Corrigendum: ILTER – The International Long-Term Ecological Research Network as a Platform for Global Coastal and Ocean Observation
- 1Instituto de Oceanografia, Universidade Federal do Rio Grande, Rio Grande, Brazil
- 2Institute for Marine Sciences and Antarctic Studies, University of Tasmania, Hobart, TAS, Australia
- 3Department of Geography and Marine Science Institute, University of California, Santa Barbara, Santa Barbara, CA, United States
- 4Department of Sciences, Roma Tre University, Rome, Italy
- 5Biology Department, Woods Hole Oceanographic Institution, Woods Hole, MA, United States
- 6Grupo de Ecologia Bêntica, Departamento de Oceanografia, Universidade Federal do Espirito Santo, Vitoria, Brazil
- 7Marine Ecology Laboratory, Institute of Biology, University of Latvia, Riga, Latvia
- 8South African Environmental Observation Network, Elwandle Coastal Node, Port Elizabeth, South Africa
- 9Coastal and Marine Research Institute, Nelson Mandela University, Port Elizabeth, South Africa
- 10Istituto Nazionale di Oceanografia e di Geofisica Sperimentale, Trieste, Italy
- 11Flanders Marine Institute, Ostend, Belgium
- 12Alfred Wegener Institute, Helmholtz Center for Polar and Marine Research, Bremerhaven, Germany
- 13Akkeshi Marine Station, Field Science Center for Northern Biosphere, Hokkaido University, Sapporo, Japan
- 14National Research Council – Institute of Marine Sciences, Venice, Italy
- 15LTSER “Zone Atelier Brest-Iroise”, Laboratoire des Sciences de l’Environnement Marin, Institut Universitaire Européen de la Mer, Plouzané, France
- 16Biologische Anstalt Helgoland, Alfred Wegener Institute, Helmholtz Center for Polar and Marine Research, Bremerhaven, Germany
- 17Dipartimento di Bioscienze e Territorio, Università del Molise, Termoli, Italy
- 18Institute of Oceanology, Bulgarian Academy of Sciences, Varna, Bulgaria
- 19LTSER “Zone Atelier Brest-Iroise”, Centre National de la Recherche Scientifique, Laboratoire LETG, Institut Universitaire Européen de la Mer, Plouzané, France
- 20Umeå Marine Sciences Center, Umeå University, Umeå, Sweden
- 21Stazione Zoologica Anton Dohrn, Naples, Italy
Understanding the threats to global biodiversity and ecosystem services posed by human impacts on coastal and marine environments requires the establishment and maintenance of ecological observatories that integrate the biological, physical, geological, and biogeochemical aspects of ecosystems. This is crucial to provide scientists and stakeholders with the support and knowledge necessary to quantify environmental change and its impact on the sustainable use of the seas and coasts. In this paper, we explore the potential for the coastal and marine components of the International Long-Term Ecological Research Network (ILTER) to fill this need for integrated global observation, and highlight how ecological observations are necessary to address the challenges posed by climate change and evolving human needs and stressors within the coastal zone. The ILTER is a global network encompassing 44 countries and 700 research sites in a variety of ecosystems across the planet, more than 100 of which are located in coastal and marine environments (ILTER-CMS). While most of the ILTER-CMS were established after the year 2000, in some cases they date back to the early 1900s. At ILTER sites, a broad variety of abiotic and biotic variables are measured, which may feed into other global initiatives. The ILTER community has produced tools to harmonize and compare measurements and methods, allowing for data integration workflows and analyses between and within individual ILTER sites. After a brief historical overview of ILTER, with emphasis on the marine component, we analyze the potential contribution of the ILTER-CMS to global coastal and ocean observation, adopting the “Strength, Weakness, Opportunity and Threats (SWOT)” approach. We also identify ways in which the in situ parameters collected at ILTER sites currently fit within the Essential Ocean Variables framework (as proposed by the Framework for Ocean Observation recommendations) and provide insights on the use of new technology in long-term studies. Final recommendations point at the need to further develop observational activities at LTER sites and improve coordination among them and with external related initiatives in order to maximize their exploitation and address present and future challenges in ocean observations.
Introduction
Human activities threaten both the natural functioning of coastal and marine ecosystems and their sustainable use by present and future generations (Worm et al., 2006; Defeo et al., 2009; Halpern et al., 2012; Howes et al., 2015; Drius et al., 2016; Malavasi et al., 2018). Developing and delivering the ecological knowledge necessary to quantify how threats to coastal ecosystems impact national and international economies, policies and the sustainable use of the sea poses a significant challenge. Gaining such ecological knowledge on a local scale requires long-term observations of both environmental and biological variables, such as temperature and species richness. Effective assessment, management and prediction at the global scale, however, requires infrastructure and ecological observatories capable of systematically integrating from a long-term, large-scale, and whole-system perspective. Developing new observing systems and strengthening existing initiatives in a coordinated, standardized, global effort is essential to address these challenges.
Marine observatories provide the infrastructure and logistical support necessary to acquire data and knowledge for these purposes. The Global Ocean Observing System (GOOS1) was established to provide a sustained, collaborative system for ocean observation that brings together in situ networks, remote sensing systems, government stakeholders, UN agencies and individual scientists. An important outcome of OceanObs’09 was the recognition of an imbalance between physical and biogeochemical/biological/ecological observations within most observing systems and the recommendation that GOOS consider how to expand the scope of observations to best address socially pressing global issues, including food security, harmful algal blooms, the spread of dead zones, and biodiversity conservation (Lindstrom et al., 2012). To that end, GOOS has established Biology and Ecosystem Essential Ocean Variables (EOVs2, Miloslavich et al., 2018). The International Long-Term Ecological Research Network (ILTER3) is uniquely poised to contribute to the need for integrated, global observation of biological and ecological aspects of coastal ecosystems. Focal areas of the ILTER coastal and marine sites (henceforth ILTER-CMS) include: the consequences of biodiversity alteration for ecosystem functioning and services, documenting productivity changes, and understanding the cumulative impacts of multiple stressors including overfishing and ocean acidification. In this paper we explore the coastal and marine component of ILTER as a platform for global coastal and ocean observation, highlighting the role of ecological observations to address the challenges posed by climate change and evolving human needs and stressors within the coastal zone. To begin, we provide a brief historical overview of ILTER and describe its organization, with emphasis on the marine component. Next, we analyze the potential contribution of the ILTER marine component to global coastal and ocean observation adopting the “Strength, Weakness, Opportunity and Threats (SWOT)” approach. From this analysis, we elaborate on two specific opportunities for ILTER-CMS to contribute to global ocean observing. One, we identify ways that the in situ parameters collected at ILTER sites fit within the EOVs framework, as proposed by the “Framework for Ocean Observation” (FOO, Lindstrom et al., 2012) recommendations, and, two, provide insights on the use of new technology in long-term studies. Finally, we recommend paths forward to address future challenges.
Historical Perspective and Overview of ILTER
The ILTER was established in 1993, 13 years after the launch of the Long-Term Ecological Research program by the National Science Foundation (NSF) of the United States, as a means of coordinating synthesis between LTER sites. Fully supported by the NSF until 2003, ILTER gradually became a self-reliant network, growing from three founding members to 34 organizations by 2006, when a 10-year strategic plan was ratified (ILTER, 2006). At that time, ILTER transitioned to a broader disciplinary approach that included researchers, managers and stakeholders (ILTER, 2006). In 2007, the ILTER Association was founded in Costa Rica, making the ILTER a legal entity with its own governance structure, unifying strategy, operational goals and by-laws (Mirtl et al., 2018). Formally, member networks join the ILTER Association.
The ILTER Network provides a globally distributed network of long-term research sites for multiple purposes and uses in the fields of ecosystem, biodiversity, critical zone, and socio-ecological research (Mirtl et al., 2018). It currently consists of 44 national networks with robust governance structures, managing more than 700 sites worldwide, with a systematic coverage of terrestrial, freshwater, and marine environments (Haase et al., 2018; Mirtl et al., 2018). This site-based research network measures a broad variety of abiotic and biotic environmental variables, which may feed into other global initiatives. LTER national networks have mainly been developed from the bottom–up and LTER sites were established for different research and monitoring purposes. The ILTER community has produced tools to harmonize and compare measurements and methods, allowing for data integration workflows and analyses between and within individual LTER sites, to ensure the highest quality interoperable services in close interaction with related regional and global research infrastructures and networks (Haase et al., 2018; Mirtl et al., 2018). Long Term Socio-economic and Ecosystem Research (LTSER) platforms emerged as initiatives aimed at enhancing the capacity of ecological knowledge combined with social science to produce useful knowledge for facing global environmental challenges (Mauz et al., 2012). This emphasis in the ILTER network reflects the desire to produce knowledge particularly useful for addressing complex environmental challenges emerging from nature-society interactions (Dick et al., 2018).
One of the goals of ILTER is to improve the comparability of site metadata and of long-term ecological data, facilitating their exchange and preservation around the world. ILTER member networks are committed to free and open data sharing (Vanderbilt et al., 2010; Vanderbilt and Gaiser, 2017), in agreement with the F.A.I.R (Findable, Accessible, Interoperable, and Reusable) principles for data management and Open Science (European Commission, 2016 ‘FAIR’; Mirtl et al., 2018; Tanhua et al., 2019). The Dynamic Ecological Information Management System Site and Dataset Registry (DEIMS-SDR4) provides a common and standardized metadata catalog for the distinct identification of observation facilities (e.g., sites, stations, sensors, datasets, persons) used by ILTER members. DEIMS-SDR also provides a web-based service to document and share scientific datasets, implements the ILTER community profile (Kliment and Oggioni, 2011), and allows the export to different XML formats (e.g., EML 2.1.1, BDP, ISO19115, INSPIRE). ILTER identified DataONE as the main facility to share and distribute ILTER data, but it also shares data through the GEOSS (Group on Earth Observation System of Systems5) Data Portal. ILTER agrees with the open data principles at the global scale in principle, but putting them into practice is still a challenging issue in most member networks and at the site level.
