- 1Scottish Marine Institute, Scottish Association for Marine Science, Oban, United Kingdom
- 2Marine Scotland Science, Aberdeen, United Kingdom
Oil and gas pipelines that lie exposed on the seabed can function as “artificial reefs,” providing habitat for fish and benthic species, including some that are listed under conservation designations. As the offshore hydrocarbon industry matures, operators and national governments must decide whether decommissioned pipelines should be left in situ or removed for onshore disposal. In most jurisdictions, there is a requirement to evaluate the environmental consequences of different pipeline decommissioning options in a comparative assessment. To do this effectively requires an understanding of the associations between pipelines and fauna. Pipeline operators routinely collect video footage for inspection and maintenance purposes using remotely operated vehicles (ROV). This footage has the potential to provide insight into interactions between the marine environment and offshore pipelines. This study uses inspection footage from eight pipelines to quantify the presence and abundance of species and features listed under a number of EU and United Kingdom conservation designations; 12 such features and species were observed on the pipelines or neighboring sediments. The soft coral Alcyonium digitatum was present in the highest densities on pipelines located on mud, while Sabellaria sp. and Echinus esculentus were more common on pipelines in sand. Gadoids, anemones and hermit crabs were also frequently observed around pipelines. The study identifies the limitations to the use of industry ROV footage for ecological purposes, but shows that with consideration of taxon size, image resolution, ROV speed and altitude, this can be a valuable approach to gain additional insights into environment-infrastructure interactions. The results suggest that removal of pipelines will remove established colonies of epibenthic species, some of which have conservation value. The ecological significance of this loss, however, must be weighed against the broader considerations during pipeline decommissioning including cost, technical feasibility and impacts to other marine users.
Introduction
Subsea pipelines are an integral part of offshore oil and gas extraction and have been installed in all major hydrocarbon basins (Guo et al., 2005). Pipelines are generally constructed from steel, with polymer or concrete coatings, and can be installed directly on the seabed (“surface laid”) or within a trench, which can be back filled naturally or artificially (Oil and Gas Uk, 2013a). Protective structures are frequently installed in association with pipelines, and can include rock armoring (referred to as “rock dump”), grout bags or articulated concrete blocks linked with wire or rope (“concrete mattresses”) (Oil and Gas Uk, 2013a).
Artificial structures, including pipelines and protective structures, introduced into marine environments, provide hard substratum, which can be colonized by sessile and mobile organisms (Baine, 2001). These “artificial reef” communities can deliver ecosystem services including water filtration, carbon sequestration and the provision of commercially exploitable biomass (Moberg and Rönnbäck, 2003; Dafforn et al., 2015). The development of artificial reef communities on shipwrecks, coastal defense structures and oil and gas platforms is well-known (Pickering et al., 1999; Jørgensen et al., 2002; Gallaway et al., 2009; Firth et al., 2014), and these structures have been shown to support a locally high abundance and biomass of fauna (Boswell et al., 2010; Claisse et al., 2014). Despite the prevalence of offshore pipelines, there is a paucity of research on the extent to which pipelines support reef-associated species, and more generally on the interactions between pipelines and local ecosystems. One example of a large-scale pipeline-ecosystem interaction is the mass accumulation of jellyfish detritus along a West African pipeline (Lebrato and Jones, 2009). In part, the lack of ecological studies on pines is due to the time and cost requirements of conducting offshore environmental surveys at the necessary spatial and temporal scales (McLean et al., 2017).
Oil and gas operators routinely collect video footage of pipelines for inspection and maintenance purposes using remotely operated vehicles (ROV). This video footage has the potential to provide insight into interactions between the marine environment and offshore pipelines (Macreadie et al., 2018; McLean et al., 2018). In Australia, ROV footage was recently used to show that fish abundance on pipelines was double that of surrounding seabed, and that pipelines can provide hard substratum habitat (McLean et al., 2017; Bond et al., 2018a,b). Similarly, in California, a pipeline supported up to seven times the fish density of the adjacent seafloor (Love and York, 2005). Unlike oil and gas platforms, marine growth removal operations are not routinely carried out on pipelines, and are only performed on localized pipeline sections where there is a suspected integrity issues (Oil and Gas Uk, 2013b), potentially allowing mature hard substratum communities to develop on pipelines.
As hydrocarbon basins mature, decisions must be made on how to decommission redundant pipelines. Unlike oil and gas platforms, there are no international regulations governing pipeline decommissioning and individual nations are able to set their own legislative agenda (DECC, 2011; Oil and Gas Uk, 2013a). In North Sea states, pipelines are not covered by the OSPAR 98/3 decision that prohibits in situ decommissioning of platforms, and instead pipeline decommissioning is considered on a case by case basis, with all feasible methods evaluated through a comparative assessment process (Oil and Gas Uk, 2013a). Potential methods for pipeline decommissioning include full/partial removal or in situ decommissioning (Oil and Gas Uk, 2013a). The environmental implications of pipeline decommissioning will depend on the pipeline location, the pipeline properties and the decommissioning methods employed. The decommissioning process has the potential to affect marine organisms through physical impacts, noise disturbances, localized sediment disturbances, release of contaminants and the addition/removal of artificial hard substratum that provides habitat and/or protection from trawling damage (Burdon et al., 2018).
To predict the environmental impacts of pipeline decommissioning, and develop an evidence base to support the comparative assessment process, interactions between marine organisms and pipelines need to be quantified. This is particularly critical where pipelines intersect a designated conservation zone or interact with protected species. The United Kingdom Continental Shelf contains a number of internationally important “Features of Conservation Importance” (FOCI), which have been identified as threatened, rare, or declining (JNCC, 2010). Such FOCI include those listed in the EU Habitats Directive (Council Directive 92/43/Eec., 1992), e.g., cold-water corals, and those within designated marine protected areas. Many of the species within the FOCI group are epibenthic, and may colonize offshore pipelines (Benson et al., 2013). Additionally, some habitats, which are recognized within the FOCI group (e.g., Sabellaria reefs), might receive protection from fishing activities from the physical presence of pipelines. As with natural hard substratum habitats, it would be expected that the benthic community, and presence of FOCI associated with the pipelines will vary according to water depth and seabed substratum. Additionally, the intensity of mobile demersal fishing in the vicinity of the pipeline is likely to influence the abundance, diversity and biomass of benthic species and FOCI (Buhl-Mortensen et al., 2016).
In instances where pipeline decommissioning has the potential to interact with FOCI, operators must demonstrate compliance with relevant national and international environmental legislation. The operator must show that their decommissioning activities “will have no likely ‘significant’ effects on the designated species or feature” (Burdon et al., 2018). Significance in this context is poorly defined in terms of thresholds of change or relevant spatial scales, posing problems for interpretation (Wilding et al., 2017). In addition to serving as an evidence base for individual decommissioning decisions, quantitative data on interactions between marine species and oil and gas infrastructure can contribute toward efforts to define thresholds of change and determine meaningful spatial and temporal scales for assessments (Wilding et al., 2017).
