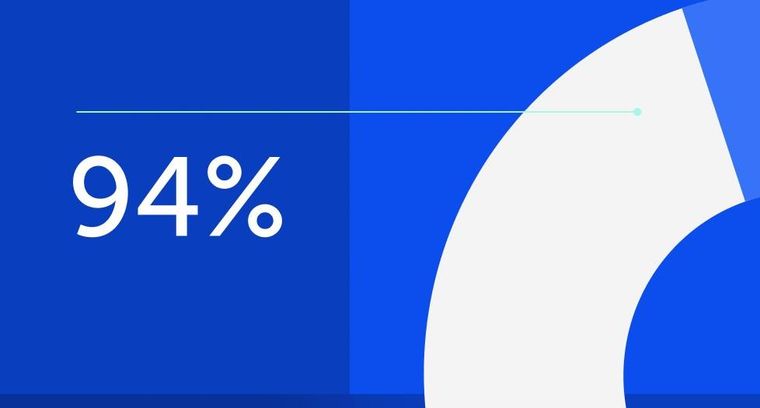
94% of researchers rate our articles as excellent or good
Learn more about the work of our research integrity team to safeguard the quality of each article we publish.
Find out more
ORIGINAL RESEARCH article
Front. Mar. Sci., 13 August 2019
Sec. Marine Ecosystem Ecology
Volume 6 - 2019 | https://doi.org/10.3389/fmars.2019.00490
Pelagic larval stages (meroplankton) of benthic invertebrates seasonally make up a significant proportion of planktonic communities, as well as determine the distribution of their benthic adult stages, yet are frequently overlooked by both plankton and benthic studies. Within the Arctic, the role of meroplanktonic larvae may be particularly important in regions of inflow from sub-Arctic regions, where they can serve as vectors of advection of temperate species into the Arctic. In this study, we describe the links between the distribution of larvae and adult benthic communities of bivalves, echinoderms, select decapods and cnidarians on the Pacific-influenced Chukchi Sea shelf during August-September in the time period 2004–2015 using traditional morphological and molecular tools to resolve taxonomic diversity. For most taxa, we observed little regional overlap between the distribution of larvae and adults of the same taxon; however, larvae of some organisms (e.g., the burrowing anemone Cerianthus sp., the sand dollar Echinarachnius parma) were only observed near populations of adult organisms. Larval stages of species not commonly observed in the Chukchi Sea benthos were also observed in the plankton; overall, shelf meroplanktonic communities were numerically dominated by larvae of coastal hard-bottom taxa, rather than local soft-bottom shelf species. Our results suggest that most larvae that we observe on the shelf are advected from other areas rather than produced locally, and most likely will not successfully settle to the benthos. Seasonality and distribution of water masses were the most important parameters shaping meroplankton communities. We discuss the implications of changing oceanographic and climatic conditions on the potential of range extensions by temperate species into the Arctic Ocean.
The Chukchi Sea is one of two inflow regions of the Arctic, providing the only connection between the temperate Pacific region and high Arctic Ocean (Figure 1). Every year, 0.8–1.2 Sv of Pacific water enter the Arctic through the Bering Strait (Woodgate, 2018), bringing in large quantities of heat, freshwater, nutrients, as well as rich pelagic communities of phyto- and zooplankton along with their predators. Unlike the Fram Strait opening in the Atlantic, the Bering Strait is narrow and shallow. The broad and shallow (<50 m) Chukchi Sea shelf serves as an impediment to Pacific organisms being advected into the Arctic Ocean, because most of this advected biomass and local production will not travel past the shelf break (Kosobokova et al., 2011; Grebmeier and Maslowski, 2014).
Figure 1. Major oceanographic features of the Chukchi Sea (modified from Stabeno et al., 2018) and locations of macrobenthic hotspots as defined by Grebmeier et al. (2015b).
Most of the flow entering the Chukchi Sea from the Pacific is dominated by a mixture of two currents: the Anadyr Current, originating on the Bering Sea slope and carrying cold, nutrient-rich oceanic water, and the Bering Shelf Current, which originates on the Bering Sea shelf and is warmer and lower in nutrients (Coachman and Aagaard, 1975). These two currents merge as they travel through the Bering Strait, forming Bering Sea-Anadyr Water, then split into three branches: one exiting north through Herald Canyon; one east of Hanna Shoal and through Barrow Canyon; and one through the Central Channel, which separates Herald Valley and Hanna Shoal (Weingartner et al., 2005; Figure 1). Due to uneven mixing of the two original currents, the western branch through Herald Valley is much richer in nutrients than the others, indicative of a higher contribution by Anadyr Water. Additionally, flowing adjacent to the Alaska coast is the highly seasonal, buoyancy-driven Alaska Coastal Current, which originates in the Gulf of Alaska, fed by river- and glacial discharge along its course, and carrying with it heat, freshwater and comparatively warmer-water organisms as far as the Beaufort Sea (Smoot and Hopcroft, 2017). Occasionally, winds carry this flow away from the coast, and during some years its signature has been observed covering the entire southern Chukchi Shelf (Pisareva et al., 2015b). Most of the water transport through the Bering Strait takes place in the summer months: during winter, the Chukchi and northern Bering Sea are completely covered by sea ice, the northward flow slows down or reverses (Woodgate et al., 2015), and the system “reverts” from an advection-dominated system to one resembling interior Arctic shelf seas (Williams and Carmack, 2015). Remnants of the cold, saline water mass that forms during ice formation remains near the sea floor on the northern Chukchi Shelf during summer as Winter Water (WW) (Weingartner et al., 2005), and is home to “resident” Chukchi Sea pelagic communities of organisms (Ershova et al., 2015a). A fourth water mass type, Siberian Coastal Water, is sometimes present in the western Chukchi Sea when the cold, buoyancy driven East-Siberian Coastal Current enters through Long Strait (Weingartner et al., 1999).
The persistent, high levels of advected and local pelagic primary production during the ice-free season on the Chukchi Shelf cannot be fully grazed by pelagic consumers, resulting in strong benthic-pelagic coupling, and very high benthic biomass in the region. In particular, the northern Bering Sea/Chukchi region is home to four benthic biomass “hotspots,” located in the pathway of the nutrient rich Anadyr water (Figure 1), characterized by a stable, exceptionally high (>20 g C m–2) biomass of macrobenthic organisms (Grebmeier et al., 2015b), which in turn provide a rich food source to a variety of higher trophic levels, such as walruses and diving sea birds. The area is also home to diverse and biomass-rich epibenthic communities (Bluhm et al., 2009; Blanchard et al., 2013).
Most benthic organisms reproduce by means of a pelagic larval stage (meroplankton), which may live in the plankton for hours to months, and allows them to disperse across wide areas. The ecological significance of planktonic larvae is two-fold: they are a dispersal stage for benthic organisms (Shanks, 2009), determining the potential of benthic species to colonize adjacent habitats, but they can also constitute a major portion of zooplankton communities in high latitude waters (Gluchowska et al., 2016), potentially competing for resources with holoplanktonic species, and serving as food source for planktonic predators (e.g., Allen, 2008; Short et al., 2013). An important biophysical process in benthic ecosystems is larval transport, which in advection-dominated systems can cause spatial decoupling between the production of local communities and settlement of juveniles. In Arctic regions highly influenced by northward flow, such as the Chukchi Sea, meroplanktonic larvae can serve as vectors of advection of sub-Arctic species into the Arctic. For sessile or slow-moving benthic species, larval transport is one of the only apparent mechanisms by which this expansion can occur (Renaud et al., 2015), which makes studies of larval communities within key inflow regions of utmost importance.
Despite being an important life stage of many key benthic species, and a seasonally significant contributor to zooplankton, meroplankton has been historically overlooked by benthic community ecologists, while plankton studies, at best, have grouped them into broad taxonomic categories. Studies on meroplankton are hampered by their temporally patchy occurrence in the plankton, as well as lack of morphological features (and/or taxonomic expertise) to assign them beyond phylum or class level. In large part due to extensive DNA barcoding efforts, such as done through the Census of Marine Life (McIntyre, 2010) and a growing richness of reference libraries (i.e., Barcode of Life Data Systems, BOLD1), it is becoming increasingly feasible to use barcoding instead of, or in addition to, morphology to describe biodiversity in marine ecosystems. Yet to date, there are only a few studies focusing on meroplankton at the species level, and even fewer from the high latitudes (i.e., Stanwell-Smith et al., 1999; Sewell, 2005; Fetzer and Arntz, 2008; Bowden et al., 2009; Heimeier et al., 2010; Sewell and Jury, 2011; Gallego et al., 2015; Silberberger et al., 2016; Brandner et al., 2017). The Pacific Arctic region is no exception; although both benthic (Grebmeier et al., 2006; Bluhm et al., 2009; Grebmeier, 2012; Iken et al., 2018) and pelagic (Hopcroft et al., 2010; Eisner et al., 2013; Ershova et al., 2015a; Pinchuk and Eisner, 2017) summer communities of the Chukchi Sea, particularly on the United States side, have been studied extensively and described in great detail during the last decades (Day et al., 2013; Sigler et al., 2017), meroplankton has been largely overlooked, with the exception of some studies focusing on specific groups (i.e., Landeira et al., 2017).
Presumably, the meroplanktonic communities in the Chukchi Sea consist of larvae both produced by the local benthic organisms, as well as advected with currents from other regions. In this study we examined the patterns in summer distribution of planktonic larvae in the Chukchi Sea region with reference to the distribution of their adult populations. To achieve previously intractable taxonomic resolution, one of the objectives of this study was to develop a time and cost-effective DNA barcoding protocol that would allow us to routinely resolve taxonomic diversity of larvae within the zooplankton and examine select taxonomic groups at or near the species level. We use these data to describe the diversity and distribution patterns of planktonic larvae on the Chukchi shelf during summer for five study years each with distinct oceanographic and thermal regimes, and with special focus on potential range expanders from the sub-Arctic. We aimed to identify the main driving factors that shape the meroplanktonic communities on the Chukchi sea shelf, anticipating that both local production and advection play important roles in the distribution and the fate of the larvae that we observe in this region.
