- 1Research Group Requirement and Welfare, Norwegian Institute of Marine Research, Bergen, Norway
- 2ASG – Animal Nutrition Group, Wageningen University & Research, Wageningen, Netherlands
Increased plant oil inclusion in aquaculture feeds has led to higher dietary phytosterol concentrations and speculation about whether this affects the metabolism and health of the fish. The mechanisms of cholesterol absorption and how phytosterols may affect this is unknown in fish. Zebrafish (Danio rerio) were used to study the effects of phytosterols on the uptake and organ distribution of dietary cholesterol in fish. One meal of diets containing a constant addition of cholesterol (cold and [4-14C] cholesterol) and varying types and concentrations of phytosterols were fed to fish in individual compartments. The fish were not previously conditioned on the experimental diets. Activity of 14C was then measured in water and fish tissues to quantify the tissue distribution and excretion of cholesterol. There were no effects of the moderate dietary concentrations of phytosterols on the excretion or tissue distribution of dietary cholesterol 24 h after the meal.
Introduction
With increased use of plant oils in diets for fish in aquaculture comes a concomitant increase in typical plant-derived components, like phytosterols (Sanden et al., 2017). Phytosterols are known to reduce the micellar solubility of cholesterol (Ikeda et al., 1989; Mel’nikov et al., 2004; Jesch and Carr, 2006; Brown et al., 2010) and thus diminish cholesterol uptake in both humans and rodents (Ikeda et al., 1988; Ostlund et al., 1999; Lin et al., 2010). It has also been shown that phytosterols can exert an effect on nuclear receptors controlling lipid metabolism and in turn influence the expression of genes involved in the uptake and excretion of cholesterol (reviewed by Calpe-Berdiel et al., 2009). Most likely, a combination of several mechanisms is responsible for the cholesterol-lowering effects of phytosterols (Smet et al., 2012). Lowering of plasma cholesterol by phytosterols has been shown for zebrafish, Danio rerio (Fatema et al., 2015), but phytosterols have not been linked to reduced cholesterol digestibility in salmonids (Miller et al., 2008). A reduction in dietary cholesterol as well as increasing dietary phytosterol:cholesterol ratios have, however, been repeatedly associated with changes in cholesterol synthesis and lipid metabolism in fish (Leaver et al., 2008; Liland et al., 2013; Norambuena et al., 2013; Kortner et al., 2014; Sanden et al., 2016). Consistent proof therefore exists of the link between dietary sterol supply and the lipid metabolism in fish, but there is less knowledge on the effects of phytosterols on the uptake of dietary cholesterol.
This study aimed to assess the acute effect of a single meal with moderate phytosterol concentrations, as found in modern commercial aquaculture fish diets, on the uptake of cholesterol in carnivore fish evolutionary adapted to having high cholesterol and low phytosterol concentrations in their diets. As controlled experiments using radio labeled feed ingredients are difficult to perform on typical adult aquacultured fish, such as Atlantic salmon (Salmo salar L.), we here present data from experiments on another carnivore fish; the zebrafish (McClure et al., 2006). The effects of a change in sterol composition on the micellar sterol composition, is thought to be one of the mechanisms of the cholesterol-lowering effect of phytosterols (Smet et al., 2012). This effect might, however, become difficult to detect in trials where fish have been conditioned on diets containing phytosterols, due to the concomitant change in tissue sterol composition, which will also affect the sterol metabolism. To be able to separate such long-term metabolic effects from the more immediate effects on micellar sterol composition and thus on the intestinal uptake of sterols, trials using an acute dose of phytosterols on non-conditioned fish is necessary. This paper thus presents proof-of-concept experiments to measure the cholesterol uptake in adults of a carnivore fish species fed diets varying in sterol composition.
Materials and Methods
Experimental Design
Both trials used diets with radiolabeled [4-14C] cholesterol and phytosterols to assess the direct effect of increasing levels of phytosterols on uptake of cholesterol in the context of a single meal. A single meal of phytosterols was administered to be able to separate immediate effects of phytosterols in the intestinal lumen from possible long-term systemic effects when phytosterols are accumulated in the tissues. Adult male zebrafish were fed individually, moved to clean tanks, and then kept overnight to allow time for uptake (see Supplementary Figure 1 for a schematic view of the setup). Both positive and a negative controls were included in the trials. A negative control group, named CON, was included in each trial and fed a diet without added phytosterols. A positive control group, named EZE, received the control feed without added phytosterols and a pharmaceutical blocker of cholesterol uptake, ezetimibe, dissolved in the water (10 μM, Santa Cruz Biotechnologies). This concentration has been shown to reduce cholesterol uptake by ∼30% (Clifton et al., 2010) and to significantly reduce the whole body cholesterol in zebrafish larvae (Baek et al., 2012). The positive control (EZE) was thus the control to verify that changes in cholesterol absorption were measurable in the current experimental setup. Twenty-four hours after feeding, fish were euthanized to determine uptake and excretion of the labeled cholesterol using a scintillation counter. The two trials differed in some ways: in trial 1, a phytosterol blend was added to the diets in graded concentrations and fish were dissected for organ samples, while in trial 2 a pure fucosterol extract diet was added, only one concentration of sterols was used, and whole fish were used for the scintillation counts. Pure fucosterol was added to the feeds in trial 2 due to its already documented effects on lipid metabolism (Hoang et al., 2012) and due to it being of interest for aquaculture nutrition with the introduction of algal sources of nutrients such as algae oil and -meal to the diets. Trial 1 was therefore able to provide us with information on tissue distribution of absorbed cholesterol. The simplified experimental procedure in trial 2 without dissections led to fewer samples per fish and dramatically shortened the handling time for sampling each fish, allowing us to have a larger amount of fish per treatment group and thus enable detection of smaller effects of the dietary treatments on cholesterol uptake than in trial 1.