The aim of this study was to repurpose industry ROV inspection footage to quantify associations between pipelines and observed marine fauna, and to discuss the extent to which pipelines may interact with benthic conservation features and species in the North Sea.
Materials and Methods
Site Description
This study focuses on pipelines in the northern North Sea region (Figure 1). The region consists of shallow (50 m) mixed water in the south and deep (200 m) stratified water in the north (De Wilde et al., 1992). The substratum is predominately sand, with areas of coarse gravel, finer sediments and muds (Breuer et al., 2004). Within the region, there is significant human activity including commercial fishing and oil and gas production (ICES, 2016).
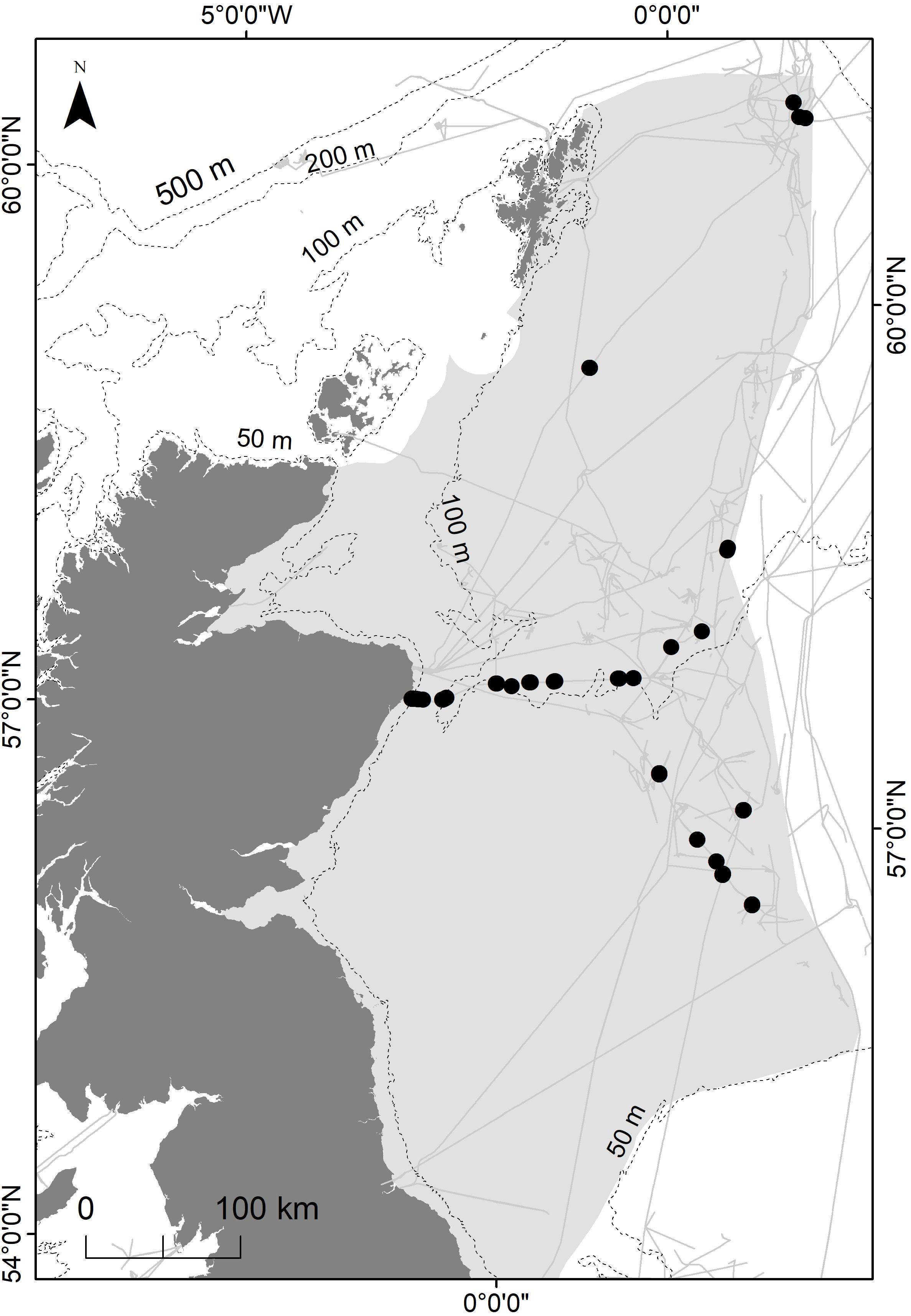
Figure 1. Oil and gas pipelines (gray lines) in the northern North Sea region (shaded area). Black circles represent the location samples of ROV footages used in this study.
Features of Conservation Importance
A list of United Kingdom FOCI was obtained from the Joint Nature Conservation Council [species1, habitats: (JNCC, 2010)]. The lists contained designations from thirteen conservations listings (Supplementary Table 1). From this list, benthic features were selected that could feasibly interact with subsea pipelines (i.e., hard-substratum associated species) and that could be identified from the survey footage. Species were selected if their known distributions corresponded to near- and offshore regions hosting subsea pipelines and if their body-size was sufficiently large to enable identification (typically > 5 cm diameter) or if they were present in high enough abundances to allow visualization (e.g., cup corals). The final list of target FOCI is shown in Supplementary Table 1. Each FOCI was categorized as a protected “species,” where each individual of a species is recognized as of conservation interest, or a “feature” where the habitat formed by the species is recognized as of conservation interest (Supplementary Table 1). “Habitat” is defined by individuals occurring at specific densities, e.g., individuals occurring with at least 30% coverage in an area of seabed that is at least 25 m2 (Irving, 2009; Morris, 2015).
ROV Footage and Sample Selection
The available ROV footage was recorded in standard definition throughout the day and night between 2013 and 2016. Footage was analyzed from eight pipelines greater than 16″ (∼406 mm) in diameter (Figure 1). All pipelines were installed prior to 2001, however data on the exact age of the pipelines were unavailable. Footage of pipelines that were fully buried, or covered by protective structures, was excluded. Pipelines that were covered by a thin sediment layer, crowning (approximately top 25% of the pipeline exposed) or fully exposed were included in the analysis. The footage comprised three concurrent views of the pipeline from starboard, port and central (top–down) cameras.