Data on meroplankton distribution were obtained from published (Hopcroft et al., 2010; Eisner et al., 2013; Ershova et al., 2015a) and unpublished zooplankton surveys, conducted during summer-fall of 2004, 2007, 2009, 2012, and 2015 (Table 1) in the Chukchi Sea. All studies used a 150 μm Bongo net (ring diameter 60 cm), which was hauled vertically from a few meters off the bottom to the surface with a wire speed of 0.5 m/s and General Oceanics Flowmeters attached at the mouth opening. For all surveys, meroplankton was counted quantitatively from formalin-preserved samples and identified to macrotaxa level (i.e., Bivalvia, Echinodermata, Polychaeta, etc.), with the exception of crab megalopa in 2012 and 2015 that were identified to species. Since crab larvae are good swimmers and can avoid nets (Porter et al., 2008), for this group we included data from selected stations collected with a semi-oblique 505 μm Bongo net, which is much better at capturing larger and faster swimming planktonic organisms. For the purposes of our study, we converted all zooplankton abundance values to ind m–2. For more details on zooplankton sample processing and enumeration, see Ershova et al. (2015a).
Macrofaunal and megafaunal abundance and biomass estimates were obtained from benthic van Veen grab and beam trawl catches, respectively, taken concurrent to zooplankton collection during each expedition (Bluhm et al., 2009; Grebmeier et al., 2015a; Iken et al., 2018). Macrofauna samples were washed over 1 mm screen and bivalves were identified to species or family level, enumerated and weighed, with typically four replicates per station. Crabs and echinoderms were sorted from beam trawl catches (7 mm mesh, 4 mm in cod end), enumerated and weighed after identification in the field; taxonomic identification was later confirmed by taxonomic experts based on voucher material where needed. Densities of the burrowing anemone Cerianthus sp. were taken from a photographic survey (Sirenko and Gagaev, 2007), since this taxon rarely is captured with trawls or grabs. For each station, the abundances of benthic invertebrates in a given taxon were combined to match the taxonomic resolution of the meroplankton identification and averaged for each station over all sampling years. While some interannual/interdecadal trends in benthic biomass are recognized (Grebmeier et al., 2018), large-scale benthic communities biomass patterns in the Chukchi Sea have remained relatively stable on an inter-annual basis (Grebmeier et al., 2015a).
Bottom depth, and depth-stratified temperature and salinity measurements were obtained for each station (collected by a Seabird 911 + CTD, with all physical data binned into 1-m intervals during post-processing). For each station, we calculated surface (top 10 m) and bottom (10 m above the seafloor to bottom) temperature and salinity values.
The distribution and properties of the water masses, as well as the overall thermal characteristics and patterns in zooplankton communities in the Chukchi Sea during the expeditions are described in detail elsewhere for all years except 2015 (Pickart et al., 2010; Ershova et al., 2015a,b; Pisareva et al., 2015a, b). Surface- and bottom- water at each station was assigned to a water mass type based on temperature and salinity characteristics in accordance with methodology for previous years (Ershova et al., 2015b). Three major water masses dominated the region (Figure 2): Alaska Coastal Water (ACW), Bering-Sea Anadyr Water (BSAW), and WW, although the boundary between the first two was not always well defined and an intermediate water mass (ACW/BSAW) was assigned to stations with mixed properties. ACW was mostly found in the eastern Chukchi, although its signature was also observed away from the coast, especially in 2009, when this water mass was spread across the entire shelf as far north as Herald Canyon (Figure 2). BSAW was widespread across the shelf during all years, although it was pushed westward in 2009. WW was observed in the northern Chukchi during all years except 2007, when the more northern regions above 70°N were not sampled. The summers of 2004 and 2007 were the warmest of the study years, with an average surface temperature (SST) of 6–8°C over the sampled stations, and with surface waters as warm as 10–12°C present along the Alaska Coast and at the entrance of the Herald Canyon region. The years 2009 and 2012 were markedly colder, with the coldest SST observed in September 2012, averaging only 3.4°C, despite the all-time low ice-minimum extent observed during that year in most parts of the Arctic. In 2013–2014 the system shifted to warmer conditions again (Wood et al., 2015), and 2015 was also characterized by warmer than average conditions.
Figure 2. Spatial distribution of sampled stations and schematicdistribution of water masses during each sampling period [figure for2004–2012 modified from Eisner et al. (2013), Ershova et al. (2015a, b), Pisareva et al. (2015a)]. Squares indicate stations where DNA barcoding of larvae was done. BSAW, Bering Sea Anadyr Water; ACW, Alaska Coastal Water; WW, Winter Water; SCW, Siberian Coastal Water.
The diversity of bivalve and echinoderm larvae was investigated using DNA barcoding. These two groups were chosen because they were among the best represented both in the meroplankton and the benthic communities, as well as had the most complete reference libraries and were challenging to identify morphologically. While barnacle and polychaete larvae were also very common in the meroplankton, the former were presumably composed of only one or two species, limited in their adult distribution to rocky coastal regions, and the reference libraries for the latter are still among the poorest. The majority of the analyzed zooplankton samples had a second replicate preserved in 97% ethanol. For a total of 26 stations, we randomly selected 20–30 individuals of each bivalve and echinoderm larva from these ethanol-preserved samples. The stations were chosen based on overall abundance of the larvae, spatial coverage, and sample quality/availability. Each individual larva was soaked for ∼10 min in MilliQ water, then transferred using sterile tools into individual wells on a 96-well plate containing 25 μl Alkaline Lysis Buffer (ALB) on ice. Bivalves were crushed with the flat blade of a sterilized micro-scalpel prior to transfer. DNA extractions were conducted using the HotShot method (Truett et al., 2000); the plates containing larvae in ALB were incubated at 95°C for 30 min, then the plates were transferred onto ice and 25 μl of Tris–HCl was added to each well. This extraction method is very quick (30 min), inexpensive, and requires only two pipetting steps with no transfer of DNA, which ensures minimal loss of material. A total of 1030 larvae were processed in this way. Massive parallel barcoding of all extracts was achieved using a high-throughput sequencing strategy. A ∼313 base pair (bp) fragment from the 5′ region of the COI gene was amplified using a single-PCR protocol using tagged versions of the highly degenerated primer set Leray-XT (Wangensteen et al., 2018), containing forward primer mlCOIintF-XT 5′-GGWACWRGWTGRACWITITAYCCYCC-3′ and reverse primer jgHCO2198 5′-TAIACYTCIGGRTGICCRAARAAYCA-3′ (Geller et al., 2013). Sample tags of 8 bp were attached to both ends of the primers. Amplification was conducted used AmpliTaq Gold DNA polymerase, with 1 μl of each 5 μM forward and reverse tagged primers, 3 μg of bovine serum albumin and 2 μl of extracted DNA in a total volume of 20 μl per sample. The PCR protocol consisted of a denaturing step of 10 min at 95°C, 35 cycles of: 94°C for 1 min, 45°C for 1 min and 72°C for 1 min, and a final extension of 5 min at 72°C. To test the DNA quality, 80 samples, selected randomly, were sequenced using Sanger Sequencing. Sequencing reactions were performed on amplicons purified with ExoSap, using the BigDye Terminator v3.1 kit (Applied Biosystems). The sequencing products were analyzed on the ABI 3130 × l genetic analyzer (Applied Biosystems). The resulting sequences were assembled and aligned in BioEdit, the ends trimmed, and searched across both BLAST and BOLD (Barcode of Life) databases for identification. The remaining samples were purified using Minelute PCR purification columns2 and pooled (96 samples per library). Ten Illumina libraries were built from the DNA pools using the NextFlex PCR-free library preparation kit (Perkin-Elmer). This protocol incorporates Illumina adapters using a ligation procedure without any further PCR step, thus minimizing biases. The resulting libraries were equalized using qPCR then sequenced on an Illumina MiSeq using a V3 2 × 250 bp kit.
Metabarcoding sequences were analyzed following a similar pipeline as in Siegenthaler et al. (2019). Initial steps and quality control were based on OBITools (Boyer et al., 2016). Paired-end reads were aligned using illuminapairedend, and reads with alignment score >40 were retained. Demultiplexing and primer removal were achieved using ngsfilter with the default options. Sequences with length outside the 300–320 bp range and containing ambiguous bases were filtered out. Obiuniq was then used to dereplicate the reads and chimeric sequences were removed using the uchime_denovo algorithm implemented in VSearch (Rognes et al., 2016). We used Swarm 2.0 (Mahé et al., 2015) with a distance value of d = 13 (Wangensteen and Turon, 2017) to cluster unique sequences into OTUs. After removal of singletons, taxonomic pre-assignment of the representative sequences of every OTU was performed using ecotag (Boyer et al., 2016). Finally, taxonomic assignment of the most abundant OTU in each sample was checked by querying the sequences against the NCBI nucleotide database using BLAST and against the BOLD database (Ratnasingham and Hebert, 2007). All taxon names were standardized to the World Register of Marine Species (Costello et al., 2013).
All mapping and analyses were performed in R, using the package vegan (Oksanen, 2013). Patterns in community structure were examined using non-metric multidimensional scaling (nMDS) of Bray-Curtis similarities between stations. Prior to analysis, abundance matrices of examined taxa (separate matrices for macrotaxa and barcoded bivalves and echinoderms) were log-transformed, and then standardized using a Wisconsin standardization. Only species/groups that contributed at least 5% of the abundances at any station were included. The resulting ordination was correlated to taxa abundances and physical characteristics using the envfit function to determine which species or groups, as well as oceanographic, spatial and temporal characteristics (surface and bottom temperature and salinity, bottom depth, latitude and latitude, day of year sampled) drove the separation of the communities. The significance (p) and goodness of fit (R2) of the correlated variables were determined via 999 permutations of the variables.
