes do not use data that are not publicly available as this undermines transparency resulting in loss of impact for the researcher, funder, and infrastructure provider. Groups who have made the effort to share their data have had far more influence on global processes, such as the Convention of Biological Diversity's Ecologically and Biologically Significant Area program, than those unwilling or unable to share their data (Bax et al., 2016). Conversely withholding data can undermine national and international agreements and prevent or delay progress toward conservation targets and sustainable use of resources (Costello et al., 2013).
Some of the most successful sharing programmes in coral reef monitoring, measured by the prominence of the publications based on their shared data, include the GCRMN, Reef Life Survey and Reef Check (e.g., Wilkinson, 2002, 2004, 2008; Stuart-Smith et al., 2017; Sully et al., 2019). But these are still relatively hierarchical, where lead researchers make the most of the shared data. As the promise of automated image analysis becomes a reality, there will be an increasing gap between data collection and its analysis, use, reanalysis, and reuse, which will require greater attention to data sharing protocols. Broader incentives for data sharing processes will come when: (a) any contributor gains benefits by accessing the data shared by their peers, whether it is to produce scientific publications, technical reports, education or communications outputs; or (b) where any external user may access the data for their purposes. The development of new, or use of existing, portals to link and share coral reef data with other datasets and resources will facilitate sharing. Existing standards and tools for sharing data can be applied, such as through the use of digital objective identifiers for datasets, implementation of FAIR principles (https://www.go-fair.org/fair-principles), or open access licenses (e.g., Creative Commons).
The Horizon—Where Will Reef Monitoring be in 10 Years?
While it is not possible to anticipate or predict all innovations that may occur in coral reef monitoring over the next 10 years, the following are imminent developments:
Monitoring for Impact
• A well-balanced monitoring network of site-based efforts integrated at regional/global levels so that local management can have data that are useful for their site management, and regional/global trends can be understood.
• improved data-reporting pipelines adopting indicators used to monitor progress against CBD, Agenda 2030 and other targets, to influence national decisions affecting coral reefs.
• improved coordination and scope of capacity development and technology transfer to deliver local benefits while building the global systems.
Integration/Coordination of Data Streams in a Single Global Network
• Within the GCRMN, but applicable to other monitoring networks, expanding coverage of level 1, and transition to levels 2 and 3 data, from local to global scales.
• a coordinated network incorporating data/inputs from diverse contributors, filling gaps, addressing challenges, and opportunities, filling the immediate priority needs for funding, capacity building, and documenting successes.
• integration of EOVs, EBVs, and other variables systems (biological, physical, climate, others) including GOOS/MBON, sensor systems, and specialized networks (e.g., I-CREOS, CREWS, GOA-ON).
• integrated, persistent monitoring using satellite, drone and in-situ unmanned vehicles, dealing with multiple resolutions (spatial), benthic discrimination, human dimensions, and involving heterogenous teams across disciplines and stakeholder groups.
Specialized Tools That May Become Standard Practice Within Years
• automated image collection and processing.
• 3D and other structure from motion image processing techniques.
• Baited Remote Underwater Video (BRUVs), including stereo applications for benthic fish and habitat structure, and for pelagic fish.
• acoustic/soundscape monitoring for coral reef health.
• coordinated multibeam acoustic surveys for exploratory surveys, mapping, and monitoring reef habitats.
• application of AUVs to multiple tasks, e.g., impacts of invasive species, coral reef structural complexity, demersal fish, and assessing diversity in reef communities.
Human Dimensions Monitoring
• Socio-economic, pressure, and human well-being variables measured at local reef dependent communities and at increasing scales.
• Socioeconomic monitoring established, conducted, and supported at the same level as biophysical monitoring.
• Social scientists being included in the strategic design and planning of monitoring activities to the same extent as natural scientists.
• Integrated monitoring established with observations that allow for better understanding of the interactions between social and ecological systems.
Summary and Key Recommendations
Significant advances have been made since OceanObs'09 (Brainard et al., 2011), though not all in the ways anticipated. In 2020, producing the global coral reef status report of the GCRMN will achieve the foundations for a global, integrated reporting, and analysis of monitoring data. Four key recommendations are synthesized as follows:
• develop the next-phase EOVs (algae, fish) and new EBVs (e.g., genetic, trait, etc) to catch up with coral cover and composition, and advance all to allow nuanced analyses relevant to understanding and managing reefs from local to national levels;
• strengthen GCRMN to incorporate the key aspects of data stewardship, accessibility and interoperability, to support visualization, modeling, and decision support;
• accelerate the advancement of remote sensing instruments to collect high radiometric quality data at high spectral resolution, relatively high spatial resolution, and at high temporal frequency;
• invest in methods, instruments, and data that can be applied by local host country stakeholders and managers, who can use the data for locally-relevant research and management.
The urgency for these improvements is high, with the very existence of coral reefs threatened by the gap between needed and committed actions to reduce greenhouse gas emissions to achieve the Paris Agreement target of limiting warming to <2°C and preferably 1.5°C. While these are viewed as aspirational in the Paris Agreement, they are recognized to be necessary to prevent the loss of 90–99% of coral reefs worldwide (Frieler et al., 2013; Heron et al., 2017, 2018).
To achieve the above by the end of the coming decade in concert with the Sustainable Development Goals and UN Decade on Ocean Science for Sustainability, we identify key areas in which expanded support is urgently needed.
Financial Support
• Engage business community through generation of innovative financial instruments, such as blue bonds, to protect, and restore coral reefs.
• Expand the community of people that relate to, feel responsible for, and might invest in coral reefs to include all major actors to support reef recovery.
Non-monetary Support
• Going the “last mile” for end-user access by ensuring that big data and automated analysis resources are available to developing country teams easily and freely.
• Development of regional capacity centers for no- or low- cost processing of biological samples including images, tissue samples for genetics, disease, etc.
• Automated image analysis using standardized categories and vocabularies.
• A global repository of data, or regional repositories of interoperable data (and to include a library of images) that are also findable and usable through OBIS.
Communication and Support
• There needs to be a balance and validation between the needs for global observations and site-based monitoring. This is particularly important for socioeconomic monitoring. Local managers and stakeholders need information that will allow them to better manage the site/area they are responsible for or have interests in, and to be able to track management effectiveness and contribution to changes.
• Communicate status and trends of coral reefs, including recovery if detected, to the broader public to keep the emphasis on coral reef monitoring and management.
Human Capacity and Skills
• There is a need to provide regular, sustained training to fill capacity-gaps in many developing countries such that local authorities have sufficient knowledge and access to infrastructure to generate, understand, and use available data/information.
