- 1Department of Environmental Sciences, University of Puerto Rico, San Juan, Puerto Rico
- 2Center for Applied Tropical Ecology and Conservation, University of Puerto Rico, San Juan, Puerto Rico
- 3Sociedad Ambiente Marino, San Juan, Puerto Rico
- 4Graduate School of Planning, University of Puerto Rico, San Juan, Puerto Rico
- 5Graduate School of Public Health, University of Puerto Rico, San Juan, Puerto Rico
- 6Department of Aquatic Ecology, University of Duisburg-Essen, Duisburg, Germany
- 7Faculty of Arts and Sciences, Harvard University, Cambridge, MA, United States
- 8Department of Biology, University of Puerto Rico, San Juan, Puerto Rico
Coral reefs are facing unprecedented global, regional and local threats that continue to degrade near-shore habitats. Water quality degradation, due to unsustainable development practices at coastal watersheds, is one of the greatest stressors across multiple spatial scales. The goal of this study was to assess near-shore coral reef benthic community spatio-temporal response to sedimentation patterns, weather, and oceanographic dynamics at Bahía Tamarindo and Punta Soldado in Culebra Island, Puerto Rico. Benthic data were collected across a distance gradient from the shore through high-resolution images at marked belt transects. Environmental data were assessed and contrasted with benthic assemblages using multivariate correlations and multiple linear regression. Coral colony abundance and coral recruit assemblages showed significant variation among seasons, sites and distance zones (PERMANOVA, p < 0.01). Species diversity (H’n) increased at both study sites with distance from shore, and the most conspicuous coral recruit species were stress-tolerant Porites astreoides, P. porites, and Siderastrea radians. Difference in coral abundance and coral recruits per site had a strong significant negative relationship with sediment characteristics and depth (p < 0.05). Near-shore coral reef benthic community structure was significantly different between sites and distance zones from shore, with depth having an important role in shaping reef zonation. Changes in benthic community structure were associated with local sediment distribution patterns emerging from human alteration of coastal watersheds and natural events that cause terrigenous sediment deposition and sand resuspension across the reef. Coral cover was significantly lower at zones more exposed to recurrent sedimentation stress (p < 0.01). It was also correlated with sediment texture (p = 0.006) and terrigenous sediment deposition (p = 0.016). Scleractinian coral cover had an inverse relationship with gorgonian and macroalgae cover. In a short-term period, a pattern of increased dominance of encrusting calcareous algae Ramicrusta textilis and invasive sponge Dictyonella funicularis were documented. Changing land use and increased frequency of extreme weather events, as a consequence of global patterns of climate change, may play an important role shaping near-shore coral reefs benthic communities and could threaten the resilience of coastal regions. Therefore, collaborative and trans-disciplinary ecosystem-based management efforts are urgently needed to effectively reduce land-based stressors and foster near-shore coral reef recovery.
Introduction
Coral reefs are affected by a wide array of global, regional, and local stressors that have led to habitat degradation worldwide during recent decades (Hughes, 1994; Gardner et al., 2003; Wilkinson and Souter, 2008). Global and regional trends of coral decline are associated to anthropogenic stressors combined with climate change-related impacts and natural disturbances (Eakin et al., 2010; Wild et al., 2011; Bozec and Mumby, 2015; Hernández-Delgado, 2015). At a local scale, land-based stressors represent a major threat to near-shore coral reefs in the Caribbean region. These land-based stressors are mostly related to increasing trends of land-use changes, coastal urban sprawl and tourism-based activities with direct effects on sediment-laden runoff and sediment distribution along coastal waters (Rogers, 1990; Larsen and Webb, 2009; Hernández-Delgado et al., 2012; Ramos-Scharrón et al., 2012, 2015; Bégin et al., 2013). This is particularly true for small tropical semi-arid islands, where an alteration of sediment delivery and distribution dynamics along near-shore reef ecosystem has been documented as a result of watershed alteration combined with changes in local weather patterns (Otaño-Cruz et al., 2017). Increased terrigenous sediment influx to coastal waters increases coral reef vulnerability and susceptibility to experience phase-shift toward alternate states, often dominated by non-reef building taxa and macroalgal assemblages (Acevedo et al., 1989; Fabricius, 2005, 2011; Bellwood and Fulton, 2008; Dudgeon et al., 2010; Hughes et al., 2010). This combination of factors can have profound permanent effects on reef ecosystem functions and services (Acevedo et al., 1989; Bellwood et al., 2004; Hughes et al., 2010). Therefore, there is a major concern regarding the potential effects of elevated terrestrial sediment input to coastal waters and changes in environmental conditions, especially on coral reef habitats historically adapted to low sedimentation levels.
The coral reef response to local human-induced disturbances depends on the frequency, duration, and distance from the source of the environmental stressor (Fabricius, 2005; Smith et al., 2008; Edmunds and Gray, 2014). Recurrent environmental disturbances have adverse implications for coral reef benthic communities by producing shifts in ecological dynamics and in the population of endangered coral species (Díaz-Ortega and Hernández-Delgado, 2014). Sedimentation stress has been associated with localized partial coral mortality, reduced coral growth rate, inhibited larval settlement, and reduced fish grazing (Loya, 1976; Nugues and Roberts, 2003a, b; Fabricius, 2005; Bellwood and Fulton, 2008). Impacted coral reefs can have loses of sensitive species, thus reinforcing phase shifts toward sediment-resistant species, and dominance by algae and other non-reef building taxa (Acevedo et al., 1989; Bellwood et al., 2004; Fabricius, 2005, 2011; Hughes et al., 2010). Changes in species composition can produce significant changes in structural complexity and functioning by reducing reef accretion and rugosity (Alvarez-Filip et al., 2013). Coral reefs exposed to recurrent sediment pulses and high turbidity levels also have limited ability to recover after chronic disturbances [e.g., bleaching caused by high sea surface temperature (SST)] and increased prevalence of coral diseases (Cróquer et al., 2002; Toledo-Hernández et al., 2007; Pollock et al., 2014; Stubler et al., 2016). Therefore, the understanding of the interactions between land and sea ecosystems and the short-term response of coral reefs community to sedimentation stressors is paramount for the development and implementation of effective and adaptive ecosystem-based management strategies to prevent further decline in live coral cover and enhance coral reef resilience (Rivera-Monroy et al., 2004; Alvarez-Filip et al., 2009; Mumby and Steneck, 2011).
Live coral cover decline across the Caribbean region has been partly attributed to increased sediment delivery to coastal waters after storms and heavy rainfall events, as a consequence of coastal watershed alteration and unsustainable development trends (Ramos-Scharrón and MacDonald, 2007; Hernández-Delgado et al., 2011, 2012, 2014b; Ramos-Scharrón et al., 2012; Sturm et al., 2014). At a broader spatial scale, the combined effects of chronic anthropogenic stressor impacts, such as declining water quality due to increased land-based source of pollution (LBSP), reduction in grazing due to overfishing and changing climate (e.g., change in rainfall patterns), have contributed to near-shore coral reef ecosystem degradation (Miller et al., 2009; Hernández-Pacheco et al., 2011; Edmunds, 2013; Jackson et al., 2014). This phenomenon illustrates the complexity and interconnectedness between the coastal and marine habitats, and thus, represents unfavorable conditions for coral reef recovery (Hughes and Connell, 1999; Rogers and Miller, 2006; Ennis et al., 2016). The implementation of mitigation and restoration projects at watershed and coral reef scales has become a management priority and the understanding of the existing land-sea and climate interconnectedness becomes critical for the implementation of rapid and effective strategies to contribute to coral reef recovery worldwide.
The goal of this study was to assess whether there was a significant spatio-temporal difference in the short-term response of benthic communities to variations in sedimentation patterns and environmental variables. Therefore, this study aimed to: (i) assess variation in coral reef benthic community structure through coral colony abundance, coral recruit abundance, percent live coral cover, octocoral, sponge and macroalgae cover in a distance gradient from the shore; and (ii) contrast spatio-temporal changes in coral colony abundance, coral recruit abundance, and coral cover with sedimentation patterns, and environmental variable dynamics.
Materials and Methods
Data Acquisition, Field Sampling, and Laboratory Analyses
Culebra Island is a mid-shelf semi-arid island located 27 km off the eastern coast of Puerto Rico, in the northeastern Caribbean Sea. The study was conducted from February 2014 to April 2015 across two leeward coral reef locations: Bahía Tamarindo (BTA, 18°18′ N, 65°19′ W) and Punta Soldado (PSO, 18°16′N, 65°17′W) (Figure 1). Both study sites are part of a long-term community-based coral reef rehabilitation effort to support reef functions and services, including the reefs’ role as fish nursery grounds (Hernández-Delgado et al., 2018). Transplant of Acropora spp. onto the reef was conducted prior to the initiation of this research in 2003. Belt transects of 10 m2 were marked parallel to the coastline and were assessed with high-resolution replicate photo-quadrat every 1 m2. Marked transects were assessed seasonally, from spring to winter, every 3 months, during a 1 year period. Each monitoring station consisted of triplicate fixed transects. Benthic assessment in BTA was conducted across a total of three replicate monitoring stations within distance zone A (<60 m from shore), with a depth range from 1 to 2 m, and three stations in distance zone B (>60 m from shore), with depth range contour from 2 to 4 m. In PSO, there were a total of two monitoring stations within zone A and B and the depth range contour was similar to BTA.
Scleractinian coral, hydrocoral, and octocoral species assemblages were assessed within each transect using photo-quadrats to identify corals to the lowest taxonomic level possible and to calculate the average abundance for each sampling station. This data was used to calculate coral colony abundance, species richness (S), species diversity index (H’n) (Shannon and Weaver, 1948), and evenness index (J’n) (Pielou, 1966) at each site and distance zone. Scleractinian coral recruit abundance was also assessed, specifically for colonies ≤4 cm in diameter for larger species (i.e., Pseudodiploria spp., Siderastrea siderea), and ≤2 cm for smaller species (i.e., Porites astreoides) (Dueñas et al., 2010). The benthic cover was assessed from high-resolution images by digitally projecting 48 regularly-distributed dots over each photo-quadrat image. Benthic components under each dot were identified, including scleractinians, hydrocorals, octocorals, sponges, macroalgae, algal turf, coralline algae (CA), and cyanobacteria, among other components (i.e., sand, pavement, rubble). Coral cover as recorded included both Scleractinians and hydrocorals. Algal turf was classified as a mix of short algae (<1 cm) and sediments (NOAA, 2015). Data was used to calculate percent benthic component cover and benthic community structure.
The environmental database included sedimentation rate, sediment texture, and composition. Sediment traps were deployed at both sites across a distance gradient from shore, within a distance of less than 10 m from marked belt transects. Sediment samples were collected on a monthly basis and processed at the laboratory. Sediment samples were oven-dried at 60°C for 24 h and total dry weight was recorded to calculate sediment accumulation rate (Edmunds and Gray, 2014; Otaño-Cruz et al., 2017). Sediment texture (silt-clay <63 μm and sand >63 μm) were analyzed through dry sieving, while loss on ignition techniques were applied to determine the organic matter (3 h oven dry 550°C), carbonate matter (3 h oven dry 950°C) and terrigenous sediment composition (Otaño-Cruz et al., 2017). Rainfall events were documented with HOBO RG3 (Onset Computers, Co.) rain gauges located in coastal watersheds near study sites, while sea surface temperature at both study sites were recorded with HOBO Watertemp Pro V2. Oceanographic hydrodynamics data, such as wave height and wind speed, were acquired from the Caribbean Integrated Coastal Ocean Observing System (CariCOOS) online database1, buoy NDBC 41056 located at Vieques sound.
Statistical Analyses
Benthic components were tested using four-way non-parametric permutational analysis of variance (PERMANOVA) and pairwise comparison for the fixed factors of seasons, time, site and distance from shore (Anderson et al., 2008). Multivariate analyses were performed in Primer v7 + PERMANOVA v1.16 software (Quest Research Ltd., Auckland, New Zealand) to analyze spatio-temporal variation in coral colony abundance, coral recruit abundance, and benthic community structure (Clarke et al., 2014; Clarke and Gorley, 2015). A significant relationship was identified by factors that had P < 0.05. The rank order of dissimilarities were calculated through Bray-Curtis resemblance from coral abundance, coral recruit abundance, and benthic community matrices. For coral recruitment, a zero-adjusted Bray-Curtis resemblance matrix was calculated, including a “dummy variable” to reduce distortion from absent species by samples (Clarke et al., 2006). Coral colony abundance, coral recruit abundance, and percent benthic community parameters were standardized to balance the contribution of common and rare species, and thus, represented the relative percentage of species for each sample. Species assemblages were also square root-transformed prior to analysis to meet assumptions of normality and homogeneity of variance (Clarke and Warwick, 2001; Gotelli and Ellison, 2013). All multivariate tests were based in 10,000 permutations (Hernández-Delgado et al., 2014a). Sigma Plot v.11 (Systat Software, Inc.) was used for graphical representation of biological data.
Ordination was performed using non-metric multi-dimensional scaling (nMDS) and principal coordinates ordination (PCO), by calculating the distance among centroids, to display the variations in benthic communities in a three-dimensional space and determine which benthic component explained spatio-temporal variation. Cluster and similarity profile test (SIMPROF) were used to identify groups with similarity differences between samples, to test the null hypothesis of no significant spatial and temporal differences on multivariate structure of benthic assemblages. Afterward, a similarity percentage (SIMPER) analysis routine was performed to determine which key taxa contributed most to similarities and spatial variation in the benthic community structure through time, within sites and distance (Clarke et al., 2014).
Environmental variables were correlated with the biological matrices using non-parametric multivariate correlation routine BEST-BIO ENV (Spearman rank correlation) to determine the best environmental variable that explained differences in coral reef benthic community spatio-temporal variation (Clarke et al., 2014). RELATE routine was used to test the relationship between coral recruit abundance and percentage macroalgal cover, with sedimentation and other abiotic variables. DISTLM was performed for multiple linear regression analysis to assess potential effects of environmental variables on coral colony abundance, coral recruit abundance, and benthic cover with a distance-based redundancy analysis (dbRDA) (Anderson et al., 2008).
Results
Coral Colony Abundance, Coral Species Richness, and Diversity
Mean coral colony abundance (±95% confidence interval) at Bahía Tamarindo and Punta Soldado near-shore coral reefs was 13.18 ± 0.92 m–2. There were significant differences in coral abundance by season (Pseudo F = 2.38, p = 0.0002), sites (Pseudo F = 36.96, p = 0.0001), and distance zones (Pseudo F = 9.98, p = 0.0001) (Table 1). Coral colony abundance was higher at BTA than PSO with a mean of 13.77 ± 0.92 m–2. The highest coral abundance value at BTA was documented a long distance zone B (farther from shore, >60 m from shore), with a mean of 17.95 ± 1.27 m–2 (Figure 2). The lowest value of coral abundance at BTA was recorded at distance zone A (near to shore, <60 m from shore) with a mean of 9.59 ± 0.94 m–2. Mean coral abundance was lowest at PSO with a mean 12.29 ± 1.29 m–2. The highest coral colony abundance value at PSO was documented near shore, zone A, with a mean of 12.78 ± 4.68 m–2. Coral abundance at both sites experienced significant variation through seasons at offshore zone (Figure 2). There were significant interaction effects between site and distance zones (Pseudo F = 18.74, p = 0.0001), suggesting the influence of the latter on the observed variation between sites. Pairwise analysis indicated that coral colony abundance had significant variation in BTA (t = 4.02, p = 0.0001) and PSO (t = 4.02, p = 0.0001) by distance zones.