The Coastal and Marine ILTER Sites (ILTER-CMS)
There are 63 coastal and 52 marine sites in the ILTER (Figure 1 and Supplementary Table S1). Based on classifications in the ILTER’s DEIMS-SDR, coastal sites include sand dunes and beaches, lagoons, estuaries, river deltas, fjords, salt marshes and mangroves, while marine sites are located on continental shelves and oceanic islands (Figure 2). Nearly half of the CMS include data records that precede the formal establishment of the ILTER (Figure 3). For example, the “Dutch Wadden Sea Area” in the Netherlands has records dating to 1872. Observations began in the Western Gulf of Finland in 1902; the Mar Piccolo of Taranto, Italy in 1914; and Shirahama, Japan in 1922. The length of these observations enhances the opportunities for ILTER-CMS to contribute to documenting global change.
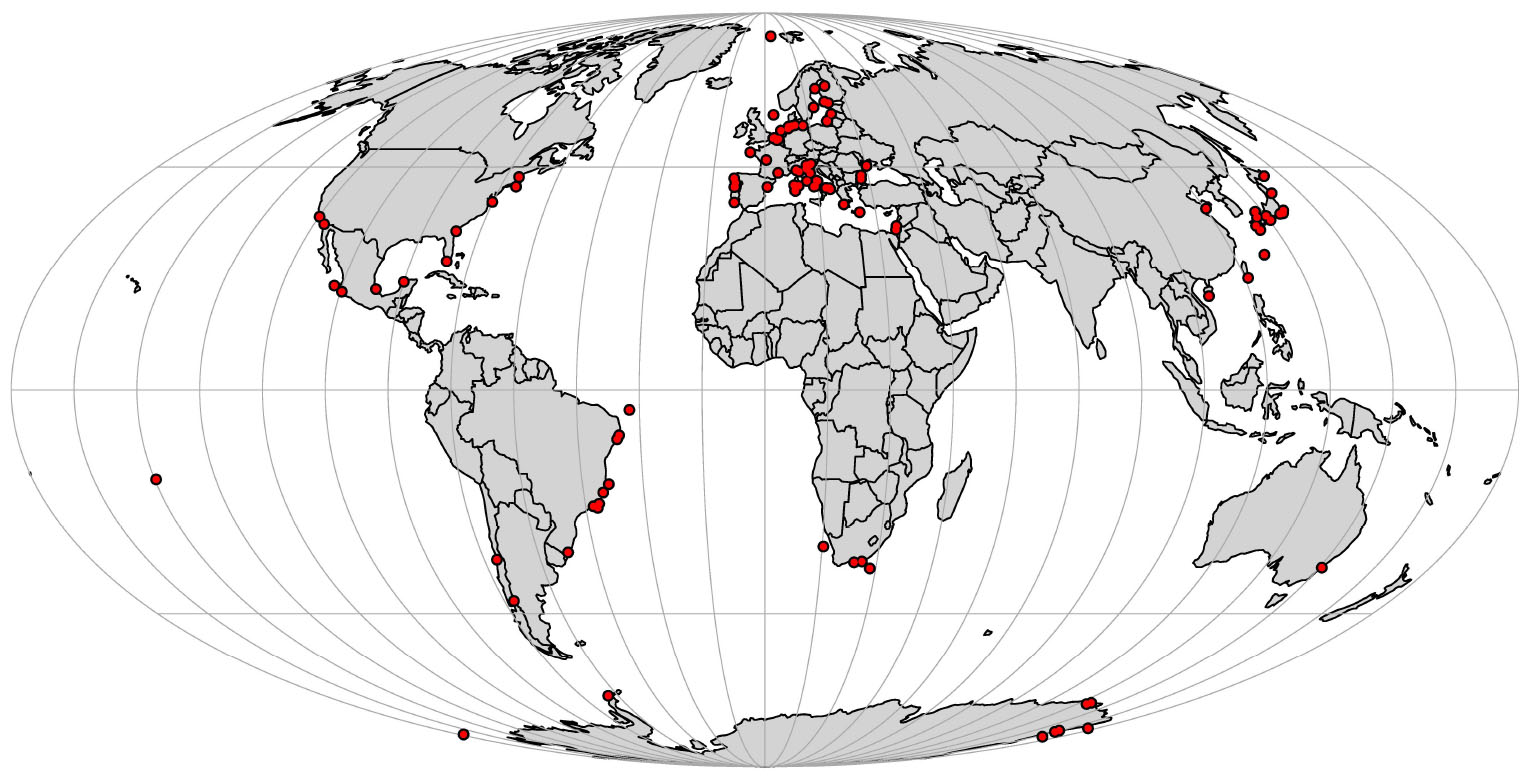
Figure 1. Worldwide distribution of coastal and marine International Long-Term Ecological Research (ILTER) sites. Based on DEIMS status as of 2nd September 2019.
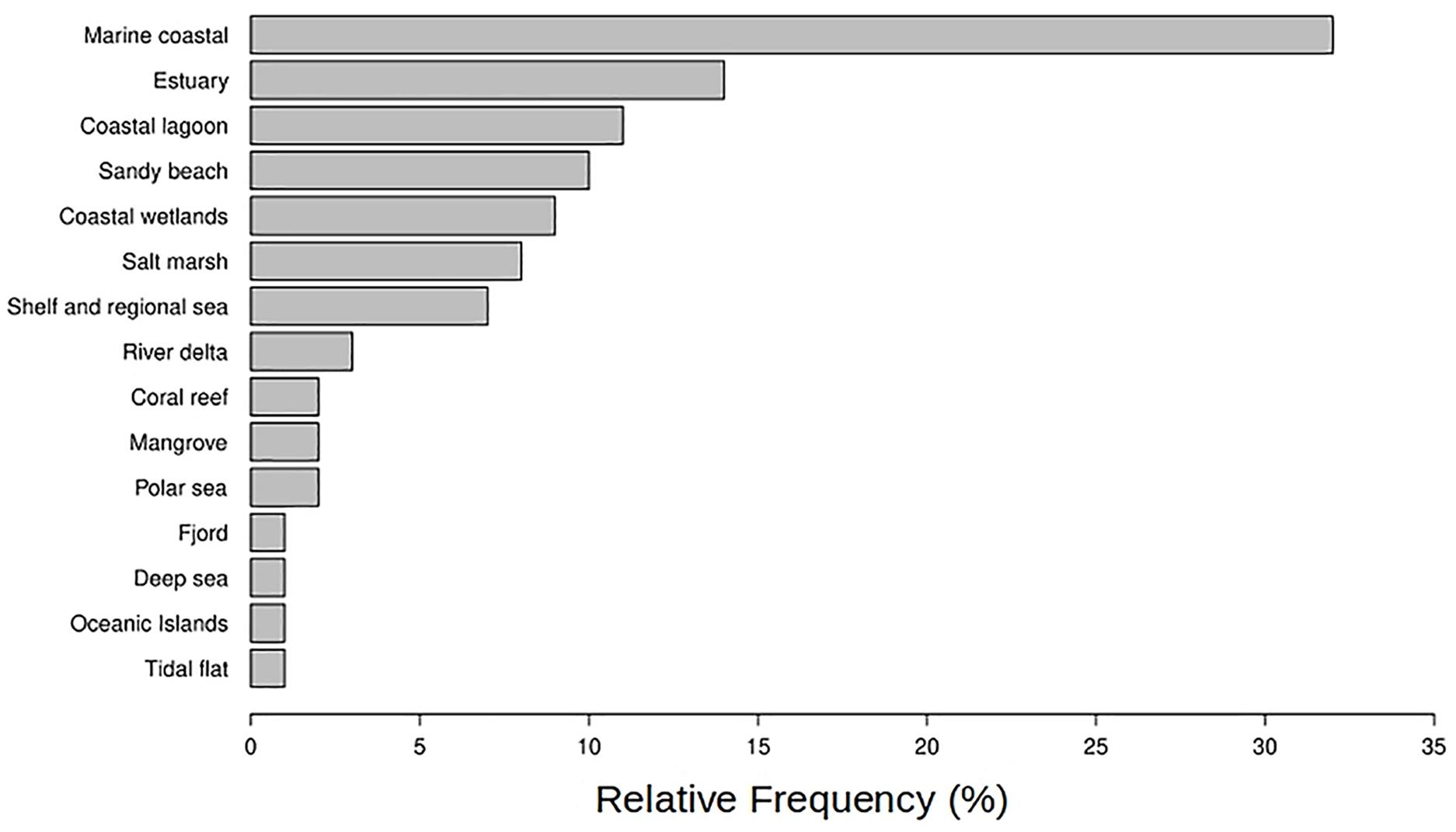
Figure 2. The diversity of habitats represented at coastal and marine International Long-Term Ecological Research sites.
