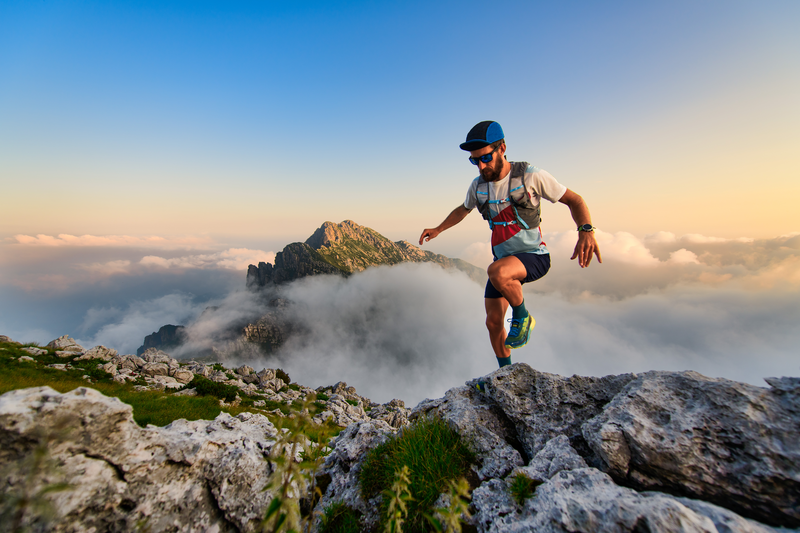
95% of researchers rate our articles as excellent or good
Learn more about the work of our research integrity team to safeguard the quality of each article we publish.
Find out more
ORIGINAL RESEARCH article
Front. Mar. Sci. , 26 February 2019
Sec. Marine Conservation and Sustainability
Volume 6 - 2019 | https://doi.org/10.3389/fmars.2019.00073
This article is part of the Research Topic SeaFlower Biosphere Reserve: New Findings and Trends in the Largest Caribbean Marine Protected Area View all 14 articles
Coral reef decline persists as a global issue with ties to climate change and human footprint. The SeaFlower Biosphere reserve includes some of the most isolated oceanic coral reefs in the Southwestern Caribbean, which provide natural experiments to test global and/or basin-wide factors affecting coral reefs. In this study, we compared coral and other substrate cover (algae, cyanobacteria, and octocorals), along population densities of keystone urchin species from two atolls (Serrana and Roncador Banks), during 1995, 2003, and 2015/2016. We also surveyed benthic foraminifera as a water quality proxy for coral growth in the last period. A steady reduction in coral cover was clearly observed at Roncador’s lagoon, but not at Serrana’s reefs, with significant differences between 1995 and 2015/2016. Percent cover of fleshy algae decreased significantly also at Roncador between 1995 and 2003 but did not change notably from 1995 to 2016 at Serrana. However, both Banks exhibited a loss in crustose coralline algae from 2003 to 2015/2016. Likewise, a reduction in bottom complexity, measured as bottom rugosity, was evident between 1995 and 2003. Roncador Bank had unprecedented high octocoral densities, which increased almost threefold from 2003 to 2015. In contrast, urchin densities were low in Roncador; only Diadema antillarum increased from 2003 to 2016 in Serrana Bank. The Foraminifera in Reef Assessment and Monitoring (FORAM) Index (FI) in the two Banks was below the range expected for healthy coral reefs. Although both Banks follow a reduction in CCA and CA cover, Roncador Bank also faces an alarming decline in coral cover, urchins and bottom complexity (rugosity) in contrast to increases in octocoral densities and potential loss of resilience and eutrophication suggested by the FI index. These unexpected findings led us to consider and discuss potential outcomes, where these reefs deteriorate (i.e., erode and drown) providing ideal conditions for octocoral growth. Hence, it is of utmost urgency to start monitoring reef budgets, octocorals and nutrient sources.
Marine biodiversity reaches its highest complexity in coral reefs. These formidable tropical ecosystems account for at least 30% of marine biodiversity at an extent of less than 1% of the planet’s surface (Roberts et al., 2002). In addition to offering a seascape of indescribable beauty, they provide significant economic benefits to countries where they are found (Costanza et al., 1997), as well as providing coastal protection from storms (i.e., hurricanes) and waves (i.e., tsunamis) (Foster et al., 2013). Direct and indirect economic profit from tourism provided the exploitation of coral reef ecosystems around the world surpass 36 billion dollars a year (Spalding et al., 2017). Yet, coral reefs face unprecedented environmental challenges (Camp et al., 2018). Thermal anomalies in the oceans lead to coral bleaching and mortality (Hughes et al., 2003; Eakin et al., 2010). Of greatest concern, the increase of atmospheric carbon dioxide (CO2) concentration alters seawater chemistry leading to ocean acidification, which includes adverse effects on the calcification rates of marine organisms including corals (Orr et al., 2005; Hoegh-Guldberg et al., 2007). These processes act in synergy with the human footprint, particularly increasing sewage and overexploitation, which reduces the resilience of marine ecosystems like coral reefs (Mumby, 2009; Graham et al., 2013; Wiedenmann et al., 2013). Failure to protect coral reefs will affect millions of livelihoods worldwide and provoke unparalleled rapid biodiversity losses in the years to come.
Biodiversity loss encompasses all marine coastal organisms (Hooper et al., 2012), in which coral reefs have become an emblematic instance of this global trend of ecosystem degradation. Recent trends, altering the composition of species assemblages at coastal marine environments (Dornelas et al., 2014), have raised new concerns about marine conservation because the emerging species turnover corresponds to less favorable ecosystem states in terms of productivity and services for humans (Pandolfi and Lovelock, 2014). Not understanding how marine communities react to many agents of deterioration and stress, climate change and human footprint, for example, prevents us from stopping degradation, managing and restoring vulnerable ecosystems like coral reefs (López-Angarita et al., 2014). In corals reefs, resilience and trophic functions appear greatly compromised (Jackson et al., 2001; Hughes et al., 2010). The worse-case scenario, in declining coral reefs, predicts high erosion and rising sea-levels leading to drowned reef structures with a few calcareous corals surviving and the hard bottom cover dominated by fleshy algae (Hughes et al., 2003; Hoegh-Guldberg et al., 2007, 2017; Perry et al., 2018). Are Caribbean coral reefs reaching this state?
The key players in coral reefs are set between top-down, grazing, and bottom-up, nutrient input controls (Littler et al., 2006). This relative dominance model allows an ideal coral-dominated shallow reef, including crustose coralline algae, under high grazing and low nutrient input (Littler and Littler, 2007). The worst case-scenario, under high nutrient input and low grazing, predicts a macroalgae dominated reef (Ledlie et al., 2007; Slattery and Lesser, 2014) or a sponge-dominated state, where dissolved organic matter is processed fueling higher trophic levels (De Goeij et al., 2013; McMurray et al., 2018). Reef decline in the Caribbean can be traced back to the time of Columbus, where reduction of herbivores like green turtles started, and more recently with the extinction of the monk seal and the mass mortalities of the black sea urchins and corals (Lessios et al., 1984; Hughes, 1994; Jackson, 1997). Basin-wide, Caribbean coral cover shrunk to 10% in less than three decades (Gardner et al., 2003). A combination of thermal anomalies, leading to coral bleaching and mortality (Eakin et al., 2010), overfishing of larger herbivores like parrotfishes (Paddack et al., 2009; Mumby et al., 2012; Loh et al., 2015), human footprint (Appeldoorn et al., 2016), including coastal pollution and eutrophication (Mora, 2008), and more recent invasive species (Lesser and Slattery, 2011; Albins and Hixon, 2013), reduced the resilience of coral reefs in the Caribbean like no other region in the world (Jackson et al., 2014).