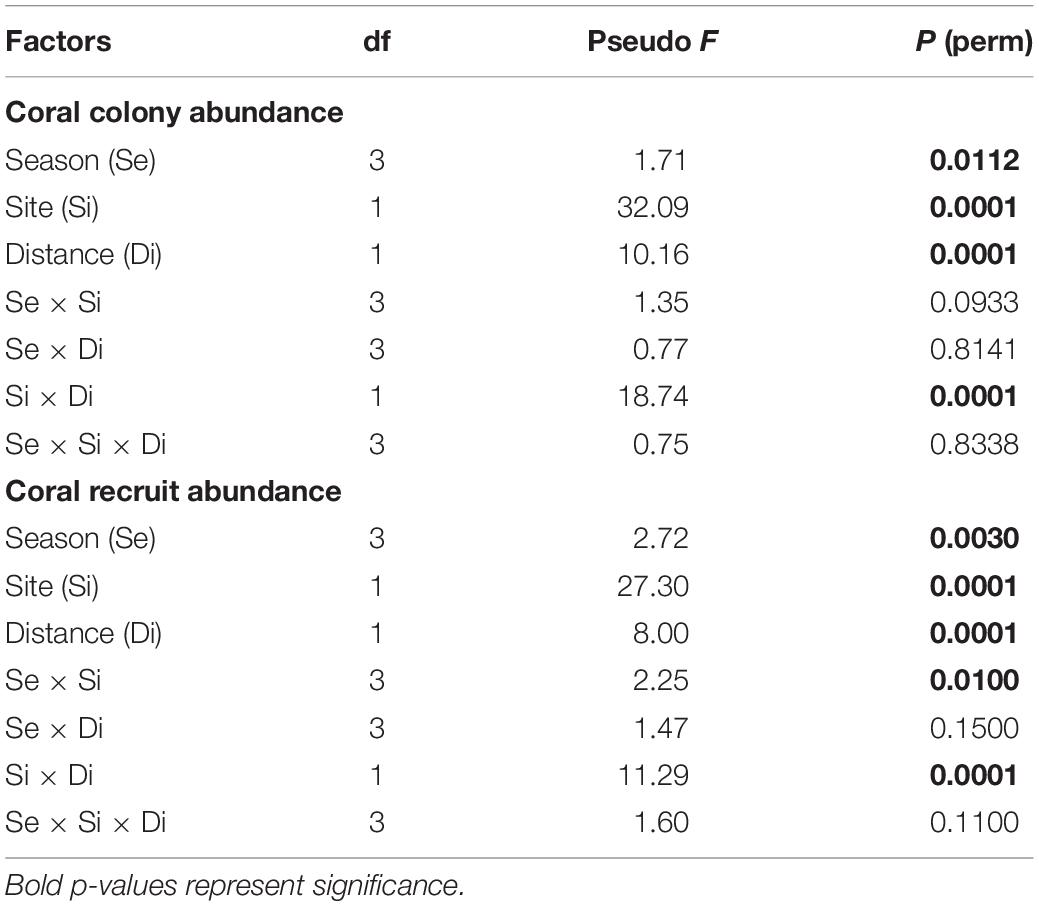
Table 1. Results of permutational analysis of variance (PERMANOVA) for coral colony abundance and coral recruit abundance.
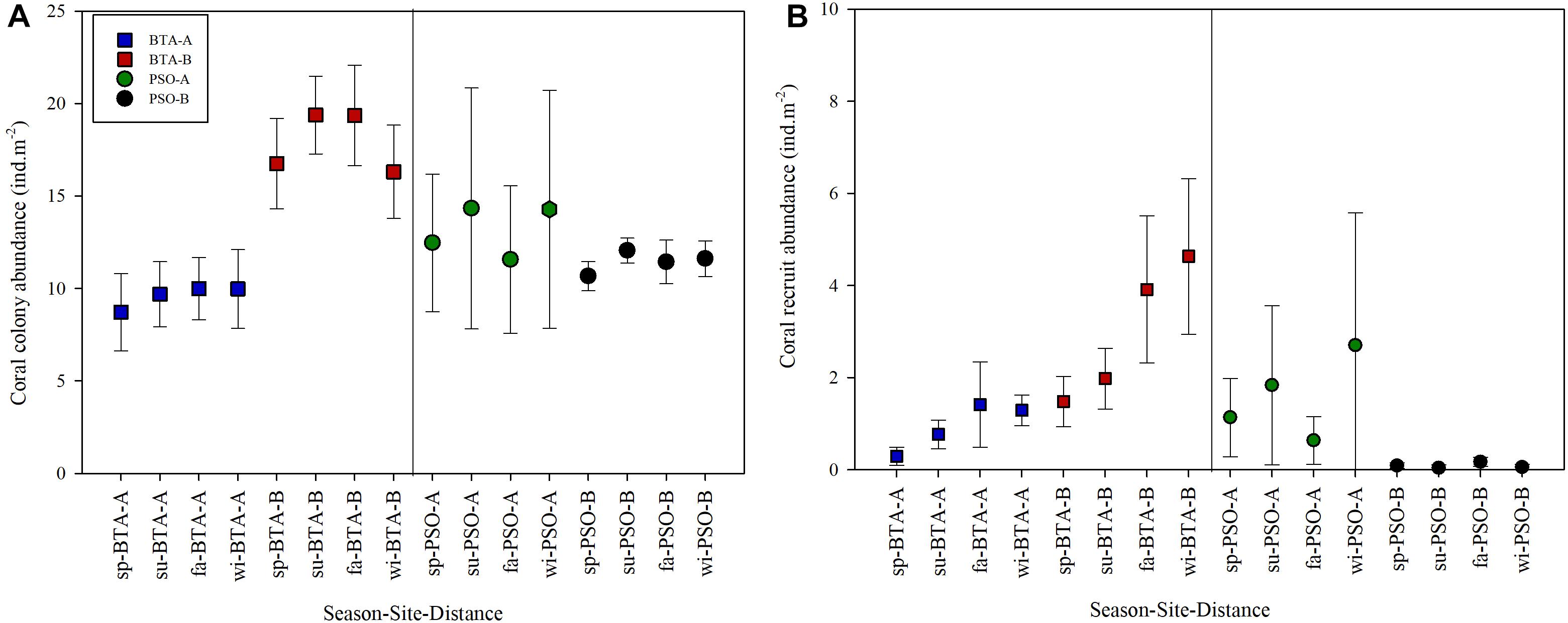
Figure 2. Mean coral colony abundance (mean ± 95% CI) (A) and coral recruit abundance (B) by season, site, and distance zone. Coral abundance includes hard corals, hydrozoans and gorgonians. Blue and red squares represent Bahía Tamarindo (BTA); green and black circles represent Punta Soldado (PSO). Seasons are defined as: sp, spring; su, summer; fa, fall; wi, winter.
A total of 66 species were documented (34 scleractinians, 2 hydrocorals, and 30 octocorals) in both study sites combined. The scleractinian coral species with highest mean relative abundance at BTA were Porites astreoides (24.54%), P. porites (11.40%), Acropora cervicornis (5.84%), Siderastrea siderea (5.76%), Pseudodiploria strigosa (5.66%), Diploria labyrinthiformis (5.03%), S. radians (4.59%), and Agaricia agaricites (3.48%), representing a total 66.30% of species contribution (Figure 3). The only species that showed a pattern of increased relative abundance through time at both distance zones was P. porites. The hydrocorals Millepora alcicornis and M. complanata had a higher percentage relative abundance at BTA than PSO, with a mean of 16.54 and 2.35%, respectively. Coral species with the highest mean relative abundance at PSO were P. astreoides (31.06%), P. porites (10.15%), O. annularis (10.14%), S. siderea (3.30%), and P. strigosa (2.53%), representing a total of 57.18%. Relative abundance D. labyrinthiformis and P. porites decreased through seasons near to shore and increased farther from shore. The coral species P. astreoides and O. annularis had higher relative abundance at reef zones farther from shore.
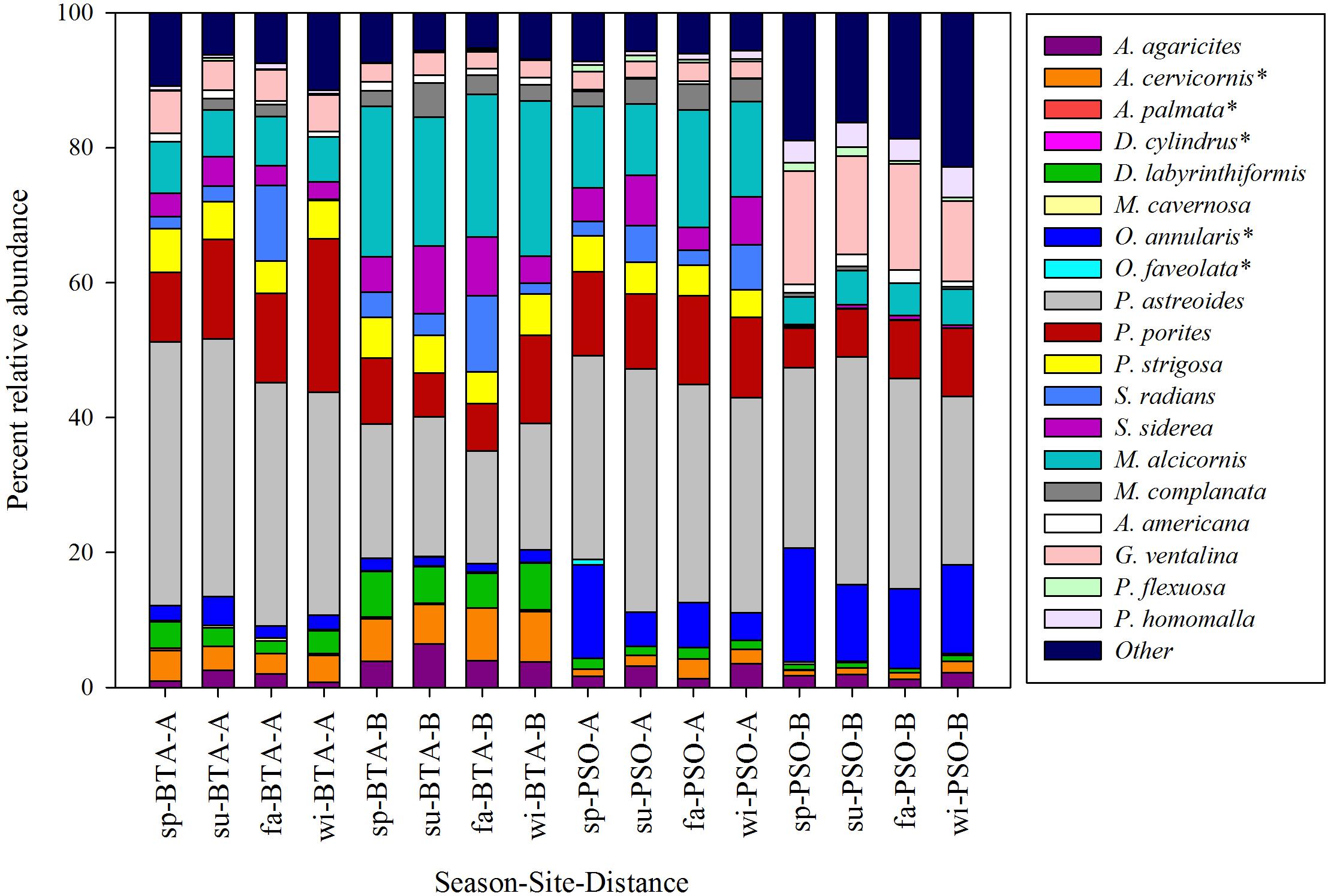
Figure 3. Percent relative abundance of scleractinians, octocorals and octocoral species by season, site and distance zones. Colors represent coral species and asterisk identifies coral species listed as threatened under the Endangered Species Act (Federal Register, 2014). Seasons are defined as: sp, spring; su, summer; fa, fall; wi, winter.
Gorgonians had a higher relative abundance at PSO than BTA, with a mean of 11.87%, mainly composed by Antillogorgia americana, Eunicea flexuosa, Gorgonia ventalina, and Plexaura homomalla (Figure 3). In contrast, gorgonians at BTA represented a mean relative abundance of 5.08%. The species that showed patterns of increased relative abundance through time at PSO at both distance zones were A. cervicornis, A. agaricites, and the octocoral P. homomalla. The SIMPER analysis revealed that the species that mostly contributed to differentiate coral colony abundance between sites were O. annularis (7.45% contribution), M. alcicornis (6.41%), P. strigosa (4.86%), and S. radians (4.83%), with a total of 23.55% of the observed variation. Differences between distance zones were mostly attributed to M. alcicornis (7.31%), P. porites (5.82%), P. astreoides (5.44%), and O. annularis (4.92%), with a total of 23.49% of the observed variation. PCO analysis identified four major groups from the coral abundance structure that represent the interaction between sites and distance zones (Figure 4). The seasonal variation of coral community within distance zone A had higher similarity between BTA and PSO due to the proximity of both clusters. In contrast, the coral community at PSO distance zone B showed a major difference between sites and distances, with clusters farther apart. The proposed PCO explains 74% of the variation between and within groups.