• Make use of the Ocean Teacher Global Academy (OTGA) platform to develop a training course on coral reef monitoring focused on EOVs, that goes from data collection to data analysis and management, allowing for real-time, on-demand training.
• Improved coordination and priority-setting for capacity development and technical transfer, perhaps through the developing IOC Clearing House Mechanism.
• Exchange visits between institutions to increase sharing of information as well as building capacity of personnel.
Complexities Involved With Monitoring a Multi-Dimensional System
• To make available a set of standard and internationally recognized protocols or “Standard Operating Procedures” with different levels of complexity that can be used as fit for purpose (e.g., budget, capabilities, specific questions) in the Ocean Best Practices platform.
• Improved linkage and communication between automated monitoring technologies—remote, aerial, surface, sub-surface, and human observers.
• The human dimensions of coral reef monitoring and management need to be better accounted for and acted on, and narrow the gap between socioeconomic monitoring and biophysical monitoring.
• Relationships, not just dependence or pressures/drivers, between people and reefs, ecosystem services and human well-being, need to be observed and addressed.
• Integrated monitoring needs to expand beyond biophysical components to include socioeconomic and human dimensions of reefs to allow for more holistic understanding of the ecosystem changes and their causes. Social-ecological system frameworks (Kittinger et al., 2012) that emphasize the links between biophysical and human systems can help this.
• Community-based monitoring should be better supported and integrated with more detailed monitoring programs to extend data generated for management and for its role in building public support for coral reef conservation.
Link Between Research and Management/Policy Needs
• Greater focus on data and products that are suitable and necessary for management at all levels, and not just on expanding streams of more and larger datasets. Linked to this is the need for improving computing infrastructure and resolving bandwidth limitations, especially in developing countries.
• In policy circles, expand status-response models to include social-ecological systems and ecosystem-based management (EBM) approaches, to examine two-way and non-linear relationships between people and coral reefs. This takes into consideration multiple causal pathways, people's stewardship of resources and interconnectedness and feedback loops of the different systems.
• Feedback of research findings to all governance systems including the local/community governance units. This has proven useful in supporting decision making process and increasing involvement of local communities in coral reef conservation.
• Increase participation of science and management experts in UN statistical committees to improve the definition and validity of indicators used to report progress against global agreements and conventions.
Author Contributions
DO conceived the paper, wrote major sections, and coordinated co-author contributions. All other authors contributed sections of the manuscript and/or significantly edited manuscript text.
Conflict of Interest Statement
The authors declare that the research was conducted in the absence of any commercial or financial relationships that could be construed as a potential conflict of interest.
The Reviewer JD declares an ongoing collaboration on a project with the author FM-K as a contribution to the collaboration on OceanObs19. The peer review was handled under the close supervision of the Chief Editors to ensure an objective process.
Acknowledgments
This paper benefited from the financial support to the authors provided by their institutions and the grants for research, monitoring and/or coordination they have secured. The development of this manuscript has been supported through ongoing work of the Global Coral Reef Monitoring Network and the Global Ocean Observing System's Biology and Ecosystems Panel, supported by the International Coral Reef Initiative, United Nations Environment Programme, the United Nations Educational, Scientific and Cultural Organization's Intergovernmental Oceanographic Commission, and Future Earth among others.
Supplementary Material
The Supplementary Material for this article can be found online at: https://www.frontiersin.org/articles/10.3389/fmars.2019.00580/full#supplementary-material
References
Aeby, G. S., Hutchinson, M., and MacGowan, P. (2008). Hawaii's Rapid Response Contingency Plan for Events of Coral Bleaching, Disease or Crown-of Thorns Starfish Outbreaks. Hawaii Division of Aquatic Resources, 85.
Ampou, E. E., Ouillon, S., Iovan, C., and Andréfouët, S. (2018). Change detection of Bunaken Island coral reefs using 15 years of very high resolution satellite images: a kaleidoscope of habitat trajectories. Mar. Pollut. Bull. 131, 83–95. doi: 10.1016/j.marpolbul.2017.10.067
Andréfouët, S., Hochberg, E., Chevillon, C., Muller-Karger, F. E., Brock, J.C, and Hu, C. (2005a). “Multi-scale remote sensing of coral reefs,” in Remote Sensing of Coastal Aquatic Environments: Technologies, Techniques and Application, eds R. L. Miller, C. E. Del Castillo, and B. A. McKee (Springer), 297–315. doi: 10.1007/1-4020-3100-9_13
Andréfouët, S., Muller-Karger, F. E., Robinson, J. A., Kranenburg, C. J., Torres-Pulliza, D., Spraggins, S., et al. (2005b). “Global assessment of modern coral reef extent and diversity for regional science and management applications: a view from space,” in Proceedings of the 10th International Coral Reef Symposium. 28 June-2 July 2004 (Okinawa).
Andréfouët, S., Muller-Karger, F. E., Robinson, J. A., Kranenburg, C. J., Torres-Pulliza, D., Spraggins, S. A., et al. (2006). “Global assessment of modern coral reef extent and diversity for regional science and management applications: a view from space,” in Proceedings of 10th International Coral Reef Symposium, 1732–1745.
Barnes, B. B., Hallock, P., Hu, C., Muller-Karger, F., Palandro, D. A., Walter, C., et al. (2015). Prediction of coral bleaching in the Florida Keys using remotely sensed data. Coral Reefs. 34:2. doi: 10.1007/s00338-015-1258-2
Bax, N. J., Appeltans, W., Brainard, R., Duffy, J. E., Dunstan, P., Hanich, Q., et al. (2018). Linking capacity development to GOOS monitoring networks to achieve sustained ocean observation. Front. Mar. Sci. 5:346. doi: 10.3389/fmars.2018.00346
Bax, N. J., Cleary, J., Donnelly, B., Dunn, D. C., Dunstan, P. K., Fuller, M., et al. (2016). Results of efforts by the Convention on Biological Diversity to describe ecologically or biologically significant marine areas. Conserv. Biol. 30, 571–581. doi: 10.1111/cobi.12649
Beck, M. W., Losada, I. J., Menéndez, P., Reguero, B. G., Díaz-Simal, P., and Fernández, F. (2018). The global flood protection savings provided by coral reefs. Nat. Commun. 9:2186. doi: 10.1038/s41467-018-04568-z
Beeden, R., Maynard, J. A., Marshall, P. A., Heron, S. F., and Willis, B. L. (2012). A framework for responding to coral disease outbreaks that facilitates adaptive management. Environ. Manage. 49, 1–13. doi: 10.1007/s00267-011-9770-9
Beijbom, O., Edmunds, P. J., Roelfsema, C., Smith, J., Kline, D. I., Neal, B. P., et al. (2015). Towards automated annotation of benthic survey images : variability of human experts and operational modes of automation. PLoS ONE 10:e0130312. doi: 10.1371/journal.pone.0130312
Bertucci, F., Parmentier, E., Lecellier, G., Hawkins, A. D., and Lecchini, D. (2016). Acoustic indices provide information on the status of coral reefs: an example from Moorea Island in the South Pacific. Sci. Rep. 6:33326. doi: 10.1038/srep33326
Beyer, H. L., Kennedy, E. V., Beger, M., Chen, C. A., Cinner, J. E., Darling, E. S., et al. (2018). Risk-sensitive planning for conserving coral reefs under rapid climate change. Conserv. Lett. 109:e12587. doi: 10.1111/conl.12587
Bouchet, P., Meeuwig, J., Huveneers, C., Langlois, T., Letessier, T., Lowry, M., et al. (2018). “Marine sampling field manual for pelagic BRUVS (Baited Remote Underwater Videos),” in Field Manuals for Marine Sampling to Monitor Australian Waters, eds R. Przeslawski and S. Foster (National Environmental Science Programme (NESP)), 105-132.
