- School of Zoology, Tel Aviv University, Tel Aviv, Israel
Identifying critical aggregation sites and behavioral patterns of imperiled species contributes to filling knowledge gaps essential for their conservation. Manta rays present a prominent example of such species, the populations of which are declining globally due to directed fishery, by-catch, and other anthropogenic stressors. Our goal was to explore manta ray aggregation sites in the Philippines in order to determine the factors governing the mantas’ visits – a knowledge gap essential to understand manta ecology, facilitate ecosystem-based fishery management, and promote sustainable manta-based ecotourism. Diving surveys, environmental conditions assessment, and autonomous cameras were employed to study manta behavior and visit patterns to a cleaning station cluster on a commonly fished seamount, visited by both Mobula birostris and Mobula alfredi. Our findings reveal several environmental conditions (e.g., sea state, moon illumination, and flow) that serve as predictors of manta presence/absence at the site. We suggest that these conditions affect both the behavior of the manta’s food (i.e., the spatial distribution of plankton) and the cleaning effectiveness of the cleaner wrasse, which consequently influence manta activity. The findings suggest a trade-off between cleaning and foraging: i.e., mantas tend to visit the cleaning stations when environmental conditions are less favorable for foraging but suitable for effective cleaning; while being absent from the cleaning stations when environmental conditions form plankton aggregations, ideal for efficient feeding. This study sheds light on manta behavior and habitat use on dynamic, small spatio-temporal scales (i.e., hundreds of meters to a few kilometers, hours to days). The acquired data may be applied in the planning of marine protected areas and in fishery management (e.g., to reduce the chances of manta bycatch by limiting fishing activities to periods of manta absence) as well as contribute to enhancing sustainable exploitation, such as ecotourism, by increasing the chances of diving encounters with manta rays.
Introduction
Knowledge of spatial and behavioral ecology is essential for effective conservation (Greggor et al., 2016; Groom et al., 2017), and particularly so for large, highly mobile species (Block et al., 2005; Jaine et al., 2014). A major challenge to facilitating fishery ecosystem-based management, sustainable exploitation, and protection, is that of the identification of critical habitats within the endangered species’ home range (Pendoley et al., 2014; McCauley et al., 2015), and an understanding of the visit patterns of these species to these habitats (Papastamatiou et al., 2012).
Manta rays present a prominent example of such wide-ranging mobile species. The largest batoid and among the largest filter-feeders in the sea, their habitat may cover vast areas (Homma, 1997). They provide diverse ecological functions for the marine environment such as control of plankton levels and “large food-falls” (O’Brien, 1979; Blumenshine and Hambright, 2003; Higgs et al., 2014; Ratnarajah et al., 2014), as well as being suppliers of nutrient subsidies (Williams et al., 2018). Likewise, manta rays are a source of livelihood for many stakeholders, such as fishermen, merchants, and tourism operators (Alava et al., 1997; Acebes, 2013; O’Malley et al., 2013). The existing conservation measures in regard to manta rays are mainly regulatory and legislative, such as the listing of the two species, Mobula alfredi and Mobula birostris, as “Vulnerable” and “Endangered,” respectively, under IUCN; their inclusion in Appendix-II in the CITES and CMS treaties; and local protection (Marshall et al., 2011). However, additional fisheries are continually being established (Croll et al., 2016) and manta ray populations are declining (Lewis et al., 2015; O’Malley et al., 2017), suggesting that these measures fall short of effective management plans, mainly due to lack of enforcement and an absence of alternative livelihood sources for the fisherfolk (Acebes and Tull, 2016).
For a management plan to be effective it must consider the ecology of the species. Mantas tend to aggregate at specific locations in order to feed and clean (Stevens, 2007; Rohner et al., 2013). Some of these locations, such as seamounts, are characterized by relatively shallow depth and high fish abundance (Morato et al., 2006; Clark, 2010), which make them attractive for fishing. Consequently, manta rays are more vulnerable in these sites to bycatch and injury (Marshall and Bennett, 2010; Amande et al., 2012). However, this tendency to aggregate also provides an opportunity for manta ray conservation (Clark et al., 2014) by way of MPA designation (Barner et al., 2015), the design of sustainable ecosystem-based fishery and protection management (Rohner et al., 2013; Stewart et al., 2016; Dill, 2017), and sound ecotourism. This requires bridging significant information gaps regarding local and regional manta behavior, ecology, habitat use, and vulnerability to human-related activities, and should encompass various spatio-temporal scales, including those relevant for dynamic management (i.e., hundreds of meters to a few kilometers and hours to days, respectively, Scales et al., 2018).
This study was carried out at a submerged seamount in the central Philippines, and sought to identify predictors of manta presence and absence at the site and to shed light on manta ecology in core habitat use areas (sensu Graham et al., 2016).
Materials and Methods
The Study Site
The study was conducted at “Manta Bowl” (locally known as Bondot Tacdogan; 12.6597°N, 123.7550°E), a submerged reef on the top of a seamount near Ticao Island, the Philippines. The seamount area is ca. 0.4 km2 and the reef flat is ∼0.06 km2, with a crest at a depth of 20 m that gently slopes down to 50 m and deeper, to a surrounding depth of ∼200 m. The Manta Bowl area experiences strong tidal currents and both upwelling and downwelling currents. The flow regime and plankton-rich water create optimal conditions for resident reef-dwelling fish, schools of semi-pelagic fish such as Jacks (family Carangidae) and megafauna such as manta rays and whale sharks. Like many diversity “hotspots,” the site is highly fished by local and regional fishermen.
Surveys were conducted on the northernmost part of the seamount, within a rectangular area (ca. 200 m by 150 m) at a depth of 22 m sloping to 28 m toward north, west and east. The area is characterized by a patchy occurrence of soft corals interspersed with several types of massive coral species. The fish community comprises mainly reef and semi-pelagic fish species, with several pelagic species regularly frequenting the area. The survey area has a large concentration of cleaning stations inhabited by the cleaner wrasses Labroides bicolor, Labroides pictoides, and Labroides dimidiatus.
Data Acquisition and Fieldwork Methodology
Data were acquired by deployment of autonomous cameras (Midland XTC-4001) near the cleaning stations.
All field work was carried out by the same two-man team. A total of 119 survey days were performed, constituting 28 days of preliminary surveys conducted in October 2012 and 91 survey days carried out between July and December 2014, which comprised the main portion of the study. A typical survey day comprised 3 dives, averaging 30–45 min/dive for the purpose of camera deployment near the selected cleaning stations. Cameras were positioned and turned on as quickly as possible to minimize disturbance. In situations in which stations were occupied by visiting megafauna species, the team would wait at a distance until the visitor had left the station, and then deploy the camera. Manta rays that were sighted during dives were not included in the analyses to avoid bias caused by diver presence.