Feed Production
The basal diet was formulated using standard feed ingredients selected for their low sterol concentrations (Table 1). Ingredients were thoroughly mixed with MilliQ water and dried in an oven at 42°C for 120 h before milling into pellets of the desired size (<1.12 mm and >0.56 mm). Both [4-14C]-labeled cholesterol (Perkin Elmer, MA, United States) and non-labeled cholesterol (>99% pure, Sigma) were coated onto the pellets with an aimed radioactivity of 0.3 MBq (18∗106 dpm) per gram of feed, in accordance with Hamre et al. (2011). Experimental diets were made by coating aliquots of the basal diet with the desired amounts of sterols. Sterols were dissolved in ethyl ether, vortexed well with the feed, and left to dry under nitrogen for ∼24 h to remove all traces of the solvent. Phytosterol concentrations were determined on a molar basis relative to the amount of cholesterol (Table 2). In trial 1, three concentrations of a phytosterol blend derived from soybean and rapeseed oil (CardioAidTM, Archer Daniels Midland Company, average molar weight: 410.3) were used to create increasing ratios of phytosterol:cholesterol (1:1, 2:1, and 3:1) (Table 2). In trial 2, a fucosterol extract (>98% pure, Santa Cruz Biotechnologies, molar weight: 412.7) diet was used in addition to the phytosterol extract in trial 1, both included in a 2:1 ratio with cholesterol (Table 2). The molar weight of the phytosterol extract was based on phytosterol analyses of the product (Supplementary Table 1), performed as described in 2.3 Feed analyses.
Feed Analyses
Samples from the feeds were taken to measure final radioactivity (3 × 10 mg) and phytosterol concentrations (one sample of ∼200 mg per feed). Total fat was estimated by extracting lipids using heptane combined with HCl (European Economic Community [EEC], 2009). Crude protein was assessed by measuring total nitrogen and using 6.25 as multiplication factor (The Association of Official Analytical Chemists [AOAC], 1995). Sterols in the feeds were analyzed with a GC-FID setup and quantified using 5-β-cholestan-3-α-ol as an internal standard as described by Liland et al. (2013) adapted from Laakso (2005).
Fish and Feeding
Since lipid metabolism may be very different between males and females (Meguro et al., 2015), only male wild type zebrafish were used in both trials to reduce variation. In trial 1, 48 fish at ∼6 months post hatch were used and 68 fish at ∼4 months post hatch were used in trial 2. Before the experiments, all fish were housed in groups of ∼50 fish in aquariums at the animal facilities at IMR, Bergen, Norway, and were fed a standard feed for zebrafish (Gemma micro 500, Skretting United States, Tooele, UT, United States). Although the trials were performed approximately 2 years apart, the conditions prior to the trials were similar; the same animal facilities and feeds were used, and stable environmental conditions were recorded throughout this whole period. Trial 1 was performed between 19th of October and 19th of November 2015, while trial 2 was performed between 5th and 20th of February 2018. All work including use of isotopes was conducted in the isotope laboratory at IMR, Bergen, Norway, where safety protocols approved by the Norwegian Radiation Protection Authority (Meldenr. M3204-12) were used. Each morning, the fish to be used that same day were transferred to the isotope laboratory and housed individually in 500 mL glass beakers filled with 200 mL preheated system water from the animal facilities. Water temperature was kept stable at 28.5°C by keeping the beakers in a water bath with temperature regulation. The fish were left to acclimatize for ∼1 h and then fed one meal, ad libitum, of their randomly allocated experimental feed and left for feeding and digestion during for 30 min. Fish were then removed from the feeding beaker, flushed with system water to remove possible traces of feed on the fish, and put into a fresh beaker with no feed (500 mL glass beaker with 300 mL system water). Twenty-four hours after the transfer, the fish were euthanized by adding tricaine methanesulfonate (TMS) from a stock solution directly to the beaker (final concentration 1 g TMS L-1). All treatments were performed each day, in randomized order and position in the water baths. The experimental procedure was conducted according to the guidelines of the Norwegian State Commission for Laboratory Animals (application number: 15/166840).
Sampling
Euthanized fish were quickly transferred to ice to slow down metabolic processes, and their weight and length measured. In trial 1, the fish were dissected to remove intestine and liver (including gallbladder). The presence of intestinal content was noted, when visible. In trial 2, only whole fish were sampled and no dissections performed. In both trials, females identified during sampling were discarded and the respective treatment repeated. Each tissue sample (intestine, liver, carcass or whole fish) was put into individual glass scintillation tubes (20 mL) and received 1–2 mL of Soluene-350 (Perkin Elmer, MA, United States). The beakers were kept at ∼60°C for 4–5 h, to accelerate the breakdown of the tissues. The samples were left to dissolve overnight, or until completely liquefied, before bleaching with 30% hydrogen peroxide (2 × 100 μL) at 50–60°C for 30 min to complete decolorization. After the removal of the fish from the beakers, the water in each beaker was homogenized with a Polytron PT-MR2100 (Kinematica AG Switzerland) for 2 min to ensure fecal matter or precipitates in the water were homogenously distributed. The exact volume of water was noted and triplicate water samples (10 mL × 3) were taken from the beakers while continuously stirring the water. Both water and tissue samples then received 10 mL Ultima Gold (Perkin Elmer, MA, United States) and were left overnight to adapt to light and temperature, according to manufacturer instructions. Radioactivity was measured (2 × 10 min) in each sample with a TRI-CARB 2000CA scintillation counter (United Technologies Packard, United Kingdom).