Non-parametric permutational multivariate analysis of variance (PERMANOVA) was applied to test community differences between pre-assigned water mass types (surface and bottom) and sampling years (function adonis). The Bray-Curtis similarity index was calculated for log-transformed abundances, similar to the previous analysis. Dispersion within groups was tested using the function betadisper; non-significant results of the test were assumed to meet the PERMANOVA assumption of equal dispersion. Pseudo-F, p and R2 values were calculated based on 999 permutations of the residuals. Significant differences between specific pairs (years and/or water masses) were determined using a permutational multivariate pairwise T-test with a Holm adjustment for the resulting p-values.
Meroplankton was observed at every sampled station and in highly variable numbers, with abundances ranging from <100 ind m–2 to over 500,000 ind m–2 (Figure 3), and composing 1–90% of total zooplankton abundance on some stations (12% on average). The highest numbers of planktonic larvae were observed in August 2004, when the average across all stations was 105,000 ± 330,000 (mean and SD) ind m–2 and the mean contribution to overall zooplankton abundance was >30%. The lowest abundances were observed in September 2012 (mean 5800 ± 11300 ind m–2, 5% of overall zooplankton). During most years, the highest numbers of larvae were observed at stations near the Alaskan Coast, or if away from the coast, in water influenced by the Alaska Coastal Current (2004 and 2009) (Figures 2, 3).
Figure 3. Meroplankton abundance and composition in the Chukchi Sea during 2004, 2007, 2009, 2012, and 2015 (A) Total abundance of meroplankton; (B) relative contribution of different taxa of meroplankton. “Other” refers to Bryozoa and Gastropoda larvae.
The most abundant meroplankton taxa across all years and stations were barnacle (Cirripedia) and bivalve larvae (Figure 3 and Table 2). Barnacle larvae were particularly abundant in the central and northwestern Chukchi, and were most numerous during August 2004, when on average they composed almost 60,000 ind m–2, in distinct contrast to 2007, when they were practically absent. Bivalves were numerous during all years, especially 2007 and 2009, and were particularly dominant in the eastern Chukchi Sea (or in waters influenced by the Alaska Coastal Current, as in 2009). Larvae of echinoderms also composed a substantial percentage of the meroplankton in some areas: particularly near the Alaska coast in 2004 and 2015, as well as the Wrangel Island/Herald Canyon region during 2009 and 2012. Abundance of polychaete larvae was highest at stations in the northeast and northwest Chukchi, as well as south of Cape Lisburne in 2007, where at several stations they dominated absolute zooplankton abundance. Decapods (shrimp zoea and juveniles, crab and hermit crab zoea, and crab megalopa) were rare in the 150-μm vertical samples, but their abundance may have been underestimated due to net avoidance, as suggested by data from the 505-μm nets (see section on “Decapods” below). Larvae from other taxa (Cnidaria, Gastropoda, Bryozoa, and Nemertea) were also recorded, but generally occurred in extremely low abundances.
Table 2. Mean abundances (ind m–2) and percent contribution of each taxon to the total meroplankton abundance.
Of 1005 DNA extracts, usable sequences were obtained for 932, or about 93%. The remaining samples either failed to produce a corresponding sequence match in the library (e.g., were identified only at the level of Eukaryota), or matched to organisms unrelated to those sampled (e.g., Homo sapiens, or Copepoda), presumably due to contamination or degradation of the target DNA, although we cannot exclude the possibility that some species are not amplified due to primer bias. The rate of success was about equal for bivalves and echinoderms. A list of 35 OTU’s was produced (Table 3); however, the number of “species” was lower, both due to more than one OTU often being assigned to the same species (different bins), as well as due to the frequent absence of reference species in the library, and consequent assignment to a higher ranking (family or order). A total of 7 OTU’s were produced for Echinodermata, and 28 for Bivalvia; 10 of the Bivalvia OTU’s were only found once.
A hotspot of adult bivalve biomass (>20 g C m–2) was located in the southwestern Chukchi Sea, and was dominated both in biomass and abundance/density by Macoma calcarea (Figure 4). In other areas, bivalve biomass was substantially lower (generally < 10 g C m–2), and abundance was dominated by a variety of species from the families Nuculanidae, Thyasiridae, Yoldiidae and the superfamily Galeommatoidea (which includes the families Lasaeidae and Montacutinae) (Table 4). In contrast, the hotspot for larval abundance was consistently observed near the Alaska coast and in waters influenced by the Alaska Coastal Current (in 2009) (Figure 5). Within the northwest Chukchi, bivalve larvae were present in 2009, but were entirely absent in 2004 and 2012. Similarly, they were found at very few stations and in very low numbers in the northeast Chukchi in 2015.
Figure 4. Distribution of (A) biomass and (B) abundance and taxonomic composition of adult bivalves in the Chukchi Sea. Symbols to the right of the legend indicate the presence of taxa in the Chukchi Sea as planktonic larvae, adults, or both.
Figure 5. Distribution of larval bivalves (top row, overall abundance; bottom row, relative contribution by different bivalve taxa in the meroplankton).
A total of 28 larval OTUs were identified, of which three were classified biogeographically as boreal-Pacific, 14 as boreal-Arctic, and the rest of unknown affinity due to lack of taxonomic resolution. The majority of the sequenced larvae (90%) belonged to only 10 OTUs, of them two species that are presumed to be of North Pacific origin (Mytilus trossulus and Mactromeris polynyma). The taxonomic composition of the larvae was not at all reflective of the co-occurring adult bivalve communities, with only 2 of the 23 most common bivalve species represented in the larval communities. The most common taxa among the larvae was the coastal species Hiatella arctica, which was found at every station, and dominated most stations south of Cape Lisburne during all years. Adults of this species were numerous only at one coastal station near Alaska and, to a lesser extent, at one station near Wrangel Island. The 4 OTUs identified as Mya spp. (of them, 2 bins of Mya truncata) were the next most dominant group, also observed at nearly every station, and especially dominant around the Cape Lisburne area in 2007, 2012, and 2015. Larvae of the dominant bivalve species in the benthos, M. calcarea, were found in relatively large numbers in the Bering Strait region and in Herald Canyon in 2009, and in low numbers in ACW in 2012, but were notably absent in other areas and during all other years. Other fairly common larvae included an OTU belonging to the superfamily Galeommatoidea, an OTU identified as belonging to the family Mactridae, and M. trossulus. The rest of the taxa (18 OTUs) together composed no more than 10% of the larval bivalve abundance at any of the stations.
The dominant echinoderms in the epibenthos across the southern Chukchi Sea were the sea stars Leptasterias spp. and Henricia sp. as well as the ophiuroid Stegophiura nodosa (Figure 6 and Table 5). The northwestern Chukchi communities (Herald Canyon area), in contrast, were dominated by the sea star Ctenodiscus crispatus and to a lesser extent the ophiuroid Ophiura sarsii, while the northeastern Chukchi Sea was heavily dominated by O. sarsii and, near the coast, by the sand dollar E. parma. Other species, such as the sea star Crossaster papposus and Lethasterias nanimensis, were locally dominant at some stations (Figure 6 and Table 5).
Figure 6. Distribution and relative contribution to abundance of the most common adult echinoderms in the benthos of the Chukchi Sea.
The spatial distribution of the echinoderm larvae was variable among years, ranging from 0 to >100,000 ind. m–2 in different locations and during different years (Figure 7A). Similar to the bivalves, the highest abundances were also observed near the Alaska coast during all years, and especially in 2004 and 2015. Larvae were observed in the southwestern Chukchi in 2009, when this area was strongly influenced by ACW, but were absent in 2004 and 2012. Similarly, echinoderm larvae were common in the Herald Canyon region in September 2009 and 2012, but were entirely absent in 2004. It is noteworthy that with the exception of a narrow band along the coast, larvae were rare or absent in the northeastern Chukchi region in 2015.
Figure 7. (A) Distribution and abundance (1000 ind m–2) of echinoderm larvae during the sampling years 2004, 2007, 2009, 2012, and 2015; numbers outside large symbols (>25) indicate values at these stations (B) Taxonomic composition of echinoderm larvae; colors indicate relative species abundances; Symbols to the right of the taxonomic legend indicate the presence of taxa in the Chukchi Sea as planktonic larvae, adults, or both, with red indicating boreal-Pacific species occasionally observed in the Southern Chukchi and blue indicating Arctic species not found in the Chukchi, but common in adjacent Arctic seas. * – presumed species, see Results section for details.
The vast majority of echinoderm larvae were ophioplutei (76%), which were matched to 5 different species of ophiuroids: O. sarsii, Ophiocten sericeum, and Ophiopholis aculeata, as well as two species from the families Amphiuridae and Ophiuridae that were absent in the reference databases. Of the remaining individuals, 21% were echinoplutei, all of which were identified as E. parma, and 3% were bipinnaria, all identified as L. nanimensis (Figure 7B). No sea cucumber larvae were observed. Larvae of the most widely distributed and abundant adult ophiuroid species within the benthos in this area, O. sarsii, were abundant in the western and northwestern Chukchi stations in 2009 and 2012, but were notably rare or absent in the eastern and northeastern Chukchi during all study years, despite this area being a major hotspot for adult abundance. Surprisingly, the western Herald Canyon region in 2009 and 2012 was dominated by larvae of O. sericeum, another common Arctic ophiuroid, although adults of this species were absent on the Chukchi shelf.
The larval echinoderm communities at southeastern stations during 2007, 2012, and 2015 were heavily dominated by an ophiuroid species identified as an Amphiuridae. The only member of this family commonly found in the Chukchi epibenthos is the Pacific species Amphiodia craterodmeta, which notably lacked a reference barcode in the BOLD database (Ratnasingham and Hebert, 2007). We assumed these larvae to most likely belong to this species; however, its adult distribution was limited to the southwestern Chukchi and did not overlap with the distribution of the larvae. One other ophiuroid larva (listed as Ophiuridae sp., brown color on Figure 7B) did not produce a species match, but was most similar (90%) to O. robusta. We deduced this species to be O. maculata, an uncommon sibling Pacific species (that also lacks a barcode in BOLD) that is the only other member of the genus Ophiura ever observed in the region. Larvae of this species were present at most stations in the southern Chukchi Sea during all years, and were the dominant meroplankton species in the Bering Strait in 2009 (abundance approaching 14,000 ind/m2), with declining numbers toward the north. O. aculeata was the least common of the ophiuroid larvae, although found in low quantities at most stations near the Bering Strait and near the Point Hope/Cape Lisburne area. Adults of this species were also relatively uncommon and restricted to just a few locations characterized by the presence of harder substrate preferred by this species as occur near Point Hope/Cape Lisburne, in the northeast Chukchi, and in Herald Canyon. Of the common ophiuroid species found in the benthos, we did not observe larvae of three: S. nodosa, Ophiacantha bidentata, and Gorgonocephalus sp. Of these, the latter two ophiuroids are believed to reproduce via external brooding or benthic/demersal larvae, which would not have been captured by our sampling (Carmack and Wassmann, 2006; Shanks, 2001).