Video Recording
Autonomous cameras were used for data acquisition in order to eliminate the risk of mantas being deterred by diver presence, as well as to provide continuous coverage of the site, enabling a “presence and absence” (rather than “presence only”) analysis. An array of cameras, each set to HD video mode and attached to a 2 kg weight, was used. Each camera was left to run for 3.5–4.3 h (until its battery was depleted), and was then retrieved. Two recording approaches were applied to account for diving safety limitations: (a) two consecutive days during which two cameras were working simultaneously at two separate sites until battery depletion, retrieved and then immediately replaced by fully charged cameras to continue recording, resulting in ∼7 h of coverage at each of the two sites; followed by (b) 1 day during which the team deployed 1–3 cameras working simultaneously at different sites, retrieved upon battery deletion, with no replacement, resulting in ∼3.5 h coverage at the sites. Cameras were deployed a total of 263 times, yielding ∼960 h of video. The time of first deployment was routinely changed between 07:00 and 10:00 to ensure sufficient coverage of the day.
Data Processing
Individual manta identification was performed by noting each animal’s unique ventral spot pattern (Marshall and Pierce, 2012) in accordance with Kitchen-Wheeler’s (2010) guidelines.
Each survey day was divided into 1-h periods. Environmental conditions and number of manta cleaning events were noted for each period.
Events were classifieds according to their time of onset. A single event was defined as the time from the appearance of an individual manta ray until it had left the cleaning station for at least 5 min. If the same individual re-visited either of the two stations more than 20 min after it had previously left it, this was considered as a separate event.
Environmental Conditions
Environmental conditions were measured, estimated, or taken from various data sources (Table 1). Parameters were considered to be continuous, binomial, or factorial in order to better represent the field and gain a deeper understanding of each state rather than to describe a linear trend.
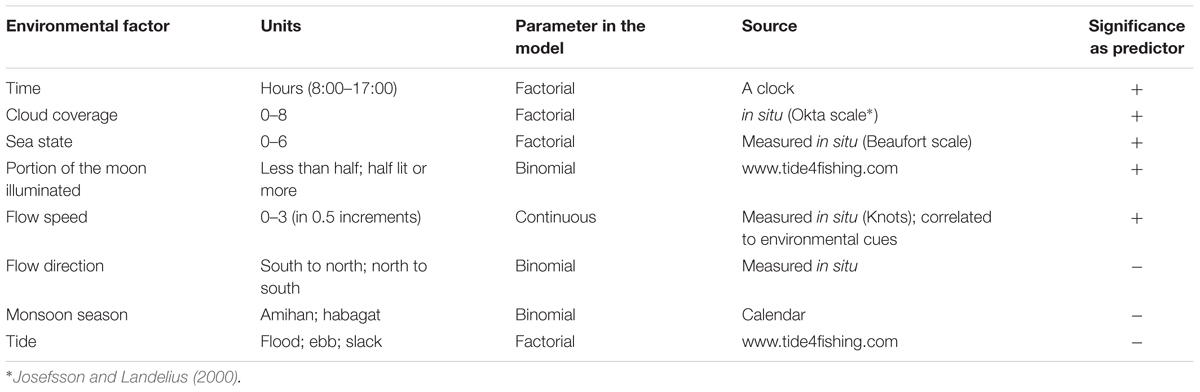
Table 1. Environmental factor, parameter statistical behavior, source, and significance as predictors of manta presence.
Cleaning Stations Identification
The identification of potential cleaning stations was made in accordance with Potts (1973): (a) by actually witnessing a cleaning interaction; (b) by locating a concentration or harem of cleaner wrasses; and (c) by observing aggression displays between adults and retreats to territorial patches.
Nine cleaning stations were identified during the preliminary phase. Two stations (Tamis Rock and Banger-II) yielded the largest number of sightings, and therefore were selected as the target sites for the data-acquisition phase of the study. The two stations were located ca. 180 m apart, facing different directions, and were very different in terms of their bathymetry; therefore, they were considered to be independent. The data obtained from the stations were not pooled but analyzed independently to enable comparison between the two.
Analysis of Environmental Factors
Environmental variables (Table 1) were tested for correlation to ensure only non-correlated factors are used for analysis.
The data were analyzed using a Generalized Linear Model (GLM; constructed in the program R), using a Negative-Binomial distribution with raw sightings per hour set as the response and environmental variables as predictors (Table 1). The model equation was Y = (Intercept) + β1 + β2 + ⋅⋅⋅...βn where (β ) is the estimate for each unique state of each variable, meaning the partial contribution of each level to the overall model, while (Y) is the estimated number of events per hour in the given conditions.
The outcome shows the partial contribution of each statistically significant factor to the probability of manta visits to the cleaning stations. All error bars and ribbons in the graphs represent standard errors; α was set to 0.05.
Model selection process was carried out individually for each cleaning station through Backward Elimination (omitting the least statistically significant variable from the model in each step), and comparison of AIC scores (Symonds and Moussalli, 2011; Tables 2, 3).
Results
A total of 876 manta visits were analyzed, and 8 environmental factors were analyzed (Table 1). A correlation was found between the Tide and Moon variables, and so Tide was not used in the analysis. The saturated models included seven variables and the final models for Tamis and Banger-II cleaning stations incorporated five and two explanatory factors, respectively (Tables 2, 3). These variables were found to have a significant effect on the number of cleaning events occurring at the study sites.
The output of the analysis shows the partial contribution of each variable on the number of estimated cleaning events at each cleaning station. Although the variables themselves were statistically significant, not all states within all variables were significant. The analysis findings were as follows:
Sea State
Both stations exhibited similarity in the trend of the effect, although not all levels were found significant. Manta presence at the Tamis Rock cleaning station was low during calm-to-moderate sea states (level 3, p = 0.04; Figure 1A). At Banger-II, the number of cleaning events was low during calm sea (level 1, p = 0.02; Figure 1A), increased during calm-to-moderate sea (level 3, p = 0.006; level 4, p = 0.01; Figure 1A), and then decreased as the sea became rougher (level 5, p = 0.01; Figure 1A). Although level 4 in the analysis for Tamis Rock was not found significant, it exhibits a strong similarity to the parallel significant level for Banger-II.
