- 1Department of Polar Biological Oceanography, Alfred Wegener Institute, Bremerhaven, Germany
- 2Geo- und Umweltwissenschaften, Eberhard Karls Universität Tübingen, Tübingen, Germany
- 3Marine Zoology, Bremen Marine Ecology, University of Bremen, Bremen, Germany
- 4Technische Informationsbibliothek, Hanover, Germany
- 5Institute of Oceanology, Polish Academy of Sciences, Sopot, Poland
Pelagic amphipods represent a large fraction of organisms entering sediment traps as so-called “swimmers.” These swimmers were sampled with sediment traps (∼200–300 m water depth) with two mooring arrays deployed at two different positions in the Long-Term Ecological Research observatory HAUSGARTEN in the northeastern Fram Strait. This sampling allowed us to investigate amphipod year-round abundances and inter-annual trends from 2000 onward. In this study, newly analyzed data from a 3-years period (August 2011–June 2014) are presented, extending this long-term investigation. In our results, the species Themisto abyssorum, T. libellula, and T. compressa dominated the swimmer biomass, corroborating previous studies. The observed increase of amphipod abundances persisted in all three species, additionally implying that Themisto compressa maintained its population off Svalbard, which appeared for the first time here after a warm anomaly in 2004–2007. This study provides evidence for changes in amphipod community patterns that can mainly be attributed to growing abundances of T. compressa. Similarly, another hyperiid, Lanceola clausii, also increased in abundance over the investigated period. For T. libellula, almost no juvenile individuals were recorded in the sampling period 2013/14, even though juveniles of this species were common in earlier records. The three more years of observations clearly suggest that recently documented environmental shifts persist in the eastern Fram Strait. They also highlight the merit of using sediment trap time series to obtain year-round data sets needed to reveal processes and range shift dynamics in the pelagic system on a long-term basis.
Introduction
The Arctic environment is in rapid transition and is severely impacted by climate change (Schiermeier, 2007; Beaugrand, 2009). The sea ice is thinning (Hansen et al., 2013; Renner et al., 2014; Krumpen et al., 2015), and its extent is shrinking, with predictions of nearly ice-free summers in the Arctic within the next 25 years (Nghiem et al., 2007; Wang and Overland, 2012; Liu et al., 2013). Decreasing sea ice in the high Arctic impacts the prevailing ice situation in the Fram Strait where sea ice, transported via the Transpolar Drift, leaves the Arctic Ocean. In addition, oceanographic surveys reveal an increase in warm water anomalies throughout the Arctic (Polyakov et al., 2005) that are largely transported via the Fram Strait. The Fram Strait is considered the main gateway to the Arctic Ocean: in its western part, the East Greenland Current transports polar water southward; in its eastern part, relatively warm water flows northward with the West Spitsbergen Current (WSC). Mixing, eddies, and recirculating water of the warm WSC add to the hydrographic complexity observed in the strait (e.g., Gascard et al., 1988; Walczowski, 2013; Von Appen et al., 2015). In the eastern part, both increasing water temperature and heat flux have been observed over the last two decades (Schauer et al., 2004, 2008; Piechura and Walczowski, 2009; Walczowski, 2013; Walczowski et al., 2017). Hence, the increasing influence of Atlantic waters in the Arctic domain, termed “Atlantification,” is extending its area of impact to a great extent through the eastern Fram Strait northward into the Arctic (Piechura and Walczowski, 2009; Beszczynska-Möller et al., 2012; Polyakov et al., 2017).
In general, alterations of environmental parameters have been shown to change plankton communities by impacting species distributions (e.g., Beaugrand, 2009) and life cycles/development (Weydmann et al., 2018). This is particularly true for the Arctic. The observed occasional warm water peaks in the eastern Fram Strait (Beszczynska-Möller et al., 2012) were accompanied by changes in phytoplankton biomass and particle flux (Bauerfeind et al., 2009; Nöthig et al., 2015; Soltwedel et al., 2016). Biogeographical shifts are also occurring in higher trophic levels, e.g., copepods in the WSC (Weydmann et al., 2014; Gluchowska et al., 2017), krill around Svalbard (Buchholz et al., 2010; Dalpadado et al., 2016), Atlantic cod in the western Greenland Sea (Christiansen et al., 2016), pteropods (Busch et al., 2015), and amphipods (Kraft et al., 2013; Dalpadado et al., 2016) in the Fram Strait. These changes consequently propagate through the Arctic food web, affecting predators such as seabirds and fish (Stempniewicz et al., 2007; Kwasniewski et al., 2012; Kortsch et al., 2015; Dalpadado et al., 2016).
Due to their abundance and their being a major prey for higher trophic levels, the three dominating pelagic amphipod species play a key role in the Arctic pelagic food web (Koszteyn et al., 1995; Dalpadado et al., 2001, 2008a,b; Auel and Werner, 2003; Melle et al., 2004). The three dominant amphipod species found in Arctic waters belong to the genus Themisto, namely the Arctic T. libellula, the Arctic-boreal T. abyssorum (both natives to the eastern Fram Strait), and the North Atlantic species T. compressa (Klekowski and Wȩsławski, 1991; Weigmann-Haass, 1997; Dalpadado et al., 2001; Dalpadado, 2002). Themisto abyssorum co-exists with T. libellula throughout the Arctic (Klekowski and Wȩsławski, 1991; Weigmann-Haass, 1997; Dalpadado et al., 2001; Dalpadado, 2002); however, T. abyssorum is thought to be more abundant in waters of Atlantic origin and therefore displays a greater tolerance of fluctuations in water temperature (Dalpadado, 2002). The distribution center of T. compressa is temperate, North Atlantic waters. It is seldom found, and only in low abundances, in the Arctic marginal seas such as the Barents Sea off Svalbard (Dalpadado, 2002) and the Greenland Sea (Weigmann-Haass, 1997) and was recorded for the first time in the eastern Fram Strait in 2004 (Kraft et al., 2013). After its establishment in the Fram Strait, abundances of T. compressa have been increasing between 2005 and 2008 (Kraft et al., 2011, 2013); this was attributed to a warm surface water anomaly in the area (Soltwedel et al., 2016).
As warmer waters of Atlantic origin have been entering the Arctic Ocean via the WSC during the last 20 years, the amphipod community composition was expected to change accordingly. Set up in 1999, the Long-Term Ecological Research (LTER) observatory HAUSGARTEN (79°N, 4°, Figure 1) has provided data to detect environmental and biological changes in the Fram Strait. Since pelagic amphipods are good indicators of the presence of distinct water masses and therefore suitable for monitoring the effect of environmental change, this study aimed to assess the temporal and spatial differences in amphipod composition in the gateway to the Arctic. We investigated swimmer time series from the LTER sediment traps during the years 2011–2014, including and building on time series previously analyzed by Kraft et al. (2011, 2013) to assess trends in the prevailing pelagic amphipod population.