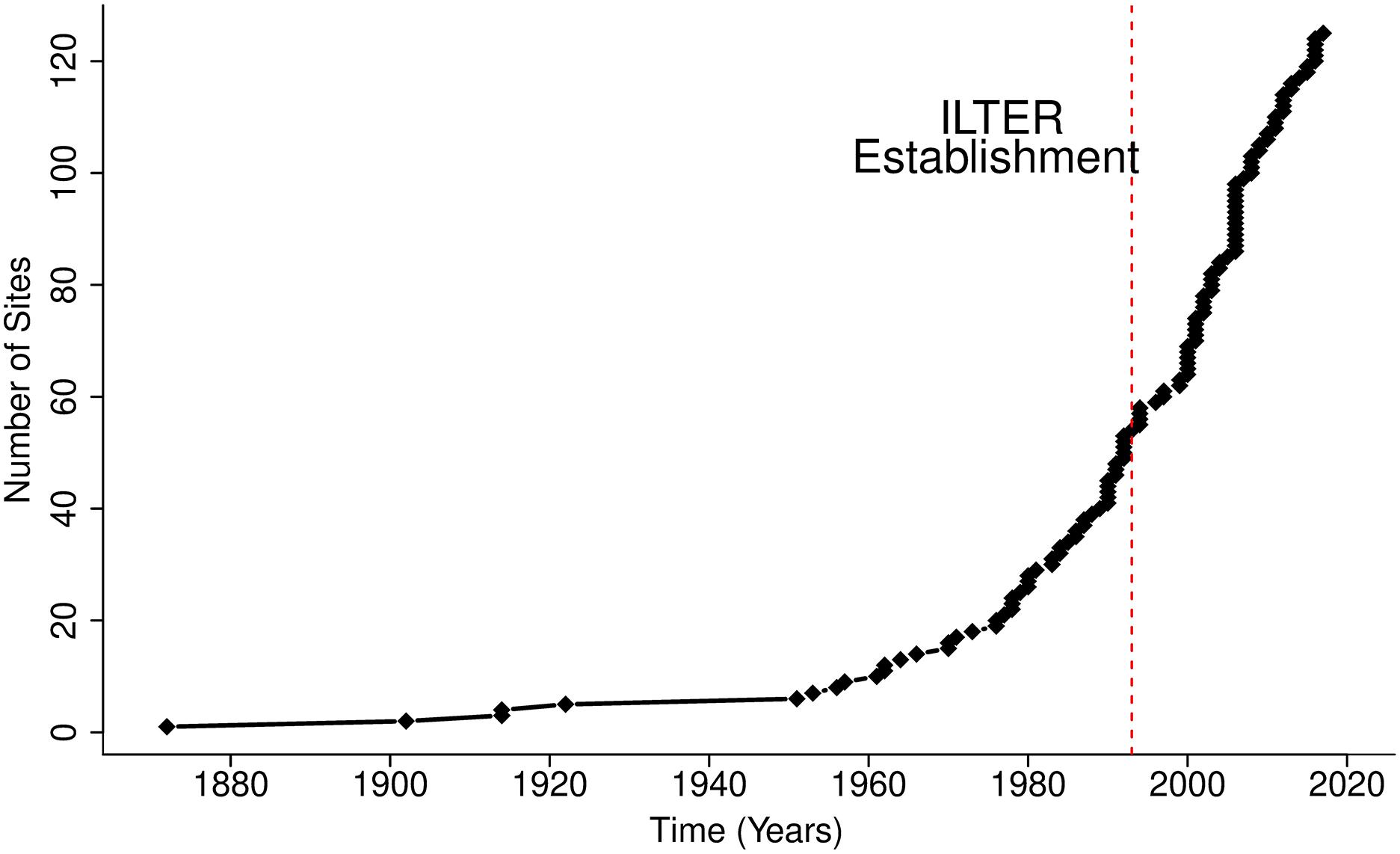
Figure 3. Timeline of the establishment of long-term coastal and marine observation sites and the implementation of the International Long-Term Ecological Research (ILTER) Program.
The ILTER-CMS are distributed from tropical-equatorial to polar regions in what can be considered a global observing system (Figure 1). There is a large concentration of sites in LTER-Europe, with broad distribution along most of the European Seas. The European LTER sites are predominantly characterized by coastal and transitional waters, such as lagoons, river deltas, estuaries, and fjords. In the Atlantic, sites are located predominantly along the United States, Caribbean, and Brazilian coasts. There is a lack of coverage for the equatorial Atlantic and the African coast, where site distribution is restricted to South Africa. The Indian Ocean has only one oceanic site located at Reunion Island. There are some sites in the South and East China Sea and a good concentration of sites around Japan. In the South Pacific, a coastal site is located in Australia and one oceanic site in Tahiti. In the East Pacific, there are no sites along South America, but only along the Mexican and North American coasts.
The main focus of the ILTER-CMS is on the primary role of ecosystem structure, function, and services in response to a wide range of environmental forcing factors, using long-term, site-based research. Consistent with the general ILTER mission, the coastal and marine sites have been established to contribute to a global, multi-disciplinary community of ecosystem observation and research capable of delivering socially relevant information on sustainable use of natural resources. To that end, ILTER-CMS represents a strong component in a global ocean observation system linking ILTER more strongly into the GOOS framework (Figure 4). ILTER-CMS and GOOS can mutually benefit from setting similar requirements and deciding on what to measure, monitor Essential Ocean Variables, and interact through the instrumentation deployment and maintenance. ILTER-CMS monitors many biological variables by classical methods, providing added value to the sensor-based measurements in the GOOS program. The LTSER with social science competence can contribute to define issues and priorities and assess the impact of observations on society (Figure 4). In the following paragraphs we will briefly analyze the potential for ILTER-CMS to contribute to global coastal and marine observations by means of a “Strengths, Weaknesses, Opportunities and Threats” (SWOT) approach (Table 1).
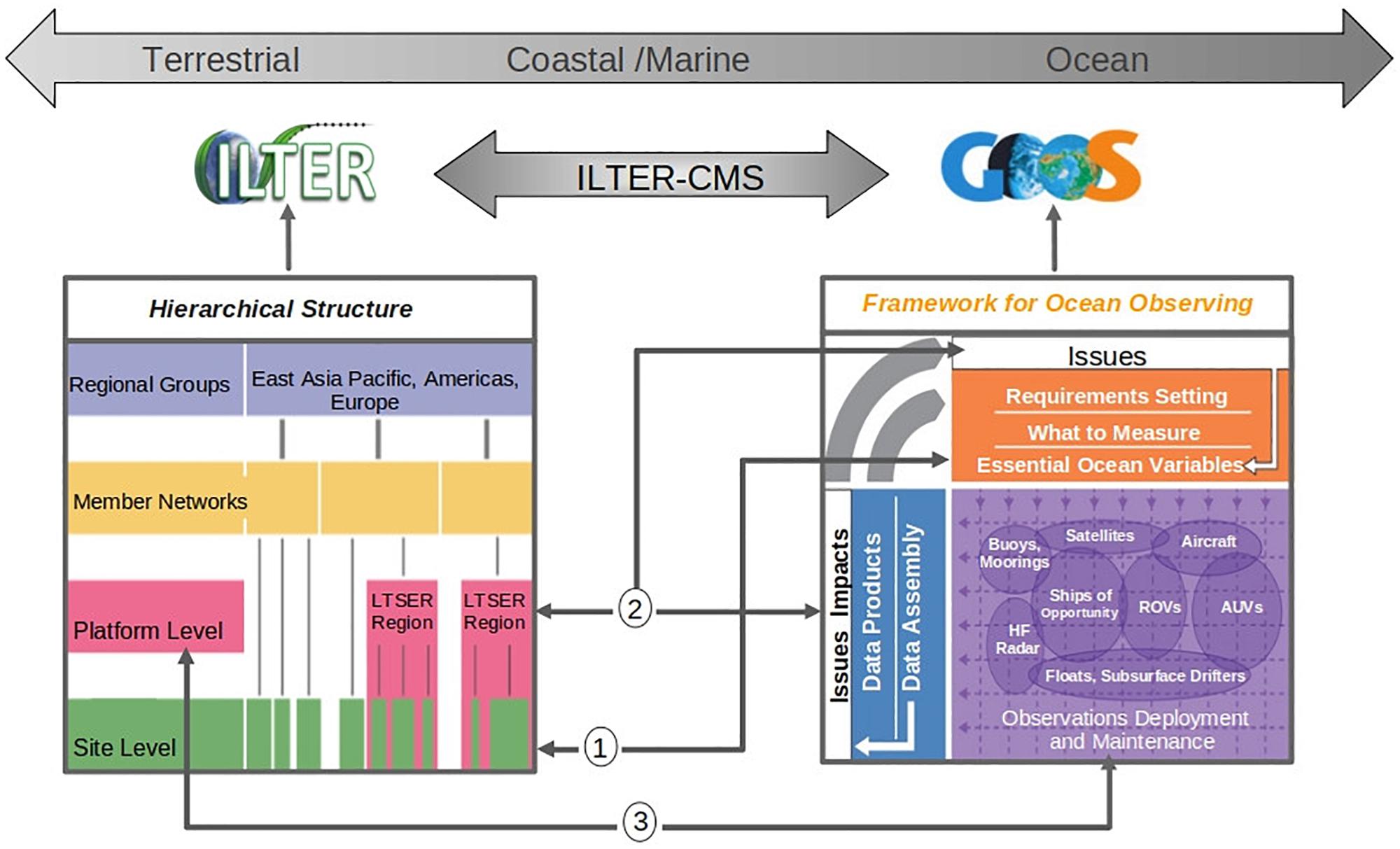
Figure 4. ILTER coastal and marine sites can provide several interaction points between the ILTER structure and the GOOS Framework. ILTER-CMS and GOOS can mutually benefit from setting similar requirements and deciding on what to measure. (1) ILTER sites can provide GOOS with monitoring of Essential Ocean Variables, including many biological variables by classical methods, providing added value to the sensor–based measurements in the GOOS program. (2) The LTSER with social science competence can contribute to define issues and priorities and assess the impact of observations on society. (3) At the platform level the interaction can take place through instrumentation deployment and maintenance.