Each pipeline was divided into 1 km “sample sections.” The length of each sample section was determined from the footage metadata, which specified the location of the ROV in terms of kilometers along the pipeline. The kilometer point location of the ROV, which was used to determine the start and end of each 1 km sample section, was linked to the view in the central camera. Sample sections were assigned as occurring on sand or mud, in shallow (up to 50 m), medium (50–120 m) or deep (120 m or more) water by overlaying the pipeline locations with bathymetry and EMODnet modeled substratum layers [Folk (1980) classification] in ArcGIS (Stevenson, 2012). For each of the six depth-substratum combinations, five 1 km sections were randomly selected. For some combinations, fewer than five 1 km sections were available, in which case the maximum number of sections was selected. The first, central and final 100 m lengths within each 1 km section were selected for analysis. In instances where only shorter sections of suitable pipeline were available, the footage was combined to equal 100 m where possible. If it was not possible to obtain 100 m from the randomly selected section, a new 1 km section was selected. For each sample section, an estimate of fishing intensity in the vicinity of the pipeline was obtained by spatially overlaying the center point of the sample section with standardized data layers of fishing intensity (fishing hours in 2015) for vessels operating mobile demersal gear (ICES, 2017). The location of sample sections is shown in Figure 1.
Analysis
All footage was viewed in VLC Media Player version 2.2.3. Central, starboard and port camera views were analyzed successively. Footage was viewed at 0.33× speed and all visible fauna were recorded. The area of pipeline/seabed within the field of view could not be recorded and identified since the ROV footage did not contain a scale. It was not possible to use the diameter of pipeline for scale, since several pipelines were partially buried and the size of the exposed section was unknown. The number of individuals per section was counted for the target FOCI “species.” The length of coverage (in meters) along the pipeline was estimated for target FOCI “features” and colonial/encrusting species. All non-target species were recorded as presence/absence. Taxon location was recorded as either pipeline or seafloor. When the same taxon was present on both the pipeline and seafloor, both instances were recorded. Taxa were identified to the lowest level of classification possible based on visual observation and known geographic and depth distribution. Species-level identifications were only assigned when image quality was sufficient that there was high confidence in the identification. Additional expert opinions were sought to confirm species-level identification for rarer species. Poor quality images were excluded from the analysis, and in instances where that was lower confidence in the species identification, a higher taxonomic designation was assigned to the observation. Observations were made on the ability to resolve taxa from ROV footage according to the speed, altitude and lighting of videos.
Data Analysis
For each taxa, the number of individuals was divided by the total length of the sample sections to provide a density estimate of individuals per unit length (linear density). The individual density estimates were combined with the coverage per unit length (linear density) estimates for the FOCI features and colonial species. Linear density, rather than areal density, were the only metrics that could be extracted from the ROV footage, given the lack of a suitable scale. Data were explored for apparent trends between density, pipeline depth and substratum type for taxa occurring on the pipelines and the adjacent seabed. Pearson’s correlation coefficients with 95% confidence intervals were used to assess the magnitude of correlations between FOCI density and three pipeline location variables: water depth, latitude and fishing intensity (Pearson, 1901). A correlation coefficient could not be calculated between Sabellaria sp. density and fishing because all four observations were from samples with a uniform fishing effort.
Results
Video footage was analyzed from eight pipelines, totaling 5.18 km and derived from 154 sample sections. The majority of sample sections (n = 150, length = 4.86 km) were on sand, with only four sections (0.325 km) available on mud (Table 1). The pipeline sections on mud occurred between 123–158 m depth, whereas the sand sections ranged between 43–160 m depth. Approximately 55% of the footage was recorded between 7 am and 7 pm. The fishing intensity associated with sample sections ranged from zero hours to 205 h per year.
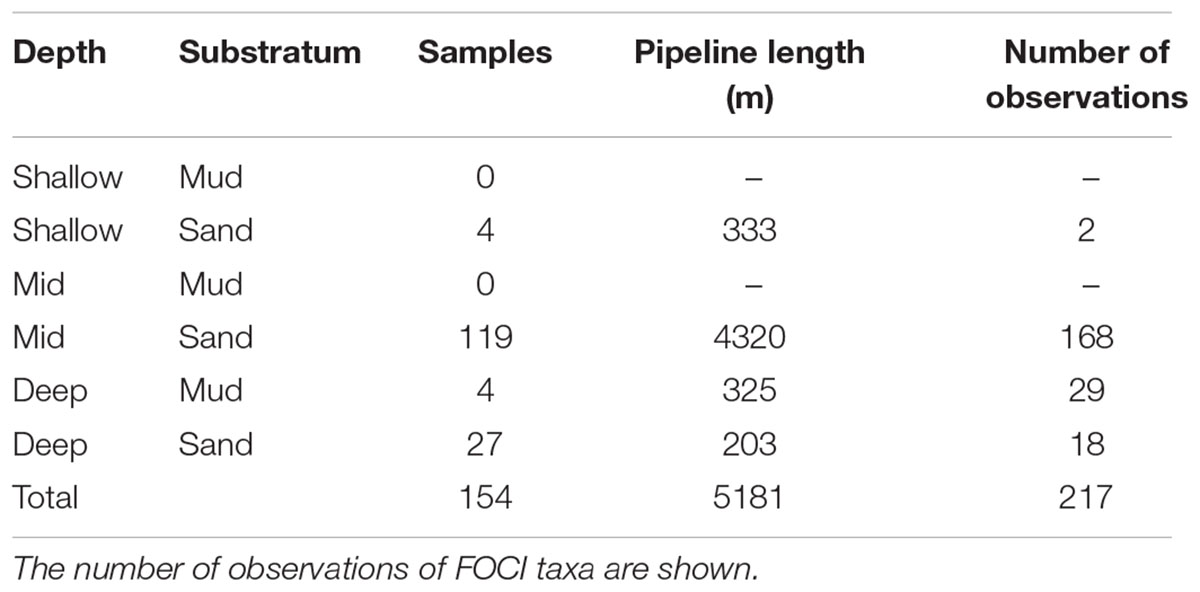
Table 1. Summary of the number ROV footage samples analyzed to identify features of conservation interest and the total length of pipeline reviewed, according to depth and substratum type.
A total of 57 taxa were identified from the video footage comprising sessile and mobile invertebrates and fish (Supplementary Table 2). Twenty-seven of the taxa could be identified to species level, while 17 could only be identified to order or above. The most frequently observed taxa were sea anemones, including the Deeplet anemones Bolocera tuediae (Johnston, 1832), Plumose anemones Metridium dianthus (Ellis, 1768) and Dahlia anemones, Urticina sp., and hermit crabs (Paguridae sp.). The majority of fish observed (and that could be identified to at least Order) were Gadoids, which were schooling around the pipelines. Ling [Molva molva (Linnaeus, 1758)] were observed under and around sections of the pipeline that lay unsupported above the substratum (“free spans”). Flatfish (Pleuronectiformes) were present on sediment adjacent to pipelines and three individual Rajiformes (Batoidea, skates/rays) were also observed on the adjacent sediment. There were no observations of non-indigenous species in any of the sample sections.