Calculations
The results in disintegrations per minute (dpm) from the scintillation counter (average dpm value of two counts of each sample) was converted to Bq for further analysis and presentation (1 Bq = 60 dpm/1000 dpm = 16.7 Bq). The value for the total fish of trial 1 was calculated as:
Total system Bq was used as an estimation of total ingested [4-14C] as well as total feed intake and was in both trials was calculated as:
Based on scintillation counts of the feeds, it was determined that fish with a Bqtotalsystem value lower than ∼33 Bq (2000 dpm) ate less than one third of an average grain of feed (data not presented). This was therefore set as a lower limit in order to separate fish that did not eat from the fish that did eat. All data is presented as mean ± standard error of the mean (SEM) if not otherwise stated.
Statistics
All statistical analyses were done using the free software environment R (R Development Core Team, 2017). Effects of dietary treatments (categorical) on the excretion of cholesterol and tissue distribution were analyzed with linear mixed effects models using the nlme package (Pinheiro et al., 2010) (nested ANOVA, random effect: sample day) followed by Tukey’s HSD using the package multcomp (Hothorn et al., 2008). For regression analyses of effects of numerical values (dietary phytosterol concentration and ratio, phytosterol dose per fish, phytosterol dose per mg bodyweight) on cholesterol excretion and tissue distribution, nlme was also used (random effects: sample day and trial), but here the EZE groups were excluded from the statistical analyses to see only the effects of sterol dose.
Results
Nutrient Composition of Feeds
Trial 1
The diets contained 48% protein and 16.5% lipid. The control diet (CON1) without added phytosterols contained 0.4 mg phytosterols per g feed, while the SMIX1:1, SMIX2:1, and SMIX3:1 diets contained 1.4, 1.3, and 3.2 mg phytosterols per g feed, respectively (Table 3). The phytosterol content of the SMIX2:1 was lower than aimed for, resulting in a lower phytosterol:cholesterol ratio in the final feed than planned for (Table 3). The aimed radioactivity per g of feed was 0.3 MBq, and the measured values were close to that, averaging around 0.27 MBq per g feed (∼89% of aimed for value, Supplementary Table 4).
Trial 2
The diets contained 48% protein and 14% lipid. The diets of trial 2, being a freshly prepared batch of feed made only few days before trial 2, had slightly lower lipid content than the diets of trial 1, possibly due to some difference in the water content of the ingredients. The control diet (CON2) without added phytosterols contained 0.6 mg phytosterols per g feed, while the SMIX and FUCO diets both contained 2.5 mg phytosterols per g feed (Table 3). The measured radioactivity values were close to the target, averaging around 0.25 MBq per g feed (∼83% of aimed for value, Supplementary Table 5).
Fish Weight and Feed Consumption
The fish of trial 1 and 2 weighed 559 ± 9.7 mg and 420.4 ± 4.8 mg, respectively. There were no significant differences between the treatments in weight or condition factor within the two trials (Supplementary Tables 4, 5). Each individual fish had a mean feed intake (calculated based on Bq in total system and Bq in feeds) equal to a mean of ∼1.8 mg of feed eaten (0.3% of bodyweight) in trial 1 and ∼1.2 mg (0.3% of bodyweight) in trial 2 (Supplementary Tables 4, 5). The actual dose of phytosterols per weight unit of each individual fish was calculated and varied from 0.0009 to 0.0078‰ of bodyweight (Supplementary Tables 4, 5).
Uptake and Excretion of Dietary Cholesterol
There was no effect of the dietary phytosterols on the excretion of [4-14C] cholesterol in neither trial 1 nor trial 2. The dietary effects on cholesterol excretion were statistically evaluated by comparing between the treatment groups as well as by using the actual dose of phytosterols based on amount of feed eaten and dietary analyses (dietary phytosterol concentration and ratio, phytosterol dose per fish, phytosterol dose per mg bodyweight) and no effects were found due to any of these parameters. The excretion of [4-14C] cholesterol plotted against the analyzed dietary phytosterol:cholesterol ratios is presented in Figure 1A. The ezetimibe treatment was very efficient at increasing the cholesterol excretion, more than doubling the mean excretion from 29.1 ± 1.7% (all non-EZE groups from both trials, n = 96) to 71.9 ± 2.5% (EZE groups from both trials, n = 20) (Figure 1B). The EZE groups had a significantly higher cholesterol excretion than the other treatment groups in both trials (p < 0.001, nested ANOVA). Of the [4-14C] cholesterol in the fish tissues (Bqtotal fish) measured in trial 1, 58.5 ± 1.8% (n = 48) was still in the intestine 24 h after the meal. The liver contained 5.3 ± 1.2% and the remaining carcass 36.2 ± 1.3% (n = 48) (Figure 2). Neither the phytosterols nor the ezetimibe treatment had any effect on the tissue distribution of the dietary cholesterol (data on [4-14C] distribution in all treatment groups in trial 1 presented in Supplementary Figure 2). Out of a total of 48 fish dissected and analyzed in trial 1, only five fish had visible traces of digesta in the intestine. However, the visible presence of digesta did not have any effect on the dpm readings of the organ, likely due to a fast absorption of dietary cholesterol into the intestinal tissue, leaving only more slowly digestible nutrients in the intestinal lumen.