Notably, the larva of only one sea star (L. nanimensis, a boreal-Pacific species) was observed within the plankton, despite many species of sea stars being commonly present in the benthic communities. In the meroplankton, L. nanimensis larvae were common just south of the Bering Strait and found in low numbers at all stations around and just south of the Cape Lisburne area; notably, the adults of this species were highly abundant just south of that cape. The stations with extremely high abundances of echinoplutei, all belonging to E. parma, were located near the northeast Alaska coast, coincident with the location of high adult abundance. Both larvae and adults of this species were also found in lower numbers elsewhere, generally overlapping in their distributions. It is noteworthy that we observed no larvae of the other sea urchin present in the Chukchi Sea, Strongylocentrotus pallidus, although not entirely surprising due to the timing of our sampling (Falk-Petersen and Lonning, 1983).
Observed decapod larvae included shrimp zoea stages of the families Hippolytidae and Pandalidae (not shown), zoea and megalopa larvae of the anomuran crab family Paguridae and zoea and megalopa stages of three brachyuran crab species: the snow crab, Chionoecetes opilio, the Arctic lyre crab, Hyas coarctatus and the helmet crab, Telmessus cheiragonus (Figure 8). Among the adult crabs, C. opilio was the most common, with adults present at nearly every sampled location. The megalopae of this species, on the other hand, were only observed in the eastern Chukchi Sea and at stations influenced by the Alaska Coastal Current. Both adults and megalopae of H. coarctatus were found throughout the Chukchi Sea, although maximum densities of larvae were observed in the area where adults were relatively scarce (northwestern Chukchi region). T. cheiragonus adults were only observed on two stations near the Alaska Coast in extremely low numbers; larvae, on the other hand, were common at stations along the coast, with abundances occasionally exceeding 50 ind m–2.
Figure 8. Distribution and abundance of (A) crab and hermit crab juveniles and adults, and (B) crab and hermit crab megalopa during 2012 and 2015 based on Bongo 505 μm collections.
Actinulae of burrowing anemones (Ceriantharia) were observed exclusively in the western Herald Canyon region in 2009 and 2012 in abundances up to 1000 ind m–2, as well as, in lower numbers near the Siberian coast at stations containing Chukchi WW (Figure 9). We did not observe these larvae in any other area during any of the years. Estimates of adult abundances are difficult to obtain for this organism, but video surveys of the area only observed adults at the same, or nearby, stations as we observed the larvae, in aggregations with densities of up to 3–4 specimens m–2 (Sirenko and Gagaev, 2007).
Non-metric multidimensional scaling of log-transformed abundance of meroplankton groups (macrotaxon level) showed a moderate separation of meroplankton communities (2D stress = 0.17), mainly driven by bivalve, barnacle, and polychaete larvae, with bivalves and barnacles driving separation along the first axis, and polychaetes and decapods driving the separation along the second axis (Figure 10A). The ordination was moderately correlated to spatial (Longitude, R2 = 0.37) and temporal (Sampling Day, R2 = 0.26) gradients, as well as sampling year (R2 = 0.39) and water mass characteristics (bottom temperature and combined water mass type) (R2 = 0.27 and 0.21, respectively), although significant overlap between categories was observed. The centroids for ACW coincided with the maximum bivalve abundances, with a decreasing contribution of bivalve larvae in BSW water, while the centroids for WW and SCW were located near stations containing maximum contribution of polychaete, echinoderm and Ceriantharia larvae. PERMANOVA showed significant differences in meroplankton community abundance between all sampling years and most water mass types (Supplementary Table 1a), as well as the interaction between them; together they accounted for 57% variability. The most pronounced differences of larval assemblages between water masses were between BSW and WW, BSW and ACW, and WW and ACW (Supplementary Table 1b).
Figure 10. nMDS ordination of log-transformed abundance of meroplankton groups with (A) relative contribution of taxa at each station; and (B) water mass types at each station (symbols represent year sampled; outer color represents bottom water mass; inner color – surface water); (C) nMDS ordination of log-transformed abundance of bivalve and echinoderm species at stations where barcoding was done; symbols represent year sampled; outer color represents bottom water mass; inner color – surface water; On all plots, vectors indicate significant (p < 0.05) correlations of taxa abundances (gray arrows)/physical parameters (red arrows) to the ordination, with length reflecting R2, and text labels the centroids for each respective Year and Water Mass. T.btm, bottom temperature; S.btm, bottom salinity; T. surf, surface temperature; S.surf, surface salinity; Lat, latitude; Long, longitude; Day.of.yr, day of year sampled; Depth, bottom depth. Water masses are listed as Bottom Water Mass/Surface Water mass: BSAW, Bering Sea Anadyr Water; ACW, Alaska Coastal Water; BSAW.ACW, Bering Sea Anadyr/Alaska Coastal Water (mix or uncertain); WW, Winter water; SCW, Siberian Coastal Water.
Non-metric multidimensional scaling analysis of bivalve and echinoderm assemblages at stations where molecular identification was done (26 stations) produced a much clearer separation (2D stress = 0.12), as well as much stronger correlations to geography (Lat, Long; R2 = 0.44 and 0.42) and oceanographic parameters [surface and bottom temperature (R2 = 0.53 and 0.29), surface and bottom salinity (R2 = 0.26 and 0.41), as well as surface water mass type (R2 = 0.49)] (Figure 10B). There was no separation by sampling years or by date sampled. The ACW influenced stations were characterized by significantly higher contribution of the bivalves Mya sp. and family Mactridae as well as echinoderms Amphiuridae and E. parma (Figure 10C). The three western Herald Canyon stations (2009 and 2012) were highly dissimilar to the rest, driven mainly by the presence of O. sericeum and O. sarsii. PERMANOVA showed significant differences in community structure between water mass types, but not sampling years, with surface and bottom water mass type together accounting for 47% of observed variability, most of it explained by surface water mass type (Supplementary Table 2a). Pairwise-comparisons showed differences in larval communities to be significant between surface ACW and BSW, ACW and MW, and BSW/ACW and MW, as well as bottom BSW and WW (Supplementary Table 2b).
Benthic species within the same phylum, or even within a family or genus, can differ significantly in their ecology and distribution patterns, both at the larval and adult stages. Our study highlights the importance of approaching meroplankton at the species level, since both spatial and temporal patterns are obscured when larvae are grouped into broad categories. Species-specific morphological features are limited in early life stages, and morphological identification to higher taxonomic ranks in our study was only possible for crabs. Our study is among the first for Arctic meroplankton to supplement the morphological identification of meroplankton with molecular barcoding to better resolve taxonomic diversity of larvae. Our lab protocol resulted in a much higher success rate (>90%) than in previous attempts, where it did not exceed 20–50% (e.g., Webb et al., 2006; Heimeier et al., 2010; Brandner et al., 2017), and we hope that our effective, simple identification protocol will pave the way for future biodiversity studies of meroplankton.
Yet, limitations of our approach remain, such as the requirement to process larvae individually, restricting the scope of any even broader-scale ecological study. A metabarcoding assay of bulk DNA extracted from whole plankton samples could circumvent this limitation, but uncertainties in the quantitative relation between sequencing read abundances and biomass per species have to be clarified before the results of such approach can be compared to previous morphological surveys. Furthermore, a current constraint of molecular identification is the gaps in the reference libraries, making a match to species level in many cases impossible. Despite significant contributions by the Census of Marine Life, Polar Barcode of Life and other efforts (Hardy et al., 2011), of the approximately 112 echinoderm and bivalve species found in the region (Sirenko and Vassilenko, 2009), barcodes are currently only available for 40 (or 35%). The numbers are even smaller for polychaetes and other less studied groups, with gaps including many abundant and ecologically significant species. We strongly encourage the scientific community to continue adding additional species to the Barcode of Life Database so as to improve the identification success of larvae (and other samples of interest) in future studies.
The summer-fall meroplanktonic communities within the Chukchi Sea were characterized by overall low diversity, yet comparable to other high latitude regions in the northern hemisphere (e.g., Fetzer and Arntz, 2008; Silberberger et al., 2016; Michelsen et al., 2017), although undoubtedly the species list would grow substantially if other groups (e.g., polychaetes) were also barcoded. Additionally, our estimates of both abundance and diversity might be underestimated due to the mesh size used and many larvae being smaller than 150 μm and would be better sampled with finer-mesh nets. Furthermore, we may underestimate larval diversity due to many larval types occurring just above the bottom, as our samples were collected 3–5 m off the seafloor. The low diversity observed in our study and other parts of the Arctic (30–50 taxa) is in contrast with Antarctic regions, where the number of distinct taxa among the larvae is often estimated to be in the hundreds (Stanwell-Smith et al., 1999; Bowden et al., 2009). The difference in species richness between our study and the Antarctic studies mentioned above could represent a real natural pattern but could also be due to a more complete seasonal coverage in those studies and/or smaller mesh sizes capturing a wider size range of larvae.