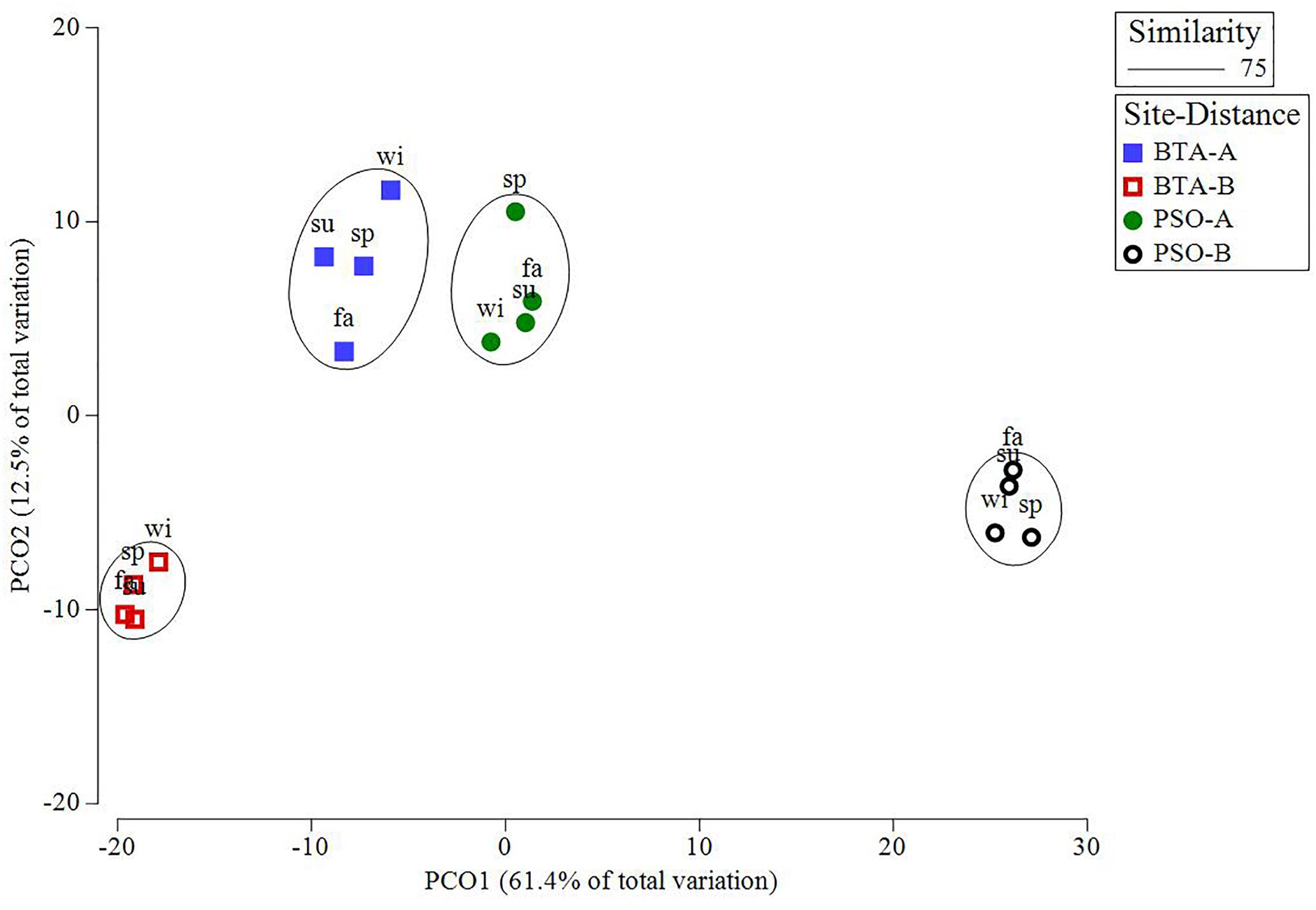
Figure 4. Principal coordinates ordination (PCO) plot of distance among centroids of coral colony abundance based on Bray-Curtis similarity matrices performed on a square root-transformed data by seasons-site-distance. Clusters represent groups with 75% similarity. Seasons are defined as: sp, spring; su, summer; fa, fall; wi, winter.
Coral species richness (S) at BTA was highest at distance zone B with a mean of 18.11 ± 1.18 (±CI 95%). Mean S decreased from spring to winter at both distance zones (Figure 5A). Overall, the highest total coral species richness between sites was recorded at PSO distance zone B with a mean of 18.63 ± 1.19. Species richness at PSO experienced seasonal variation along both distance zones (Figure 5B). Species richness showed significant differences by distance zones (Pseudo F = 1.37, p = 0.0010), but no difference was recorded between seasons (Pseudo F = 1.41, p = 0.2300), or sites (Pseudo F = 0.91, p = 0.3300).
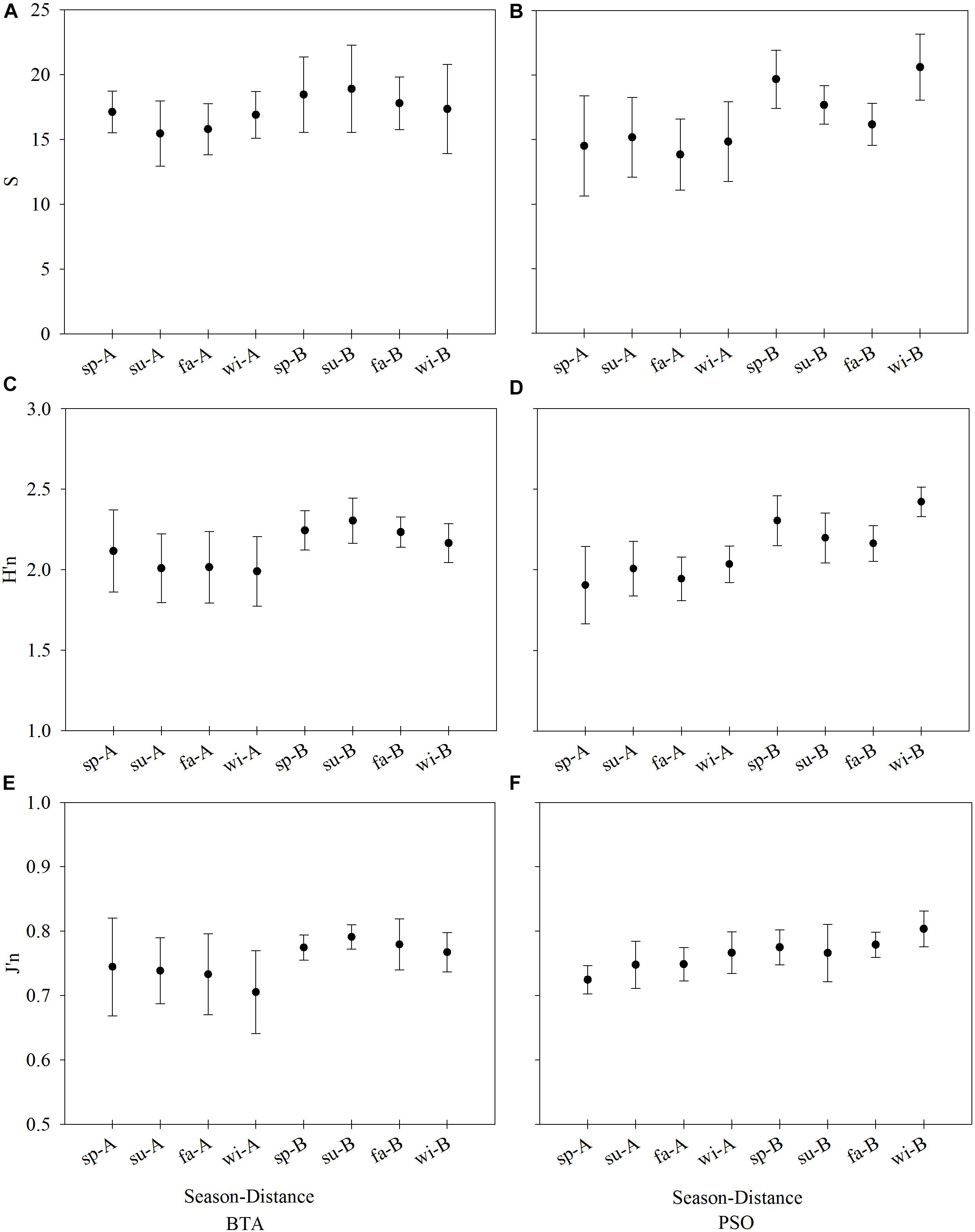
Figure 5. Coral species richness (S, A,B), diversity index (H’n, C,D) and evenness (J’n, E,F) by site, season, and distance zones (mean ± 95% CI). Left column graphs represent Bahía Tamarindo (BTA) and right column Punta Soldado (PSO). Seasons are defined as: sp, spring; su, summer; fa, fall; wi, winter.
Coral species diversity (H’n) showed contrasting patterns by sites. In BTA, H’n was higher farther from shore, with the highest values recorded during the summer with a mean of 2.30 ± 0.14 (Figure 5C). At both distance zones, H’n declined gradually from spring to winter season, representing a short-term percent change of -5.98%. The highest H’n between sites was recorded at PSO farther from shore with a mean of 2.27 ± 0.07. At PSO, H’n increased from spring to winter at both distance zones (Figure 5D). Coral diversity at both sites had lower values at shallower, near-to-shore areas. There were significant differences by distance zones (Pseudo F = 32.22, p = 0.0010), but no difference was recorded between seasons (Pseudo F = 0.44, p = 0.7200) or sites (Pseudo F = 0.04, p = 0.8500). The evenness (J’n) showed that BTA had a higher difference in species dominance between distance zones, with a higher evenness farther from shore (Figure 5E). J’n at BTA showed a pattern of rapid decline at distance zone A through seasons, reflecting greater changes in species dominance. J’n at PSO had similar patterns of increasing evenness across both distance zones through seasons (Figure 5F). There was a significant difference in J’n by distance zones (Pseudo F = 12.24, p = 0.0020).
Coral Recruit Abundance
Coral recruit community structure showed statistically significant differences among seasons (Pseudo F = 2.72, p = 0.0030), site (Pseudo F = 23.30, p = 0.0001), and distance (Pseudo F = 8.00, p = 0.0001) (Table 1). There were also significant differences between the interactions of site and distance (Pseudo F = 11.29, p = 0.0001). Coral recruit abundance was higher in BTA at areas farther from shore with a mean of 1.96 ± 0.46 m–2. Mean coral recruit abundance at BTA increased from 0.88 m–2 in spring to 2.96 m–2 in winter. Coral recruit abundance near to shore increased from 0.28 (spring) to 1.28 m–2 (winter), while farther from shore increased from 1.47 to 4.63 m–2 (Figure 2). In contrast, at PSO, coral recruit abundance was higher on reef areas closer to shore. Mean recruit abundance increased from 0.60 m–2 in spring to 1.38 m–2 in winter. Recruit abundance near to shore increased from 1.13 to 2.7 m–2 and farther from shore decreased gradually from 0.08 to 0.05 m–2. A total of 11 coral species recruited at both study sites and the species with highest percentage relative abundance were Siderastrea radians (45.1%), S. siderea (23.1%), and followed by P. astreoides (19.2%) (Table 2). No coral recruits of large reef-building coral species were documented at BTA through the study period.
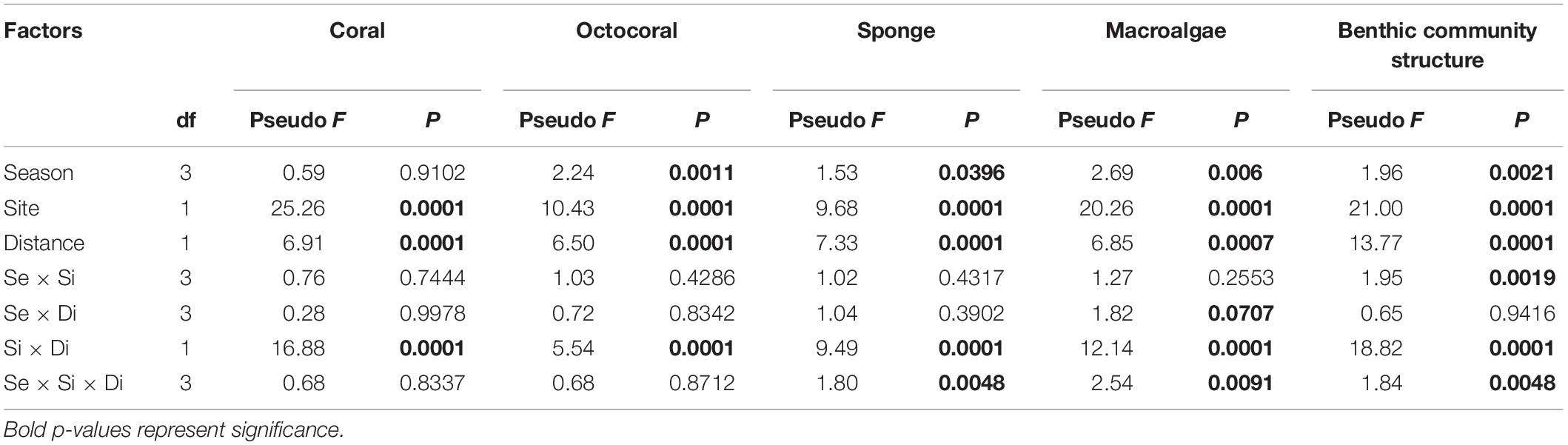
Table 2. Results of permutational analysis of variance (PERMANOVA) for benthic components by factors.
PCO analysis identified two major groups which distinguished different coral recruit abundance patterns of PSO distance zone B from zone A, and from BTA among all seasons (Figure 6). There was also one outlier event identified for PSO zone B during the summer season, particularly due to a significant reduction recorded with a mean recruit abundance of 0.4 m–2. However, SIMPROF analysis only identified two distinct groups for coral recruit abundance. The calculated PCO explains 83.2% of the total variation. Also, SIMPER analysis showed the three species that contributed to explain 79% of the differences of coral recruit abundance patterns between sites were S. radians (32.41% individual contribution), P. astreoides (23.93%), and S. siderea (22.42%). The species that contributed to explain 73% of the differences between distance zones were P. astreoides (27.83%), S. siderea (24.42%), and S. radians (21.21%). The average dissimilarity was 49.69 between sites and 61.01 between distance zones.
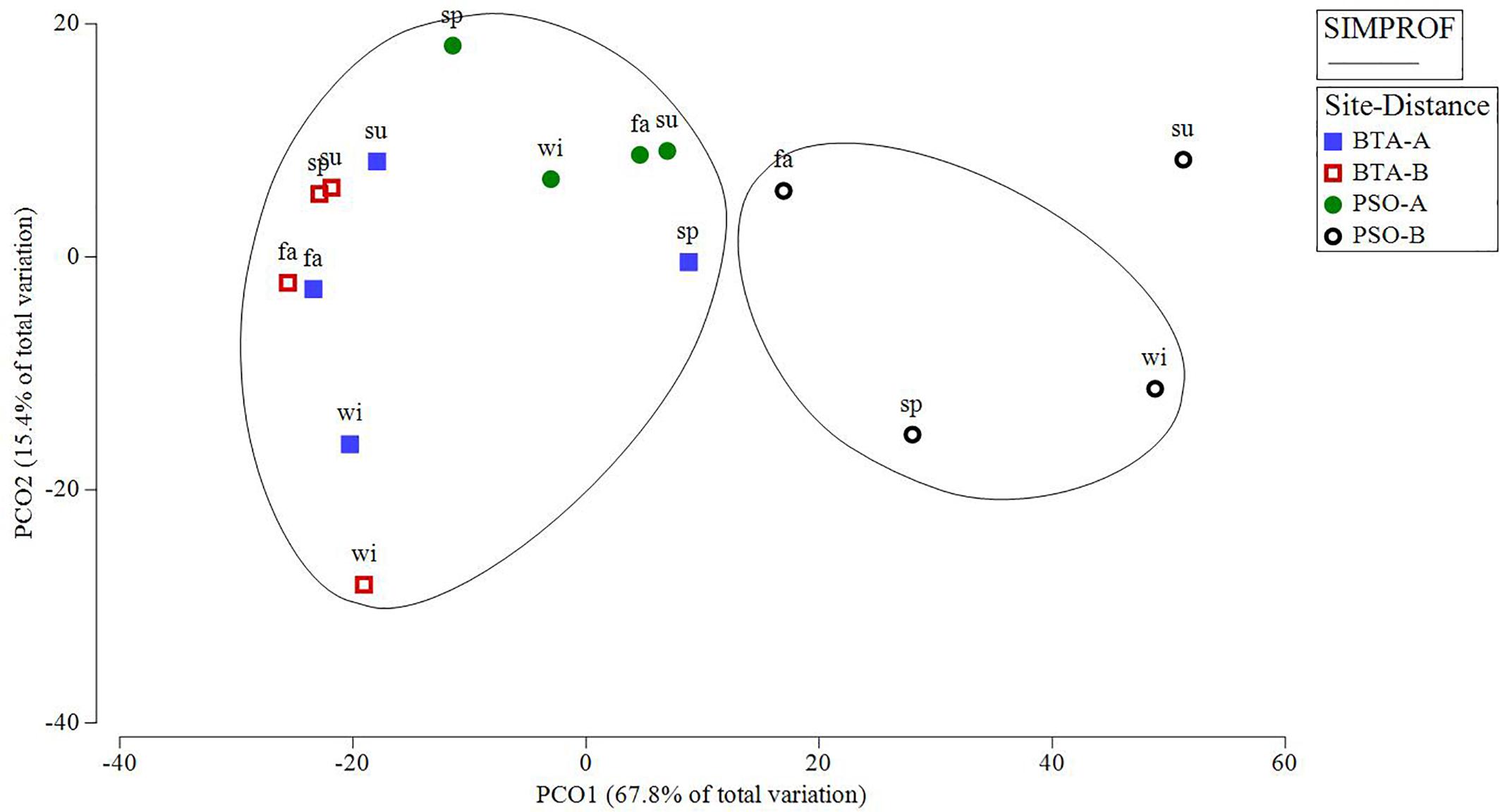
Figure 6. PCO plot of distance among centroids of coral recruit abundance based on Bray-Curtis similarity matrices performed on square root-transformed data by seasons-site-distance clusters. Clusters represent significant SIMPROF groups (∼75% similarity within groups). This model explained 83.2% of the observed spatio-temporal variation in coral recruit abundance by site and distance zone. Blue and red squares represent Bahía Tamarindo (BTA); green and black represent Punta Soldado (PSO). Seasons are defined as: sp, spring; su, summer; fa, fall; wi, winter.