Strengths
• ILTER coastal and marine sites are uniquely poised to contribute to biological and ecosystem EOVs by virtue of the existing ILTER governance, infrastructure, and research resources (both human and technological).
• The long time span of many ILTER-CMS members enables the identification of global trends (e.g., warming) and local pressures (e.g., nutrient loads) against the background of natural variation. Recent evidence of global changes and impacts from marine organisms and ecosystems are mainly derived from global databases of in situ observations (Poloczanska et al., 2016), stressing the value of global networks such as ILTER-CMS.
• A marked diversity and wide range of partners and institutions across the globe characterize ILTER-CMS, guaranteeing multi-disciplinary data acquisition, analysis, integration and synthesis, and cost-effective sustainable observations.
• The ILTER Network provides a platform for discussion among experts and stakeholders on key oceanographic and ecological themes, optimizing active links or developing new ones with a diverse array of global and regional processes and initiatives. Indeed, since its onset, ILTER had adopted a more interdisciplinary approach to research and monitoring, recognizing that in dealing with environmental problems, natural and human systems cannot be considered as separate entities (Dick et al., 2018). ILTER-CMS provides and fosters the use of standard protocols and open access data. This is critical for the study of climate change and its effect on biota and ecosystems. Additionally, ILTER-CMS already monitors Physical, Biochemical, and Biological/Ecological EOVs.
Weaknesses
• Most LTER sites and national networks have been developed from the bottom–up. The different research and monitoring aims, some of which may have changed over time, as well as the wide variety of ecosystem types, infrastructure, instrumentation and technological development may all hinder comparisons within and across networks, sites and scales.
• ILTER-CMS is a relatively new network, with 50% of the sites being established after the year 2000. Environmental and socio-ecological issues are often regional and not yet clearly defined at the global scale.
• Despite recent efforts (Haase et al., 2018) and progress with the development of the DEIMS-SDR, the harmonization of data and metadata for CMS is still far from complete. Standardized/transparent data management procedures cannot easily be implemented in a number of locations and datasets are often not readily available. The obstacles include inadequate funding, a lack of training opportunities, and/or hesitancy to submit data to internationally accepted repositories.
• ILTER-CMS was not designed to be an operational monitoring system but to study ecosystem and biodiversity. Consequently, there is not final consensus about the variables to be measured and the methodologies and sampling schemes that should be adopted.
Opportunities
• ILTER-CMS has the governance structure to coordinate with management and policy programs, interact and link with other large-scale initiatives, create interfaces between the different approaches from the various communities, and establish a co-located network of sites within similar ecosystem typologies, with shared research and monitoring tasks for multi-purpose uses (Haase et al., 2018). The ILTER-CMS can contribute substantially to merging frameworks that are behind different global research and monitoring initiatives, producing guidelines for future site-based long-term research and monitoring. The site network could generate connected ecosystem monitoring methodologies and datasets, supporting, as well, synergies in the use of costly infrastructures, through cross-initiative collaborative research.
• Stakeholder interactions play a key role in implementing ILTER outcomes for sustainable regional and local development in light of global trends, and for true integration of the sites into local/regional innovation systems, where the societal and human dimension is considered together with the scientific one. Long-term ecosystem studies, in comparison to the public funding they need, possess a disproportionately high capacity to inform policymakers about relevant environmental issues (Hughes et al., 2017). The ILTER-CMS represent ideal places for establishing pilot integrated biological observatories where the use of new technologies for ocean observation can also be promoted and where the information that technologies make available can be tested and compared to develop standardized approaches for their use (e.g., high throughput molecular or imaging techniques). With enhanced standardization and increased adoption across coastal and marine sites, the ILTER offers the opportunity to monitor EOVs at a global scale.
• The interaction between modelers and observers provides the opportunity to better design and plan new long-term observation initiatives. Several sites record periods of decades and thus a large number of different past conditions, which can be used to calibrate and validate various kinds of models. Model-generated environmental parameters can help to reduce temporal and spatial observation gaps. Advanced models are vital to create reliable (future) scenarios that facilitate the understanding of ecosystem functioning and evolution and are necessary to improve links between science and policy makers. Observations can be used to generate operational models needed to sustainably manage and protect marine and coastal ecosystems (ODS 14.2) by indicating areas of adverse impacts, habitat loss, and changes in ocean state that are relevant for ecosystems services. ILTER can strengthen capacity building by providing site access and training on advanced ecosystem monitoring and management. This is an invaluable opportunity to attract young scientists from around the globe and to teach a new generation of scientists innovative ways to use resources, recognize the relevance of these kinds of activities, and maintain and develop the network with a long-term perspective.
• ILTER-CMS could be an ideal context for the development of citizen science (CS) activities (Irwin, 1995; Bonney et al., 2009). CS can (i) improve the frequency and geographical coverage of observations, and (ii) improve pedagogy and communication at the science/society/policy interface by involving citizens, managers or different stakeholders in a research program. Hands-on involvement of people in research and monitoring activities is more effective than communicating about science and its benefits. Increasingly apps can be used to assist the citizen scientists to report data in a standardized manner (e.g., the Secchi disk project6 or beach observer). CS programs can yield significant results (see, e.g., Abbott et al., 2018) and could be applied, for example, in programs with divers, sailors, and beach-goers looking at biodiversity in coastal zones and collecting data of great interest to ILTER and contributing to GOOS.
• ILTER Network may provide improved data management at site-level and enhanced data flow toward global and European marine data infrastructure like the Ocean Biogeographic Information System (OBIS) and the European Marine Observation and Data Network (EMODnet). ILTER may make use as well of the standardization tools provided by World Register of Marine Species (WoRMS) and the improved analytical capacity provided by marine virtual research environments constructed under the European Research Infrastructure (ESFRI) by LifeWatch-ERIC and the European Open Science Cloud.
• The concepts of EOVs and Essential Biodiversity variables (EBVs) could facilitate the task of identifying key variables for LTER coastal and marine biological observations (Miloslavich et al., 2018; Navarro et al., 2018). ILTER-CMS needs to assess the readiness levels of the EOVs to assure implementation of an operational system. These concepts could be merged with the Ecosystem Integrity framework (Müller, 2005), at the moment adopted by ILTER, based on a comprehensive set of abiotic variables for identifying drivers of biodiversity changes within the context of ecosystem structures and processes (Haase et al., 2018).
Threats
• Long-term initiatives are difficult to maintain, and they need to be cost-effective and sustainable at the country level. Reduction of ILTER-CMS activities at some sites could produce temporal and spatial gaps that would hamper the functionality and relevance of the network.
• A failure in engaging users and stakeholders could limit the full exploitation of ILTER-CMS services and products. The lack of transfer of knowledge and understanding to policy makers and society would prevent informed decision making regarding the long-term safeguarding and effective management of marine ecosystem services.
• ILTER Network has historically been more oriented toward “terrestrial,” inland ecosystems: conceptual frameworks, harmonization, and data models not well-suited for the marine component may discourage future ocean initiatives.
• Future ILTER-CMS activities must be sustained by young scientists. A failure to make ILTER-CMS attractive and meaningful for early career researchers (and the next generation of stakeholders) could be a major threat for the long-term sustainability of integrated coastal and marine ecological observatories.
In the following two sections, we elaborate on two specific opportunities for ILTER-CMS in contributing to global ocean observing: Essential Ocean Variables and Emerging Technology.
Essential Ocean Variables and the ILTER Contribution to Observation, Science and Management Programs
Many programs and initiatives at the regional and global level are dedicated to the study of coastal and marine environments and they express a common need to harmonize and coordinate observations to allow comparison and synthesis across ecosystems and scales. In this respect, efforts need to be dedicated to enable interoperability and to create interfaces among the different initiatives. In particular, consolidated global networks, such as GOOS and ILTER, should be leaders in proposing and demonstrating how interoperability and linking of conceptual frameworks could be tackled.
We argue that one way to achieve this integration is through the use of Essential Ecosystem Variables (EEVs) or EOVs. One of the outputs of the OceanObs’09 conference was the adoption of FOO (Lindstrom et al., 2012). The FOO proposed the use of routine and sustained observations of physical, biogeochemical and biological EOVs. GOOS has adopted the FOO recommendations, and recently the Biology and Ecosystem Panel has approved a set of EOVs for global sustained observations of biodiversity and ecosystem change (Miloslavich et al., 2018). This is also in line with a recent joint proposition of ILTER and the Group on Earth Observations Biodiversity Observation Network (GEOBON) to merge ecosystem integrity and EBVs to serve as an improved guideline for future long-term environmental research and monitoring (Haase et al., 2018).
As a first step, we conducted a survey involving ILTER-CMS site managers in order to record how many sites already monitor the EOVs proposed by the GOOS. The GOOS Panels have identified 11 Physical, 9 Biogeochemical, and 11 Biological/Ecosystem essential variables. The results of the survey were quite encouraging (Figure 5). Eleven EOVs are observed routinely in more than 50% of the surveyed sites. Surface and sub-surface temperature and salinity are among the most observed physical EOVs, but a good number of sites also measure sea state (42%), surface (42%) and sub-surface currents (42%) and sea surface height (38%). The most common physical EOVs within ILTER-CMS sites (Figure 5) are already in a mature readiness level. For the biogeochemical EOVs, nutrients, oxygen and particulate matter are measured in more than 50% of the sites, while inorganic carbon (40%), dissolved organic carbon (40%) and ocean color (32%) are also well-represented. Nutrients and oxygen are at a mature level while particular matter can vary from concept to mature depending on the environment. Phytoplankton biomass and diversity, the abundance and distribution of benthic invertebrates, fish and zooplankton are the biological/ecosystem EOVs measured at 50% or more sites. These EOVs have a varying degree of readiness level, ranging from concept to mature. Other biological/ecosystem EOVs are site specific and reflect habitat specificity. Therefore, it is expected that seagrass, hard coral, and mangrove covers would only be measured at a few sites.