Bourne, D., Ainsworth, T., Pollock, J., and Willis, B. (2014). Towards a better understanding of white syndrome and their causes on Indo_Pacific coral reefs. Coral Reefs 34, 233–242. doi: 10.1007/s00338-014-1239-x
Brainard, R., Bainbridge, S., Brinkman, R., Eakin, C. M., Field, M., Gattuso, J.-P., et al. (2011). “An international network of coral reef ecosystem observing systems (I-CREOS),” in Conference: OceanObs'09: Sustained Ocean Observations and Information for Society. doi: 10.5270/OceanObs09.cwp.09
Bruno, J. F., and Selig, E. R. (2007). Regional decline of coral cover in the indo-pacific: timing, extent, and subregional comparisons. PLoS ONE 2:e711. doi: 10.1371/journal.pone.0000711
Bunce, L., and Pomeroy, R. (2003). Socioeconomic Monitoring Guidelines for Coastal Managers in the Caribbean. World Commission of Protected Areas & Australian Institute of Marine Science.
Bunce, L., Townsely, P., Pomeroy, R., and Pollnac, R. (2000). Socioeconomic Manual for Coral Reef Management. Townsville, QLD: Australian Institute of Marine Science and IUCN.
Burke, L., Bryant, D., McManus, J., and Spalding, M. (2008). Reefs at Risk. World Resources Institute (WRI), 56.
Burke, L. K., Reytar, M. S., and Spalding, M. (2011). Reefs at Risk Revisited. Washington, DC: World Resources Institute, 130.
Burns, J., Delparte, D., Gates, R., and Takabayashi, M. (2015). Integrating structure-from-motion photogrammetry with geospatial software as a novel technique for quantifying 3D ecological characteristics of coral reefs. PeerJ 3:e1077. doi: 10.7717/peerj.1077
Butler, J., Butler, M. J., and Gaff, H. (2017). Snap, crackle, and pop: acoustic-based model estimation of snapping shrimp populations in healthy and degraded hard-bottom habitats. Ecol. Indic. 77, 377–385. doi: 10.1016/j.ecolind.2017.02.041
Casella, E., Collin, A., Harris, D., Ferse, S., Bejarano, S., Parravicini, V., et al. (2016). Mapping coral reefs using consumer-grade drones and structure from motion photogrammetry techniques. Coral Reefs 36:1. doi: 10.1007/s00338-016-1522-0
Chirayath, V., and Earle, S. (2016). Drones that see through waves - developing new tools in coastal marine conservation. Aquat. Conserv. Mar. Freshw. Ecosyst. 26, 237–250. doi: 10.1002/aqc.2654
Costello, M. J., Michener, W. K., Gahegan, M., Zhang, Z.-Q., and Bourne, P. E. (2013). Biodiversity data should be published, cited, and peer reviewed. Trends Ecol. Evol. 28, 454–461. doi: 10.1016/j.tree.2013.05.002
Daszak, P., Cunningham, A., and Hyatt, A. (2000). Emerging infectious diseases of wildlife– threats to biodiversity and human health. Science 287, 443–449. doi: 10.1126/science.287.5452.443
De Pooter, D., Appeltans, W., Bailly, N., Bristol, S., Deneudt, K., Eliezer, M., et al. (2017). Toward a new data standard for combined marine biological and environmental datasets - expanding OBIS beyond species occurrences. Biodivers. Data J. 5:e10989. doi: 10.3897/BDJ.5.e10989
Díaz-Pérez, L., Rodríguez-Zaragoza, F. A., Ortiz, M., Cupul-Magaña, A. L., Carriquiry, J. D., Ríos-Jara, E., et al. (2016). Coral reef health indices versus the biological, ecological and functional diversity of fish and coral assemblages in the Caribbean sea. PLoS ONE 11:e0161812. doi: 10.1371/journal.pone.0161812
Dobson, A., and Foufopoulos, J. (2001). Emerging infectious pathogens of wildlife. Philos. Trans. R. Soc. Lond. B Biol. Sci. 356, 1001–1012. doi: 10.1098/rstb.2001.0900
Eakin, M. C., Carl Nim, J., Russell Brainard, E., Christoph, A, Chris, E, Dwight Gledhill, K., et al. (2010). “Monitoring coral reefs from space,” in The Oceanography Society Magazine, Special Volume: The Future of Oceanography From Space. December 2010. 119–133. doi: 10.5670/oceanog.2010.10
Edgar, G. J., Banks, S. A., Bessudo, S., Cortés, J., Guzmán, H. M., Henderson, S., et al. (2011). Variation in reef fish and invertebrate communities with level of protection from fishing across the Eastern Tropical Pacific seascape. Global Ecol. Biogeogr. 20, 730–743. doi: 10.1111/j.1466-8238.2010.00642.x
Edgar, G. J., and Barrett, N. S. (2012). An assessment of population responses of common inshore fishes and invertebrates following declaration of five Australian marine protected areas. Environ. Conserv. 39, 271–281. doi: 10.1017/S0376892912000185
Edgar, G. J., and Stuart-Smith, R. D. (2014). Systematic global assessment of reef fish communities by the Reef Life Survey program. Sci. Data 1:140007. doi: 10.1038/sdata.2014.7
Edwards, C. B., Eynaud, Y., Williams, P., Pedersen, N. E., Zglicynski, B. J, Gleason, A. C. R., et al. (2017). Large-area imaging reveals biologically driven non-random spatial patterns of corals at a remote reef. Coral Reefs 36:1291. doi: 10.1007/s00338-017-1624-3
Ferrari, R., Bryson, M., Bridge, T., Hustache, J., Williams, S. B., Byrne, M., et al. (2016). Quantifying the response of structural complexity and community composition to environmental change in marine communities. Global Change Biol. 22, 1965–1975. doi: 10.1111/gcb.13197
Ferreira, P. A., Pinto, J., Dias, J., Sousa, P. J., and Lourenco, P. (2017). Rapid Environmental Picture Atlantic exercise 2016. Field Report. 1–7. doi: 10.1109/OCEANSE.2017.8084800
Fletcher, P. J., Kelble, C. R., Nuttle, W. K., and Kiker, G. A. (2014). Using the integrated ecosystem assessment framework to build consensus and transfer information to managers. Ecol. Indic. 44, 11–25. doi: 10.1016/j.ecolind.2014.03.024
Fletcher, P. J., Spranger, M., Hendee, J. C., Li, Y., Clark, M., and Kiker, G. A. (2015). Decision tools for coral reef managers: using participatory decision support to integrate potential climate impacts and informed decision making. Global Ecol. Conserv. 4, 491–504. doi: 10.1016/j.gecco.2015.09.003
Freeman, L. A., and Freeman, S. E. (2016). Rapidly obtained ecosystem indicators from coral reef soundscapes. Mar. Ecol. Prog. Ser. 561, 69–82. doi: 10.3354/meps11938
Frieler, K., Meinshausen, M., Golly, A., Mengel, M., Lebek, K., Don- ner, S. D., et al. (2013). Limiting global warming to 2 degrees C is unlikely to save most coral reefs. Nat. Climate Change 3, 165–170. doi: 10.1038/nclimate1674
GCRMN (2018a). GCRMN Implementation and Governance Plan. International Coral Reef Initiative (ICRI).
