- 1Department of Arctic and Marine Biology, Faculty of Biosciences, Fisheries and Economics, UiT – The Arctic University of Norway, Tromsø, Norway
- 2SINTEF Ocean, Trondheim, Norway
The northern Svalbard shelf region is part of the Atlantic advective contiguous domain along which nutrients, phyto- and mesozooplankton are advected with Atlantic Water from the Norwegian Sea along the Norwegian shelf break and into the Arctic Ocean. By applying the SINMOD model, we investigated how much mesozooplankton may be advected into the northern Svalbard shelf region. We also compared this supply with the local mesozooplankton production. To achieve this, we selected a box north of Svalbard and calculated the in- and outflux of Atlantic Calanus finmarchicus and Arctic Calanus glacialis. The average biomass inside the box ranged between 0.5 and 3.0 g C month–2 in March and August, respectively. Annually, 18.8 g C month–2 of advected (and locally produced) mesozooplankton would be available for predators inside the box before it is advected out. The advection of mesozooplankton reached 12 times more than the average biomass within the box. The model projects significance variability in mesozooplankton advection which may be explained by the hitherto non-quantified recirculation in the northern Fram Strait and differences in the geographic origin of the mesozooplankton source population. The results imply that grazing upon mesozooplankton in the Atlantic advective contiguous domain north of Svalbard is greatly advantageous for pelagic predators. It could represent an important food source for fish, birds, and whales. It is suggested that mesozooplankton encountered on the shelf north of Svalbard may derive from populations along the North Norwegian shelf break, in some years as far south as the Lofoten/Vesterålen region. This illustrates the extent and significance of the Atlantic advective contiguous domain for the European shelf of the Arctic Ocean which apparently depends on significant food supply through expatriates. Primary production on the shelf is lower than C consumption and thus the European shelf of the AO is presumably net-heterotrophic.
Introduction
The North Atlantic Current transports large amounts of phytoplankton and nutrients along the slope off and on the Northern-Norwegian shelf. For the connection between subarctic regions and the Arctic Ocean (AO), the transport of long-lived mesozooplankton from the eastern realms of the Norwegian Sea northward is of particular interest (Wassmann et al., 2015). For the most part, the mesozooplankton biomass is comprised by the copepod Calanus finmarchicus (Tande, 1991; Slagstad et al., 1999) and modeled estimates suggest that about 1.5 million t C year–1 of C. finmarchicus leave the shelf off northern Norway. One branch enters the southern and central Barents Sea while the main flux is directed along the eastern Fram Strait and the slope and shelf of western Svalbard (Gluchowska et al., 2017; Figure 1). North of Svalbard, Atlantic Water and its mesozooplankton biomass is diverted into two branches (Basedow et al., 2018). One transports mesozooplankton biomass toward the western Fram Strait, Greenland, and partly back toward the Norwegian Sea. The other branch moves mesozooplankton biomass eastward along the shelf of northern Svalbard (Basedow et al., 2018) and along the central AO slope toward the Lomonosov Ridge (Hirche and Kosobokova, 2007; Figure 1). The transport is part of the Atlantic contiguous advective domain (Wassmann et al., 2015; Hunt et al., 2016) that connects the boreal zone off the Lofoten/Vesterålen region with the European Arctic Corridor (northeastern Greenland to the Eastern Kara Sea), comprising the hydrographically most active sector of the central AO (Rudels et al., 2004; Polyakov et al., 2017). The Atlantic contiguous advective domain is joined by the Arctic contiguous advective domain east of Franz Josefs Land (transporting C. glacialis from the northern Barents Sea) and from there, both domains carry on in concert toward the origin of the Lomonosov Ridge (Figure 2).
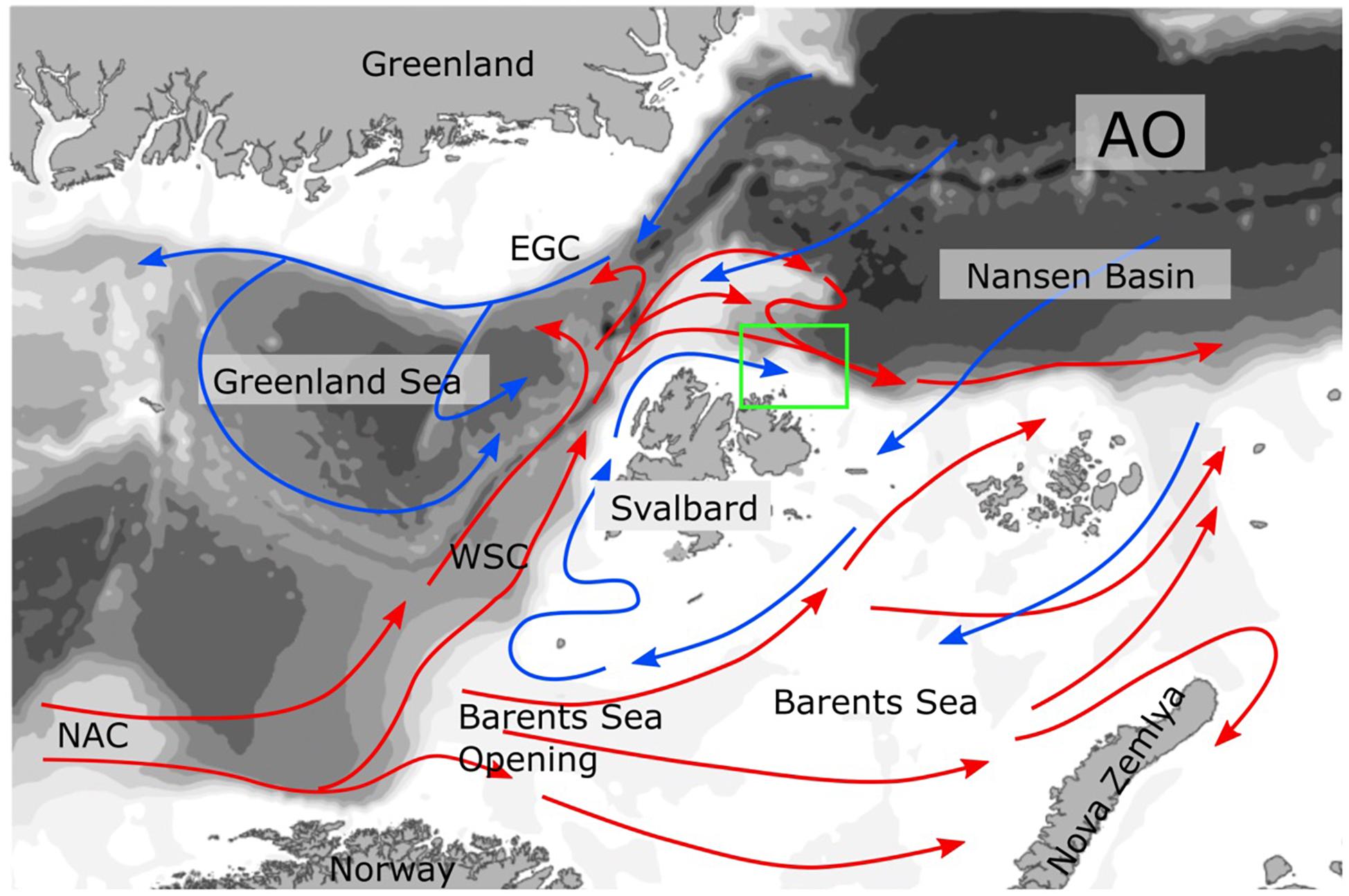
Figure 1. Currents in the Northern Norway, Fram Strait, Barents Sea, and Arctic Ocean (AO) region. The warm currents dominated by Atlantic Water are marked in read, for example the North Atlantic Current (NAC), and West Spitsbergen Current (WSC). The cold Arctic Water currents are in blue, for example the East Greenland Current (EGC). In the Lofoten/Vesterålen region the NAC touches the North Norwegian shelf and forms a strong slope current.