Increased nutrient and sediment load in coastal areas are recognized as major drivers of coral reef deterioration worldwide (Fabricius, 2011; Sherman et al., 2016). In addition, coastal pollution can lead to disturbances in the coral microbiome and disease-related mortality (Klaus et al., 2007; Montilla et al., 2016). Traditional methods to evaluate reef condition do not effectively reflect the current water quality in terms of nutrients, due to a delayed response by the long-lived coral community and the associated costs of long-term monitoring (Cooper et al., 2009). Recently, the benthic foraminiferal assemblage has emerged as an economic and reliable alternative to assess water quality to support growth of symbiotic and calcifying organisms on coral reefs (Hallock et al., 2003). The “Foraminifera in Reef Assessment and Monitoring (FORAM) Index” (FI), is an efficient and cost-effective measure of water and sediment quality based on the assemblage of foraminiferal shells (Hallock, 2012). On oligotrophic and clear waters with abundance of hypercalcifying mixotrophs, such as hard corals, the shells of larger (symbiont-bearing) foraminifera account for a high proportion of sediment composition (Hallock, 1999). When nutrients increase the shells of smaller heterotrophic species dominate the assemblage, if conditions lead to accumulation of organic matter, stress-tolerant or opportunistic taxa can abound (Cockey et al., 1996).
Roncador and Serrana (locally known as “Islas Cayo,” hereafter referred as Banks) comprise two of the few true Darwinian atolls in the Caribbean (Figure 1). Surrounded by depths of over two thousand meters, these atolls enclose shallow lagoons (5–15 m), where most of the coral growth stands (Milliman, 1969; Diaz et al., 1996b). An atoll is the climax of an oceanic coral reef complex developing on an extinct volcano (Darwin, 1842). The network-like distribution of patch reefs in these lagoons respond to their positioning with respect to the lagoonal terrace, a sand Bank off the back reef, and the water motion exposure (Geister and Díaz, 1996). These Banks have the same fractal patterning formed by corals at Indo-Pacific atolls’ lagoons (Blakeway and Hamblin, 2015), which remarks the singularity of this type of reef formation in Caribbean reefs. This rare coral patch formation composed mostly of Orbicella (instead of Acropora in the Indo-Pacific) species (O. annularis, O. faveolata and O. franksi) with some colonies attaining large sizes (Diaz et al., 1996a; Sánchez et al., 2005; Foster et al., 2013). This habitat includes a different community of benthic organisms and fish compared to the deepest, most exposed external environments of the atolls and barrier reefs of the area (Sánchez et al., 1997a; Díaz-Pulido et al., 2004; Velásquez and Sánchez, 2015; Sánchez, 2016; Gonzalez-Zapata et al., 2018).
Figure 1. Study area. (A) Partial map of the Southwestern Caribbean showing the geographical location of the studied Banks in the San Andrés, Providencia and Santa Catalina Archipelago (Colombia) and details of the study sites: (B) Serrana (2016) and (C) Roncador Banks (2015) (red squares, exact positions in the Supplementary Table 1) (Images from NASA).
The SeaFlower Biosphere Reserve is the largest Marine Protected Area (MPA) in the Caribbean and the second in Latin-America (Guarderas et al., 2008). These include islands and cays, two barrier-reef systems and seven atolls similar to Roncador and Serrana (Diaz et al., 1996a; Sánchez et al., 2005). The geographic zoning of the MPA was the result of an exemplary collaboration between scientists, politicians, and community involvement since 1999 to 2005 (Friedlander et al., 2003; Sánchez et al., 2005; Schrope, 2008; Taylor et al., 2013; Ramirez, 2016). Due to the extension of the MPA, however, the area is rarely explored beyond the larger Islands, San Andrés, Providencia and Santa Catalina (Taylor et al., 2013). Quantitative surveys of corals and other benthic organisms began with scientific expeditions led by the research groups of Invemar funded by Colciencias in 1994–1995 (Diaz et al., 1996b; Sánchez et al., 1997a, 1998; Díaz-Pulido et al., 2004), following by planning of the MPA (Coralina- Gobernación del Archipiélago de San Andrés, Providencia y Santa Catalina-The Ocean Conservancy) between 2000 and 2003 (Friedlander et al., 2003; Sánchez et al., 2005) and recently thanks to a nation-wide interest (SeaFlower Expeditions: Comisión Colombiana del Océano-CCO/Dimar, Coralina, Gobernación del Archipiélago de San Andrés, Providencia y Santa Catalina [Secretaria de Pesca y Agricultura], ColombiaBIO-Colciencias and diverse Colombian universities and ONGs). This research aimed to study trends in coral and other substrate cover between similar sites in the lagoons within Serrana and Roncador Banks, using previously available data from 1995 to 2003 and combining it with data taken during the years 2015 and 2016. In addition, we estimated the FI to assess the effect of nutrient concentration on Serrana (2016) and Roncador (2015) Banks to support recruitment and proliferation of calcifying, photosynthesizing holobionts. These surveys spanned 20 years of change in Caribbean reefs, which provide an unprecedented long-term account of ecosystem trends in some of the most isolated reefs in the region.
Roncador Bank is an elongated atoll of about 13 km in length (NW-SW) and 6.5 km wide. The peripheral windward reef extends without interruption along its entire length (∼11 km), with considerable stretches of its crest emerging at low tides (Figures 1A,B). Serrana Bank is an extended Bank of triangular shape, originated from an annular atoll, which was partially dissected to leeward by subsidence of the sea floor. This Bank measures 15.5 km in the SW-NE direction and 33.4 km in the W-N direction, where a well-developed, 50 km long, peripheral reef encloses a large lagoon basin by the N, E, and S, that connects by the W to the open sea (Figure 1B) (Milliman, 1969; Diaz et al., 1996a; Geister and Díaz, 1996; Díaz et al., 2000; Sánchez et al., 2005; Taylor et al., 2013). We revisited the area during September 2015 (Roncador, 12 sites: Figure 1A) and August 2016 (Serrana, 13 sites: Figure 1B). Details of sampling sites during May 1995 (Díaz-Pulido et al., 2004), April–May 2003 (Sánchez et al., 2005) and 2015/2016 (this study) are included in the Supplementary Table 1. The surveyed sites during 2015/2016 were positioned as close as possible to the previous expeditions using GPS coordinates (see Supplementary Table 1). The sheltered environments in these atolls include the highest relief environments in the lagoon and leeward terrace, with the largest coral cover and colony sizes (e.g., Figure 2).
Figure 2. Map of Roncador Bank environments emphasizing high relief sheltered coral communities (modified from: Diaz et al., 1996a; Sánchez et al., 2005). Inset photo showing a portion of the atoll (courtesy of J. Geister).