Features of Conservation Importance (FOCI)
Twelve FOCI were observed on the pipelines and adjacent seabed. Four FOCI were only observed on sediments, seven on sediments and pipelines and one (Crinoids) observed only on pipelines (Table 2). Of the FOCI observed on pipelines, the soft coral Alcyonium digitatum was present in the highest densities on pipelines in mud substrata, while Sabellaria sp. and Echinus esculentus were present in the greatest density on pipelines in sand substrata. Of the FOCI recorded on the sediment, burrowed communities and Virgularia mirabilis showed the highest densities in areas classified as mud, while Sabellaria sp. dominated sand areas (Table 2). The correlations between FOCI density and water depth varied from positive to negative (Table 2). The strongest correlations (>0.5) indicated an increase in density with water depth for the following four FOCI: the anemone Actinauge richardi and Crinoids on pipelines, and burrowed communities and Sabellidae worms on adjacent seabeds. However, the 95% confidence intervals for these correlation coefficients were large, suggesting that no correlation or a weak correlation between density and water depth were also possible. Similarly, the confidence intervals around the correlations coefficients for FOCI density against sample latitude and fishing intensity indicated that there were no relationships between these variables. The density of the common urchin E. esculentus on adjacent seabeds was the only FOCI to show a moderate to strong positive correlation with latitude (r = 0.70, 95% CI: 0.36–0.88).
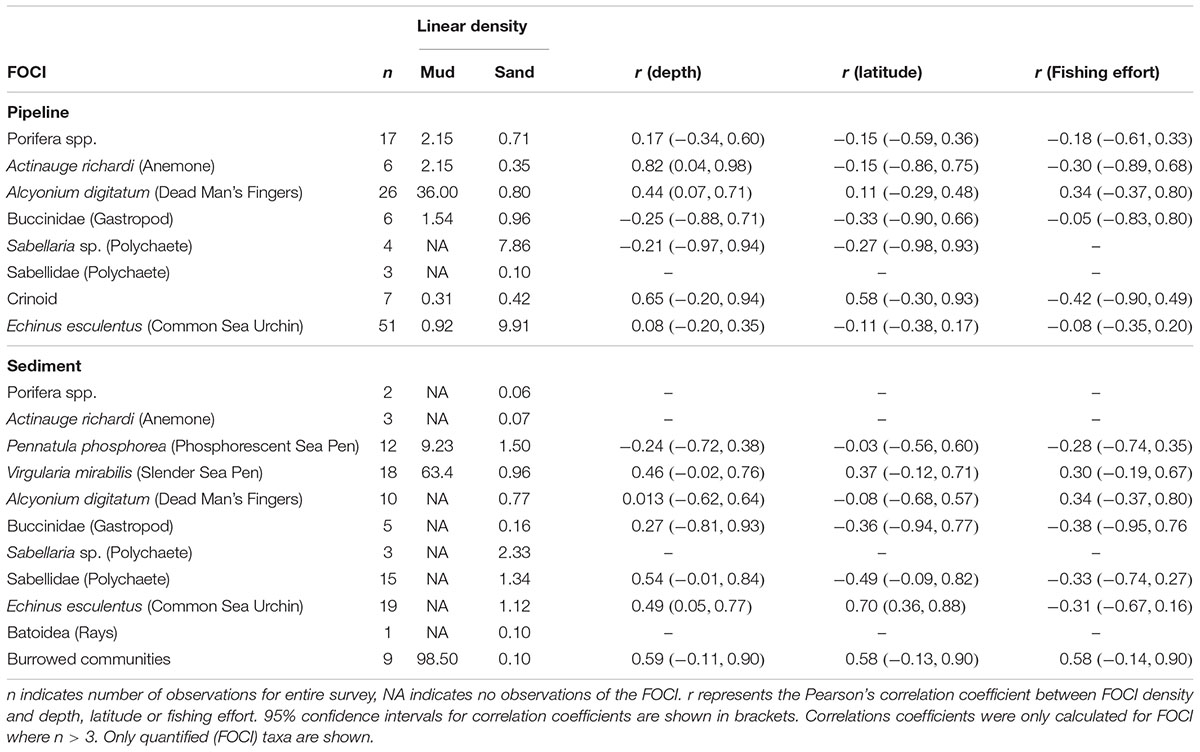
Table 2. Linear density (individuals/coverage per 100 m) of FOCI observed on pipelines and seabed, according to substratum type.
ROV Footage Characteristics
The ability to identify and quantify fauna associated with pipelines from video footage was influenced by the camera angles, ROV altitude and speed and illumination. Frequently, a section of pipeline could be observed in the port and starboard views up to 5 s in advance of the central view because of the angle of the lateral cameras. The angle of lateral cameras also influenced the amount of seabed visible either side of the pipeline. Typically, up to 1 m of seabed was visible either side of the pipeline, but this was substantially reduced when the lateral cameras were angled toward the pipeline.
The altitude of the ROV used to obtain the video footage affected the field of view. Altitude ranged from 0.36 to 2.75 m above the seabed (Figure 2A). Only two samples had an altitude of > 2.5 m. By comparing the cumulative length of pipeline analyzed with the cumulative number of observation for different ROV altitudes, it can be seen that there is a sharp increase in the number of observations between 0.5 and 0.6 m above the seabed, despite a steady increase in the length of pipeline analyzed (Figure 2). The majority of FOCI and other taxa records were obtained from samples sections with ROV altitudes of 1–1.5 m, with approximately half the number of observations obtained for sample sections with ROV altitudes of < 0.5 m or between 1.5 and 2.0 m. The clarity of the video footage was strongly affected by the speed of the ROV and the associated lighting. The speed of the ROV determined the extent of motion blur in the resultant footage. Speed data were only available for video footage from one of the operators and ranged from 0.03 to 1.48 km h-1. Generally, the footage was evenly and adequately illuminated, however, on occasion one of the lateral camera lights was switched off to allow better visualization of gaps between the pipeline and seafloor (free spans) for structural assessment purposes.