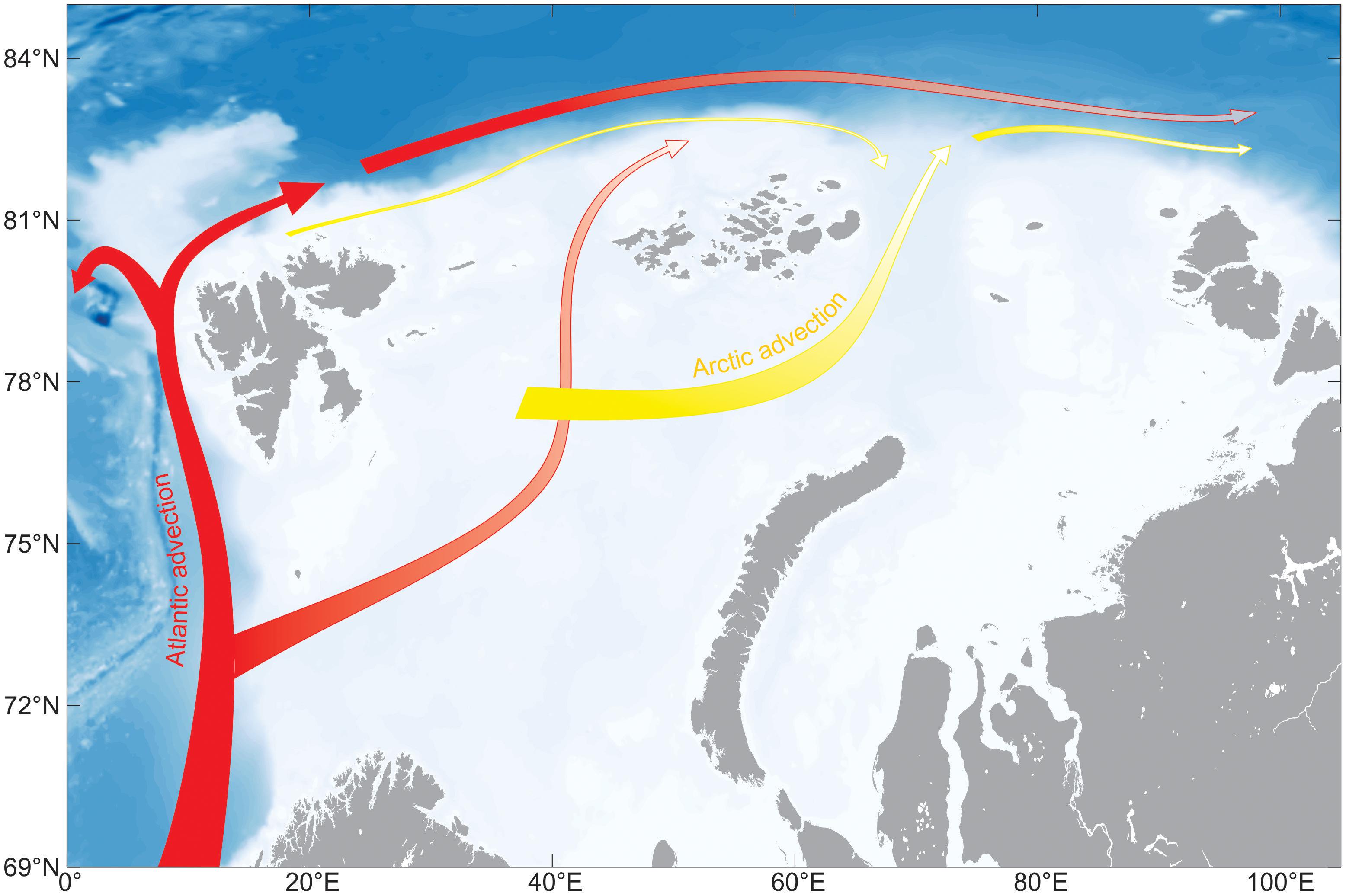
Figure 2. The upper layer advective domain of the European sector of the Arctic Ocean. The Atlantic (red) and Arctic (yellow) advective domains are shown.
However, because this transport has not yet been adequately quantified based on observational data (but see Basedow et al., 2018), mesozooplankton contribution to the AO is poorly defined (Wassmann et al., 2015), and future changes in Arctic zooplankton communities are difficult to appraise, let alone to observe and project. We applied the coupled physical-chemical-biological -models system SINMOD (Slagstad and McClimans, 2005; Wassmann et al., 2006) to investigate the transport of the Atlantic C. finmarchicus toward and along the northern shelf of Svalbard (Slagstad et al., 2011, 2015). In addition, we investigated the transport of the Arctic copepod C. glacialis that dominates in the arctic waters east of the Svalbard Archipelago. C. glacialis is transported along the Svalbard shelf by cold water masses through the Svalbard Coastal Current toward northern Svalbard (Figure 2). We ask (a) how much mesozooplankton biomass is transported along the Northern Svalbard region, (b) to what extent this advective transport is connected to the Norwegian shelf, and (c) what are the consequences of advection of mesozooplankton for planktonic composition at the edge of the AO (Nansen Basin).
Materials and Methods
The model (SINMOD) applied in this work is a coupled hydrodynamic and ecological model system with a particle tracking module that takes current velocities from the hydrodynamic model. A comprehensive description of the physical and ecosystem and food web model is found in Slagstad and McClimans (2005) and Wassmann et al. (2006). A short description, including recent deviations, is given here, but more information can be found in references given. The hydrodynamic model is based on the primitive Navier–Stokes equations and is established on a z-grid (Slagstad and McClimans, 2005; Slagstad et al., 2015).
The model structure was created for the Barents Sea ecosystem. State variables include nitrate, ammonium, silicate, diatoms, autotrophic, flagellates, bacteria, hetertrophic nanoflagellates, microzooplankton and two mesozooplankters: the Atlantic Calanus finmarchicus and the artic C. glacialis. Parameter values were set for modeling the carbon flux in this region. SINMOD calculates Gross Primary Production (GPP), new production (NP), the f ratio (NP/GPP), and secondary production of two key mesozooplankton species. The secondary production is calculated from grazed phytoplankton, minus egestion, and respiration losses. For details of the biological model, see Wassmann et al. (2006). The model contains additional compartments for sinking detritus (fast and slow), dissolved organic carbon and the sediment. The model uses constant stoichiometry [C:N ratio equal 7.6 was used, average data from the Barents Sea (Reigstad et al., 2002)].
The particle tracking module advect particles using a Runge–Kutta 4th order computational scheme. Most of the Atlantic Water deeper than 500 m in the model domain (e.g., Norwegian Sea) was populated with particles. Advection of the particles started April 1st each year. These state variables are calculated as a product of water flux and concentration through the selected boundaries. The time step was 1800 s.
The model set-up encompassed the Nordic Seas (located north of Iceland and south of Svalbard), the central AO and the Eurasian shelf (see Slagstad et al., 2015) and uses a horizontal grid point distance of 20 km. The model has 25 vertical levels. The vertical level thickness increases from 5 to 10 m near the surface to 500 m below 100 m. A total of 8 tidal components were imposed by specifying these (elevation and currents) at the open boundaries. Data were taken from TPXO 7.1 model of global ocean tides1. The ERA INTERIM reanalysis (Dee et al., 2011)2 data (wind, sea level air pressure, air temperature, cloud cover, and humidity) were used to force SINMOD.
Data on freshwater discharges from rivers and land along the Norwegian coast and Svalbard were collected from simulations by the Norwegian Water Resources and Energy Directorate3. The simulations were performed using a version of the HBV-model in 1 km horizontal resolution (Beldring et al., 2003). For Arctic Rivers, data were obtained from R-ArcticNet (Vörösmarty et al., 1996, 1998) available through http://www.r-arcticnet.sr.unh.edu/v4.0/main.html.
Initial values of temperature and salinity were taken from World Ocean Circulation Experiment (WOCE) Global Data Resource Version 3.04 using a spin-up phase of 26 years prior to the start of the simulation in this work. A comprehensive description of the WOCE data system can be found in Lindstrom (2001).
The model calculates the transport of the two calanoid copepods into and out of as well as their production and respiration inside the box (Figure 1). In backtracking mode SINMOD also tried to approximate the drift pathways of C. finmarchicus type particles until April, the main reproductive period.
Results
The modeled biomass of C. finmarchicus and C. glacialis for the months June and September showed widely different distribution and advection patterns for the two species (Figure 3). In June C. finmarchicus had recruited a large biomass in the eastern Norwegian Sea and north of the Norwegian shelf while late arrivers of the advected local C. finmarchicus are found west and north of Svalbard (Figure 3, upper left). In September the advection of C. finmarchicus from northern Norway to the Barents Sea and further along Svalbard to the AO was clearly detectable, stretching as far as to the Franz-Josefs-Land archipelago (Figure 3, lower left). The core population of C. glacialis is found in the north-eastern Barents Sea, Kara Sea, and Fram Strait. As compared to June C. glacialis had increased its biomass significantly in September (Figure 3, right panel). The basic distribution patterns of both copepods suggest that they dominate in different regions. The maximum biomass concentrations of C. glacialis and C. finmarchicus were similar.