Algal and coral (scleractinian) percentage cover, as well as reef rugosity values were included from two previous studies taken in 1995 and 2003 (Díaz-Pulido et al., 2004; Sánchez et al., 2005). We measured reef rugosity as an environmental variable indicator of wave-motion energy and the chronic disturbance effect of waves (Aronson and Precht, 1995). Rugosity, a measurement of bottom topography also known as simple surface roughness index, which acts as a proxy of reef degradation (Bozec et al., 2015), was estimated from the ratio of linear length of a chain that was laid out in a straight line along the bottom following all the vertical relief to its length when stretched out 10 m (Sánchez et al., 1997b). We used a modified band transect technique (Dodge et al., 1982; Etnoyer et al., 2010) to estimate algae and coral cover during 2015 at Roncador and 2016 at Serrana. Equivalent methods for cover estimation were used in 1995 and 2003 surveys (Díaz-Pulido et al., 2004; Sánchez et al., 2005). At each site (See Figures 1A,B and Supplementary Table 1 for details) a 25 m × 1 m band was surveyed using a 50 × 50 cm photoquadrat, which facilitated the quantification of the benthic components. Following the 2003 survey (Sánchez et al., 2005), the 50 × 50 cm quadrat was positioned four times in a square fashion to complete a 1 × 1 m quadrat, that was surveyed at 10 random points, to complete a total of 10 m2 per band. For each site, algal and coral cover were estimated manually by digitizing the areas from the photo quadrats using ImageJ (Schneider et al., 2012; López-Angarita et al., 2014). Benthic cover was categorized as follows, hard coral (HC), crustose coralline algae (CCA), calcareous algae (CA), fleshy algae (FA), cyanobacteria (CB), other invertebrates, sponges, octocorals, rock, rubble and sand (see Supplementary Table 1). Hard corals included scleractinian and other hard corals such as Millepora spp. and Stylaster roseus. Algae genera for CA included Amphiroa, Halimeda, Galaxaura and Penicillus. Algae belonging to genera Turbinaria, Caulerpa, Dyctiota, Lobophora, Mycrodictium and Sargassum composed the FA category. We evaluated cover percentage differences in HC, CCA, CA, FA, CB and rugosity index (RI) per site at a mid-water reefs at Roncador (12–23 m) and shallow reefs at Serrana (2–13 m) across the 3 years of sampling, by obtaining bootstrapped (n = 1000 with replacement) confidence intervals (95% CI) for each mean (see Supplementary Table 3), to smooth confounding effects due to differences in sampling unbalanced sample sizes, repeated measures and seasonal variability. Data for cover percentage comparisons at Roncador mid-water reefs came from 8 stations (12–22 m) in 1995, 14 stations (12–23 m) in 2003 and 13 stations (8–18 m) in 2015. Likewise, data for the above comparisons at Serrana shallow reefs came from 12 stations (3–13 m) in 1995, 36 stations (2–13 m) in 2003 and 13 stations (2–11 m) in 2016.
In situ estimates of octocoral density in the quadrats were made by counting the individual erect colonies within each quadrat and then averaging the density values of the 10 random points for each surveyed station following the same methods as in 2003 (Sánchez and Wirshing, 2005; Sánchez et al., 2005; Etnoyer et al., 2010) (see Supplementary Table 4). Octocoral density changes were assessed by obtaining bootstrapped (n = 1000 with replacement) confidence intervals (95% CI) for each mean value (see Supplementary Table 3) at Roncador between 2003 and 2015, and Serrana between 2003 and 2016.
During the expeditions to Roncador (2015) and Serrana (2016) Banks, samples of superficial sandy sediment were collected at four different sites in each Bank (6–12 m). The samples were stored in plastic containers (20 ml), fixed with absolute ethanol, subsequently dried (>24 h, 60°C) and thoroughly mixed. From each sample, we took a 0.1 g subsample, placed it in a 90 mm-diameter petri dish and examined it using a Leica EZ4 stereoscope. We removed and counted all foraminifera until a minimum of 150 individuals were found in all the samples combined for each station, excluding heavily worn and reworked specimens. The tests were placed onto cardboard micropaleontological faunal slides, counted, identified by genus and sorted into three functional groups: symbiont-bearing, opportunistic and other small heterotrophic taxa; the proportions of each functional groups were then used to calculate the average FI (Hallock et al., 2003). The FI ranges from 1 to 10, where FI < 2 indicates unfavorable conditions for coral growth, 2 < FI < 4 represents the limit for coral growth and unsuitable for recovery and FI > 4 permits coral growth and recovery.
Sea urchin densities, namely of Diadema antillarum and Echinometra viridis, were estimated by counting the number of urchins within each quadrat along the transect, then averaging the density values for each surveyed station following the same methods as in 2003 (Coyer et al., 1993; Sánchez and Wirshing, 2005; Sánchez et al., 2005) (see Supplementary Table 6). We compared Sea urchin density by obtaining bootstrapped (n = 1000 with replacement) confidence intervals (95% CI) for each mean (see Supplementary Table 3) at Roncador between 2003 and 2015, and Serrana between 2003 and 2016.
Roncador Bank mid-water reefs experienced a mean decline in CCA (10.13%) and FA (53.41%), but not HC (22.58%), from 1995 to 2015 (CCA: 2.15%, FA: 28.88%, HC: 9.85% ). Mean CB increased significantly from 1995 (1.73%) to 2003 (13.20%) but decreased in 2015 (3.99%) close to its 1995 value. Mean CA did not change significantly over the three periods surveyed (6.51, 7.89, and 3.99%) at this Bank. Meanwhile, mean CCA in Serrana Bank shallow reefs from 1995 (5.05%) lowered significantly to 0.25% in 2016. During 2003 mean FA (48.53%) and HC (35.73%) reduced significantly as compared to 2003 period (FA: 25.83%, HC: 19.24%) in this Bank, but not when compared to 2016 (FA: 43.24%, HC: 20.88%). Mean CB significantly raised from 1995 (0.58%) to 2003 (9.15%) but fell back to 1.97% in 2016. (Figure 3, see Supplementary Tables 1–3 for complete data sets). Cover by CA fell down from 6.5% in 1995 to 3.9% in 2015 at Roncador mid-water reefs, and from 7.3 to 3.2% at Serrana shallow reefs for the 1995–2016 period. CB reached its highest cover during 2003 at both Banks, 13.2% at Roncador and 9.2% at Serrana, while remaining below 5% at Roncador in 1995/2015 and 2% at Serrana in 1995/2016. CCA diminished from 10.1% in 1995 to 2.2% in 2015 at Roncador, and from 5.1 to 0.3% at Serrana from 1995 to 2016. FA was the highest at Roncador during 1995 with 53.4% but diminished by 24.5% in 2015, with a slight decline of 2.8% from 2003 to 2015. Similarly, HC cover at this Bank decrease from 22.6% in 1995 to 14.9% in 2003, and to 9.9% during 2016; a continuous reduction in HC cover of 12.7% over 20 years. FA at Serrana fluctuated from 48.5% in 1995 down to 25.8% in 2003 and then up to 43.2% in 2016. However, despite a 21.5% HC mean reduction at Serrana shallow reefs from 1995 to 2003, its HC cover did not differ significantly between 1995 and 2016, when reached a mean of 20.9%.
Figure 3. Calcareous algae (CA), cyanobacteria (CB), crustose coralline algae (CCA), fleshy algae (FA) and hard coral (HC) cover (%) means with 95% CI for the data collected for three periods at (A) Roncador (1995–2003–2015) and (B) Serrana (1995–2003–2016) Banks.