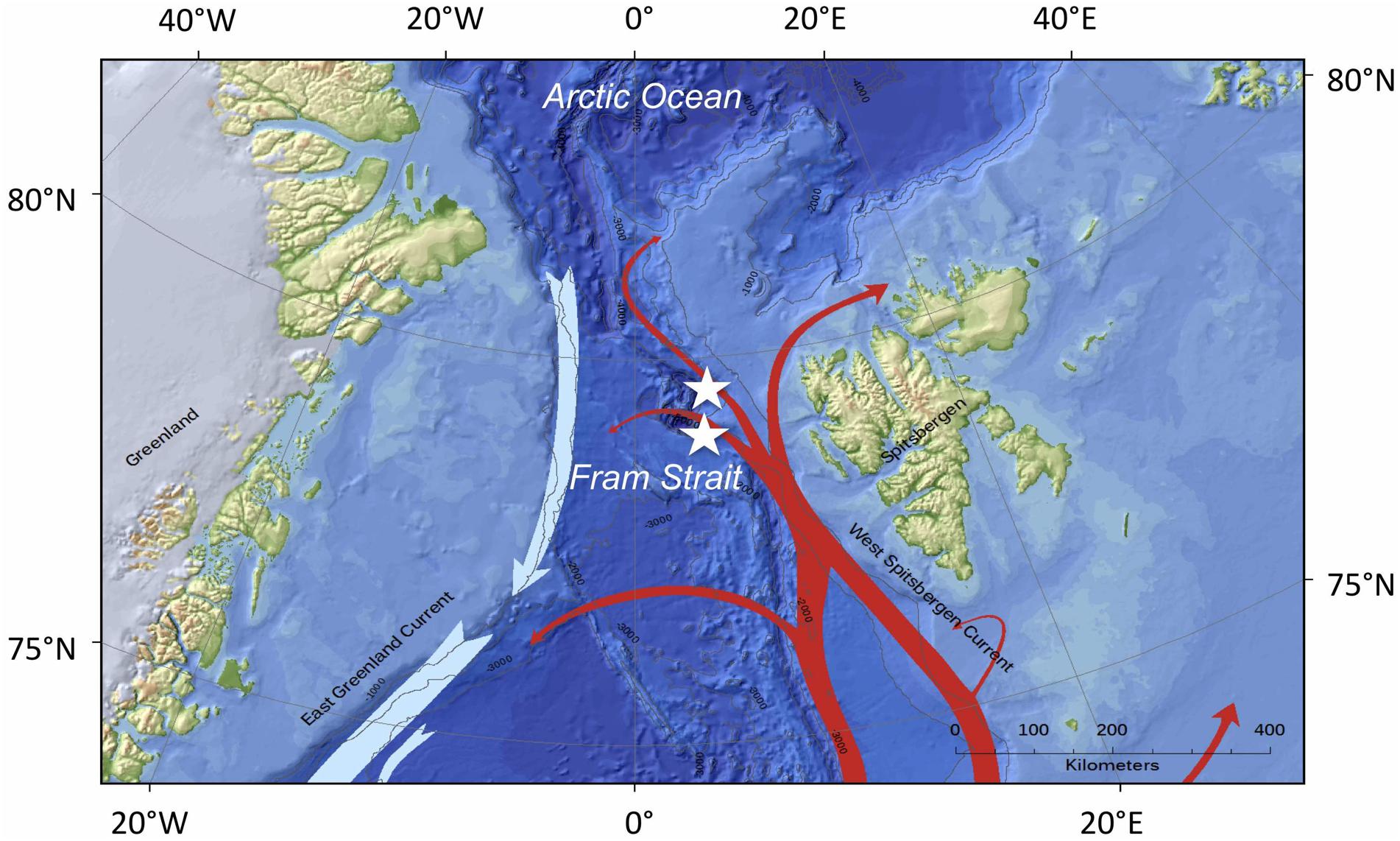
Figure 1. Schematic map of the marine circulation patterns in the Fram Strait. The West Spitsbergen Current is delineated by red arrows; the East Greenland Current is depicted by light blue arrows. The stars in the center indicate the two mooring positions, North (upper asterisk) and Central (lower asterisk), in the LTER observatory HAUSGARTEN. The map was created using ArcGIS 10.3 and based on the General Bathymetric Chart of the Oceans (GEBCO)-08 grid, version 20100927, http://www.gebco.net, with permission from the British Oceanographic Data Centre (BODC).
Materials and Methods
All samples were collected using automatic Kiel sediment traps (K/MT 234; K.U.M. Umwelt- und Meerestechnik Kiel GmbH) with an opening of 0.5 m2 and 20 collection cups. Two different localities were sampled over the period from September 2000 to July 2014 (Figure 1); sampling intervals lasted from summer to the following summer. The moorings were located at approximately 79°00′ N, 04°19′ E and 79°44′ N 04°30′ E. The sampling depth of the upper traps that were used for the present analysis were located between 190 and 280 m water depth. Traps were retrieved during 16 expeditions to the Arctic LTER observatory HAUSGARTEN located the eastern Fram Strait. Details of positions and traps for each year are found in Table 1. Collector cups of the sediment traps were filled with filtered, sterile North Sea water at an adjusted salinity of 40 psu and poisoned with HgCl2 (0.14% final solution). Automatic sampling was set to rotate to new collectors every 7–26 days during times of high primary and secondary production (May-September), with longer sampling intervals (up to 32 days) during other months (see Supplementary Table S1 for more details of sampling intervals).
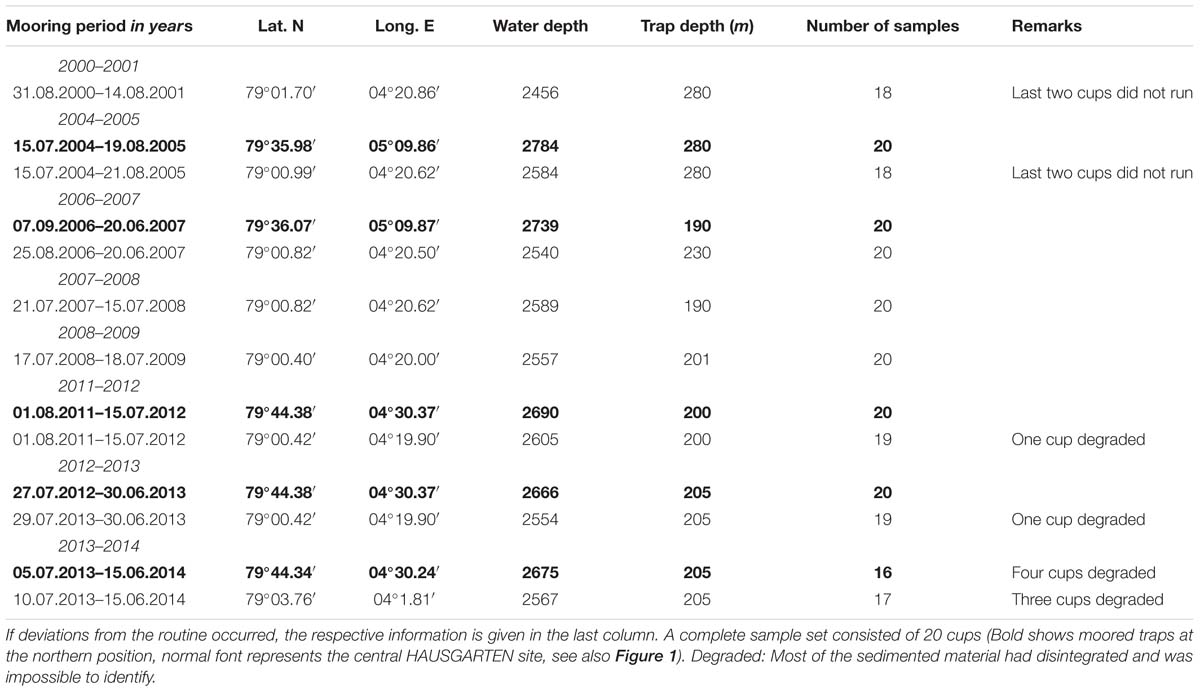
Table 1. Location, sampling time, water depth, and trap depth of moored sediment traps in the HAUSGARTEN and Greenland Sea analyzed for their amphipod composition with multivariate analyses.
Collected amphipods were removed and rinsed under a dissecting microscope (Olympus SZX10, magnification 20–50×). They were identified to species level and life stage and counted. For T. abyssorum and T. compressa, specimens <8 mm were considered as juveniles, whereas for T. libellula, the range <11 mm was chosen (lower size-limit: 2 mm, respectively), based on Kraft et al. (2011). In addition, numbers of Lanceola clausii were evaluated, because it was the most abundant amphipod after the three Themisto species.
We observed erratic peaks in amphipod abundances, especially in T. abyssorum (see also results Figure 3), suggesting an underestimation of individuals in some of the samples. Reasons for this are discussed below.
Incorporating data obtained in the study period 2000–2009, multivariate analysis was carried out using PRIMER 6 (Plymouth Marine Laboratory, United Kingdom) (Clarke and Gorley, 2005) to visualize the similarity between the abundances of the different Themisto species obtained in every sample for the different sampling periods and sites. The averaged amphipod flux data (including rare species) were standardized and square root transformed to avoid large values (abundant species) overwhelming the analysis. Nevertheless, the rare species only marginally contributed to the clustering. Thereafter, similarities between the groups of sample sets were checked. Comparing these sample sets, a resemblance matrix was generated applying the Bray-Curtis measure, because this measure was shown to apply best to marine data (Field et al., 1982). Based on the resemblance matrix, a multidimensional scaling (MDS) plot was generated for the established spatial and temporal criteria (Field et al., 1982; Clarke and Gorley, 2005). Similarity Percentage (SIMPER) routines were applied to highlight the species mainly responsible for the differences between the sample clusters (Clarke and Gorley, 2005).
Results
Amphipod Swimmer Composition in Traps 2011–2014
Analyzing six sediment traps of three consecutive years, a total of 10,906 specimens comprising seven different amphipod species were found in the traps – in order of decreasing abundance: T. abyssorum, T. libellula, T. compressa (Figure 2), L. clausii, Eusirus holmii, Hyperia medusarum, and Gammarus wilkitzkii, representing four different families (Hyperiidae, Lanceolidae, Eusiridae, and Gammaridae) (see Supplementary Table S1 for all and Supplementary Table S2 for rare species abundances).