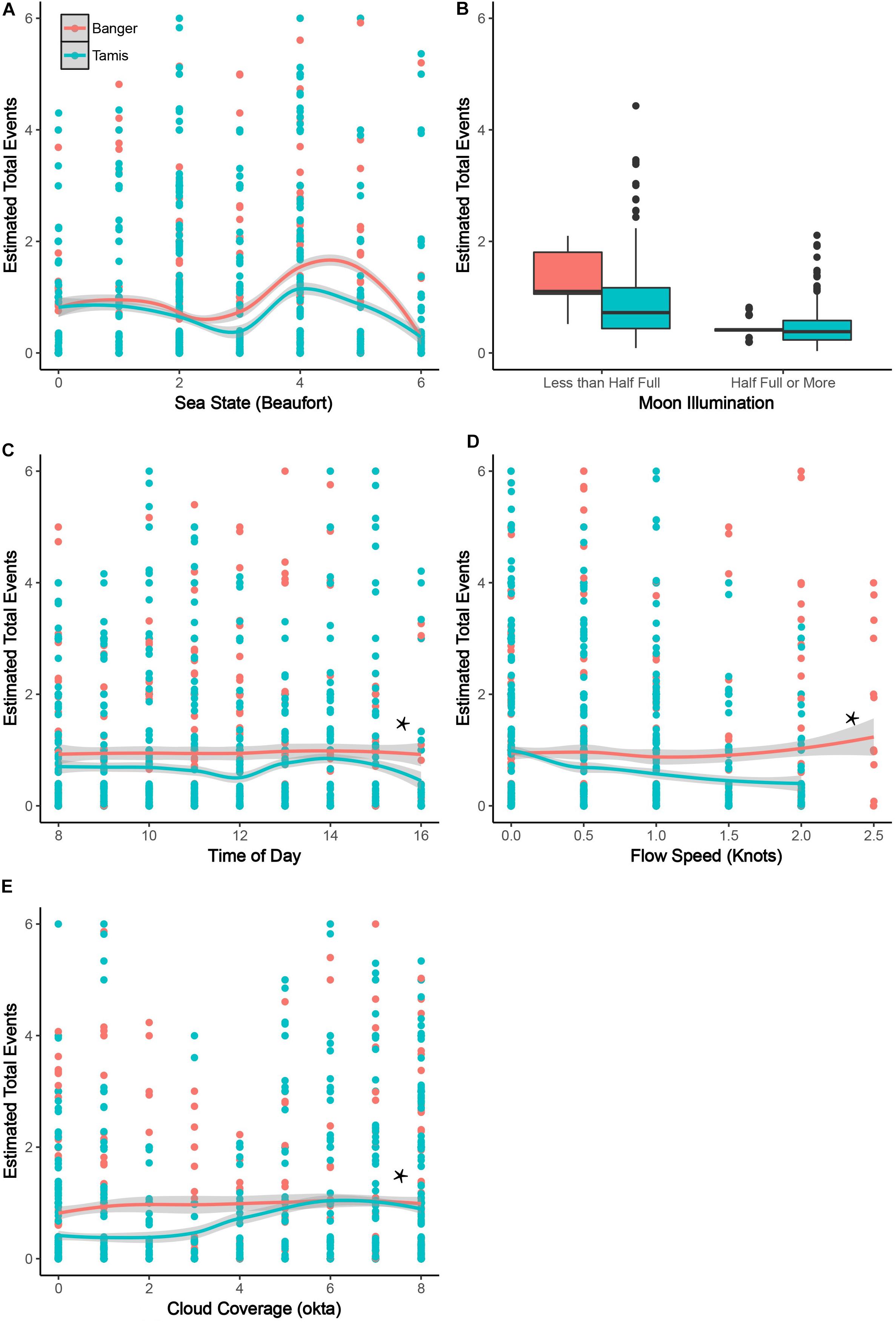
Figure 1. Generalized linear model output – Partial effect of environmental factors on manta presence at the two cleaning stations (Banger-II and Tamis Rock) in the Manta Bowl seamount. Results of the analysis of the interaction between environmental factors and manta cleaning events in Tamis Rock (colored blue) and Banger-II (colored red). Each plot presents the partial contribution of the factor as a predictor of manta cleaning events. Factors in plots (A,B) were found significant in both cleaning stations. Factors in plots (C–E) (marked with Asterisk) were only found significant in Tamis Rock station. In each plot the y-axis represents number of cleaning events witnessed during the state represented in the x-axis. All error bars and ribbons in the graphs represent standard errors; α was set to 0.05.
Moon Illumination
Manta presence at the cleaning stations was significantly higher when the moon was less than half full at both Tamis Rock (p = 0.009; Figure 1B) and Banger-II (p = 6.8e-13; Figure 1B).
Time of Day
Manta ray presence was significantly lower at 12:00 and 16:00 (p = 0.05; p = 0.01, respectively; Figure 1C) at Tamis Rock. The factor was found marginally significant at Banger-II station.
Flow Speed
Manta presence showed a reverse correlation to the flow speed at Tamis Rock (p = 0.007; Figure 1D), with fewer mantas present at the cleaning station as the flow speed increased. This factor was not found significant at Banger-II.
Cloud Coverage
Higher manta presence in the cleaning stations correlated with higher levels of cloud coverage at Tamis Rock (levels 5, 6, 7, and 8; p = 3.14e-07, p = 0.008, p = 0.01, and p = 0.001, respectively; Figure 1E). This factor was not found significant at Banger-II station.
Discussion
Five environmental factors were found to be potential predictors of manta ray presence/absence at the cleaning stations: Sea state, Moon illumination, Time of day, Flow speed, and Cloud coverage. Due to a correlation between the Tide and Moon factors, only the Moon variable was analyzed. These factors affect the marine environment mainly via (a) light characteristic in the water (Cohen and Forward, 2005; Gernez and Antoine, 2009), and (b) hydrodynamic processes (Kingsford et al., 1991; Rinke et al., 2007). How these factors affect manta behavior may derive from these species’ aggregation behavior, which is associated with various aspects of manta life-history traits – cleaning, feeding, and courtship (Stevens, 2007; Anderson et al., 2011; Marshall et al., 2011). Observations suggest that these activities are distinct in time (Rohner et al., 2013) and place (O’Shea et al., 2010, present study). For instance, no feeding occurs while at the cleaning stations.
Being large planktivores, mantas consume large quantities of plankton (Motta et al., 2010) and thus need also to allocate significant time for feeding. Needing to feed in an energy efficient manner (Dewar et al., 2008; Meekan et al., 2015), mantas take advantage of concentrated patches of plankton (Armstrong et al., 2016), which are often formed by mass coordinated movement triggered by various environmental cues (Gibson, 1992; Nelson et al., 1997; Sims et al., 2005; Rohner et al., 2015). This need may be even more extreme in oligotrophic waters (Pennington et al., 2006; Zerbini et al., 2006), such as in the Philippines, where plankton abundance is extremely low and spatio-temporally varied (Lalli and Parsons, 1997). Cleaning is another important time-consuming need for the mantas’ well-being (Ros et al., 2011), and may be triggered by parasite load (Grutter, 1999; Treasurer, 2002) and their physical health (Bshary, 2003; Waldie et al., 2011). While some animal species may locate a cleaning station by accident, and need to rediscover it periodically (Schofield et al., 2017), some manta rays are seen at the same stations repeatedly for many years, to the point that they may even be considered as “temporal residents” (Yano et al., 1999; Deakos et al., 2011), suggesting that not only do mantas clean at specific times, but they also seek specific stations.