Based on the surveys performed in 1995 at Roncador Bank mid-water reefs (Díaz-Pulido et al., 2004), a total of 20.0% HC cover was attributed to Orbicella franksi, Agaricia agaricites, O. annularis, Pseudodiploria strigosa, Colphophyllia natans, Siderastrea siderea, Montastraea cavernosa, Millepora alcicornis and Porites porites. Comparing the datasets from the 2003 surveys taken at Roncador Bank, all previously reported species were present and dominant at mid-water reefs, with the exception of P. astreoides and M. cavernosa, but all species with an overall lower coral cover (15.5%). During 2015, the main coral species were O. annularis, O. faveolata, A. agaricites, P. astreoides and O. franksi. These species remained as the most abundant species despite an apparent decline of HC cover (12.7%) when compared to the 1995 dataset from Roncador Bank. On the other hand, Serrana Bank showed an apparent trend of loss and posterior recovery of HC cover. In 1995 this Bank’s 32% of HC cover was represented by Orbicella spp., A. agaricites, S. siderea, C. natans, Diploria labyrinthiformis and P. strigosa. In 2003, Orbicella spp., A. agaricites, S. siderea along P. astreoides and P. porites, composed 15.5% of HC cover at this Bank. Lastly, in 2016 O. annularis, O. faveolata, S. siderea, P. astreoides and Agaricia spp. colonies composed over 17.0% of HC cover at Serrana shallow reefs, where O. annularis was the main responsible for the increase in mean HC cover, along the appearance of Agaricia spp. colonies in the quadrats.
There was an apparent decrease in rugosity at both Banks when comparing data sets between 1995 and 2003. However, there was not an apparent change in rugosity when comparing the datasets between 2003 and those taken in 2015/2016. (Figure 4). A 52 and 55% reduction of rugosity occurred at Roncador mid-water and Serrana shallow reefs from 1995 to 2015 and 2016, respectively.
Figure 4. Bottom complexity. Rugosity index means with 95% CI for three periods at (A) Roncador and (B) Serrana Banks.
The density in Roncador ranged between 3.5 and 15.1 ind m−2 in 2003 and 11.3–68.5 ind m−2 in 2015, the highest value for Serrana only reached 13.9 ind m−2 in a survey during 2003 (see Suplemmentary Table 4). Although the octocoral densities between Roncador and Serrana showed strong spatial variation, their temporal patterns within surveyed sites are more complex. The highest value for octocoral density reported for 2003 was 15.1 ind m−2, while in 2015 was 68.5 ind m−2, almost 5 times the highest value in 2003. At Roncador Bank, octocoral density increased from 11.2 ind m−2 during 2003 to 36.4 ind m−2 in 2015, a 225% increment. Contrarily, Serrana Bank did not change its octocoral density over a 11-year span, with 1.9 ind m−2 in 2003 to 1.5 ind m−2 in 2016 (Figure 5A). In general, density values for Serrana were lower than Roncador, which included a peak value reaching 13.9 ind m−2 in 2003 and 6.5 ind m−2 in 2016. The sites where octocoral densities increased were located inside the reef lagoon. Serrana had the lowest values with low variation among sites (Figure 5A). Although, we did not survey densities per species, at both Banks Antillogorgia bipinnata was overall the most abundant gorgonian coral followed by the soft coral Briareum asbestinum and plexaurid gorgonians belonging to the genera Plexaura, Pseudoplexaura and Eunicea.
Figure 5. Octocoral and Benthic foraminifera abundance. (A) Octocoral densities (ind m−2) means with 95% CI in Roncador and Serrana during 2003 and 2015/2016. (B) The average FI ± SD for Roncador 2015 and Serrana 2016. Horizontal lines represent critical point for analysis (Hallock et al., 2003)].
We examined a total of 8 sediment samples, counted 1363 foraminifera tests and identified 34 genera at both Banks: 7 symbiont-bearing, 3 opportunistic and 24 other small heterotrophic. The most abundant genera for each functional group were Laevipeneroplis, Bolivina and Triloculina, respectively (See Supplementary Table 5). The small heterotrophic taxa dominated the foraminifera community with 86% of all individuals, followed by 10% of symbiont-bearing and 4% of opportunistic. The average FI found for Roncador was 2.5 and 3 for Serrana (Figure 5B).
Urchins were seldom found at both Serrana and Roncador Banks, sometimes absent in many sites during 2015 and 2016 surveys. Similar densities of the sea urchin D. antillarum were found at Roncador during 2003 (0.01 ind m−2) with respect to 2015 (0.01 ind m−2), while its density was higher in Serrana 2016 (0.15 ind m−2) than in 2003 (0.04 ind m−2). Despite these trends, sea urchin density did not change significantly over those periods (Figures 6A,B). On the contrary, no individual of E. viridis was recorded in Roncador 2015 and Serrana 2016, showing a reduction in its density when compared to 2003 (see Supplementary Table 6).
Figure 6. Sea Urchin abundance. Species mean density (ind m−2) with 95% CI for two periods at (A) Roncador 2003/2005 and (B) Serrana Banks 2003/2016.
Our results demonstrate a significant reduction in CCA and HC percent cover, which included massive (Orbicella spp.) and encrusting/smaller (Agaricia spp., P. astreoides) reef building species, particularly at Roncador Bank mid water-reefs. Likewise, a reduction in benthic complexity (rugosity), was evident between 1995 and 2003 at both Roncador and Serrana atolls. The Banks were different in terms of gorgonian densities, whit a nearly one order of magnitude higher in Roncador and a trend to increase. Sea urchin densities were overall low at both Banks. The FI at both Banks, a proxy of water quality and reef resilience, was below the range expected for coral reefs, which are apparently isolated from human waste water and/or sources of eutrophication (FI > 4). FI values found, between 2 and 4, corresponded to reefs with water conditions from marginal to unsuitable for recovery of coral communities after disturbance (Hallock et al., 2003). This means nutrient availability does not favor the recruitment and growth of mixotrophic and calcifying organisms like hard corals (Hallock, 2012), compromising reef health in the long term. Those values are common at fringing reefs along the continental coast of Colombia affected by constant runoff and nutrient input from pollution (Velásquez et al., 2011; López-Angarita et al., 2014). Although both Banks show a decrease in CA and CCA, Roncador Bank mid-water reefs additionally face an alarming decline in overall coral cover. The correspondence of declining CCA, FA and general coral cover, water quality and bottom complexity (rugosity) with increases in octocoral is even more alarming in an already declining reef system.
Although remote reefs represent an exemplary case to assess reef health, due to its isolation from direct human disturbances, our results evidenced a clear decline in CCA an overall HC cover from 1995 to 2015 at Roncador mid-water reefs. During the year 2003, a mass mortality of hard corals was also observed in the lagoon of Serrana Bank (Sánchez et al., 2010), whereas coral in Roncador appeared healthier that year (Sánchez et al., 2005). Rugosity for both Roncador and Serrana Banks decreased dramatically between 1995 and 2003. The 1997–1998 El Niño Southern Oscillation led to mass coral bleaching and subsequently coral mortalities as well as the appearance of numerous diseases affecting corals (Weil, 2004), which most likely also impacted the two Banks. In terms of direct external effects, these reefs face the Caribbean current, NW direction, and at least part of the year receive diluted waters influenced by fresh-water runoff from the continental coast (Beier et al., 2017). Coastal waters coming from the Colombian coast are increasingly polluted with nutrients coming from rivers, which promotes coral degradation and harmful algae blooms (Restrepo et al., 2006; Coronado-Franco et al., 2018). Moreover, direct impacts from continental eutrophic waters on these Banks are likely. Complex seasonal water circulation around the archipelago (Garay et al., 1987) can also transport nutrients from the nearby island of San Andrés, where overpopulation and eutrophication is on the rise (Gavio et al., 2010; Mancera-Pineda et al., 2014). In addition, regional currents may be introducing alien objects or drifters, which can contribute as a source or external organic and inorganic material into San Andres (Mancera-Pineda et al., 2014), The case in point of drifters demonstrates that external threats can have detrimental impacts to isolated reef sites such as Serrana and Roncador.