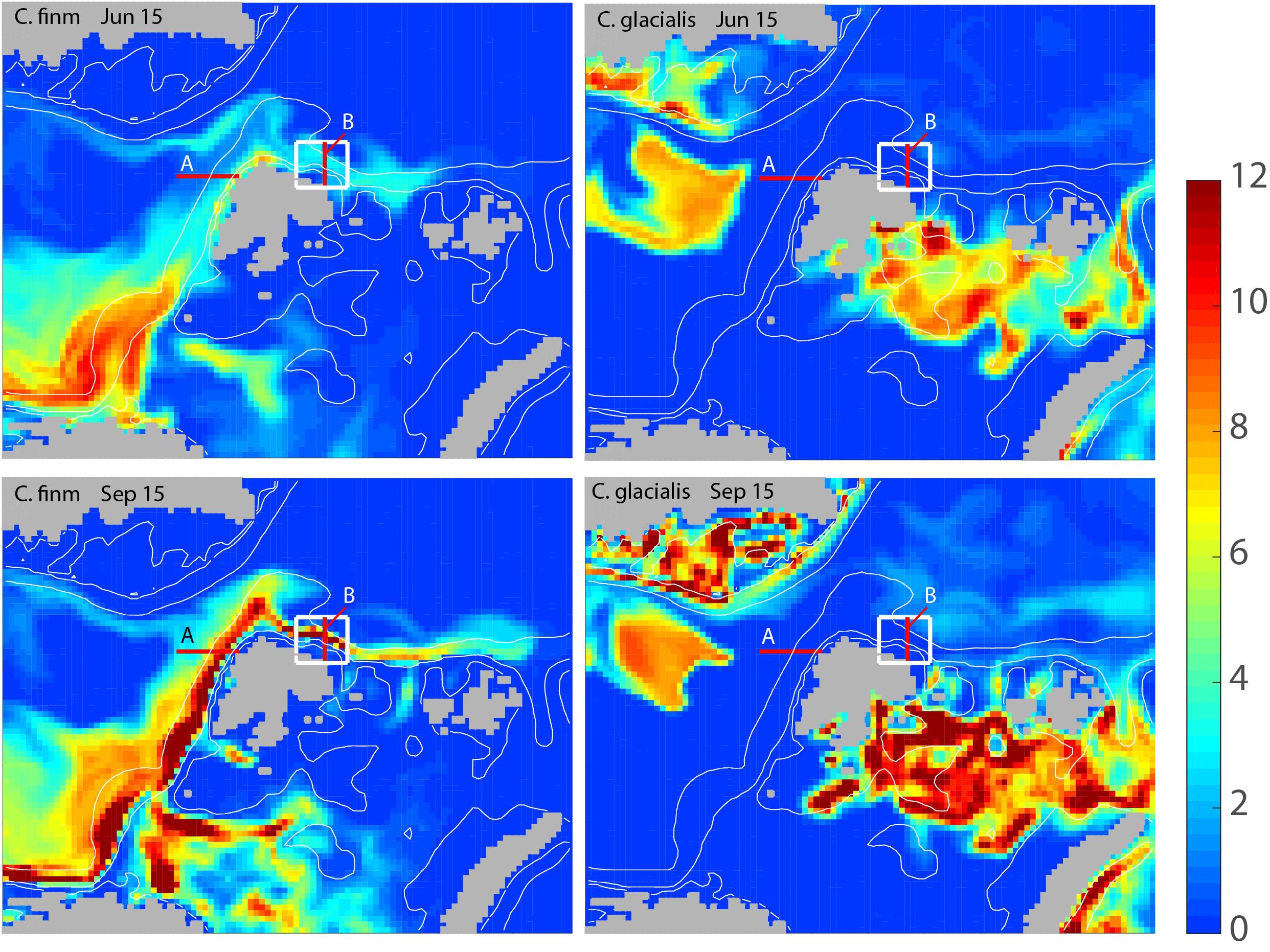
Figure 3. Modeled, integrated biomass of Calanus finmarchicus (left column) and Calanus glacialis (right column) for the months June (upper panel) and September (lower panel) of 2006 around Svalbard and adjacent arctic waters (g C month–2). Locations for sections A and B (red) and the box (white) north of Svalbard for which the advection of mesozooplankton is calculated are also shown.
The monthly flux of C. glacialis and C. finmarchicus over the years 2005 and 2014 was investigated across the western Svalbard shelf (Section A) and across the shelf and slope north of Svalbard (Sections B) (see Figure 3). For both species and sections obvious seasonal and interannual variability in flux were detected (Figure 4). The flux of C. finmarchicus across section A was about twice as high as across section B (Figure 4), suggesting that roughly half of the advected biomass was advected west and south in the northern Fram Strait. The flux of C. glacialis across section A was negligible (not shown) and that through section B must be based upon recruitment north of Svalbard and the adjacent AO. The flux of C. glacialis across section B was an order of magnitude lower than that of C. finmarchicus (Figure 4). The flux of C. glacialis and C. finmarchicus across section B and eastward into the interior of the AO was recurrent (Figure 4).
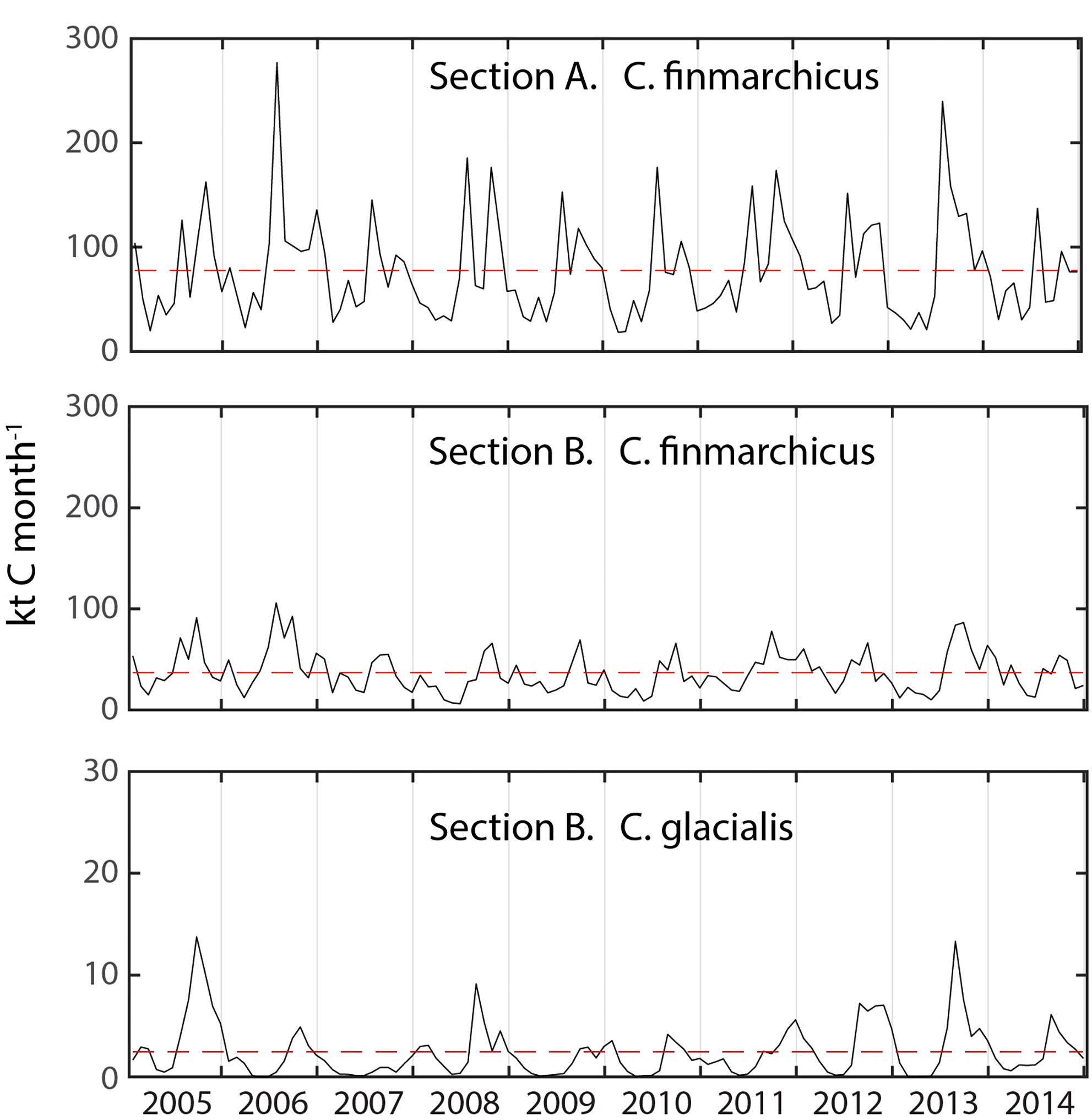
Figure 4. The monthly modeled flux (kt C month–1) of C. finmarchicus across sections A and B and C. glacialis through the section B from 2005 to 2015. Note scale differences and potential reversals of flux. Hatched time line shows the mean flux for the simulation period.
The biomass, flux, production and mortality of C. glacialis and C. finmarchicus were estimated for a box (25,600 million month–2) on the northern Svalbard shelf and slope (Figure 3 and Table 1). The average monthly biomass of C. finmarchicus over the period 2005–2014 declined from about 1.4 to 0.7 g C month–2 from January to May, increased 3–4 times from May to August (about 3 g C month–2) and declined again by May next year (Figure 5). The average biomass of C. glacialis over the period 2005–2014 was less than 0.3 g C month–2, was low in winter, increased in summer and peaked along with that of C. finmarchicus in August-September (Figure 5). There was a significant interannual variability in standing stock for both species, in particular for C. finmarchicus in summer (Figure 5).