Benthic Components Percent Cover and Community Structure
Live coral cover was significantly higher on BTA zone B with a mean percent cover of 17.87 ± 0.67%, and a gradual reduction was recorded in a short-term period (Figure 7A). Percent coral cover at zone A, near to shore, remained relatively stable through seasons. Coral cover at PSO had the lowest percentage cover farther from shore with a mean of 7.84% and it slightly decreased through time at both distance zones. Percentage coral cover was significantly different between sites (Pseudo F = 25.26, p = 0.0010), distance (Pseudo F = 13.77, p = 0.0010), and the interaction site by distance (Pseudo F = 18.82, p = 0.001) (Table 3).
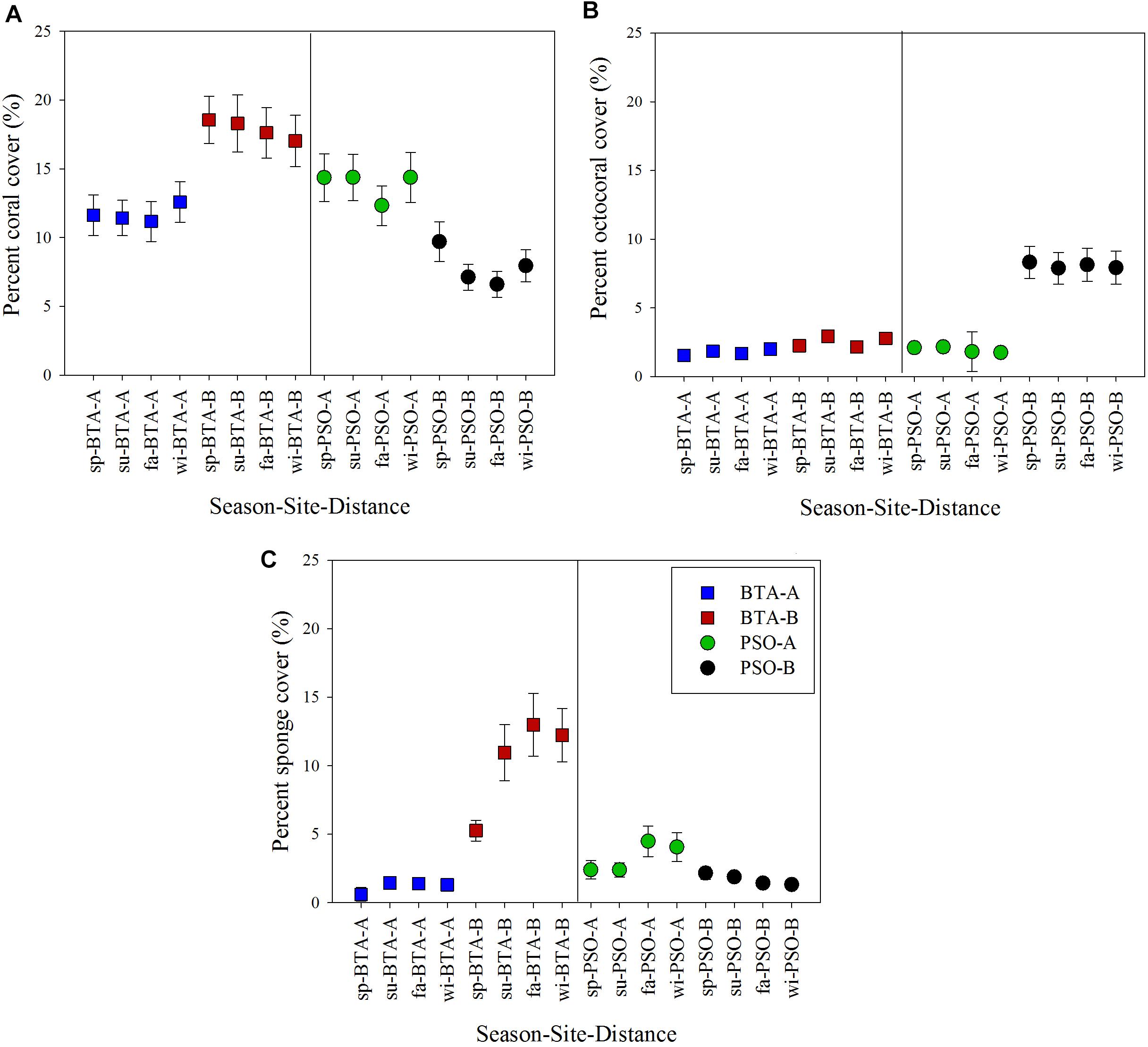
Figure 7. Coral reef benthic components cover across a distance gradient from shore and seasons (mean ± 95% CI). Percent coral cover (Scleractininas + hydrocorals) (A), octocoral cover (B), and sponge cover (C). Blue and red squares represent Bahía Tamarindo (BTA); green and black represent Punta Soldado (PSO). Seasons are defined as: sp, spring; su, summer; fa, fall; wi, winter.
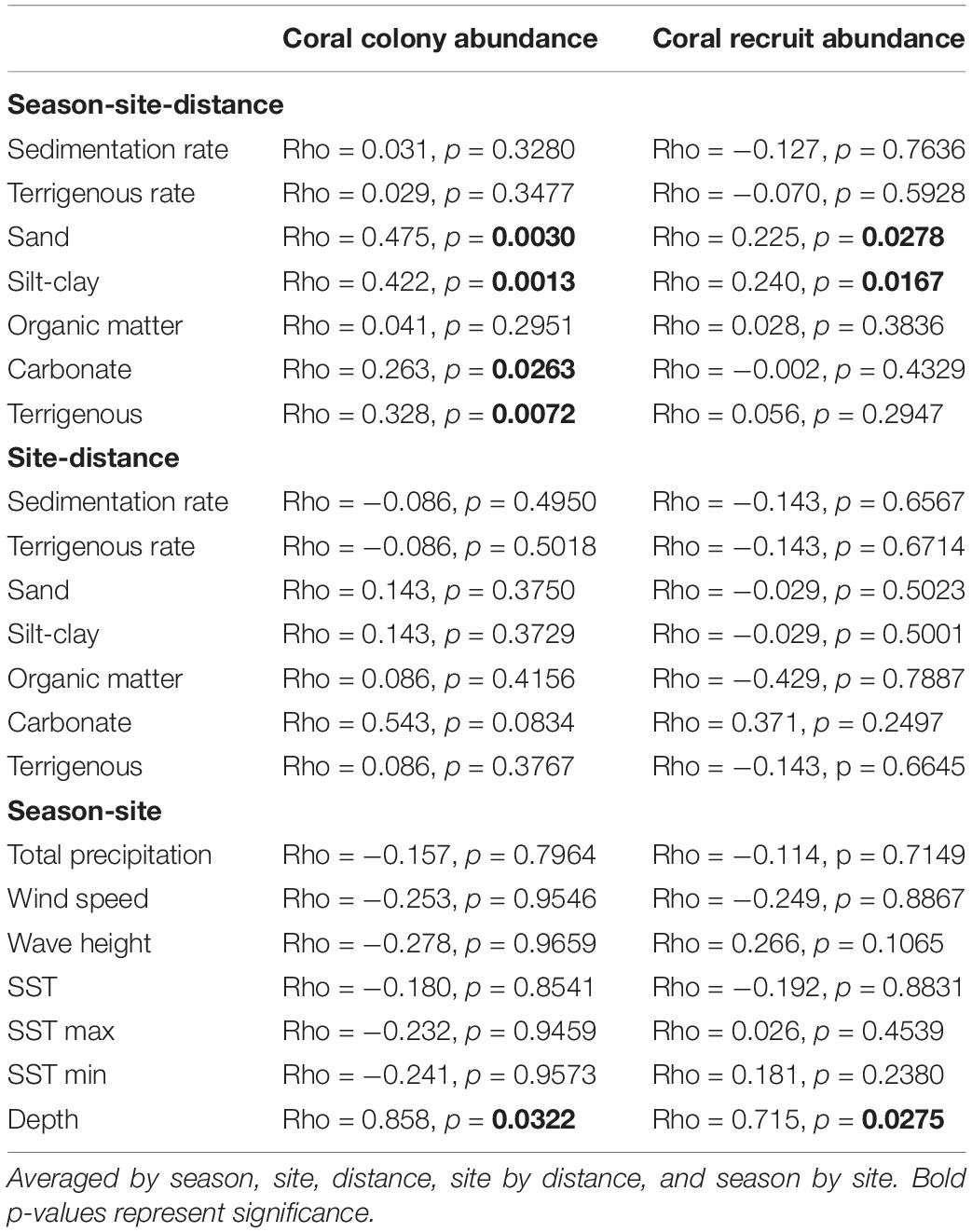
Table 3. Summary of RELATE (spearman rank) correlation matrix for coral colony abundance, coral recruit abundance and environmental variables.
Stress-tolerant coral species, P. astreoides and P. porites, had higher percent relative cover in BTA zone A than distance zone B, and PSO, with a mean of 4.28 and 2.71%, respectively. The hydrocoral M. alcicornis had a higher percent cover farther from shore (Figure 8). Percentage cover of A. cervicornis was higher in distance zone B with a mean cover of 1.95% and increased from spring 1.56% to winter 2.35%. In PSO, the species with higher percentage cover near to shore was P. astreoides with a mean of 2.51% and O. annularis farther from shore with a mean of 3.63%. The similarity percentage (SIMPER) analysis revealed that 8 species constituted 75% of the observed variations in percent coral cover between sites and distance zones. The species that had major contributions to variations between sites and distance zones were M. alcicornis, O. annularis, P. porites, and A. cervicornis. There was an average dissimilarity between sites of 57.11 and 49.85% between distances zones.
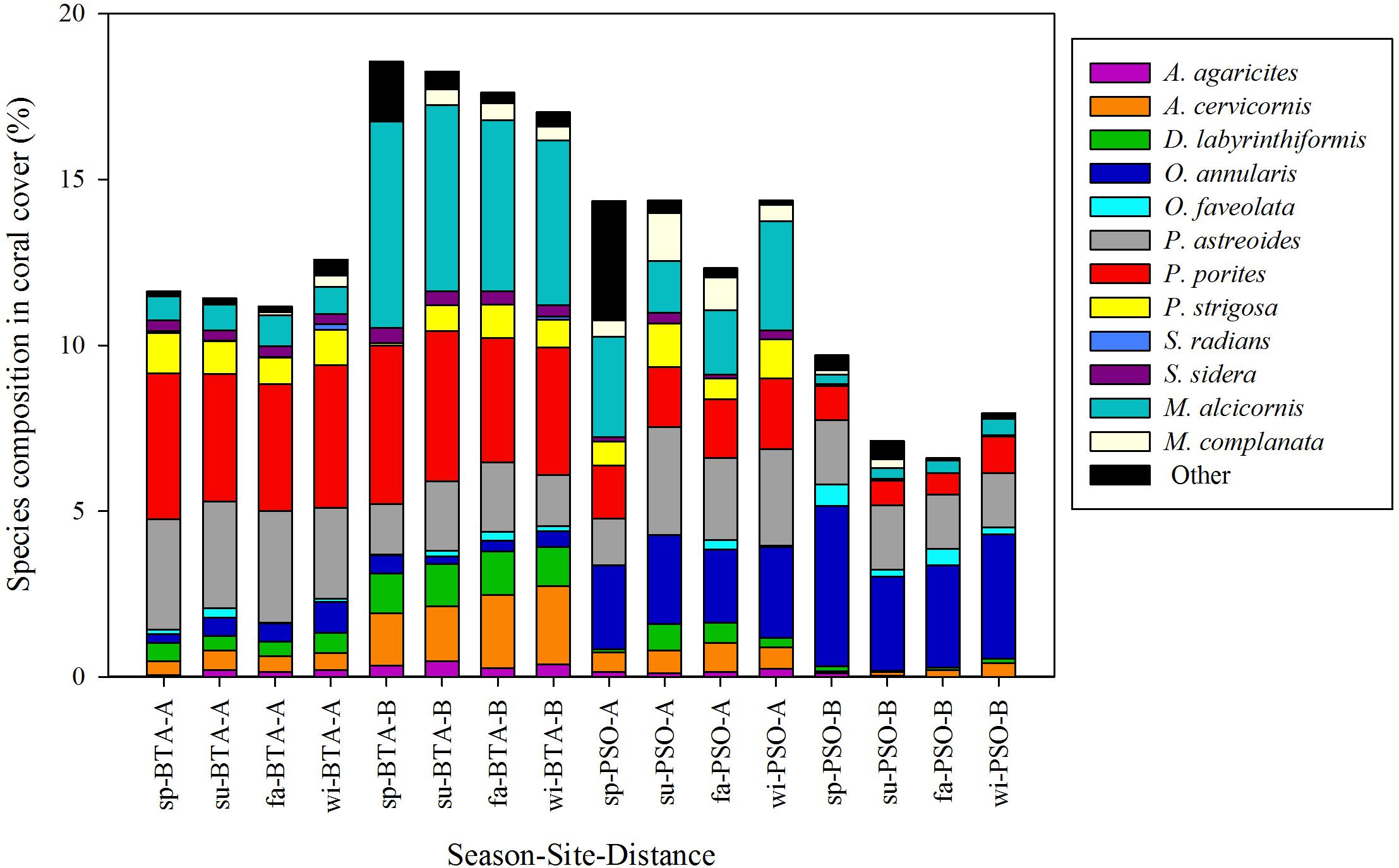
Figure 8. Percent species composition of Scleractinian and hydrocoral species. Most dominant species among site, distance zone and seasons identified by colors. Seasons are defined as: sp, spring; su, summer; fa, fall; wi, winter.