Gordon, T. A. C., Harding, H. R., Wong, K. E., Merchant, N. D., Meekan, M. G., McCormick, M. I., et al. (2018). Habitat degradation negatively affects auditory settlement behavior of coral reef fishes. Proc. Natl. Acad. Sci. 115, 5193–5198. doi: 10.1073/pnas.1719291115
Gramer, L. J., Hendee, J. C., Thompson, N. B., and Fletcher, P. (2016). “Better living through physics: mapping reef resilience with site-specific ecological forecasts,” in Proc 13th Int Coral Reef Symp (Honolulu, HI).
Grasmueck, M., Eberli, G. P., Viggiano, D. A., Correa, T., Rathwell, G., and Luo, J. (2006). Autonomous underwater vehicle (AUV) mapping reveals coral mound distribution, morphology, and oceanography in deep water of the Straits of Florida. Geophys. Res. Lett. 33, 1–6. doi: 10.1029/2006GL027734
Gudka, M., and Obura, D. O. (2017). “The 2016 coral bleaching event in the Western Indian Ocean – overview,” in Coral reef status report for the Western Indian Ocean. Global Coral Reef Monitoring Network (GCRMN)/International Coral Reef Initiative (ICRI), eds D. O. Obura, et al., 22–37.
Harvell, C. D., Jordan-Dahlgren, E., Merkel, S., Rosenberg, E., Raymundo, L., Smith, G., et al. (2007). Coral disease, environ- mental drivers, and the balance between coral and microbial associates. Oceanography 20, 172–195. doi: 10.5670/oceanog.2007.91
Harvell, C. D., Kim, K., Burkholder, J., Coldwell, R. R., Epstein, P. R., Grimes, D. J., et al. (1999). Emerging ma- rine diseases: climate links and anthropogenic factors. Science 285, 1505–1510. doi: 10.1126/science.285.5433.1505
Hedley, J. D., Chris, R, Vittorio, B, Claudia1, G, Tiit, K, Stuart, P., et al. (2018). Coral reef applications of Sentinel-2: coverage, characteristics, bathymetry and benthic mapping with comparison to Landsat 8. Remote Sens. Environ. 216, 598–614. doi: 10.1016/j.rse.2018.07.014
Hendee, J., Gramer, L., Heron, S., Jankulak, M., Amornthammarong, N., Shoemaker, M., et al. (2012). “Wireless architectures for coral reef environmental monitoring,” in Proceedings of the 12th International Coral Reef Symposium (Cairns).
Hendee, J., Gramer, L., Manzello, D., and Jankulak, M. (2008). “Ecological forecasting for coral reef ecosystems,” Proc 11th Int Coral Reef Symp (Ft. Lauderdale, FL), 534–538.
Heron, S. C., Eakin, C. M., Douvere, F., Anderson, K., Day, J. C., Geiger, E., et al. (2017). Impacts of Climate Change on World Heritage Coral Reefs: A First Global Scientific Assessment. Paris: UNESCO World Heritage Centre.
Heron, S. C., van Hooidonk, R., Maynard, J., Anderson, K., Day, J. C., Geiger, E., et al. (2018). Impacts of Climate Change on World Heritage Coral Reefs: Update to the First Global Scientific Assessment. Paris: UNESCO World Heritage Centre.
Hodgson, G. (1999). A global assessment of human effects on coral reefs. Mar. Poll. Bull. 38:345. doi: 10.1016/S0025-326X(99)00002-8
Hodgson, G. J., Kiene, W., Maun, L., Mihaly, J., Liebeler, J., Shuman, C., et al. (2016). Reef Check Instruction Manual: A Guide to Reef Check Coral Reef Monitoring. Marina Del Rey, CA: Reef Check Foundation, 86.
Hoegh-Guldberg, O. (2015). Reviving the Ocean Economy: the Case for Action - 2015. Geneva: WWF International, Gland, 60.
Hoegh-Guldberg, O., Poloczanska, E. S., Skirving, W., and Dove, S. (2017). Coral reef ecosystems under climate change and ocean acidification. Front. Mar. Sci. 4:158. doi: 10.3389/fmars.2017.00158
Hughes, T. P., Barnes, M. L., Bellwood, D. R., Cinner, J. E., Cumming, G. S., Jackson, J. B. C., et al. (2017). Coral reefs in the Anthropocene. Nature 546, 82–90. doi: 10.1038/nature22901
IAEA (2015). Ocean Acidification Impacts on Coastal Communities - Bridging the Gap Between Ocean Acidification and Economic Valuation. Report from the Third International Workshop. Oceanographic Museum, Principality of Monaco. January 2015. International Atomic Energy Association (IAEA)/Ocean Acidification International Coorination Center OA-ICC, 32.
ICRI (2018). Proceedings of the 33rd General meeting, of the International Coral Reef Initiative. Monaco, 5–7.