Meroplankton abundance within summer-fall zooplankton communities in the Chukchi Sea ranged over four orders of magnitude (from <100 ind m–2 to over 500,000 ind m–2), and was characterized by extremely large spatial and inter-annual variability, although we recognize the challenge of comparing inter-annual data with such different spatial coverage. The high variability between locations and sampling years is a consequence of the inherently patchy distribution of larvae in time and space, due to the limited larval duration and episodic reproduction events in many benthic organisms (Shanks, 2009). For example, the exceptionally high abundance of barnacle larvae observed in 2004 was likely due to the earlier timing of the sampling by 3–4 weeks that year compared to other years, as in other Arctic regions, peak abundances of barnacle larvae have been recorded early in the summer (Brandner et al., 2017; Michelsen et al., 2017). A study on decapod larvae in the Chukchi Sea spanning two consecutive years similarly found abundances to differ over several orders of magnitude, which the authors explain by the slightly different timing of the cruises (Landeira et al., 2017). Although significant differences in larval assemblages were observed between years at the macrotaxa level, we did not observe any temporal trends over the examined time span of changes in abundances or composition, with each year being distinct in larval composition from the others. Yet, even with the high levels of variability there were persistent trends in larval distribution, mostly associated with water mass distribution, and especially when communities were examined at the species level. ACW was the “hotspot” for larval abundance and diversity during most years, characterized by a community composed mainly of bivalve and echinoderm larvae of coastal and North Pacific origin. In contrast, Bering Sea Anadyr Water was dominated by barnacle larvae, and generally carried lower quantities of meroplankton (with the exception of 2004). Similarly, distinct meroplankton groups, containing a higher contribution of decapod and polychaete larvae, were similarly observed in the northeast (Herald Canyon) and northwest Chukchi at stations containing WW. Future climate related changes in the relative transport and characteristics of these water masses into the Chukchi will also affect the composition of the larval communities carried within them.
It is noteworthy how few of the dominant benthic species, many of which presumably reproduce via pelagic larvae, we observed in the meroplankton. One possible explanation is that the reproductive window for these species falls outside of our sampling periods, all of which were during late summer-fall. Long-term sediment trap data from the northeastern Chukchi Sea, however, showed meroplankton abundance (mostly represented by polychaetes and barnacles) to peak around September, coinciding with our sampling periods (Lelande et al., in review). However, in other regions of the Arctic, the main meroplankton peaks often occur during or shortly after the spring bloom (Kuklinski et al., 2013; Stübner et al., 2016). A recent seasonal study in a Svalbard fjord observed two major abundance peaks for most meroplankton groups, with the first one occurring early in the spring, and the second in the summer-fall (Stübner et al., 2016), which could indicate different timing of reproduction for different species within the same phylum. The spawning of the sea urchin S. pallidus, for example, in the northeast Atlantic occurs in early spring (Falk-Petersen and Lonning, 1983), which likely explains the absence of the larvae of this species in our samples. Another explanation is that the planktonic larvae are too short-lived or do not rise up sufficiently from the seafloor to even be captured with nets, or do not reproduce via a planktonic larva altogether. Thorson’s Rule (Thorson, 1950; Mileikovsky, 1971) predicted a decline in the incidence of pelagic development with latitude, although these studies did not take into account organisms with planktonic lecitotrophy, and this rule has been partially disputed by newer evidence (Stanwell-Smith et al., 1999; Marshall et al., 2012; Landeira et al., 2017). However, as an example in support of the absence of pelagic larvae, recent live observations have suggested that S. nodosa may have brooding development (Lauren Sutton, University of Alaska Fairbanks, pers. obs.), rather than a typical pluteus larva, and the fact that we never observed larvae of this very common ophiuroid in any of our samples supports this observation. Within the bivalves, three of the most common families found in the Chukchi (Nuculanidae, Nuculidae, and Yoldiidae) belong to the subclass Protobranchia, which reproduce via a lecitotrophic pericalymma larva, rather than the typical bivalve veliger. These larvae are short-lived (hours to days) and stay near the bottom, which likely explains why we did not observe them in our net samples. The species that we did consistently observe in the meroplankton across the study area during most study years are likely characterized by an extended period of reproduction, or multiple overlapping spawning periods, as well as long larval duration. Larvae of H. arctica, M. calcarea, and Mya spp., as well as Cerianthus sp., were observed over periods of 5–8 months during the course of a year in other high latitude regions (Von Oertzen, 1972; Couwelaar, 2003; Brandner et al., 2017).
Inversely, for some species that we observed in the meroplankton no data were available on the distribution of adult forms. For example, the deep-dwelling clam families Myidae and Mactridae, both of which were among the most numerous bivalves in the meroplankton, cannot be adequately sampled by van Veen grab (Jay et al., 2014), so no estimates of distribution and abundance of these groups exist for the Chukchi Sea other than from the stomach contents of marine mammals. In the Bering Sea, Mya spp. and Mactromeris (=Spisula) polynyma (the only member of the family Mactridae in the Pacific sub-Arctic) are among the dominant prey items for walruses (Sheffield and Grebmeier, 2009; Jay et al., 2014) and bearded seals (Frost and Lowry, 1980), and M. polynyma is harvested by native communities in northwest Alaska (Magdanz et al., 2007). Walruses within the Chukchi Sea, however, mostly feed on other organisms (Sheffield and Grebmeier, 2009), suggesting that these clams are less common as adults in the benthos in this area. Similar to marine mammal diet analysis, the distribution of larvae of these groups may indirectly provide important insight on the distributions of adults within the benthos, although possible advection of larvae needs to be accounted for.
One striking, if not entirely surprising, result of our study was the distinct spatial mismatch between most adult benthic populations and their larval stages. Notable exceptions to this were organisms such as Cerianthus sp. and the sand dollar E. parma, whose larvae were only found close to the adult populations, suggesting either recent spawning from this local population as a source or larval retention by means of local oceanographic features and/or larval behavior. Larval behavior (e.g., vertical migration, response to turbulence, or chemical signals) coupled with local hydrodynamics can sometimes result in much shorter dispersal distances than predicted by larval duration solely, favoring retention of propagules closer to their spawning grounds (Shanks, 2009; Gaylord et al., 2013). Consistent with our observations of high densities of larvae near adult populations, it has been documented that E. parma larvae react to chemical cues produced by adults, which contributes to the dense aggregations of this species in the benthos (Pearce and Scheibling, 1990). It is also noteworthy that no significant sand dollar populations are present upstream from these locations.
However, most other larval types that we observed were presumed to be far from their points of origin. Most significantly, the meroplanktonic communities were heavily dominated by coastal forms (together Cirripedia and H. arctica composed on average >75% of meroplankton abundance). Therefore, the absence of these taxa on the Chukchi Sea shelf is most likely due to a lack of suitable substrate rather than larval supply. This is supported by the observations of dense patches of newly settled recruits of H. arctica covering moorings – an artificial hard substrate – in the south-central Chukchi Sea (K. Iken, pers. obs.). The high density of larvae of these hard-bottom coastal organisms that we observed across the studied area during all years reflects their life strategy: high fecundity, extended periods of reproduction, and long larval durations (Grantham et al., 2015), which is likely what makes them so common and successful in nearshore environments across all Arctic- and sub-Arctic seas.
Some of the other more common larvae presumably belonged to Pacific expatriates, such as the ophiuroids A. craterodmeta and O. maculata, and the crab T. cheiragonus, adults of which are rarely observed outside of the southern Chukchi Sea (Smirnov, 1994; Sirenko and Gagaev, 2007), although a large density of this species has been observed in kelp habitats in Peard Bay (Iken et al., personal observation). On the contrary, the Arctic ophiuroid O. sericeum is practically absent on the Chukchi shelf, but very numerous in the adjacent Beaufort and East-Siberian seas. The numerous larvae of this species that we observed in Herald Canyon is likely a consequence of local circulation patterns, which occasionally carry water from the East Siberian Sea into this region (Pisareva et al., 2015b). Therefore, its scarcity in the benthos in that region is not a consequence of lack of larval supply, but may be related to competition with O. sarsii or other dominant epibenthic species (Ravelo et al., 2017). While larval forms of some of the most abundant benthic species were numerous in the plankton (i.e., M. calcarea or O. sarsii), they were generally observed outside of the areas with the highest adult abundances.
A mismatch between adult and larval distribution is not surprising given the dispersive nature of the pelagic larval stage. Larval dispersal distance can be highly variable across taxa, dictated in large part by the time a larva spends in the water column prior to settling, ranging from a few minutes to several months (Shanks, 2009). Data on size distributions and developmental stages of larval populations could predict how recently these larvae were produced. Unfortunately, pelagic larval duration and dispersal distance are known only for a handful of species, and closely related species often show disparate dispersal strategies (Levin and Bridges, 1995), so it is difficult to predict duration without empirical evidence. Temperature also affects pelagic larval duration so that larvae in cold waters spend more time in the water column prior to settling and consequently can disperse much greater distances (O’Connor et al., 2007). This has major implications for the Chukchi and other Arctic seas where larvae could, in theory, disperse over longer distances than their boreal compatriots. Conversely, the recent warming seawater temperatures may result in larvae spending less time in the warming surface water and settling more quickly to benthos, and Arctic species settling farther to the south than dictated by their life history strategy.
The Chukchi Sea is a special environment within the Arctic in that at least during summer, it represents a direct extension of the Bering Sea and North Pacific. This Pacific connection is unique compared with the rest of the Arctic shelf seas that are either influenced by Atlantic inflow (i.e., Barents/Kara Seas), and/or are governed by local processes (i.e., interior shelves) (Williams and Carmack, 2015). As a result, the Chukchi Sea is characterized by unique pelagic communities, heavily dominated by Pacific expatriates. Even the species that are shared with adjacent Arctic seas may represent distinct populations of Pacific origin within the Chukchi Sea (Nelson et al., 2009; Ershova et al., 2015b). For holozooplankton, Pacific zooplankton species are occasionally observed in the surface waters of the deep Arctic Basin, but they never compose a significant part of the communities in contrast to Atlantic expatriates, such as C. finmarchicus (Kosobokova et al., 2011). Thus, the Chukchi Sea serves as a chokepoint for these species invading the Arctic. As with holoplanktonic expatriates, some meroplanktonic larvae get transported from the Chukchi Sea to the basins (Smoot and Hopcroft, 2017), but only eurybathic species would be able to settle at depths beyond the shelf break. Among the meroplankton taxa identified to species level, we did not detect deep-water species from the North Pacific. The long residence time on the Bering and Chukchi shelves likely inhibits the propagation of larvae of deep-water benthic species from the North Pacific to the Arctic Ocean, while larvae of most deep-water species likely never reach surface waters necessary to carry them northward in the first place.