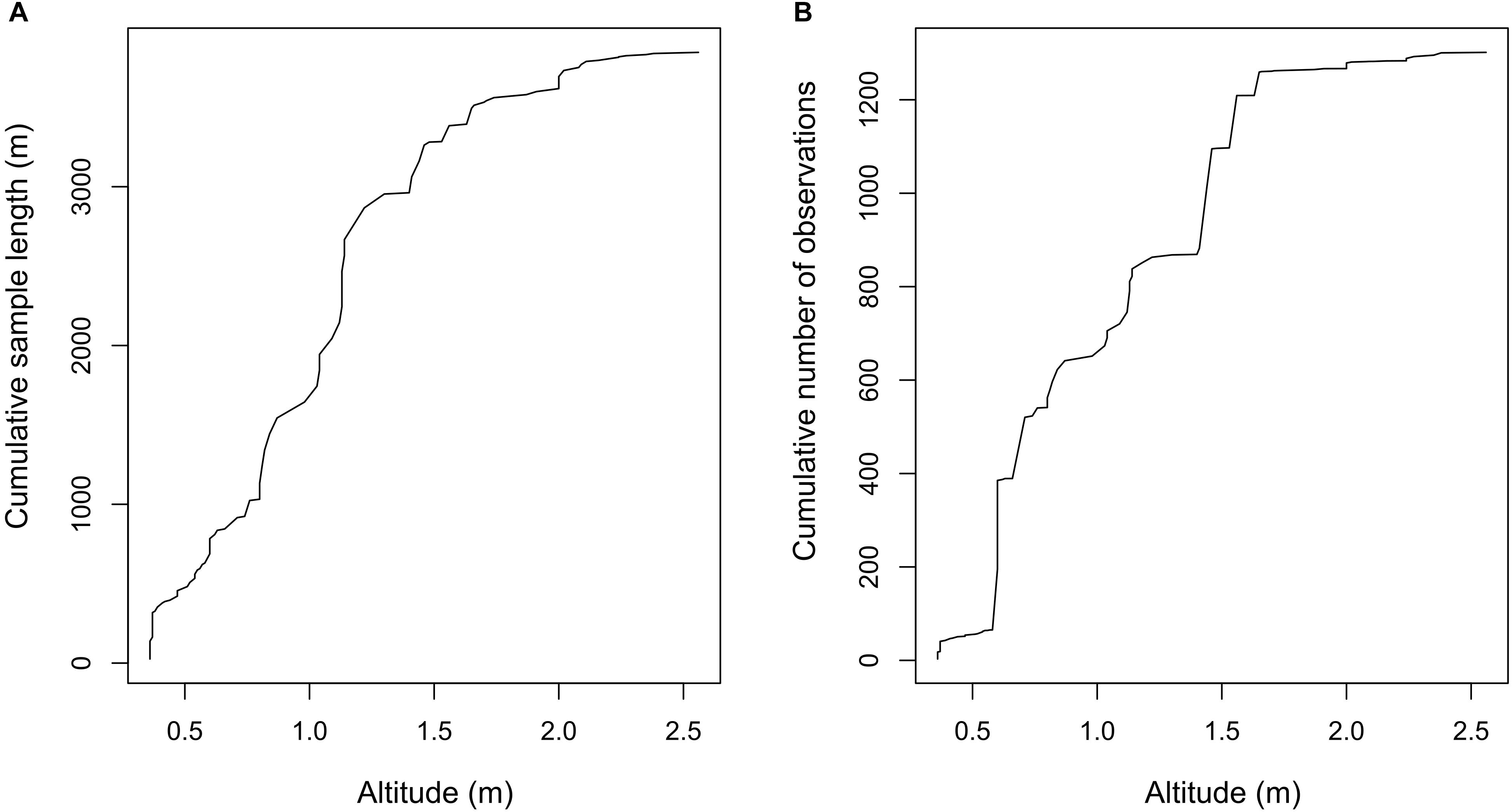
Figure 2. Cumulative length of pipeline analyzed in samples (A) and cumulative number of observations for all taxa and features of conservation interest (B) according to the altitude of the ROV used to obtain video footage of pipelines.
Discussion
Quantitative data on the associations between marine fauna and subsea pipelines are essential to understand the environmental consequences of installing and removing pipelines, and to support the sustainable management of anthropogenic activities in the marine environment. This study provides one of the first quantitative assessments of fish and benthic species associated with North Sea pipelines. The observation of approximately 60 taxa demonstrates that pipelines do provide habitat for a variety of marine fauna within the North Sea. The species observed were typical of North Sea hard substratum communities (Reiss et al., 2010), and are similar to the epifaunal assemblages documented on North Sea oil and gas platforms, offshore wind turbines and shipwrecks (Zintzen et al., 2008; De Mesel et al., 2015; van der Stap et al., 2016). The video footage used in this study was restricted to pipelines located in the northern North Sea. As with other artificial and natural substrata, it would be expected that the epifaunal assemblages associated with pipelines will exhibit spatial differences due to variations in substratum type, fishing intensity, current regime and stability of subsea pipelines between the southern and northern North Sea (Angus and Moore, 1982; Gass and Roberts, 2006; Reiss et al., 2010). The positive correlation observed between the common urchin E. esculentus and latitude suggests that for some species, spatial differences in pipeline assemblages can operate over small spatial scales. However, for the majority of observed FOCI there were no apparent relationships between density and pipeline depth, fishing intensity or latitude. However, the lack of apparent relationships obtained in this study may be a result of the small sample size, limited number of observations and the intrinsic limits to opportunistic reuse of ROV inspection footage in this study. Access to additional pipeline footage, and a targeted sampling approach would be required to further quantify the effect of pipeline location on FOCI presence and abundance. In addition to pipeline location, pipeline properties, including size, construction material, and burial status are likely to influence the community composition and abundance of epifauna. The data obtained in the current study were insufficient to quantify relationships between individual pipeline properties and the abundance of epifauna.
A number of fish species were observed in the vicinity of the subsea pipelines. The presence of ling underneath North Sea pipeline free spans (sections of pipeline unsupported by the substratum) is consistent with observations of fish aggregations under free spanning pipelines sections in Australian and Californian waters (Love and York, 2005; McLean et al., 2017; Bond et al., 2018c). Pipeline free spans are likely to offer shelter or refuge for the fish species observed in these habitats. Ling are generally solitary fish that move between crevices (e.g., free spans) and open-water habitats within a defined “home range” (Løkkeborg et al., 2000). Ling predate on other fish and invertebrates, including crustaceans and starfish (Husebø et al., 2002). The creation of crevices (through free spans) that lie in close proximity to a food supply, in the form of epifauna and other fish on the pipelines, is likely to drive the observed aggregations of ling. The presence of other gadoid fish around pipelines supports previous suggestions that aggregations of commercial fishing activity around subsea pipelines are a result of artificial reef effects of pipelines (Rouse et al., 2017).
Six of the taxa observed on the North Sea pipelines are listed under conservation designations, with an additional six taxa that would [if present at densities specified by the EU habitats Directive (Mcleod et al., 2009)] form habitat types that are protected under conservation legislation. It is likely that the true number of FOCI associated with pipelines is greater than reported in this study. Several FOCI (e.g., the amphipod Arrhis phyllonyx) are too small to be identified from video footage, and thus will have been excluded. Additionally, there are likely to be regional differences in FOCI presence and those that are restricted to the southern and central North Sea will not have been observed in the present study. The level of legal protection afforded to each FOCI varies according to the legislation/scheme under which it is designated. For those FOCI listed under the EU Habitats Directive, the United Kingdom Government is obliged to maintain or restore them at a favorable conservation status. This means that the range of FOCI must be stable or increasing and that the population is maintaining itself on a long-term basis. The EU Habitats Directive specifically excludes the use of non-natural substratum as candidate sites for the creation of marine protected areas, regardless of whether the feature or species are present at the necessary density to qualify as a habitat under Annex I (Mcleod et al., 2009).