The models of coral degradation usually consider a shift from coral to algae dominance in degrading coral reefs (Mumby, 2009; Graham et al., 2013; Slattery and Lesser, 2014), a signal not clearly evidenced in our study area. It is important to mention that detailed observations on Serrana and Roncador reefs, made over 50 years ago (1966), describe these reef communities as both coral and CCA-dominated, which included abundant stands of species today endangered like Acropora cervicornis and A. palmata, and only mentioning a reduction on fish abundances in comparison to observation from 1945 (Milliman, 1969). Coral and CCA percent cover decreased at Roncador mid-water reefs whereas only CCA decreased at Serrana shallow reefs which kept CCA, FA and HC close to their values in 1995. A pattern unexpected in an area devoid of direct human influence, which follow the world-wide decline of marine wilderness (Jones et al., 2018) and coral reef degradation (Bruno and Valdivia, 2016). The rise of slimy cyanobacterial mats, as those observed in these Caribbean reefs, comprise another phase shift related to increases in both eutrophication and water temperature during the last 40 years (De Bakker et al., 2017). In addition, large predator fishes, such as groupers, have been depleted by unsustainable fisheries throughout the entire SeaFlower reserve (Prada et al., 2007), with Roncador bank exhibiting the lowest abundance values in the archipelago (Hooker et al., 2011). Overall, corals reefs are experimenting a more complex degradation scenario than previously thought, where even far-flung reefs seem to be unable to cope with large-scale stressor such as global warming/sea level rise and ocean acidification (Perry et al., 2013, 2018). This situation could be acting in synergy with the tectonic activity leading to subsidence in the Caribbean (Khan et al., 2017), which could be different between Serrana and Rocandor Banks. In fact, Roncador Bank has been predicted to be more vulnerable to sea-level rise impacts than Serrana (Andrade et al., 2011). In addition, the small size of Roncador Bank, together with strong water motion, coral degradation and eutrophication, makes it prone to erosion and sediment loss, which suggest a similar reef demise as the case of Serranilla Bank (Triffleman et al., 1992). An interpretation of coral reefs declining, in both coral and algae whereas octocorals thrive, needs a more complex scenario with multiple stressors, where ocean acidification, sea-level and reef erosion should be taken into account (Pendleton et al., 2016).
A strong differentiation between octocoral densities from Roncador and Serrana Banks, on a spatial and temporal scale, was clearly found. Overall, density values in Roncador mid-water reefs were almost five times higher than those in Serrana shallow reefs, reaching up to 68.5 ind m−2 whereas the highest in the later was 13.9 ind m−2 (see Supplementary Table 3). These values are consistent with previous studies reporting similar ranges (2.95–20.6 ind m−2) for octocoral densities recently estimated in the Caribbean (Privitera-Johnson et al., 2015), except for station 17 in Roncador that showed unusually higher density than those previously reported (68.5 ind m−2). Interestingly, Roncador mid-water reefs showed higher mean octocoral densities (see Supplementary Table 4) than those reported in many Caribbean regions (Etnoyer et al., 2010; Sánchez, 2016) and those (9 ind m−2) reported for SE Sulawesi assemblages (Rowley, 2018). It is widely known that habitat complexity significantly affects the assembly of benthic communities (Sánchez et al., 1997a) and in this study we see a dramatic decrease in habitat complexity through the measured rugosity index. Since these indexes reflect the growth dynamics of hard corals and reef erosion (Bozec et al., 2015), we can conclude that the percent cover of scleractinian community has been receding in the last years, whereas changes in octocoral densities tend to increase. This is a striking pattern because if hard coral cover continues to decrease and octocorals increase, or at least remain constant; it means that their relative abundance in the community increases as well, and such trend could dominate the community in a hypothetical phase shift scenario altering the functional and trophic structure of the reef community (Norström et al., 2009; Lenz et al., 2015). These growing gorgonian assemblages can also change in composition due to the relative abundance of associate predators (e.g., Cyphoma) or species susceptibility to diseases (Sánchez, 2016).
In the last two decades Caribbean reefs have experienced thermal anomalies of up to 16 weeks as during 2005 and 2010, including coral bleaching throughout the province and scleractinian coral mortality, coinciding with the record of hurricanes, with four category 5 hurricanes, including Katrina (Wilkinson and Souter, 2008; Eakin et al., 2010). However, gorgonian corals (Octocorallia), one of the features of the Caribbean reef province, exhibited negligible mortality and high resilience after bleaching (Lasker and Sánchez, 2002; Lasker, 2003; Prada et al., 2010). Octocorals have also shown to be very resistant to ocean acidification in experimental settings (Enochs et al., 2016), even below saturation of Ωcalcite (Gómez et al., 2015). Other octocorals, such as the soft coral species of the Red Sea, have also shown resistance to high pCO2 values due to the protective properties of their tissues (Gabay et al., 2013, 2014). In short, octocorals, due to their resistance to bleaching and acidification, together with a heterothrophic feeding strategy in most Caribbean species (Baker et al., 2015; Sánchez et al., 2019), should be included in the list of climate change/human footprint survivors. Yet, these organisms have been rarely considered as reef-building corals, although a large colony can contribute as much calcium carbonate, at its holdfast, as a small scleractinian coral species (Kocurko, 1987). Octocoral-dominated reefs already exists at naturally acidified waters (Inoue et al., 2013). Should we consider an octocoral-algae phase shift in Caribbean reefs?
Although both Banks followed a loss in coral cover, topographic complexity (rugosity) and resilience (FI index), Roncador Bank mid-water reefs face a steady decline. These unexpected findings led us to consider the scenario of some of these reefs starting to lose complexity, whereas providing ideal conditions for octocoral recruitment, settlement, successful establishment and subsequent growth. Other SeaFlower reef complexes, like San Andrés Island, have already shown the effects of coastal erosion and eutrophication (Gavio et al., 2010). Situations predicted to increase with the human footprint and under a climate change scenario (Schönberg et al., 2017), hence bioerosion and reef budgets are rarely considered in monitoring programs (Perry et al., 2013). Serrana Bank shallow reefs, a larger reef-complex with signs of HC recovery, provides some hope in terms of its withstanding HC cover, yet a more detailed monitoring is needed.
Another concern is the apparent eutrophication of the Banks as seen in the coral/algae abundances and FORAM index. Due to lack of information, foreign sources of nutrients should not be ignored and deserve further study. Yet, a more likely scenario could be related with the cycle of dissolved organic carbon (DOC), fleshy algae and the microbial community (Pawlik et al., 2016), which maintains the algal competitive abundance and promotes coral degradation (Haas et al., 2016). Coral diseases are prevalent in the two Banks and mass mortalities have been observed in Serrana Bank (Sánchez et al., 2005, 2010). Monitoring the sources of nutrients in these Banks, along with the other components of reef resilience (i.e., herbivorous fish), can bring important information for, urgently needed, adaptive management in these deteriorating coral reefs.