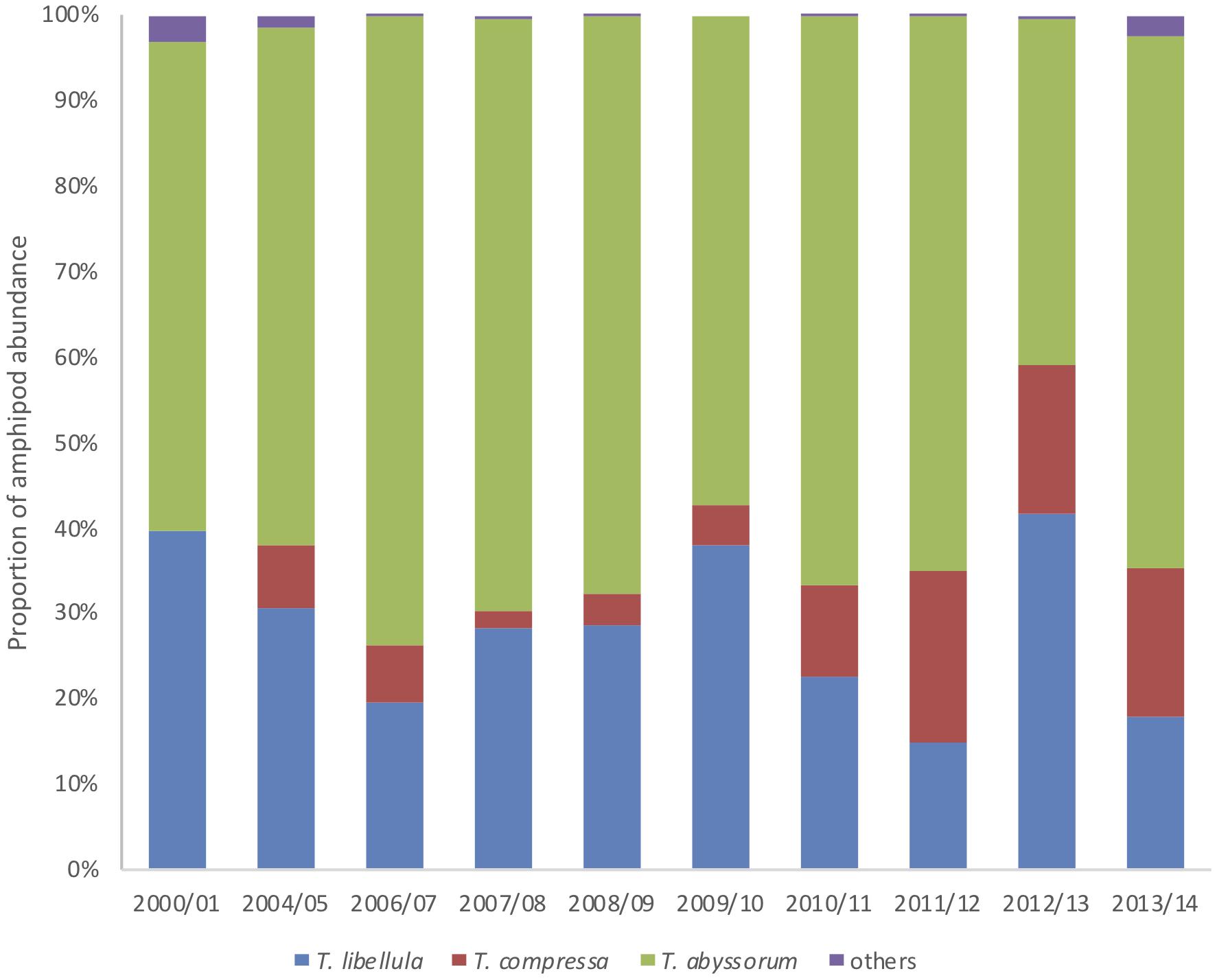
Figure 2. Proportion of amphipod abundances (%) at HAUSGARTEN Central Station per sampling period. Details for the years 2009/10 and 2010/11 can be found in the Supplementary Material.
The hyperiid genus Themisto dominated the epipelagic amphipod counts by >97%. The three dominating pelagic Themisto spp. showed significant seasonal, inter-annual and spatial variability over the studied period 2011–2014 (Figure 3), with high abundances in summer and lower numbers in winter. The highest peaks of T. libellula were observed at the end of summer (August–September), at both sites, in most cases with maximal abundances in periods of maximum ice cover. Monthly abundances of T. abyssorum were more variable regionally; for the northern HAUSGARTEN site they were highest in August, whereas in the central site they were highest in June for 2012 and in September for 2013, coinciding with ice-free periods. Overall, T. abyssorum dominated the amphipod community by >50%. However, in 2012/13, T. abyssorum and T. libellula were present in nearly equal proportions (∼40%, respectively), over the entire year at both HAUSGARTEN sites. Whereas the two native Themisto species were present and dominating throughout the year, T. compressa was absent in the trap samples over long periods in winter (November–February), reappearing in spring. Abundances of this North Atlantic species remained elevated compared to the mid-2000s, with noteworthy counts in late summer 2011. L. clausii was the most abundant amphipod species after the three Themisto spp. with 19 specimens collected between 2011 and 2014. Previously, this species was absent, but, similarly to T. compressa – it became more abundant although to a much lower degree.
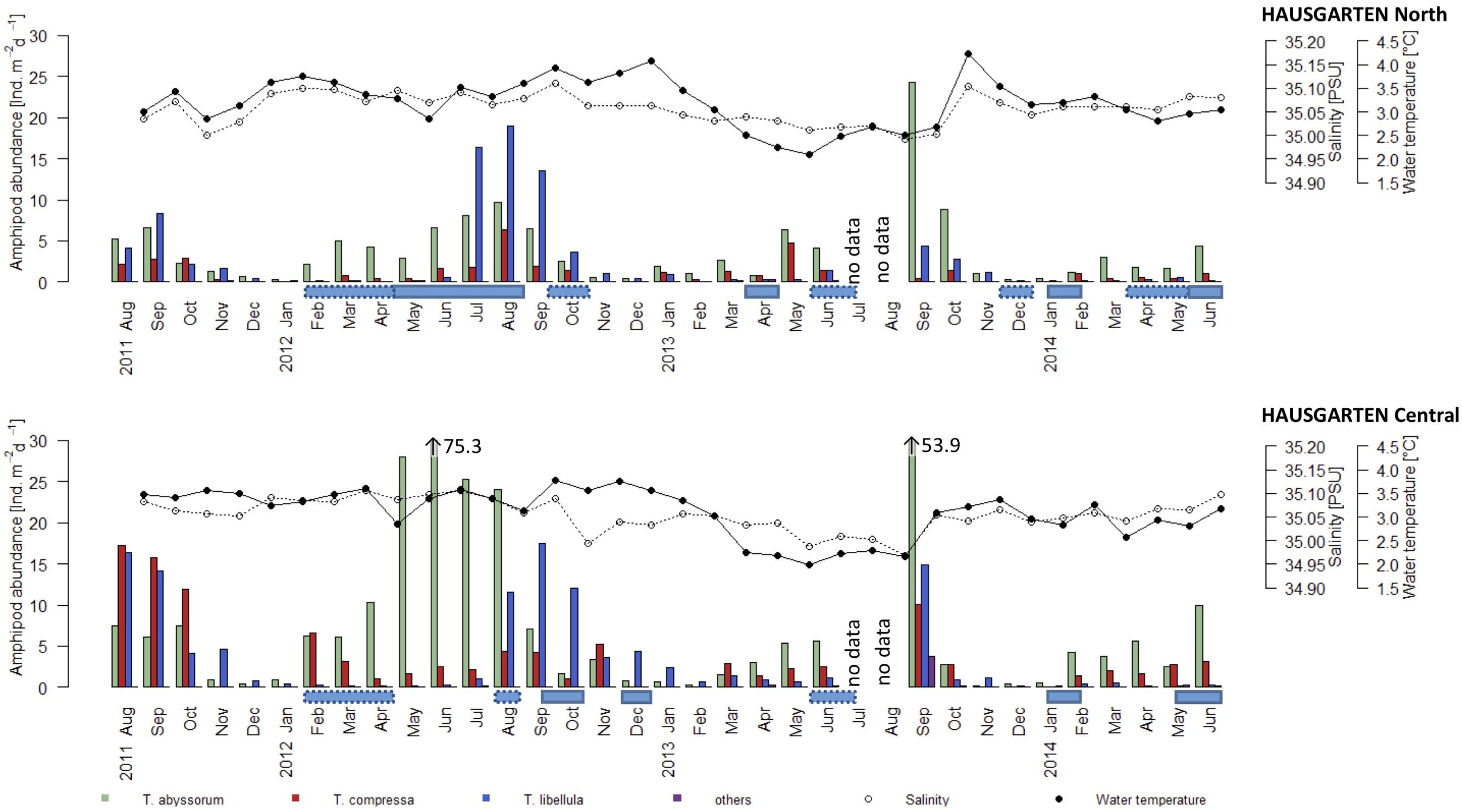
Figure 3. Progression of mean monthly amphipod abundance (Ind.m−2d−1) in HAUSGARTEN sediment traps along a latitudinal gradient: northern (upper panel) and central position (lower panel). Note the off-scale values of Themisto abyssorum in the lower panel (dashed boxes = periods of occasional ice cover, solid boxes = permanent ice cover). Connected dots represent the salinity (open circles) and temperature (filled circles) values across the years (for amphipod species color codes, see Figure 2).
Population Structures (2011–2014), Sex Ratio and Life Stages of Themisto spp.