IPBES (2019). “Summary for policymakers of the global assessment report on biodiversity and ecosystem services,” in Intergovernmental Science-Policy Platform on Biodiversity and Ecosystem Services, eds S. Díaz, J. Settele, E. S. Brondizio E.S., H. T. Ngo, M. Guéze, J. Agard, A. Arneth, P. Balvanera, K. A. Brauman, S. H. M. Butchart, K. M. A. Chan, L. A. Garibaldi, K. Ichii, J. Liu, S. M. Subramanian, G. F. Midgley, P. Miloslavich, Z. Molnár, D. Obura, A. Pfaff, S. Polasky, A. Purvis, J. Razzaque, B. Reyers, R. Roy Chowdhury, Y. J. Shin, I. J. Visseren-Hamakers, K. J. Willis, and C. N. Zayas (Bonn: IPBES secretariat), 39.
IPCC (2018). “Global warming of 1.5°C,” in An IPCC Special Report on the Impacts of Global Warming of 1.5°C Above Pre-Industrial Levels and Related Global Greenhouse Gas Emission Pathways, in the Context of Strengthening the Global Response to the Threat of Climate Change, Sustainable Development, and Efforts to Eradicate Poverty, eds V. Masson-Delmotte, P. Zhai, H. O. Pörtner, D. Roberts, J. Skea, P. R. Shukla, A. Pirani, W. Moufouma-Okia, C. Péan, R. Pidcock, S. Connors, J. B. R. Matthews, Y. Chen, X. Zhou, M. I. Gomis, E. Lonnoy, T. Maycock, M. Tignor, and T. Waterfield.
Jackson, J. B. C., Donovan, M., Cramer, K., and Lam, V. (2014). Status and Trends of Caribbean Coral Reefs: 1970-2012. GCRMN/ICRI/UNEP/IUCN, 245.
James, L. C., Marzloff, M. P., Barrett, N., Friedman, A., and Johnson, C. R. (2017). Changes in deep reef benthic community composition across a latitudinal and environmental gradient in temperate Eastern Australia. Mar. Ecol. Prog. Ser. 565, 35–52. doi: 10.3354/meps11989
Jokiel, P. L, Rodgers, K. S., Brown, E. K., Kenyon, J. C., Aeby, G., Smith, W. R., et al. (2015). Comparison of methods used to estimate coral cover in the Hawaiian Islands. PeerJ 3:e954. doi: 10.7717/peerj.954
Kaplan, M. B., Lammers, M. O., Zang, E., and Mooney, T. A. (2018). Acoustic and biological trends on coral reefs off Maui, Hawaii. Coral Reefs 37, 121–133. doi: 10.1007/s00338-017-1638-x
Kaplan, M. B., Mooney, T. A., Partan, J., and Solow, A. R. (2015). Coral reef species assemblages are associated with ambient soundscapes. Mar. Ecol. Prog. Ser. 533, 93–107. doi: 10.3354/meps11382
Kelble, C. R., Loomis, D. K., Lovelace, S., Nuttle, W. K., Ortner, P. B., Fletcher, P., et al. (2013). The EBM-DPSER conceptual model: integrating ecosystem services into the DPSIR framework. PLoS ONE 2013:e70766. doi: 10.1371/journal.pone.0070766
Kennedy, E. V., Holderied, M. W., Mair, J. M., Guzman, H. M., and Simpson, S. D. (2010). Spatial patterns in reef-generated noise relate to habitats and communities: evidence from a Panamanian case study. J. Exp. Mar. Bio. Ecol. 395, 85–92. doi: 10.1016/j.jembe.2010.08.017
Kittinger, J. N., Finkbeiner, E. M., Glazier, E. W., and Crowder, L. B. (2012). Human dimensions of coral reef social-ecological systems. Ecol. Soci. 17:17. doi: 10.5751/ES-05115-170417
Knowlton, N., Brainard, R. E., Fisher, R., Moews, M., Plaisance, L., and Caley, M. J. (2010). “Coral reef biodiversity,” in Life in the World's Ocean: Diversity, Distribution, and Abundance, ed A. McIntyre (Wiley-Blackwell), 65–78. doi: 10.1002/9781444325508.ch4
Koparan, C., Koc, A. B., Privette, V. C., and Sawyer, C. B. (2018). In situ water quality measurements using an unmanned aerial vehicle (UAV) system. Water 10:264. doi: 10.3390/w10030264
Langlois, T., Williams, J., Monk, J., Bouchet, P., Currey, L., Goetze, J., et al. (2018). “Marine sampling field manual for benthic stereo BRUVS (Baited Remote Underwater Videos),” in Field Manuals for Marine Sampling to Monitor Australian Waters, eds R. Przeslawski and S. Foster (National Environmental Science Programme (NESP)), 82–104.
Levy, J., Hunter, C., Lukacazyk, T., and Franklin, E. C. (2018). Assessing the spatial distribution of coral bleaching using small unmanned aerial systems. Coral Reefs 37, 373–387. doi: 10.1007/s00338-018-1662-5
Lillis, A., and Mooney, T. A. (2018). Snapping shrimp sound production patterns on Caribbean coral reefs: relationships with celestial cycles and environmental variables. Coral Reefs 37, 597–607. doi: 10.1007/s00338-018-1684-z
Ling, S. D., Mahon, I., Marzloff, M. P., Pizarro, O., Johnson, C. R., and Williams, S. B. (2016). Stereo-imaging AUV detects trends in sea urchin abundance on deep overgrazed reefs. Limnol. Oceanogr. Methods 14, 293–304. doi: 10.1002/lom3.10089
Lirman, D., Schopmeyer, S., Manzello, D., Gramer, L. J., Precht, W. F., Muller-Karger, F., et al. (2011). Severe 2010 cold-water event caused unprecedented mortality to corals of the florida reef tract and reversed previous survivorship patterns. PLoS ONE 6:e23047. doi: 10.1371/journal.pone.0023047
Liu, G., Scott Heron, F., Mark Eakin, C., Frank Muller-Karger, F., Maria Vega-Rodriguez, S., De La Jacqueline, L., et al. (2014). Reef-scale thermal stress monitoring of coral ecosystems: New 5-km global products from NOAA coral reef watch. Remote Sens. 6, 11579–11606. doi: 10.3390/rs61111579
Lucieer, V., Picard, K., Siwabessy, J., Jordan, A., Tran, M., and Monk, J. (2018). “Seafloor mapping field manual for multibeam sonar,” in Field Manuals for Marine Sampling to Monitor Australian Waters, eds R. Przeslawski and S. Foster (National Environmental Science Programme (NESP)), 42–64.
Magris, R. A., Treml, E. A., Pressey, R. L., and Weeks, R. (2015). Integrating multiple species connectivity and habitat quality into conservation planning for coral reefs. Ecography 38, 1–16. doi: 10.1111/ecog.01507
Marshall, P., and Schuttenberg, H. (2006). A Reef Manager's Guide to Coral Bleaching. NOAA/GBRMPA/IUCN, 178.
