- 1BioCost Research Group, Departamento de Bioloxía, Facultade de Ciencias, Universidade da Coruña, A Coruña, Spain
- 2Instituto de Ciencias Ambientales y Evolutivas, Facultad de Ciencias, Universidad Austral de Chile, Valdivia, Chile
- 3UMI EBEA 3614, Evolutionary Biology and Ecology of Algae, CNRS, PUCCh, UACH, Station Biologique de Roscoff, Sorbonne Université, Roscoff, France
Maerl beds are one of the world’s key coastal ecosystems and are threatened by human activities and global change. In this study, the genetic diversity and structure of one of the major European maerl-forming species, Phymatolithon calcareum, was studied using eight microsatellite markers. Two sampling scales (global: North East Atlantic and regional: Galicia) were investigated and fifteen maerl beds from Atlantic Europe were sampled. At the regional-scale the location of sites outside and within four estuaries allowed to test for the influence of coastal configuration on population connectivity and genetic diversity. Results suggested that clonal reproduction plays an important role in the population dynamics of P. calcareum maerl beds. Clonality was variable among populations, even within the same region. At the European scale, these differences in clonality cannot be explained by the geographic or latitudinal distribution of the populations studied. A significant genetic differentiation was found among almost all population pairs and a positive correlation between geographic and genetic distances showed the limited dispersal capacity of P. calcareum. Moreover, a very clear pattern of genetic structure was revealed at the regional scale between populations located within and at the mouth of the estuaries. Genetic differentiation among estuaries was less marked for the sites located in outer-zones compared to those located in the inner-zones. In addition, variation in level of clonality linked to seascape was also observed: populations situated in the outer-zones of the estuaries were less clonal than those in the inner-zones. Finally, populations from the same estuary generally shared one or several mutilocus genotypes.
Introduction
The majority of plants involve both sexual and asexual/clonal reproduction (Halkett et al., 2005; Vallejo-Marín et al., 2010). The balance between the two modes of reproduction varies widely between species and even between populations of the same species, highly influencing demography and genetic structure (Eckert, 2002; Halkett et al., 2005). Most asexual reproductive modes, such as vegetative propagation, bypass meiosis and do not involve recombination. In this case, asexual offspring (ramets) are exact genetic copies of the parent genotypes (produced by sexual reproduction) with exceptions linked to somatic mutations (Harper, 1981; Eckert, 2002; Vallejo-Marín et al., 2010). All ramets produced clonally, as well as the original parental individual, are part of the same genetic unit also called a genet (Vallejo-Marín et al., 2010). In dense populations inhabiting stable environments, extensive clonal spread has been observed (Arnaud-Haond et al., 2010). Within these populations, increasing competition among genets for space or resources through time could lead to an overwhelming dominance of one or a few clonal lineages: the more ecologically competent excluding the less fit lineage (Eriksson, 1993; Arnaud-Haond et al., 2010; Becheler et al., 2014). By combining both sexual and clonal mode of reproduction, partially asexual organisms can potentially generate new recombinant genets at each generation avoiding then the accumulation of deleterious mutations while being able to maintain the best performing genotypes over time scales far exceeding sexual generation times (Otto and Lenormand, 2002; Arnaud-Haond et al., 2012). Asexual reproduction has been reported to be more important at the limit of the species range distribution, where adverse environmental conditions can limit sexual reproduction referred to as geographic parthenogenesis in Kearney (2005). In brown, red, and green macroalgae, ramets constitute free-living forms after thallus fragmentation (Fucus: Johannesson et al., 2011; Agarophyton, previously known as Gracilaria: Guillemin et al., 2008; and Caulerpa: Ceccherelli and Cinelli, 1999). These drifting fragments (mature or vegetative) are considered as the major mechanism for long distance dispersal in macroalgae (review in Macaya et al., 2016).
Traits linked to the reproductive system and migration ultimately generate the spatial pattern of genotype distributions, hence influencing the intraspecific competition for resources utilization and the species capacity to buffer negative impacts of stochastic environmental changes. Estimating the rate of sex is central in predicting the resilience of partially asexual organisms since different evolutionary consequences are expected in asexual and sexual populations after large perturbations (Hörandl, 2006). Resilience of asexual populations could be favored on the short term since large clones have a higher probability to survive bottlenecks and high clonality may somewhat buffer the loss of variation linked to severe population size reduction (Diaz-Almela et al., 2008). On the contrary, sexual populations in which genetic diversity could be quickly replenished, could show better resilience on a long term scale than asexual ones (Hörandl, 2006). Information about genetic diversity, population connectivity, and the impact of the reproductive system on genotype assemblages is then a prerequisite to assess population resilience to climatic changes or the direct impact of human activities and design effective management plans for keystone species (Gaines et al., 2010; Segelbacher et al., 2010). The use of genetic data has been shown to be of paramount importance in defining optimal number and size of Nature Reserves (Soulé and Simberloff, 1986) and establish long term management practices and ecological restoration programs (Schwartz et al., 2007).
In the marine realm, the maerl beds (also known as rhodolith beds) function as autogenic ecosystem engineers (Barbera et al., 2003). The complex nature of the three-dimensional calcified habitat structure generated by the maerl forming species host a large variety of organisms, the associated communities presenting an exceptional biological and functional diversity (Foster, 2001; Barbera et al., 2003; Grall et al., 2006; Peña et al., 2014a). Maerl beds provide a unique habitat to many commercially important crustacean, bivalve and fish species (Grall and Hall-Spencer, 2003; Grall et al., 2006) and can be seen as one of largest stores of carbon in the biosphere (Birkett et al., 1998). Worldwide, maerl-forming species are threatened due to anthropogenic disturbances (e.g., large-scale extraction, dredging, destructive fishing, and fish and mussel farming) as well as global climatic changes (e.g., ocean warming and acidification; Barbera et al., 2003; Grall and Hall-Spencer, 2003; Peña and Bárbara, 2008; Nelson, 2009; Brodie et al., 2014). Rhodolith beds regression would presumably result in more unfavorable conditions for many benthic organisms due to the loss of habitat and food resources (Airoldi et al., 2008; Cloern et al., 2016). Maerl-forming species are partially asexual organisms that combine the characteristic complex sexual life cycle of haploid-diploid red algae with vegetative fragmentation (see Figure 1). The importance of sexual vs. asexual reproduction varies between species and even between regions within species; and some authors proposed that for the most common species forming maerl beds along the temperate coast of North European (NE) Atlantic, asexual reproduction by fragmentation is the main mechanism of population renewal and maintenance (Bosence, 1976; Irvine and Chamberlain, 1994; Peña et al., 2014b; Pardo et al., 2017). Maerl lifespan can reach hundreds of years (i.e., living rhodolith’s age estimated between a few hundred years up to thousand years, depending on the species and methodology used; Littler et al., 1991; Foster, 2001; Goldberg, 2006; McConnico et al., 2014) and populations of secular clones could exhibit low resilience to disturbances (McConnico et al., 2014). Despite their ecological significance, the maerl bed’s dynamics of recruitment, maintenance, and main mechanisms of dispersion and colonization are not well understood. In particular, knowledge on the impact of clonal propagation, scales of dispersal, and gene flow on spatial genetic diversity of these habitat-forming algae is required to develop relevant management strategies. Moreover, habitat differences such as those found between estuarine and open sea habitats may also be important features shaping the pattern of genetic differentiation at a local scale (as for example in a fucoid alga: Coleman et al., 2018).
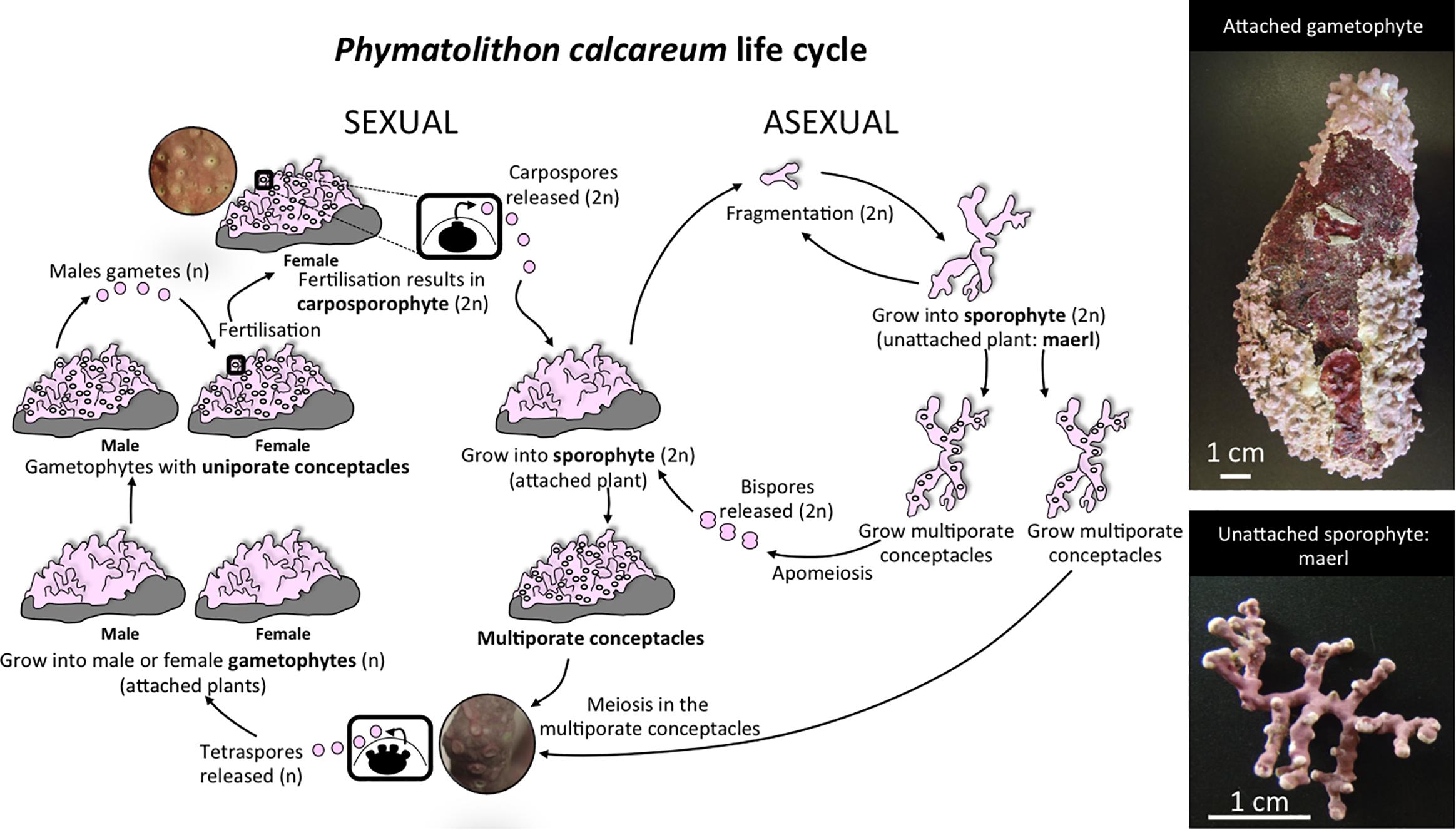
Figure 1. The typical life cycle of maerl-forming species, including Phymatolithon calcareum. Sexual reproduction typically involves an alternation between diploid sporophytes and haploid male and female gametophytes. Regardless of their phase or sex, all spore recruits grow into encrusting-forms. The sporophyte (2n) produces, through meiosis, tetraspores (n) that are released and further develop as gametophytes. Male gametophytes (n) produce gametes by mitosis that are released and fertilize the gametes of female gametophytes (n) produced in uniporate conceptacles. Fertilization results in the formation of a zygote, which undergoes a complex series of changes that ultimately result in a diploid carposporophyte, retained on the female thallus. Carposphorophyte produces carpospores (2n) mitotically, which develop as sporophytes when they are released, completing the sexual cycle. Branches of encrusting growth-forms can break and grow into large unattached thalli that characterize the maerl (generally only reports of sporophytic maerl plants, Cabioch, 1969, 1970; Mendoza and Cabioch, 1998; Pardo et al., 2017). Fragmentation of branches from free-living maerl thalli can also occur enhancing the sporophyte vegetative reproduction (Bosence, 1976; Peña et al., 2014b; Pardo et al., 2017). Attached and unattached sporophyte thalli can also reproduce asexually by apomeiotic uninucleate bispores (Irvine and Chamberlain, 1994). Photos of an attached gametophyte (specimen with sexual uniporate conceptacles from Molène Archipelago described in Pardo et al., 2017, photograph by Cristina Pardo) and an unattached sporophyte (specimen with sexual multiporate conceptacles from Morlaix used in our study, photograph by Ignacio Bárbara) are given in the upper right hand corner and the lower right hand corner, respectively. Close-up from sexual uniporate conceptacles and multiporate conceptacles are given in circular inserts within the sexual life cycle. Please note that sporophytes of red algae producing tetraspores are commonly named tetrasporophytes in the literature.