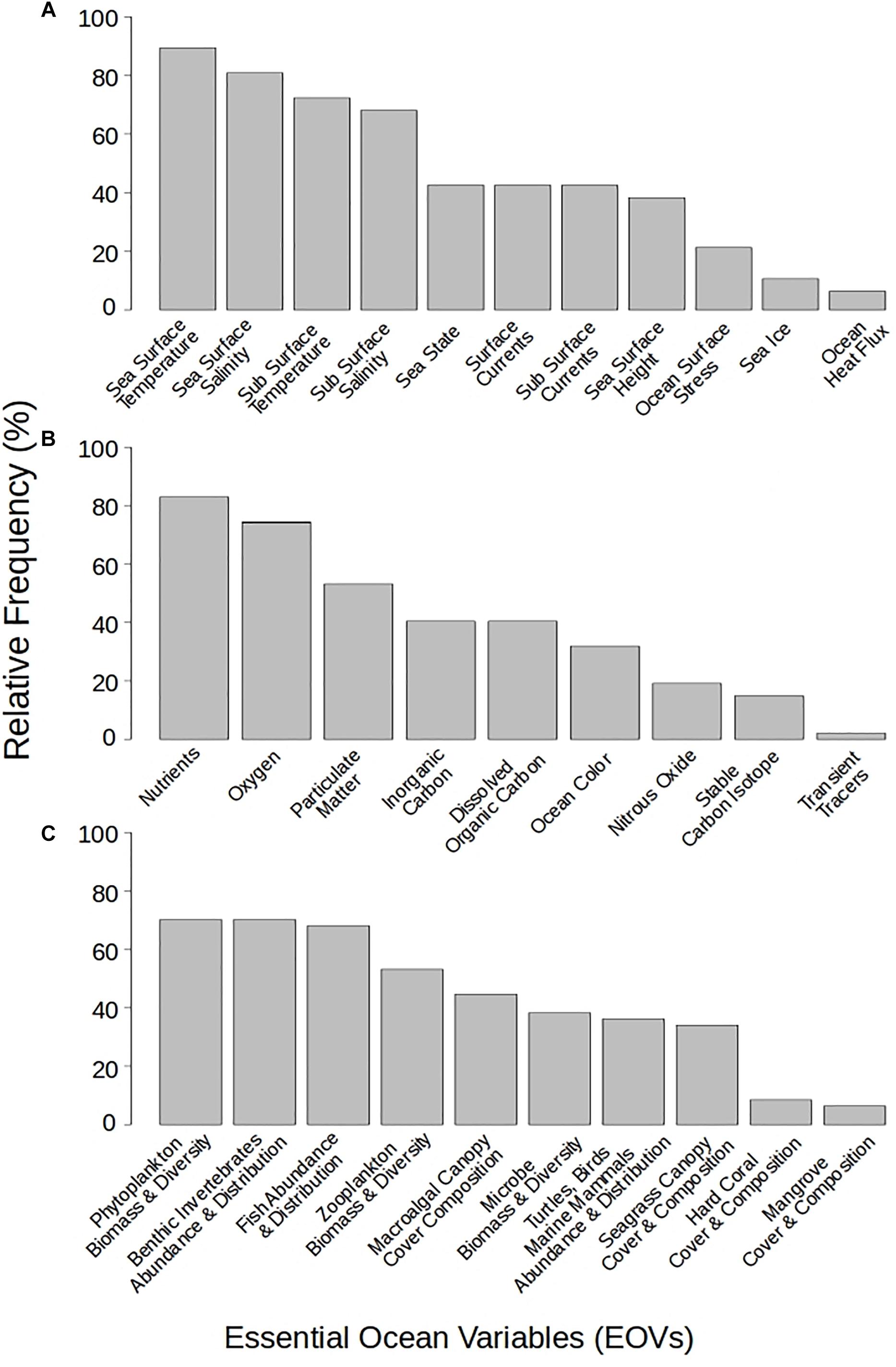
Figure 5. Frequency of coastal and marine International Long-Term Ecological Research (ILTER) sites that observe Essential Ocean Variables recommended by the GOOS Biology and Ecosystem Panel. Physical variables (A), biogeochemical variables (B), and biological/ecosystem variables (C).
ILTER coastal and marine sites also measure several other variables that are relevant for coastal and marine ecosystems, but are not indicated as EOVs. Among them: physical (e.g., incident wave height and cumulative wave energy, and currents along the entire water column parameters), water quality (e.g., transparency, turbidity, sediment concentration, and composition) and biogeochemical and biological parameters (redox potential and water pH, CO2 fluxes to and from the atmosphere, primary productivity, toxic phytoplankton composition, and production of toxins).
These results show that a comprehensive coastal and marine ecosystem monitoring system could benefit from collaboration and synergy with the ILTER network. At this time, when GOOS has just defined biological/ecological EOVs and is working on implementation strategies and coordination of observations, the ILTER network already provides infrastructure and logistical support to conduct monitoring of coastal and marine ecosystems around the world.
Another possible contribution of the ILTER-CMS observations is the establishment of integrated supersites. Supersites are focal points for instrument intensive research, ideally suited for co-location with small-scale experiments and specialized observations, and situated in areas that will provide important information on environmental change, i.e., critical zones. Co-locating ILTER-CMS supersites with GOOS-proposed Sentinel sites (PICO-I, 2012) would link the mechanistic ecological and biophysical understanding from the ILTER with an ocean observatory framework that explicitly seeks to understand the influence of anthropogenic pressures and the roles of ecosystem services in that location. ILTER has sites in most Sentinel Sites including some of the most stressed ones: Greenland-North-Baltic Seas/Bay of Biscay; Indonesian Archipelago-South China Sea; and, East China/Yellow Seas. Thus, the ILTER-CMS is poised to provide observations of scientific and societal benefit immediately to vulnerable regions.
Long-term ecological time series are crucial for setting realistic baselines and limits in the classification systems used for assessing ecosystem environmental status. The 115 globally-distributed coastal and marine sites of the ILTER provide an exceptional observation platform for the GOOS-defined EOVs and invaluable information for several regional and global programs. This integration could benefit the European Water Framework Directive (WFD) and the EU Marine Strategy Framework Directive (MSFD), the accomplishment of the Aichi Targets of the Convention for Biological Diversity (CBD), the Intergovernmental Panel for Climate Change (IPCC), the Intergovernmental Science-Policy Platform on Biodiversity and Ecosystem Services (IPBES) and the United Nations World Ocean Assessment. Information on coastal and marine ecosystems is urgently required to address the UN Sustainable Development Goal (SDG) 14.
ILTER coastal and marine sites can also provide information for science programs such as Future Earth Coasts, formerly LOICZ (Land-Ocean Interactions in the Coastal Zone) programme. Future Earth Coasts was launched by IGBP (International Geosphere-Biosphere Programme) and IHDP (International Human Dimensions Programme) as an international research project and global expert network exploring the pressures and social-environmental impacts of global environmental change in coastal zones. ILTER-CMS can also contribute to the Marine Biodiversity Observation Network (MBON), a thematic component of GEO BON, that aims to coordinate, promote and augment the capabilities of present and future national and international observing systems to characterize and monitor diversity of marine life at the genetic, species, and ecosystem levels using a broad array of in situ and remote sensing observations (Duffy et al., 2013; Muller-Karger et al., 2018). ILTER-CMS sites are located in 20 of the 66 Large Marine Ecosystem (LME, Sherman, 1991) around the world and can contribute to identify areas of the oceans for conservation purposes and enable ecosystem-based management to provide a collaborative approach to management of resources within ecologically-bounded transnational areas. Data collected at ILTER-CMS could be streamed into the OBIS and contribute to increasingly free and open access biodiversity and biogeographic data and information on marine life. ILTER can harbor and link with many other long-term coastal and ocean ecosystem monitoring programs, which may be explicitly reported also on DEIMS – SDR, in each site description. Furthermore, there is a well-established bottom–up procedure from the request of a site to become an LTER site, to the assessment of its suitability, i.e., of the possession of specific requisites fulfilling the LTER network requirements, which occurs at the national level, to the admittance to the national network and hence to ILTER.
New Technologies for ILTER
One aspect determining the future success of the ILTER will be its ability to adopt and leverage emerging technologies at all levels of organization. Many of these technological developments represent opportunities to catalyze education of and collaboration between young scientists across LTER sites, strengthening the network and thus mitigate identified threats in the SWOT-analysis (Table 1). Mature technologies that are economic to implement can directly contribute to counteract currently identified weaknesses by better geographical coverage and harmonized data quality. This spans innovative in situ technologies developed at individual sites to global data networks that enable novel syntheses via increasingly powerful computational technologies or leverage citizen science. Here we consider some of these technologies, building up through the hierarchical organization of ILTER (see Figure 4 in Mirtl et al., 2018).