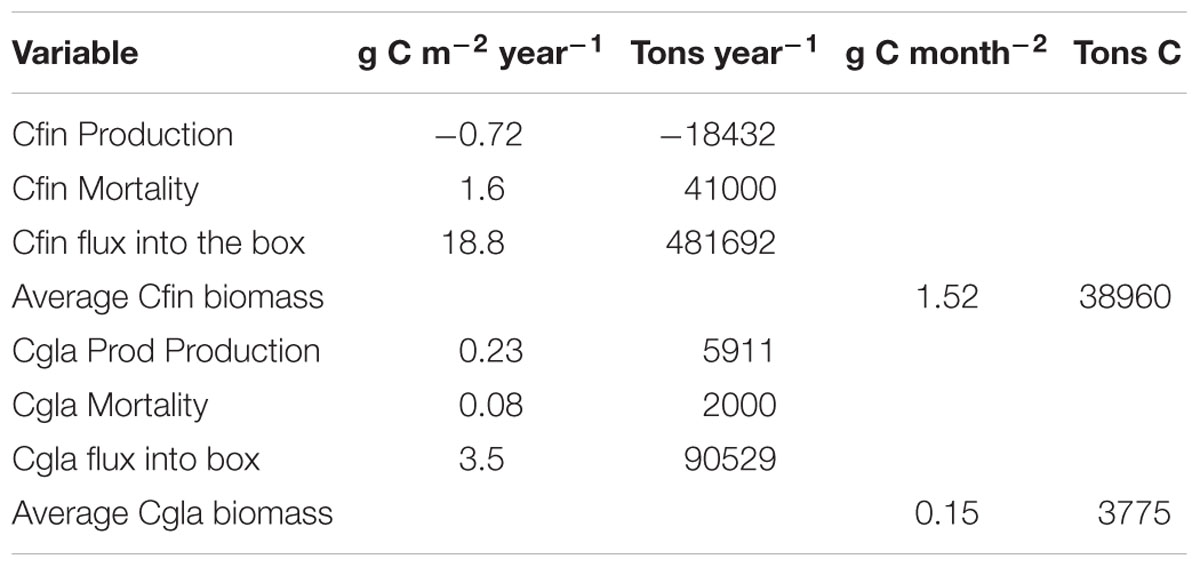
Table 1. Production (growth-respiration), mortality, influx and average biomass of Calanus finmarchicus (Cfin) and Calanus glacialis (Cgla) in/into the box north of Svalbard (see Figure 3). The box area is 25,600 million month–2.
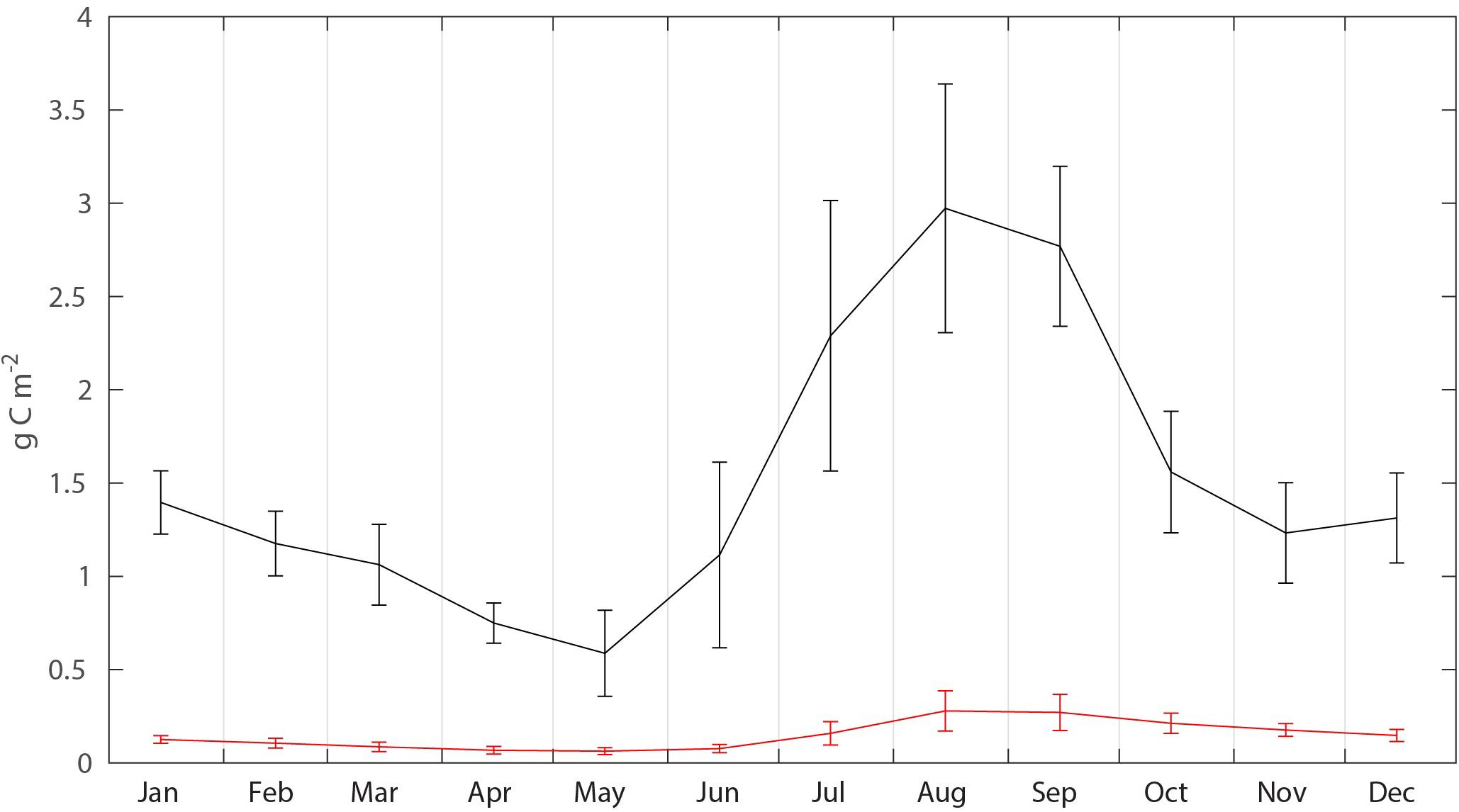
Figure 5. Average seasonal (2005–2015) C. finmarchicus (black) and C. glacialis (red) biomass (g C month–2) in the box, with monthly standard deviations.
The average annual biomass of C. finmarchicus and C. glacialis inside the box for the selected period was 38,960 and 3,775 (t C), respectively. Mesozooplankton biomass north of Svalbard is thus dominated by C. finmarchicus of North Atlantic origin. The average annual flux into the box of C. finmarchicus and C. glacialis in the period 2005–2014 was 18.8 and 3.6 g C month–2, respectively. This indicates that significant amounts of C. finmarchicus biomass are advected into the region while there is net export of C. glacialis. The average production (growth – respiration) of C. finmarchicus inside the box was negative: −0.72 g C month–2 year–1. However, average production of C. glacialis inside the box was positive: 0.23 g C month–2 year–1, contributing to a net export from the box. A similar picture was provided for mortality. Inside the box it was 27,620 and 3,775 t C year–1 for C. finmarchicus and C. glacialis, respectively.
The flux of C. finmarchicus dominated the annual budget for import and export of the two species in and out of the box (Figure 6). Most of the import of C. finmarchicus came with the Atlantic Water from the west, with 87% (419,360 t C) being exported annually to the east. Most of the C. glacialis import was from the north (Figure 6), although biomass came from the west and south. All export (94,600 t C) was to the east. The export is bigger than then import, caused by production inside the box.