Among the NE Atlantic maerl beds, recent assessments using molecular approaches detected up to ten maerl-forming species (Peña et al., 2011, 2015; Pardo et al., 2014a, 2017; Hernández-Kantún et al., 2015). Most species show a restrained geographic distribution, while Phymatolithon calcareum (Pallas) W. H. Adey and D. L. McKibbin is confirmed as one of the most widespread builder of maerl beds in the temperate waters of this area (from Norway to the Iberian Peninsula, Carro et al., 2014; Pardo et al., 2014a). The existence of cryptic species within the same maerl bed (e.g., Carro et al., 2014) and the lack of genetic markers available for these non-model plants have, to date, restrained our capacity to develop population genetic studies. The main objective of our work was to determine the impact of clonal propagation on diversity and genotypic assemblage in P. calcareum populations sampled from fifteen sites along the temperate NE Atlantic coast, using eight microsatellites developed by Pardo et al. (2014b). In species with haploid-diploid life cycle, high rate of asexual reproduction can lead to an overdominance of one of the phases (Guillemin et al., 2008; Krueger-Hadfield et al., 2016). The level of clonality of P. calcareum populations was estimated by combining direct observations of reproductive structures, appraisal of the sporophytes / gametophytes ratio and classical estimates of genetic and genotypic diversity (Eckert, 2002; Arnaud-Haond et al., 2007). Our sampling covers the main distribution area of this maerl-forming species, from the British Isles to the Iberian Peninsula. In this study, we aim to answer the following questions: (i) what is the level of clonality of P. calcareum populations and its variation among populations?; (ii) what is the effect of clonality on the genetic diversity and the genotypic assemblage at a large scale, and is there any geographical trend linked to latitude?; (iii) at the local scale of the NW Iberian Peninsula (Galicia), how is the seascape shaping the patterns of genetic and genotypic differentiation within and among estuaries?
Materials and Methods
Model Species
Phymatolithon calcareum is a non-geniculate coralline red alga encountered along the coast of the North Atlantic (from Norway to Atlantic Iberian Peninsula) and Mediterranean Sea. It is further considered one of the major maerl species, forming vast beds in temperate/cold waters within its distribution range (Pardo et al., 2014a) and is also listed as species whose exploitation requires management (in Annex V of Eriksson and Fröborg, 1996). The species’ complex sexual life cycle involves an alternation between two types of independent functional individuals: the diploid sporophyte and haploid, dioicious gametophytes (Figure 1). Phymatolithon calcareum is also able to reproduce asexually via vegetative fragmentation as well as more rarely by apomeiotic uninucleate bispores (Figure 1). All spores (haploids or diploids) grow into encrusting-forms (Figure 1) and even though the formation of bispores has also been reported in maerl-forming species, the presence of crusts has generally been associated with the occurrence of sexual reproduction in this species (Cabioch, 1969, 1970; Mendoza and Cabioch, 1998). While there are reports of fertile plants (both gametophytes and sporophytes) in the Mediterranean (Bressan and Babbini, 2003; Wolf et al., 2016), along the North European Atlantic (NE Atlantic), crustose forms of P. calcareum are very rare and have so far almost exclusively been observed in the French region of Brittany, growing as small epilithic crusts on pebbles or dead maerl (Mendoza and Cabioch, 1998; Peña et al., 2014b; Pardo et al., 2017). North European Atlantic maerl beds of P. calcareum are typically composed of free-living diploid sporophyte thalli that originated from broken branches initially growing on tetrasporophyte crusts (Figure 1). Maerl plants continue to grow, fragment, and multiply during their life with occasional production of tetrasporangial conceptacles (Cabioch, 1969, 1970; Bosence, 1976; Irvine and Chamberlain, 1994; Mendoza and Cabioch, 1998; Peña et al., 2014b; Pardo et al., 2017) (Figure 1). Further, the low abundance of encrusting individuals or free-living rhodoliths bearing reproductive organs have led to the conclusion that P. calcareum rhodolith beds were highly clonal and asexual reproduction by fragmentation of the own free-living maerl plants has been proposed as the main mechanism of their population renewal and maintenance (Bosence, 1976; Irvine and Chamberlain, 1994; Peña et al., 2014b; Pardo et al., 2017).
Sampling, DNA Extraction, Molecular-Based Species Detection and Genotyping
Maerl samples were collected along the NE Atlantic coasts between February and July 2011 in fifteen maerl beds distributed from the British Isles to Southern Portugal; eight of them located within protected areas (e.g., Natura 20001) (Supplementary Table S1). To assess the pattern of genetic and genotypic diversity at different spatial scales, a hierarchical sampling design was applied. At the scale of the North Atlantic, four different geographic regions were defined according to the principal biogeographic provinces delimited in Spalding et al. (2007): the British Isles, Atlantic France, Atlantic Spain, and Southern Portugal (Supplementary Table S1). Except for South Portugal, three to four areas were sampled within each region: three areas in the British Isles (Northern Ireland, Wales, and England), three areas in Atlantic France (Northern Brittany, Western Brittany, and Southern Brittany), and four areas in Atlantic Spain (Ría de Muros e Noia, Ría de Arousa, Ría de Pontevedra, and Ría de Vigo) (Supplementary Table S1 and Figure 2). Southwards, maerl beds composed of P. calcareum are found in the Algarve region, where only one bed could be sampled (Carro et al., 2014; Pardo et al., 2014a) (Supplementary Table S1 and Figure 2). At the regional scale of Galicia, sampling was performed in the outermost zone (hereafter referred to as “outer-zone”) and the middle sector (hereafter referred to as “inner-zone”) of four estuaries (Figure 2). In our study, the sampled outer-zones were San Francisco, Barbafeita, Illa de Ons, and Illas Cíes; and the sampled inner-zones were Bornalle, Benencia, Tulla and Con de Pego (in Ría de Muros e Noia, Ría de Arousa, Ría de Pontevedra, and Ría de Vigo, respectively; Figure 2 and Supplementary Table S1).
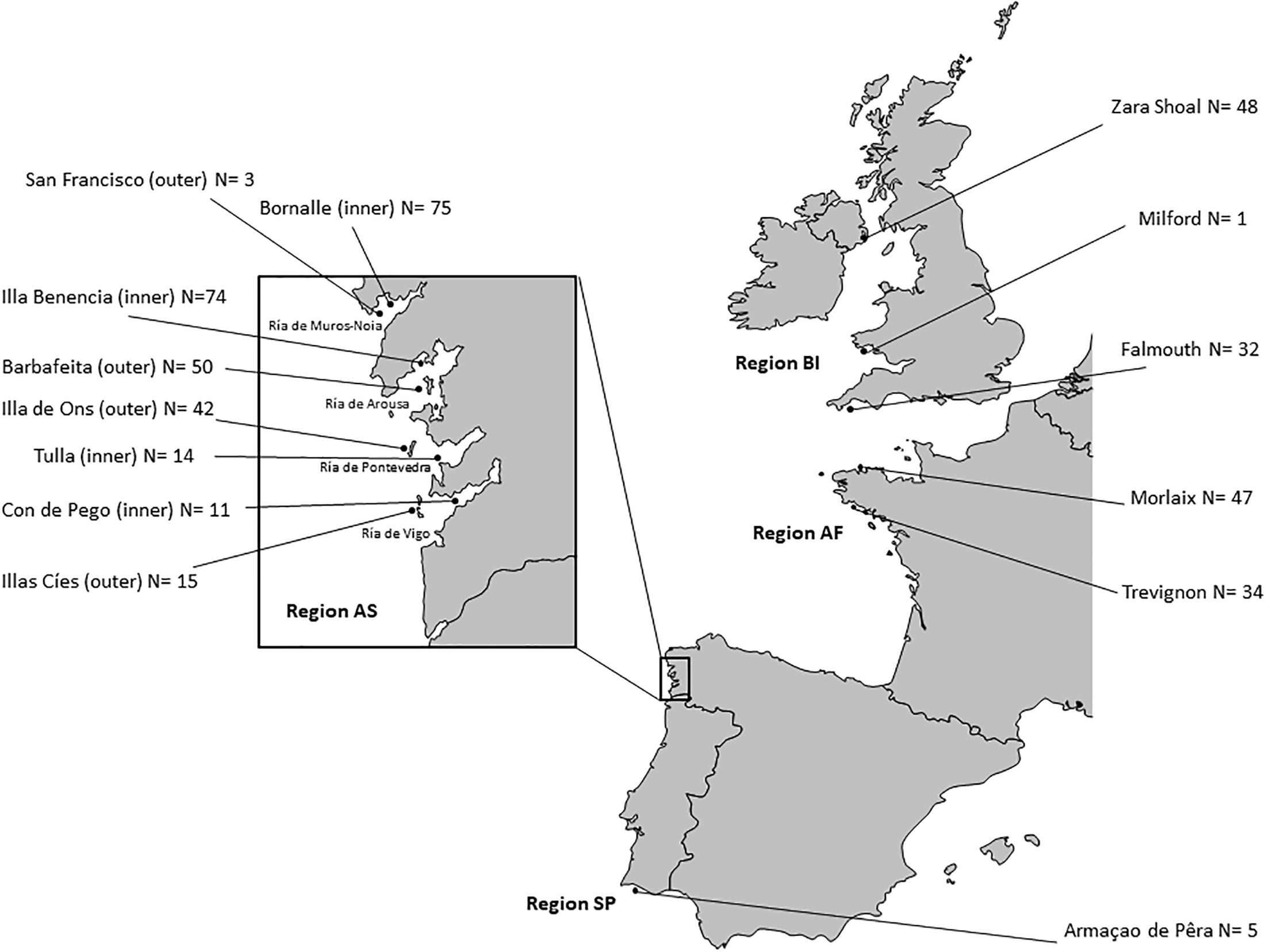
Figure 2. Studied maerl beds, where Phymatolithon calcareum samples were collected and successfully scored for eight microsatellite markers (N, number of samples).
Maerl samples were collected by scuba diving at 3.9–20 m of depth. At each site, the sampling design consisted of 12–16 sampling points separated by 1–3 m along a linear transect in the maerl bed (i.e., a total transect length of 14–50 m). In each sampling point of the linear transect, three to six samples were collected randomly. The number of maerl samples collected per transect varied depending on the maerl bed’s density. Once in the laboratory, specimens were air-dried and stored in silica for later observation and DNA extraction. Each individual was observed under a stereomicroscope and the presence of uniporate and multiporate conceptacles was used to define the phase (i.e., haploid gametophyte or diploid sporophyte; Figure 1). Genomic DNA extraction was performed using the NucleoSpin 96 Tissue kit (Macherey-Nagel, Düren, Germany) following the manufacturer’s protocol. For all samples, a fragment of 950 base pairs (bp) of the plastidial gene photosystem II reaction center protein D1 (psbA) was amplified using the primers psbA-F1 and psbA-R2 from Yoon et al. (2002). Additionally, in some specimens, a fragment of 664 bp of the 5′ end of the mitochondrial gene cytochrome oxidase I (COI-5P) was amplified using the primers GazF1 and GazR1 or GCorR3 (Saunders, 2005; Pardo et al., 2014a). PCR conditions and thermal profiles followed those in Saunders and McDevit (2012) and Pardo et al. (2014a). Comparisons of the psbA and COI-5P sequences to the DNA barcodes available for the neotype specimen (voucher BM000712373, Pardo et al., 2014a; Peña et al., 2014b) were used to identify P calcareum samples.
Eight microsatellite loci developed by Pardo et al. (2014b) were amplified in two multiplex reactions for all samples identified as P. calcareum. Multiplexed PCR products were genotyped on an Applied Biosystems 3730XL DNA analyzer at Macrogen (Seoul, Korea) and scored using the GeneMarker® v1.7.0 software (SoftGenetics, State College, PA, United States). The raw data supporting the conclusions of this manuscript are available in Supplementary Table S2 and can also be downloaded from Dryad (doi: 10.5061/dryad.ds2714g). Prior to analyses, raw data were checked for null alleles using a maximum-likelihood estimator with the software ML-NULLFREQ (Kalinowski and Taper, 2006).