Examination of the occurrences of the different life stages (Figure 4) showed noteworthy proportions of juveniles of T. abyssorum (up to 8% of the total specimens). These were found at each site both in winter and summer, with higher proportions in winter. Focusing on the sample sites, higher proportions of juvenile T. abyssorum were observed at the northern HAUSGARTEN site compared to the central location. For T. libellula, juveniles did not reach more than 1% of the total individuals. Not a single juvenile specimen of T. compressa was recorded in this study during winter, and only very few occurred in summer (max. 1% of the total individuals). Females were always dominant for all three species, with a maximum value of 96% in T. libellula. For both T. abyssorum and T. libellula, the sex ratio was even more skewed toward female dominance in summer than in winter at both sites. The opposite was true for T. compressa, where the largest proportions of females occurred in winter at both sites.
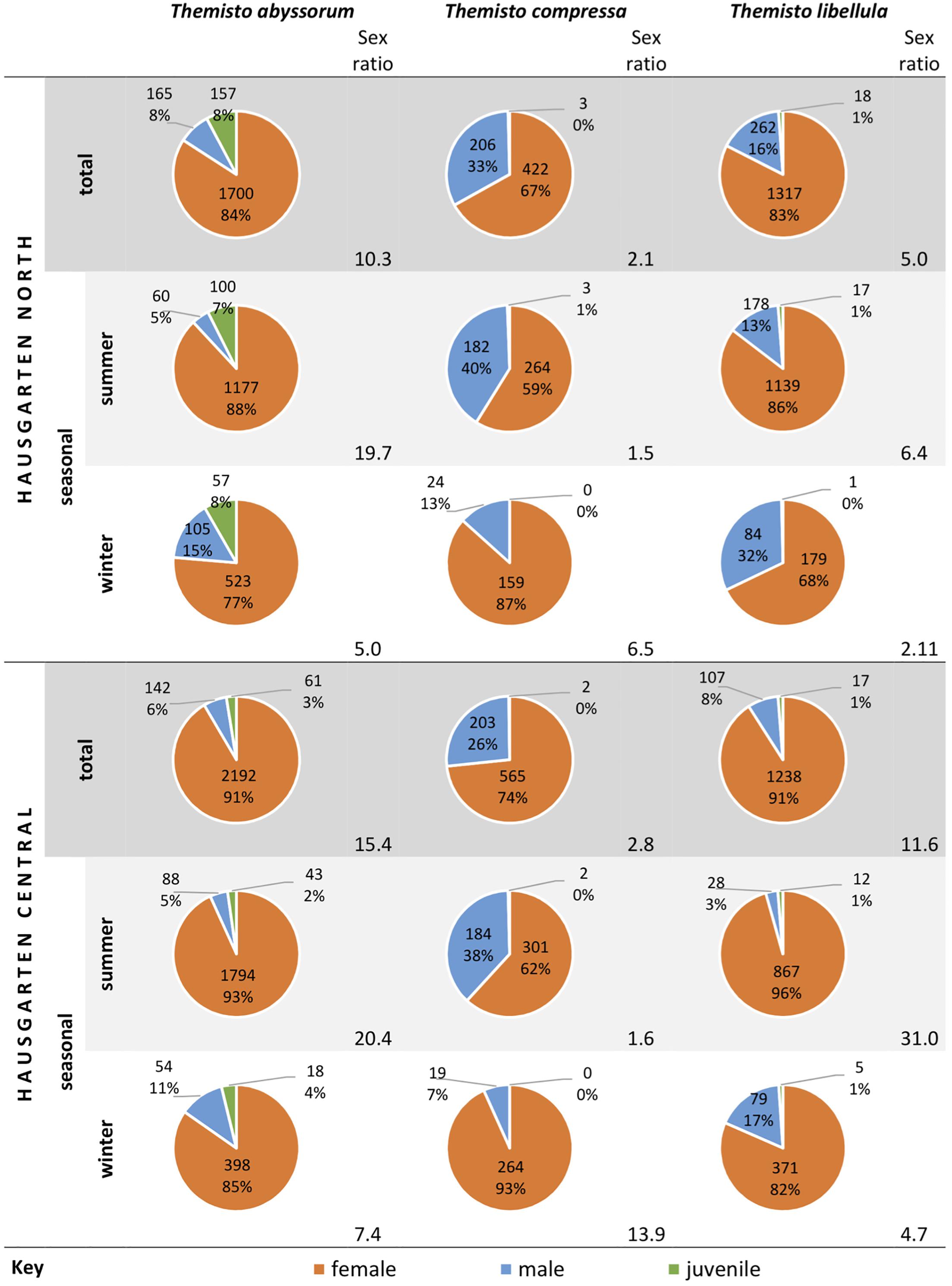
Figure 4. Total and seasonal percentages of gender and maturity of the Themisto species between 2011 and 2014; absolute numbers are counts, relative numbers are proportions of total counts. Sex ratios = female counts/male counts. Missing data were replaced with comparative means of the respective month.
Trends in Themisto spp. Abundances Over the Entire Sampling Period (2000–2014)
We combined our abundance data from the different years of observations (2011–2014) with the data (2000–2011) of Kraft’s (2010) Ph.D. thesis and detected a compositional dissimilarity of the amphipod community between different sampling periods. A separation of earlier samples from those collected for this study is apparent (Figure 5). Altogether, the ordination plot suggests three different clusters: the 2000/01 sample, both 2012/13 samples, and the remaining samples. SIMPER analysis (Supplementary Table S3) showed a clear trend of increased contributions of high Themisto compressa abundances toward the right domain of the ordination chart (Figure 5). Furthermore, a tendency of increasing T. libellula abundances toward the upper domain of the chart could be identified, and vice versa for T. abyssorum. The exclusive position of the sample on the very left in the MDS-plot (2000/01 in Figure 5) was mainly due to the absence of T. compressa in that sampling period. The joint isolated positions of the 2012/13 samples were caused by increased abundances of T. compressa and T. libellula, as well as by relatively low abundances of T. abyssorum, compared to other years.
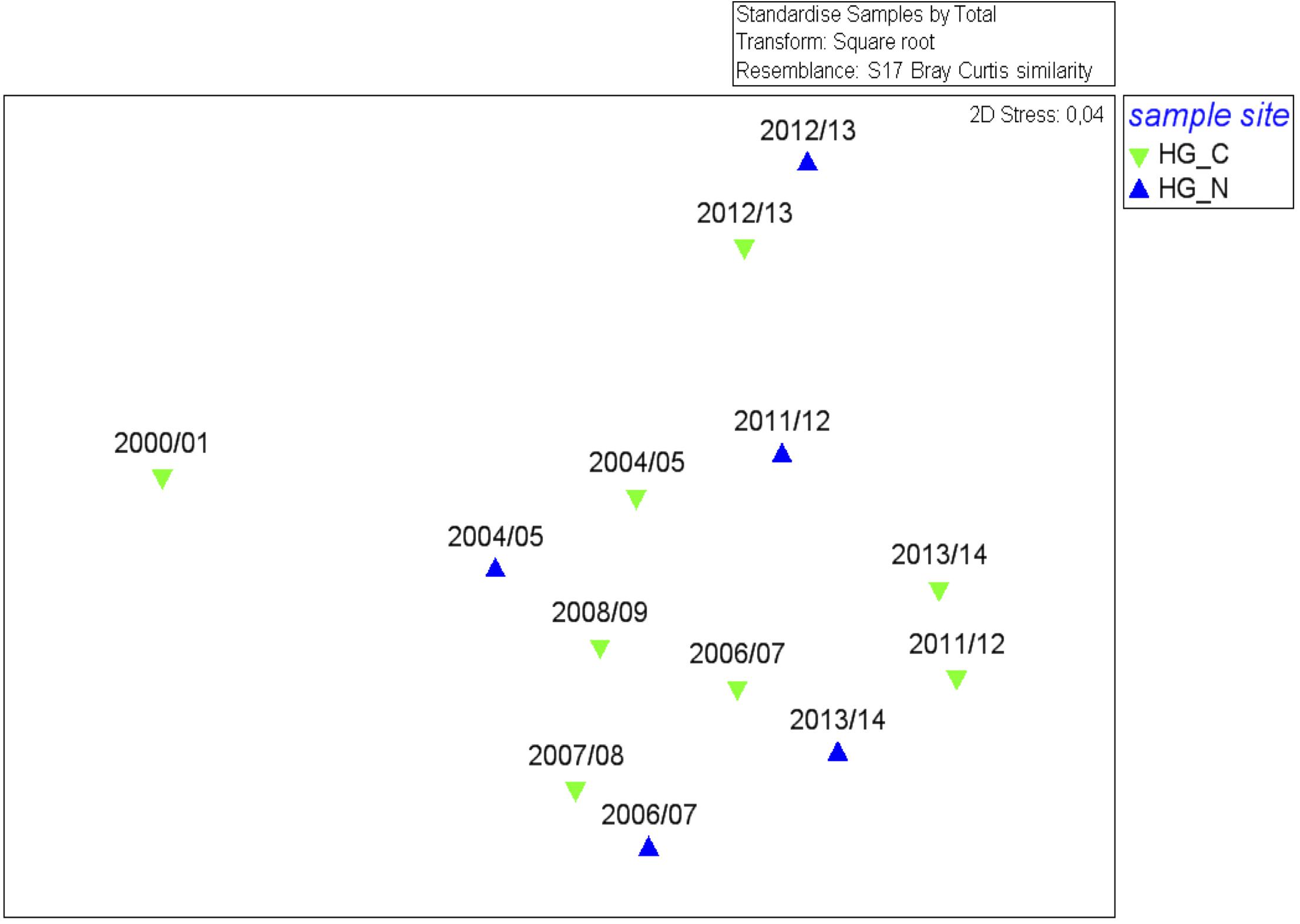
Figure 5. Multi-Dimensional Scaling plot of mean amphipod abundances (Ind.m−2d−1) in HAUSGARTEN sediment traps (2000–2014) based on the Bray Curtis similarity measure. Blue upright triangles indicate the northern sampling locations, green inverted triangles represent the central moorings. Data ranging from 2000 to 2009 were obtained and analyzed by Kraft (2010).