Octocorals mostly dominated benthic cover at PSO farther from shore with a mean of 8.10% (Figure 7B). The octocoral cover near to shore had similar patterns at PSO and BTA with an overall mean cover of 1.80%. Sponges had higher percentage cover at BTA with a mean of 5.77%. Sponges had a pattern of increasing percentage cover at areas farther from shore, reaching benthic cover of up to 12% during the fall and winter season (Figure 7C). The gray encrusting and invading sponge species Dictyonella funicularis had a significant contribution at BTA farther from shore, with an increasing benthic cover from spring (0.02%) to fall (7.81%). This species was documented overgrowing dead or diseased A. cervicornis colonies, among other benthic components. In contrast, D. funicularis had higher percent cover at PSO closer to shore, reef area that experienced an increase in sponge cover from spring to winter. Octocoral and sponge cover showed a significant difference among seasons, sites, and distance zones (p < 0.05) (Table 3). Sponge community also had a significant interaction between site and distance (p < 0.05).
Macroalgae and algal turf were important components of the community composition. Macroalgal assemblages had significant differences among season, sites, distance and the interaction site by distance (p < 0.05) (Table 3). Higher percent macroalgae cover was recorded at PSO, especially at areas farther from shore, where it reached up to 35% (Figure 9A). Macroalgae dominance at PSO occurred while the encrusting and invasive red algae Ramicrusta textilis overgrew dead skeletons of the O. annularis species complex. Reef zones adjacent to the shoreline were dominated by Dictyota spp. algae, which reached its highest cover at BTA with 14%. Algae composition at BTA also had a high dominance of turf, which constituted a mean cover of 37.51%, and it was significantly higher at zone A with its highest level recorded during the fall season of 51.67% (Figure 9B). Meanwhile, on distance zone B, the most prominent algae cover were turf and crustose coralline algae (CCA), Porolithon spp., since these constituted a mean cover of 28.66 and 9.53%, respectively. The percentage cover of CCA at BTA farther from shore increased through seasons (Figure 9C). Cyanobacterial cover also had an important influence on BTA zone B with increased percentage cover form spring (3.91%) to summer (6.99%) (Figure 9D).
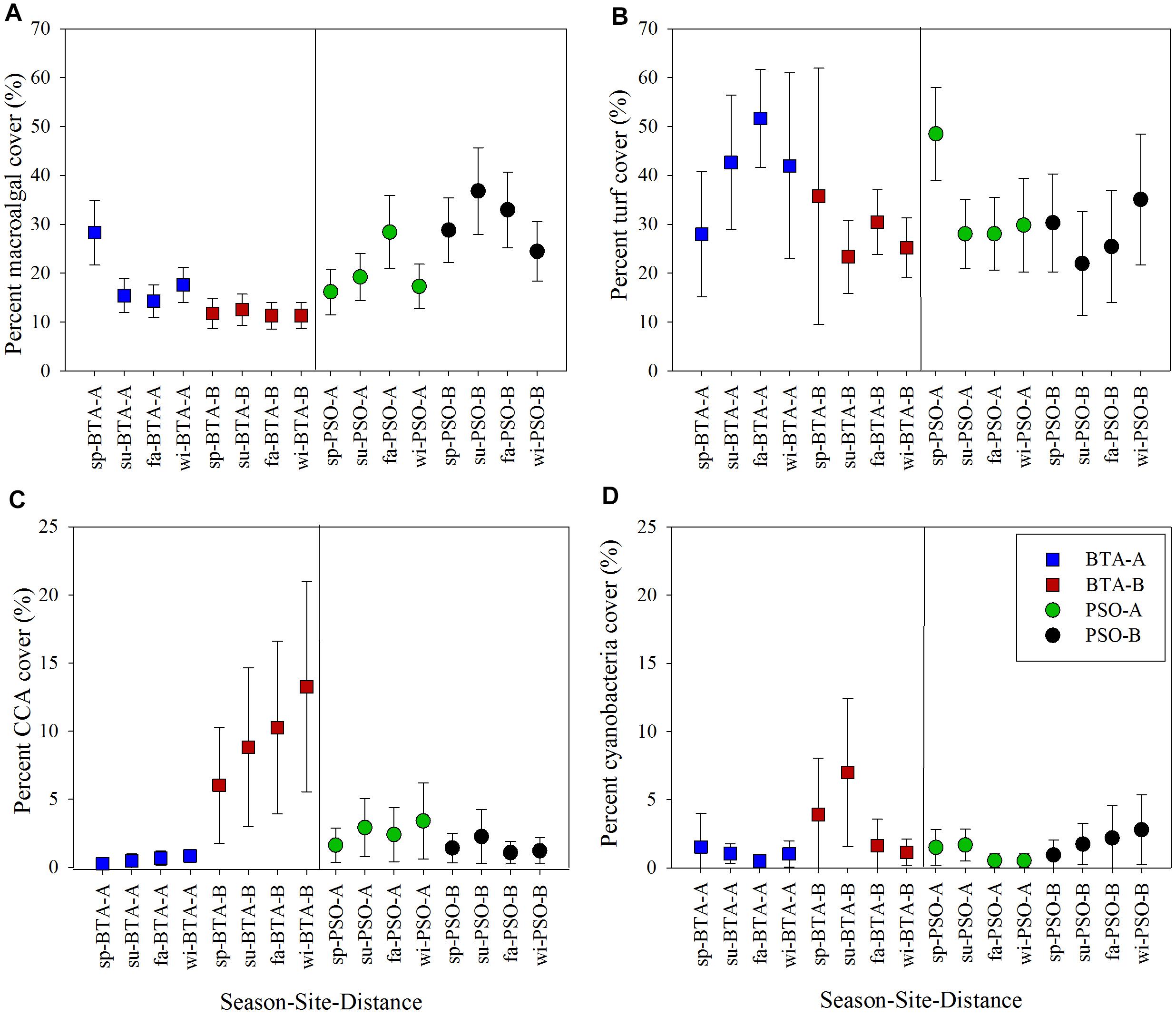
Figure 9. Coral reef benthic components cover across a distance gradient from shore, site, and seasons (mean ± 95% CI). Percent macroalgal cover (A), turf (B), crustose coralline algae (CCA) (C), and cyanobacteria cover (D). Blue and red squares represent Bahía Tamarindo (BTA); green and black represent Punta Soldado (PSO). Seasons are defined as: sp, spring; su, summer; fa, fall; wi, winter.
Coral reef benthic community structure presented a statistically significant difference among seasons (Pseudo F = 1.96, p = 0.0020), sites (Pseudo F = 21.00, p = 0.0010), distance (Pseudo F = 13.07, p = 0.0010), and within the interactions season by site (Pseudo F = 1.95, p = 0.0070), and site by distance (Pseudo F = 18.82, p = 0.0010) (Table 3). All of the benthic components analyzed in this study showed significant differences in the interaction of site by distance. The PCO analysis identified four major groups that distinguished benthic community structure between sites and distance zone (Figure 10). Benthic communities were similar between sites at areas near to shore and greater differences between benthic communities were identified farther from shore.
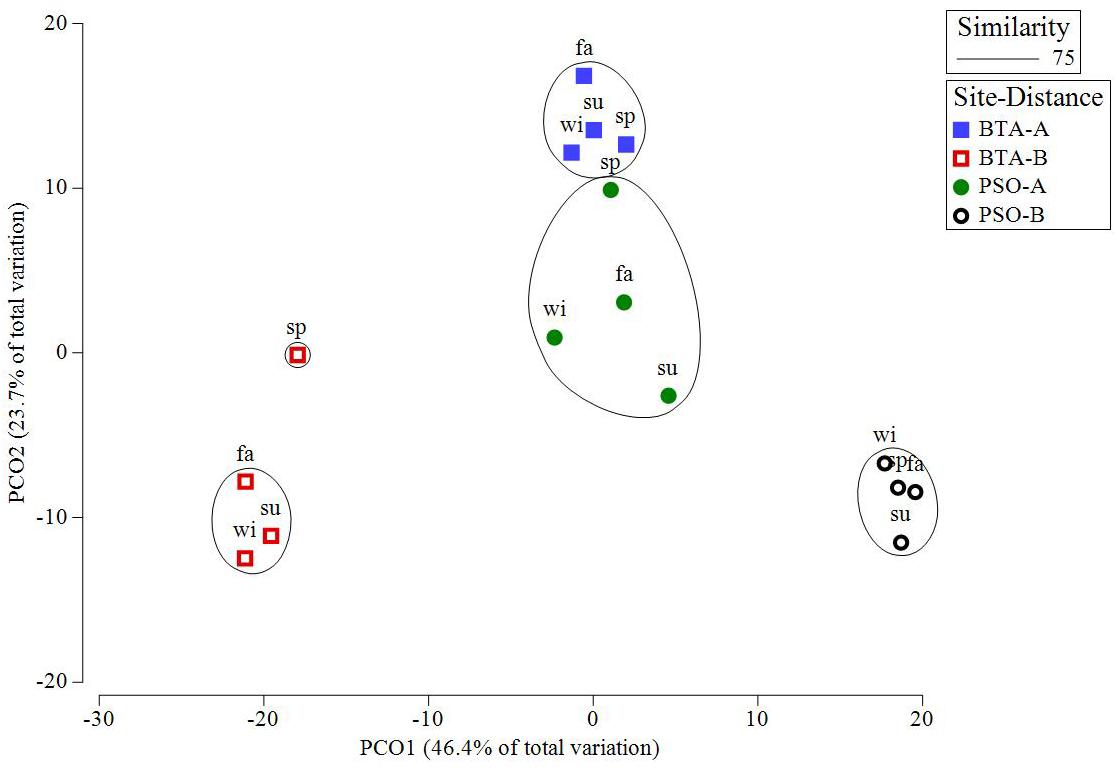
Figure 10. Principal coordinates ordination (PCO) plot of benthic community structure on site by distance based on Bray-Curtis similarity matrix. Cluster represent 75% of similarity within groups. This model explained 75% of the observed spatio-temporal variation in benthic cover. Blue and red squares represent Bahía Tamarindo (BTA); green and black represent Punta Soldado (PSO). Seasons are defined as: sp, spring; su, summer; fa, fall; wi, winter.
Benthic Community Structure and Environmental Variables
Among the environmental variables assessed, coral colony abundance had a significant negative correlation with sand (>63 μm) (RELATE, Rho = 0.475, p = 0.002), silt-clay (<63 μm) (Rho = 0.422, p = 0.003), carbonate (Rho = 0.263, p = 0.026), and terrigenous sediment content (Rho = 0.328, p = 0.009) (Table 3). Coral colony abundance had a significant positive interaction with depth (Rho = 0.858, p = 0.037) at BTA and a negative interaction at PSO. The nMDS bubble plot showed that sand and terrigenous sediment had a significant negative association with spatial configuration of coral colony abundance at BTA, while silt-clay had a negative significant association with coral colony abundance at PSO reef (Appendix 1). These patterns suggest that sediment texture differed significantly between sites (PERMANOVA, Pseudo F = 31.26, p = 0.001) and for the interaction site by distance from shore (Pseudo F = 5.52, p = 0.030). Multiple linear regressions analyses (visualized in dbDRA diagram) identified sand sediment particle distribution (r2 = 0.33, p = 0.0007) as the most significant environmental variable that explained 49% of the total spatial and temporal variation of coral abundance at nearshore reefs (Figure 11A).
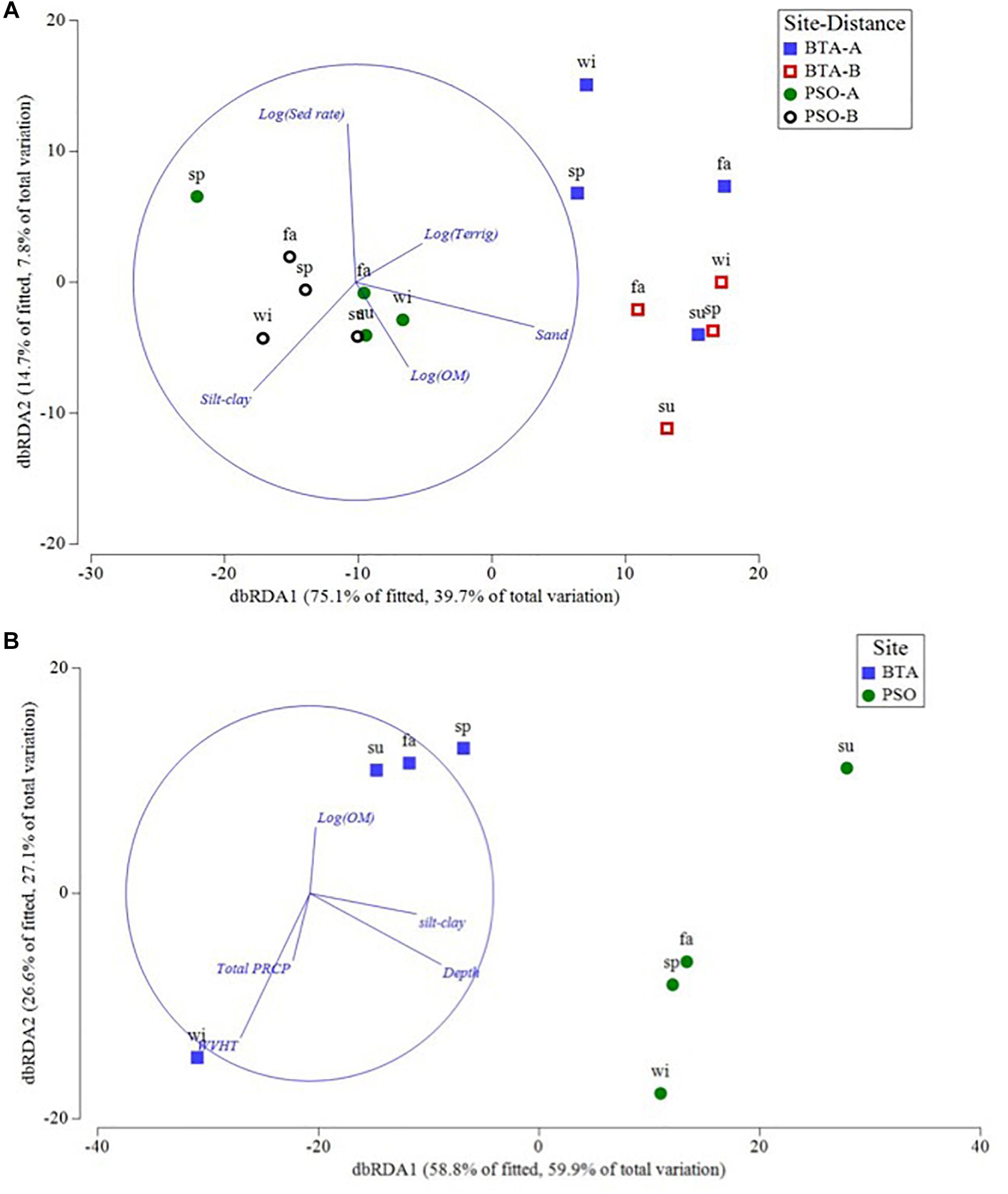
Figure 11. Plot of redundancy analysis (dbRDA) for coral colony abundance (A) and coral recruit abundance (B). Vectors represent environmental variables that best explains variation in coral abundance structure (A) between site by distance zone, and in coral recruit abundance (B) between sites. Blue and red squares represent Bahía Tamarindo (BTA); green and black represent Punta Soldado (PSO). Seasons are defined as: sp, spring; su, summer; fa, fall; wi, winter.