Clonal Diversity
First, in order to assess the capacity of the eight microsatellite loci genotyped to discriminate all possible distinct MultiLocus Genotypes (MLGs) in each site, a jackknife resampling of loci (1000 permutations) was used to estimate the average number of distinct MLGs that can be detected using the GENECLONE v.2.0 software (Arnaud-Haond and Belkhir, 2007). Herewith, the accumulation curve of the clonal diversity (R; genotypic diversity index; calculated as R = (G – 1)/(N – 1), with G being the number of MLGs detected in the population and N the total number of individuals sampled; Dorken and Eckert, 2001) given the number of loci genotyped was plotted. The level of clonality within each site was estimated by calculating the number of MLGs and their Psex using the GENECLONE v.2.0 software (Arnaud-Haond and Belkhir, 2007), where Psex is the probability that a given MLG detected in N samples derived from distinct sexual reproductive events (i.e., thus actually belonging to different genets) (Arnaud-Haond et al., 2007). In order to limit overestimation of events of asexual reproduction in the investigated maerl beds, identical MLGs were considered as ramets of the same genet only when Psex fell below a threshold value fixed at 0.05. Indeed, for molecular markers with limited polymorphism and low statistical power, some distinct lineages could falsely appear identical (Arnaud-Haond et al., 2007). The genotypic diversity (Rgenets) was calculated only taking into accounts the different genets as Rgenets = (Ggenets – 1)/(N – 1), with Ggenets being the number of genets detected in the population (including all repeated MLGs with Psex > 0.05) and N the total number of individuals sampled (Dorken and Eckert, 2001). Additionally, the effectiveness of our sampling effort to properly estimate the genotypic diversity in P. calcareum beds was tested using a resampling procedure where R values were estimated for multiple random combination of sampling units, for sample sizes ranging from 1 to N (N being equal to 75, the maximum sampling size within the data set; Bornalle, Table 1). The rate of change in R with increasing sampling size N was then plotted to estimate the number of sampled individuals required to yield R values approaching the asymptote (Arnaud-Haond and Belkhir, 2007).
Genetic Diversity and Structure
Note that all analyses of genetic diversity and structure were only carried out for sites with N ≥ 10 P. calcareum (11 sites, Table 1) and that the site of Falmouth was not included since specimens sampled in this site were possibly triploids (more details are given at the beginning of the results section). Two data sets were constituted: a first a data set including all the sampled individuals (hereafter named “ramets”) and a second one where repeated MLGs with Psex lower than 0.05 were removed (hereafter named “genets”). The following analyses were implemented using both, the “ramets” and the “genets” data sets.
For each site, number of alleles (Na), number of private alleles (Nap), observed heterozygosity (Ho), and unbiased expected heterozygosity (He) were computed for each locus and over all loci using GenAlEx v.6.501 (Peakall and Smouse, 2012). Allelic richness (AR) was estimated with a rarefaction approach using the software HP-RARE v.1 (Kalinowski, 2005). Estimations of AR were calculated standardizing the sample size to N = 11 for the “ramets” and N = 4 for the “genets” data set.
For each population, linkage disequilibrium (LD) was assessed using the association index in MultiLocus v.1.2. (Agapow and Burt, 2001). To test departure from random associations between loci, the observed dataset was compared with 1000 simulated datasets, in which recombination was imposed by randomly reshuffling the alleles, independently for each locus, among individuals (Agapow and Burt, 2001). Tests for departure from Hardy-Weinberg equilibrium were performed using FSTAT v.2.9.3.2 (Goudet, 1995). FIS was calculated for each locus and over all loci according to Weir and Cockerham (1984) and significance was tested by running 1000 permutations of alleles, among individuals, within samples. To investigate population differentiation, Weir and Cockerham’s unbiased measure of FST was estimated over all loci using FSTAT v.2.9.3.2 (Goudet, 1995). To test isolation by distance (IBD) a Mantel test was carried out using 999 permutations in GenAlEx v.6.501 (Peakall and Smouse, 2012). As suggested by Rousset (1997) for sampling schemes undertaken in at a two-dimensional scale, FST / (1- FST) was plotted against the logarithm of the geographical distance between each populations pair.
At the regional scale of Galicia, a nested analysis of molecular variance (AMOVA) was implemented using ARLEQUIN v.3.1 (Excoffier et al., 2005) to test for the partition of genetic variance within sites, between sites within estuaries, and among estuaries. In addition, differences between sites located in the outer, and inner-zone of the estuaries were tested for several genetic measures (R, AR, Ho, He, and FIS) using non-parametric Mann–Whitney tests with STATGRAPHICS2.
Results
Of the 1142 maerl plants sequenced, 455 samples were identified as P. calcareum. The percentage of P. calcareum observed was very variable depending on the site under study, ranging from 96% in Benencia (Galicia) to 0% in Brest (Western Brittany) (Supplementary Table S1).
Most of the P. calcareum plants were vegetative even if some sporophytes (44 over the 455 studied, 10%, Supplementary Table S2) were identified thanks to the occurrence of sexual structures (i.e., presence of multiporate conceptacles, see Figure 1). No mature gametophytes, characterized by uniporate conceptacles (Figure 1), were detected. Of the 455 P. calcareum individuals, 447 were successfully scored for the eight microsatellite loci (Table 1) and diploid individuals (i.e., sporophytes) were defined as individuals showing a heterozygous genotype for at least one locus (Guillemin et al., 2008; Krueger-Hadfield et al., 2016). Non-mature plants were all characterized by genotypes showing at least one heterozygous locus and were classified as sporophytes (Supplementary Table S2). All populations, except Falmouth, were composed exclusively of diploid sporophytes. All specimens collected in Falmouth (32 plants, Table 1 and Supplementary Table S1) displayed a triploid profile in three of the eight loci scored (PC-2, PC-3, and PC-7, Pardo et al., 2014b) and were considered as possible triploid sporophytes. In Falmouth, clonal diversity (G and R) was estimated by directly using the triploid data set (Menken et al., 1995).
Missing Data and Power of Clone Detection of the Eight Microsatellites Genotyped
Of all the individuals genotyped, only four did not amplify at all eight microsatellite loci and were not included in our analyses (Table 1). One individual did not amplify for the locus PC-8 while three individuals did not amplify for the locus PC-6 (Supplementary Table S2). When only considering the individuals scored at every locus, results from ML-NULLFREQ (Kalinowski and Taper, 2006) showed evidence of null alleles at the loci PC-6 (null allele frequency = 20.58%) and PC-8 (null allele frequency = 24.41%). Even if missing data can have an impact on the estimation of genotypic frequencies (Gourraud et al., 2004), we chose to keep all loci in the subsequent analyses since (i) our capacity to detect distinct MLGs was limited at some sites, even when all eight microsatellite loci were included (see Supplementary Figure S1), and (ii) it has been demonstrated that including loci with low to moderate frequencies of null alleles (i.e., <20–30%) does not profoundly affect classical and Bayesian population genetic analyses of population structure (Séré et al., 2014).
The power of the eight microsatellite loci genotyped to discriminate all possible distinct MLG was sufficient at five sites of the study area (Zara Shoal, Illa de Ons, Illas Cies, Tulla, and Bornalle; Supplementary Figure S1), as the accumulation curve of the clonal diversity reached a plateau at values lower than eight. In contrast, the curve obtained for three sites (Barbafeita, Benencia, and Falmouth; Supplementary Figure S1) did not show the typical asymptotic growth nor a plateau. Likewise, in Con de Pego, Morlaix, and Trevignon (Supplementary Figure S1) the accumulation curve did not reach a plateau even when using the eight loci available. Therefore, it is possible that our estimation of clonal diversity in these last six sites may be less accurate.
In sites where more than ten maerl plants of P. calcareum were sampled (11 sites), the number of MLGs varied from two (Falmouth, 32 samples) to 33 (Zara Shoal, 48 samples) (Table 1). For most repeated MLGs, Psex values were lower than 0.05, suggesting that a high number of them were ramets of the same genet (Supplementary Table S2). However, in the six sites where the power of the eight microsatellites genotyped for MLG distinction was low (Barbafeita, Benencia, Falmouth, Con de Pego, Morlaix, and Trevignon; Supplementary Figure S1), some repeated MLGs had Psex values higher than 0.05 and were considered as the possible outcome of sexual reproduction and kept as different genets (Table 1 and Supplementary Table S1). Except for Benencia, within the aforementioned sites, only a few repeated MLGs show Psex > 0.05, and our estimation of clonal diversity is likely unaffected (Table 1). In Benencia, 17 copies of repeated MLGs could not be considered to come from asexual reproduction (Table 1).
Clonal Identity
In two sites where less than ten maerl plants of P. calcareum were sampled (Armaçao de Pêra in South Portugal and San Francisco in Galicia), all samples belonged to distinct MLGs (Table 1). In the eleven sites where more than ten P. calcareum were sampled, a typical signature of clonality was observed with a number of genets much lower than the total number of samples (Table 1). Zara Shoal and Illa de Ons showed a relatively high clonal diversity (R = Rgenets = 0.681 and 0.634, respectively), while the site of Falmouth was the less clonally diverse, with a R of only 0.032 (Table 1). Because of the lack of power of our markers, in Benencia, its clonal diversity was more difficult to estimate and the value of Rgenets was six times higher (0.274) than the value of R (0.041) (Table 1). Clonal diversity was highly variable within regions and even within estuaries (for example, in Ría de Pontevedra, Rgenets from Illa de Ons is three times higher than from Tulla, Table 1).
Interestingly, our study revealed that in seven cases two MLGs within the same site were differentiated by a single mutational step and that in three cases these pairs of MLGs belonged each to one rare genotype and one frequent one (Supplementary Table S2). In Falmouth, the difference between the rare MLG (N = 1) and the frequent MLG (N = 31) occurred at a locus where three alleles were detected (PC-3; Supplementary Table S2).
Most MLGs, were private to one sampling site (Figure 3). Only four MLGs were shared between sites and generally these were only encountered among close by sampling sites located in Galicia (Figure 3). MLG015 was the most commonly sampled genotype (sampled 103 times, equivalent to 23% of all genotyped P. calcareum individuals) and the only MLG encountered in two biogeographic provinces: Atlantic Spain and Atlantic France (Figures 2, 3).
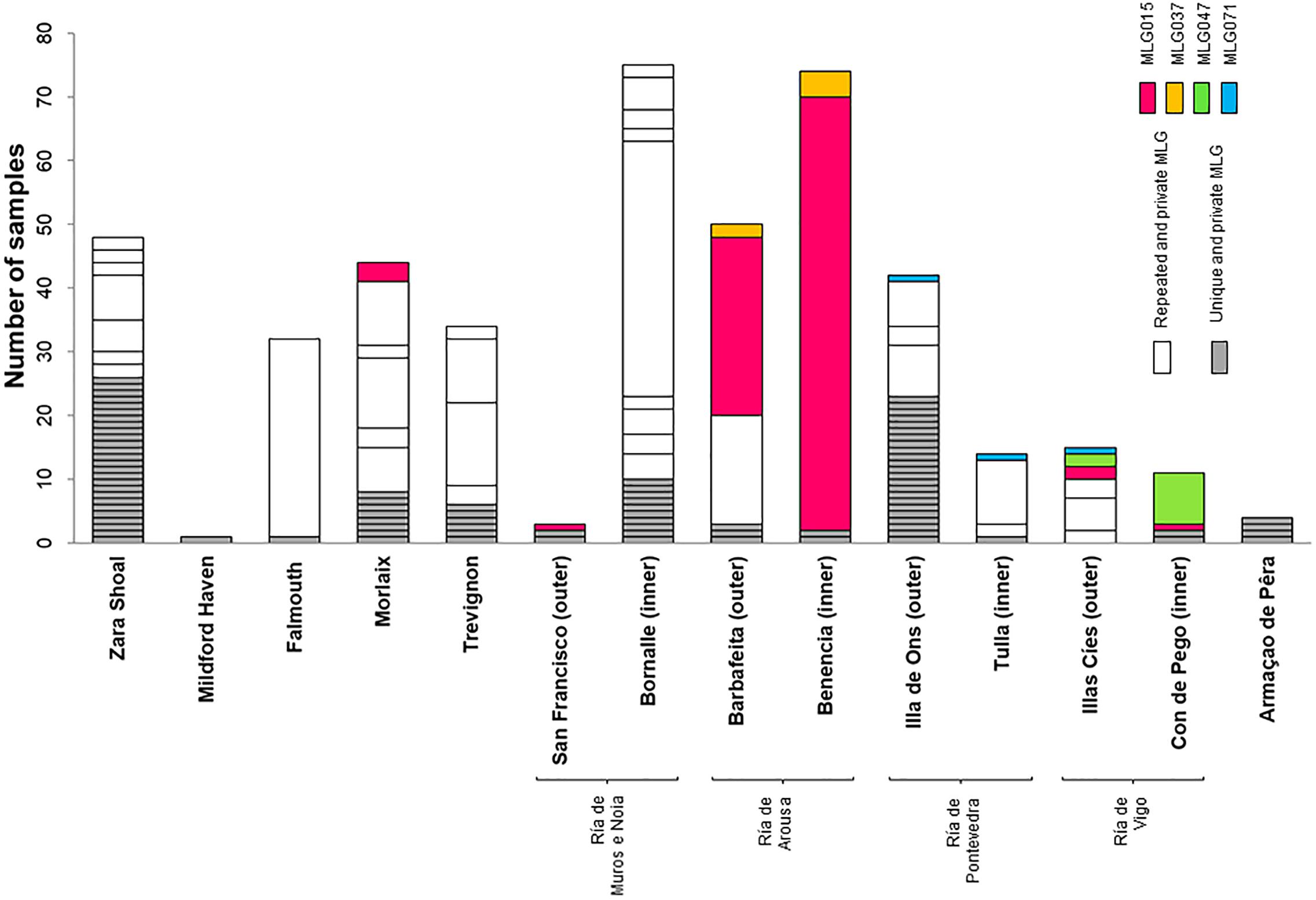
Figure 3. MultiLocus Genotypes (MLGs) observed for eight microsatellite markers in Phymatolithon calcareum from fourteen study sites. Private and unique MLGs are shown in gray while private repeated MLGs and are shown in white. The different colors represent MLGs shared between sites.