Maynard, J., van Hooidonk, R., Eakin, C. M., Puotinen, M., Garren, M., Williams, G., et al. (2015). Projections of climate conditions that increase coral disease susceptibility and pathogen abundance and virulence. Nat. Clim. Chan. 5, 688–694. doi: 10.1038/nclimate2625
Maynard, J. A., Anthony, K. R. N., Harvell, C. D., Burgman, M. A., Beeden, R., Sweatman, H., et al. (2011). Predicting outbreaks of a climate-driven coral disease in the Great Barrier Reef. Coral Reefs 30, 485–495. doi: 10.1007/s00338-010-0708-0
McCarthy, M. J., Kaitlyn Colna, E., Mahmoud El-Mezayen, M., Abdiel Laureano-Rosario, E., Méndez-Lázaro, P., Frank Muller-Karger, E., et al. (2017). Satellite remote sensing for coastal management: a review of successful applications. Environ. Manage. 60, 323–339. doi: 10.1007/s00267-017-0880-x
Miloslavich, P., Bax, N. J., and Simmons, S. E. (2018a). Essential ocean variables for global sustained observations of biodiversity and ecosystem changes. Glob. Change Biol. 2018, 1–18. doi: 10.1111/gcb.14108
Miloslavich, P., Seeyave, S., Muller-Karger, F., Bax, N., Ali, E., Delgado, C., et al. (2018b). Challenges for global ocean observation: the need for increased human capacity. J. Operat. Oceanogr. 2018, 1–20. doi: 10.1080/1755876X.2018.1526463
Miloslavich, P., Tom, W., Paul, S., Edward Vanden, B., Kristin, K., Patrick, H. N., et al. (2016). “Extent of assessment of marine biological diversity,” in The First Global Integrated Marine Assessment - World Ocean Assessment I). Chapter 35. (Coordinators) Lorna Inniss and Alan Simcock The Group of Experts of the Regular Process. C. United Nations. Available online at: http://www.un.org/Depts/los/global_reporting/WOA_RegProcess.htm
Monk, J., Barrett, N., Bridge, T., Carroll, A., and Friedman, A. (2018). 4. Marine Sampling Field Manual for Auvs (Autonomous Underwater Vehicles). Nespmarine. Edu. Au. 2018, 65–81.
Moore, F., and Best, B. (2001). “Coral reef crisis: causes and consequences,” in Papers Presented at a Symposium held at the 2001 Annual Meeting of the American Association for the Advancement of Science (New York, NY).
Moritz, C., Vii, J., Lee Long, W., Tamelander, J., Thomassin, A., and Planes, S. (eds.). (2018). Status and Trends of Coral Reefs of the Pacific. Global Coral Reef Monitoring Network, 220. Available online at: www.gcrmn.net
Moses, C. S., Serge, A., Christine, K. J., and Frank, E. (2009). Regional estimates of reef carbonate dynamics and productivity using Landsat 7 ETM+, and potential impacts from ocean acidification. Mar. Ecol. Prog. Ser. 380, 103–115. doi: 10.3354/meps07920
Muller-Karger, F., Roffer, M., Walker, N., Oliver, M., Schofield, O., Abbott, M., et al. (2013). Satellite remote sensing in support of an integrated ocean observing system. Geosci. Remote Sens. Magaz. 1:18. doi: 10.1109/MGRS.2013.2289656
Muller-Karger, F. E., Erin, H., Christiana, A., Turpie, K., Roberts, D. A., Siegel, D., et al. (2018a). Satellite sensor requirements for monitoring essential biodiversity variables of coastal ecosystems. Ecol. Appl. 28, 749–760. doi: 10.1002/eap.1682
Muller-Karger, F. E., Miloslavich, P., Bax, N., Simmons, S. E., Costello, M. J., Pinto, I. S., et al. (2018b). Advancing marine biological observations and data requirements of the complementary essential ocean variables (EOVs) and essential biodiversity variables (EBVs) frameworks. Front. Mar. Sci. 5:211. doi: 10.3389/fmars.2018.00211
Mumby, P. J., and Alasdair Edwards, J. (2002). Mapping marine environments with IKONOS imagery: enhanced spatial resolution can deliver greater thematic accuracy. Remote Sens. Environ. 82, 248–257. doi: 10.1016/S0034-4257(02)00041-X
Naidu, R., Muller-Karger, F., and McCarthy, M. (2017). “Mapping of benthic habitats in Komave, Coral coast using worldview-2 satellite imagery,” in Climate Change Impacts and Adaptation Strategies for Coastal Communities. Climate Change Management, ed W. Leal Filho (Cham: Springer), 337–355. doi: 10.1007/978-3-319-70703-7_18
Nedelec, S. L., Simpson, S. D., Holderied, M., Radford, A. N., Lecellier, G., Radford, C., et al. (2015). Soundscapes and living communities in coral reefs: temporal and spatial variation. Mar. Ecol. Prog. Ser. 524, 125–135. doi: 10.3354/meps11175
Newton, J. A., Feely, R. A., Jewett, E. B., Williamson, P., and Mathis, J. (2015). Global Ocean Acidification Observing Network: Requirements and Governance Plan. Second Edition, GOA-ON. Available online at: http://www.goa-on.org/docs/GOA-ON_plan_print.pdf
Obura, D. O., Gudka, M., Abdou Rabi, F., Bacha Gian, S., Bigot, L., Bijoux, J., et al. (2017). Coral Reef Status Report for the Western Indian Ocean. Global Coral Reef Monitoring Network (GCRMN)/International Coral Reef Initiative (ICRI), 144.