Researcher Level
International Long-Term Ecological Research Network sites are ideal incubators for the development and/or deployment of emerging technologies. As noted in the introduction, the general sampling rate of eco/bio/geo/chemical phenomena has long lagged behind the rapid data collection possible by physical oceanographers. In the last two decades, however, there has been a rapid development of novel high-throughput technologies. These include chemical, imaging, acoustic and molecular sensor systems that have enabled continuous and long-term measurement of new parameters that are of great interest (Johnson et al., 2007). Examples include nitrate (Johnson and Coletti, 2002), ammonium (Plant et al., 2009), and pH (Seidel et al., 2008; Martz et al., 2010) sensors. Similarly, advances in rapid image collection and processing have enabled cataloging and enumeration of phytoplankton and zooplankton biodiversity at tidal to interannual scales (Olson and Sosik, 2007; Sosik and Olson, 2007; Faillettaz et al., 2016; Hunter-Cevera et al., 2016), while high-throughput molecular analyses facilitate biodiversity assessments of unprecedented taxonomic resolution, facilitating the regular monitoring of taxa that were not reliably identifiable previously (Stern et al., 2018). This, in turn, provides the potential for unique insights into the diversity and function of marine foodwebs (e.g., Leray et al., 2015).
One major trend that will shape the future of ILTER research is the continued miniaturization of electronics and improvements to memory and batteries. For example, the Imaging FlowCytobot is now half as large, weighs half as much, and consumes one-third of the power of the original (Olson et al., 2017). This technology has now been commercialized (McLane Research Laboratories, Inc.), is in routine use at the Northeast U.S. Shelf (NES) LTER site and other time series locations in the U.S., and has a growing user base around the world. Continued advances along this trajectory will enable novel biological and ecological studies. For example, embedded sensors within organisms have enabled metabolic and physiological studies (McGaw et al., 2018); underwater tracking technology has revealed ecological interactions between predator, prey and the environment (Osterback et al., 2013). The rich contextual data at the ILTER sites provide an ideal framework to develop this new technology and interpret the data it produces.
In addition to developments of in situ technologies, ILTER sites are also promoting advances in remote sensing technologies that are critical for characterizing ecosystems at large scales. For example, in coastal dune systems the use of high-resolution remote sensed imagery (LiDAR – Light Detection and Ranging – and orthophotos) is helping to explain the invasion success of some alien species and the roles of propagule pressure, abiotic, and biotic factors in coastal landscapes (Bazzichetto et al., 2018). The use of remotely sensed data may make it possible to model the invader-landscape relationship over a large geographic extent and to highlight the coastal sectors that are most likely to be invaded in the future.
Site Level
Across individual ILTER sites (which may comprise a number of individual locations), autonomous systems, robotics, machine learning, and advances in -omics technologies will drive discovery in the coming decades.
The maturation and miniaturization of robotic platforms (taken here to be all manner of autonomous mobile platforms, including drifters, profiling floats, buoyancy gliders, propeller-driven autonomous underwater vehicles, and unmanned aerial systems, cf. Nidzieko et al., 2018) will gradually enable their incorporation into a broader range of research. There are three barriers to more widespread adoption at the moment. One, the maturation of suitable sensors, as mentioned above, is necessary prior to incorporation onto mobile platforms. Two, most platforms are still not “turnkey” devices and thus require specialized operator knowledge. And three, costs are still prohibitive for acquisition of more mature technologies. The popular trend of the DIY/makerspace ethos may result in less costly, yet still capable platforms that can be readily employed in coastal and marine research. Once deployed, however, the ability of robots to do “dull, dirty, dangerous” tasks will improve both the temporal and spatial measurements collected across an LTER site. For example, the time-consuming task of manually counting kelp forest biomass is only conducted monthly across all 10 of the individual sites within the Santa Barbara Coastal LTER and measurements are done on subsampled transects within a kelp forest. Underwater and/or aerial platforms could be used to survey the entire 70 km coastline on a weekly basis, enabling better understanding spatial patterns in synchrony and disturbance (Bell et al., 2015).
Machine learning will enable the deployment of technologies that have heretofore been too data-rich to make significant impacts to understanding biology and ecology within LTER sites. We give two examples: passive acoustics and flow cytometers. The use of passive acoustics across a broad sound spectrum has not been feasible due to limitations in memory, battery power, and processing capabilities. With the advances mentioned above and the maturation of machine learning techniques in parallel with increasingly powerful computers (at both the personal and cloud/cluster level), tackling the processing requirements of such large datasets in now feasible (Mooney et al., 2017). We envision that monitoring the soundscape within ILTER-CMS will become one of the most widely adopted technologies in the coming decade. Flow cytometry techniques similarly produce massive amounts of data and have traditionally required extensive manual efforts to classify cytometric and image data into quantified products; the potential for automation of these tasks across ILTER sites could revolutionize researcher’s understanding of variations in plankton biodiversity.
Finally, at the site level, emerging molecular methods will bring major insights by identifying important microbial actors, determining their interactions, and providing deeper insights into biogeochemical processes at ILTER sites. One example of this technology is the MBARI Environmental Sample Processor (Scholin et al., 2009), which has been used to autonomously collect and preserve microbial samples (Ottesen et al., 2011), revealing complex shifts in microbial communities over very short time scales (Needham et al., 2018). See McQuillan and Robidart (2017) for a comprehensive review of recent advances. Importantly, these technological advances are multiplicative: not only are the measurement techniques novel and advanced, but these measurements can also be conducted adaptively in response to the feature or event of interest (Harvey et al., 2012). Furthermore, these technologies can present advantages in accuracy, efficiency, and cost (Danovaro et al., 2016). It is important though that all new observing technologies pass through the four stages of evolution of a sustained Ocean Observing system (Nowlin et al., 2001).
Regional/Global Levels
While many of the roles of technology at the researcher and site levels will provide fundamental disciplinary discoveries and enable the establishment of new time series for incorporation into ILTER core products, several aspects of technology will drive the interdisciplinary discoveries that integrate multiple sites at regional and/or global levels. First, common metadata and easy, rapid access to data assets are essential to synthesis efforts. Improvements to cloud storage and data discovery tools will be critical to this end (Buck et al., 2019). As these tools mature, and perhaps converge from bespoke applications into more generic platforms, ILTER data will become an integral component of the broader suite of data products provided from regional observing systems, operational (and data assimilative) forecasts, numerical hindcasts, and weather data that researchers draw upon.
The major challenge to delivering the sound ecological knowledge necessary to address human impacts will require incorporating patterns/trends from site-level records into observed and predicted global climate models. This is not a trivial task, because it requires both scaling up local measurements to discover and explain emergent patterns (that might only be detectable within the distributed network of the ILTER) while also scaling down from climate predictions to expectations/hypotheses of what might be observed at individual site. Synthesis using cross ILTER site have contributed to the knowledge of ecosystem spatial and temporal variability (e.g., Bestelmeyer et al., 2011), and serve as example that this challenge can be addressed. Advances in computational capabilities will certainly be an asset to this end, but ultimately the larger hurdle may be not be technological be rather operational, as described in the threats above.
Recommendations and Challenges
ILTER coastal and marine sites has great potential to contribute to global coastal and ocean observation. Here we provide recommendations to improve the opportunities for ILTER-CMS to enhance collaboration among researchers, institutions and governments and funding agencies in support of long-term ocean observing initiatives.
(1) Recommendations to the network members and related organizations:
• Regional nodes should promote the expansion of coastal and marine sites by strengthening networks of marine scientists.
• Financial and educational support necessary to minimize the coverage gaps of ILTER-CMS (Figure 1), especially in developing countries should be addressed.
• Members should provide a quality-assured web-based data archiving, effective data retrieval and relevant data products within the network; promote the use of best-available statistical tools for data analysis and synthesis; and build up their respective infrastructures for sharing data and data handling, analysis and visualization.
• The ILTER-CMS need to harmonize and coordinate long-term environmental (both biotic and abiotic) observations in line with the EOVs to the extent possible, to allow comparisons within and across networks, sites and scales. These efforts should consider socio-ecological aspects, as well.
• Launch or improve coordination and integration of observations across scales (e.g., from coast to open sea) in concert with other international observing networks.
• Develop standard operating procedures, adopt guidelines for measurement program design, and establish routines for recurrent intercalibration exercises for all subject fields in line with the OceanBestPractices (OBP7). Such activities may include increasing the use of shared infrastructure, protocols and data platforms; and developing metadata and dataset harmonization/interoperability necessary to foster and facilitate sharing and open access.
• Define a consistent overarching research and monitoring framework, taking into account the wide range of ILTER-CMS typologies. LTER has historically been more oriented toward terrestrial, inland ecosystems, therefore coastal and marine conceptual frameworks, harmonization and data models still need improvement and should be strengthened and better integrated.
• Strengthen the communication with relevant stakeholders to improve the role of ILTER-CMS network as a data and knowledge provider for society.
• Promote citizen science initiatives to raise awareness about the broad importance of ILTER-CMS research activities. A collaboration with existing citizen science networks, e.g., ECSA (European Citizen Science Association) and other observation networks should be encouraged.
• Seek consensus for the adoption of parameters and monitoring methods in order to overcome fragmentation between sites, improve interfaces among networks (e.g., GOOS) and promote cooperation on shared environmental issues and targets.
• Further develop existing biological observations through the implementation of new technological and -omic approaches and improve their integration with physical and chemical observing systems and modeling initiatives.
• Provide the knowledge needed by policy makers and society for informed decision-making regarding the long-term safeguarding and effective management and sustainability of coastal and marine ecosystem services.
(2) General recommendations to government, funding agencies, and other organizations:
• Partners should address the need to support and protect existing LTER sites, recruit technical workforce, allow for their development and improvement, and increase their number for better spatial coverage, while recognizing funding limitations.
• Support formation of expert working groups providing urgent scientific environmental knowledge for society, capacity strengthening, education, and training.
• Support technological innovation to implement in situ observing systems, develop smart technologies for cost-effective automated monitoring of biological variables, and transition from research to operational status.
• Engage society in the definition of relevant research questions to strengthen the science/society/policy interface.
• Engage governments in prioritizing and sustaining ILTER-CMS activities as well as engage users, stakeholders, and other existing observing networks to fully exploit ILTER services and products, and demonstrate the impact on science and society.