In contrast to holozooplankton that permanently associate with hydrography (Pisareva et al., 2015a), meroplankton recruit to the seafloor and need to encounter conditions matching their habitat preference. We document the arrival of high densities of larvae of hard bottom species such the bivalve H. arctica and barnacles, but the predominantly fine sediment type (mud and silt), characteristic of the Chukchi Shelf (Feder et al., 1994; Grebmeier et al., 2015a), seems to largely preclude the settlement of such species. Since most of the sampling within our study has been conducted far off shore, we are limited in the knowledge of the distribution of the adults and larvae of these species in coastal domain of the study region. However, offshore the potential for successful range expansions through the Bering Strait is essentially limited to soft-bottom shelf species, such as many crabs, polychaetes, ophiuroids or bivalves. Larvae of species with potential of range expansion that we have already observed in our study include the North Pacific echinoderms L. nanimensis, O. maculata, and A. craterodmeta, the bivalves S. polynyma and M. trossulus and the crab T. cheraigonus. In support of this trend, adults of M. trossulus have been observed in the Northeastern Chukchi Sea in the late 1990s, whereas historically they had been absent in this area (Feder et al., 2003). The remainder of the advected larvae that fail to eventually settle to the benthos, play the same ecological role of advected holoplanktonic expatriates that cannot complete their life cycle in the Arctic, and instead become prey for planktonic predators or a sink to the benthos as detritus (Carmack and Wassmann, 2006).
The distinct mismatch between larval and adult benthic communities within the Chukchi Sea suggests that advection is the main factor driving larval distribution in this region. Our results suggest the vast majority of the larvae in terms of their abundance that we observe on the Chukchi Sea shelf during summer months are advected “visitors” from neighboring regions: from the North Pacific through the Bering Strait, from adjacent Arctic seas, and most significantly, from hard bottom coastal areas. The absence or rarity of adult forms of these advected species on the Chukchi Shelf is an indication that the vast majority of their larvae will not settle successfully and will become a food source for pelagic predators or a carbon sink to the benthos.
The datasets generated and compiled for this study can be found in the Mendeley Data Repository at http://dx.doi.org/10.17632/rhd9z8x86h.1.
EE, RD, and BB conceived the study. EE, RH, and CS collected and analyzed the zooplankton data. BB, JG, and KI collected and analyzed the benthic data. EE, RD, and OW completed the molecular work. OW completed the bioinformatics. EE performed the data analysis and wrote the manuscript with contributions from OW, RD, BB, KI, RH, CS, and JG.
This research has been jointly funded by the UiT – The Arctic University of Norway and the Tromsø Research Foundation under the project “Arctic Seasonal Ice Zone Ecology,” project number 01vm/h15. The collection and processing of 2015 samples by KI, RH, and CS was done within the framework the AMBON program funded through a National Ocean Partnership Program (NOPP Grant NA14NOS0120158) by the National Oceanic and Atmospheric Administration (NOAA), the Bureau of Ocean Energy Management, and the Shell Exploration & Production, under management of the Integrated Ocean Observing System (IOOS). The 2004, 2009, and 2012 samples were collected within the framework of the RUSALCA program with funds from the NOAA under cooperative agreements NA17RJ1224, NA13OAR4320056, and NA08OAR4320870 with the University of Alaska. The publication charges for this article were funded by the publication fund of the UiT – The Arctic University of Norway.
The authors declare that the research was conducted in the absence of any commercial or financial relationships that could be construed as a potential conflict of interest.
We thank Kim Præbel for providing lab facilities and advising with the development of the molecular protocol, Kyle Dilliplaine and Cheryl Hopcroft for providing lab support, Seth Danielsen for providing CTD data for the 2015 AMBON expedition. We also thank Sarah Hardy for providing ethanol samples and Lisa Eisner for providing the zooplankton data from the BASIS expedition. We acknowledge the support in the field during all expeditions, which contributed data to this manuscript. We also thank the two reviewers for their helpful comments on improving the manuscript.
The Supplementary Material for this article can be found online at: https://www.frontiersin.org/articles/10.3389/fmars.2019.00490/full#supplementary-material
Allen, J. D. (2008). Size-specific predation on marine invertebrate larvae. Biol. Bull. 214, 42–49. doi: 10.2307/25066658
Blanchard, A. L., Parris, C. L., Knowlton, A. L., and Wade, N. R. (2013). Benthic ecology of the northeastern Chukchi Sea. Part II. Spatial variation of megafaunal community structure, 2009-2010. Cont. Shelf Res. 67, 67–76. doi: 10.1016/j.csr.2013.04.031
Bluhm, B., Iken, K., Mincks Hardy, S., Sirenko, B., and Holladay, B. (2009). Community structure of epibenthic megafauna in the Chukchi Sea. Aquat. Biol. 7, 269–293. doi: 10.3354/ab00198
Bowden, D. A., Clarke, A., and Peck, L. S. (2009). Seasonal variation in the diversity and abundance of pelagic larvae of Antarctic marine invertebrates. Mar. Biol. 156, 2033–2047. doi: 10.1007/s00227-009-1235-1239
Boyer, F., Mercier, C., Bonin, A., Le Bras, Y., Taberlet, P., and Coissac, E. (2016). Obitools: a Unix-inspired software package for DNA metabarcoding. Mol. Ecol. Resour. 16, 176–182. doi: 10.1111/1755-0998.12428
Brandner, M. M., Stübner, E., Reed, A. J., Gabrielsen, T. M., and Thatje, S. (2017). Seasonality of bivalve larvae within a high Arctic fjord. Polar Biol. 40, 263–276. doi: 10.1007/s00300-016-1950-x
Carmack, E., and Wassmann, P. (2006). Food webs and physical-biological coupling on pan-Arctic shelves: unifying concepts and comprehensive perspectives. Prog. Oceanogr. 71, 446–477. doi: 10.1016/j.pocean.2006.10.004
Coachman, L. K., and Aagaard, K. (1975). Bering Strait: The Regional Physical Oceanography. Seattle, WA: University of Washington.
Costello, M. J., Bouchet, P., Boxshall, G., Fauchald, K., Gordon, D., Hoeksema, B. W., et al. (2013). Global coordination and standardisation in marine biodiversity through the World Register of Marine Species (WoRMS) and related databases. PLoS One 8:e51629. doi: 10.1371/journal.pone.0051629
Couwelaar, M. (2003). Zooplankton and Micronekton of the North Sea. Available at: http://species-identification.org/ (accessed April 20, 2019).
Day, R. H., Weingartner, T. J., Hopcroft, R. R., Aerts, L. A. M., Blanchard, A. L., Gall, A. E., et al. (2013). The offshore northeastern Chukchi Sea, Alaska: a complex high-latitude ecosystem. Cont. Shelf. Res. 67, 147–165. doi: 10.1016/j.csr.2013.02.002
Eisner, L., Hillgruber, N., Martinson, E., and Maselko, J. (2013). Pelagic fish and zooplankton species assemblages in relation to water mass characteristics in the northern Bering and southeast Chukchi seas. Polar Biol. 36, 87–113. doi: 10.1007/s00300-012-1241-0
Ershova, E. A., Hopcroft, R. R., and Kosobokova, K. N. (2015a). Inter-annual variability of summer mesozooplankton communities of the western Chukchi Sea: 2004–2012. Polar. Biol. 38, 1461–1481. doi: 10.1007/s00300-015-1709
Ershova, E. A., Hopcroft, R. R., Kosobokova, K. N., Matsuno, K., Nelson, R. J., Yamaguchi, A., et al. (2015b). Long-term changes in summer zooplankton communities of the Western Chukchi Sea, 1945–2012. Oceanography 28, 100–115. doi: 10.5670/oceanog.2015.60
Falk-Petersen, I. B., and Lonning, S. (1983). Reproductive cycles of two closely related sea urchin species, Strongylocentrotus droebachiensis (O.F. Müller) and Strongylocentrotus pallidus (G.O. sars). Sarsia 68, 157–164. doi: 10.1080/00364827.1983.10420567
Feder, H. M., Naidu, A. S., Jewett, S. C., Hameedi, J. M., Johnson, W. R., and Whitledge, T. E. (1994). The northeastern Chukchi Sea: benthos-environmental interactions. Mar. Ecol. Prog. Ser. 111, 171–190. doi: 10.3354/meps111171
Feder, H. M., Norton, D. W., and Geller, J. B. (2003). A review of apparent 20th century changes in the presence of mussels (Mytilus trossulus) and macroalgae in Arctic Alaska, and of historical and paleontological evidence used to relate mollusc distributions to climate change. Arctic 56, 391–407. doi: 10.14430/arctic636
Fetzer, I., and Arntz, W. E. (2008). Reproductive strategies of benthic invertebrates in the Kara Sea (Russian Arctic): adaptation of reproduction modes to cold water. Mar. Ecol. Prog. Ser. 356, 189–202. doi: 10.3354/meps07271
Frost, K. J., and Lowry, L. F. (1980). Feeding of ribbon seals (Phoca fasciata) in the Bering Sea in spring. Can. J. Zool. 58, 1601–1607. doi: 10.1139/z80-219
Gallego, R., Heimeier, D., Lavery, S., and Sewell, M. A. (2015). The meroplankton communities from the coastal Ross Sea: a latitudinal study. Hydrobiologia 761, 195–209. doi: 10.1007/s10750-015-2487
Gaylord, B., Hodin, J., and Ferner, M. C. (2013). Turbulent shear spurs settlement in larval sea urchins. Proc. Natl. Acad. Sci. U.S.A. 110, 6901–6906. doi: 10.1073/pnas.1220680110
Geller, J., Meyer, C., Parker, M., and Hawk, H. (2013). Redesign of PCR primers for mitochondrial cytochrome c oxidase subunit I for marine invertebrates and application in all-taxa biotic surveys. Mol. Ecol. Resour. 13, 851–861. doi: 10.1111/1755-0998.12138
Gluchowska, M., Kwasniewski, S., Prominska, A., Olszewska, A., Goszczko, I., Falk-Petersen, S., et al. (2016). Zooplankton in Svalbard fjords on the Atlantic–Arctic boundary. Polar Biol. 39, 1785–1802. doi: 10.1007/s00300-016-1991-1
Grantham, B. A., Eckert, G. L., and Shanks, A. L. (2015). Dispersal potential of marine invertebrates in diverse habitats. Ecol. Appl. 13, 108–116. doi: 10.1890/1051-0761(2003)013%5B0108:dpomii%5D2.0.co;2
Grebmeier, J. M., Bluhm, B. A., Cooper, L. W., Denisenko, S. G., Iken, K., Kedra, M., et al. (2015a). Time-series benthic community composition and biomass and associated environmental characteristics in the Chukchi Sea during the RUSALCA 2004–2012 program. Oceanography 28, 116–133. doi: 10.5670/oceanog.2015.61
Grebmeier, J. M., Bluhm, B. A., Cooper, L. W., Danielson, S. L., Arrigo, K. R., Blanchard, A. L., et al. (2015b). Ecosystem characteristics and processes facilitating persistent macrobenthic biomass hotspots and associated benthivory in the Pacific Arctic. Prog. Oceanogr. 136, 92–114. doi: 10.1016/j.pocean.2015.05.006
Grebmeier, J., Frey, K., Cooper, L., and Kêdra, M. (2018). Trends in benthic macrofaunal populations, seasonal sea ice persistence, and bottom water temperatures in the bering strait region. Oceanography 31, 136–151. doi: 10.5670/oceanog.2018.224
Grebmeier, J. M. (2012). Shifting patterns of life in the Pacific Arctic and sub-Arctic seas. Ann. Rev. Mar. Sci. 4, 63–78. doi: 10.1146/annurev-marine-120710-100926
Grebmeier, J. M., Cooper, L. W., Feder, H. M., and Sirenko, B. I. (2006). Ecosystem dynamics of the Pacific-influenced Northern Bering and Chukchi Seas in the Amerasian Arctic. Prog. Oceanogr. 71, 331–361. doi: 10.1016/j.pocean.2006.10.001
Grebmeier, J. M., and Maslowski, W. (2014). The Pacific Arctic Region: Ecosystem Status and Trends in a Rapidly Changing Environment. Berlin: Springer.