Assuming that both cleaning and feeding are crucial for the mantas’ survival, there is probably a trade-off between the two activities (Schofield et al., 2017). Consequently, we suggest that the mantas’ decision to visit a cleaning station is probably dictated by: (1) feeding effectiveness (i.e., plankton availability, distribution, and concentration) (Dewar et al., 2008; Marshall and Bennett, 2010; Armstrong et al., 2016); and (2) cleaning effectiveness (i.e., cleaner fish activity hours and service quality) (Bshary and Schäffer, 2002; Oliver et al., 2011; Rohner et al., 2013). We suggest that the above-noted environmental factors affect both plankton density and concentration and the service quality of the cleaner wrasses, creating conditions in which it becomes more favorable for mantas either to feed or to clean, and thereby indirectly influencing the mantas’ behavior and presence at cleaning stations (Figure 2).
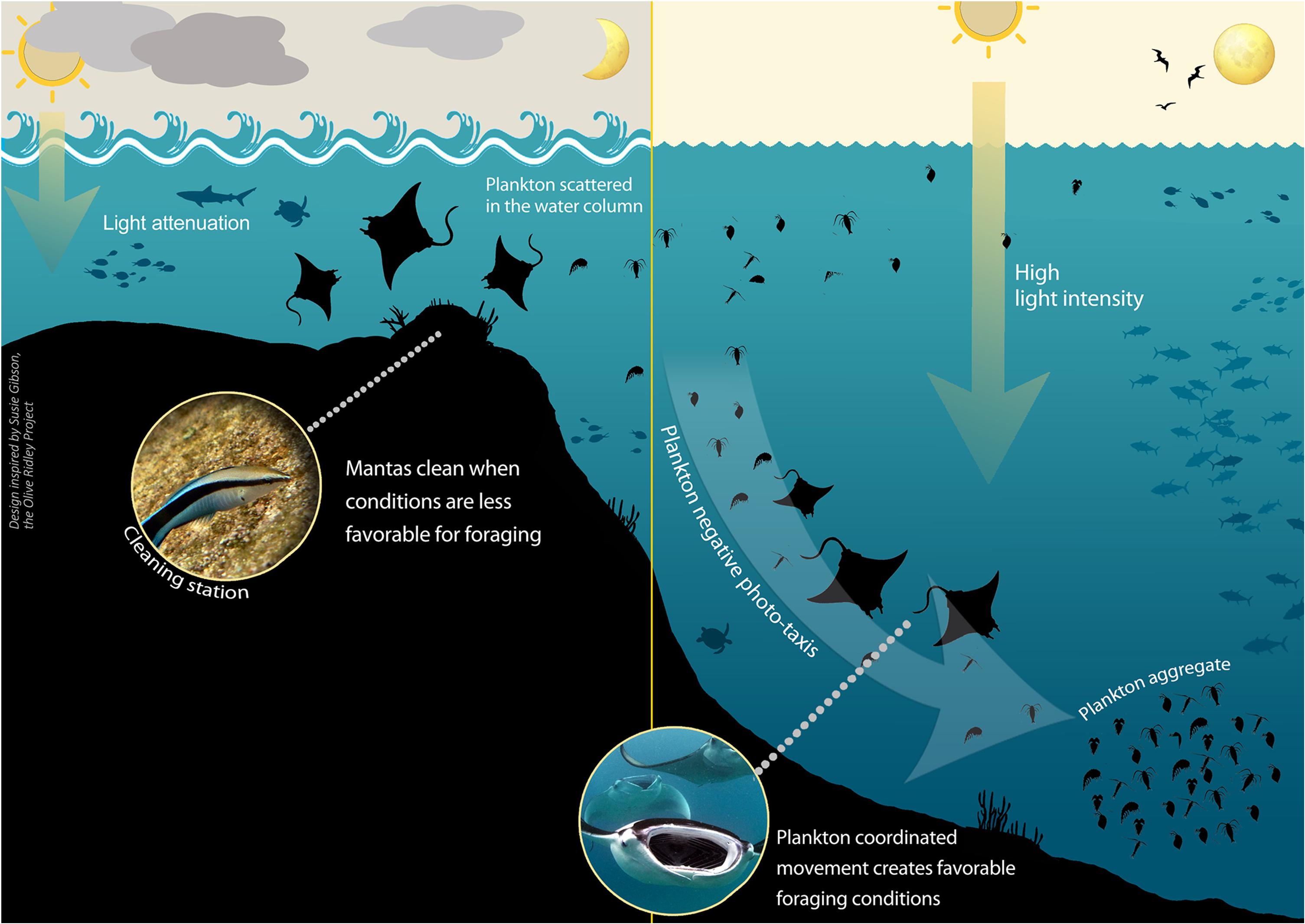
Figure 2. Environmental conditions dictating manta ray behavior and location. A summary of the proposed mechanism of how Time of day (particularly the angle of the sun), Sea state, Cloud coverage, and Moon illumination affect plankton movement and distribution, and hence manta presence at the cleaning stations. At times of high light intensity plankton undergo mass coordinated movement, thus their density and concentration increase, facilitating prime foraging conditions and resulting in a decline in manta presence at the cleaning stations. Light intensity is reduced by moderate to high cloud coverage, reduced angle of the sun (affected by time of day), less illuminated moon phases, and moderate sea state. Not pictured but addressed in the study are the effects of cleaner wrasse presence and strong turbulence.
Feeding Effectiveness
Manta feeding effectiveness is dictated by the density and concentration of its planktonic prey (Sims et al., 2005; Armstrong et al., 2016). Plankton are greatly affected by the light characteristic via phototaxis (Hardy and Bainbridge, 1954; Bollens et al., 1992; Harrison et al., 2013). Hence, plankton patches of high density and large biomass can be formed as a response to the time of day (Yahel et al., 2005), water turbidity (Gernez and Antoine, 2009; Hieronymi and Macke, 2010), cloud coverage (Stramska and Dickey, 1998; Anthony et al., 2004), and the illumination of the moon (Cohen and Forward, 2005; Benoit-Bird et al., 2009). The concentration and density of plankton in the water are also affected by hydrodynamic processes (Ledbetter, 1979; Evans and Taylor, 1980) such as Langmuir circulation or other up/downwelling flows (Kingsford et al., 1991; Rinke et al., 2007). High or low plankton density can thus be the result of large-scale turbulence and directional water motion (Denman and Gargett, 1983; Yen and Bundock, 1997).