The role of pipelines in maintaining FOCI ranges and/or stable populations will depend, partly, on the connectivity between pipelines and other suitable FOCI habitats. Artificial structures in the North Sea, including oil platforms and wind turbines, are thought to provide a network of highly connected hard substrata (Hyder et al., 2017; Henry et al., 2018). Pipelines have traditionally been excluded from North Sea connectivity/larval dispersal models (Hyder et al., 2017), but our results, documenting the presence of marine fauna on pipelines, suggest that pipelines will, to some extent, contribute to the connected network of some taxa. The extended linear presence of pipelines over the seabed, connecting larger areas of artificial hard substrate (i.e., platforms), could mean that pipelines facilitate dispersal of epifauna, particularly those characterized by short-lived larval or non-larvae based reproduction (Mineur et al., 2012).
The decommissioning of pipelines poses particular challenges in the North Sea because of the wide-spread spatial distribution of pipelines, the potential hazard that pipelines pose for commercial trawlers, and the costs and technical challenges of removing pipelines (Side, 1999; Oil and Gas Uk, 2013a; Rouse et al., 2018). Operators and regulators must ensure that pipeline decommissioning practices and/or policies balance these potentially conflicting challenges, as well as accounting for environmental concerns. The results from this study suggest that removal of decommissioned pipelines will eliminate established habitat for a number of epibenthic species, some of which have conservation value. Other pipeline decommissioning methods, including trenching or rock dumping would also be expected to eliminate this established habitat, however, rock dumping would provide additional substrate which could be colonized by epibenthic species. In situ decommissioning of pipelines, with minimal intervention, would be expected to cause the lowest level of disturbance for established epifauna and fish. However, where pipelines are removed or trenched, it may be expected that soft-sediment habitats and species would recover (Dernie et al., 2003). The exact impacts of pipeline decommissioning will depend on the methods used and the sensitivity of FOCI and other marine species to disturbance (Burdon et al., 2018). Furthermore, the ecological significance of local disturbances to marine fauna and FOCI through pipeline decommissioning must be considered within the context of the total footprint of pipelines in the North Sea, and the extent to which pipelines contribute to connectivity between hard substrata. On the United Kingdom Continental Shelf, pipelines occupy approximately 12 km2, equating to <0.01% of the total area (Rouse et al., 2018). The total contribution of pipelines to hard substrata habitats in the North Sea is, therefore, expected to be extremely small. However, footprints of human activities do not necessarily have a linear relationship with pressures, and small habitat fragments can contribute substantially to ecosystem functions (Jules and Shahani, 2003; Halpern et al., 2008). The inclusion of pipelines, with associated data on faunal abundance, in ecosystem models could determine whether or not pipelines make any meaningful contribution to regional ecosystem process and populations of FOCI.
Remotely operated vehicles video footage was successfully used in this study to quantify FOCI and record the presence of other marine fauna associated with pipelines. The repurposing of video footage, originally obtained for integrity assessments, for ecological analysis presented a number of challenges as have been documented previously by Gormley et al. (2018) and Macreadie et al. (2018). The lack of a suitable scale in the footage meant that the area within the field of view could not be calculated and faunal counts could not be scaled by area. The discrepancy between the central and lateral camera views led to inconsistencies in the section of pipeline assessed for each sample according to each camera view. The changing altitude of the ROV also limited the ability to identify taxa. Whilst the ROV being close to the pipeline potentially improved views of individuals and allowed for greater taxonomic resolution, it also limited the field of view either side of the pipeline and thus any individuals present there. Additionally, the altitude denoted the height above the seafloor, not above the pipeline. Therefore, footage over larger structures results in a higher recorded altitude but not necessarily a greater distance between the ROV and the structure. There are a number of small, relatively inexpensive, modifications that could be made to ROV survey design to improve the value for ROV footage for ecological analysis. With sufficient data on location, altitude and rotational parameters of the camera, it is possible to scale flat surface within images (Durden et al., 2016), however, the addition of scaling lasers to ROV would provide a more efficient methods of scaling images. Additionally, maintaining a constant ROV speed over pipelines and the collection of video/still photographs in high definition would increase the value of the footage for ecological analysis. These improvements, and access to additional pipeline ROV footage, would allow for the relationships between different pipeline properties and FOCI abundance, according to different regions of the North Sea to be quantified and provide a further evidence base to support decommissioning practices and policies.
Author Contributions
SR conceived the project and drafted the manuscript. NL performed video analysis and data collection, and contributed to writing methods. PH obtained the data, provided project oversight and guidance, and commented on draft manuscript. TW conceived the project, provided technical advice and oversight, and reviewed the manuscript.
Funding
This work was funded by the Natural Environment Research Council’s Oil and Gas Innovation Programme (NE/P016537/1).
Conflict of Interest Statement
The authors declare that the research was conducted in the absence of any commercial or financial relationships that could be construed as a potential conflict of interest.
Acknowledgments
The authors would like to acknowledge Mike Robertson and Adrian Tait (MSS) for assistance with taxa identification.
Supplementary Material
The Supplementary Material for this article can be found online at: https://www.frontiersin.org/articles/10.3389/fmars.2019.00200/full#supplementary-material
Footnotes
References
Angus, N. M., and Moore, R. L. (1982). “Scour repair methods in the southern north sea,” in Proceedings of the Offshore Technology Conference, (Houston, TX: Offshore Technology Conference).
Baine, M. (2001). Artificial reefs: a review of their design, application, management and performance. Ocean Coast. Manag. 44, 241–259. doi: 10.1016/s0964-5691(01)00048-5
Benson, A., Foster-Smith, B., Gubbay, S., and Hendrick, V. (2013). “Background document on Sabelleria spinulosa reefs,” in Proceedings of the Ospar Biodiversity Series Report, (London).
Bond, T., Langlois, T. J., Partridge, J. C., Birt, M. J., Malseed, B. E., Smith, L., et al. (2018a). Diel shifts and habitat associations of fish assemblages on a subsea pipeline. Fish. Res. 206, 220–234. doi: 10.1016/j.fishres.2018.05.011
Bond, T., Partridge, J. C., Taylor, M. D., Langlois, T. J., Malseed, B. E., Smith, L. D., et al. (2018b). Fish associated with a subsea pipeline and adjacent seafloor of the North West Shelf of Western Australia. Mar. Environ. Res. 141, 53–65. doi: 10.1016/j.marenvres.2018.08.003
Bond, T., Prince, J., Partridge, J. C., White, D., and Mclean, D. L. (2018c). “The value of subsea pipelines to marine biodiversity,” in Proceedings of the Offshore Technology Conference Asia, (Kuala Lumpur: Offshore Technology Conference).