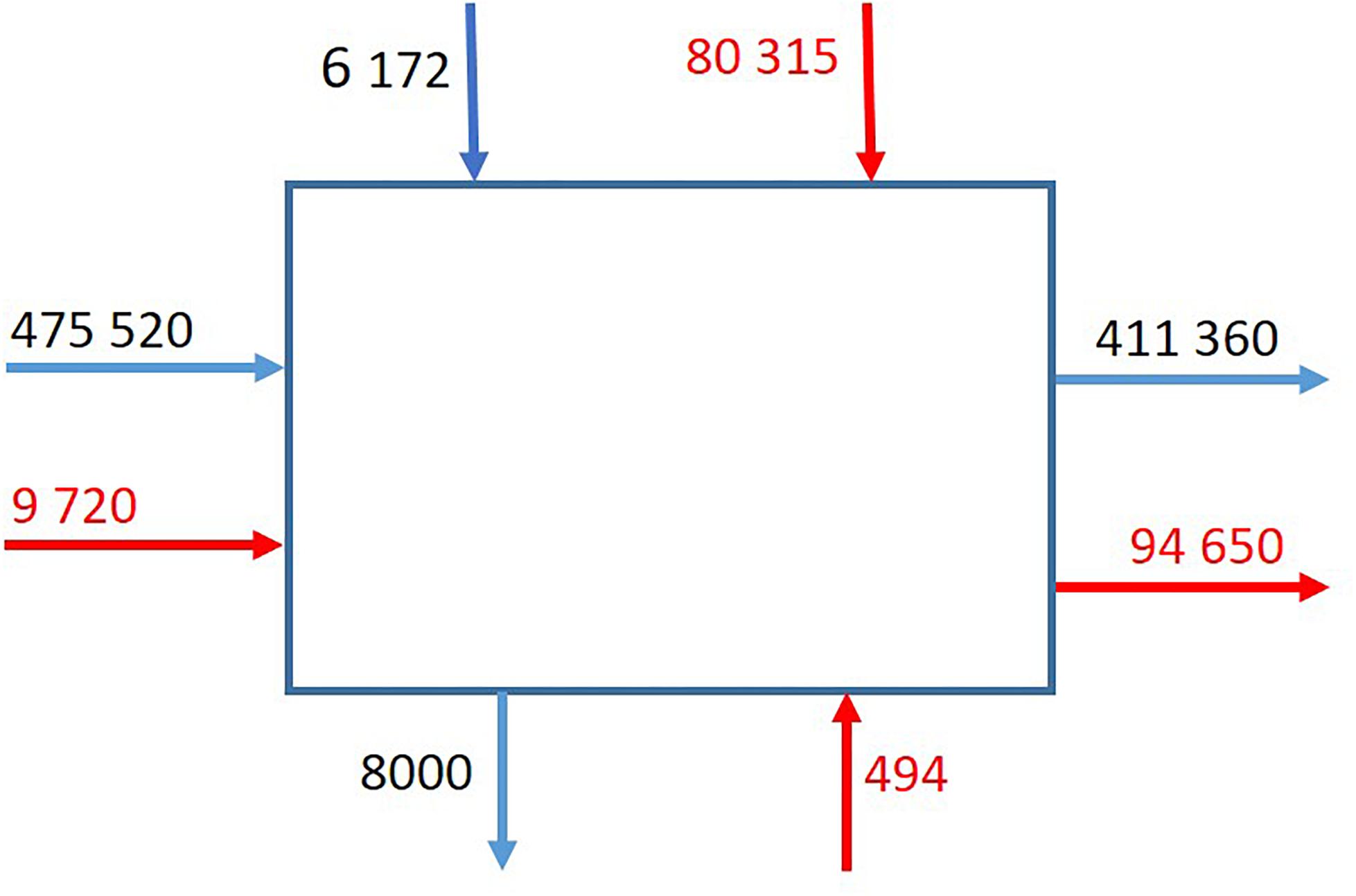
Figure 6. The average annual flux (t C) of C. finmarchicus (red) and C. glacialis (black) into the box from the west, north and south, and out toward to the east.
The model in backtracking mode was applied to identify where mesozooplankton-sized particles in September originated from in spring (April). The particles derived from the shelf break of western Svalbard, the Barents Sea shelf break toward the Norwegian Sea and the north Norwegian shelf (Figure 7). Zooplankton-type particles north of Svalbard (in September) derived from variable recruitment regions in spring (Figure 8).
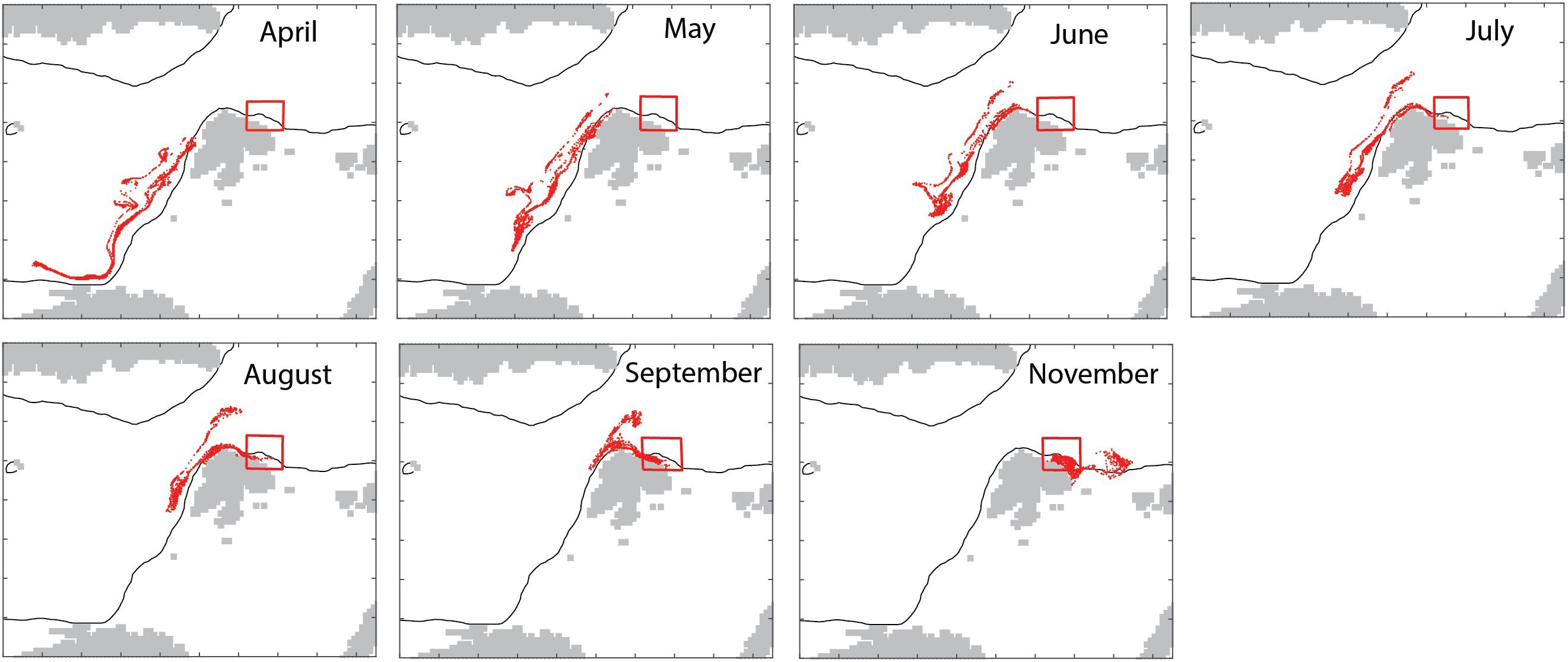
Figure 7. Backtracking to April (15/4, upper left) of particles located in the box (shown) in September 1999 and forward tracking of those particles into November 1999 (bottom right).
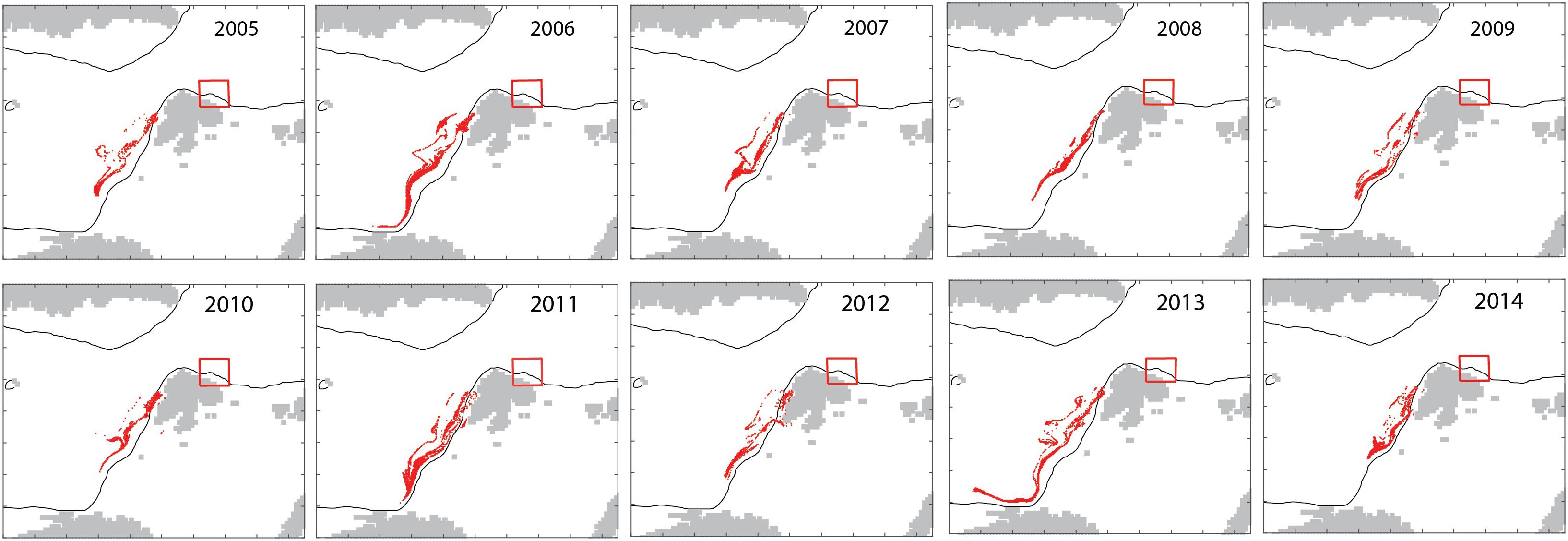
Figure 8. Interannual variability in the April 15 locations (red dots) of mesozooplankton particles that are located north of Svalbard (red box) on October 15 for each year 2005–2014.