We found that manta ray presence at the cleaning stations was correlated with those conditions that may cause low light intensity over a short time scale (i.e., hours), affected mainly by the time of day, sea surface state, and cloud coverage; or long time scale (i.e., days), affected by the moon illumination. Under low-light conditions, plankton concentration will be low, as there will be no plankton mass coordinated movement due to a lack of environmental cues. When the plankton are scattered, foraging will be less efficient (Armstrong et al., 2016), and it will therefore be more beneficial for the manta rays to visit the cleaning stations (Figure 2).
In contrast, mantas were notably absent from the cleaning stations at midday and when the sky was clear of clouds, as well as during periods when the moon was more than half full. Under these conditions, light intensity in the water is high, resulting in the formation of plankton aggregations, triggering manta foraging behavior (Armstrong et al., 2016), and the consequent absence of mantas from the cleaning stations (Figure 2).
Cleaning Effectiveness
Cleaning effectiveness is mostly affected by the performance of the cleaner wrasses. Cleaning at the study site was found to be performed almost solely by the diurnal blue streak cleaner wrasse Labroides dimidiatus, whose activity hours are from sunrise to sunset (Slobodkin and Fishelson, 1974). Cleaner wrasses are also affected by hydrodynamic processes, such high flow speed, which may impair the cleaning performance (Bellwood and Wainwright, 2001; Oliver et al., 2011), and so may cause the client to leave the station (Bshary and Schäffer, 2002). Consequently, both the cleaner wrasses’ activity hours and their ability to perform may affect the mantas’ decision to visit the cleaning stations.
We found that manta ray presence at the cleaning stations correlated with the activity hours of the cleaner wrasses and with conditions of relatively slow water flow (Figures 1C,D). Under these conditions, the cleaner wrasses’ more effective service may attract clients to the stations (Bshary and Schäffer, 2002). In contrast, mantas were notably absent from the cleaning stations around 16:00 h; during rough seas; and when the flow speed increased. Under these conditions, the cleaner wrasses’ performance will be impaired, leading to clients leaving the station.
Conclusion
This study integrates a wide range of findings regarding both biotic and abiotic aspects of the marine environment, with new observations based on presence and absence datasets rather than presence only datasets. This innovative approach provides insights into the manta rays’ ecology and behavior: notably, their decision-making regarding energy-efficient feeding or effective cleaning. Five environmental factors, which affect either the mantas’ planktonic prey or the cleaner wrasses via phototactic and hydrodynamic induced processes (Figure 2), were found to be successful predictors of manta ray presence/absence at cleaning stations. Although manta behavior is probably also affected by other factors, the five above-noted factors seem to play a significant part in dictating their behavior.
Implications for Manta Ray Conservation
Identifying the critical habitats of manta rays, such as off-shore cleaning stations, and understanding the patterns of their visits there, are essential for the effective ecosystem-based management and conservation of manta rays. The accessible depth and rich fauna of these habitats make them attractive for fishing and, consequently, mantas visiting there are more vulnerable to fishing, bycatch and, incidental injury. However, the same sites can also be harnessed to the design of sustainable fishery management and protection.
Our findings suggest that several easily measured environmental factors can serve as predictors of manta ray behavior and habitat use, i.e., their presence at cleaning stations. The use of these predictors can be applied as an efficient ecosystem-based management tool to minimize manta ray bycatch or incidental injury, by, for instance, limiting fishing in the vicinity of the cleaning stations during times of high likelihood of manta presence. Likewise, the same prediction-based tools may be used to improve manta-based ecotourism, by embarking on manta encounter dives at sites and times where manta presence is more likely, and thereby increasing the chances of a manta encounter, and improving ecotourism. Such applications may promote socio-ecological setups that enable manta population recovery while also maintaining coastal communities’ livelihood. In contrast to total fishery bans, which may drive entire populations and towns to hunger and unemployment, or to the emergence of illegal “underground markets,” such setups have a higher chance of successful implementation.
Data Availability
The raw data supporting the conclusions of this manuscript will be made available by the authors, without undue reservation, to any qualified researcher.
Author Contributions
YB designed the study concept, performed the fieldwork and analyses, and drafted the manuscript. AA supervised the scientific integrity, provided insights into the study’s scientific and ecological significance, and revised the manuscript.
Funding
This work was supported by the “Rufford Foundation” (Grant Number 14464-1).
Conflict of Interest Statement
The authors declare that the research was conducted in the absence of any commercial or financial relationships that could be construed as a potential conflict of interest.
Acknowledgments
The authors wish to thank Mr. Medel Silvosa and Mr. Marvin Mondrano for their role in the study’s fieldwork, Dr. Simon Oliver of TSRPC, Liat Shenhav and Or Givan of Tel-Aviv University, and Dotan Shalev of Marathon Results. The authors also wish to thank Naomi Paz and Natalie Levy for their assistance, and Rico Calleja, Jessica Noelle Wong, and the people at Ticao Island Resort, the Philippines, for their support and assistance.
Footnotes
References
Acebes, J. M. (2013). Hunting “Big Fish”: A Marine Environmental History of a Contested Fishery in the Bohol sea. Ph.D. thesis, Murdoch University, Perth.
Acebes, J. M. V., and Tull, M. (2016). The history and characteristics of the mobulid ray fishery in the Bohol Sea, Philippines. PLoS One 11:e0161444. doi: 10.1371/journal.pone.0161444
Alava, M. N. R., Dolumbaló, E. R. Z., Yaptinchay, A. A., and Trono, R. B. (1997). “Fishery and trade of whale sharks and manta rays in the Bohol Sea, Philippines,” in Elasmobranch Biodiversity, Conservation and Management: Proceedings of the International Seminar and Workshop, eds S. L. Fowler, T. M. Reed, and F. A. Dipper (Gland: IUCN), 132–148.