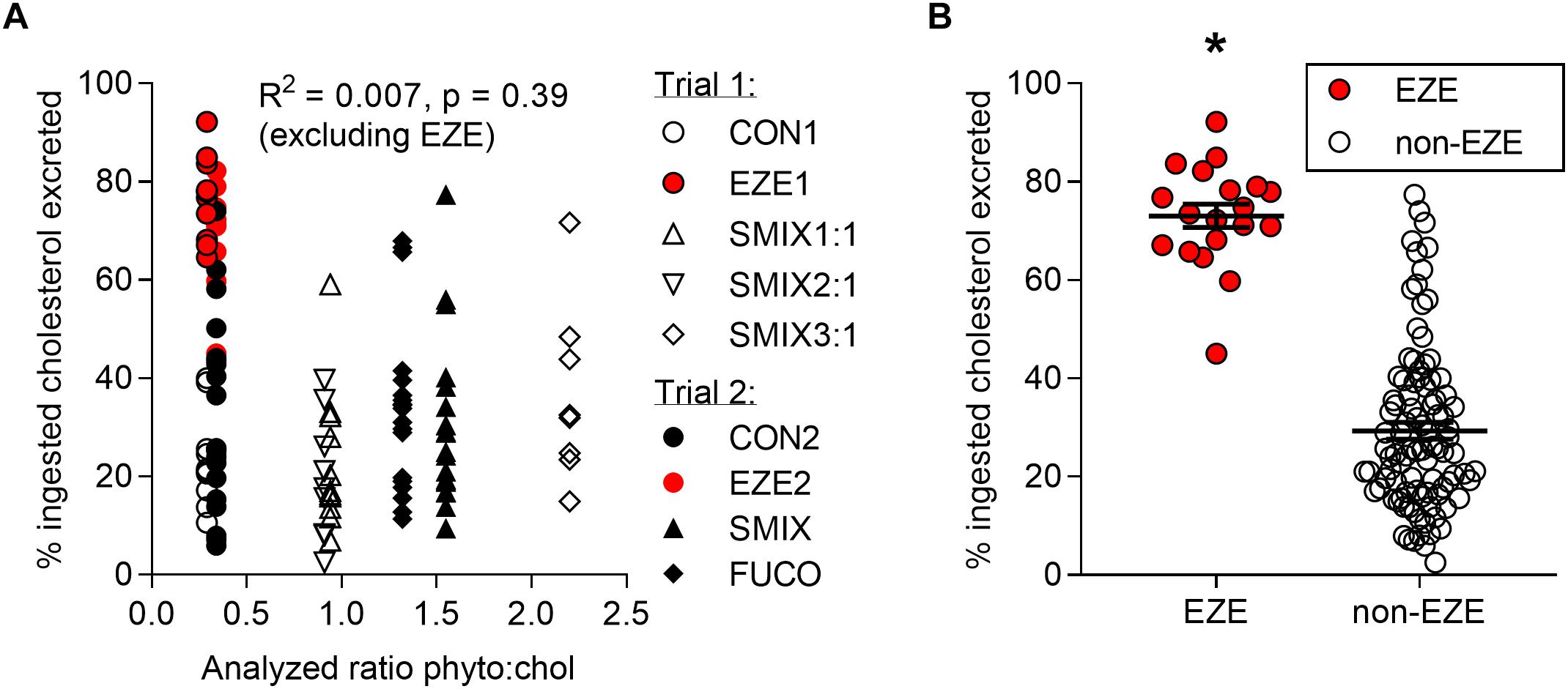
Figure 1. Excreted [4-14C] cholesterol (as percent of ingested [4-14C] cholesterol) of fish from both trials. (A) Individual values plotted against their respective analyzed dietary ratios of phytosterols:cholesterol (no effect of dietary phytosterol:cholesterol ratio on the excretion of exogenous cholesterol). CON, control diet without added phytosterols; SMIX, diet with phytosterol added as a sterol extract from soybean/rapeseed; FUCO, diet with phytosterol added as pure fucosterol. (B) Individual values as well as mean ± SEM plotted as either not exposed to ezetimibe in the water (non-EZE, n = 96) or exposed to ezetimibe (EZE, n = 20). The fish exposed to ezetimibe had a significantly higher excretion of cholesterol, as indicated by ∗ (p < 0.001, nested ANOVA).