The implications of high octocoral densities in Roncador are of the utmost importance for understanding the future of Caribbean reefs. First it is important to note that Roncador mid-water reefs, and even Serrana shallow reefs, show significantly lower densities of sponges as compared to continental reefs (Zea, 2001), where increased suspended particles promote sponge abundance and dominance (Valderrama and Zea, 2003; Loh et al., 2015). Novel community assemblages may include new trophic and habitat provisioning opportunities (Lugo et al., 2000) and could even transform the storm resistance regimes (Lenz et al., 2015). To fully understand the nature of octocoral densities, as well as how environmental conditions change by high octocoral abundances, it is paramount to set a baseline of recruitment survivorship and dynamics in future monitoring programs. Specifically, future studies in the area should address the hypothesis of the stock-recruitment within the octocoral canopy (Privitera-Johnson et al., 2015), which suggests that once larvae leave the parental colonies, they will get caught up beneath the canopy of the octocoral community promoting settlement and eventually densities of adult colonies.
Caribbean atolls, such as Roncador and Serrana Banks, developed remarkable areas of high relief coral communities in their lagoons and terraces (e.g., Figure 2), but today live coral cover averages less than 10% at Roncador mid-water reefs, when in 1995 was 22.6%, and less than 21% at Serrana shallow reefs, when in 1995 was 35.4%. These Banks, isolated from human populations and rivers, comprised the climax of thousands of years of a reef-building process, which is disappearing in the last few decades in the absence of a clear causal explanation. The fate of these and many coral reefs in the Caribbean are beyond alarming. The need of more sophisticated means of reef monitoring, including reef budgets and nutrients (i.e., carbon sources), is urgently needed toward adaptive management of Caribbean MPAs.
JS conceived the study. FG-Z, LG-C, and MGn collected the samples. FG-Z, LG-C, NP, PR, LN, MGn, DV, and AS processed the imagery. MG-C analyzed the samples. MG-C, PR, LG-C, and DV performed the data analyses. JS and MG-C wrote the manuscript with contributions from LG-C and DV. All authors edited the manuscript before submission.
This work was supported by the ColombiaBIO-Colciencias (Convenio 341-2016, Universidad de los Andes- Comisión Colombiana del Océano-CCO) and Corporación para el Desarrollo Sostenible del Departamento Archipiélago de San Andrés Providencia y Santa Catalina, CORALINA-Universidad de los Andes (Convenios No. 13, 2014 and No. 21, 2015). Additional Funding sources included COLCIENCIAS (Grant No. 120465944147) and Vicerrectoría de Investigaciones, Programas de Investigación (Completando la teoría del origen de las especies de Darwin: especiación ecológica en octocorales, desde niños a doctores) (UniAndes).
The authors declare that the research was conducted in the absence of any commercial or financial relationships that could be construed as a potential conflict of interest.
We greatly acknowledge the SeaFlower Expeditions (2015–2016) organizers and sponsors: Comisión Colombiana del Océano-CCO/Dimar (J. Soltau, J. Sintura, A. Chadid, R. Carranza and their team), Colombian Navy (ARC Providencia and crew, Serrana Expedition), Coralina (N. Bolaños, Erik Castro and their team), Secretaria de Pesca y Agricultura (Gobernación del departamento de San Andrés, Providencia y Santa Catalina), ColombiaBIO-Colciencias (F. García, L. Ayala and team). Centro de Servicios Compartidos, Universidad de los Andes, efficiently managed resources for the Serrana expedition. We express great appreciation to T. González and A. Iñiguez for contributing in the field and/or processing digital imagery and estimating coral cover. Members of BIOMMAR, and O. Ruíz and J. Andrade who helped with logistics. Comments and criticism from three reviewers greatly improved the corrected manuscript.
The Supplementary Material for this article can be found online at: https://www.frontiersin.org/articles/10.3389/fmars.2019.00073/full#supplementary-material
Albins, M. A., and Hixon, M. A. (2013). Worst case scenario: potential long-term effects of invasive predatory lionfish (Pterois volitans) on atlantic and caribbean coral-reef communities. Environ. Biol. Fishes 96, 1151–1157. doi: 10.1007/s10641-011-9795-1
Andrade, C. A., Pinzón, V. M., and Manzanillo, I. (2011). Effects of sea-level rise due to climatic changes on the beaches of the Cays of the San Andres Archipelago. Sci. Bull. 29, 62–74.
Appeldoorn, R., Ballantine, D., Bejarano, I., Carlo, M., Nemeth, M., Otero, E., et al. (2016). Mesophotic coral ecosystems under anthropogenic stress: a case study at Ponce, Puerto Rico. Coral Reefs 35, 63–75. doi: 10.1007/s00338-015-1360-5
Aronson, R. B., and Precht, W. F. (1995). Landscape patterns of reef coral diversity: a test of the intermediate disturbance hypothesis. J. Exp. Mar. Biol. Ecol. 192, 1–14. doi: 10.1016/0022-0981(95)00052-S
Baker, D. M., Freeman, C. J., Knowlton, N., Thacker, R. W., Kim, K., and Fogel, M. L. (2015). Productivity links morphology, symbiont specificity and bleaching in the evolution of Caribbean octocoral symbioses. ISME J. 9, 2620–2629. doi: 10.1038/ismej.2015.71
Beier, E., Bernal, G., Ruiz-Ochoa, M., and Barton, E. D. (2017). Freshwater exchanges and surface salinity in the Colombian basin, Caribbean Sea. PLoS One 12:e0182116. doi: 10.1371/journal.pone.0182116
Blakeway, D., and Hamblin, M. G. (2015). Self-generated morphology in lagoon reefs. PeerJ 3:e935. doi: 10.7717/peerj.935
Bozec, Y.-M., Alvarez-Filip, L., and Mumby, P. J. (2015). The dynamics of architectural complexity on coral reefs under climate change. Glob. Change Biol. 21, 223–235. doi: 10.1111/gcb.12698
Bruno, J. F., and Valdivia, A. (2016). Coral reef degradation is not correlated with local human population density. Sci. Rep. 6:29778. doi: 10.1038/srep29778
Camp, E. F., Schoepf, V., Mumby, P. J., Hardtke, L. A., Rodolfo-Metalpa, R., Smith, D. J., et al. (2018). The future of coral reefs subject to rapid climate change: lessons from natural extreme environments. Front. Mar. Sci. 5:4. doi: 10.3389/fmars.2018.00433
Cockey, E., Hallock, P., and Lidz, B. H. (1996). Decadal-scale changes in benthic foraminiferal assemblages off Key Largo, Florida. Coral Reefs 15, 237–248. doi: 10.1007/BF01787458
Cooper, T. F., Gilmour, J. P., and Fabricius, K. E. (2009). Bioindicators of changes in water quality on coral reefs: review and recommendations for monitoring programmes. Coral Reefs 28, 589–606. doi: 10.1007/s00338-009-0512-x
Coronado-Franco, K. V., Selvaraj, J. J., and Mancera Pineda, J. E. (2018). Algal blooms detection in Colombian Caribbean Sea using MODIS imagery. Mar. Pollut. Bull. 133, 791–798. doi: 10.1016/j.marpolbul.2018.06.021
Costanza, R., d’Arge, R., de Groot, R., Farber, S., Grasso, M., Hannon, B., et al. (1997). The value of the world’s ecosystem services and natural capital. Nature 387, 253–260. doi: 10.1038/387253a0
Coyer, J. A., Ambrose, R. F., Engle, J. M., and Carroll, J. C. (1993). Interactions between corals and algae on a temperate zone rocky reef: mediation by sea urchins. J. Exp. Mar. Biol. Ecol. 167, 21–37. doi: 10.1016/0022-0981(93)90181-M
Darwin, C. (1842). The Structure and Distribution of Coral Reefs. Berkeley, CA: University of California Press, 214.