Hardy, S. M., Carr, C. M., Hardman, M., Steinke, D., Corstorphine, E., and Mah, C. (2011). Biodiversity and phylogeography of Arctic marine fauna: Insights from molecular tools. Mar. Biodivers. 41, 195–210. doi: 10.1007/s12526-010-0056-x
Heimeier, D., Lavery, S., and Sewell, M. A. (2010). Using DNA barcoding and phylogenetics to identify Antarctic invertebrate larvae: lessons from a large scale study. Mar. Genomics 3, 165–177. doi: 10.1016/j.margen.2010.09.004
Hopcroft, R. R., Kosobokova, K. N., and Pinchuk, A. I. (2010). Zooplankton community patterns in the Chukchi Sea during summer 2004. Deep. Res. Part II Top. Stud. Oceanogr. 57, 27–39. doi: 10.1016/j.dsr2.2009.08.003
Iken, K., Mueter, F., Grebmeier, J. M., Cooper, L. W., Danielson, S. L., and Bluhm, B. A. (2018). Developing an observational design for epibenthos and fish assemblages in the Chukchi Sea. Deep. Res. Part II Top. Stud. Oceanogr. 162, 180–190. doi: 10.1016/j.dsr2.2018.11.005
Jay, C. V., Grebmeier, J. M., Fischbach, A. S., McDonald, T. L., Cooper, L. W., and Hornsby, F. (2014). Pacific walrus (Odobenus rosmarus divergens) resource selection in the northern Bering Sea. PLoS One 9:e93035. doi: 10.1371/journal.pone.0093035
Kędra, M., Włodarska-Kowalczuk, M., and Węsławski, J. M. (2010). Decadal change in macrobenthic soft-bottom community structure in a high Arctic fjord (Kongsfjorden, Svalbard). Polar Biol. 33, 1–11. doi: 10.1007/s00300-009-0679-1
Kosobokova, K. N., Hopcroft, R. R., and Hirche, H. J. (2011). Patterns of zooplankton diversity through the depths of the Arctic’s central basins. Mar. Biodivers. 41, 29–50. doi: 10.1007/s12526-010-0057-9
Kuklinski, P., Berge, J., McFadden, L., Dmoch, K., Zajaczkowski, M., Nygård, H., et al. (2013). Seasonality of occurrence and recruitment of Arctic marine benthic invertebrate larvae in relation to environmental variables. Polar Biol. 36, 549–560. doi: 10.1007/s00300-012-1283-3
Landeira, J. M., Matsuno, K., Yamaguchi, A., Hirawake, T., and Kikuchi, T. (2017). Abundance, development stage, and size of decapod larvae through the Bering and Chukchi Seas during summer. Polar Biol. 40, 1805–1819. doi: 10.1007/s00300-017-2103-6
Levin, L. A., and Bridges, T. S. (1995). “Pattern and Diversity in Reproduction and Development,” in Ecology of Marine Invertebrate Larvae. Boca Raton, FL: CRC Press, Inc., 1–48.
Magdanz, J., Braem, N., Robbins, B., and Koster, D. (2007). Subsistence Harvests in Northwest Alaska, Kivalina and Noatak. Kotzebue, AK: Alaska Department of Fish and Game Division of Subsistence.
Mahé, F., Rognes, T., Quince, C., de Vargas, C., and Dunthorn, M. (2015). Swarm v2: highly-scalable and high-resolution amplicon clustering. PeerJ 3:e1420. doi: 10.7717/peerj.1420
Marshall, D. J., Krug, P. J., Kupriyanova, E. K., Byrne, M., and Emlet, R. B. (2012). The biogeography of marine invertebrate life histories. Annu. Rev. Ecol. Evol. Syst. 43, 97–114. doi: 10.1146/annurev-ecolsys-102710-145004
McIntyre, A. D. (2010). Life in the World’s Oceans: Diversity, Distribution, and Abundance. Hoboken, HOB: Wiley-Blackwell.
Michelsen, H. K., Svensen, C., Reigstad, M., Nilssen, E. M., and Pedersen, T. (2017). Seasonal dynamics of meroplankton in a high-latitude fjord. J. Mar. Syst. 168, 17–30. doi: 10.1016/j.jmarsys.2016.12.001
Mileikovsky, S. A. (1971). Types of larval development in marine bottom invertebrates, their distribution and ecological significance: a re-evaluation. Mar. Biol. 10, 193–213. doi: 10.1007/BF00352809
Nelson, R. J., Carmack, E. C., McLaughlin, F. A., and Cooper, G. A. (2009). Penetration of pacific zooplankton into the western arctic ocean tracked with molecular population genetics. Mar. Ecol. Prog. Ser. 381, 129–138. doi: 10.3354/meps07940
O’Connor, M. I., Bruno, J. F., Gaines, S. D., Halpern, B. S., Lester, S. E., Kinlan, B. P., et al. (2007). Temperature control of larval dispersal and the implications for marine ecology, evolution, and conservation. Proc. Natl. Acad. Sci. 104, 1266–1271. doi: 10.1073/pnas.0603422104
Pearce, C. M., and Scheibling, R. E. (1990). Induction of settlement and metamorphosis in the sand dollar Echinarachnius parma: evidence for an adult-associated factor. Mar. Biol. 107, 363–369. doi: 10.1007/BF01319838
Pickart, R. S., Pratt, L. J., Torres, D. J., Whitledge, T. E., Proshutinsky, A. Y., Aagaard, K., et al. (2010). Evolution and dynamics of the flow through herald canyon in the western Chukchi Sea. Deep. Res. Part II Top. Stud. Oceanogr. 57, 5–26. doi: 10.1016/j.dsr2.2009.08.002
Pinchuk, A. I., and Eisner, L. B. (2017). Spatial heterogeneity in zooplankton summer distribution in the eastern Chukchi Sea in 2012–2013 as a result of large-scale interactions of water masses. Deep. Res. Part II Top. Stud. Oceanogr. 135, 27–39. doi: 10.1016/j.dsr2.2016.11.003
Pisareva, M. N., Pickart, R. S., Iken, K., Ershova, E., Grebmeier, J., Cooper, L., et al. (2015a). The relationship between patterns of benthic fauna and zooplankton in the Chukchi Sea and physical forcing. Oceanography 28, 68–83. doi: 10.5670/oceanog.2015.58
Pisareva, M. N., Pickart, R. S., Spall, M. A., Nobre, C., Torres, D. J., Moore, G. W. K., et al. (2015b). Flow of Pacific water in the western Chukchi Sea: results from the 2009 RUSALCA expedition. Deep. Res. Part I Oceanogr. Res. Pap. 105, 53–73. doi: 10.1016/j.dsr.2015.08.011
Porter, S. S., Eckert, G. L., Byron, C. J., and Fisher, J. L. (2008). Comparison of light traps and plankton tows for sampling brachyuran crab larvae in an Alaskan Fjord. J. Crustac. Biol. 28, 175–179. doi: 10.1651/06-2818R.1
Ratnasingham, S., and Hebert, P. D. N. (2007). The Barcode of Life Data System (www.barcodinglife.org). Mol. Ecol. Notes 7, 355–364. doi: 10.1111/j.1471-8286.2006.01678.x
Ravelo, A. M., Konar, B., Bluhm, B., and Iken, K. (2017). Growth and production of the brittle stars Ophiura sarsii and Ophiocten sericeum (Echinodermata: Ophiuroidea). Cont. Shelf Res. 139, 9–20. doi: 10.1016/j.csr.2017.03.011
Renaud, P. E., Sejr, M. K., Bluhm, B. A., Sirenko, B., and Ellingsen, I. H. (2015). The future of Arctic benthos: expansion, invasion, and biodiversity. Prog. Oceanogr. 139, 244–257. doi: 10.1016/j.pocean.2015.07.007
Rognes, T., Flouri, T., Nichols, B., Quince, C., and Mahé, F. (2016). VSEARCH: a versatile open source tool for metagenomics. PeerJ 4:e2584. doi: 10.7717/peerj.2584
Sewell, M. A. (2005). Examination of the meroplankton community in the south-western Ross Sea, Antarctica, using a collapsible plankton net. Polar Biol. 28, 119–131. doi: 10.1007/s00300-004-0670-9
Sewell, M. A., and Jury, J. A. (2011). Seasonal patterns in diversity and abundance of the high antarctic meroplankton: plankton sampling using a Ross sea desalination plant. Limnol. Oceanogr. 56, 1667–1681. doi: 10.4319/lo.2011.56.5.1667
Shanks, A. L. (2001). An Identification Guide to the Larval Marine Invertebrates of the Pacific Northwest. Corvallis, OR: Oregon State University Press, 272.