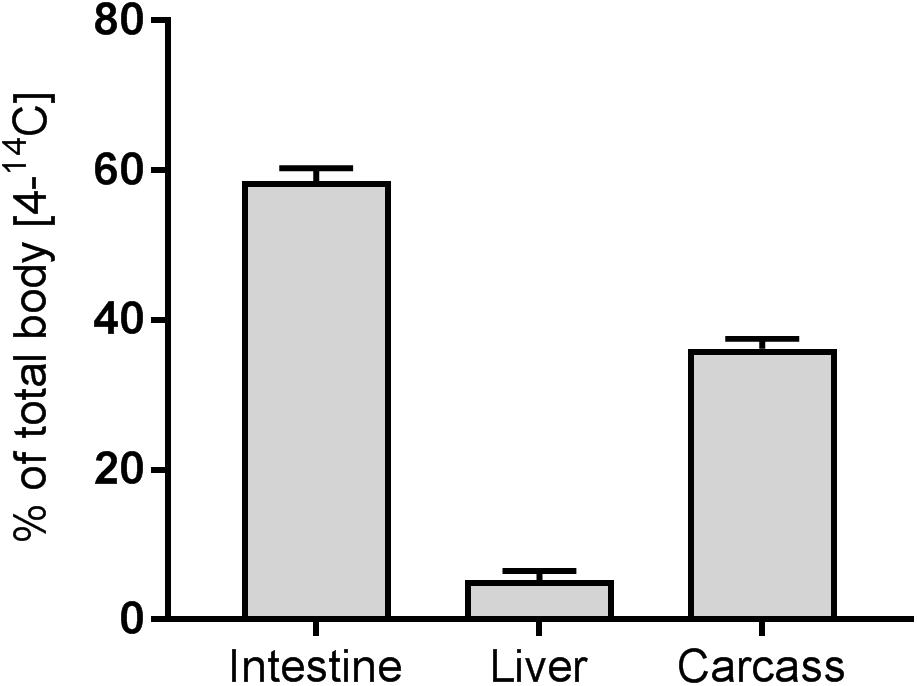
Figure 2. Tissue distribution of [4-14C] cholesterol (as percent of total fish [4-14C] cholesterol) measured in fish from trial 1. Data presented as mean ± SEM (n = 49). There were no dietary effects on the tissue distribution of [4-14C] cholesterol.
Discussion
Increased replacement of dietary fish oil with plant oils in aquaculture-grown carnivore fish increases dietary phytosterol concentrations while decreasing the cholesterol content of the diets. A typical fishmeal and fish oil diet has a phytosterol:cholesterol ratio of ∼0.1 (Liland et al., 2013), while modern aquaculture diets for, e.g., Atlantic salmon have average phytosterol:cholesterol ratios of 1.5 (ranging from 1 to 2.5 in analyses done on Norwegian commercial salmonid feeds in 2016) (Sanden et al., 2017). It has been a concern whether this shift in sterol composition could lead to a lowered availability of dietary cholesterol and that compensatory mechanisms to produce more cholesterol could lead to unwanted metabolic effects in the fish (Liland et al., 2013). The current study thus aimed to use zebrafish as a model of carnivore fish to gain more information on how uptake mechanisms of cholesterol in a single meal in fish are affected when fed diets with relatively low content of cholesterol and moderate dietary concentrations of phytosterols. By using an acute dose of phytosterols on fish not previously conditioned to the diets, the more immediate effects of dietary sterols, such as their effect on micellar sterol distribution, will be more visible. This is in contrast to what is possible to see in a more long-term feeding trial, where metabolic effects due to the changes in tissue sterol composition might become more dominating.
Phytosterols have been thoroughly proven to lower plasma cholesterol of mammals (Laraki et al., 1991; Plat et al., 2000; Matvienko et al., 2002; Doornbos et al., 2006) and some studies also indicate the same in fish (Couto et al., 2015b; Fatema et al., 2015; Sissener et al., 2018). Long-term feeding with phytosterols has shown a lowered plasma cholesterol in juvenile sea bass (Dicentrarchus labrax) (Couto et al., 2015b) and Atlantic salmon (Sissener et al., 2018), but not in on-growing sea bass or juvenile or on-growing seabream (Sparus aurata) (Couto et al., 2014a,b, 2015a). To measure the actual uptake of exogenous cholesterol in the presence of phytosterols is not commonly done, as it requires different methods than normal digestibility studies and normally the use of a tracer such as stable isotopes. The studies that have quantified the uptake of sterols report phytosterols to be inefficiently absorbed (≤5%) compared to cholesterol (45–54%) in humans (Salen et al., 1970; Bosner et al., 1999). In the current trial 70.9% of dietary cholesterol was taken up 24 h post ingestion, indicating a higher cholesterol absorption in fish than in humans. The measurements of cholesterol uptake are, however, likely influenced by factors such as dietary cholesterol concentrations as well as variations due to different methods used for measuring cholesterol uptake in humans than in fish.
In Atlantic salmon, both the dietary cholesterol content and the ratio of phytosterols:cholesterol (not phytosterol concentrations alone) affected the plasma cholesterol, with increasing phytosterol:cholesterol ratios lowering the plasma cholesterol in a linear manner (Sissener et al., 2018). The same study showed that dietary cholesterol concentrations as well as the dietary phytosterol:cholesterol ratios can affect the tissue cholesterol concentrations (Sissener et al., 2018). Dietary phytosterol has, on the other hand, not been shown to affect digestibility (Miller et al., 2008) or retention (Sissener et al., 2018) of cholesterol in Atlantic salmon. The cholesterol in the body is, however, largely composed of endogenously produced cholesterol and most of the cholesterol absorbed through the intestine is endogenously derived biliary cholesterol (Turley and Dietschy, 2003). The current trial only measured the uptake of dietary cholesterol after a single meal of the experimental diets and shows that phytosterol:cholesterol ratios up to 2.2 do not affect the uptake of exogenously derived cholesterol. The current results, combined with a lack of effects on cholesterol retention and digestibility in other trials (Miller et al., 2008; Sissener et al., 2018), indicate that a moderate dose of phytosterols does not affect the absorption of neither exogenously nor endogenously derived cholesterol in fish. The lack of dramatic effects of modest changes in the dietary sterol composition is likely due to the plasticity of the cholesterol metabolism, being able to quickly adapt to differing sterol concentrations to maintain stable cellular cholesterol concentrations (Kortner et al., 2014). The current trial looked at the effects of an acute dose of phytosterols in fish not previously conditioned on the experimental diets, but long-term feeding with phytosterols in fish indicate that also a continued addition of phytosterols in the diets is without large effects on cholesterol uptake, but then measured as total cholesterol (exogenous + endogenous cholesterol) (Couto et al., 2014a,b, 2015a,b; Miller et al., 2008; Sissener et al., 2018). Although zebrafish is a recognized model for use in studies on lipid metabolism (Carten and Farber, 2009), the results of the current trial would need to be replicated in the target species to be able to give a more definitive conclusion concerning specific species.