Author Contributions
JM, NN, AA, AB, AK, AP, and AZ helped to conceive the manuscript, coordinated the author contributions, wrote and edited the manuscript, and contributed to tables and figures. SB, EB, TB, BC, KD, EE, MN, OR, MS, TS, HS, AdS, KS, PS, AnS, and JW contributed to manuscript ideas and text.
Funding
JM was supported by a CNPq fellowship (Grant No. 310047/2016-1) and by PELD Estuário da Lagoa dos Patos e Costa Adjacente (CNPq/CAPES/FAPERGS). SB was supported by US NSF (Grant #OCE-1655686). AB was supported by CAPES/CNPq/FAPES grant no. 441243/2016-9 to PELD Coastal Habitats of Espírito Santo as part of the Brazilian LTER program. HS was supported by US NSF (Grant #CCF-1539256 and #OCE-1655686), Simons Foundation (Grant #561126) and US NOAA/CINAR (Cooperative Agreement NA14OAR4320158).
Conflict of Interest Statement
The authors declare that the research was conducted in the absence of any commercial or financial relationships that could be construed as a potential conflict of interest.
Acknowledgments
The authors acknowledge the contribution of all ILTER-CMS that have provided updated information to the Dynamic Ecological Information Management System Site and Dataset Registry (DEIMS-SDR) and that responded the EOV questionnaire.
Supplementary Material
The Supplementary Material for this article can be found online at: https://www.frontiersin.org/articles/10.3389/fmars.2019.00527/full#supplementary-material
Footnotes
- ^ http://goosocean.org/
- ^ http://www.goosocean.org/eov
- ^ https://www.ilter.network/
- ^ http://data.lter-europe.net/deims/
- ^ http://www.geoportal.org/
- ^ http://www.secchidisk.org/
- ^ https://www.oceanbestpractices.net/
References
Abbott, B. W., Moatar, F., Gauthier, O., Fovet, O., Antoine, V., and Ragueneau, O. (2018). Trends and seasonality of river nutrients in agricultural catchments: 18 years of weekly citizen science in France. Sci. Total Environ. 624, 845–858. doi: 10.1016/j.scitotenv.2017.12.176
Bazzichetto, M., Malavasi, M., Barták, V., Acosta, A. T. R., Moudrý, V., and Carranza, M. L. (2018). Modeling plant invasion on Mediterranean coastal landscapes: an integrative approach using remotely sensed data. Landsc. Urban Plan. 171, 98–106. doi: 10.1016/j.landurbplan.2017.11.006
Bell, T. W., Cavanaugh, K. C., Reed, D. C., and Siegel, D. A. (2015). Geographical variability in the controls of giant kelp biomass dynamics. J. Biogeogr. 42, 2010–2021. doi: 10.1111/jbi.12550
Bestelmeyer, B. T., Ellison, A. M., Fraser, W. R., Gorman, K. B., Holbrook, S. J., Laney, C. M., et al. (2011). Analysis of abrupt transitions in ecological systems. Ecosphere 2:129. doi: 10.1890/ES11-00216.1
Bonney, R., Ballard, H., Jordan, R., Mc Callie, E., Phillips, T., Shirk, J., et al. (2009). CAISE Report on Public Participation in Scientific Research. A CAISE Inquiry Group Report. Washington, DC: Center for Advancement of Informal Science Education.
Buck, J. J. H., Bainbridge, S. J., Burger, E. F., Kraberg, A. C., Casari, M., Casey, K. S., et al. (2019). Ocean data product integration through innovation-the next level of data interoperability. Front. Mar. Sci. 6:32. doi: 10.3389/fmars.2019.00032
Danovaro, R., Carugati, L., Berzano, M., Cahill, A., Carvalho, S., Chenuil, A., et al. (2016). Implementing and innovating marine monitoring approaches for assessing marine environmental status. Front. Mar. Sci. 3:213. doi: 10.3389/fmars.2016.00213
Defeo, O., McLachlan, A., Schoeman, D. S., Schlacher, T. A., Dugan, J., Jones, A., et al. (2009). Threats to sandy beach ecosystems: a review. Estuar. Coast. Shelf Sci. 81, 1–12. doi: 10.1016/j.ecss.2008.09.022
Dick, J., Orenstein, D. E., Holzer, J. M., Wohner, C., Achard, A.-L., Andrews, C., et al. (2018). What is socio-ecological research delivering? A literature survey across 25 international LTSER platforms. Sci. Total Environ. 62, 1225–1240. doi: 10.1016/j.scitotenv.2017.11.324
Drius, M., Carranza, M. L., Stanisci, A., and Jones, L. (2016). The role of Italian coastal dunes as carbon sinks and diversity sources. A multi-service perspective. Appl. Geogr. 75, 127–136. doi: 10.1016/j.apgeog.2016.08.007
Duffy, J. E., Amaral-Zettler, L. A., Fautin, D. G., Paulay, G., Rynearson, T. A., Sosik, H. M., et al. (2013). Envisioning a national marine biodiversity observation network. Bioscience 63, 350–361. doi: 10.1525/bio.2013.63.5.8
European Commission (2016). H2020 Programme - Guidelines on FAIR Data Management in Horizon 2020. Available at: http://ec.europa.eu/research/participants/data/ref/h2020/grants_manual/hi/oa_pilot/h2020-hi-oa-data-mgt_en.pdf (accessed August 13, 2019).
Faillettaz, R., Picheral, M., Luo, J. Y., Guigand, C., Cowen, R. K., and Irisson, J.-O. (2016). Imperfect automatic image classification successfully describes plankton distribution patterns. Methods Oceanogr. 15, 60–77. doi: 10.1016/j.mio.2016.04.003
Haase, P., Tonkin, J. D., Stoll, S., Burkhard, B., Frenzel, M., Geijzendorffer, I. R., et al. (2018). The next generation of site-based long-term ecological monitoring: linking essential biodiversity variables and ecosystem integrity. Sci. Total Environ. 613–614, 1376–1384. doi: 10.1016/j.scitotenv.2017.08.111
Halpern, B. S., Frazier, M., Potapenko, J., Casey, K. S., Koenig, K., Longo, C., et al. (2012). Spatial and temporal changes in cumulative human impacts on the world’s ocean. Nat. Comm. 6:7615. doi: 10.1038/nccomms8615
Harvey, J. B. J., Ryan, J. P., Marin, R., Preston, C. M., Alvarado, N., Scholin, C. A., et al. (2012). Robotic sampling, in situ monitoring and molecular detection of marine zooplankton. J. Exp. Mar. Biol. Ecol. 413, 60–70. doi: 10.1016/j.jembe.2011.11.022
Howes, E. L., Joos, F., Eakin, C. M., and Gattuso, J.-P. (2015). An updated synthesis of the observed and projected impacts of climate change on the chemical, physical and biological processes in the oceans. Front. Mar. Sci. 2:36. doi: 10.3389/fmars.2015.00036
Hughes, B. R., Beas-Luna, R., Barner, A. K., Brewitt, K., Brumbaugh, D. R., Cerny-Chipman, E. B., et al. (2017). Long-Term studies contribute disproportionately to ecology and policy. Bioscience 67, 271–281. doi: 10.1093/biosci/biw185
Hunter-Cevera, K. R., Neubert, M. G., Olson, R. J., Solow, A. R., Shalapyonok, A., and Sosik, H. M. (2016). Physiological and ecological drivers of early spring blooms of a coastal phytoplankter. Science 354, 326–329. doi: 10.1126/science.aaf8536
ILTER (2006). ILTER Strategic Plan: International long-term ecological research network - Strategic Plan. Available at: http://www.lter-europe.net/document-archive/central/ECOLEC-D-08-00262.pdf (accessed August 13, 2019).
Irwin, A. (1995). Citizen Science: A Study of People, Expertise and Sustainable Development. New York, NY: Routledge.
Johnson, K. S., and Coletti, L. J. (2002). In situ ultraviolet spectrophotometry for high resolution and long-term monitoring of nitrate, bromide and bisulfide in the ocean. Deep Sea Res. I 49, 1291–1305. doi: 10.1016/s0967-0637(02)00020-1
Johnson, K. S., Needoba, J. A., Riser, S. C., and Showers, W. J. (2007). Chemical sensor networks for the aquatic environment. Chem. Rev. 107, 623–640. doi: 10.1021/cr050354e
Kliment, T., and Oggioni, A. (2011). Metadatabase: EnvEurope Metadata specification for Dataset Level. EnvEurope (LIFE08 ENV/IT/000339) Project Report PD.A1.1.4. Available at: http://www.enveurope.eu/misc/PD_1_1_4_Kliment_Metadatabase_201112_final_v1.0.pdf (accessed August 13, 2019).
Leray, M., Meyer, C. P., and Mills, S. C. (2015). Metabarcoding dietary analysis of coral dwelling predatory fish demonstrates the minor contribution of coral mutualists to their highly partitioned, generalist diet. PeerJ 3:e1047. doi: 10.7717/peerj.1047
Lindstrom, E., Gunn, J., Fischer, A., McCurdy, A., Glover, L., Alverson, K., et al. (2012). A Framework for Ocean Observing. By the Task Team for an Integrated Framework for Sustained Ocean Observing, UNESCO 2012, IOC/INF-1284. Available at: http://www.oceanobs09.net/foo/FOO_Report.pdf (accessed August 13, 2019).