Discussion
The advection of Atlantic Water along the Norwegian shelf and into the Barents Sea and adjacent AO is one of the most significant features of the European Arctic Corridor were by far the greatest water exchanges into and out of the AO take place (e.g., Smedsrud et al., 2013; Polyakov et al., 2017). In the Atlantic Water a considerable amount of nutrients and biogenic matter is advected into the AO (Popova et al., 2013). Biogenic C advection may be 5–50 times bigger than local primary production along the advective pathway. Along with this advection, large amount of mesozooplankton enter the AO ecosystem that partly depends upon additional food supply. Being partly dependent upon food supply from subarctic regions of a quantification of mesozooplankton advection into the AO, its seasonal features and connection to the North Atlantic are essential to comprehend the regulation of the biota and the dynamics of the AO ecosystem.
Mesozooplankton Transport Along the Northern Svalbard Region
Large amounts of mesozooplankton, for the most part C. finmarchicus, are transported along with Atlantic Water from the Norwegian Sea, along northern Norway and the western Fram Strait (Basedow et al., 2018). In the vicinity of the north-western Svalbard region, some of the Atlantic Water is recirculated toward Greenland, so that about half of the advected zooplankton biomass may be retained inside the northern Fram Strait region while the remaining biomass is advected on and along the continental slope of the Nansen Basin toward the Lomonosov Ridge (Hirche and Kosobokova, 2007; Bluhm et al., 2015). This biomass transport is dominated by C. finmarchicus north of Spitsbergen (Figure 3A), but toward the east more and more of the mesozooplankton biomass becomes dominated by the arctic species C. glacialis. This is particularly the case east of the St. Anna Trough (the largest submarine valley of the AO, between the Franz-Josefs-Land archipelago and Novaya Zemlya) where much of the C. glacialis production in the northern Barents Sea (Figure 3B) was advected eastward into the AO (see Figure 2). The mesozooplankton biomass north of Svalbard was lowest in May and highest in August and September (Figure 5). The model projected significant variability between years, reflecting both variable reproduction and assumed variation in recirculation in the northern Fram Strait. The seasonal and annual variability of mesozooplankton transport through section A in the eastern Fram Strait was not directly translated to the eastward transport along northern Svalbard through section B (Figure 4). The amplitude along section B decreased and not all maxima through section A were found in sector B. In general, recirculation in the Fram Straight is inadequately known (e.g., Marnela et al., 2013; Hattermann et al., 2016; Wekerle et al., 2017). Thus, also the advection of mesozooplankton into the Fram Strait and, particularly, how much of this biomass enters the adjacent AO is challenging. The application of a model seemed a fruitful first step before more precise estimates may be provided. According to the SINMOD model about 50% of the advected mesozooplankton biomass through sector A of the eastern Fram Strait entered the central AO through section B (Figure 4). The model further suggests that the flux of C. glacialis trough section B reflected the low local production on the north-western Svalbard shelf with its strong Atlantic Water inflow.
On average, about 480,000 t C of C. finmarchicus were transported into of the box from the east and north per year, respectively (Figure 6). The inflow of C. finmarchicus with Atlantic Water to northern Svalbard estimated from Laser Optical Plankton Counter and Multinett samples (Basedow et al., 2018) was on the order of 500,000 t C year–1, which compares well to our modeled estimates. This considerable biomass will support ecosystems along the European shelf edge to the AO. It implies that Atlantic mesozooplankton, advected from outside the AO, plays a significant role as an allochthonous food source for polar marine ecosystems from the Eurasian shelf break. The strength of this supply decreases toward the Lomonosov Ridge, while advection of arctic mesozooplankton from the northern Barents Sea and local production plays an increasingly important role along the Eurasian shelf (Hirche and Kosobokova, 2007). The advection of C. finmarchicus into the Eurasian AO shelves is unidirectional, since C. finmarchicus cannot reproduce successfully and the advection has been characterized as a death trail (Wassmann et al., 2015). Once C. finmarchicus enters the AO they persist until grazed or die. Because of the high advected biomass the grazing capacity of the expatriated mesozooplankton population will match the reduced primary production toward the second part of the annual cycle, diminishing the likelihood of algal blooms. However, climate change and the loss of sea ice (Overland and Wang, 2013; Onarheim et al., 2014) will support significant future increases in primary production along the Eurasian shelves (Wassmann et al., 2010; Slagstad et al., 2011; Ivanov et al., 2012) and more allochthonous zooplankton production may be expected. This scenario is true for both C. finmarchicus [caused by increased Atlantification (warming and increased primary production), Polyakov et al., 2017] and C. glacialis (increased primary and secondary production, Slagstad et al., 2015).
Advective Zooplankton Transport North of Svalbard Is Connected to the Norwegian Shelf
The simulated seasonal variability of mesozooplankton north of Svalbard showed a wide maximum in August and September, i.e., several months after the spring bloom. As C. finmarchicus reproduces in spring the cause must be allochthonous. To figure out the source of the advected mesozooplankton we applied the model in backtracking mode to identify where mesozooplankton-sized particles in September originated from in spring (April). The results indicated that the particles derived from the shelf break of western Svalbard, the Barents Sea opening (Figure 7). April is an important month for the spawning and recruitment of C. finmarchicus and the new recruits from these regions have drifted to northern Svalbard by early autumn. This finding demonstrates that northern Svalbard and the Eurasian rim of the AO are directly connected to the Norwegian Sea and the strong North Atlantic Current that follows the continental shelf northward. Static regions are linked to geography, but they may be linked by contiguous domains of shared function that facilitate material transports and share key ecological features. In general, such features are termed contiguous domains (Carmack and Wassmann, 2006). All contiguous domains have lengths of several thousand km and pass often through multiple biogeographic regions. Their components share (i) a common boundary or set of properties and (ii) are connected, over defined scales, in time and space. The Lofoten/Vesterålen region of northern Norway and the Eurasian rim of the AO thus form the Atlantic advective contiguous domain that supports the AO with nutrients, phytoplankton biomass and, particularly, long-lived zooplankton (Wassmann et al., 2015). As such, the northern Svalbard shelf is part of the Arctic inflow shelves which play an important role for the transfer of water masses of Atlantic origin (Smedsrud et al., 2013), with the nutrient availability and primary production (Randelhoff et al., 2018) as well as the advection of pelagic organisms (Wassmann et al., 2015). The Atlantic contiguous advective domain is not a constant, but rather dynamic feature that continuously exports and imports biogenic compounds and planktonic organism on its pathway toward the AO. The Atlantic advective contiguous domain may be one of the most dynamic features of the AO with significant biogeochemical cycling along its pathway.
The advection of mesozooplankton toward the northern Svalbard shelf region is an annually recurrent phenomenon. The model suggested that the influx the influx was characterized by significant annual variability (Figures 4, 5). The cause for the interannual variability is unknown. It appears to reflect variable recirculation patterns in the northern Fram Strait, but could also be caused by variable recruitment, advective supply from the North Norwegian shelf, influx into the Barents Sea and grazing along the advective pathway. Over the course of years, zooplankton-type particles north of Svalbard (in September) may derive from variable recruitment regions in spring and advection drives this variability. In some years, they may be derived from the boundary between the Barents Sea and the Norwegian Sea (2005, 2008, and 2012), while in other years (2006, 2011, and 2013), they may be derived from the region south-east of the Lofoten Islands (Figure 8). Together these regions form the > 3000 km long Atlantic advective contiguous domain through which advection crosses several biogeographic regions, light regimes and productive regions during the year. Certain sections of the sub-boreal, sub-arctic, and arctic regions can thus not be understood and managed separately, but must be considered as functional units of the contiguous domain. The central Norwegian Sea is considered the “home region” of C. finmarchicus and the recirculation in northern Fram Strait moves expatriates back into the source region of the species. Expatriates that enter the shelf of northern Svalbard are, however, lost for good and represent a food supply for the AO that is lost from the Atlantic Ocean.
Significance of Mesozooplankton Advection for the Pelagic
The increase in mesozooplankton biomass north of Svalbard in August and September reflects, for the most, growth and production of cohorts that spawned along the southern section of the Atlantic contiguous advective domain. This cohort commences in spring and is particularly strong in the Lofoten/Vesterålen region. While the cohort from the previous year is exported or lost from the region of northern Svalbard, as reflected declining biomass (October to May, Figure 5) in spring a new cohort is advected northward, arriving there for full in August and September. Despite these cohorts, Figure 5. Suggests that the advective supply of mesozooplankton to the northern Svalbard shelf is continuous as verified by Basedow et al. (2018). This advection of zooplankton fuels pelagic life, also during winter (Daase et al., 2014; Falk-Petersen et al., 2014; Berge et al., 2015; Blachowiak-Samolyk et al., 2015). The Atlantic contiguous advective domain is thus characterized by pulsing C. finmarchicus cohorts. The advent of the spring pulse has a strong grazing impact on the phytoplankton bloom north of Svalbard that takes place in late summer. Also, along this pulse (for details of the highly variable biogeochemical processes along the drift) the grazing impact is continuously strong. The grazing impact in the southern section of the Atlantic contiguous advective domain was reported to be so strong that diatoms blooms do not occur, despite strong silicate depletion that suggests a diatom production rate of close to 100 g C month–2 (Ratkova et al., 1999; Slagstad et al., 1999). Consequently, chlorophyll concentrations may stay low throughout the productive season along the North-Norwegian shelf break and toward northern Svalbard (Wassmann et al., 1999). The spring bloom north of Svalbard takes place in May/June, i.e., when the model predicted that the mesozooplankton biomass was lowest, consistent with the findings of Søreide et al. (2010). This suggests that a potential for strong pelagic-benthic coupling and strong benthic production exists in the early stages of the productive season. The post bloom period north of Svalbard is thus characterized by heavy grazing, executed by the new approaching mesozooplankton cohorts and thus chlorophyll concentrations stay low. The Arctic contiguous advective domain, dominated for the most of C. glacialis, appears to be less pulsed and more continuous (C. glacialis has a 2- to 3-year life cycle, Kosobokova, 1999), with the population of C. glacialis in the northern Barents and Kara seas as the main source.