Interestingly, the current trial did not find any effect of neither the ezetimibe treatment nor dietary phytosterol on the tissue distribution of the dietary cholesterol. The clear inhibiting effect of ezetimibe on the uptake of cholesterol emphasizes that ezetimibe uniquely blocks the intestinal absorption of cholesterol. However, the lack of changes on cholesterol tissue distribution of ezetimibe demonstrates that the fate of absorbed cholesterol is a process not easily disturbed. The hepatic production and proper circulation of cholesterol will therefore likely become more important when fish eat less cholesterol. The tissue distribution of dietary cholesterol seems to be evolutionary conserved as it was similar to what has been seen in rats (Borgström, 1968), where ∼45% of exogenous cholesterol was found in the gastrointestinal system and under 10% in the liver after 24 h.
Author Contributions
MvS, NS, BT, ØS, and NL participated in the planning and design of the trials. MvS, NS, ØS, and ØR participated in the execution of feeding trials. MvS, NS, and NL analyzed the samples. MvS and NL did data treatment and statistics. MvS and NL were the main authors of the manuscript. NS, ØR, ØS, and BT contributed with comments and corrections to the manuscript.
Funding
The study was funded by the Norwegian Research Councils HAVBRUK Program (Aquafly steroler: project number: 244253/E40) and Institute of Marine Research (IMR), Bergen, Norway.
Conflict of Interest Statement
The authors declare that the research was conducted in the absence of any commercial or financial relationships that could be construed as a potential conflict of interest.
Acknowledgments
Thanks to Silvia Rinaldi for her work with the zebrafish in trial 2 and to Arna Kazazic for her help feeding and taking care of the zebrafish.
Supplementary Material
The Supplementary Material for this article can be found online at: https://www.frontiersin.org/articles/10.3389/fmars.2019.00092/full#supplementary-material
References
Baek, J. S., Fang, L., Li, A. C., and Miller, Y. I. (2012). Ezetimibe and simvastatin reduce cholesterol levels in zebrafish larvae fed a high-cholesterol diet. Cholesterol 2012:564705. doi: 10.1155/2012/564705
Borgström, B. (1968). Quantitative aspects of the intestinal absorption and metabolism of cholesterol and β-sitosterol in the rat. J. Lipid Res. 9, 473–481.
Bosner, M. S., Lange, L. G., Stenson, W. F., and Ostlund, R. E. (1999). Percent cholesterol absorption in normal women and men quantified with dual stable isotopic tracers and negative ion mass spectrometry. J. Lipid Res. 40, 302–308.
Brown, A. W., Hang, J., Dussault, P. H., and Carr, T. P. (2010). Phytosterol ester constituents affect micellar cholesterol solubility in model bile. Lipids 45, 855–862. doi: 10.1007/s11745-010-3456-6
Calpe-Berdiel, L., Escolà-Gil, J. C., and Blanco-Vaca, F. (2009). New insights into the molecular actions of plant sterols and stanols in cholesterol metabolism. Atherosclerosis 203, 18–31. doi: 10.1016/j.atherosclerosis.2008.06.026
Carten, J. D., and Farber, S. A. (2009). A new model system swims into focus: using the zebrafish to visualize intestinal metabolism in vivo. Clin. Lipidol. 4, 501–515. doi: 10.2217/clp.09.40
Clifton, J. D., Lucumi, E., Myers, M. C., Napper, A., Hama, K., Farber, S. A., et al. (2010). Identification of novel inhibitors of dietary lipid absorption using zebrafish. PLoS One 5:e12386. doi: 10.1371/journal.pone.0012386
Couto, A., Kortner, T. M., Penn, M., Bakke, A. M., Krogdahl,Å., and Oliva-Teles, A. (2014a). Effects of dietary phytosterols and soy saponins on growth, feed utilization efficiency and intestinal integrity of gilthead sea bream (Sparus aurata) juveniles. Aquaculture 432, 295–303. doi: 10.1016/j.aquaculture.2014.05.009
Couto, A., Kortner, T. M., Penn, M., Bakke, A. M., Krogdahl,Å., and Oliva-Teles, A. (2014b). Effects of dietary soy saponins and phytosterols on gilthead sea bream (Sparus aurata) during the on-growing period. Anim. Feed Sci. Technol. 198, 203–214. doi: 10.1016/j.anifeedsci.2014.09.005
Couto, A., Kortner, T. M., Penn, M., Bakke, A. M., Krogdahl,Å., and Oliva-Teles, A. (2015a). Dietary saponins and phytosterols do not affect growth, intestinal morphology and immune response of on-growing European sea bass (Dicentrarchus labrax). Aquacult. Nutr. 21, 970–982. doi: 10.1111/anu.12220
Couto, A., Kortner, T. M., Penn, M., Ostby, G., Bakke, A. M., Krogdahl, Å., et al. (2015b). Saponins and phytosterols in diets for European sea bass (Dicentrarchus labrax) juveniles: effects on growth, intestinal morphology and physiology. Aquacult. Nutr. 21, 180–193. doi: 10.1111/anu.12146
Doornbos, A. M. E., Meynen, E. M., Duchateau, G., Van der Knaap, H. C. M., and Trautwein, E. A. (2006). Intake occasion affects the serum cholesterol lowering of a plant sterol-enriched single-dose yoghurt drink in mildly hypercholesterolaemic subjects. Eur. J. Clin. Nutr. 60, 325–333. doi: 10.1038/sj.ejcn.1602318
European Economic Community [EEC] (2009). Commission Regulation (EC) No 152/2009 of 27 January 2009 Laying Down the Methods of Sampling and Analysis for the Official Control of Feed. Available at: https://eur-lex.europa.eu/legal-content/EN/TXT/PDF/?uri=CELEX:02009R0152-20170524&qid=1549036706310&from=EN.