Coral recruit abundance had a strongly significant negative relationship with sand (RELATE, Rho = 0.225, p = 0.017) silt-clay (Rho = 0.24, p = 0.021), and depth (Rho = 0.715, p = 0.032) (Table 3). Multiple linear regression analysis showed that silt-clay (r2 = 0.18, p = 0.020) had a significant association with coral recruit spatial and temporal array. Furthermore, coral recruit abundance also had a strongly significant relationship with wave height (r2 = 0.52, p = 0.0270) and depth (r2 = 0.45, p = 0.0250) for season by site interaction (Figure 10B). Vectors superimposed showed that coral recruit abundance was mostly influenced by silt-clay (<63 μm) and depth at PSO, where higher recruit abundance was documented at shallower areas closer to shore (Figure 11B). On the other hand, wave height had a stronger correlation to recruit abundance at BTA during the winter season, combined to a lesser extent with total precipitation. These environmental associations explain 87% of the total variation for coral recruit abundance. During the study period, peak SST reached up to 30°C, representing an anomaly of +1.35°C in relation to the mean monthly maximum, and might have also contributed to negatively impact coral abundance through undetected coral tissue loss, as coral decline followed high SST episodes. No bleaching was observed during the study period. However, there was no significant relationship between coral recruit abundance and SST (r2 = 0.71, p = 0.37).
The non-parametric correlation BEST BIOENV (Spearman rank) analyses identified two groups of sediment variables that best correlated with coral reef benthic components, composed of sand and carbonate (Rho = 0.42), and sand, organic matter, carbonate, and terrigenous sediment (Rho = 0.412). Changes in percentage coral cover through the study period, 2014 to 2015, correlated with the variation of sand, silt-clay, carbonate, and terrigenous sediment content (p < 0.05) (Table 4). Based on our temporal and spatial analysis, there was a significant negative relationship between coral and macroalgal cover (r2 = 0.70, p < 0.0001). A similar pattern was documented between coral and octocoral cover (r2 = 0.462, p = 0.003). Spatial and temporal variations of macroalgal cover had a significant relationship with most of the sediment variables (p < 0.05), except organic matter (Table 4). Depth gradient across the reefs was correlated with macroalgal cover between seasons by site (Rho = 0.71, p = 0.036).
Sponge cover variation at nearshore reefs had a strong correlation with most of the sediment characteristics assessed, including sedimentation rate (Rho = 0.433, p = 0.002) and terrigenous rate (Rho = 0.371, p = 0.0001) (Table 4). Crustose coralline algae had similar correlations and had significant relationships to sedimentation rate (Rho = 0.256, p = 0.042), terrigenous rate (Rho = 0.295, p = 0.042), among other sediment dynamics, and depth (p < 0.05) (Table 3). Increased cyanobacteria cover, especially at BTA farther from shore, correlated with increased organic matter content (Rho = 0.262, p = 0.012) for the interaction site by distance from shore.
Discussion
Near-shore coral reefs are experiencing rapid and significant spatial and temporal ecological changes in apparent response to human-induced degradation of coastal watersheds and interconnected coastal and marine habitats. In the context of current and forecasted climate change trends, such influences become increasingly significant. This study was primarily correlational and does not prove cause and effect. However, in the absence of other major disturbances during the study period of time (i.e., bleaching, disease outbreaks, hurricanes), documented land-based sediment pulses may have been one of the most important factors causing damaging effects on corals. Documented spatio-temporal patterns of coral reef benthic community structure near shore (<120 m from shore) had associations with sedimentation dynamics and interacting hydrodynamic forces. Sediment characteristics had an important role shaping benthic communities across a distance gradient from shore were sediment texture, categorized as silt-clay (<63 μm) and sand (>63 μm), and terrigenous sediments content (Tables 3, 4). Results from this study suggest that significant spatio-temporal differences at near-shore coral reefs were associated with changing environmental conditions, in combination with increasing influences of coastal hydrodynamics.
Coral colony abundance in this study showed significant spatial and temporal differences and it was significantly associated with sand distribution patterns. Abundance decline during fall and winter seasons was related to the occurrence of extreme and acute weather events caused by a tropical trough and tropical storms that impacted the northern Caribbean region from August to November 2014. These atmospheric events produced the combined effect of sediment-laden runoff from disturbed coastal watersheds and increased wave action on shallow reefs (Otaño-Cruz et al., 2017). Coral reef benthic community structure and its ecological response is not only associated with variation in land-derived sediment input and distribution dynamics but is also related to bathymetry, as well as changes in local weather and oceanographic conditions. Weather and oceanographic dynamics that influence wave height and energy are the main drivers of sand sediment resuspension and transport (Hernández-Cruz et al., 2009; Field et al., 2011; Edmunds and Gray, 2014).
Coral species richness and diversity declined in a short-term period, especially at areas subjected to recurrent runoff and sedimentation stress. Further, seasonal variation was observed in multiple parameters, which also fluctuated between sites and distance zones, suggesting combined effects of natural seasonal variability at the studied spatial scales and terrestrial influences associated with changing land use and human-influenced runoff pulse dynamics. The scleractinian species recorded in BTA and PSO shallow reefs approximately represent two-thirds of the total number of species known for the northeastern region of Puerto Rico (Hernández-Delgado, 2000). The most conspicuous species at reef areas under continual sediment stress were P. astreoides and P. porites. The Porites spp. complex and the Siderastrea spp. complex have been recognized as species with high tolerance to sediment due to their ability to effectively reject particles, and they are becoming more abundant on shallow reefs throughout the Caribbean (Loya, 1976; Cortés and Risk, 1985; Torres and Morelock, 2002; Green et al., 2008; Ennis et al., 2016). Other common coral species at both study sites were A. cervicornis, D. labyrinthiformis, and O. annularis, abundant at reef zones less subjected to sedimentation stress (Figure 3). Transplanting, that was conducted prior to the initiation of this research, produced a high abundance of Acropora spp. across the study sites, showing that these species successfully established in areas less exposed to waves and land-based anthropogenic stress (Figures 3, 8).
Rare and sensitive coral species have disappeared from locations affected by chronic sedimentation regimes across northeastern Puerto Rico (Hernández-Delgado, 2000). Over the last few decades, multiple Caribbean coral reefs have shown similar trends of declining coral diversity and coral abundance at sites impacted by recurrent pulses of sedimentation and LBSP (Loya, 1976; Cortés and Risk, 1985; Pastorok and Bilyard, 1985; Acevedo et al., 1989; Ennis et al., 2016). LBSP effects on coral reefs do not always reflect a discrete spatial gradient from shore since it mostly depends on the characteristics of marine sediment deposited across the reef (Pastorok and Bilyard, 1985) and on the ecosystem capability to process and adapt to sediment, organic matter and nutrient influxes (Lirman and Fong, 2007; Olds et al., 2018). Such changes might have adverse long-term repercussions due to reduced reef accretion and increased vulnerability to future and extreme climate scenarios (Knowlton, 2001; Edmunds, 2010; Hoegh-Guldberg et al., 2014). Degradation of the reef’s structural complexity could further affect social-ecological services provided to coastal communities, including sustaining fish assemblages (Alvarez-Filip et al., 2009; Graham and Nash, 2013; Newman et al., 2015).
Coral recruit abundance of stress-tolerant species, such as S. radians, S. siderea, and P. astreoides, have become dominant in this study, as in many other locations in Puerto Rico. The increasing dominance of brooder species recruits has also been documented across the wider Caribbean (Lirman and Fong, 2007; Green et al., 2008; Edmunds, 2010; Hernández-Delgado et al., 2014a), which could be, not only the result of local environmental changes, but also the result of large-scale phenomena, such as climate change. This trend might be responsible for the overall shift in species composition as community trajectory is skewed toward ephemeral, fast growing, and stress-tolerant species. Under such regime, coral reefs experience a multiplicity of threats and recurrent changes in environmental conditions, thus limiting the success of reef-building species recruit settlement, such as O. annularis and Acropora spp. (Hernández-Delgado, 2000; Van Woesik and Jordán-Garza, 2011). In PSO reef, coral recruit abundance declined on the reef zone farthest from shore (>60 m) and it had a significant relationship with increased the proportion of silt-clay sediment deposition after an extensive deforestation event that disturbed the adjacent coastal watershed. Increased sediment influx and distribution of fine sediments through PSO reef was documented after strong precipitation events that were followed by wind-induced waves and currents that transported fine, land-based sediments until they were deposited in calmer waters (Otaño-Cruz et al., 2017). The effects of cold front events, characteristic of the winter season across the northern Caribbean, can produce strong long-period swells and a significant increase in sedimentation rate by both sediment bedload transport and resuspension (Otaño-Cruz et al., 2017). Previous studies have shown similar patterns of reduced or inhibited recruitment under sediment stress (Pastorok and Bilyard, 1985; Edmunds and Gray, 2014).
Other factors that affect coral recruit abundance are thermal stress (Edmunds, 2004; Van Woesik and Jordán-Garza, 2011), out-competition by fast-growing macroalgae (Nugues et al., 2004), and cyanobacteria (Fong and Paul, 2014). Algal dominance can inhibit coral larval settlement, predominantly when combined with deposited sediments, disturbing long-term coral reef resilience and their ability to recover after disturbance (Birrell et al., 2005; Kuffner et al., 2006; Vermij, 2006; Fong and Paul, 2014; Stubler et al., 2016). Extreme precipitation events have also been identified as a key factor that can trigger land-derived sediment pulses and distribution along near-shore coral reefs (Otaño-Cruz et al., 2017). Increased frequency of extreme rainfall events can have deleterious impacts on local coral populations and on nearshore coral rehabilitation efforts (Hernández-Delgado et al., 2014b). This combination of factors operating at multiple temporal and spatial scales can interact as long-term drivers of coral reef species composition change and should be further addressed.
Trends of live coral cover decline worldwide have often been associated with synergistic and complex local and regional chronic factors (i.e., disease, sedimentation, LBSP, increasing SST). Their combined effects with natural stochastic factors (i.e., hurricanes) have resulted in the long-term loss of primary reef-building species (i.e., O. annularis complex) and in shifts in species composition through the last few decades (Pandolfi et al., 2003; Rogers and Miller, 2006; Knowlton and Jackson, 2008; Miller et al., 2009; Hernández-Pacheco et al., 2011). Although the primary cause of coral decline in the Caribbean has been coral disease (Aronson and Precht, 2001a, b; Weil and Rogers, 2011), terrestrial sediment input is one of the main threats to near-shore coral reefs (Ogston et al., 2004; Hernández-Cruz et al., 2009; Ennis et al., 2016; Otaño-Cruz et al., 2017). Documented differences in percentage coral cover near shore were mostly associated with local sedimentation dynamics, principally with sand and silt-clay sediment distribution patterns. Coral cover decline at reef areas subjected to higher terrigenous sediment accumulation suggests an important relationship between coral reef health and changes in coastal watershed management, weather and local oceanographic dynamics. Documented mean live coral cover of 12.82% positions Culebra Island nearshore reefs below mean coral cover reported for the Caribbean region of 16.80% (Jackson et al., 2014), though this study was limited to shallow fringing reef systems which are often characterized by lower mean percentage live coral cover. However, the observed inverse relationship between live coral and macroalgal cover at nearshore reefs is a strong ecological indicator of degraded water quality conditions resulting from runoff pulse events and other land-derived stressors. Results from this study validate previous studies conducted across the Caribbean region that have recognized that increased human activities and unsustainable development along coastal watersheds can have major negative consequences on live coral cover and coral reef benthic community structure (Acevedo et al., 1989; Fabricius, 2005; Smith et al., 2008; Risk and Edinger, 2011; Bégin et al., 2013; Oleson et al., 2018).
Sediment accumulation on coral surfaces, especially fine sediments, can produce significant adverse physiological responses as a consequence of energy relocation, required to achieve rejection of sediment particles through the production of mucus and ciliary action (Acevedo et al., 1989; Telesnicki and Goldberg, 1995; Woolfe and Larcombe, 1999; Fabricius, 2011). Coral abilities differ between species and coral morphologies, with branching, meandering, and large coral colonies being more tolerant to sediment accumulation (Rogers, 1990; Fabricius, 2005; Sanders and Baron-Szabo, 2005). Field studies conducted at Costa Rica (Cortés and Risk, 1985) and southwest Puerto Rico (Torres and Morelock, 2002) documented that the O. annularis complex experienced significant reduction of growth rates and live tissue cover with increased terrigenous sediment accumulation. Similar trends were evidenced in this study where massive O. annularis composed the lowest live coral cover, and in contrast, P. astreoides and P. porites had the highest benthic cover. Benthic cover dominance by species that can survive in sub-optimal conditions suggests that these reefs have already experienced changes due to a chronic, recurrent land-based stressors.
Other benthic components that correlated with terrigenous sediment deposition were macroalgae, calcareous algae, and sponge percentage cover. Macroalgae, turf, and calcareous algae cover experienced variation in benthic cover in a short time period, particularly the fast-growing Dictyota spp. and encrusting calcareous algae Ramicrusta textilis, which were documented overgrowing dead coral skeleton. In 2011, R. textilis was first documented in Puerto Rican coral reefs (Ballantine et al., 2011; Ballantine and Ruiz, 2013), and continues to outcompete and overgrow multiple coral species (Ballantine et al., 2016). This encrusting species has been documented overgrowing at least 14 species of scleractinian corals, gorgonians, hydrocorals and other algae (Eckrich et al., 2010) and has become a critical factor adversely influencing coral assemblages across the Caribbean region. Its role should be carefully studied. Increased sediment deposition could inhibit fish grazing and promote the growth of macroalgae and filamentous algal turfs (Bellwood and Fulton, 2008). Macroalgae can also proliferate under high nutrient concentration from runoff (Cloern, 2001), under low herbivory pressure due to the slow recovery of Diadema antillarum (Ruiz-Ramos et al., 2011; Rodríguez-Barreras et al., 2018), overfishing (Hernández-Delgado et al., 2006), or due to a combination of any of these factors (Littler et al., 2006). This suggests that coral reef trophic condition is also a critical co-factor, in combination with climate change-related impacts, in shaping coral reef benthic assemblages.
Increased frequency and severity of sedimentation stress and LBSP can increase sponge competition for space and could favor benthic cover of resistant species, thus leading to alternate dominant states (López-Victoria et al., 2006; Chadwick and Morrow, 2011; González-Rivero et al., 2011). Even though the increased cover of encrusting sponge D. funicularis has been recently reported on Caribbean reefs (García-Sais et al., 2016; Kramer et al., 2016), its distribution and coral out-competition effects are still unknown. High octocoral cover documented in deeper areas at PSO concurs with previous studies that have shown increased dominance of octocorals at deeper reefs (Sánchez et al., 1997). Results from this study show that there was an inverse relationship between coral and octocoral cover.
A possible limitation of the study was the lack of resources to assess benthic community and environmental variables at a larger spatial scale to contrast various land uses and coastal management strategies, and the response of coral reefs. Future research could address larger spatial scales and multiple environmental factors affecting water quality, such as nutrient concentration, to identify which factors exert greater influence in coral abundance, coral recruit patterns, coral cover, and macroalgae cover at local and regional levels. It is also imperative to address potential effects of increased frequency of extreme events, including intense storms and wave action, in the context of changing land use and other localized human-driven influences. Hurricanes can have highly destructive mechanical impacts to coral reefs (Woodley et al., 1981; Fenner, 1991; Toledo-Hernández et al., 2018), and in the context of changing land use, they can magnify sediment delivery and resuspension along coastal coral reefs and associated ecosystems.