Genetic Diversity and Structure of the Maerl Beds at the Two Spatial Scales Studied
All data on multilocus genetic diversity estimates are given in Table 2. No clear patterns of latitudinal variation of genetic and genotypic diversity were observed at the scale of the NE Atlantic. The mean number of alleles varied between 1.63 and 3.50, and the highest values (Na > 3.00) were found in Trevignon, Illa de Ons and Illas Cíes (Table 2). The higher values of allelic richness (AR) were observed in Illa de Ons and Illas Cíes, when all individuals were included in the analyses (“ramets” data set; AR > 3.00, Table 2). Levels of observed heterozygosity (Ho) were usually higher than unbiased expected heterozygosity (He) and the highest values of Ho were detected in Galicia, in the populations from Tulla and Illas Cíes (Table 2). Significant excess of heterozygotes was indeed detected in five sites when all individuals were included in the analyses (“ramets” data set; Trevignon, Barbafeita, Benencia, Tulla, and Con de Pego; Table 2). If only genets were taken into account, FIS values retrieved were higher than those for the “ramets” data set at all sites, and significant excess of heterozygotes was detected in Trevignon and Benencia (“genets” data set; Table 2). Among ramets, LD values were significant for all sites except Benencia, while among genets, roughly half of the study sites showed significant LD (; Table 2). When all these analyses were performed without the two loci with a non-negligible frequency of null alleles (PC-6 and PC-8), the results did not change substantially except for the fact that all FIS were negative and significant, and all values were significant among the ramets data set (data not shown).
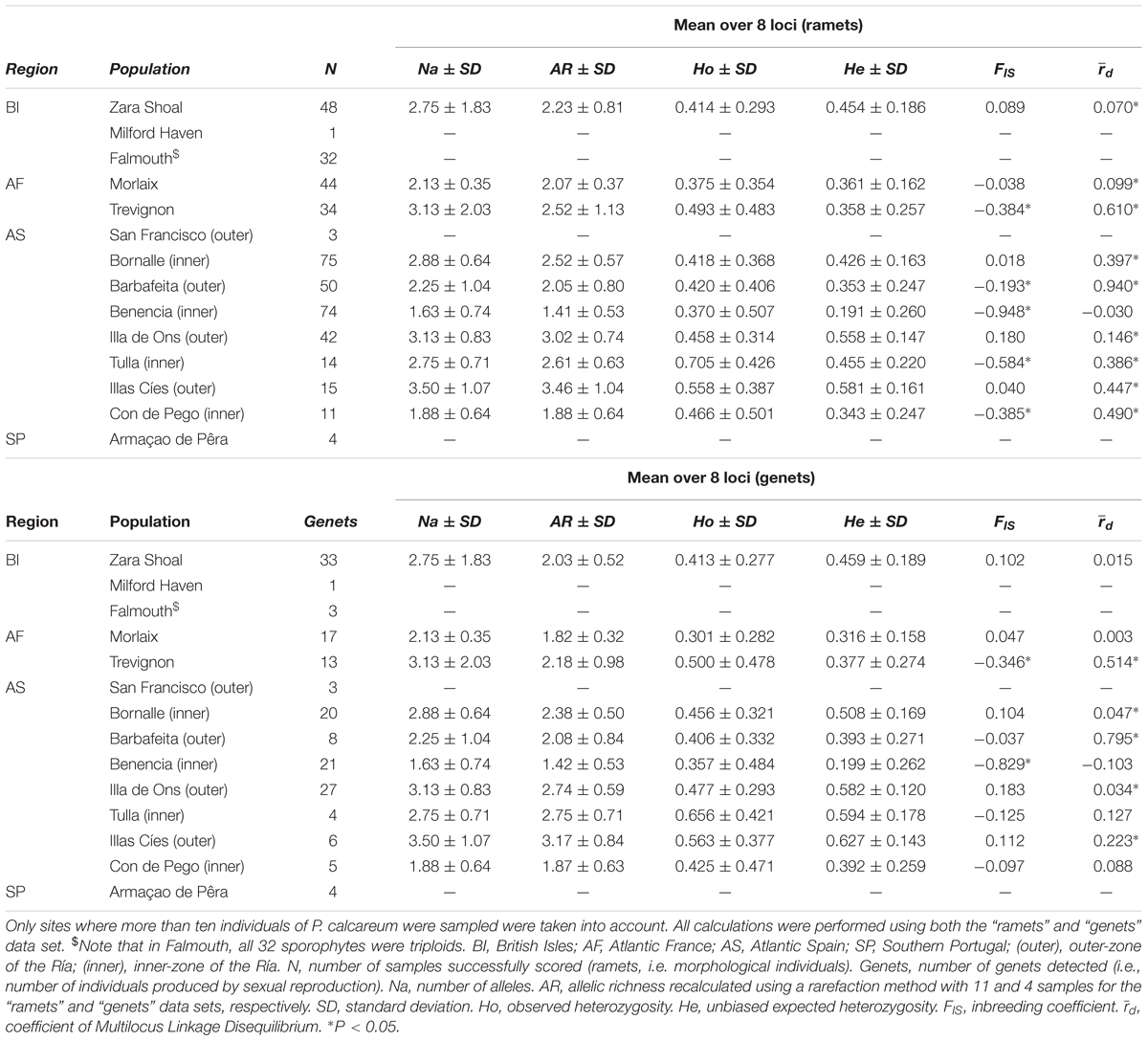
Table 2. Genetic diversity estimates, inbreeding coefficient and linkage disequilibrium calculated for eight polymorphic microsatellite markers in ten sites where Phymatolithon calcareum were sampled along the Atlantic coasts of Europe.
Private alleles were encountered in all regions except in Atlantic France and the percentage of private alleles was very high in Southern Portugal (12.5% of private allele; Supplementary Table S3). For the “ramets” data set, all pairwise FST values calculated among sites were significantly different from zero, and ranged from 0.11 to 0.61 (Supplementary Table S4A). Similarly, FST values calculated among sites using the “genets” data set were all positive and significant, except among some sites sampled in Galicia and between Tulla and Trevignon (Supplementary Table S4B). Concerning the relationship between genetic and geographical distance, significant patterns of IBD were observed for both, the “ramets” (Mantel test, P = 0.01) and the “genets” data set (Mantel test, P = 0.0001) (Supplementary Figures S2A,B).
At the local scale of Galicia, clear differences in genetic diversity and structure were observed between sites located in the outer and in inner-zones of the estuaries (Table 2 and Figure 4). First, for both data sets, parametric Mann–Whitney tests (P < 0.05) showed a significantly higher allelic richness in the outer-zones (“ramets” AR = 2.838 ± 0.211; “genets” AR = 2.663 ± 0.175), than in the inner-zones of the estuaries (“ramets” AR = 1.975 ± 0.156; “genets” AR = 2.008 ± 0.168). Expected heterozygosity (He) was also significantly higher (Mann-Whitney test, P < 0.05) in the outer than in the inner-zones of the estuaries for both data sets (“ramets” He = 0.497 ± 0.043 and 0.330 ± 0.052; “genets” He = 0.534 ± 0.043 and 0.395 ± 0.057; results given for the outer and inner-zones, respectively). The FIS values showed a clear excess of heterozygotes only in the inner-zones (inner-zones: “ramets” FIS = -0.396 ± 0.168, “genets” FIS = -0.227 ± 0.158; outer-zones: “ramets” FIS = 0.021 ± 0.116, “genets” FIS = 0.070 ± 0.111), and differences between the outer and inner-zones were significant in both data sets (Mann–Whitney test, P < 0.05). The mean FST values between sites from the same estuary and between outer estuarine sites were low (FST < 0.176; Figure 4). On the other hand, FST values between inner estuarine sites were much higher (FST = 0.456; Figure 4). The results of the AMOVA showed that genetic variation among estuaries (≈19 and 16%; “ramets” and “genets” data set, respectively) is slightly higher than among sites within estuaries (≈14 and 7%; “ramets” and “genets” data set, respectively) whatever the data set under study (Supplementary Tables S5A,B).
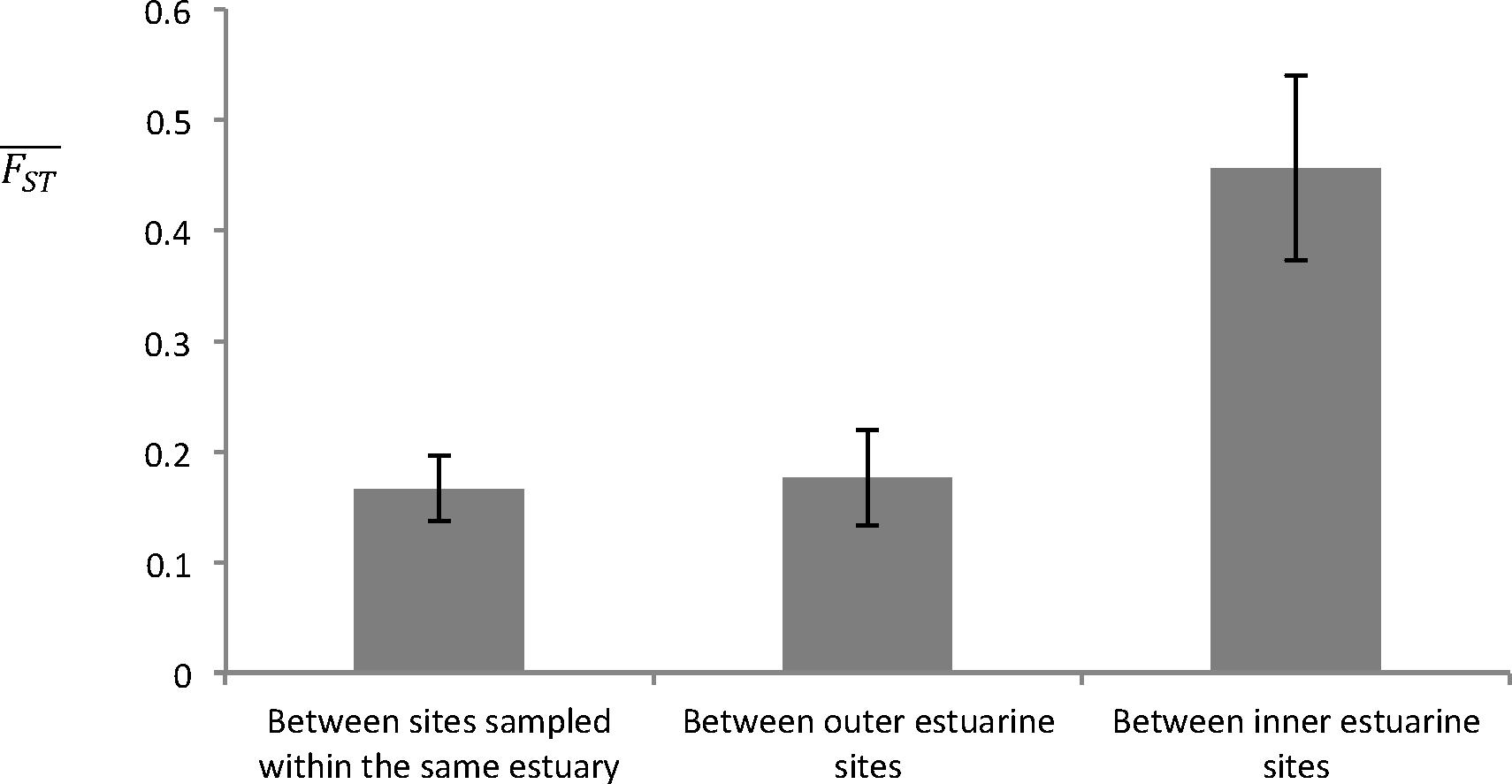
Figure 4. Mean (and SE) FST values calculated between sites within the same estuary, between outer estuarine sites, and between inner estuarine sites in Galicia using eight microsatellite loci. The two populations from the Ría de Muros e Noia, San Francisco and Bornalle, were not included since only three specimens of Phymatolithon calcareum were sampled in San Francisco (i.e., outer-zone, see Figure 2). FST values correspond to the ones calculated using the whole data set (i.e., the “ramets” data set).