Fatema, M., Chen, Z., and Wei, G. (2015). β-sitosterol reduce cholesterol levels in high cholesterol diet fed zebrafish. Int. J. Nat. Soc. Sci. 2, 53–65.
Hamre, K., Lukram, I. M., Ronnestad, I., Nordgreen, A., and Sæle, Ø. (2011). Pre-digestion of dietary lipids has only minor effects on absorption, retention and metabolism in larval stages of Atlantic cod (Gadus morhua). Br. J. Nutr. 105, 846–856. doi: 10.1017/S0007114510004459
Hoang, M. H., Jia, Y., Jun, H. J., Lee, J. H., Lee, B. Y., and Lee, S. J. (2012). Fucosterol is a selective Liver X receptor modulator that regulates the expression of key genes in cholesterol homeostasis in macrophages, hepatocytes, and intestinal cells. J. Agric. Food Chem. 60, 11567–11575. doi: 10.1021/jf3019084
Hothorn, T., Bretz, F., and Westfall, P. (2008). Simultaneous inference in general parametric models. Biom. J. 50, 346–363. doi: 10.1002/bimj.200810425
Ikeda, I., Tanabe, Y., and Sugano, M. (1989). Effects of sitosterol and sitostanol on micellar solubility of cholesterol. J. Nutr. Sci. Vitaminol. 35, 361–369. doi: 10.3177/jnsv.35.361
Ikeda, I., Tanaka, K., Sugano, M., Vahouny, G. V., and Gallo, L. L. (1988). Inhibition of cholesterol absorption in rats by plant sterols. J. Lipid Res. 29, 1573–1582.
Jesch, E. D., and Carr, T. P. (2006). Sitosterol reduces micellar cholesterol solubility in model bile. Nutr. Res. 26, 579–584. doi: 10.1016/j.nutres.2006.08.006
Kortner, T. M., Bjorkhem, I., Krasnov, A., Timmerhaus, G., and Krogdahl, A. (2014). Dietary cholesterol supplementation to a plant-based diet suppresses the complete pathway of cholesterol synthesis and induces bile acid production in Atlantic salmon (Salmo salar L.). Br. J. Nutr. 111, 2089–2103. doi: 10.1017/S0007114514000373
Laakso, P. (2005). Analysis of sterols from various food matrices. Eur. J. Lipid Sci. Technol. 107, 402–410. doi: 10.1002/ejlt.200501134
Laraki, L., Pelletier, X., and Debry, G. (1991). Effects of dietary cholesterol and phytosterol overload on Wistar rat plasma lipids. Ann. Nutr. Metab. 35, 221–225. doi: 10.1159/000177649
Leaver, M. J., Villeneuve, L. A., Obach, A., Jensen, L., Bron, J. E., Tocher, D. R., et al. (2008). Functional genomics reveals increases in cholesterol biosynthetic genes and highly unsaturated fatty acid biosynthesis after dietary substitution of fish oil with vegetable oils in Atlantic salmon (Salmo salar). BMC Genomics 9:299. doi: 10.1186/1471-2164-9-299
Liland, N. S., Espe, M., Rosenlund, G., Waagbo, R., Hjelle, J. I., Lie, O., et al. (2013). High levels of dietary phytosterols affect lipid metabolism and increase liver and plasma TAG in Atlantic salmon (Salmo salar L.). Br. J. Nutr. 110, 1958–1967. doi: 10.1017/S0007114513001347
Lin, X., Racette, S. B., Lefevre, M., Spearie, C. A., Most, M., Ma, L., et al. (2010). The effects of phytosterols present in natural food matrices on cholesterol metabolism and LDL-cholesterol: a controlled feeding trial. Eur. J. Clin. Nutr. 64, 1481–1487. doi: 10.1038/ejcn.2010.180
Matvienko, O. A., Lewis, D. S., Swanson, M., Arndt, B., Rainwater, D. L., Stewart, J., et al. (2002). A single daily dose of soybean phytosterols in ground beef decreases serum total cholesterol and LDL cholesterol in young, mildly hypercholesterolemic men. Am. J. Clin. Nutr. 76, 57–64. doi: 10.1093/ajcn/76.1.57
McClure, M. M., McIntyre, P. B., and McCune, A. R. (2006). Notes on the natural diet and habitat of eight danionin fishes, including the zebrafish Danio rerio. J. Fish Biol. 69, 553–570. doi: 10.1111/j.1095-8649.2006.01125.x
Meguro, S., Hasumura, T., and Hase, T. (2015). Body fat accumulation in zebrafish is induced by a diet rich in fat and reduced by supplementation with green tea extract. PLoS One 10:e0120142. doi: 10.1371/journal.pone.0120142
Mel’nikov, S. M., ten Hoorn, J., and Eijkelenboom, A. (2004). Effect of phytosterols and phytostanols on the solubilization of cholesterol by dietary mixed micelles: an in vitro study. Chem. Phys. Lipids 127, 121–141. doi: 10.1016/j.chemphyslip.2003.09.015
Miller, M. R., Nichols, P. D., and Carter, C. G. (2008). The digestibility and accumulation of dietary phytosterols in Atlantic salmon (Salmo salar L.) smolt fed diets with replacement plant oils. Lipids 43, 549–557. doi: 10.1007/s11745-008-3175-4