The potential influence of sedimentation in determining coral reef trajectories highlights the need for a broader understanding of sedimentation dynamics and of coral reef social-ecological responses under variable environmental scenarios, in order to inform decisions and policies to reduce local stressors, improve water quality, and effectively conserve and restore threatened coral reefs.
Author Contributions
AO-C, AM-A, EH-D, EC, and JO-Z conceived and designed the study. AO-C, AM-A, NG-R, DD-M, and EB performed the coral reef monitoring, field sampling, and analysis. AO-C, AM-A, EH-D, EC, and JO-Z analyzed the data. AO-C, AM-A, and EH-D wrote the manuscript.
Funding
This study was possible by the partial funding provided by the CATEC NSF-HDR-0734826, the University of Puerto Rico, Río Piedras Campus, Dean’s Office of Graduate Studies and Research, and Sociedad Ambiente Marino.
Conflict of Interest Statement
The authors declare that the research was conducted in the absence of any commercial or financial relationships that could be construed as a potential conflict of interest.
Acknowledgments
Our appreciation to the Coral Reef Research Group of the Center of Applied Tropical Ecology and Conservation (CATEC) of the University of Puerto Rico, volunteers from the student’s chapter of Sociedad Ambiente Marino (CESAM) for their continuous and enthusiastic assistance with coral reef monitoring, sediment sample collection, and analyses. We thank CATEC, the Department of Environmental Sciences, and the Institute for Tropical Ecosystem Studies for supporting this project by providing access to materials, equipment, and laboratory facilities. Our deepest gratitude to local NGO’s Sociedad Ambiente Marino and CORALations for providing lodging and logistic support. Also, many thanks to Culebra Island community members and collaborators who provided support during site visits, data collection, outreach efforts, conservation initiatives, and much more.
Supplementary Material
The Supplementary Material for this article can be found online at: https://www.frontiersin.org/articles/10.3389/fmars.2019.00551/full#supplementary-material
Footnotes
References
Acevedo, R., Morelock, J., and Olivieri, R. (1989). Modification of coral reef zonation by terrigenous sediment stress. PALAIOS 4, 92–100. doi: 10.2307/3514736
Alvarez-Filip, L., Carricart-Ganivet, J. P., Horta-Puga, G., and Iglesias-Prieto, R. (2013). Shift in coral-assemblages composition do not ensure persistence of reef functionality. Sci. Rep. 3:3486. doi: 10.1038/srep03486
Alvarez-Filip, L., Dulvy, N., Gill, J., Cote, I., and Watkinson, A. (2009). Flattening of Caribbean coral reefs: region-wide declines in architectural complexity. Proc. R. Soc. 276, 3019–3025. doi: 10.1098/rspb.20090339
Anderson, M., Gorley, R., and Clarke, K. (2008). PERMANOVA+ for PRIMER: Guide to Software and Statistical Methods. Plymouth: PRIMER-E.
Aronson, R. B., and Precht, W. F. (2001a). “Evolutionary palaeoecology of Caribbean coral reefs,” in Evolutionary Paleoecology: the Ecological Context of Macro-Evolutionary Change, eds W. D. Allmon and D. J. Bottjer (New York, NY: Columbia University Press), 171–233. doi: 10.7312/allm10994-011
Aronson, R. B., and Precht, W. F. (2001b). White-band disease and the changing face of Caribbean coral reefs. Hydrobiologia 460, 25–38. doi: 10.1023/A:1013103928980
Ballantine, D., Athanasiadis, A., and Ruiz, H. (2011). Notes on the epibenthic marine algae of Puerto Rico X. Additions to the Flora. Botanica Marina 54, 293–302. doi: 10.1515/bot.2011.039
Ballantine, D., and Ruiz, H. (2013). A unique red algal reefs formation in Puerto Rico. Coral Reefs 32:411. doi: 10.1007/s0038-013-1016-2
Ballantine, D., Ruiz, H., Lozada-Troche, C., and Norris, J. (2016). The genus Ramicrusta (Peyssonnelliales, Rhodophyta) in the Caribbean Sea, including Ramicrusta bonairensis sp. nov and Ramicrusta monensis sp. nov. Botanica Marina 56, 417–431. doi: 10.1515/bot-2016-0086
Bégin, C., Wurzbacher, J., and Coté, I. (2013). Variation in benthic communities of eastern Caribbean in relation to surface sediment composition. Mar. Biol. 160, 343–353. doi: 10.1007/s00227-012-2092-5
Bellwood, D., Hughes, T., Folke, C., and Nystrom, M. (2004). Confronting the coral reef crisis. Nature 429, 827–833. doi: 10.1038/nature02691
Bellwood, D. R., and Fulton, C. J. (2008). Sediment-mediated suppression of herbivory on coral reefs: decreasing resilience to rising sea levels and climate change? Limnol. Oceanogr. 53, 2695–2701. doi: 10.4319/lo.2008.53.6.2695
Birrell, C., McCook, L., and Willis, B. (2005). Effects of algal turfs and sediment on coral settlements. Mar. Pollut. Bull. 51, 408–414. doi: 10.1016/j.marpolbul.2004.10.022
Bozec, Y. M., and Mumby, P. J. (2015). Synergistic impacts of global warming on the resilience of coral reefs. Philos. Trans. R. Soc. B 370:20130267. doi: 10.1098/rstb.2013.0267
Chadwick, N. E., and Morrow, K. M. (2011). “Competition among sessile organisms on coral reefs,” in Corals Reefs: an Ecosystem in Transition, eds Z. Dubinsk and N. Stambler (New York, NY: Springer), 347–372. doi: 10.1007/978-94-007-0114-4_20
Clarke, K. R., Gorley, R. N., Somerfield, P. J., and Warwick, R. M. (2014). Changes in Marine Communities: an Approach to Statistical Analysis and Interpretation, 3rd Edn. Plymouth: PRIMER-R.
Clarke, K. R., Somerfield, P. J., and Chapman, M. G. (2006). On resemblance measure for ecological studies, including taxonomic dissimilarities and a zero-adjusted Bray-Curtis coefficient for denuded assemblages. J. Exp. Mar. Biol. Ecol. 300, 55–80. doi: 10.1016/j.jembe.2005.12.017
Clarke, K. R., and Warwick, R. M. (2001). Change in Marine Communities: An Approach to Statistical Analysis and Interpretation, 2nd Edn. Plymouth: PRIMER-E, Ltd., Plymouth Marine Laboratory.
Cloern, J. (2001). Our evolving conceptual model of the coastal eutrophication problem. Mar. Ecol. Prog. Ser. 210, 223–253. doi: 10.3354/meps210223
Cortés, J., and Risk, M. (1985). A reef under siltation stress: Cahuita, Costa Rica. Bull. Mar. Sci. 36, 339–356.
Cróquer, A., Villamizar, E., and Noriega, N. (2002). Environmental factors affecting tissue regeneration of the reef – building coral Montastraea annularis (Faviidae) at Los Roques National Park, Venezuela. Rev. Biol. Trop. 50, 1055–1065.
Díaz-Ortega, G., and Hernández-Delgado, E. (2014). Unsustainable land-based source pollution in a climate change: a roadblock to the conservation and recovery of elkhorn coral Acropora palmata (Lamarck 1816). Nat. Resourc. 5, 561–581. doi: 10.4236/nr.2014.510050
Dudgeon, S. R., Aronson, R. B., Bruno, J., and Precth, W. (2010). Phase shifts and stable states on coral reefs. Mar. Ecol. Prog. Ser. 413, 201–216. doi: 10.3354/meps08751
Dueñas, L., Montenegro, J., Acosta, A., Cárdenas, F., Sepúlveda, M., Vidal, A., et al. (2010). Guide to Scleractinean Coral Recruits From the Caribbean. INVEMAR Seride de Documentos Generales No. 42. Bogotá: XPRESS Estudio Gráfico y Digital.
Eakin, C., Morgan, J., Heron, S., Smith, T., Liu, G., Weil, E., et al. (2010). Caribbean coral in crisis: record thermal stress, bleaching and mortality in 2005. PLoS One 5:e13969. doi: 10.1371/journal.pone.0013969
Eckrich, C., Peachey, R., and Engel, M. (2010). Crustose, calcareous algal bloom (Ramicrusta sp.) overgrowing scleractinian corals, gorgonians, a hydrocoral, sponges, and other algae in Lac Bay, Bonaire, Dutch Caribbean. Coral Reefs 30:131. doi: 10.1007/s00338-010-0683-5
Edmunds, P. (2004). Juvenile coral population dynamics track rising seawater temperature on a Caribbean reef. Mar. Ecol. Prog. Ser. 269, 111–119. doi: 10.3354/meps269111
Edmunds, P. (2010). Population biology of Porites astreoides and Diploria strigosa on a shallow Caribbean reef. Mar. Ecol. Prog. Ser. 418, 87–104. doi: 10.3354/meps08823
Edmunds, P. (2013). Decadal-scale changes in the community structure of coral reefs of St. John, US Virgin Islands. Mar. Ecol. Prog. Ser. 489, 107–123. doi: 10.3354/meps10424
Edmunds, P., and Gray, S. (2014). The effects of storms, heavy rains and sedimentation on the shallow coral reefs in St. John, US Virgin Islands. Hydrobiologia 734, 143–158. doi: 10.1007/s10750-014-1876-7
Ennis, R., Brandt, M., Wilson, K., and Smith, T. (2016). Coral reef health response to chronic and acute changes in water quality in St. Thomas, United States Virgin Islands. Mar. Pollut. Bull. 111, 418–427. doi: 10.1016/j.marpolbul.2016.07.033
Fabricius, K. (2005). Effects of terrestrial runoff on the ecology of corals and coral reefs: review and synthesis. Mar. Pollut. Bull. 50, 125–146. doi: 10.1016/j.marpolbul.2004.11.028
Fabricius, K. (2011). “Factors determining the resilience of coral reefs to eutrophication: a review and conceptual model,” in Coral Reefs: An Ecosystem in Transition, eds Z. Dubinsky and N. Stambler (New York, NY: Springer), 493–505. doi: 10.1007/978-94-007-0114-4_28
Fenner, D. P. (1991). Effects of hurricante Gilbert on coral reefs, fishes and spsicnes at Cozumel, Mexico. Bull. Mar. Sci. 48, 719–730.
Field, M. E., Ogston, A. S., and Storlazzi, C. D. (2011). Rising sea level may cause decline of fringing coral reefs. EOS 92, 273–280. doi: 10.1029/2011EO330001
Fong, P., and Paul, V. J. (2014). “Coral reef algae,” in Corals Reefs: An Ecosystem in Transition, eds Z. Dubinsky and N. Stambler (New York, NY: Springer), 241–272. doi: 10.1007/978-94-007-0114-4_17
García-Sais, J., Esteves, R., William, S., Sabater-Clavell, J., and Carlo, M. (2016). Monitoring of Coral Reef Communities from Natural Reserves in Puerto Rico: 2016. Silver Spring, MD: NOAA, 1–241.
Gardner, T., Cote, I., Gill, J., Grant, A., and Watkinson, A. (2003). Long-term region-wide declines in Caribbean Corals. Science 301, 958–960. doi: 10.1126/science.1086050
González-Rivero, M., Yakob, L., and Mumby, P. (2011). The role of sponge competition on coral reef alternative steady states. Ecol. Model. 222, 1847–1853. doi: 10.1016/j.ecolmodel.2011.03.020
Gotelli, N. J., and Ellison, G. N. (eds) (2013). A Primer of Ecological Statistics, 2nd Edn. Massachusetts, MA: Sunderland.
Graham, N., and Nash, K. (2013). The importance of structural complexity in coral reefs ecosystems. Coral Reefs 32, 315–326. doi: 10.1007/s00338-012-0984-y
Green, D., Edmunds, P., and Carpenter, R. (2008). Increasing relative abundance of Porites astreoides on Caribbean reefs mediated by an overall decline in coral cover. Mar. Ecol. Prog. Ser. 359, 1–10. doi: 10.3354/meps07454
Hernández-Cruz, R., Sherman, C., Weil, E., and Yoshioka, P. (2009). Spatial and temporal patterns in reef sediment accumulation and composition, southwestern insular shelf of Puerto Rico. Caribbean J. Sci. 2-3, 138–150. doi: 10.18475/cjos.v45i2.a3
Hernández-Delgado, E., Ramos-Scharron, C., Guerrrero-Pérez, C., Lucking, M., Laureano, R., Méndez-Lázaro, P., et al. (2012). “Long-term impacts of non-sustainable tourism and urban development in small tropical islands coastal habitat in a changing climate: lessons learned from Puerto Rico,” in Visions for Global Tourism Industry- Creating and Sustaining Competitive Strategies, ed. M. Kasimoglu (London: InTech Publications), 358–398. doi: 10.5772/38140
Hernández-Delgado, E., Rosado, B., and Sabat, A. (2006). “Management failures and coral decline threatens fish functional groups recovery patterns in the Luis Peña channel no-take natural reserve,” in Proceedings 57th Gulf and Caribbean Fisheries Institute, (Culebra Island, PR), 577–605.
Hernández-Delgado, E. A. (2000). Effects of Anthropogenic Stress Gradients in the Structure of Coral Reef Epibenthic and Fish Communities. Ph.D. Dissertation, University of Puerto Rico: San Juan, PR.
Hernández-Delgado, E. A. (2015). The emerging threats of climate change on tropical coastal ecosystem services, public health, local economies and livelihood sustainability of small islands: cumulative impacts and synergies. Mar. Pollut. Bull. 101, 5–28. doi: 10.1016/j.marpolbul.2015.09.018
Hernández-Delgado, E. A., González-Ramos, C., and Alejandro-Camis, P. (2014a). Large-scale coral recruitment patterns on Mona Island Puerto Rico: evidence of a transitional community trajectory after massive coral bleaching and mortality. Rev. Biol. Trop. 62, 49–64.
Hérnandez-Delgado, E. A., Hutchinson-Delgado, Y., Laureano, R., Hernández-Pacheco, R., Ruiz-Maldonado, T., Oms, J., et al. (2011). “Sediment stress, water turbidity and sewage impacts on threatened Elkhorn coral (Acropora palmata) stands at Vega Baja, Puerto Rico,” in Proceedings of the 63rd Gulf and Caribbean Fisheries Institute, Puerto Rico, 83–92.
Hernández-Delgado, E. A., Mercado-Molina, A., Alejandro-Camis, P., Candelas-Sánchez, F., Fonseca-Miranda, J., González-Ramos, C., et al. (2014b). Community-based coral reef rehabilitation in a changing climate: lessons learned from hurricanes, extreme rainfall, and changing land use impacts. Open J. Ecol. 4, 918–944. doi: 10.4236/oje.2014.414077
Hernández-Delgado, E. A., Mercado-Molina, A. E., and Suleimán-Ramos, S. E. (2018). “Multi-disciplinary lessons learned from low-tech coral farming and reef rehabilitation: I. Best management practices,” in Proceedings of the Coral in Changing World, eds C. Duque and E. Tello Camacho (Croatia: InTech), 213–244. doi: 10.5772/intechopen.73151
Hernández-Pacheco, R., Hernández-Delgado, E., and Sabat, A. (2011). Demographics of bleaching in a major Caribbean reef-building coral: Montastraea annularis. Ecosphere 2, 1–13. doi: 10.1890/ES10-00065.1
Hoegh-Guldberg, O., Cai, E. S., Poloczanska, P. G., Brewer, S., Sundby, K., Hilmi, V. J., et al. (eds) (2014). “Chapter 30. The ocean,” in Climate Change 2014: Impacts, Adaptation, and Vulnerability. Part B: Regional Aspects. Contribution of Working Group II to the Fifth Assessment Report of the Intergovernmental Panel on Climate Change, Vol. 2, eds V. R. Barros, C. B. Field, D. J. Dokken, M. D. Mastrandrea, K. J. Mach, T. E. Bilir, et al. (Cambridge: Cambridge University Press), 1655–1731.