Discussion
Because of the existence of various cryptic species in the maerl beds of the NE Atlantic (Pardo et al., 2014a), Phymatolithon calcareum specimens cannot be identified using taxonomical criteria based on morphology. This limits the ability to plan a homogeneous population genetic sampling strategy and, indeed, only a few to no specimens of P. calcareum could be collected at four of the fifteen sampled sites. Notwithstanding this limitation, our results show clear signature of clonality affecting both the maerl beds population dynamics (i.e., all beds dominated by the tetrasporophytic phase of the life cycle) as well as genetic diversity and structure. High levels of genetic differentiation suggest that recruitment occurs mostly locally with dispersal being limited by geographic distance but also by coastal configuration and water dynamics. Nevertheless, some rare long-distance dispersal events may have spread clones of P. calcareum over larger areas (e.g., one clone located in two biogeographic regions). We propose a complex interplay between the outcomes of sexual/asexual reproduction, mutation, and hybridization as a possible explanation to the pattern of genetic and genotypic diversity in this maerl forming species.
Slow Accumulation of Genotypic Diversity in a Long-Lived Clonal Maerl Forming Species
The low genotypic diversity, complete absence of gametophytes, and low number of samples producing spores revealed in this study confirms that Phymatolithon calcareum propagates primarily via clonal fragmentation. The pattern of genotypic diversity was highly variable among populations confirming previous reports of geographical variation of phenotypic occurrence of sexual P. calcareum thalli (reports of fertile sporangial maerl plants or small crustose gametophytes: Cabioch, 1969, 1970; Mendoza and Cabioch, 1998; Peña et al., 2014b; Pardo et al., 2017). However, the genetic estimates of sexual/asexual reproduction provided by the current study do not vary accordingly to the frequency of sexual phenotypes reported previously. Indeed, sexually mature thalli have never been reported in some well-studied maerl beds of Galicia (Peña et al., 2014b, 2015; Pardo et al., 2017) or the British Isles (Irvine and Chamberlain, 1994); while there are few reports of phenotypic occurrence of sexual reproduction from Brittany (Cabioch, 1969, 1970; Mendoza and Cabioch, 1998; Peña et al., 2014b; Pardo et al., 2017) and these are common in the Mediterranean (Bressan and Babbini, 2003; Wolf et al., 2016). In our study, values of genotypic diversity detected in Brittany (Morlaix and Trevignon) were generally similar or lower to those measured in Galicia, a result opposite to the one expected based on direct observation of mature thalli (see Pardo et al., 2017). Maerl beds, ten meters thick, can extend over several square-kilometers. This indicates great temporal stability, lasting probably over thousands of years (e.g., Brittany; Grall and Hall-Spencer, 2003). Such temporal scale may explain why neither production of sexual structures nor sporelings recruitments has ever been observed in some maerl beds. Even if extremely uncommon, successful recruitment of new genets generated by sexual reproduction may have considerable implications on population genotypic diversity in such long-lived sexual/asexual organisms (terrestrial plants: Eriksson and Fröborg, 1996; Stehlik and Holderegger, 2000; seagrasses: Alberto et al., 2005; Arnaud-Haond et al., 2012, 2014; soft coral: McFadden, 1997). As stated by Hurst and Peck (1996), “a little sex may go a long way” and very low rates of recruitment have been shown to produce high genetic variation in long-lived clonal plant (Eriksson and Fröborg, 1996; Stehlik and Holderegger, 2000). Thus, the maintenance of substantial numbers of different genotypes in some population could be explained by the combined effects of large population size and slow renewal of the populations.
Except in Falmouth, where P. calcareum thalli were recognized as putative triploids, all samples from this species were diploid in the maerl beds studied. Moreover, six of the ten beds dominated by diploid tetrasporophytes displayed strong heterozygote excess. An uncoupling of the complex haploid-diploid life cycle and an overdominance of one of the phases (generally dominance of the diploid tetrasporophytes over the haploid gametophytes) has previously been recorded in clonal populations of red algae (Sosa et al., 1998; Guillemin et al., 2008; Kamiya and West, 2010; Krueger-Hadfield et al., 2016). In those algal populations as in other plant and animal species (Balloux et al., 2003), heterozygote excess was also detected. Even if recent theoretical studies have shown that neutral dynamics of genotype frequencies in partially clonal organism may explain transient negative values of FIS (Reichel et al., 2016), heterozygote excess in clonal organisms has classically been related to either passive accumulation of somatic mutations over time (Klekowski, 1997; Ally et al., 2008; Ardehed et al., 2015) or advantage related to heterozygosity (Balloux et al., 2003; Guillemin et al., 2008; Krueger-Hadfield et al., 2016). These three explanations (i.e., neutral transient dynamics of the FIS, accumulation of somatic mutations and heterozygote advantage) may account for the excess of heterozygotes observed in P. calcareum.
The Special Case of Triploid Clones in the Phymatolithon calcareum Bed From Falmouth
Hybridization and introgression events could be leading to changes in reproductive mode, producing new, generally obligate, asexual genotypes (Gastony, 1986; Tucker et al., 2013). Supporting this idea, in algae, hybrids of genetically distinct entities have been repeatedly associated with the formation of asexual lineages (in red algae: Kamiya and West, 2008; Kamiya et al., 2011, 2017; in green algae: Ogawa et al., 2015; and in brown algae: Coyer et al., 2006) and with sterility linked to changes in ploidy level (e.g., polyploidy and aneuploidy; Coyer et al., 2006; Montecinos et al., 2017b). Interestingly, the P. calcareum bed from Falmouth populated by putative triploids is also the population presenting the lowest genotypic diversity and of the only two genotypes identified there, one seems to have arisen from mutations and not from sexual reproduction and recombination. We, thus, propose that in P. calcareum, genetic variation results mostly from successful settlement of sexually produced genotypes and accumulation of new somatic mutations in long-lived clones but that rare events of past hybridization and introgression could have been at the origin of some obligate asexual lineages. The identification of the second potential parental species could not be deduced from our data set. In Falmouth, only two species were detected in the maerl beds, P. calcareum, and Lithothamnion corallioides (Carro et al., 2014; Pardo et al., 2017). However, other species of Phymatolithon, such as P. lamii or P. laevigatum, have also been reported in the area (Pardo et al., 2017). Resequencing of regional maerl specimens from the British Isles and Atlantic France for several unlinked genetic markers and the search for incongruence between them could be a first step to test for the existence of hybridization in Falmouth and identify the second parental species (Montecinos et al., 2017a).
Large-Scale Distribution of Genetic Diversity: Restricted Dispersal but No Clear Influence of Latitude or Historical Climatic Oscillations
At the large European scale, a significant pattern of isolation by distance was detected in P. calcareum, confirming the limited dispersal capacity of spores and gametes observed in various sexually reproducing macroalgae (Durrant et al., 2014). It seems that even low levels of gene flow, probably more through movement of small rhodoliths or detached branches than through spore recruitments, have been able to connect populations over the whole P. calcareum distribution area. Maerl beds appear to be quite patchy nowadays, but rhodolith dominated benthic communities have reached much more extensive development in the past, colonizing most of the temperate platforms (e.g., during late Miocene; Halfar and Mutti, 2005), and it is possible that stepping-stone migration between large beds could have been more common in the past.
In the NE Atlantic, P. calcareum is steadily replaced by other rhodolith forming species in both the north of the British Isles region and south of Galicia (Carro et al., 2014; Pardo et al., 2014a; Peña et al., 2015) and the most southern and northern sampling sites in the present study are located at the species distribution edges. However, we did not detect any significant pattern of variation of genotypic or genetic diversity with latitude that could be explained by geographic parthenogenesis (Eckert, 2002) or rapid poleward recolonization after the Last Glacial Maximum from genetically rich, lower latitude refugia (Maggs et al., 2008; Neiva et al., 2016). Nonetheless in macroalgae, shifts toward asexual reproduction have been detected in populations living in highly stressful environments such as in brackish waters of the Baltic Sea (Bergström et al., 2003) and at high intertidal limits and/or at range edges (Suneson, 1982; Fierst et al., 2010; Krueger-Hadfield et al., 2013; Oppliger et al., 2014). Other hypotheses, as the intermediate disturbance theory, further attempt to explain changes in genetic and genotypic diversity along species ranges (e.g., in the seagrass Zostera muelleri, Macreadie et al., 2014). Genotypic diversity is expected to be higher in habitats with intermediate disturbance levels where recurrent opening of accessible space allows for the recruitment of new seedlings. In the NE Atlantic, differences in recurrence intervals of hurricane-strength storms or surge-events (Hickey, 2011) may explain in part the high variability in genotypic diversity among our sampling sites.
Successive expansion and contraction events occurring during the Pleistocene have led to a gradient in genetic diversity among most marine species distributed along the NE Atlantic coasts, with high genetic diversity and distinctiveness characterizing populations at the rear edge (i.e., Iberian Peninsula and Algarve) and high genetic homogeneity at the leading edge (i.e., British Isles) (Maggs et al., 2008; Neiva et al., 2016). The sampling site of Armaçao de Pêra, located in Algarve and corresponding to the southernmost edge of P. calcareum’s distribution in the Atlantic (Carro et al., 2014; Pardo et al., 2014a), presented high genotypic diversity and high numbers of private alleles. This result may be related to long-term stability and isolation of the maerl beds located in South Portugal (the potential rear edge) during the past glacial and interglacial climatic oscillations. However, no latitudinal gradient of genetic diversity could be identified in P. calcareum since most northern beds did not have reduced genetic diversity when compared to the ones from the Iberian Peninsula.
Processes Driving Clonal Distribution at Small Scales: Coastal Configuration, Currents, and Storm Incidence
Most of the clones observed in the present study were restricted to one site. This aggregated distribution of clones supports direct observations made by Cabioch (1970) in Brittany proposing that ramets coming from fragments of overgrown rhodoliths mostly accumulate locally and are not necessarily rapidly swept away from their parental plants by currents. Field observations and experimentations have shown that rhodoliths are easily moved over scales of tens of centimeters by wind-propagated waves or disturbances due to bioturbation caused by fish and invertebrates, and can even be transported over meters by swell generated by strong winds (Marrack, 1999 and references therein). Anthropogenic activities such as bivalve dredging are known to alter the structure complexity of maerl beds in the NE Atlantic (Barbera et al., 2003) and could also facilitate the local spread of rhodoliths (but see Hall-Spencer et al., 2003).
At the regional scale, coastal topography and hydrodynamics (waves, tides, and currents) seem to control the rate of recruitment of new sexual or asexual propagules and the gene flow between estuarian and coastal populations. The more protected inner-zones of estuaries were characterized by lower genetic and genotypic diversity than coastal outer-zones. This pattern could be linked to a lower rate of recruitment of sexual seedlings in the inner-zones of the estuaries and is indeed concordant with the higher excess of heterozygotes detected in these populations (expected for predominantly asexual populations; Balloux et al., 2003). In the region of Rías Baixas, connectivity along the coast was reduced between populations located in the inner zone compared to those of the outer-zone. Strikingly similar results have been reported in a brown alga living both inside and outside estuaries along the Australian coast (Hormosira banksii, Coleman et al., 2018). In H. banksii, patterns of connectivity were linked to coastal topography and water exchange between estuaries and the open coast, where populations inside estuaries acted as sinks of migrants. Inner-zones sampled in our study present a combination of estuarine and marine characteristics while the outer-zones’ hydrodynamics are governed by open-ocean water movement (deCastro et al., 2000; García-Gil et al., 2000; Iglesias and Carballo, 2009; Bernabeu et al., 2012). The geographical limit of each zone varies depending on the seasonal upwelling-downwelling sequences that define the patterns of circulation in the estuaries (Torres and Barton, 2007). Strong waves and swell affect the outer-zones of Rías Baixas (Bernabeu et al., 2012) and frequent disturbances could enhance recruitment of new genotypes that can be flush inside the Rías by powerful scouring bottom currents during the upwelling season (Iglesias and Carballo, 2009). Once trapped in the estuaries inner-zones, rhodoliths seem to mostly reproduce asexually. Similarly, a higher rate of asexual reproduction of H. banksia has been measured within estuaries when compared to areas along the open coast (Coleman et al., 2018). Authors further proposed that estuarine populations could have originated from floating thalli, teared off from neighboring open coast populations after large-scale storms.