De Bakker, D. M., Van Duyl, F. C., Bak, R. P., Nugues, M. M., Nieuwland, G., and Meesters, E. H. (2017). 40 Years of benthic community change on the Caribbean reefs of Curaçao and Bonaire: the rise of slimy cyanobacterial mats. Coral Reefs 36, 355–367. doi: 10.1007/s00338-016-1534-9
De Goeij, J. M., Van Oevelen, D., Vermeij, M. J., Osinga, R., Middelburg, J. J., de Goeij, A. F., et al. (2013). Surviving in a marine desert: the sponge loop retains resources within coral reefs. Science 342, 108–110. doi: 10.1126/science.1241981
Díaz, J. M., Barrios, L. M., Cendales, M. H., Garzón-Ferreira, J., Geister, J., Parra-Velandia, F., et al. (2000). Áreas coralinas de Colombia. Invemar.
Diaz, J. M., Diaz, G., Garzon-Ferreira, J., Geister, J., Sánchez, J. A., and Zea, S. (1996a). Atlas de los Arrecifes Coralinos del Caribe colombiano. I. Archipiélago de San Andrés y Providencia. Santa Mart: INVEMAR.
Diaz, J. M., Sánchez, J. A., Garzon-Ferreira, J., and Zea, S. (1996b). Morphology and marine habitats from two southwestern Caribbean atolls: albuquerque and Courtown. Atoll Res. Bull. 435, 1–33.
Díaz-Pulido, G., Sánchez, J. A., Zea, S., Díaz, J. M., et al. (2004). Esquemas de distribución espacial en la comunidad bentónica de arrecifes coralinos continentales y oceánicos del Caribe colombiano. Rev. Acad. Colomb. Cienc. Exactas 108, 337–347.
Dodge, R. E., Logan, A., and Antonius, A. (1982). Quantitative reef assessment studies in Bermuda: a comparison of methods and preliminary results. Bull. Mar. Sci. 32, 745–760.
Dornelas, M., Gotelli, N. J., McGill, B., Shimadzu, H., Moyes, F., Sievers, C., et al. (2014). Assemblage time series reveal biodiversity change but not systematic loss. Science 344, 296–299. doi: 10.1126/science.1248484
Eakin, C. M., Morgan, J. A., Heron, S. F., Smith, T. B., Liu, G., Alvarez-Filip, L., et al. (2010). Caribbean corals in crisis: record thermal stress, bleaching, and mortality in 2005. PLoS One 5:e13969. doi: 10.1371/journal.pone.0013969
Enochs, I. C., Manzello, D. P., Wirshing, H. H., Carlton, R., and Serafy, J. (2016). Micro-CT analysis of the Caribbean octocoral Eunicea flexuosa subjected to elevated p CO 2. ICES J. Mar. Sci. J. Cons. 73, 910–919. doi: 10.1093/icesjms/fsv159
Etnoyer, P., Wirshing, H., and Sánchez, J. A. (2010). Rapid assessment of octocoral diversity and habitat on Saba Bank, Netherlands antilles. PLoS One 5:e10668. doi: 10.1371/journal.pone.0010668
Fabricius, K. E. (2011). “Factors determining the resilience of coral reefs to eutrophication: a review and conceptual model,” in Coral Reefs: An Ecosystem in Transition, eds Z. Dubinsky and N. Stambler (Berlin: Springer), 493–505.
Foster, N. L., Baums, I. B., Sánchez, J. A., Paris, C. B., Chollett, I., Agudelo, C. L., et al. (2013). Hurricane-driven patterns of clonality in an ecosystem engineer: the Caribbean coral Montastraea annularis. PLoS One 8:e53283. doi: 10.1371/journal.pone.0053283
Friedlander, A., Sladek-Nowlis, J., Sánchez, J. A., Appeldoorn, R., Usseglio, P., Mccormick, C., et al. (2003). Designing effective marine protected areas in old providence and santa catalina islands, san andrés archipelago, colombia, using biological and sociological information. Conserv. Biol. 17, 1769–1784. doi: 10.1111/j.1523-1739.2003.00338.x
Gabay, Y., Benayahu, Y., and Fine, M. (2013). Does elevated p CO2 affect reef octocorals? Ecol. Evol. 3, 465–473. doi: 10.1002/ece3.351
Gabay, Y., Fine, M., Barkay, Z., and Benayahu, Y. (2014). Octocoral tissue provides protection from declining oceanic pH. PLoS One 9:e91553. doi: 10.1371/journal.pone.0091553
Garay, J., Francisco, C., Carlos, A., and Jairo, A. (1987). Estudio oceanográfico del área insular y oceánica del Caribe colombiano de San Andrés y Providencia y cayos vecinos. CIOH Sci. Bull. 9:33.
Gardner, T. A., Cote, I. M., Gill, J. A., Grant, A., and Watkinson, A. R. (2003). Long-term region-wide declines in Caribbean corals. Science 301, 958–961. doi: 10.1126/science.1086050
Gavio, B., Palmer-Cantillo, S., and Mancera, J. E. (2010). Historical analysis (2000–2005) of the coastal water quality in San Andrés Island, sea flower biosphere reserve, Caribbean Colombia. Mar. Pollut. Bull. 60, 1018–1030. doi: 10.1016/j.marpolbul.2010.01.025
Geister, J., and Díaz, J. M. (1996). “A field guide to the atolls and reefs of San Andres and Providencia (Colombia),” in Proceedings of the 8th International Coral Reef Symposium, Panama City, 235–236.
Gómez, C. E., Paul, V. J., Ritson-Williams, R., Muehllehner, N., Langdon, C., and Sánchez, J. A. (2015). Responses of the tropical gorgonian coral Eunicea fusca to ocean acidification conditions. Coral Reefs 34, 451–460. doi: 10.1007/s00338-014-1241-3
Gonzalez-Zapata, F. L., Bongaerts, P., Ramírez-Portilla, C., Adu-Oppong, B., Walljasper, G., Reyes, A., et al. (2018). Holobiont diversity in a reef-building coral over its entire depth range in the mesophotic zone. Front. Mar. Sci. 5:29. doi: 10.3389/fmars.2018.00029
Graham, N. A., Bellwood, D. R., Cinner, J. E., Hughes, T. P., Norström, A. V., and Nyström, M. (2013). Managing resilience to reverse phase shifts in coral reefs. Front. Ecol. Environ. 11, 541–548. doi: 10.1890/120305
Guarderas, A. P., Hacker, S. D., and Lubchenco, J. (2008). Current status of marine protected areas in latin america and the caribbean. Conserv. Biol. 22, 1630–1640. doi: 10.1111/j.1523-1739.2008.01023.x
Haas, A. F., Fairoz, M. F. M., Kelly, L. W., Nelson, C. E., Dinsdale, E. A., Edwards, R. A., et al. (2016). Global microbialization of coral reefs. Nat. Microbiol. 1:16042. doi: 10.1038/nmicrobiol.2016.42
Hallock, P. (1999). “Symbiont-bearing Foraminifera,” in Modern Foraminifera, ed. B. K. S. Gupta (Dordrecht: Springer), 123–139.
Hallock, P. (2012). “The FoRAM index revisited: uses, challenges, and limitations,” in Proceedings of the 12th International Coral Reef Symposium, Cairns.
Hallock, P., Lidz, B. H., Cockey-Burkhard, E. M., and Donnelly, K. B. (2003). “Foraminifera as bioindicators in coral reef assessment and monitoring: the FORAM index,” in Coastal Monitoring through Partnerships, eds B. D. Melzian, V. Engle, M. McAlister, and S. S. Sandhu (Berlin: Springer), 221–238.