Discussion
General Long-Term Trends of Themisto spp. at LTER HAUSGARTEN in the Eastern Fram Strait
The focus of this study was to verify the trends in dominating pelagic amphipod swimmer abundances recorded by Kraft et al. (2011, 2013) by investigating three more consecutive years (2011–2014) of amphipod sampling at two locations within the LTER observatory HAUSGARTEN. As amphipod species are more abundant in particular water masses (Dalpadado et al., 2001), they may serve as sentinel species to detect changes in the pelagic environment. With this new dataset, we also analyzed the population structure of the three dominating amphipod species.
The trends of respective abundances of the two native Themisto species – the boreal T. abyssorum and the Arctic T. libellula – as well of that of the intruding T. compressa, corroborated the observations from the period 2000–2012 described by Kraft et al. (2013). In the period 2000–2012, total amphipod counts increased by a factor of 14 during and after a warm anomaly (Supplementary Figure S1) observed in the HAUSGARTEN area, and counts remained equally high until 2014 (Figures 2, 3) despite the more stable water temperatures (Supplementary Figure S1).
Bottom-up and top-down processes similarly could have caused this tremendous increase in amphipod counts. Soltwedel et al. (2016) reported a slight increase of chlorophyll a biomass in the Fram Strait’s surface waters as well as in the water column. Hence, they suggested an increase of phytoplankton biomass in the region from 2008 onward (Soltwedel et al., 2016). These findings are complemented by Nöthig et al. (2015) documenting increasing chlorophyll a concentrations in the WSC since the 1990s during summer months. This development provided more food for pelagic herbivorous copepods, which in the Fram Strait mainly comprises the genus Calanus (Hirche et al., 1994; Mumm et al., 1998; Hop et al., 2006; Hildebrandt et al., 2014). It has been shown that egg production in Calanus glacialis was positively correlated to chlorophyll a concentration (Hirche et al., 1994). Supporting this, an increase of ∼50% in copepod abundances in central HAUSGARTEN sediment taps was evident between 2000 and 2014 (Nöthig et al., unpublished data). It is thus possible that raptorial carnivores, such as amphipods of the genus Themisto (Dalpadado et al., 2008b; Kraft et al., 2015), found sufficient prey, which might have caused the continuously increasing amphipod abundances. However, we do not know whether the 50% increase in food availability would have been enough to sustain the very high numbers of amphipods we report. One could speculate that, in addition, amphipods were not so heavily preyed upon by higher trophic levels.
Similarly, a top-down mechanism could have caused increased amphipod abundances. Amphipods are important prey for fish (e.g., capelin, cod), birds (e.g., little auk), and marine mammals (e.g., ringed seal) (Klekowski and Wȩsławski, 1991; Dalpadado et al., 2001, 2008b; Auel and Werner, 2003; Melle et al., 2004). For the Barents Sea, Dalpadado et al. (2001) suggested a strong predator-prey interaction between amphipods and fish such as cod and capelin. They further demonstrated that low abundances in predatory fish were accompanied by increased amphipod stocks, and vice versa (Dalpadado et al., 2001). Hence, it is very likely that in this trophic interaction, the release of predation pressure results in the recovery of amphipods preyed upon. Dalpadado et al. (2001) also suggested that this mechanism mainly controls amphipod populations in the Barents Sea. According to the Norwegian Directorate of Fisheries (2015), catches of capelin, haddock, and herring declined between 2010 and 2014, whereas Atlantic cod (Gadus morhua) catches increased by ∼30% for the same time frame. Given the high commercial value of Atlantic cod and haddock (Norwegian Directorate of Fisheries, 2015), a recently observed northward shift of Atlantic cod (Christiansen et al., 2016) and an enormous increase of fishing vessel sightings near Svalbard (Bergmann and Klages, 2012), the potential fishing pressure may remain high, relieving the amphipods from predation impact.
The three dominant pelagic Themisto species showed significant seasonal, inter-annual and spatial variability (Figure 3), with high abundances in summer and lower numbers in winter. Overall, T. abyssorum dominated the amphipod community by >50% during the period 2011–2014, corresponding to Kraft et al. (2011) results. However, in 2012/13, T. abyssorum and T. libellula were present in nearly equal proportions (∼40%, respectively) at both HAUSGARTEN sites. This sampling period was characterized by extraordinary ocean temperatures starting with a warm winter followed by a pronounced temperature drop, with cold water prevailing the entire summer of 2013 (Walczowski et al., 2017). It has been demonstrated that T. abyssorum was more abundant than T. libellula when warm WSC water predominated (Koszteyn et al., 1995; Dalpadado, 2002; Dalpadado et al., 2008a,b, 2016). Hence, the broad impact of the WSC may explain the observed predominance of T. abyssorum at the HAUSGARTEN, potentially coupled with increased reproductive rates and/or less predation mortality as discussed above. High reproductive rates were observed at both sites over the study period as indicated by the high proportions of juveniles compared with the two other species and their ubiquity over both seasons.
Whereas the two common species in the study area were present and dominating throughout the year, T. compressa was absent in the trap samples over long periods in winter (November–February). The reappearance of this species in spring may thus indicate an “allochthonous origin” as speculated by Kraft et al. (2013). Abundances of this North Atlantic species remained elevated compared to the mid-2000s, with noteworthy counts in late summer 2011, which may be attributed to inflow of warmer Atlantic water causing higher ocean temperatures in the eastern Fram Strait between 2011 and 2012 (Beszczynska-Möller et al., 2012; Walczowski, 2013; Gluchowska et al., 2017). This warm event has also been shown to be related to a substantial increase in abundances of the Atlantic-associated copepod Calanus finmarchicus in the WSC (Gluchowska et al., 2017). Irrespective of its winter absences, T. compressa appears to have become a common species in the eastern Fram Strait. To date, however, the species has not yet been recorded in the central Arctic (Kosobokova et al., 2011; Kosobokova, personal communication). L. clausii was the most abundant amphipod species after the three Themisto spp. with 19 specimens collected between 2011 and 2014. Previously, it was absent, but, – similarly to T. compressa in July 2004 – it became more abundant, although on a lower scale.
In this study we observed erratic peaks in amphipod abundances, especially in T. abyssorum (see Figure 3). Swarms or high density aggregations of T. abyssorum, T. compressa and T. libellula have previously been recorded both on the seafloor and in the water column (Lampitt et al., 1993; Vinogradov, 1999; Angel and Pugh, 2000). We assume that swarms of Themisto spp. were present at the sediment traps no later than the end of July 2013 (Figure 3), accumulating in the instruments’ funnels, filling the sample cups to the top, and thus exceeding the poison’s capacity to preserve the samples and resulting in degraded samples (Lee et al., 1992). A funnel full of swimmers would also explain the prolonged event lasting until early September 2013, with swimmer material filling up the next sample cup entirely when it was exposed for sampling. Strikingly, degraded samples occurred concurrently at both the central and the northern locations between July and early September 2013, for which similar mechanisms causing the degradation can be assumed. Furthermore, we suggest that due to degradation, significant numbers of amphipods are not included in the data, possibly leading to an underestimation of the maximum abundances recorded. In this context, the occasional T. abyssorum abundance peaks as in June 2012 and September 2013 (75.3 and 53.9 Ind. m−2d−1, respectively; Figure 3) were unexpected and can similarly be considered records of swarming events.