Mirroring the results obtained with estimates of population divergence (FST), a few MLGs were shared between geographically close populations located in the estuaries outer-zones and those from the inner-zone and the mouth of estuaries. However, one MLG was extremely common in Galicia and spread over the whole sampled region of the Rías Baixas. Since ramets constitute free-living forms after thallus fragmentation in P. calcareum, the geographical range colonized by a single MLG cannot be used to estimate the age of clones (as for example, in the case of Posidonia oceanica, a clonal seagrass reproducing asexually via stolon growth; Arnaud-Haond et al., 2012). Growth rate of rhodoliths is considered as extremely low (as low as ∼1 mm per year for P. calcareum maerl; Adey and McKibbin, 1970; Blake and Maggs, 2003) and even ramets lifespan can reach hundreds of years (Foster, 2001; Goldberg, 2006). Speed of formation of new maerl beds have never been estimated. However, the radio carbon age of surface maerl thalli sampled near Trevignon was estimated to be of more than 1000 years BP (Before Present) while thalli sampled deeper in the same maerl bed reached almost 6000 years BP (Grall and Hall-Spencer, 2003). These results have leaded to the hypothesis that the maerl beds observed in Brittany have accumulated slowly during the las 10.000 years (i.e., since the end of the last glaciation; Grall and Hall-Spencer, 2003). Interestingly, the estuaries of the Rías Baixas have also reached their current coast lines and bathymetry 11.000 years ago (Méndez and Vilas, 2005). Because of these antecedents, we propose that the huge spatial and numerical dominance of the MLG015 genet can only have been established over a very long period of time and that extended distribution of some genotypes probably correspond to very old genets that have slowly spread over time to adjacent beds and even over whole region due to episodic large-scale disturbance such as hurricane-like storms. Extreme longevity of genets has been reported in other slow growing clonal organisms and genets age reach tens of thousands of years in the quaking aspen (Kemperman and Barnes, 1976) and in the seagrass P. oceanica (Arnaud-Haond et al., 2012).
Consequences for the Management and Conservation of Phymatolithon calcareum
Maerl beds are categorized as “vulnerable” in the European Red List of Habitats (Gubbay et al., 2016) and as threatened and/or declining habitats in the OSPAR list (Hall-Spencer et al., 2010), while P. calcareum is only listed in Annex V of the European Community Habitats Directive 19923 as a species whose exploitation requires management. Our study reveals that some clones of P. calcareum might be very old and that its genenotypic diversity has been accumulated over large temporal scales. We therefore question the long term impact of the exploitation of this species and advocate that maerl beds of the NE Atlantic should be considered a non-renewable resource with extremely poor recovery potential (see also Barbera et al., 2003; Hall-Spencer et al., 2010).
Eight of the 15 maerl beds studied were located in areas with legal protection status. However, strong genetic structure and the generally highly restricted distribution of clones (and alleles) stress the necessity for broader scale protection network, involving all countries with a NE Atlantic shoreline. Maerl beds lack any conservation status in some regions such as South Portugal. Likewise, results obtained at the regional scale indicate that the current protection measures, which are mainly restricted to two maerl beds located within a National Park (Illa Ons and Illas Cíes), may not be enough to preserve the high genetic diversity and complex structure found in Galicia. Galician maerl beds are located in areas characterized by high human population density, critically impacted by agriculture and industrial activities, and a reduction of areas colonized by rhodoliths have been observed during the last decades in the Rías Baixas (Peña and Bárbara, 2008). Negative impact of ocean acidification on maerl beds have also been postulated (Brodie et al., 2014) and, under the current scenario of global changes, maerl beds of high latitudes in the NE Atlantic could decline and particular attention should be given to beds located in Brittany and the British Isles, especially regarding their huge ecological and economical relevance.
Data Availability
This manuscript contains previously unpublished data. GENBANK accession numbers of psbA and COI-5P sequences are given in the text (i.e., legend of the Supplementary Table S1) and the genotype profile of the 455 samples of the maerl bed forming red algae Phymatolithon calcareum scored for eight microsatellite markers are available in Supplementary Table S2 and can also be downloaded from Dryad (doi: 10.5061/dryad.ds2714g).
Author Contributions
RB designed the study. CP, VP, IB, and RB conducted the field work. CP generated the data. CP, M-LG, and MV performed the data analyses. CP, MV, and M-LG wrote the manuscript. All authors read, commented, and agreed on the manuscript.
Funding
This research was supported by Spain’s Ministerio de Economía y Competitividad (CTM2010-18787) and Xunta de Galicia (10MMA103003PR). ASSEMBLE (grant agreement no. 227799) provided support for sampling in Brittany. CP acknowledges support by Xunta de Galicia (Axudas á etapa predoutoral do Plan I2C 2011) and Spain’s Ministerio de Educación (Programa FPU 2010; Programa FPU-Estancias Breves 2013). VP acknowledges support by Universidade da Coruña Universidade da Coruña (Programa Campus Industrial de Ferrol). ML and M-LG acknowledge support from Clonix and Clonix2 (France: ANR11-BSV7-00704 and ANR-18-CE32-0001-05).
Conflict of Interest Statement
The authors declare that the research was conducted in the absence of any commercial or financial relationships that could be construed as a potential conflict of interest.
The reviewer SK declared a past co-authorship with several of the authors to the handling Editor.
Acknowledgments
We are grateful to Belén Carro for her help in the Laboratory, to Sophie Martin, Philippe Potin, and Thierry Comtet for the discussions on maerl beds growth rate and radiocarbon dating and to the three reviewers for their constructive comments.
Supplementary Material
The Supplementary Material for this article can be found online at: https://www.frontiersin.org/articles/10.3389/fmars.2019.00149/full#supplementary-material
Footnotes
- ^http://ec.europa.eu/environment/nature/natura2000/index_en.htm
- ^http://www.statgraphics.net/
- ^http://ec.europa.eu/environment/nature/legislation/habitatsdirective/index_en.htm#enlargement
References
Adey, W. H., and McKibbin, D. L. (1970). Studies on the maerl species Phymatolithon calcareum (pallas) nov. comb. and Lithothamnium coralloides crouan in the ría de vigo. Bot. Mar. 13, 100–106. doi: 10.1515/botm.1970.13.2.100
Agapow, P. M., and Burt, A. (2001). Indices of multilocus linkage disequilibrium. Mol. Ecol. Notes 1, 101–102. doi: 10.1046/j.1471-8278.2000.00014.x
Airoldi, L., Balata, D., and Beck, M. W. (2008). The gray zone: relationships between habitat loss and marine diversity and their applications in conservation. J. Exp. Mar. Biol. Ecol. 366, 8–15. doi: 10.1016/j.jembe.2008.07.034
Alberto, F., Gouveia, L., Arnaud-Haond, S., Pérez-Lloréns, J. L., Duarte, C. M., and Serrao, E. A. (2005). Within-population spatial genetic structure, neighbourhood size and clonal subrange in the seagrass Cymodocea nodosa. Mol. Ecol. 14, 2669–2681. doi: 10.1111/j.1365-294X.2005.02640.x
Ally, D., Ritland, K., and Otto, S. P. (2008). Can clone size serve as a proxy for clone age? An exploration using microsatellite divergence in Populus tremuloides. Mol. Ecol. 17, 4897–4911. doi: 10.1111/j.1365-294X.2008.03962.x
Ardehed, A., Johansson, D., Schagerström, E., Kautsky, L., Johannesson, K., and Pereyra, R. T. (2015). Complex spatial clonal structure in the macroalgae Fucus radicans with both sexual and asexual recruitment. Ecol. Evol. 5, 4233–4245. doi: 10.1002/ece3.1629
Arnaud-Haond, S., and Belkhir, K. (2007). GENCLONE: a computer program to analyse genotypic data, test for clonality and describe spatial clonal organization. Mol. Ecol. Notes 7, 15–17. doi: 10.1111/j.1471-8286.2006.01522.x
Arnaud-Haond, S., Duarte, C. M., Alberto, F., and Serrão, E. A. (2007). Standardizing methods to address clonality in population studies. Mol. Ecol. 16, 5115–5139. doi: 10.1111/j.1365-294X.2007.03535.x
Arnaud-Haond, S., Duarte, C. M., Diaz-Almela, E., Marbà, N., Sintes, T., and Serrão, E. A. (2012). Implications of extreme life span in clonal organisms: millenary clones in meadows of the threatened seagrass Posidonia oceanica. PLoS One 7:e30454. doi: 10.1371/journal.pone.0030454
Arnaud-Haond, S., Marbà, N., Diaz-Almela, E., Serrão, E. A., and Duarte, C. M. (2010). Comparative analysis of stability-genetic diversity in seagrass (Posidonia oceanica) meadows yields unexpected results. Estuar. Coast. 33, 878–889. doi: 10.1007/s12237-009-9238-9
Arnaud-Haond, S., Moalic, Y., Hernández-García, E., Eguiluz, V. M., Alberto, F., Serrño, E. A., et al. (2014). Disentangling the influence of mutation and migration in clonal seagrasses using the genetic diversity spectrum for microsatellites. J. Hered. 105, 532–541. doi: 10.1093/jhered/esu015
Balloux, F., Lehmann, L., and de Meeûs, T. (2003). The population genetics of clonal and partially clonal diploids. Genetics 164, 1635–1644.
Barbera, C., Bordehore, C., Borg, J. A., Glemarec, M., Grall, J., Hall-Spencer, J. M., et al. (2003). Conservation and management of northeast atlantic and mediterranean maerl beds. Aquat. Conserv. 13, S65–S76. doi: 10.1002/aqc.569
Becheler, R., Benkara, E., Moalic, Y., Hily, C., and Arnaud-Haond, S. (2014). Scaling of processes shaping the clonal dynamics and genetic mosaic of seagrasses through temporal genetic monitoring. Heredity 112, 114–121. doi: 10.1038/hdy.2013.82
Bergström, L., Bruno, E., Eklund, B., and Kautsky, L. (2003). Reproductive strategies of Ceramium tenuicorne near its inner limit in the brackish Baltic Sea. Bot. Mar. 46, 125–131. doi: 10.1515/BOT.2003.013
Bernabeu, A. M., Lersundi-Kanpistegi, A. V., and Vilas, F. (2012). Gradation from oceanic to estuarine beaches in a ría environment: a case study in the ría de vigo. Estuar. Coast. Shelf Sci. 102, 60–69. doi: 10.1016/j.ecss.2012.03.001
Birkett, D. A., Maggs, C., and Dring, M. J. (1998). MAERL: an Overview of Dynamics and Sensitivity Characteristics for Conservation Management of Marine SACs. scottish association for marine science: UK Marine SACs Project.
Blake, C., and Maggs, C. A. (2003). Comparative growth rates and internal banding periodicity of maerl species (Corallinales, Rhodophyta) from northern Europe. Phycologia 42, 606–612. doi: 10.2216/i0031-8884-42-6-606.1
Bosence, D. W. (1976). Ecological studies on two unattached coralline algae from western ireland. Palaeontology 19, 365–395.
Bressan, G., and Babbini, L. (2003). Biodiversità marina delle coste italiane: corallinales del mar mediterraneo: guida alla determinazione. Biol. Mar. Medit. 10, 1–237.
Brodie, J., Williamson, C. J., Smale, D. A., Kamenos, N. A., Mieszkowska, N., Santos, R., et al. (2014). The future of the northeast atlantic benthic flora in a high CO2 world. Ecol. Evol. 4, 2787–2798. doi: 10.1002/ece3.1105
Cabioch, J. (1969). Les fonds de maërl de la baie de morlaix et leur peuplement végétal. Cah. Biol. Mar. 10, 139–161.
Cabioch, J. (1970). Le maërl des côtes de bretagne et le problème de sa survie. Penn Ar Bed 7, 421–429.