Annually 18.8 g C month–2 of advected (and locally produced) mesozooplankton could be available for mesozooplankton predators before advected out again, implying that far greater amounts of feed are available along the Atlantic contiguous advective domain than outside the domain. Zooplankton predators inside the box experience, on average, 1.4 g C month–2 of prey biomass during the year. However, the advection of mesozooplankton is about 12 times bigger than the average concentration, implying that mesozooplankton in the Atlantic advective contiguous domain represents an important food source. It could represent a continuous replenished food source for pelagic fish, birds and marine mammals. Feeding in a stream of food or utilizing accumulation of feed in regions where eddies are prominent is a well-known phenomenon in coastal regions of northern Alaska. Here about 1/3 of the zooplankton biomass advected through Bering Strait seem to be grazed by bowhead whales see, calculations in Wassmann et al. (2015) and Moore et al. (2018). Similar scenarios may also exist north of Svalbard, but the feeding impact of marine mammals there is not yet available. For the increasing population of young cod recently reported from the region (e.g., Fossheim et al., 2015), the feeding conditions north of Svalbard are good, if not excellent. Their availability is not so much based upon the feed concentration, but on the flux of feed through the area that consistently replenishes the feeding concentration. Along advective contiguous domains feeding on the flux of advected food is thus an excellent feeding strategy. Thus, large amounts of mesozooplankton prey may sustain fish, birds and marine mammals at the western Eurasian perimeter of the AO basins.
Outlook
The advection of Atlantic Water, including its nutrients, phyto- and zooplankton, plays a significant role for the ecosystem function of the AO that needs obviously dedicated investigations. The SINMOD model projects advection mesozooplankton biomass that contributes to the heterotrophic nature of the Eurasian rim of the AO. However, the model has shortcomings that should be kept in mind. Its hydrodynamic model has a set-up using 20 km horizontal grid point distance. This a rather course resolution in the complex hydrographical region of the Fram Strait. The horizontal (and vertical) resolution is probably the most important factor for improving the model’s ability to improve the flow field. Further, the mortality of the mesozooplankton is difficult to assess. In lack of other alternatives, we assumed this parameter as a constant, but it will certainly vary through the season, with the variable presence of pelagic feeders (such as young cod) and from year to year.
The principle situation along the European Arctic Corridor is not unique. On the opposite Pacific side of the AO, a similar advective contiguous domain is found (Wassmann et al., 2015). Moore et al. (2018) described how zooplankton-rich water of Pacific origin flows through Bering Strait in the southern Chuckie Sea and along the shelf of northern Alaska, supporting the good feeding condition for whales. The pelagic-benthic coupling in the region is tied to the Pacific through flow because of continuous feed supply from the south. The “Arctic Pulses” model of Moore et al. (2018) and the “Advective” model of Grebmeier et al. (2015) for the Pacific opening of the AO have been launched. The results of the present investigation also illustrate the significance of the advective contiguous domain concept for the productivity, phenology, and food supply of the perimeter of the central AO. As climate change causes changes in the atlantification (Polyakov et al., 2005) and “pacification,” major changes in the food availability along the AO shelf breaks may be expected. Similar scenarios for food supply for pelagic carnivores, birds and marine mammals seem to exist for Arctic inflow shelves. However, the comparatively shallow environments on the Pacific side results in larger share of the food consumed by benthos.
Author Contributions
PW initiated the research, interpreted the results, and wrote the manuscript. DS and IE stood for the modeling work.
Conflict of Interest Statement
The authors declare that the research was conducted in the absence of any commercial or financial relationships that could be construed as a potential conflict of interest.
Funding
This publication was a part of the CARBONBRIDGE project, funded by the Norwegian Research Council (No. 226415). This publication was also a contribution to the project ARCTOS LoVe Marine Eco, supported by Equinor and ARCEx (No. 228107), and the research group Arctic SIZE (http://site.uit.no/arcticsize/).
Footnotes
- ^ http://www.coas.oregonstate.edu/research/po/research/tide/global.html
- ^ www.ecmwf.int
- ^ www.nve.no
- ^ http://www.nodc.noaa.gov
References
Basedow, S. L., Sundfjord, A., von Appen, W.-J., Halvorsen, E., Kwasniewski, S., and Reigstad, M. (2018). Seasonal variation in transport of zooplankton into the arctic basin through the atlantic gateway, fram strait. Front. Mar. Sci. 5:194. doi: 10.3389/fmars.2018.00194
Beldring, S., Engeland, K., Roald, L. A., Sælthun, N. R., and Voksø, A. (2003). Estimation of parameters in a distributed precipitation-runoff model for Norway. Hydrol. Earth Syst. Sci. 7, 304–316. doi: 10.5194/hess-7-304-2003
Berge, J., Daase, M., Renaud, P. E., Ambrose, W. G., Darnis, G., Last, K. S., et al. (2015). Unexpected levels of biological activity during the polar night offer new perspectives on a warming Arctic. Curr. Biol. 25, 2555–2561. doi: 10.1016/j.cub.2015.08.024
Blachowiak-Samolyk, K., Wiktor, J. M., Hegseth, E. N., Wold, A., Falk-Petersen, S., and Kubiszyn, A. M. (2015). Winter tales: the dark side of planktonic life. Polar. Biol. 38, 23–36. doi: 10.1007/s00300-014-1597-4
Bluhm, B., Kosobokova, K., and Carmack, E. (2015). A tale of two basins: an integrated physical and biological perspective of the deep Arctic Ocean. Prog. Oceanogr. 139, 89–121. doi: 10.1016/j.pocean.2015.07.011
Carmack, E., and Wassmann, P. (2006). Food webs and physical-biological coupling on pan-arctic shelves: comprehensive perspectives, unifying concepts and future research. Prog. Oceanogr. 71, 446–477. doi: 10.1016/j.pocean.2006.10.004
Daase, M., and Varpe, Ø, and Falk-Petersen, S. (2014). Non-consumptive mortality in copepods: occurrence of Calanus spp. carcasses in the Arctic Ocean during winter. J. Plankton Res. 36, 129–144. doi: 10.1093/plankt/fbt079
Dee, D. P., Uppala, S. M., Simmons, A. J., Berrisford, P., Poli, P., Kobayashi, S., et al. (2011). The ERA-Interim reanalysis: configuration and performance of the data assimilation system. Q. J. Royal Met. Soc. 137, 553–597.