Amande, M. J., Chassot, E., Chavance, P., Murua, H., de Molina, A. D., and Bez, N. (2012). Precision in bycatch estimates: the case of tuna purse-seine fisheries in the Indian Ocean. ICES J. Mar. Sci. 69, 1501–1510. doi: 10.1093/icesjms/fss106
Anderson, R. C., Adam, M. S., and Goes, J. I. (2011). From monsoons to mantas: seasonal distribution of Manta alfredi in the Maldives. Fish. Oceanogr. 20, 104–113. doi: 10.1111/j.1365-2419.2011.00571.x
Anthony, K. R., Ridd, P. V., Orpin, A. R., Larcombe, P., and Lough, J. (2004). Temporal variation of light availability in coastal benthic habitats: effects of clouds, turbidity, and tides. Limnol. Oceanogr. 49, 2201–2211. doi: 10.4319/lo.2004.49.6.2201
Armstrong, A. O., Armstrong, A. J., Jaine, F. R., Couturier, L. I., Fiora, K., Uribe-Palomino, J., et al. (2016). Prey density threshold and tidal influence on reef manta ray foraging at an aggregation site on the Great Barrier Reef. PLoS One 11:e0153393. doi: 10.1371/journal.pone.0153393
Barner, A. K., Lubchenco, J., Costello, C., Gaines, S. D., Leland, A., Jenks, B., et al. (2015). Solutions for recovering and sustaining the bounty of the ocean: combining fishery reforms, rights-based fisheries management, and marine reserves. Oceanography 28, 252–263. doi: 10.5670/oceanog.2015.51
Bellwood, D., and Wainwright, P. (2001). Locomotion in labrid fishes: implications for habitat use and cross-shelf biogeography on the Great Barrier Reef. Coral Reefs 20, 139–150. doi: 10.1007/s003380100156
Benoit-Bird, K. J., Au, W. W., and Wisdoma, D. W. (2009). Nocturnal light and lunar cycle effects on diel migration of micronekton. Limnol. Oceanogr. 54, 1789–1800. doi: 10.4319/lo.2009.54.5.1789
Block, B. A., Teo, S. L., Walli, A., Boustany, A., Stokesbury, M. J., Farwell, C. J., et al. (2005). Electronic tagging and population structure of Atlantic bluefin tuna. Nature 434, 1121–1127. doi: 10.1038/nature03463
Blumenshine, S. C., and Hambright, K. D. (2003). Top-down control in pelagic systems: a role for invertebrate predation. Hydrobiologia 491, 347–356. doi: 10.1023/A:1024422121048
Bollens, S. M., Frost, B. W., Thoreson, D. S., and Watts, S. J. (1992). Diel vertical migration in zooplankton: field evidence in support of the predator avoidance hypothesis. Hydrobiologia 234, 33–39. doi: 10.1007/BF00010777
Bshary, R. (2003). The cleaner wrasse, Labroides dimidiatus, is a key organism for reef fish diversity at Ras Mohammed National Park, Egypt. J. Anim. Ecol. 72, 169–176. doi: 10.1046/j.1365-2656.2003.00683.x
Bshary, R., and Schäffer, D. (2002). Choosy reef fish select cleaner fish that provide high-quality service. Anim. Behav. 63, 557–564. doi: 10.1006/anbe.2001.1923
Clark, M. R., Rowden, A. A., Schlacher, T. A., Guinotte, J., Dunstan, P. K., Williams, A., et al. (2014). Identifying ecologically or biologically significant areas (EBSA): a systematic method and its application to seamounts in the South Pacific Ocean. Ocean Coast. Manag. 91, 65–79. doi: 10.1016/j.ocecoaman.2014.01.016
Clark, T. B. (2010). Abundance, Home Range, and Movement Patterns of Manta Rays (Manta alfredi, M. birostris) in Hawai′i. Ph.D. dissertation, University of Hawaii at Manoa, Honolulu, HI.
Cohen, J. H., and Forward, R. B. (2005). Diel vertical migration of the marine copepod Calanopia americana. I. Twilight DVM and its relationship to the diel light cycle. Mar. Biol. 147, 387–398. doi: 10.1007/s00227-005-1569-x
Croll, D. A., Dewar, H., Dulvy, N. K., Fernando, D., Francis, M. P., Galván-Magaña, F., et al. (2016). Vulnerabilities and fisheries impacts: the uncertain future of manta and devil rays. Aquat. Conserv. Mar. Freshw. Ecosyst. 26, 562–575. doi: 10.1002/aqc.2591
Deakos, M. H., Baker, J. D., and Bejder, L. (2011). Characteristics of a manta ray Manta alfredi population off Maui, Hawaii, and implications for management. Mar. Ecol. Prog. Ser. 429, 245–260. doi: 10.3354/meps09085
Denman, K. L., and Gargett, A. E. (1983). Time and space scales of vertical mixing and advection of phytoplankton in the upper ocean. Limnol. Oceanogr. 28, 801–815. doi: 10.4319/lo.1983.28.5.0801
Dewar, H., Mous, P., Domeier, M., Muljadi, A., Pet, J., and Whitty, J. (2008). Movements and site fidelity of the giant manta ray, Manta birostris, in the Komodo Marine Park, Indonesia. Mar. Biol. 155, 121–133. doi: 10.1007/s00227-008-0988-x
Dill, L. M. (2017). Behavioural ecology and marine conservation: a bridge over troubled water? ICES J. Mar. Sci. 74, 1514–1521. doi: 10.1093/icesjms/fsx034
Evans, G. T., and Taylor, F. J. R. (1980). Phytoplankton accumulation in Langmuir cells1. Limnol. Oceanogr. 25, 840–845. doi: 10.4319/lo.1980.25.5.0840
Gernez, P., and Antoine, D. (2009). Field characterization of wave-induced underwater light field fluctuations. J. Geophys. Res. Oceans 114, 1–15.
Gibson, R. N. (1992). “Tidally-synchronised behaviour in marine fishes,” in Rhythms in Fishes, ed. M. A. Ali (Boston, MA: Springer), 63–81.
Graham, F., Rynne, P., Estevanez, M., Luo, J., Ault, J. S., and Hammerschlag, N. (2016). Use of marine protected areas and exclusive economic zones in the subtropical western North Atlantic Ocean by large highly mobile sharks. Divers. Distrib. 22, 534–546. doi: 10.1111/ddi.12425
Greggor, A. L., Berger-Tal, O., Blumstein, D. T., Angeloni, L., Bessa-Gomes, C., Blackwell, B. F., et al. (2016). Research priorities from animal behaviour for maximising conservation progress. Trends Ecol. Evol. 31, 953–964. doi: 10.1016/j.tree.2016.09.001
Groom, C., White, N. E., Mitchell, N., Roberts, J. D., and Mawson, P. (2017). Assessing the spatial ecology and resource use of a mobile and endangered species in an urbanized landscape using satellite telemetry and DNA faecal metabarcoding. Ibis 159, 390–405. doi: 10.1111/ibi.12442
Hardy, A. C., and Bainbridge, R. (1954). Experimental observations on the vertical migrations of plankton animals. J. Mar. Biol. Assoc. U.K. 33, 409–448. doi: 10.1017/S0025315400008456
Harrison, P. M., Gutowsky, L. F. G., Martins, E. G., Patterson, D. A., Leake, A., Cooke, S. J., et al. (2013). Diel vertical migration of adult burbot: a dynamic trade-off among feeding opportunity, predation avoidance, and bioenergetic gain. Can. J. Fish. Aquat. Sci. 70, 1765–1774. doi: 10.1139/cjfas-2013-0183
Hieronymi, M., and Macke, A. (2010). Spatiotemporal underwater light field fluctuations in the open ocean. J. Eur. Opt. Soc. Rapid Publ. 5. doi: 10.2971/jeos.2010.10019s
Higgs, N. D., Gates, A. R., and Jones, D. O. (2014). Fish food in the deep sea: revisiting the role of large food-falls. PLoS One 9:e96016. doi: 10.1371/journal.pone.0096016
Homma, K. (1997). “Biology of the manta ray, Manta birostris Walbaum, in the Indo-Pacific,” in Proceedings of the 5th Indo Pacific Fish Conference, Nouméa, 1997, eds B. Séret, and J.-Y. Sire (Paris: Societe’ Franc,aise d’Ichthyologie), 209–216.