Carro, B., Lopez, L., Peña, V., Bárbara, I., and Barreiro, R. (2014). DNA barcoding allows the accurate assessment of European maerl diversity: a proof-of-concept study. Phytotaxa 190, 176–189. doi: 10.11646/phytotaxa.190.1.12
Ceccherelli, G., and Cinelli, F. (1999). The role of vegetative fragmentation in dispersal of the invasive alga Caulerpa taxifolia in the Mediterranean. Mar. Ecol. Prog. Ser. 182, 299–303. doi: 10.3354/meps182299
Cloern, J. E., Abreu, P. C., Carstensen, J., Chauvaud, L., Elmgren, R., Grall, J., et al. (2016). Human activities and climate variability drive fast-paced change across the world’s estuarine–coastal ecosystems. Glob. Chang. Biol. 22, 513–529. doi: 10.1111/gcb.13059
Coleman, M. A., Clark, J. S., Doblin, M. A., Bishop, M. J., and Kelaher, B. P. (2018). Genetic differentiation between estuarine and open coast ecotypes of a dominant ecosystem engineer. Mar. Freshwater Res. doi: 10.1071/MF17392
Coyer, J. A., Hoarau, G., Pearson, G. A., Serrao, E. A., Stam, W. T., and Olsen, J. L. (2006). Convergent adaptation to a marginal habitat by homoploid hybrids and polyploid ecads in the seaweed genus Fucus. Biol. Lett. 2, 405–408. doi: 10.1098/rsbl.2006.0489
deCastro, M., Gómez-Gesteira, M., Prego, R., Taboada, J. J., Montero, P., Herbello, P., et al. (2000). Wind and tidal influence on water circulation in a galician ria (NW Spain). Estuar. Coast. Shelf Sci. 51, 161–176. doi: 10.1006/ecss.2000.0619
Diaz-Almela, E., Marba, N., Alvarez, E., Santiago, R., Holmer, M., Grau, A., et al. (2008). Benthic input rates predict seagrass (Posidonia oceanica) fish farm-induced decline. Mar. Pollut. Bull. 56, 1332–1342. doi: 10.1016/j.marpolbul.2008.03.022
Dorken, M. E., and Eckert, C. G. (2001). Severely reduced sexual reproduction in northern populations of a clonal plant, Decodon verticillatus (Lythraceae). J. Ecol. 89, 339–350. doi: 10.1046/j.1365-2745.2001.00558.x
Durrant, H. M., Burridge, C. P., Kelaher, B. P., Barrett, N. S., Edgar, G. J., and Coleman, M. A. (2014). Implications of macroalgal isolation by distance for networks of marine protected areas. Conserv. Biol. 28, 438–445. doi: 10.1111/cobi.12203
Eckert, C. G. (2002). The loss of sex in clonal plants. Evol. Ecol. 15, 501–520. doi: 10.1023/A:1016005519651
Eriksson, O. (1993). Dynamics of genets in clonal plants. Trends Ecol. Evol. 8, 313–316. doi: 10.1016/0169-5347(93)90237-J
Eriksson, O., and Fröborg, H. (1996). “Windows of opportunity” for recruitment in long-lived clonal plants: experimental studies of seedling establishment in Vaccinium shrubs. Can. J. Bot. 74, 1369–1374. doi: 10.1139/b96-166
Excoffier, L., Laval, G., and Schneider, S. (2005). Arlequin ver. 3.0: an integrated software package for population genetics data analysis. Evol. Bioinform. 1, 47–50. doi: 10.1177/117693430500100003
Fierst, J. L., Kübler, J. E., and Dudgeon, S. R. (2010). Spatial distribution and reproductive phenology of sexual and asexual Mastocarpus papillatus (Rhodophyta). Phycologia 49, 274–282. doi: 10.2216/PH09-41.1
Foster, M. (2001). Rhodoliths: between rocks and soft places. J. Phycol. 37, 659–667. doi: 10.1046/j.1529-8817.2001.00195.x
Gaines, S. D., White, C., Carr, M. H., and Palumbi, S. R. (2010). Designing marine reserve networks for both conservation and fisheries management. Proc. Natl. Acad. Sci. U.S.A. 107, 18286–18293. doi: 10.1073/pnas.0906473107
García-Gil, S., Durán, R., and Vilas, F. (2000). Side scan sonar image and geologic interpretation of the ría de Pontevedra seafloor (Galicia, NW Spain). Sci. Mar. 64, 393–402. doi: 10.3989/scimar.2000.64n4393
Gastony, G. J. (1986). Electrophoretic evidence for the origin of fern species by unreduced spores. Am. J. Bot. 73, 1563–1569. doi: 10.1002/j.1537-2197.1986.tb10907.x
Goldberg, N. (2006). Age estimates and description of rhodoliths from Esperance Bay, Western Australia. J. Mar. Biol. Assoc. U.K. 86, 1291–1296. doi: 10.1017/S0025315406014317
Goudet, J. (1995). FSTAT (version 1.2): a computer program to calculate F-statistics. J. Hered. 86, 485–486. doi: 10.1093/oxfordjournals.jhered.a111627
Gourraud, P.-A., Génin, E., and Cambon-Thomsen, A. (2004). Handling missing values in population data: consequences for maximum likelihood estimation of haplotype frequencies. Eur. J. Hum. Genet. 12, 805–812. doi: 10.1038/sj.ejhg.5201233
Grall, J., and Hall-Spencer, J. M. (2003). Problems facing maerl conservation in Brittany. Aquat. Conserv. 13, S55–S64. doi: 10.1002/aqc.568
Grall, J., Le Loc’h, F., Guyonnet, B., and Riera, P. (2006). Community structure and food web based on stable isotopes (δ15N and δ13C) analysis of a North Eastern Atlantic maerl bed. J. Exp. Mar. Biol. Ecol. 338, 1–15. doi: 10.1016/j.jembe.2006.06.013
Gubbay, S., Sanders, N., Haynes, T., Janssen, J. A. M., Rodwell, J. R., Nieto, A., et al. (2016). European Red List of Habitats. Part 1: Marine Habitats. Luxembourg: European Union,
Guillemin, M.-L., Faugeron, S., Destombe, C., Viard, F., Correa, J. A., and Valero, M. (2008). Genetic variation in wild and cultivated populations of the haploid- diploid red alga Gracilaria chilensis: how farming practices favor asexual reproduction and heterozygosity. Evolution 62, 1500–1519. doi: 10.1111/j.1558-5646.2008.00373.x
Halfar, J., and Mutti, M. (2005). Global dominance of coralline red-algal facies: a response to miocene oceanographic events. Geology 33, 481–484. doi: 10.1130/G21462.1
Halkett, F., Simon, J.-C., and Balloux, F. (2005). Tackling the population genetics of clonal and partially clonal organisms. Trends Ecol. Evol. 20, 194–201. doi: 10.1016/j.tree.2005.01.001
Hall-Spencer, J., Grall, J., Moore, P. G., and Atkinson, R. J. A. (2003). Bivalve fishing and maerl-bed conservation in France and the UK- retrospect and prospect. Aquat. Conserv. 13, 33–41. doi: 10.1002/aqc.566
Hall-Spencer, J. M., Kelly, J., and Maggs, C. A. (2010). Background Document for Maërl Beds,” in OSPAR Commission Report 491/201036pp. Available at:http://qsr2010.ospar.org/media/assessments/Species/P00491_maerl.pdf
Harper, J. L. (1981). “The concept of population in modular organisms,” in Theoretical Ecology, ed. R. M. May (Oxford: Blackwell), 53–77.
Hernández-Kantún, J. J., Rindi, F., Adey, W. H., Heesch, S., Peña, V., Le Gall, L., et al. (2015). Sequencing type material resolves the identity and distribution of the generitype Lithophyllum incrustans, and related European species L. hibernicum and L. bathyporum (Corallinales. Rhodophyta). J. Phycol. 51, 791–807. doi: 10.1111/jpy.12319
Hickey, K. (2011). The Impact of Hurricanes on the Weather of Western Europe, Recent Hurricane Research - Climate, Dynamics, and Societal Impacts. Available at: https://www.intechopen.com/books/recent-hurricane-research-climate-dynamics-and-societal-impacts/the-impact-of-hurricanes-on-the-weather-of-western-europe
Hörandl, E. (2006). The complex causality of geographical parthenogenesis. New Phytol. 171, 525–538. doi: 10.1111/j.1469-8137.2006.01769.x
Hurst, L. D., and Peck, J. R. (1996). Recent advances in understanding of the evolution and maintenance of sex. Trends Ecol. Evol. 11, 46–52. doi: 10.1016/0169-5347(96)81041-X
Iglesias, G., and Carballo, R. (2009). Seasonality of the circulation in the ría de muros (NW Spain). J. Mar. Syst. 78, 94–108. doi: 10.1016/j.jmarsys.2009.04.002
Irvine, L. M., and Chamberlain, Y. M. (1994). Seaweeds of the British Isles Vol. 1: Rhodophyta, Part 2B Corallinales, Hildenbrandiales. London: The Natural History Museum.
Johannesson, K., Johansson, D., Larsson, K. H., Huenchuñir, C. J., Perus, J., Forslund, H., et al. (2011). Frequent clonality in fucoids (Fucus radicans and Fucus vesiculosus; Fucales, Phaeophyceae) in the Baltic Sea. J. Phycol. 47, 990–998. doi: 10.1111/j.1529-8817.2011.01032.x
Kalinowski, S. (2005). HP-RARE 1.0: a computer program for performing rarefaction on measures of allelic richness. Mol. Ecol. Notes 5, 187–189. doi: 10.1111/j.1471-8286.2004.00845.x
Kalinowski, S., and Taper, M. (2006). Maximum likelihood estimation of the frequency of null alleles at microsatellite loci. Conserv. Genet. 7, 991–995. doi: 10.1017/S0016672312000341
Kamiya, M., Saba, E., and West, J. A. (2017). Marginal distribution and high heterozygosity of asexual Caloglossa vieillardii (Delesseriaceae, Rhodophyta) along the Australian coasts. J. Phycol. 53, 1283–1293. doi: 10.1111/jpy.12580
Kamiya, M., and West, J. A. (2008). Origin of apomictic red algae: outcrossing studies of different strains in Caloglossa monosticha (Ceramiales, Rhodophyta). J. Phycol. 44, 977–984. doi: 10.1111/j.1529-8817.2008.00551.x
Kamiya, M., and West, J. A. (2010). “Investigations on reproductive affinities in red algae,” in Red Algae in the Genomic Age, eds J. Seckbach and D. J. Chapman (Dordrecht: Springer), 77–109. doi: 10.1007/978-90-481-3795-4_5
Kamiya, M., West, J. A., and Hara, Y. (2011). Induction of apomixis by outcrossing between genetically divergent entities of Caloglossa leprieurii (Ceramiales, Rhodophyta) and evidence of hybrid apomicts in nature. J. Phycol. 47, 753–762. doi: 10.1111/j.1529-8817.2011.01016.x
Kearney, M. (2005). Hybridization, glaciation and geographical parthenogenesis. Trends Ecol. Evol. 20, 495–502. doi: 10.1016/j.tree.2005.06.005
Kemperman, J. A., and Barnes, B. V. (1976). Clone size in American aspens. Can. J. Bot. 54, 2603–2607. doi: 10.1139/b76-280
Klekowski, E. J. (1997). “Somatic mutation theory of clonality,” in The Ecology and Evolution of Clonal Plants, eds H. De Kroon and J. Van Groenendael (Leiden: Backhuys Publishers), 227–241.
Krueger-Hadfield, S. A., Kollars, N. M., Byers, J. E., Greig, T. W., Hammann, M., Murray, D. C., et al. (2016). Invasion of novel habitats uncouples haplo-diplontic life cycles. Mol. Ecol. 25, 3801–3816. doi: 10.1111/mec.13718
Krueger-Hadfield, S. A., Kübler, J. E., and Dudgeon, S. R. (2013). Reproductive effort of Mastocarpus papillatus (Rhodophyta) along the California coast1. J.Phycol. 49, 271–281. doi: 10.1111/jpy.12034
Littler, M. M., Littler, D. S., and Hanisak, M. D. (1991). Deep-water rhodolith distribution, productivity, and growth history at sites of formation and subsequent degradation. J. Exp. Mar. Biol. Ecol. 150, 163–182. doi: 10.1016/0022-0981(91)90066-6
Macaya, E. C., López, B., Tala, F., Tellier, F., and Thiel, M. (2016). “Float and raft: role of buoyant seaweeds in the phylogeography and genetic structure of non-buoyant associated for a,” in Seaweed Phylogeography, eds Z. M. Hu and C. I. Fraser (Dordrecht: Springer), 97–130.