Population Structure of Themisto spp. in the Eastern Fram Strait During 2011–2014
Noteworthy proportions of juveniles of T. abyssorum (up to 8% of the total individuals) were recorded, which is consistent with Kraft et al. (2012). These were higher at the northern HAUSGARTEN site compared to the central location (Figure 4). This difference between sites coincides with a difference of ca. + 0.1°C in mean water temperature between August 2011 and June 2014 at the northern station compared to the central station and differences in sea ice cover. Juveniles were present in both seasons at both sites (contradicting Kraft, 2010), indicating more than one spawning period per year, as discussed by Koszteyn et al. (1995). This outcome contrasts with Kraft (2010), who obtained a seasonal pattern for juvenile T. abyssorum abundances, hence suggesting a seasonal migration of juveniles or lower reproductive rates. Not a single juvenile specimen of T. compressa was recorded in this study during winter, and only very few occurred in summer (max. 1% of the total specimen count), indicating no or only limited reproduction in the area; this is supported by Kraft et al. (2012). However, the record of Kraft et al. (2013) of brooding females in the traps may indicate that reproduction of T. compressa in the area is possible, but still rare. Very low numbers of juvenile T. libellula were recorded, irrespective of location and season (max. 1% of the total specimen count), whereas other investigations reported elevated numbers of juveniles between May and June (Percy, 1993; Koszteyn et al., 1995; Dale et al., 2006; Kraft et al., 2012). This may imply unfavorable reproductive conditions for the true Arctic T. libellula in a warming environment, as suggested by Dalpadado et al. (2016).
Maturity studies based on net hauls (e.g., Williams and Robins, 1981; Koszteyn et al., 1995; Dalpadado, 2002; Dale et al., 2006; Dalpadado et al., 2016) do not provide year-round data sets as do sediment trap catches (e.g., Kraft et al., 2012). Whereas net catches conducted in the Barents Sea by Dalpadado (2002) between August-September 1993 yielded juvenile proportions of up to 88% for T. abyssorum and 80% for T. libellula, a maximum percentage of 8% was recorded for T. abyssorum herein. Large relative numbers of juveniles have also been found in similar studies in the Greenland and Barents seas (e.g., Koszteyn et al., 1995; Dale et al., 2006). On the other hand, size distributions found herein agree with other sediment trap-approaches, such as Kraft et al. (2012), who generally observed very few juveniles. The different approaches target different depths, and it is known that Themisto spp. are often segregated by depth in the water column according to sex and life stage (Williams and Robins, 1981; Wȩsławski et al., 2006). Varying criteria for classifying life stages (juveniles, immature adults, mature adults) (Williams and Robins, 1981; Percy, 1993; Wȩsławski et al., 2006) and seasonal migratory behavior (Percy, 1993; Kraft, 2010) may also account for the large discrepancies in the outcomes.
Community Changes of Themisto spp. at LTER HAUSGARTEN in the Eastern Fram Strait
By combining data on pelagic amphipods species from this study (2011–2014) with Kraft (2010) Themisto spp. and other pelagic amphipod data sets from her Ph.D. thesis (2000–2011), we obtained a broader view of the amphipod community development between 2000 and 2014. Within this time frame, a separation of older and more recent samples is apparent (Figure 5), indicating a development of the system.
According to the similarity analyses of species composition between the different years and sites, the trend of increased T. compressa proportions most probably caused the dissimilarity over years. In general, the system has changed to a state of higher T. compressa abundances reflected by an increasing contribution of this species to the observed cluster patterns. Thus, the lack of T. compressa in the 2000/01 sample resulted in its strong dissimilarity with other samples. Furthermore, increased T. libellula abundances mainly contributed to the vertical separation of the samples with increasing numbers in the upper domain. Similarly, high T. abyssorum abundances contributed to the vertical clustering with highest values in the lower domain of the chart. However, these trends do not seem to indicate a continuous temporal development as discussed for T. compressa. These trends are reflected in the 2012/13 samples because their dissimilarity was mainly due to high cluster contributions of increased abundances of T. compressa and T. libellula as well as to low abundances of T. abyssorum. Interestingly, the 2012/13 winter was characterized by notably high ocean temperatures followed by a subsequent cold summer (Supplementary Figure S1, as mentioned in Gluchowska et al., 2017). We speculate that the abundances of T. libellula did not appear to be affected by increased abundances of the intruding T. compressa, at least under cold water conditions; however, abundances of T. abyssorum appeared to be adversely influenced (see also Stempniewicz et al., 2007). We further speculate that even though T. abyssorum is assumed to tolerate a high temperature gradient and show high abundances in Atlantic water masses (Dalpadado, 2002), competition between T. compressa and T. abyssorum may play a role, given their similar sizes and ecological roles (e.g., carnivorous feeding type – see Kraft et al., 2015).
Variations and possible shifts in amphipod proportions of the three dominant pelagic hyperiids are evident based on our long-term data series. The occurrence of the North Atlantic species T. compressa (Kraft et al., 2013) continued until 2014, which may be attributed to higher water temperatures (Supplementary Figure S1; Walczowski et al., 2017). The latter was confirmed by the observation that the abundances observed at the central HAUSGARTEN site were considerably higher than at the northern location. The system is evidently shifting toward a warm, more North Atlantic-influenced state (Gluchowska et al., 2017), potentially causing Arctic species to decline (Dalpadado et al., 2016). This is corroborated by the low numbers of juveniles of the true Arctic T. libellula in the sampling period 2013/14, even though previously, these were commonly detected. The replacement of the larger, lipid-rich T. libellula by the sub-Arctic and temperate species T. abyssorum and T. compressa in the Arctic could change the food chain pattern with possible consequences for fish, whale and bird populations that depend on this species as major prey. For example, little Auks (Alle alle) feed predominantly on the largest size class of T. libellula (Lønne and Gabrielsen, 1992), and hence, the other Themisto species cannot eventually act as substitutes because of their smaller size.
Warming water temperatures are a likely cause of the increasing amphipod abundances between 2000 and 2014 that are potentially affecting trophic interactions and increasing competition between Themisto spp. Thus, more temperate species evidently extended their range into the Arctic, as we demonstrated by the seasonal establishment of the North Atlantic species T. compressa. Other, previously unsampled species are newly appearing in the Fram Strait sediment traps, with the most abundant being the hyperiid L. clausii. These outcomes suggest ongoing environmental shifts taking place in the seasonally ice-covered eastern Fram Strait. For a better understanding of species interactions and for firm predictions regarding future pelagic communities, more regionally and temporally extensive studies on the topic are urgently needed.
Data Availability
The datasets for the 2011–2014 data can be found in the supplementary information. The existing data (2000–2011) can be found in the PANGAEA repository: Oceanographic data sets see Bauerfeind et al. (2015), amphipod numbers see Kraft et al. (2012).
Author Contributions
FS wrote the manuscript together with E-MN and CH. AK, AB-M, NK, and EB provided the data of amphipods, hydrography as well as trap, and mooring logistics and analyzed the respective data sets. All authors involved in discussions about the amphipod development in the Fram Strait in the long-term perspective of environmental change in the Arctic Ocean and revised and approved the manuscript.
Funding
This study was funded by the German Federal Ministry of Education and Research (project: 03F0629A). CH was funded by the German Science Foundation/Deutsche Forschungsgemeinschaft (DFG) with the project HA 7627/1-1 within the Priority Programme SPP-1158 on Antarctic Research with Comparable Investigations in Arctic Sea Ice Areas.
Conflict of Interest Statement
The authors declare that the research was conducted in the absence of any commercial or financial relationships that could be construed as a potential conflict of interest.
Acknowledgments
We thank C. Lorenzen and E. Bonk (Alfred Wegener Institute, Helmholtz Centre for Polar and Marine Research) for lab assistance and the tedious work of swimmer picking. We thank all the helpers picking out swimmers from the traps. We would also like to thank the AWI Physical Oceanography section, the AWI Deep Sea group, and the captains and crews of FS Polarstern and MSMerian for their support during all the years. Thanks also to Marcia M. Gowing for the language check as well as Michaela Grote for support in generating Figure 3.
Supplementary Material
The Supplementary Material for this article can be found online at: https://www.frontiersin.org/articles/10.3389/fmars.2019.00311/full#supplementary-material
References
Angel, M. V., and Pugh, P. R. (2000). “Quantification of diel vertical migration by macroplankton and micronektonic taxa in the northeast Atlantic,” in Proceedings of the 34th European Marine Biology Symposium on Island, Ocean and Deep-Sea Biology, eds M. B. Jones, J. M. N. Azevedo, A. I. Neto, A. C. Costa, and A. M. Frias Martins (Ponta Delgarda: Kluwer Academic), 161–179. doi: 10.1007/978-94-017-1982-7_16
Auel, H., and Werner, I. (2003). Feeding, respiration and life history of the hyperiid amphipod Themisto libellula in the Arctic marginal ice zone of the Greenland Sea. J. Exp. Mar. Biol. Ecol. 296, 183–197. doi: 10.1016/s0022-0981(03)00321-6
Bauerfeind, E.,von Appen, W.-J., Soltwedel, T., Sablotny, B., and Lochthofen, N. (2015). Physical oceanography and current meter data from moorings at HAUSGARTEN, 2001-2014. London: Pangaea, doi: 10.1594/PANGAEA.150005.