Jaine, F. R. A., Rohner, C. A., Weeks, S. J., Couturier, L. I. E., Bennett, M. B., Townsend, K. A., et al. (2014). Movements and habitat use of reef manta rays off eastern Australia: offshore excursions, deep diving and eddy affinity revealed by satellite telemetry. Mar. Ecol. Prog. Ser. 510, 73–86. doi: 10.3354/meps10910
Josefsson, W., and Landelius, T. (2000). Effect of clouds on UV irradiance: as estimated from cloud amount, cloud type, precipitation, global radiation and sunshine duration. J. Geophys. Res. Atmos. 105, 4927–4935. doi: 10.1029/1999JD900255
Kingsford, M. J., Wolanski, E., and Choat, J. H. (1991). Influence of tidally induced fronts and Langmuir circulations on distribution and movements of presettlement fishes around a coral reef. Mar. Biol. 109, 167–180. doi: 10.1007/BF01320244
Kitchen-Wheeler, A. M. (2010). Visual identification of individual manta ray (Manta alfredi) in the Maldives Islands, Western Indian Ocean. Mar. Biol. Res. 6, 351–363. doi: 10.1080/17451000903233763
Lalli, C., and Parsons, T. R. (1997). Biological Oceanography: An Introduction. Amsterdam: Elsevier.
Ledbetter, M. (1979). Langmuir circulations and plankton patchiness. Ecol. Model. 7, 289–310. doi: 10.1016/0304-3800(79)90039-5
Lewis, S. A., Setiasih, N., Fahmi, F., Dharmadi, D., O’Malley, M. P., Campbell, S. J., et al. (2015). Assessing Indonesian manta and devil ray populations through historical landings and fishing community interviews. PeerJ 3:e1642. doi: 10.13140/RG.2.1.3033.0323
Marshall, A. D., and Bennett, M. B. (2010). The frequency and effect of shark-inflicted bite injuries to the reef manta ray Manta alfredi. Afr. J. Mar. Sci. 32, 573–580. doi: 10.2989/1814232X.2010.538152
Marshall, A. D., Dudgeon, C. L., and Bennett, M. B. (2011). Size and structure of a photographically identified population of manta rays Manta alfredi in southern Mozambique. Mar. Biol. 158, 1111–1124. doi: 10.1007/s00227-011-1634-6
Marshall, A. D., and Pierce, S. J. (2012). The use and abuse of photographic identification in sharks and rays. J. Fish Biol. 80, 1361–1379. doi: 10.1111/j.1095-8649.2012.03244.x
McCauley, D. J., Pinsky, M. L., Palumbi, S. R., Estes, J. A., Joyce, F. H., and Warner, R. R. (2015). Marine defaunation: animal loss in the global ocean. Science 347:1255641. doi: 10.1126/science.1255641
Meekan, M., Fuiman, L., Davis, R., Berger, Y., and Thums, M. (2015). Swimming strategy and body plan of the world’s largest fish: implications for foraging efficiency and thermoregulation. Front. Mar. Sci. 2:64. doi: 10.3389/fmars.2015.00064
Morato, T., Cheung, W. W., and Pitcher, T. J. (2006). Vulnerability of seamount fish to fishing: fuzzy analysis of life-history attributes. J. Fish Biol. 68, 209–221. doi: 10.1111/j.0022-1112.2006.00894.x
Motta, P. J., Maslanka, M., Hueter, R. E., Davis, R. L., De la Parra, R., Mulvany, S. L., et al. (2010). Feeding anatomy, filter-feeding rate, and diet of whale sharks Rhincodon typus during surface ram filter feeding off the Yucatan Peninsula, Mexico. Zoology 113, 199–212. doi: 10.1016/j.zool.2009.12.001
Nelson, D. R., McKibben, J. N., Strong, W. R., Lowe, C. G., Sisneros, J. A., Schroeder, D. M., et al. (1997). An acoustic tracking of a megamouth shark, Megachasma pelagios: a crepuscular vertical migrator. Environ. Biol. Fishes 49, 389–399. doi: 10.1023/A:1007369619576
O’Brien, W. J. (1979). The predator-prey interaction of planktivorous fish and zooplankton: recent research with planktivorous fish and their zooplankton prey shows the evolutionary thrust and parry of the predator-prey relationship. Am. Sci. 67, 572–581.