Norambuena, F., Lewis, M., Hamid, N. K. A., Hermon, K., Donald, J. A., and Turchini, G. M. (2013). Fish oil replacement in current aquaculture feed: is cholesterol a hidden treasure for fish nutrition? PLoS One 8:e81705. doi: 10.1371/journal.pone.0081705
Ostlund, R. E., Spilburg, C. A., and Stenson, W. F. (1999). Sitostanol administered in lecithin micelles potently reduces cholesterol absorption in humans. Am. J. Clin. Nutr. 70, 826–831. doi: 10.1093/ajcn/70.5.826
Pinheiro, J., Bates, D., DebRoy, S., Sarkar, D., and R Development Core Team (2010). Nlme: Linear and Nonlinear Mixed Effects Models. R Package Version 3.1-137. Available at: https://CRAN.R-project.org/package=nlme
Plat, J., Van Onselen, E. N. M., Van Heugten, M. M. A., and Mensink, R. P. (2000). Effects on serum lipids, lipoproteins and fat soluble antioxidant concentrations of consumption frequency of margarines and shortenings enriched with plant stanol esters. Eur. J. Clin. Nutr. 54, 671–677. doi: 10.1038/sj.ejcn.1601071
R Development Core Team (2017). R: A Language and Environment for Statistical Computing. Vienna: R Foundation for Statistical Computing. Available at: http://www.R-project.org/
Salen, G., Ahrens, E. H., and Grundy, S. M. (1970). Metabolism of β-sitosterol in man. J. Clin. Invest. 49, 952–967. doi: 10.1172/JCI106315
Sanden, M., Hemre, G.-I., Måge, A., Lunestad, B. T., Espe, M., Lie, K. K., et al. (2017). Årsrapport for Prøver Innsamlet i 2016 PROGRAM for Overvåking av Fiskefôr. Bergen: NIFES, 51.
Sanden, M., Liland, N. S., Saele, O., Rosenlund, G., Du, S., Torstensen, B. E., et al. (2016). Minor lipid metabolic perturbations in the liver of Atlantic salmon (Salmo salar L.) caused by suboptimal dietary content of nutrients from fish oil. Fish Physiol. Biochem. 42, 1463–1480. doi: 10.1007/s10695-016-0233-3
Sissener, N. H., Rosenlund, G., Stubhaug, I., and Liland, N. S. (2018). Tissue sterol composition in Atlantic salmon (Salmo salar L.) depends on the dietary cholesterol content and on the dietary phytosterol:cholesterol ratio, but not on the dietary phytosterol content. Br. J. Nutr. 119, 599–609. doi: 10.1017/S0007114517003853
Smet, E. D., Mensink, R. P., and Plat, J. (2012). Effects of plant sterols and stanols on intestinal cholesterol metabolism: suggested mechanisms from past to present. Mol. Nutr. Food Res. 56, 1058–1072. doi: 10.1002/mnfr.201100722
The Association of Official Analytical Chemists [AOAC] (1995). AOAC Official Methods of Analysis, Crude Protein in Meat and Meat Products, Combustion Method, 16th Edn. Available at: https://assets.thermofisher.com/TFS-Assets/CMD/brochures/FL-42237-OEA-FlashSmart-Official-Methods-FL42237-EN.pdf
Keywords: phytosterols, cholesterol, cholesterol uptake, zebrafish, aquaculture
Citation: van Spankeren M, Sibinga N, Reinshol Ø, Torstensen BE, Sæle Ø and Liland NS (2019) A Single Meal Containing Phytosterols Does Not Affect the Uptake or Tissue Distribution of Cholesterol in Zebrafish (Danio rerio). Front. Mar. Sci. 6:92. doi: 10.3389/fmars.2019.00092
Received: 14 August 2018; Accepted: 18 February 2019;
Published: 11 March 2019.
Edited by:
Ivan Viegas, University of Coimbra, PortugalReviewed by:
Fotini Kokou, Ben-Gurion University of the Negev, IsraelSofia Engrola, Centro de Ciências do Mar (CCMAR), Portugal
Copyright © 2019 van Spankeren, Sibinga, Reinshol, Torstensen, Sæle and Liland. This is an open-access article distributed under the terms of the Creative Commons Attribution License (CC BY). The use, distribution or reproduction in other forums is permitted, provided the original author(s) and the copyright owner(s) are credited and that the original publication in this journal is cited, in accordance with accepted academic practice. No use, distribution or reproduction is permitted which does not comply with these terms.
*Correspondence: Nina S. Liland, nina.liland@hi.no
†Present address: Nathaniel Sibinga, Aquatic Animal Health Program, Department of Microbiology and Immunology, College of Veterinary Medicine, Cornell University, Ithaca, NY, United States; Øyvind Reinshol, Norges Fiskerimuseum, Bergen, Norway; Bente E. Torstensen, Nofima, Fyllingsdalen, Norway