Palandro, D., Andréfouët, S., Dustan, P., and Muller-Karger, F. E. (2003a). Change detection in coral reef communities using the Ikonos sensor and historic aerial photographs. Int. J. Remote Sens. 24, 873–878. doi: 10.1080/0143116021000009895
Palandro, D., Andréfouët, S., Müller-Karger, F. E., Dustan, P., Hu, C., and Hallock, P. (2003b). Detection of changes in coral reef communities using Landsat 5/TM and Landsat 7/ETM+ Data. Canad. J. Remote Sens. 29, 201–209. doi: 10.5589/m02-095
Palandro, D., Hu, C., Andréfouët, S., and Müller-Karger, F. E. (2004). Synoptic water clarity assessment in the Florida Keys using diffuse attenuation coefficient estimated from Landsat imagery. Hydrobiologia 530–531, 489–493. doi: 10.1007/s10750-004-2691-3
Parsons, M. J. G., Salgado Kent, C. P., Recalde-Salas, A., and McCauley, R. D. (2016). Fish choruses off Port Hedland, Western Australia. Bioacoustics 4622, 1–18. doi: 10.1080/09524622.2016.1227940
Patrício, J., Elliott, M., Mazik, K., Papadopoulou, K.-N., and Smith, C. J. (2016). DPSIR—two decades of trying to develop a unifying framework for marine environmental management? Front. Mar. Sci. 3:225. doi: 10.3389/fmars.2016.00177
Pereira, H. M., Ferrier, S., Walters, M., Geller, G. N., Jongman, R. H. G., Scholes, R. J., et al. (2013). Essential biodiversity variables. Science 339, 277–278. doi: 10.1126/science.1229931
Piercy, J. J. B., Codling, E. A., Hill, A. J., Smith, D. J., and Simpson, S. D. (2014). Habitat quality affects sound production and likely distance of detection on coral reefs. Mar. Ecol. Prog. Ser. 516, 35–47. doi: 10.3354/meps10986
Pieretti, N., Farina, A., and Morri, D. (2011). A new methodology to infer the singing activity of an avian community: the Acoustic Complexity Index (ACI). Ecol. Indic. 11, 868–873. doi: 10.1016/j.ecolind.2010.11.005
Precht, W. F., Gintert, B. E., Robbart, M. L., Fura, R., and van Woesik, R. (2016). Unprecedented disease-related coral mortality in Southeastern Florida. Sci. Rep. 6:31374. doi: 10.1038/srep31374
Przeslawski, R., Foster, S., Monk, J., Barrett, N., Bouchet, P., Carroll, A., et al. (2019). A suite of field manuals for marine sampling to monitor Australian waters. Front. Mar. Sci. 6. doi: 10.3389/fmars.2019.00177
Raymundo, L. J., Couch, C. S., Harvell, C. D., Raymundo, J., Bruckner, A. W., Work, T. M., et al. (2008). “Coral disease handbook guidelines for assessment, monitoring and management,” in Coral Reef Targeted Research and Capacity Building for Management Program (Brisbane, QLD).
Roelfsema, C., and Phinn, S. (2010). Integrating field data with high spatial resolution multispectral satellite imagery for calibration and validation of coral reef benthic community maps. J. Appl. Remote Sens. 4:1. doi: 10.1117/1.3430107
Secretariat of the Convention on Biological Diversity (2014). An Updated Synthesis of the Impacts of Ocean Acidification on Marine Biodiversity, Montreal, Technical Series No. 75, 99.
Seiler, J., Williams, A., and Barrett, N. (2012). Assessing size, abundance and habitat preferences of the Ocean Perch Helicolenus percoides using a AUV-borne stereo camera system. Fish. Res. 129–130, 64–72. doi: 10.1016/j.fishres.2012.06.011
Servick, K. (2014). Eavesdropping on ecosystems. Science 343, 834–837. doi: 10.1126/science.343.6173.834
Smith, J. E., Brainard, R., Carter, A., Grillo, S., Edwards, C., Harris, J., et al. (2016). Re-evaluating the health of coral reef communities: baselines and evidence for human impacts across the central Pacific. Proc. R. Soc. B 283:20151985. doi: 10.1098/rspb.2015.1985
Snelgrove, P., Vanden Berghe, E., Miloslavich, P., Bailly, N., Brandt, A., Bucklin, A., et al. (2016). Global Patterns in Marine Biodiversity. In: The First Global Integrated Marine Assessment - World Ocean Assessment). Chapter 34. (Coordinators) Lorna Inniss and Alan Simcock The Group of Experts of the Regular Process. C. United Nations. Available online at: http://www.un.org/Depts/los/global_reporting/WOA_RegProcess.htm
Soto, I., Andrefouet, S., Hu, C., Muller-Karger, F. E., Wall, C. C., Sheng, J., et al. (2009). Physical connectivity in the Mesoamerican Barrier Reef System inferred from 9 years of ocean color observations. Coral Reefs. 28, 415–425. doi: 10.1007/s00338-009-0465-0
Soto, R., Inia, M., Muller-Karger, F., Hallock, P., and Hu, C. (2011). Sea surface temperature variability in the Florida Keys and its relationship to coral cover. J. Mar. Biol. 2011, 1–10. doi: 10.1155/2011/981723
Spalding, M., Burke, L., Wood, S. A., Ashpole, J., Hutchison, J., and Ermgassen, P. (2017). Mapping the global value and distribution of coral reef tourism. Mar. Pol. 82, 104–113. doi: 10.1016/j.marpol.2017.05.014
Staaterman, E., Ogburn, M. B., Altieri, A. H., Brandl, S. J., Whippo, R., Seemann, J., et al. (2017). Bioacoustic measurements complement visual biodiversity surveys: preliminary evidence from four shallow marine habitats. Mar. Ecol. Prog. Ser. 575, 207–215. doi: 10.3354/meps12188
Staaterman, E., Paris, C. B., DeFerrari, H. A., Mann, D. A., Rice, A. N., and D'Alessandro, E. K. (2014). Celestial patterns in marine soundscapes. Mar. Ecol. Prog. Ser. 508, 17–32. doi: 10.3354/meps10911
Stal, C., Bourgeois, J., De Maeyer, P., De Mulder, G., and De Wulf, A. (2012). “Test case on the quality analysis of structure from motion in airborne applications,” in Conference: Proceedings of the 32nd EARSeL symposium ‘Advances in Geosciences'(Mykonos).
Stuart-Smith, R. D., Edgar, G. J., Barrett, N. S., Bates, A. E., Baker, S. C., Bax, N. J., et al. (2017). Assessing National Biodiversity trends for rocky and coral reefs through the integration of citizen science and scientific monitoring programs. BioScience 67, 134–146. doi: 10.1093/biosci/biw180
Sully, S., Burkepile, D. E., Donovan, M. K., Hodgson, G., and Van Woesik, R. (2019). A global analysis of coral bleaching over the past two decades. Nat. Commun. 10:1264. doi: 10.1038/s41467-019-09238-2
Tittensor, D. P., Walpole, M., Hill, S. L. L., Boyce, D. G., Britten, G. L., Burgess, N. D., et al. (2014). A mid-term analysis of progress toward international biodiversity targets. Science 346, 241–244. doi: 10.1126/science.1257484
UNE/WCMC (2016). UN Environment's World Conservation Monitoring Centre/WCMC Ocean Data Viewer. Available online at: http://data.unep-wcmc.org/datasets/1
UNEP/SPAW (2017). GCRMN-Caribbean Guidelines For Integrated Coral Reef Monitoring. Protocol Concerning Specially Protected Areas and Wildlife (SPAW) in the Wider Caribbean Region. UN Environment.