Bauerfeind, E., Nöthig, E.-M., Beszczynska, A., Fahl, K., Kaleschke, L., Kreker, K., et al. (2009). Particle sedimentation patterns in the eastern fram strait during 2000-2005: results from the Arctic long-term observatory hausgarten. Deep Sea Res. Part I 56, 1471–1487. doi: 10.1016/j.dsr.2009.04.011
Beaugrand, G. (2009). Decadal changes in climate and ecosystems in the North Atlantic Ocean and adjacent seas. Deep Sea Res. Part II 56, 656–673. doi: 10.1016/j.dsr2.2008.12.022
Bergmann, M., and Klages, M. (2012). Increase of litter at the Arctic Deep-Sea observatory hausgarten. Mar. Pollut. Bull. 64, 2734–2741. doi: 10.1016/j.marpolbul.2012.09.018
Beszczynska-Möller, A., Fahrbach, E., Schauer, U., and Hansen, E. (2012). Variability in Atlantic water temperature and transport at the entrance to the Arctic Ocean, 1997-2010. Ices J. Mar. Sci. 69, 852–863. doi: 10.1093/icesjms/fss056
Buchholz, F., Buchholz, C., and Weslawski, J. M. (2010). Ten years after: krill as indicator of changes in the macro-zooplankton comunities of two Arctic fjords. Polar Biol. 33, 101–113. doi: 10.1007/s00300-009-0688-0
Busch, K., Bauerfeind, E., and Nöthig, E.-M. (2015). Pteropod sedimentation patterns in different water depths observed with moored sediment traps over a 4-year period at the LTER station hausgarten in eastern fram strait. Polar Biol. 38, 845–859. doi: 10.1007/s00300-015-1644-9
Christiansen, J. S., Bonsdorff, E., Byrkjedal, I., Fevolden, S.-E., Karamushko, O. V., Lynghammar, A., et al. (2016). Novel biodiversity baselines outpace models of fish distribution in Arctic waters. Sci. Nat. 103:8. doi: 10.1007/s00114-016-1332-9
Clarke, K. R., and Gorley, R. N. (2005). PRIMER: Getting started with v6. Plymouth: PRIMER-E Ltd., 1–15.
Dale, K., Falk-Petersen, S., Hop, H., and Fevolden, S. E. (2006). Population dynamics and body composition of the Arctic hyperiid amphipod Themisto libellula in Svalbard fjords. Polar Biol. 29, 1063–1070. doi: 10.1007/s00300-006-0150-5
Dalpadado, P. (2002). Inter-specific variations in distribution, abundance and possible life-cycle patterns of Themisto spp. (Amphipoda) in the Barents Sea. Polar Biol. 25, 656–666.
Dalpadado, P., Borkner, N., Bogstad, B., and Mehl, S. (2001). Distribution of Themisto (Amphipoda) spp. in the Barents Sea and predator-prey interactions. ICES J. Mar. Sci. 58, 876–895. doi: 10.1006/jmsc.2001.1078
Dalpadado, P., Ellertsen, B., and Johannessen, S. (2008a). Inter-specific variations in distribution, abundance and reproduction strategies of krill and amphipods in the Marginal Ice Zone of the Barents Sea. Deep Sea Res. Part II 55, 2257–2265. doi: 10.1016/j.dsr2.2008.05.015
Dalpadado, P., Yamaguchi, A., Ellertsen, B., and Johannessen, S. (2008b). Trophic interactions of macro-zooplankton (krill and amphipods) in the Marginal Ice Zone of the Barents Sea. Deep-Sea Res. Part II 55, 2266–2274. doi: 10.1016/j.dsr2.2008.05.016
Dalpadado, P., Hop, H., Roenning, J., Pavlov, V., Sperfeld, E., Buchholz, F., et al. (2016). Distribution and abundance of euphausiids and pelagic amphipods in Kongsfjorden, Isfjorden and Rijpfjorden (Svalbard) and changes in their relative importance as key prey in a warming marine ecosystem. Polar Biol. 39, 1765–1784. doi: 10.1007/s00300-015-1874-x
Field, J. G., Clarke, K. R., and Warwick, R. M. (1982). A practical strategy for analysing multispecies distribution patterns. Mar. Ecol. Prog. Ser. 8, 37–52. doi: 10.3354/meps008037
Gascard, J.-C., Kergomard, C., Jeannin, P.-F., and Fily, M. (1988). Diagnostic study of the Fram Strait marginal ice zone during summer from 1983 and 1984 Marginal Ice Zone experiment lagrangian observations. J. Geophys. Res. 93, 3613–3641.
Gluchowska, M., Dalpadado, P., Beszczynska-Möller, A., Olszewska, A., Ingvaldsen, R. B., and Kwasniewski, S. (2017). Interannual zooplankton variability in the main pathways of the Atlantic water flow into the Arctic Ocean (Fram Strait and Barents Sea branches). ICES J. Mar. Sci. 74, 1921–1936. doi: 10.1093/icesjms/fsx033
Hansen, E., Gerland, S., Granskog, M. A., Pavlova, O., Renner, A. H. H., Haapala, J., et al. (2013). Thinning of Arctic sea ice observed in fram strait: 1990-2011. J. Geophys. Res. 118, 5202–5221. doi: 10.1002/jgrc.20393
Hildebrandt, N., Niehoff, B., and Sartoris, F. J. (2014). Long-term effects of elevated CO2 and temperature on the arctic calanoid copepods Calanus glacialis and C. hyperboreus. Mar. Pollut. Bull. 80, 59–70. doi: 10.1016/j.marpolbul.2014.01.050
Hirche, H. J., Hagen, W., Mumm, N., and Richter, C. (1994). The Northeast Water Polynya, Greenland Sea - III. meso- and macrozooplankton distribution and production of dominant herbivorous copepods during spring. Polar Biol. 14, 491–503.
Hop, H., StigFalk, P., Harald, S., Slawek, K., Vladimir, P., Olga, P., et al. (2006). Physical and biological characteristics of the pelagic system across fram strait to kongsfjorden. Prog. Oceanogr. 71, 182–231. doi: 10.1016/j.pocean.2006.09.007
Klekowski, R. Z., and Wȩsławski, J. M. (1991). Atlas of the Marine Fauna of southern Spitsbergen Invertebrates Part 1, Vol. 2. Gdańsk: Polish Academy of Sciences.
Kortsch, S., Primicerio, R., Fossheim, M., Dolgov, A. V., and Aschan, M. (2015). Climate change alters the struccture of arctic marine food webs due to poleward shifts of boreal generalists. Proc. R. Soc. B 282:20151546. doi: 10.1098/rspb.2015.1546
Kosobokova, K. N., Hopcroft, R. R., and Hirche, H.-J. (2011). Patterns of zooplankton diversity through the depths of the Arctic’s central basins. Mar. Biodivers. 41, 29–50. doi: 10.1007/s12526-010-0057-9
Koszteyn, J., Timofeev, S., Wȩsławski, J. M., and Malinga, B. (1995). Size structure of Themisto abyssorum Boeck and Themisto libellula (Mandt) populations in European Arctic seas. Polar Biol. 15, 85–92. doi: 10.1007/bf00241046
Kraft, A. (2010). Amphipods observed in sediment trap samples in the eastern Fram Strait (Arctic Ocean) - Community patterns in relation to climate variability. Master Thesis. Bremen: University of Applied Sciences Bremen, 1–129.
Kraft, A., Bauerfeind, E., and Nöthig, E.-M. (2011). Amphipod abundance in sediment trap samples at the long-term observatory hausgarten (Fram Strait, ∼79°N/4°E). Variability in species community patterns. Mar. Biodiver. 41, 353–364. doi: 10.1007/s12526-010-0052-1
Kraft, A., Bauerfeind, E., Nöthig, E.-M., and Bathmann, U. V. (2012). Size structure and life cycle patterns of dominant pelagic amphipods collected as swimmers in sediment traps in the eastern fram strait. J. Mar. Syst. 95, 1–15. doi: 10.1016/j.jmarsys.2011.12.006
Kraft, A., Graeve, M., Janssen, D., Greenacre, M., and Falk-Petersen, S. (2015). Arctic pelagic amphipods: lipid dynamics and life strategy. J. Plankton Res. 37, 790–807. doi: 10.1093/plankt/fbv052
Kraft, A., Nöthig, E.-M., Bauerfeind, E., Wildish, D. J., Pohle, G. W., Bathmann, U. V., et al. (2013). First evidence of reproductive success in a southern invader indicates possible community shifts among Arctic zooplankton. Mar. Ecol. Prog. Ser. 493, 291–296. doi: 10.3354/meps10507
Krumpen, T., Gerdes, R., Haas, C., Hendricks, S., Herber, A., Selyuzhenok, V., et al. (2015). Recent summer sea ice thickness surveys in the fram strait and associated volume fluxes. Cryosphere Discuss. 9, 5171–5202. doi: 10.5194/tcd-9-5171-2015
Kwasniewski, S., Gluchowska, M., Walkusz, W., Karnovsky, N. J., Jakubas, D., Wojczulanis-Jakubas, K., et al. (2012). Interannual changes in zooplankton on the West Spitsbergen Shelf in relation to hydrography and their consequences for the diet of planktivorous seabirds. ICES J. Mar. Sci. 69, 890–901. doi: 10.1093/icesjms/fss076
Lampitt, R. S., Wishner, K. F., Turley, C. M., and Angel, M. V. (1993). Marine snow studies in the Northeast Atlantic Ocean: distribution, composition and role as a food source for migrating plankton. Mar. Biol. 116, 689–702. doi: 10.1007/bf00355486
Lee, C., Hedges, J. I., Wakeham, S. G., and Zhu, N. (1992). Effectiveness of various treatments in retarding microbial activity in sediment trap material and their effects on the collection of swimmers. Limnol. Oceanogr. 37, 117–130. doi: 10.4319/lo.1992.37.1.0117
Liu, J., Song, M., Horton, R. M., and Hu, Y. (2013). Reducing spread in climate model projections of a September ice-free Arctic. Proc. Natl. Acad. Sci. U.S.A. 110, 12571–12576. doi: 10.1073/pnas.1219716110
Lønne, O. J., and Gabrielsen, G. W. (1992). Summer diet of seabirds feeding in sea-ice-covered waters near Svalbard. Polar Biol. 12, 685–692.