Malavasi, M., Acosta, A. T. R., Carranza, M. L., Bartolozzi, L., Basset, A., Bassignana, M., et al. (2018). Plant invasions in Italy: an integrative approach using the european lifewatch infrastructure database. Ecol. Indic. 91, 182–188. doi: 10.1016/j.ecolind.2018.03.038
Martz, T. R., Connery, J. G., and Johnson, K. S. (2010). Testing the honeywell durafet® for seawater ph applications. Limnol. Oceanogr. Methods 8, 172–184. doi: 10.4319/lom.2010.8.172
Mauz, I., Peltola, T., Granjou, C., van Bommel, S., and Buijs, A. (2012). How scientific visions matter: insights from three long-term socio-ecological research (LTSER) platforms under construction in Europe. Environ. Sci. Pol. 19, 90–99. doi: 10.1016/j.envsci.2012.02.005
McGaw, I. J., Steell, S. C., Van Leeuwen, T. E., Eliason, E. J., and Cooke, S. J. (2018). Application of miniature heart rate data loggers for use in large free-moving decapod crustaceans: method development and validation. Physiol. Biochem. Zool. 91, 731–739. doi: 10.1086/695839
McQuillan, J. S., and Robidart, J. C. (2017). Molecular-biological sensing in aquatic environments: recent developments and emerging capabilities. Curr. Opin. Biotechnol. 45, 43–50. doi: 10.1016/j.copbio.2016.11.022
Miloslavich, P., Bax, N. J., Simmons, S. E., Klein, E., Appeltans, W., Aburto-Oropeza, O., et al. (2018). Essential ocean variables for global sustained observations of biodiversity and ecosystem changes. Glob. Change Biol. 24, 2416–2433. doi: 10.1111/gcb.14108
Mirtl, M., Borer, E. T., Djukic, I., Forsius, M., Haubold, H., Hugo, W., et al. (2018). Genesis, goals and achievements of long-term ecological research at the global scale: a critical review of ILTER and future directions. Sci. Total Environ. 626, 1439–1462. doi: 10.1016/j.scitotenv.2017.12.001
Mooney, T. A., Lillis, A., Kaplan, M. B., Suca, J., and Lammers, M. (2017). Variability in coral reef soundscapes, spatiotemporal differences, biophysical and behavioral drivers, and associations with local biota. J. Acoust. Soc. Am. 141, 3939–3939. doi: 10.1121/1.4988920
Muller-Karger, F. E., Miloslavich, P., Bax, N. J., Simmons, S., Costello, M. J., Sousa Pinto, I., et al. (2018). Advancing marine biological observations and data requirements of the complementary essential ocean variables (EOVs) and essential biodiversity variables (EBVs) frameworks. Front. Mar. Sci. 5:211. doi: 10.3389/fmars.2018.00211
Müller, F. (2005). Indicating ecosystem and landscape organisation. Ecol. Indic. 5, 280–294. doi: 10.1016/j.ecolind.2005.03.017
Navarro, L., Fernández, N., Guerra, C., Guralnick, R., Kissling, W. D., Londoño, M. C., et al. (2018). Monitoring biodiversity change through effective global coordination. Curr. Opin. Environ. Sust. 29, 158–169. doi: 10.1016/j.cosust.2018.02.005
Needham, D. M., Fichot, E. B., Wang, E., Berdjeb, L., Cram, J. A., Fichot, C. G., et al. (2018). Dynamics and interactions of highly resolved marine plankton via automated high-frequency sampling. ISME J. 12, 2417–2432. doi: 10.1038/s41396-018-0169-y
Nidzieko, N. J., Todd, R. E., and Edwards, C. R. (2018). “ALPS in coastal oceanography,” in ALPS II - Autonomous and Lagrangian Platforms and Sensors. A Report of the ALPS II Workshop, eds D. Rudnick, D. Costa, K. Johnson, C. Lee, and M.-L. Timmermans (La Jolla, CA: National Oceanographic Partnership Program).
Nowlin, W. D. Jr., Briscoe, M., Smith, N., McPhaden, M., Roemmich, D., Chapman, P., et al. (2001). Evolution of a sustained ocean observing system. Bull. Am. Met. Soc. 82, 1369–1376.
Olson, R. J., Shalapyonok, A., Kalb, D. J., Graves, S. W., and Sosik, H. M. (2017). Imaging FlowCytobot modified for high throughput by in-line acoustic focusing of sample particles. Limnol. Oceanogr. Methods 15, 867–874. doi: 10.1002/lom3.10205
Olson, R. J., and Sosik, H. M. (2007). A submersible imaging-in-flow instrument to analyze nano-and microplankton: imaging flowcytobot. Limnol. Oceanogr. Methods 5, 195–203. doi: 10.4319/lom.2007.5.195
Osterback, A.-M. K., Frechette, D. M., Shelton, A. O., Hayes, S. A., Bond, M. H., Shaffer, S. A., et al. (2013). High predation on small populations: avian predation on imperiled salmonids. Ecosphere 4:116. doi: 10.1890/ES13-00100.1
Ottesen, E. A., Marin, R., Preston, C. M., Young, C. R., Ryan, J. P., Scholin, C. A., et al. (2011). Metatranscriptomic analysis of autonomously collected and preserved marine bacterioplankton. ISME J. 5, 1881–1895. doi: 10.1038/ismej.2011.70
PICO-I (2012). Requirements for Global Implementation of the Strategic Plan for Coastal GOOS. Available at: http://goosocean.org/index.php?option=com_oe&task=viewDocumentRecord&docID=7702 (accessed August 13, 2019).
Plant, J., Johnson, K., Needoba, J., and Coletti, L. (2009). NH4-Digiscan: an in situ and laboratory ammonium analyzer for estuarine, coastal and shelf waters. Limnol. Oceanogr. Methods 7, 144–156. doi: 10.4319/lom.2009.7.144
Poloczanska, E. S., Burrows, M. T., Brown, C. J., García Molinos, J., Halpern, B. S., Hoegh-Guldberg, O., et al. (2016). Responses of marine organisms to climate change across oceans. Front. Mar. Sci. 3:62. doi: 10.3389/fmars.2016.00062
Scholin, C., Doucette, G., Jensen, S., Roman, B., Pargett, D., and Marin, R. III, et al. (2009). Remote detection of marine microbes, small invertebrates, harmful algae, and biotoxins using the environmental sample processor (ESP). Oceanography 22, 158–167. doi: 10.5670/oceanog.2009.46
Seidel, M. P., DeGrandpre, M. D., and Dickson, A. G. (2008). A sensor for in situ indicator-based measurements of seawater pH. Mar. Chem. 109, 18–28. doi: 10.1016/j.marchem.2007.11.013
Sherman, K. (1991). The large marine ecosystem concept: research and management strategy for living marine resources. Ecol. Appl. 1, 349–360. doi: 10.2307/1941896
Sosik, H. M., and Olson, R. J. (2007). Automated taxonomic classification of phytoplankton sampled with imaging-in-flow cytometry. Limnol. Oceanogr. Methods 5, 204–216. doi: 10.4319/lom.2007.5.204
Stern, R., Kraberg, A., Bresnan, E., Kooistra, W. H. C. F., Lovejoy, C., Montresor, M., et al. (2018). Molecular analyses of protists in long-term observation programmes—current status and future perspectives. J. Plankton Res. 40, 519–536. doi: 10.1093/plankt/fby035
Tanhua, T., Pouliquen, S., Hausman, J., O’Brien, K., Bricher, P., de Bruin, T., et al. (2019). Ocean FAIR data services. Front. Mar. Sci. 6:440. doi: 10.3389/fmars.2019.00440
Vanderbilt, K. L., Blankman, D., Guo, X., He, H., Lin, C.-C., Lu, S.-S., et al. (2010). A multilingual metadata catalog for the ILTER: issues and approaches. Ecol. Inform. 5, 187–193. doi: 10.1016/j.ecoinf.2010.02.002
Vanderbilt, K. L., and Gaiser, E. (2017). The international long term ecological research network: a platform for collaboration. Ecosphere 8:e01697. doi: 10.1002/ecs2.1697
Keywords: climate change, marine ecosystems, ecology, EOVs, SWOT, DEIMS
Citation: Muelbert JH, Nidzieko NJ, Acosta ATR, Beaulieu SE, Bernardino AF, Boikova E, Bornman TG, Cataletto B, Deneudt K, Eliason E, Kraberg A, Nakaoka M, Pugnetti A, Ragueneau O, Scharfe M, Soltwedel T, Sosik HM, Stanisci A, Stefanova K, Stéphan P, Stier A, Wikner J and Zingone A (2019) ILTER – The International Long-Term Ecological Research Network as a Platform for Global Coastal and Ocean Observation. Front. Mar. Sci. 6:527. doi: 10.3389/fmars.2019.00527
Received: 13 November 2018; Accepted: 12 August 2019;
Published: 28 August 2019.
Edited by:
Laura Lorenzoni, University of South Florida, United StatesReviewed by:
Craig E. Nelson, University of Hawai‘i at Mānoa, United StatesMaria Kavanaugh, Oregon State University, United States
Copyright © 2019 Muelbert, Nidzieko, Acosta, Beaulieu, Bernardino, Boikova, Bornman, Cataletto, Deneudt, Eliason, Kraberg, Nakaoka, Pugnetti, Ragueneau, Scharfe, Soltwedel, Sosik, Stanisci, Stefanova, Stéphan, Stier, Wikner and Zingone. This is an open-access article distributed under the terms of the Creative Commons Attribution License (CC BY). The use, distribution or reproduction in other forums is permitted, provided the original author(s) and the copyright owner(s) are credited and that the original publication in this journal is cited, in accordance with accepted academic practice. No use, distribution or reproduction is permitted which does not comply with these terms.
*Correspondence: José H. Muelbert, am11ZWxiZXJ0QGZ1cmcuYnI=