Boswell, K. M., Wells, R. J. D., Cowan, J. J. H., and Wilson, C. A. (2010). Biomass, density, and size distributions of fishes associated with a large-scale artificial reef complex in the gulf of mexico. Bull. Mar. Sci. 86, 879–889. doi: 10.5343/bms.2010.1026
Breuer, E., Stevenson, A. G., Howe, J. A., Carroll, J., and Shimmield, G. B. (2004). Drill cutting accumulations in the Northern and Central North Sea: a review of environmental interactions and chemical fate. Mar. Pollut. Bull. 48, 12–25. doi: 10.1016/j.marpolbul.2003.08.009
Buhl-Mortensen, L., Neat, F., Koen-Alonso, M., Hvingel, C., and Holte, B. (2016). Fishing impacts on benthic ecosystems: an introduction to the 2014 Ices symposium special issue. ICES J. Mar. Sci. 73, i1–i4.
Burdon, D., Barnard, S., Boyes, S. J., and Elliott, M. (2018). Oil and gas infrastructure decommissioning in marine protected areas: system complexity, analysis and challenges. Mar. Pollut. Bull. 135, 739–758. doi: 10.1016/j.marpolbul.2018.07.077
Claisse, J. T., Pondella, D. J., Love, M., Zahn, L. A., Williams, C. M., Williams, J. P., et al. (2014). Oil platforms off California are among the most productive marine fish habitats globally. Proc. Natl. Acad. Sci. U.S.A. 111, 15462–15467. doi: 10.1073/pnas.1411477111
Council Directive 92/43/Eec. (1992). Council Directive 92/43/EEC of the 21st May 1992 on the conservation of natural habitats of wild fauna and flora. Official J. Eur. Commun. L206, 0007–0050.
Dafforn, K. A., Glasby, T. M., Airoldi, L., Rivero, N. K., Mayer-Pinto, M., and Johnston, E. L. (2015). Marine urbanization: an ecological framework for designing multifunctional artificial structures. Front. Ecol. Environ. 13:82–90. doi: 10.1890/140050
De Mesel, I., Kerckhof, F., Norro, A., Rumes, B., and Degraer, S. (2015). Succession and seasonal dynamics of the epifauna community on offshore wind farm foundations and their role as stepping stones for non-indigenous species. Hydrobiologia 756, 37–50. doi: 10.1007/s10750-014-2157-1
De Wilde, P. A. W. J., Jenness, M. I., and Duineveld, G. C. A. (1992). Introduction into the ecosystem of the North Sea: hydrography, biota, and food web relationships. Netherland J. Aquat. Ecol. 26, 7–18. doi: 10.1007/bf02298024
DECC (2011). Decommissioning of Offshore Oil and Gas Installations and Pipelines Under the Petroleum Act 1998. Guidance Notes. Aberdeen: Department of Energy and Climate Change. doi: 10.1007/bf02298024
Dernie, K. M., Kaiser, M., Richardson, E., and Warwick, R. (2003). Recovery of soft sediment communities and habitat following physical disturbance. J. Exp. Mar. Biol. Ecol. 285–286, 415–434. doi: 10.1016/s0022-0981(02)00541-5
Durden, J. M., Schoening, T., Althaus, F., Friedman, A., Garcia, R., Glover, A. G., et al. (2016). “Perspectives in visual imaging for marine biology and ecology: from acquisition to understanding,” in Oceanography and Marine Biology, eds R. J. A. Atkinson and R. N. Gibson (Boca Raton, FL: CRC Press).
Firth, L., Thompson, R., Bohn, K., Abbiati, M., Airoldi, L., Bouma, T., et al. (2014). Between a rock and a hard place: environmental and engineering considerations when designing coastal defence structures. Coast. Eng. 87, 122–135. doi: 10.1016/j.coastaleng.2013.10.015
Gallaway, B. J., Szedlmayer, S. T., and Gazey, W. J. (2009). A life history review for red snapper in the Gulf of Mexico with an evaluation of the importance of offshore petroleum platforms and other artificial reefs. Rev. Fish. Sci. 17, 48–67. doi: 10.1080/10641260802160717
Gass, S. E., and Roberts, J. M. (2006). The occurrence of the cold-water coral Lophelia pertusa (Scleractinia) on oil and gas platforms in the North Sea: colony growth, recruitment and environmental controls on distribution. Mar. Pollut. Bull. 52, 549–559. doi: 10.1016/j.marpolbul.2005.10.002
Gormley, K., Mclellan, F., Mccabe, C., Hinton, C., Ferris, J., Kline, D., et al. (2018). Automated image analysis of offshore infrastructure marine biofouling. J. Mar. Sci. Eng. 6:2. doi: 10.3390/jmse6010002
Guo, B., Song, S., Ghalambor, A., and Chacko, J. (2005). Offshore Pipelines. Burlington, VT: Elsevier. doi: 10.3390/jmse6010002
Halpern, B. S., Walbridge, S., Selkoe, K. A., Kappel, C. V., Micheli, F., D’agrosa, C., et al. (2008). A global map of human impact on marine ecosystems. Science 319, 948–952.
Henry, L.-A., Mayorga-Adame, C. G., Fox, A. D., Polton, J. A., Ferris, J. S., Mclellan, F., et al. (2018). Ocean sprawl facilitates dispersal and connectivity of protected species. Sci. Rep. 8:11346. doi: 10.1038/s41598-018-29575-4
Husebø,Å, Nøttestad, L., Fosså, J., Furevik, D., and Jørgensen, S. (2002). Distribution and abundance of fish in deep-sea coral habitats. Hydrobiologia 471, 91–99.
Hyder, K., Van Der Molen, J., Garcia, L., Callaway, A., Posen, P., Wright, S., et al. (2017). Assessing the Ecological Connectivity Between Man-made Structures in the North Sea (EcoConnect). Norwich: CEFAS Laboratory.
ICES (2016). Greater North Sea Ecoregion - Ecosystem Overview. Ices Advice 2018, Book 6. Copenhagen: ICES.
ICES (2017). Spatial Data Layers of Fishing Intensity/Pressure per Gear Type for Surface and Subsurface Abrasion, for the Years 2009 to 2016 in the Ospar Area. Copenhagen: ICES.
Irving, R. (2009). The Identification of the Main Characteristics of Stony Reef Habitats Under the Habitats Directive. Summary Report of an Inter-agency Workshop 26-27th March 2008.
Jørgensen, T., Løkkeborg, S., and Soldal, A. V. (2002). Residence of fish in the vicinity of a decommissioned oil platform in the North Sea. ICES J. Mar. Sci. 59, S288–S293.