Melle, W., Ellertsen, B., and Skjoldal, H. R. (2004). “Zooplankton: the link to higher trophic levels,” in The Norwegian Sea Ecosystem, ed. H. Skjoldal (Trondheim: Tapir Academic Press), 137–202.
Mumm, N., Auel, H., Hanssen, H., Hagen, W., Richter, C., Hirche, H.-J., et al. (1998). Breaking the ice: large-scale distribution of mesozooplankton after a decade of Arctic and Transpolar cruises. Polar Biol. 20, 189–197. doi: 10.1007/s003000050295
Nghiem, S. V., Rigor, I. G., Perovich, D. K., Clemente-Colón, P., Weatherly, J. W., and Neumann, G. (2007). Rapid reduction of Arctic perennial sea ice. Geophys. Res. Lett. 34, 1–6.
Norwegian Directorate of Fisheries (2015). Økonomiske Og Biologiske Nøkkeltal Frå Dei Norske Fiskeria 2014 (Economic and Biological Figures from Norwegian Fisheries 2014). Bergen: Norwegian Directorate of Fisheries.
Nöthig, E. M., Bracher, A., Engel, A., Metfies, K., Niehoff, B., Peeken, I., et al. (2015). Summertime plankton ecology in Fram Strait - a compilation of long- and short-term observations. Polar Res. 34:23349. doi: 10.3402/polar.v34.23349
Percy, J. A. (1993). Reproduction and growth of the Arctic hyperiid amphipod Themisto libellula Mandt. Polar Biol. 13, 131–139.
Piechura, J., and Walczowski, W. (2009). Warming of the West Spitsbergen current and sea ice north of Svalbard. Oceanologia 5, 147–164. doi: 10.1002/ece3.1160
Polyakov, I. V., Beszczynska, A., Carmack, E. C., Dmitrenko, I. A., Fahrbach, E., Frolov, I. E., et al. (2005). One more step toward a warmer Arctic. Geophys. Res. Lett. 32, 1–4.
Polyakov, I. V., Pnyushkov, A. V., Alkire, M. B., Ashik, I. M., Baumann, T. M., Carmack, E. C., et al. (2017). Greater role for Atlantic inflows on sea-ice loss in the Eurasian Basin of the Arctic Ocean. Science 356, 285–291. doi: 10.1126/science.aai8204
Renner, A. H. H., Gerland, S., Haas, C., Spreen, G., Beckers, J. F., Hansen, E., et al. (2014). Evidence of Arctic sea ice thinning from direct observations. Geophys. Res. Lett. 41, 5029–5036. doi: 10.1002/2014gl060369
Schauer, U., Beszczynska-Möller, A., Walczowski, W., Fahrbach, E., Piechura, J., and Hansen, E. (2008). “Variation of measured heat flow through the fram strait between 1997 and 2006,” in Arctic-subarctic Ocean Fluxes: Defining the Role of the Northern Seas in Climate, eds R. R. Dickson, J. Meincke, and P. Rhines (Berlin: Springer), 65–85. doi: 10.1007/978-1-4020-6774-7_4
Schauer, U., Fahrbach, E., Osterhus, S., and Rohardt, G. (2004). Arctic warming through the Fram Strait: oceanic heat transport from 3 years of measurements. J. Geophys. Res. 109. doi: 10.1029/2003JC001823.
Soltwedel, T., Bauerfeind, E., Bergmann, M., Bracher, A., Budaeva, N., Busch, K., et al. (2016). Natural variability or anthropogenically-induced variation? Insights from 15 years of multidisciplinary observations at the arctic open-ocean LTER site hausgarten. Ecol. Indic. 65, 89–102. doi: 10.1016/j.ecolind.2015.10.001
Stempniewicz, L., Błachowiak-Samołyk, K., and Wȩsławski, J. M. (2007). Impact of climate change on zooplankton communities, seabird populations and arctic terrestrial ecosystem - A scenario. Deep Sea Res. Part II 54, 2934–2945. doi: 10.1016/j.dsr2.2007.08.012
Vinogradov, G. M. (1999). Deep-sea near-bottom swarms of pelagic amphipods Themisto: observations from submersibles. Sarsia 84, 465–467. doi: 10.1080/00364827.1999.10807352
Von Appen, W.-J., Schauer, U., Somavilla, R., Bauerfeind, E., and Beszczynska-Möller, A. (2015). Exchange of warming deep waters across fram strait. Deep Sea Res. Part I 103, 86–100. doi: 10.1016/j.dsr.2015.06.003
Walczowski, W. (2013). Frontal structures in the West Spitsbergen current margins. Ocean Sci. 9, 957–975. doi: 10.5194/os-9-957-2013
Walczowski, W., Beszczynska-Möller, A., Wieczorek, P., Merchel, M., and Grynczel, A. (2017). Oceanographic observations in the Nordic Sea and fram strait in 2016 under the IO PAN long-term monitoring program AREX. Oceanologica 59, 187–194. doi: 10.1016/j.oceano.2016.12.003
Wang, M., and Overland, J. E. (2012). A sea ice free summer Arctic within 30 years: an update from CMIP5 models. Geophys. Res. Lett. 39, 6–11.
Weigmann-Haass, R. (1997). Verbreitung von Makrozooplankton in der Grönlandsee im Spätherbst 1988 (Crustacea: Ostracoda, Hyperiidea [Amphipoda], Euphausiacea). Helgoländer Meeresuntersuchungen 51, 69–82. doi: 10.1007/bf02908755
Wȩsławski, J. M., Kwasniewski, S., Stempniewicz, L., and Blachowiak-Samolyk, K. (2006). Biodiversity and energy transfer to top trophic levels in two contrasting Arctic fjords. Pol. Polar Res. 27, 259–278.
Weydmann, A., Carstensen, J., Goszczko, I., Dmoch, K., Olszewska, A., and Kwasniewski, S. (2014). Shift towards the dominance of boreal species in the Arctic: inter-annual and spatial zooplankton variability in the West Spitsbergen current. Mar. Ecol. Prog. Series 501, 41–52. doi: 10.3354/meps10694
Weydmann, A., Carstensen, J., Goszczko, I., Dmoch, K., Olszewska, A., and Kwasniewski, S. (2018). Warming of Subarctic waters accelerates development of a key marine zooplankton Calanus finmarchicus. Glob. Change Biol. 24, 172–183. doi: 10.1111/gcb.13864
Keywords: sediment traps, hyperiids – pelagic amphipods, Arctic marine ecology, biodiversity, range shifts
Citation: Schröter F, Havermans C, Kraft A, Knüppel N, Beszczynska-Möller A, Bauerfeind E and Nöthig E-M (2019) Pelagic Amphipods in the Eastern Fram Strait With Continuing Presence of Themisto compressa Based on Sediment Trap Time Series. Front. Mar. Sci. 6:311. doi: 10.3389/fmars.2019.00311
Received: 26 February 2019; Accepted: 24 May 2019;
Published: 14 June 2019.
Edited by:
Maria Vernet, University of California, San Diego, United StatesReviewed by:
Alexei Pinchuk, University of Alaska Fairbanks, United StatesBerge Jørgen, UiT The Arctic University of Norway, Norway
Copyright © 2019 Schröter, Havermans, Kraft, Knüppel, Beszczynska-Möller, Bauerfeind and Nöthig. This is an open-access article distributed under the terms of the Creative Commons Attribution License (CC BY). The use, distribution or reproduction in other forums is permitted, provided the original author(s) and the copyright owner(s) are credited and that the original publication in this journal is cited, in accordance with accepted academic practice. No use, distribution or reproduction is permitted which does not comply with these terms.
*Correspondence: Eva-Maria Nöthig, RXZhLU1hcmlhLk5vZXRoaWdAYXdpLmRl