Oliver, S. P., Hussey, N. E., Turner, J. R., and Beckett, A. J. (2011). Oceanic sharks clean at coastal seamount. PLoS One 6:e14755. doi: 10.1371/journal.pone.0014755
O’Malley, M. P., Lee-Brooks, K., and Medd, H. B. (2013). The global economic impact of manta ray watching tourism. PLoS One 8:e65051. doi: 10.1371/journal.pone.0065051
O’Malley, M. P., Townsend, K. A., Hilton, P., Heinrichs, S., and Stewart, J. D. (2017). Characterization of the trade in manta and devil ray gill plates in China and South-east Asia through trader surveys. Aquat. Conserv. Mar. Freshw. Ecosyst. 27, 394–413. doi: 10.1002/aqc.2670
O’Shea, O. R., Kingsford, M. J., and Seymour, J. (2010). Tide-related periodicity of manta rays and sharks to cleaning stations on a coral reef. Mar. Freshw. Res. 61, 65–73. doi: 10.1071/MF08301
Papastamatiou, Y. P., DeSalles, P. A., and McCauley, D. J. (2012). Area-restricted searching by manta rays and their response to spatial scale in lagoon habitats. Mar. Ecol. Prog. Ser. 456, 233–244. doi: 10.3354/meps09721
Pendoley, K. L., Schofield, G., Whittock, P. A., Ierodiaconou, D., and Hays, G. C. (2014). Protected species use of a coastal marine turtle migratory corridor connecting Australian MPAs. Mar. Biol. 161, 1455–1466. doi: 10.1007/s00227-014-2433-7
Pennington, J. T., Mahoney, K. L., Kuwahara, V. S., Kolber, D. D., Calienes, R., and Chavez, F. P. (2006). Primary production in the eastern tropical Pacific: a review. Prog. Oceanogr. 69, 285–317. doi: 10.1016/j.pocean.2006.03.012
Potts, G. W. (1973). The ethology of Labroides dimidiatus (cuv. & val.)(Labridae, Pisces) on Aldabra. Anim. Behav. 21, 250–291. doi: 10.1016/S0003-3472(73)80068-5
Ratnarajah, L., Bowie, A. R., Lannuzel, D., Meiners, K. M., and Nicol, S. (2014). The biogeochemical role of baleen whales and krill in Southern Ocean nutrient cycling. PLoS One 9:e114067. doi: 10.1371/journal.pone.0114067
Rinke, K., Hübner, I., Petzoldt, T., Rolinski, S., KÖNIG-RINKE, M. A. R. I. E., Post, J., et al. (2007). How internal waves influence the vertical distribution of zooplankton. Freshw. Biol. 52, 137–144. doi: 10.1111/j.1365-2427.2006.01687.x
Rohner, C. A., Armstrong, A. J., Pierce, S. J., Prebble, C. E., Cagua, E. F., Cochran, J. E., et al. (2015). Whale sharks target dense prey patches of sergestid shrimp off Tanzania. J. Plankton Res. 37, 352–362. doi: 10.1093/plankt/fbv010
Rohner, C. A., Pierce, S. J., Marshall, A. D., Weeks, S. J., Bennett, M. B., and Richardson, A. J. (2013). Trends in sightings and environmental influences on a coastal aggregation of manta rays and whale sharks. Mar. Ecol. Prog. Ser. 482, 153–168. doi: 10.3354/meps10290
Ros, A. F., Lusa, J., Meyer, M., Soares, M., Oliveira, R. F., Brossard, M., et al. (2011). Does access to the bluestreak cleaner wrasse Labroides dimidiatus affect indicators of stress and health in resident reef fishes in the Red Sea? Horm. Behav. 59, 151–158. doi: 10.1016/j.yhbeh.2010.11.006
Scales, K. L., Hazen, E. L., Jacox, M. G., Castruccio, F., Maxwell, S. M., Lewison, R. L., et al. (2018). Fisheries bycatch risk to marine megafauna is intensified in Lagrangian coherent structures. Proc. Natl. Acad. Sci. U.S.A. 115, 7362–7367. doi: 10.1073/pnas.1801270115
Schofield, G., Papafitsoros, K., Haughey, R., and Katselidis, K. (2017). Aerial and underwater surveys reveal temporal variation in cleaning-station use by sea turtles at a temperate breeding area. Mar. Ecol. Prog. Ser. 575, 153–164. doi: 10.3354/meps12193
Sims, D. W., Southall, E. J., Tarling, G. A., and Metcalfe, J. D. (2005). Habitat-specific normal and reverse diel vertical migration in the plankton-feeding basking shark. J. Anim. Ecol. 74, 755–761. doi: 10.1111/j.1365-2656.2005.00971.x
Slobodkin, L. B., and Fishelson, L. (1974). The effect of the cleaner-fish Labroides dimidiatus on the point diversity of fishes on the reef front at Eilat. Am. Nat. 108, 369–376. doi: 10.1086/282914
Stevens, J. D. (2007). Whale shark (Rhincodon typus) biology and ecology: a review of the primary literature. Fish. Res. 84, 4–9. doi: 10.1016/j.fishres.2006.11.008
Stewart, J. D., Beale, C. S., Fernando, D., Sianipar, A. B., Burton, R. S., Semmens, B. X., et al. (2016). Spatial ecology and conservation of Manta birostris in the Indo-Pacific. Biol. Conserv. 200, 178–183. doi: 10.1016/j.biocon.2016.05.016
Stramska, M., and Dickey, T. D. (1998). Short-term variability of the underwater light field in the oligotrophic ocean in response to surface waves and clouds. Deep Sea Res. Part I Oceanogr. Res. Pap. 45, 1393–1410. doi: 10.1016/S0967-0637(98)00020-X
Symonds, M. R., and Moussalli, A. (2011). A brief guide to model selection, multimodel inference and model averaging in behavioural ecology using Akaike’s information criterion. Behav. Ecol. Sociobiol. 65, 13–21. doi: 10.1007/s00265-010-1037-6
Treasurer, J. W. (2002). A review of potential pathogens of sea lice and the application of cleaner fish in biological control. Pest Manag. Sci. 58, 546–558. doi: 10.1002/ps.509
Waldie, P. A., Blomberg, S. P., Cheney, K. L., Goldizen, A. W., and Grutter, A. S. (2011). Long-term effects of the cleaner fish Labroides dimidiatus on coral reef fish communities. PLoS One 6:e21201. doi: 10.1371/journal.pone.0021201
Williams, J. J., Papastamatiou, Y. P., Caselle, J. E., Bradley, D., and Jacoby, D. M. (2018). Mobile marine predators: an understudied source of nutrients to coral reefs in an unfished atoll. Proc. Biol. Sci. 285:20172456. doi: 10.1098/rspb.2017.2456
Yahel, R., Yahel, G., Berman, T., Jaffe, J. S., and Genin, A. (2005). Diel pattern with abrupt crepuscular changes of zooplankton over a coral reef. Limnol. Oceanogr. 50, 930–944. doi: 10.4319/lo.2005.50.3.0930
Yano, K., Sato, F., and Takahashi, T. (1999). Observations of mating behavior of the manta ray, Manta birostris, at the Ogasawara Islands, Japan. Ichthyol. Res. 46, 289–296. doi: 10.1007/BF02678515
Yen, J., and Bundock, E. A. (1997). “Aggregate behavior in zooplankton: Phototactic swarming in four developmental stages of Coullana canadensis (Copepoda, Harpacticoida),” in Animal Groups in Three Dimensions: How Species Aggregate, eds J. K. Parrish, and W. M. Hamner (New York, NY: Cambridge University Press), 143–162.
Keywords: seamounts, conservation biology, ecotourism, spatial ecology, cleaning, remote cameras
Citation: Barr Y and Abelson A (2019) Feeding – Cleaning Trade-Off: Manta Ray “Decision-Making” as a Conservation Tool. Front. Mar. Sci. 6:88. doi: 10.3389/fmars.2019.00088
Received: 01 August 2018; Accepted: 14 February 2019;
Published: 15 March 2019.
Edited by:
Lyne Morissette, M – Expertise Marine, CanadaReviewed by:
Rebecca Ruth McIntosh, Phillip Island Nature Parks, AustraliaGail Schofield, Queen Mary University of London, United Kingdom
Copyright © 2019 Barr and Abelson. This is an open-access article distributed under the terms of the Creative Commons Attribution License (CC BY). The use, distribution or reproduction in other forums is permitted, provided the original author(s) and the copyright owner(s) are credited and that the original publication in this journal is cited, in accordance with accepted academic practice. No use, distribution or reproduction is permitted which does not comply with these terms.
*Correspondence: Yotam Barr, yotamanta@gmail.com