Falk-Petersen, S., Pavlov, V., Berge, J., Cottier, F., Kovacs, K. M., and Lydersen, C. (2014). At the rainbow’s end: high productivity fueled by winter upwelling along an Arctic shelf. Polar Biol. 38, 5–11. doi: 10.1007/s00300-014-1482-1
Fossheim, M., Primicerio, R., Johannesen, E., Ingvaldsen, R. B., Aschan, M. M., and Dolgov, A. V. (2015). Recent warming leads to a rapid borealization of fish communities in the Arctic. Nat. Clim. Change 5, 673–677. doi: 10.1038/nclimate2647
Gluchowska, M., Dalpadado, P., Beszczynska-Möller, A., Olszewska, A., Ingvaldsen, R. B., and Kwasniewski, S. (2017). Interannual zooplankton variability in the main pathways of the Atlantic water flow into the Arctic Ocean (Fram Strait and Barents Sea branches). ICES J. Mar. Sci. 74, 1921–1936. doi: 10.1093/icesjms/fsx033
Grebmeier, J. M., Bluhm, B. A., Cooper, L. W., Danielson, S. L., Arrigo, K. R., Blanchard, A. L., et al. (2015). Ecosystem characteristics and processes facilitating persistent macrobenthic biomass hotspots and associated benthivory in the Pacific Arctic. Prog. Oceanogr. 136, 92–114. doi: 10.1016/j.pocean.2015.05.006
Hattermann, T., Isachsen, P. E., Appen, W.-J. V., Albertsen, J., and Sundfjord, A. (2016). Eddy-driven recirculation of Atlantic Water in Fram Strait. Geophys. Res. Lett. 43, 3406–3414. doi: 10.1002/2016GL068323
Hirche, H.-J., and Kosobokova, K. (2007). Distribution of Calanus finmarchicus in the northern North Atlantic and Arctic Ocean—Expatriation and potential colonization. Deep-Sea Res. II 54, 2729–2747. doi: 10.1016/j.dsr2.2007.08.006
Hunt, G. L., Drinkwater, K. F., Arrigo, K., Berge, J., Daly, K. L., Danielson, S., et al. (2016). Advection in polar and sub-polar environments: impacts on high latitude marine ecosystems. Prog. Oceanogr. 149, 40–81. doi: 10.1016/j.pocean.2016.10.004
Ivanov, V., Alexeev, V. A., Repina, I., Koldunov, N. V., and Smirnov, A. (2012). Tracing Atlantic water signature in the Arctic sea ice cover east of Svalbard. Adv. Meteorol. 2012:201818. doi: 10.1155/2012/201818
Kosobokova, K. (1999). The reproductive cycle and life history of the Arctic copepod Calanus glacialis in the White Sea. Polar Biol. 22, 254–263. doi: 10.1007/s003000050418
Lindstrom, E. J. (2001). “Developing the WOCE Global Data System,” in Ocean Circulation and Climate - Observing and Modelling the Global Ocean, eds G. Siedler, J. Church, and J. Gould (Cambridge, MA: Academic Press), 181–190. doi: 10.1016/s0074-6142(01)80118-2
Marnela, M., Rudels, B., Houssais, M.-N., Beszczynska-Möller, A., and Eriksson, P. B. (2013). Recirculation in the Fram Strait and transports of water in and north of the Fram Strait derived from CTD data. Ocean Sci. 9, 499–519. doi: 10.5194/os-9-499-2013
Moore, S., Stabeno, P. J., Grebmeier, J. M., and Okkonen, S. R. (2018). The Arctic marine pulses model: linking annual oceanographic processes to contiguous ecological domains in the Pacific Arctic. Deep Sea Res. Part 2 Topic. Stud. Oceanogr. 152, 8–21. doi: 10.1016/j.dsr2.2016.10.011
Onarheim, I. H., Smedsrud, L. H., Ingvaldsen, R. B., and Nilsen, F. (2014). Loss of sea ice during winter north of Svalbard. Tellus A 66:23933. doi: 10.3402/tellusa.v66.23933
Overland, J. E., and Wang, M. (2013). When will the summer Arctic be nearly sea ice-free? Geophys. Res. Lett. 40, 2097–2101. doi: 10.1002/grl50316
Polyakov, I. V., Beszczynska, A., Carmack, E. C., Dmitrenko, I. A., Fahrbach, E., Frolov, I. V., et al. (2005). One more step toward a warmer Arctic. Geophys. Res. Lett. 32:L17605. doi: 10.1029/2005GL023740
Polyakov, I. V., Pnyushkov, A. V., Alkire, M. B., Ashik, I. M., Baumann, T. M., Carmack, E. M., et al. (2017). Greater role for Atlantic inflows on sea-ice loss in the Eurasian Basin of the Arctic Ocean. Science 356, 285–291. doi: 10.1126/science.aai8204
Popova, E. E., Yool, A., and Aksenov, Y. (2013). Role of advection in Arctic Ocean lower trophic dynamics. J.Geophys. Res. Oceans 118, 1571–1586. doi: 10.1002/jgrc.20126
Randelhoff, A., Reigstad, M., Chieric, I. M., Sundfjord, A., Ivanov, V., Cape, M., et al. (2018). Seasonality of the physical and biogeochemical hydrography in the inflow to the Arctic Ocean through Fram Strait. Front. Mar. Sci. 5:224. doi: 10.3389/fmars.2018.00224
Ratkova, T. N., Wassmann, P., Verity, P. G., and Andreassen, I. (1999). Abundance and biomass of pico-, nano- and microplankton along a transect on Nordvestbanken, north Norwegian shelf, in 1994. Sarsia 84, 213–226. doi: 10.1080/00364827.1999.10420427
Reigstad, M., Wexels Riser, C., Øygarden, S., Wassmann, P., and Rey, F. (2002). Variation in hydrography, nutrients and suspended biomass in the marginal ice zone and the central Barents Sea. J. Mar. Syst. 38, 9–29. doi: 10.1016/s0924-7963(02)00167-7
Rudels, B., Jones, P., Schauer, U., and Eriksson, P. (2004). Atlantic sources of the Arctic Ocean surface and halocline waters. Polar Res. 23, 181–208. doi: 10.3402/polar.v23i2.6278
Slagstad, D., Ellingsen, I. H., and Wassmann, P. (2011). Evaluating primary and secondary production in an Arctic Ocean void of summer sea ice: an experimental simulation approach. Progr. Ocean. 90, 117–131. doi: 10.1016/j.pocean.2011.02.009
Slagstad, D., and McClimans, T. A. (2005). Modeling the ecosystem dynamics of the Barents Sea including the marginal ice zone: I. Physical and chemical oceanography. J. Mar. Syst. 58, 1–18. doi: 10.1016/j.jmarsys.2005.05.005
Slagstad, D., Tande, K., and Wassmann, P. (1999). Modelled carbon fluxes as validated by field data on the north Norwegian shelf during the productive period in 1994. Sarsia 84, 303–317. doi: 10.1080/00364827.1999.10420434
Slagstad, D., Wassmann, P. F. J., and Ellingsen, I. (2015). Physical constrains and productivity in the future Arctic Ocean. Front. Mar. Sci. 2:85. doi: 10.3389/fmars.2015.00085
Smedsrud, L. H., Esau, I., Ingvaldsen, R. B., Eldevik, T., Haugan, P. M., Li, C., et al. (2013). The role of the Barents Sea in the Arctic climate system. Rev. Geophys. 51, 415–449. doi: 10.1002/rog.20017
Søreide, J. E., Leu, E., Berge, J., Graeve, M., and Falk-Petersen, S. (2010). Timing of blooms, algal food quality and Calanus glacialis reproduction and growth in a changing Arctic. Global Chem. Biol. 19, 3154–3163. doi: 10.1111/j.1365-2486.2010.02175.x
Tande, K. S. (1991). Calanus in North Norwegian fjords and in the Barents Sea. Polar Res. 10, 389–408. doi: 10.3402/polar.v10i2.6754
Vörösmarty, C. J., Fekete, B., and Tucker, B. A. (1996). River Discharge Database, Version 1.0 (RivDIS v1.0), Volumes 0 through 6. A Contribution to IHP-V Theme 1. Paris: UNESCO.
Vörösmarty, C. J., Fekete, B., and Tucker, B. A. (1998). River Discharge Database, Version 1.1 (RivDIS v1.0 Supplement). Durham, NH: Institute for the Study of Earth, Oceans, and Space and University of New Hampshire.
Wassmann, P., Andreassen, I., and Rey, F. (1999). Seasonal variation of nutrients and suspended biomass along a transect on Nordvestbanken, north Norwegian shelf, in 1994. Sarsia 84, 199–212. doi: 10.1080/00364827.1999.10420426
Wassmann, P., Carmack, E., Slagstad, D., Kosobokova, K., Drinkwater, K., Ellingsen, I., et al. (2015). The contiguous domains of Arctic Ocean advection: trails of life and death. Prog. Oceanogr. 139, 42–65. doi: 10.1016/j.pocean.2015.06.011
Wassmann, P., Slagstad, D., and Ellingsen, I. (2010). Primary production and climatic variability in the European sector of the Arctic Ocean prior to 2007: preliminary results. Polar Biol. 33, 1641–1650. doi: 10.1007/s00300-010-0839-3
Wassmann, P., Slagstad, D., Wexels Riser, C., and Reigstad, M. (2006). Modelling the ecosystem dynamics of the marginal ice zone and central Barents Sea. II. Carbon flux and interannual variability. J. Mar. Systems 59, 1–24. doi: 10.1016/j.jmarsys.2005.05.006
Keywords: Arctic Ocean, zooplankton, Calanus finmarchicus, advection, contiguous domains, harvestable production
Citation: Wassmann P, Slagstad D and Ellingsen I (2019) Advection of Mesozooplankton Into the Northern Svalbard Shelf Region. Front. Mar. Sci. 6:458. doi: 10.3389/fmars.2019.00458
Received: 04 December 2018; Accepted: 09 July 2019;
Published: 14 August 2019.
Edited by:
Maria Vernet, University of California, San Diego, United StatesReviewed by:
Carin Ashjian, Woods Hole Oceanographic Institution, United StatesAndrea Pinones, Universidad Austral de Chile, Chile
Robin Macurda Ross, University of California, Santa Barbara, United States
Copyright © 2019 Wassmann, Slagstad and Ellingsen. This is an open-access article distributed under the terms of the Creative Commons Attribution License (CC BY). The use, distribution or reproduction in other forums is permitted, provided the original author(s) and the copyright owner(s) are credited and that the original publication in this journal is cited, in accordance with accepted academic practice. No use, distribution or reproduction is permitted which does not comply with these terms.
*Correspondence: Paul Wassmann, cGF1bC53YXNzbWFubkB1aXQubm8=