Macreadie, P. I., York, P. H., and Sherman, C. D. (2014). Resilience of Zostera muelleri seagrass to small-scale disturbances: the relative importance of asexual versus sexual recovery. Ecol. Evol. 4, 450–461. doi: 10.1002/ece3.933
Maggs, C. A., Castilho, R., Foltz, D., Henzler, C., Jolly, M. T., Kelly, J., et al. (2008). Evaluating signatures of glacial refugia for North Atlantic benthic marine taxa. Ecology 89, S108–S122. doi: 10.1890/08-0257.1
Marrack, E. C. (1999). The relationship between water motion and living rhodolith beds in the southwestern Gulf of California, Mexico. Palaios 14, 159–171. doi: 10.2307/3515371
McConnico, L. A., Foster, M. S., Steller, D. L., and Riosmena-Rodríguez, R. (2014). Population biology of a long-lived rhodolith: the consequences of becoming old and large. Mar. Ecol. Prog. Ser. 504, 109–118. doi: 10.3354/meps10780
McFadden, C. S. (1997). Contributions of sexual and asexual reproduction to population structure in the clonal soft coral, Alcyonium rudyi. Evolution 51, 112–126. doi: 10.1111/j.1558-5646.1997.tb02393.x
Méndez, G., and Vilas, F. (2005). Geological antecedents of the rias baixas (galicia, northwest iberian peninsula). J. Mar. Syst. 54, 195–207. doi: 10.1016/j.jmarsys.2004.07.012
Mendoza, M. L., and Cabioch, J. (1998). Étude comparée de la reproduction de Phymatolithon calcareum (pallas) adey & mcKibbin et Lithothamnion corallioides (P. & H. Crouan) P. & H. Crouan (Corallinales, Rhodophyta), et reconsidérations sur le définition des genres. Can. J. Bot. 76, 1433–1445. doi: 10.1139/b98-116
Menken, S. B., Smit, E., and Nijs, H. J. (1995). Genetical population structure in plants: gene flow between diploid sexual and triploid asexual dandelions (Taraxacum section ruderalia). Evolution 49, 1108–1118. doi: 10.1111/j.1558-5646.1995.tb04437.x
Montecinos, A. E., Couceiro, L., Peters, A. F., Desrut, A., Valero, M., and Guillemin, M.-L. (2017a). Species delimitation and phylogeographic analyses in the Ectocarpus subgroup siliculosi (Ectocarpales, Phaeophyceae). J. Phycol. 53, 17–31. doi: 10.1111/jpy.12452
Montecinos, A. E., Guillemin, M.-L., Couceiro, L., Peters, A. F., Stoeckel, S., and Valero, M. (2017b). Hybridization between two cryptic filamentous brown seaweeds along the shore: analysing pre- and post-zygotic barriers in populations of individuals with varying ploidy levels. Mol. Ecol. 26, 3497–3512. doi: 10.1111/mec.14098
Neiva, J., Serrão, E. A., Assis, J., Pearson, G. A., Coyer, J. A., Olsen, J. L., et al. (2016). “Climate oscillations, range shifts and phylogeographic patterns of North Atlantic Fucaceae,” in Seaweed Phylogeography, eds Z. M. Hu and C. I. Fraser (Dordrecht: Springer), 279–308.
Nelson, W. A. (2009). Calcified macroalgae - critical to coastal ecosystems and vulnerable to change: a review. Mar. Freshwater Res. 60, 787–801. doi: 10.1071/MF08335
Ogawa, T., Ohki, K., and Kamiya, M. (2015). High heterozygosity and phenotypic variation of zoids in apomictic Ulva prolifera (Ulvophyceae) from brackish environments. Aquat. Bot. 120, 185–192. doi: 10.1016/j.aquabot.2014.05.015
Oppliger, L. V., Von Dassow, P., Bouchemousse, S., Robuchon, M., Valero, M., Correa, J. A., et al. (2014). Alteration of sexual reproduction and genetic diversity in the kelp species Laminaria digitata at the southern limit of its range. PLoS One 9:e102518. doi: 10.1371/journal.pone.0102518
Otto, S. P., and Lenormand, T. (2002). Resolving the paradox of sex and recombination. Nat. Rev. Genet. 3, 252–261. doi: 10.1038/nrg761
Pardo, C., Bárbara, I., Barreiro, R., and Peña, V. (2017). Insights into species diversity of associated crustose coralline algae (Corallinophycidae, Rhodophyta) with atlantic european maerl beds using DNA barcoding. An. Jardín Bot. Mad. 74:e059. doi: 10.3989/ajbm.2459
Pardo, C., Lopez, L., Peña, V., Hernández-Kantún, J., Le Gall, L., Bárbara, I., et al. (2014a). A multilocus species delimitation reveals a striking number of species of coralline algae forming maerl in the OSPAR maritime area. PLoS One 9:e104073. doi: 10.1371/journal.pone.0104073
Pardo, C., Peña, V., Bárbara, I., Valero, M., and Barreiro, R. (2014b). Development and multiplexing of the first microsatellite markers in a coralline red alga (Phymatolithon calcareum, Rhodophyta). Phycologia 53, 474–479. doi: 10.2216/14-031.1
Peakall, R., and Smouse, P. (2012). GenAlEx 6.5: genetic analysis in excel. population genetic software for teaching and research-an update. Bioinformatics 28, 2537–2539. doi: 10.1093/bioinformatics/bts460
Peña, V., Adey, W. H., Riosmena-Rodríguez, R., Jung, M.-Y., Choi, H. G., Afonso Carrillo, J., et al. (2011). Mesophyllum sphaericum sp. nov (Corallinales, Rhodophyta): a new maërl-forming species from the northeast Atlantic. J. Phycol. 47, 911–927. doi: 10.1111/j.1529-8817.2011.01015.x
Peña, V., and Bárbara, I. (2008). Maërl community in the north-western Iberian peninsula: a review of floristic studies and long-term changes. Aquat. Conserv. 18, 339–366. doi: 10.1002/aqc.847
Peña, V., Barbara, I., Grall, J., Maggs, C. A., and Hall-Spencer, J. M. (2014a). The diversity of seaweeds on maerl in the NE Atlantic. Mar. Biodiv. 44, 533–551. doi: 10.1007/s12526-014-0214-7
Peña, V., Hernández-Kantún, J. J., Grall, J., Pardo, C., López, L., Bárbara, I., et al. (2014b). Detection of gametophytes in the maerl-forming species Phymatolithon calcareum (Melobesioideae, Corallinales) assessed by DNA barcoding. Cryptog. Algol. 35, 15–25. doi: 10.7872/crya.v35.iss1.2014.15
Peña, V., Pardo, C., López, L., Carro, B., Hernández-Kantún, J., Adey, W. H., et al. (2015). Phymatolithon lusitanicum sp. nov. (Hapalidiales, Rhodophyta): the third most abundant maerl-forming species in the atlantic iberian peninsula. Cryptog. Algol. 36, 1–31.
Reichel, K., Masson, J.-P., Malrieu, F., Arnaud-Haond, S., and Stoeckel, S. (2016). Rare sex or out of reach equilibrium? The dynamics of fis in partially clonal organisms. BMC Genet. 17:76. doi: 10.1186/s12863-016-0388-z
Rousset, F. (1997). Genetic differentiation and estimation of gene flow from F-statistics under isolation by distance. Gene. Mol. Biol. 145, 1219–1228.
Saunders, G. W. (2005). Applying DNA barcoding to red macroalgae: a preliminary appraisal holds promise for future applications. Philos. T. R. Soc. B 360, 1879–1888. doi: 10.1098/rstb.2005.1719
Saunders, G. W., and McDevit, D. C. (2012). “Methods for DNA barcoding photosynthetic protists emphasizing the macroalgae and diatoms,” in DNA Barcodes: Methods and Protocols, eds W. J. Kress and D. L. Erickson (Totowa, NJ: Humana Press), 207–222.
Schwartz, M. K., Luikart, G., and Waples, R. S. (2007). Genetic monitoring as a promising tool for conservation and management. Trends Ecol. Evol. 22, 25–33. doi: 10.1016/j.tree.2006.08.009
Segelbacher, G., Cushman, S. A., Epperson, B. K., Fortin, M.-J., Francois, O., Hardy, O. J., et al. (2010). Applications of landscape genetics in conservation biology: concepts and challenges. Conserv. Genet. 11, 375–385. doi: 10.1007/s10592-009-0044-5
Séré, M., Kaboré, J., Jamonneau, V., Belem, A. M. G., Ayala, F. J., and De Meeûs, T. (2014). Null allele, allelic dropouts or rare sex detection in clonal organisms: simulations and application to real data sets of pathogenic microbes. Parasite. Vector. 7, 331–344. doi: 10.1186/1756-3305-7-331
Sosa, P., Valero, M., Batista, F., and Gonzalez-Perez, M. (1998). Genetic structure of natural populations of Gelidium species: a re-evaluation of results. J. Appl. Phycol. 10, 279–284. doi: 10.1023/A:1008092023549
Soulé, M. E., and Simberloff, D. (1986). What do genetics and ecology tell us about the design of nature reserves? Biol. Cons. 35, 19–40. doi: 10.1016/0006-3207(86)90025-X
Spalding, M. D., Fox, H. E., Allen, G. R., Davidson, N., Ferdaña, Z. A., Finlayson, M. A. X., et al. (2007). Marine ecoregions of the world: a bioregionalization of coastal and shelf areas. BioScience 57, 573–583. doi: 10.1641/B570707
Stehlik, I., and Holderegger, R. (2000). Spatial genetic structure and clonal diversity of Anemone nemorosa in late successional deciduous woodlands of central europe. J. Ecol. 88, 424–435. doi: 10.1046/j.1365-2745.2000.00458.x
Suneson, S. (1982). The culture of bisporangial plants of Dermatolithon litorale (suneson) hamel et lemoine (Rhodophyta, Corallinaceae). Br. Phycol. J. 17, 107–116. doi: 10.1080/00071618200650121
Torres, R., and Barton, E. D. (2007). Onset of the Iberian upwelling along the Galician coast. Cont. Shelf Res. 27, 1759–1778. doi: 10.1016/j.csr.2007.02.005
Tucker, A. E., Ackerman, M. S., Eads, B. D., Xu, S., and Lynch, M. (2013). Population-genomic insights into the evolutionary origin and fate of obligately asexual Daphnia pulex. Proc. Natl. Acad. Sci. U.S.A. 110, 15740–15745. doi: 10.1073/pnas.1313388110
Vallejo-Marín, M., Dorken, M. E., and Barrett, S. C. H. (2010). The ecological and evolutionary consequences of clonality for plant mating. Annu. Rev. Ecol. Evol. Sci. 41, 193–213. doi: 10.1146/annurev.ecolsys.110308.120258
Weir, B. S., and Cockerham, C. C. (1984). Estimating F-statistics for the analysis of population structure. Evolution 38, 1358–1370.
Wolf, M. A., Falace, A., Kaleb, S., and Moro, I. (2016). Molecular data confirm the existence of attached crustose tetrasporangial thalli in Phymatolithon calcareum (Melobesioideae, Hapalidiaceae, Rhodophyta) from the mediterranean Sea. Aquat. Bot. 134, 75–81. doi: 10.1016/j.aquabot.2016.07.006
Keywords: coralline red algae, conservation, ecosystem engineer, genetic and genotypic diversity, mating system, microsatellite, North European Atlantic, rhodolith
Citation: Pardo C, Guillemin M-L, Peña V, Bárbara I, Valero M and Barreiro R (2019) Local Coastal Configuration Rather Than Latitudinal Gradient Shape Clonal Diversity and Genetic Structure of Phymatolithon calcareum Maerl Beds in North European Atlantic. Front. Mar. Sci. 6:149. doi: 10.3389/fmars.2019.00149
Received: 01 November 2018; Accepted: 08 March 2019;
Published: 29 March 2019.
Edited by:
Kathryn Schoenrock, National University of Ireland Galway, IrelandReviewed by:
Anne Chenuil, Centre National de la Recherche Scientifique (CNRS), FranceStacy Krueger, University of Alabama at Birmingham, United States
Copyright © 2019 Pardo, Guillemin, Peña, Bárbara, Valero and Barreiro. This is an open-access article distributed under the terms of the Creative Commons Attribution License (CC BY). The use, distribution or reproduction in other forums is permitted, provided the original author(s) and the copyright owner(s) are credited and that the original publication in this journal is cited, in accordance with accepted academic practice. No use, distribution or reproduction is permitted which does not comply with these terms.
*Correspondence: Marie-Laure Guillemin, bWFyaWVsYXVyZS5ndWlsbGVtaW5AZ21haWwuY29t
†These authors have contributed equally to this work