Jules, E. S., and Shahani, P. (2003). A broader ecological context to habitat fragmentation: why matrix habitat is more important than we thought. J. Veg. Sci. 14, 459–464. doi: 10.1111/j.1654-1103.2003.tb02172.x
Lebrato, M., and Jones, D. O. B. (2009). Mass deposition event of Pyrosoma atlanticum carcasses off Ivory Coast (West Africa). Limnol. Oceanogr. 54, 1197–1209. doi: 10.4319/lo.2009.54.4.1197
Løkkeborg, S., Skajaa, K., and Fernö, A. (2000). Food-search strategy in ling (Molva molva L.): crepuscular activity and use of space. J. Exp. Mar. Biol. Ecol. 247, 195–208. doi: 10.1016/s0022-0981(00)00148-9
Love, M. S., and York, A. (2005). A comparison of the fish assemblages associated with an oil/gas pipeline and adjacent seafloor in the Santa Barbara Channel, Southern California Bight. Bull. Mar. Sci. 77, 101–118. doi: 10.3160/0038-3872-111.2.101
Macreadie, P. I., Mclean, D. L., Thomson, P. G., Partridge, J. C., Jones, D. O. B., Gates, A. R., et al. (2018). Eyes in the sea: unlocking the mysteries of the ocean using industrial, remotely operated vehicles (Rovs). Sci. Total Environ. 634, 1077–1091. doi: 10.1016/j.scitotenv.2018.04.049
McLean, D. L., Macreadie, P., White, D. J., Thomson, P. G., Fowler, A., Gates, A. R., et al. (2018). “Understanding the global scientific value of industry rov data, to quantify marine ecology and guide offshore decommissioning strategies,” in Proceedings of the Offshore Technology Conference Asia, (Kuala Lumpur: Offshore Technology Conference).
McLean, D. L., Partridge, J. C., Bond, T., Birt, M. J., Bornt, K. R., and Langlois, T. J. (2017). Using industry Rov videos to assess fish associations with subsea pipelines. Cont. Shelf Res. 141, 76–97. doi: 10.1016/j.csr.2017.05.006
Mcleod, C. R., Yeo, M., Brown, A. E., Burn, A. J., Hopkins, J. J., and Way, S. F. (2009). The Habitats Directive: Selection of Special Areas of Conservation in the UK. Peterborough: JNCC. doi: 10.1016/j.csr.2017.05.006
Mineur, F., Cottier-Cook, E., Minchin, D., Bohn, K., Macleod, A., and Maggs, C. (2012). “Changing coasts: marine aliens and artificial structures,” in Oceanography and Marine Biology: An Annual Review, eds R. N. Gibson, R. J. A. Atkinson, J. D. M. Gordon, and R. N. Hughes (Abingdon, GB: CRC Press), 189–234. doi: 10.1201/b12157-5
Moberg, F., and Rönnbäck, P. (2003). Ecosystem services of the tropical seascape: interactions, substitutions and restoration. Ocean Coast. Manag. 46, 27–46. doi: 10.1016/s0964-5691(02)00119-9
Morris, E. (2015). Defining Annex I Biogenic Modiolus Modiolus Reef Habitat Under the Habitats Directive: Report of an Inter-agency Workshop, March 4th and 5th 2014. JNCC Report No. 531, Peterborough.
Oil and Gas Uk (2013a). Decommissioning of Pipelines in the North Sea Region. Aberdeen: Oil and Gas Uk.
Oil and Gas Uk (2013b). The Managment of Marine Growth During Decommissioning. Aberdeen: Oil and Gas Uk.
Pearson, K. (1901). Mathematical contributions to the theory of evolution. Vii. on the correlation of characters not quantitatively. Philos. Trans. R. Soc. 196, 1–47.
Pickering, H., Whitmarsh, D., and Jensen, A. (1999). Artificial reefs as a tool to aid rehabilitation of coastal ecosystems: investigating the potential. Mar. Pollut. Bull. 37, 505–514. doi: 10.1016/s0025-326x(98)00121-0
Reiss, H., Degraer, S., Duineveld, G. C. A., Kröncke, I., Aldridge, J., Craeymeersch, J. A., et al. (2010). Spatial patterns of infauna, epifauna, and demersal fish communities in the North Sea. ICES J. Mar. Sci. 67, 278–293. doi: 10.1093/icesjms/fsp253
Rouse, S., Kafas, A., Catarino, R., and Hayes, P. (2017). Commercial fisheries interactions with oil and gas pipelines in the North Sea: considerations for decommissioning. ICES J. Mar. Sci. 75, 279–286. doi: 10.1093/icesjms/fsx121
Rouse, S., Kafas, A., Hayes, P., and Wilding, T. A. (2018). Offshore pipeline decommissioning: scale and context. Mar. Pollut. Bull. 129, 241–244. doi: 10.1016/j.marpolbul.2018.02.041
Side, J. (1999). Issues arising from the westhaven tragedy concerning fishing gear and pipeline interactions. Underwater Technol. 24, 3–9. doi: 10.3723/175605499783259875
Stevenson, A. (2012). The European marine observation and data network: geological data. Baltica 25, 87–90. doi: 10.1111/geb.12729
van der Stap, T., Coolen, J. W. P., and Lindeboom, H. J. (2016). Marine fouling assemblages on offshore gas platforms in the southern north sea: effects of depth and distance from shore on biodiversity. PLoS One 11:e0146324. doi: 10.1371/journal.pone.0146324
Wilding, T. A., Gill, A. B., Boon, A., Sheehan, E., Dauvin, J. C., Pezy, J.-P., et al. (2017). Turning off the Drip (‘Data-rich, information-poor’) – rationalising monitoring with a focus on marine renewable energy developments and the benthos. Renew. Sustain. Energy Rev. 74, 848–859. doi: 10.1016/j.rser.2017.03.013
Keywords: artificial reef, pipelines, marine spatial planning, decommissioning, benthic
Citation: Rouse S, Lacey NC, Hayes P and Wilding TA (2019) Benthic Conservation Features and Species Associated With Subsea Pipelines: Considerations for Decommissioning. Front. Mar. Sci. 6:200. doi: 10.3389/fmars.2019.00200
Received: 31 August 2018; Accepted: 01 April 2019;
Published: 16 April 2019.
Edited by:
Andrew James Guerin, Newcastle University, United KingdomReviewed by:
Andrew Russell Gates, University of Southampton, United KingdomDianne McLean, The University of Western Australia, Australia
Copyright © 2019 Rouse, Lacey, Hayes and Wilding. This is an open-access article distributed under the terms of the Creative Commons Attribution License (CC BY). The use, distribution or reproduction in other forums is permitted, provided the original author(s) and the copyright owner(s) are credited and that the original publication in this journal is cited, in accordance with accepted academic practice. No use, distribution or reproduction is permitted which does not comply with these terms.
*Correspondence: Sally Rouse, c2FsbHkucm91c2VAc2Ftcy5hYy51aw==