Shanks, A. L. (2009). Pelagic larval duration and dispersal distance revisited. Biol. Bull. 216, 373–385. doi: 10.2307/25548167
Sheffield, G., and Grebmeier, J. M. (2009). Pacific walrus (Odobenus rosmarus divergens): differential prey digestion and diet. Mar. Mammal Sci. 25, 761–777. doi: 10.1111/j.1748-7692.2009.00316.x
Short, J., Metaxas, A., and Daigle, R. M. (2013). Predation of larval benthic invertebrates in St George’s Bay, Nova Scotia. J. Mar. Biol. Assoc. U.K. 93, 591–599. doi: 10.1017/s0025315412000768
Siegenthaler, A., Wangensteen, O. S., Benvenuto, C., Campos, J., and Mariani, S. (2019). DNA metabarcoding unveils multiscale trophic variation in a widespread coastal opportunist. Mol. Ecol. 28, 232–249. doi: 10.1111/mec.14886
Sigler, M. F., Mueter, F. J., Bluhm, B. A., Busby, M. S., Cokelet, E. D., Danielson, S. L., et al. (2017). Late summer zoogeography of the northern Bering and Chukchi seas. Deep. Res. Part II Top. Stud. Oceanogr. 135, 168–189. doi: 10.1016/j.dsr2.2016.03.005
Silberberger, M. J., Renaud, P. E., Espinasse, B., and Reiss, H. (2016). Spatial and temporal structure of the meroplankton community in a sub-Arctic shelf system. Mar. Ecol. Prog. Ser. 555, 79–93. doi: 10.3354/meps11818
Sirenko, B. I. (2009). “The present state of investigations of the Chukchi Sea fauna,” in Ecosystems and Biological Resources of the Chukchi Sea and Adjacent Areas. Explorations of the Fauna of the Sea, Vol. 64, ed. B. I. Sirenkocpesnm (St. Petersburg: Zoological Institute of RAS), 5–27.
Sirenko, B. I., and Gagaev, S. Y. (2007). Unusual abundance of macrobenthos and biological invasions in the Chukchi Sea. Russ. J. Mar. Biol. 33, 355–364. doi: 10.1134/S1063074007060016
Sirenko, B. I., and Vassilenko, S. V. (2009). Fauna and zoogeography of benthos of the Chukchi Sea. St. Petersburg: Russian Academy of Sciences, Zoological Institute.
Smirnov, A. V. (1994). “Arctic echinoderms: composition, distribution and history of the fauna,” in Echinoderms Through Time. Proceedings of a conference held in Dijon, France, 6-10 September 1993, eds D. Bruno, A. Guille, J.-P. Feral, and M. Rouxcpesnm, (Rotterdam: A. A. Balkema), 135–143.
Smoot, C. A., and Hopcroft, R. R. (2017). Cross-shelf gradients of epipelagic zooplankton communities of the Beaufort Sea and the influence of localized hydrographic features. J. Plankton Res. 39, 65–75. doi: 10.1093/plankt/fbw080
Stabeno, P., Kachel, N., Ladd, C., and Woodgate, R. (2018). Flow Patterns in the Eastern Chukchi Sea: 2010–2015. J. Geophys. Res. Ocean. 123, 1177–1195. doi: 10.1002/2017JC013135
Stanwell-Smith, D., Peck, L. S., Clarke, A., Murray, A. W. A., and Todd, C. D. (1999). The distribution, abundance and seasonality of pelagic marine invertebrate larvae in the maritime Antarctic. Philos. Trans. R. Soc. B Biol. Sci. 354, 471–484. doi: 10.1098/rstb.1999.0398
Stübner, E. I., Søreide, J. E., Reigstad, M., Marquardt, M., and Blachowiak-Samolyk, K. (2016). Year-round meroplankton dynamics in high-Arctic Svalbard. J. Plankton Res. 38, 522–536. doi: 10.1093/planktfbv124
Thorson, G. (1950). Reproductive and larval ecology of marine bottom invertebrates. Biol. Rev. 25, 1–45. doi: 10.1111/j.1469-185X.1950.tb00585.x
Truett, G., Walker, A., Truett, A., Mynatt, R., Heeger, P., and Warman, M. (2000). Preparation of PCR-quality DNA with hot sodium hydroxide and tris (HOTSHOT). Biotechniques 29, 52–54. doi: 10.2144/00291bm09
Tyler, P. A., and Gage, J. D. (1982). The reproductive biology of Ophiacantha bidentata (Echinodermata: Ophiuroidea) from the rockall trough. J. Mar. Biol. Assoc. 62, 45–55. doi: 10.1017/S0025315400020099
Väinölä, R. (2003). Repeated trans-Arctic invasions in littoral bivalves: molecular zoogeography of the Macoma balthica complex. Mar. Biol. 143, 935–946. doi: 10.1007/s00227-003-1137-1
Von Oertzen, J. A. (1972). Cycles and rates of reproduction of six Baltic Sea bivalves of different zoogeographical origin. Mar. Biol. 14, 143–149. doi: 10.1007/BF00373213
Wangensteen, O. S., Palacín, C., Guardiola, M., and Turon, X. (2018). DNA metabarcoding of littoral hard-bottom communities: high diversity and database gaps revealed by two molecular markers. PeerJ 6:e4705. doi: 10.7717/peerj.4705
Wangensteen, O. S., and Turon, X. (2017). “Metabarcoding techniques for assessing biodiversity of marine animal forests,” in Marine Animal Forests: The Ecology of Benthic Biodiversity Hotspots, eds S. Rossi, L. Bramanti, A. Gori, and C. O. Saco del Vallecpesnm, (Berlin: springer), 445–473. doi: 10.1007/978-3-319-21012-4_53
Webb, K. E., Barnes, D. K. A., Clark, M. S., and Bowden, D. A. (2006). DNA barcoding: a molecular tool to identify Antarctic marine larvae. Deep Res. Part II Top. Stud. Oceanogr. 53, 1053–1060. doi: 10.1016/j.dsr2.2006.02.013
Weingartner, T., Aagaard, K., Woodgate, R., Danielson, S., Sasaki, Y., and Cavalieri, D. (2005). Circulation on the north central Chukchi Sea shelf. Deep. Res. Part II Top. Stud. Oceanogr. 52, 3150–3174. doi: 10.1016/j.dsr2.2005.10.015
Weingartner, T. J., Danielson, S., Sasaki, Y., Pavlov, V., and Kulakov, M. (1999). The siberian coastal current: a wind- and buoyancy-forced Arctic coastal current. J. Geophys. Res. Ocean. 104, 29697–29713. doi: 10.1029/1999JC900161
Williams, W. J., and Carmack, E. C. (2015). The “interior” shelves of the Arctic Ocean: physical oceanographic setting, climatology and effects of sea-ice retreat on cross-shelf exchange. Prog. Oceanogr. 139, 24–41. doi: 10.1016/j.pocean.2015.07.008
Wood, K. R., Bond, N. A., Danielson, S. L., Overland, J. E., Salo, S. A., Stabeno, P. J., et al. (2015). A decade of environmental change in the Pacific Arctic region. Prog. Oceanogr. 136, 12–31. doi: 10.1016/j.pocean.2015.05.005
Woodgate, R., Stafford, K., and Prahl, F. (2015). A synthesis of year-round iinterdisciplinary mooring measurements in the Bering Strait (1990–2014) and the RUSALCA years (2004–2011). Oceanography 28, 46–67. doi: 10.5670/oceanog.2015.57
Keywords: meroplankton, zooplankton, Chukchi Sea, Pacific Arctic, DNA barcoding, benthic ecology, planktonic larvae
Citation: Ershova EA, Descoteaux R, Wangensteen OS, Iken K, Hopcroft RR, Smoot C, Grebmeier JM and Bluhm BA (2019) Diversity and Distribution of Meroplanktonic Larvae in the Pacific Arctic and Connectivity With Adult Benthic Invertebrate Communities. Front. Mar. Sci. 6:490. doi: 10.3389/fmars.2019.00490
Received: 24 May 2019; Accepted: 22 July 2019;
Published: 13 August 2019.
Edited by:
Heliana Teixeira, University of Aveiro, PortugalReviewed by:
Jose Maria Landeira, Hiroshima University, JapanCopyright © 2019 Ershova, Descoteaux, Wangensteen, Iken, Hopcroft, Smoot, Grebmeier and Bluhm. This is an open-access article distributed under the terms of the Creative Commons Attribution License (CC BY). The use, distribution or reproduction in other forums is permitted, provided the original author(s) and the copyright owner(s) are credited and that the original publication in this journal is cited, in accordance with accepted academic practice. No use, distribution or reproduction is permitted which does not comply with these terms.
*Correspondence: E. A. Ershova, ZWxpemF2ZXRhLmVyc2hvdmFAdWl0Lm5v
Disclaimer: All claims expressed in this article are solely those of the authors and do not necessarily represent those of their affiliated organizations, or those of the publisher, the editors and the reviewers. Any product that may be evaluated in this article or claim that may be made by its manufacturer is not guaranteed or endorsed by the publisher.
Research integrity at Frontiers
Learn more about the work of our research integrity team to safeguard the quality of each article we publish.