UNESCO (2012). A Framework for Ocean Observing. By the Task Team for an Integrated Framework for Sustained Ocean Observing. UNESCO2012, IOC/INF-1284.
van Hooidonk, R., Maynard, J., Tamelander, J., Gove, J., Ahmadia, G., Raymundo, L., et al. (2016). Local-scale projections of coralreef futures and implications ofthe Paris Agreement. Sci. Rep. 2016, 1–8. doi: 10.1038/srep39666
Vega-Rodriguez, M., Müller-Karger, F. E., Hallock, P., Quiles-Perez, G. A., Eakin, C. M., Colella, M., et al. (2015). Influence of water-temperature variability on stony coral diversity in Florida Keys patch reefs. Mar. Ecol. Prog. Ser. 528, 173–186. doi: 10.3354/meps11268
Wabnitz, C. C., Andréfouët, S., Frank, A., and Muller, K. (2010). Measuring progress towards global marine conservation targets. Front. Ecol. Environ. 8:109. doi: 10.1890/080109
Wabnitz, C. C., Serge, A., Torres-Pulliza, D., Muller-Karger, F. E., and Kramer, P. (2008). Regional-scale seagrass habitat mapping in the Wider Caribbean region using Landsat sensors: applications to conservation and ecology. Remote Sens. Environ. 112, 3455–3467. doi: 10.1016/j.rse.2008.01.020
Walton, C., Hayes, N., and Gilliam, D. (2018). Ipacts of a regional, multi-year, multi-species coral disease outbreak in Southeast Florida. Front. Mar. Sci. 5:323. doi: 10.3389/fmars.2018.00323
Wilkinson, C. (ed.). (2000). Status of Coral Reefs of the World: 2000. Townsville, QLD: Australian Institute of Marine Science.
Wilkinson, C. (ed.). (2002). Status of Coral Reefs of the World: 2002. Townsville, QLD: Australian Institute of Marine Science.
Wilkinson, C. (ed.). (2004). Status of Coral Reefs of the World: 2004. Volume 1. Townsville, QLD: Australian Institute of Marine Science.
Wilkinson, C. (ed.). (2008). Status of Coral Reefs of the World: 2008. Townsville, QLD: Australian Institute of Marine Science.
Wilkinson, C., Salvat, B., Eakin, C. M., Brathwaite, A., Francini-Filho, R., Webster, N., et al. (2016). “Tropical and sub-tropical coral reefs (Chapter 43),” in World Ocean Assessment, 42.
Williams, I. D, Couch, C. S., Beijbom, O., Oliver, T. A., Vargas-Angel, B., Schumacher, B. D., et al. (2019). Leveraging automated image analysis tools to transform our capacity to assess status and trends on coral reefs. Front. Mar. Sci. 2019:222. doi: 10.3389/fmars.2019.00222
Wirt, K. E., Hallock, P., Palandro, D., and Semon Lunz, K. (2015). Potential habitat of Acropora spp on reef of Florida Puerto Rico and the US Virgin Islands. Global Ecol. Conserv. 2, 242–255. doi: 10.1016/j.gecco.2014.12.001
WOA (2016). The First Global Integrated Marine Assessment World Ocean Assessment I; by the Group of Experts of the Regular Process. United Nations General Assembly and its Regular Process for Global Reporting and Assessment of the State of the Marine Environment, including Socioeconomic Aspects.
Wongbusarakum, S., and Heenan, A (2019) Integrated Monitoring with SocMon/SEM-Pasifika: Principles Process: Global Coral Reef Monitoring Network (GCRMN) Socio-economic Monitoring for Coastal Management (SocMon) Methodological Updates. Silver Spring, MD: GCRMN SocMon, NOAA Coral Reef Conservation Program.
Wongbusarakum, S., Madeira, E.M., and Hartanto, H. (2014). Strengthening the Social Impacts of Sustainable Landscapes Programs: A Practitioner's Guidebook to Strengthen and Monitor Human Well-Being Outcomes. Arlington, VA: The Nature Conservancy.
Woodley, C. M., Bruckner, A. W., McLenon, A. L., Higgins, J. L., Galloway, S. B., and Nicholson, J. H. (2008). Field Manual for Investigating Coral Disease Outbreaks. US Department of Commerce, National Oceanic, and Atmospheric Administration National Ocean Service.
Work, T., and Aeby, G. (2006). Systematically describing gross lesions in corals. Dis. Aquat. Org. 70, 155–160. doi: 10.3354/dao070155
Work, T. M., Russell, R., and Aeby, G. S. (2012). “Tissue loss (white syndrome) in the coral Montipora capitata is a dynamic disease with multiple host responses and potential causes,” in Proceedings of the Royal Society B: Biological Sciences, Vol. 279 (London: Royal Society Publishing), 8. doi: 10.1098/rspb.2012.1827
Keywords: ecological monitoring, coral reef, climate change, Essential Ocean Variables (EOV), social-ecological system, GOOS
Citation: Obura DO, Aeby G, Amornthammarong N, Appeltans W, Bax N, Bishop J, Brainard RE, Chan S, Fletcher P, Gordon TAC, Gramer L, Gudka M, Halas J, Hendee J, Hodgson G, Huang D, Jankulak M, Jones A, Kimura T, Levy J, Miloslavich P, Chou LM, Muller-Karger F, Osuka K, Samoilys M, Simpson SD, Tun K and Wongbusarakum S (2019) Coral Reef Monitoring, Reef Assessment Technologies, and Ecosystem-Based Management. Front. Mar. Sci. 6:580. doi: 10.3389/fmars.2019.00580
Received: 12 December 2018; Accepted: 30 August 2019;
Published: 19 September 2019.
Edited by:
Eric Delory, Oceanic Platform of the Canary Islands, SpainReviewed by:
J. Emmett Duffy, Smithsonian Environmental Research Center (SI), United StatesClaire M. Spillman, Bureau of Meteorology, Australia
Copyright © 2019 Obura, Aeby, Amornthammarong, Appeltans, Bax, Bishop, Brainard, Chan, Fletcher, Gordon, Gramer, Gudka, Halas, Hendee, Hodgson, Huang, Jankulak, Jones, Kimura, Levy, Miloslavich, Chou, Muller-Karger, Osuka, Samoilys, Simpson, Tun and Wongbusarakum. This is an open-access article distributed under the terms of the Creative Commons Attribution License (CC BY). The use, distribution or reproduction in other forums is permitted, provided the original author(s) and the copyright owner(s) are credited and that the original publication in this journal is cited, in accordance with accepted academic practice. No use, distribution or reproduction is permitted which does not comply with these terms.
*Correspondence: David O. Obura, dobura@cordioea.net