Hughes, T., and Connell, J. (1999). Multiple stressors on coral reefs: a long-term perspective. Limnol. Oceanogr. 44, 932–940. doi: 10.43/lo.1999.44.3_part_2.0932
Hughes, T., Graham, N., Jackson, J., Mumby, P., and Steneck, R. (2010). Raising the challenge of sustaining coral reef resilience. Trends Ecol. Evol. 25, 633–642. doi: 10.1016/j.tree.2010.07.011
Hughes, T. P. (1994). Catastrophes, phase-shifts, and large-scale degradation of a Caribbean coral reef. Science 265, 1547–1551. doi: 10.1126/science.265.5178.1547
Jackson, J., Donovan, M., Cramer, K., and Lam, W. (eds) (2014). Status and Trends of Caribbean Coral Reefs: 1970-2012. Gland: IUCN.
Knowlton, N. (2001). The future of coral reefs. Proc. Natl. Acad. Sci. U.S.A. 98, 5419–5425. doi: 10.1073/pnas.091092998
Knowlton, N., and Jackson, J. (2008). Shifting baselines, local impacts, and global change on coral reefs. PLoS Biol. 6:e54. doi: 10.1371/journal.pbio.0060054
Kramer, P., Atis, M., Schill, S., Williams, S., Freid, E., Moore, G., et al. (2016). Baseline Ecological Inventory for Three Bays National Park, Haiti. Arlington, TX: The Nature Conservancy, 1–180.
Kuffner, I., Walters, I., Becerro, M., Paul, V., Ritson-Williams, R., and Beach, K. (2006). Inhibition of coral recruitment by macroalgae and cyanobacteria. Mar. Ecol. Prog. Ser. 323, 107–111. doi: 10.3354/meps323107
Larsen, M. C., and Webb, R. M. (2009). Potential effects of runoff, fluvial sediment and nutrient discharges on the coral reefs of Puerto Rico. J. Coast. Res. 25, 189–208. doi: 10.2112/07-0920.1
Lirman, D., and Fong, P. (2007). Is proximity to land-based sources of coral stressors an appropriate measure of risk to coral reefs? An example from the Florida Reef Tract. Mar. Pollut. Bull. 54, 779–791. doi: 10.1016/j.marpolbul.2006.12.014
Littler, M., Littler, D., and Brooks, B. (2006). Harmful algae on tropical coral reefs, bottom-up eutrophication and top-down herbivory. Harmful Algae 5, 565–585. doi: 10.1016/j.hal.2005.11.003
López-Victoria, M., Zea, S., and Weil, E. (2006). Competition for space between encrusting excavating Caribbean sponge and other coral reef organisms. Mar. Ecol. Prog. Ser. 312, 113–121. doi: 10.3354/meps312113
Loya, Y. (1976). Effects of water turbidity and sedimentation on the community structure of Puerto Rican corals. Bull. Mar. Sci. 26, 450–466.
Miller, J., Muller, E., Rogers, C., Waara, R., Atkinson, A., Whelan, K. R., et al. (2009). Coral disease following massive bleaching in 2005 causes 60% decline in coral cover on reefs in the US Virgin Islands. Coral Reefs 28, 925–937. doi: 10.1007/s00338-009-0531-7
Mumby, P. L., and Steneck, R. S. (2011). “The resilience of coral reefs and its implication for reef management,” in Coral Reefs: An Ecosystem in Transition, eds Z. Dubinsky and N. Stambler (New York, NY: Springer), 509–519. doi: 10.1007/978-94-007-0114-4_29
Newman, S., Meesters, E., Dryden, C., Williams, S., Sanchez, C., Mumby, P., et al. (2015). Reef flattening effects on total richness and species response in the Caribbean. J. Anim. Ecol. 84, 1678–1689. doi: 10.1111/1365-2656.12429
NOAA (2015). Shallow-Water Benthic Habitats of Northeast Puerto Rico and Culebra Island. NOAA Technical Memorandum NOS NCCOS 200. Silver Spring, MD: NOAA, 112.
Nugues, M., Smith, G., van Hooidonk, R., Seabra, M., and Bak, R. (2004). Algal contact as a trigger for coral disease. Ecol. Lett. 7, 919–923. doi: 10.1111/j.1461-0248.2004.00651.x
Nugues, M. M., and Roberts, C. M. (2003a). Coral mortality and interaction with algae on relation to sedimentation. Coral Reefs 22, 507–516. doi: 10.1007/s00338-003-0338-x
Nugues, M. M., and Roberts, C. M. (2003b). Partial mortality in massive reef corals as an indicator of sediment stress on coral reefs. Mar. Pollut. Bull. 46, 314–323. doi: 10.1016/S0025-326X(02)00402-2
Ogston, A. S., Storlazzi, C. D., Field, M. E., and Presto, M. K. (2004). Sediment resuspension and transport patterns on a fringing reef flat, Molokai, Hawaii. Coral Reefs 23, 559–569. doi: 10.1007/s00338-004-0415-9
Olds, A., Nagelkerken, I., Huijibers, C., Gilby, B., Pittma, S., and Schalacher, T. (2018). “Connectivity in coastal seascapes,” in Seascape Ecology, ed. S. Pittman (New Jersey, NJ: John Wiley & Sons), 261–292.
Oleson, K., Falinski, K., Audas, D., Coccia-Schillo, S., Groves, P., Teneva, L., et al. (2018). “Linking landscape and seascape conditions: science, tools and management,” in Seascape Ecology, ed. S. Pittman (New Jersey, NJ: John Wiley & Sons), 319–364.
Otaño-Cruz, A., Montañez-Acuña, A. A., Torres-López, V., Hernández-Figueroa, E. M., and Hernández-Delgado, E. A. (2017). Effects of changing weather, oceanographic conditions, and land uses on spatio-temporal variation of sedimentation dynamics along near-shore coral reefs. Front. Mar. Sci. 4:249. doi: 10.3389/fmars.2017.00249
Pandolfi, J., Bradbury, R., Sala, E., Hughes, T., Bjorndal, K., Cooke, R., et al. (2003). Global trajectories of the long-term decline of coral reef ecosystems. Science 301, 955–957. doi: 10.1126/science.1085706
Pastorok, R., and Bilyard, G. (1985). Effects of sewage pollution on coral-reef communities. Mar. Ecol. 21, 175–189. doi: 10.3354/meps021175
Pielou, E. (1966). The measurement of diversity in different types of biological collections. J. Theor. Biol. 13, 131–144. doi: 10.1016/0022-5193(66)90013-0
Pollock, J., Lamb, J., Field, S., Heron, S., Schaffelke, B., and Shedrawi, G. (2014). Sediment and turbidity associated with offshore dredging increase coral disease prevalence on nearby reefs. PLoS One 9:e102498. doi: 10.1371/journal.pone.0102498
Ramos-Scharrón, C., Amador, J., and Hernandez-Delgado, E. (2012). “An interdisciplinary erosion mitigation approach for coral reef protection – a case study form the Eastern Caribbean,” in Marine Ecosystems, ed. A. Cruzado (London: InTech Publications), 127–160. doi: 10.5772/35709
Ramos-Scharrón, C., and MacDonald, L. (2007). Development and application of GIS-based sediment budget model. J. Environ. Manag. 84, 157–172. doi: 10.1016/j.jenvman.2006.05.019
Ramos-Scharrón, C., Torres-Pulliza, D., and Hernández-Delgado, E. (2015). Watershed- and island wide-scale land cover changes in Puerto Rico (1930-2004) and their potential effects on coral reef ecosystems. Sci. Total Environ. 506-507, 241–251. doi: 10.1016/j.scitotenv.2014.11.016
Risk, M. J., and Edinger, E. (2011). “Impacts of sediment on coral reefs,” in Encyclopedia of Modern Coral Reefs, ed. D. Hopely (Dordrecht: Springer), 575–585. doi: 10.1007/978-90-481-2639-2_25
Rivera-Monroy, V. H., Twilley, R. H., Bone, D., Childers, D., Coronado-Molina, C., Feller, I. C., et al. (2004). A conceptual framework to develop long-term ecological research and management objective in the wider Caribbean region. BioScience 54, 843–856.
Rodríguez-Barreras, R., Montañez-Acuña, A., Otaño-Cruz, A., and Ling, D. S. (2018). Apparent stability of a low-density Diadema antillarum regime for Puerto Rican coral reefs. ICES J. Mar. Sci. 75, 2193–2201. doi: 10.1093/icesjms/fsy093
Rogers, C. (1990). Response of coral reefs and reef organisms to sedimentation. Mar. Ecol. Prog. Ser. 62, 185–202. doi: 10.3354/meps062185
Rogers, C., and Miller, J. (2006). Permanent ‘phase shifts’ or reversible declines in coral cover? Lack of recovery of two coral reefs in St. John, US Virgin Islands. Mar. Ecol. Prog. Ser. 306, 103–114. doi: 10.3354/meps306103
Ruiz-Ramos, D., Hernández-Delgado, E. A., and Schizas, N. (2011). Population status of the long-spine sea urchin, Diadema antillarum in Puerto Rico 20 years after a mass mortality event. Bull. Mar. Sci. 87, 113–127. doi: 10.5343/bms.2010.1038
Sánchez, J., Día, J., and Zea, S. (1997). Gorgonian communities in two contrasting environments on oceanic atolls of the Southwestern Caribbean. Bull. Mar. Sci. 61, 453–465.
Sanders, D., and Baron-Szabo, R. (2005). Scleractinian assemblages under sediment input: their characteristics and relation to the nutrient input concept. Palaeogeogr. Palaeoclimatol. Palaeoecol. 261, 139–181. doi: 10.106/j.palaeo.2004.10.008
Shannon, C. E., and Weaver, W. (1948). The Mathematical Theory of Communication. Urbana, IL: University of Illinois Press.
Smith, T., Nemeth, R., Blondeau, J., Calnan, J. M., Kadison, E., and Herzlieb, S. (2008). Assessing coral reef health across onshore to offshore stress gradients in the US Virgin Islands. Mar. Pollut. Bull. 56, 1983–1991. doi: 10.1016/j.marpollbul.2008.08.015
Stubler, A., Stevens, A., and Peterson, B. (2016). Using community-wide recruitment and succession patterns to assess sediment stress on Jamaican coral reefs. J. Mar. Biol. Ecol. 474, 29–38. doi: 10.1016/j.jembe.2015.09.018
Sturm, P., Viqueira-Rios, R., Meyer-Comas, L., Hernández-Delgado, E., González-Ramos, C., Montañez-Acuña, A., et al. (2014). Culebra Community Watershed Action Plan for Water Quality and Coral Reefs. Silver Spring, MD: NOAA, 76.
Telesnicki, G., and Goldberg, W. (1995). Effects of turbidity on the photosynthesis and respiration of two south Florida reef coral species. Bull. Mar. Sci. 57, 527–0539. doi: 10.1371/journal.pone.0107195
Toledo-Hernández, C., Ruiz-Diaz, C. P., Hernández-Delgado, E. A., and Suleimán-Ramos, S. E. (2018). Devastation of 15-year old community-based coral farming and reef-restoration sites in Puerto Rico by major hurricanes Irma and María. Caribbean Nat. 53, 1–6.
Toledo-Hernández, C., Sabat, A. M., and Zuluaga-Montero, A. (2007). Density, size structure and asperigillosis prevalence in Gorgonia ventalina at six localities in Puerto Rico. Mar. Biol. 152, 527–535. doi: 10.1007/s00227-007-0699-8
Torres, J., and Morelock, J. (2002). Effect of terrigenous sediment influx on coral cover and linear extension rates of three Caribbean massive coral species. Caribbean J. Sci. 38, 222–229.
Van Woesik, R., and Jordán-Garza, A. (2011). Coral population in a rapidly changing environment. J. Exp. Mar. Biol. 408, 11–20. doi: 10.1016/j.jembe.2011.07.022
Vermij, M. (2006). Early life history of dynamics of Caribbean coral species on artificial substratum: the importance of competition, growth, and variation in life-history strategy. Coral Reefs 25, 59–71. doi: 10.1007/s00338-005-0056-7
Weil, E., and Rogers, C. (2011). “Coral reef diseases in the Atlantic-Caribbean,” in Corals Reefs: An Ecosystem in Transition, eds Z. Dubinsky and N. Stambler (New York, NY: Springer), 465–491. doi: 10.1007/978-94-007-0114-4_27
Wild, C., Hoegh-Guldberg, O., Naumann, M. S., Colombo-Pallota, F., Ateweberhan, M., Fitt, W. K., et al. (2011). Climate change impedes scleractinian corals as primary reef ecosystem engineers. Mar. Freshw. Res. 62, 205–215. doi: 10.1071/MF10254
Wilkinson, C., and Souter, D. (2008). Status of Caribbean Coral Reefs After Bleaching and Hurricanes in 2005. Townsville, QLD: Global Coral Reef Monitoring Network and Reef and Rainforest Research Center, 152.
Woodley, J. D., Chornesky, E. A., Clifford, P. A., Jackson, J. B., Kaufman, L. S., Knowlton, N., et al. (1981). Hurricane Allen’s impact on Jamaican coral reefs. Science 214, 749–755. doi: 10.1126/science.214.4522.749
Keywords: benthic cover, coral abundance, coral diversity, coral recruit, coral reef community structure, environmental stressors, near-shore coral reefs, sedimentation dynamics
Citation: Otaño-Cruz A, Montañez-Acuña AA, García-Rodríguez NM, Díaz-Morales DM, Benson E, Cuevas E, Ortiz-Zayas J and Hernández-Delgado EA (2019) Caribbean Near-Shore Coral Reef Benthic Community Response to Changes on Sedimentation Dynamics and Environmental Conditions. Front. Mar. Sci. 6:551. doi: 10.3389/fmars.2019.00551
Received: 08 November 2018; Accepted: 21 August 2019;
Published: 06 September 2019.
Edited by:
Edward Jeremy Hind-Ozan, Department for Environment, Food and Rural Affairs, United KingdomReviewed by:
Xiaoshou Liu, Ocean University of China, ChinaDouglas Fenner, Independent Researcher, Pago Pago, American Samoa
Copyright © 2019 Otaño-Cruz, Montañez-Acuña, García-Rodríguez, Díaz-Morales, Benson, Cuevas, Ortiz-Zayas and Hernández-Delgado. This is an open-access article distributed under the terms of the Creative Commons Attribution License (CC BY). The use, distribution or reproduction in other forums is permitted, provided the original author(s) and the copyright owner(s) are credited and that the original publication in this journal is cited, in accordance with accepted academic practice. No use, distribution or reproduction is permitted which does not comply with these terms.
*Correspondence: Edwin A. Hernández-Delgado, edwin.hernandezdelgado@gmail.com