Hoegh-Guldberg, O., Mumby, P. J., Hooten, A. J., Steneck, R. S., Greenfield, P., Gomez, E., et al. (2007). Coral reefs under rapid climate change and ocean acidification. Science 318, 1737–1742. doi: 10.1126/science.1152509
Hoegh-Guldberg, O., Poloczanska, E. S., Skirving, W., and Dove, S. (2017). Coral reef ecosystems under climate change and ocean acidification. Front. Mar. Sci. 4:158. doi: 10.3389/fmars.2017.00158
Hooker, H., Santos-Martinez, A., and Taylor, E. (2011). “Abundancias de grandes serranidos en la reserva de biosfera seaflower,” in Proceedings of the 64th Gulf and Caribbean Fisheries Institute, Puerto Morelos, 237–240.
Hooper, D. U., Adair, E. C., Cardinale, B. J., Byrnes, J. E., Hungate, B. A., Matulich, K. L., et al. (2012). A global synthesis reveals biodiversity loss as a major driver of ecosystem change. Nature 486, 105–108. doi: 10.1038/nature11118
Hughes, T. P. (1994). Catastrophes, phase shifts, and large-scale degradation of a caribbean coral reef. Science 265, 1547–1551. doi: 10.1126/science.265.5178.1547
Hughes, T. P., Baird, A. H., Bellwood, D. R., Card, M., Connolly, S. R., Folke, C., et al. (2003). Climate change, human impacts, and the resilience of coral reefs. Science 301, 929–933. doi: 10.1126/science.1085046
Hughes, T. P., Graham, N. A. J., Jackson, J. B. C., Mumby, P. J., and Steneck, R. S. (2010). Rising to the challenge of sustaining coral reef resilience. Trends Ecol. Evol. 25, 633–642. doi: 10.1016/j.tree.2010.07.011
Inoue, S., Kayanne, H., Yamamoto, S., and Kurihara, H. (2013). Spatial community shift from hard to soft corals in acidified water. Nat. Clim. Change 3, 683–687. doi: 10.1038/nclimate1855
Jackson, J. B. C., Donovan, M. K., Cramer, K. L., and Lam, V. V. (2014). Status and Trends of Caribbean Coral Reefs. Washington, D.C: Global Coral Reef Monitoring Network.
Jackson, J. B. C., Kirby, M. X., Berger, W. H., Bjorndal, K. A., Botsford, L. W., Bourque, B. J., et al. (2001). Historical overfishing and the recent collapse of coastal ecosystems. Science 293, 629–638. doi: 10.1126/science.1059199
Jones, K. R., Klein, C. J., Halpern, B. S., Venter, O., Grantham, H., Kuempel, C. D., et al. (2018). The location and protection status of earth’s diminishing marine wilderness. Curr. Biol. 28, 2506.e3–2512.e3. doi: 10.1016/j.cub.2018.06.010
Khan, N. S., Ashe, E., Horton, B. P., Dutton, A., Kopp, R. E., Brocard, G., et al. (2017). Drivers of holocene sea-level change in the caribbean. Quat. Sci. Rev. 155, 13–36. doi: 10.1016/j.quascirev.2016.08.032
Klaus, J. S., Janse, I., Heikoop, J. M., Sanford, R. A., and Fouke, B. W. (2007). Coral microbial communities, zooxanthellae and mucus along gradients of seawater depth and coastal pollution. Environ. Microbiol. 9, 1291–1305. doi: 10.1111/j.1462-2920.2007.01249.x
Kocurko, M. J. (1987). Shalow-water octocorallia and related submarine lithification, San Andres island, Colombia, Tex. J. Sci. 39, 349–365.
Lasker, H. R. (2003). Zooxanthella densities within a Caribbean octocoral during bleaching and non-bleaching years. Coral Reefs 22, 23–26.
Lasker, H. R., and Sánchez, J. A. (2002). “Allometry and astogeny of modular organisms,” in Reproductive Biology of Invertebrates Progress in Asexual Reproduction, ed. R. N. Hughes (New York, NY: John Wiley), 207–253.
Ledlie, M. H., Graham, N. A. J., Bythell, J. C., Wilson, S. K., Jennings, S., Polunin, N. V. C., et al. (2007). Phase shifts and the role of herbivory in the resilience of coral reefs. Coral Reefs 26, 641–653. doi: 10.1007/s00338-007-0230-1
Lenz, E. A., Bramanti, L., Lasker, H. R., and Edmunds, P. J. (2015). Long-term variation of octocoral populations in St. John, US Virgin Islands. Coral Reefs 34, 1099–1109. doi: 10.1007/s00338-015-1315-x
Lesser, M. P., and Slattery, M. (2011). Phase shift to algal dominated communities at mesophotic depths associated with lionfish (Pterois volitans) invasion on a Bahamian coral reef. Biol. Invasions 13, 1855–1868. doi: 10.1007/s10530-011-0005-z
Lessios, H. A., Robertson, D. R., and Cubit, J. D. (1984). Spread of diadema mass mortality through the caribbean. Science 226, 335–337. doi: 10.1126/science.226.4672.335
Littler, M. M., and Littler, D. S. (2007). Assessment of coral reefs using herbivory/nutrient assays and indicator groups of benthic primary producers: a critical synthesis, proposed protocols, and critique of management strategies. Aquat. Conserv. Mar. Freshw. Ecosyst. 17, 195–215. doi: 10.1002/aqc.790
Littler, M. M., Littler, D. S., and Brooks, B. L. (2006). Harmful algae on tropical coral reefs: bottom-up eutrophication and top-down herbivory. Harmful Algae 5, 565–585. doi: 10.1016/j.hal.2005.11.003
Loh, T.-L., McMurray, S. E., Henkel, T. P., Vicente, J., and Pawlik, J. R. (2015). Indirect effects of overfishing on Caribbean reefs: sponges overgrow reef-building corals. PeerJ 3:e901. doi: 10.7717/peerj.901
López-Angarita, J., Moreno-Sánchez, R., Maldonado, J. H., and Sánchez, J. A. (2014). Evaluating linked social-ecological systems in marine protected areas. Conserv. Lett. 7, 241–252. doi: 10.1111/conl.12063
Lugo, A. E., Rogers, C. S., and Nixon, S. W. (2000). Hurricanes, coral reefs and rainforests: resistance, ruin and recovery in the Caribbean. Ambio 29, 106–115. doi: 10.1579/0044-7447-29.2.106
Mancera-Pineda, J. E., Montalvo-Talaigua, M., and Gavio, B. (2014). Potentially toxic dinoflagellates associated to drift in san andres Island, international biosphere reservation – Seaflower. Caldasia 36, 139–156. doi: 10.15446/caldasia.v36n1.43896
McMurray, S. E., Stubler, A. D., Erwin, P. M., Finelli, C. M., and Pawlik, J. R. (2018). A test of the sponge-loop hypothesis for emergent Caribbean reef sponges. Mar. Ecol. Prog. Ser. 588, 1–14. doi: 10.3354/meps12466
Milliman, J. D. (1969). Four southwerstern caribbean atolls: courtown cays, alburquerque cays, Roncador Bank and Serrana Bank. Atoll Res. Bull. 129, 1–41. doi: 10.5479/si.00775630.129.1
Montilla, L. M., Ramos, R., García, E., and Cróquer, A. (2016). Caribbean yellow band disease compromises the activity of catalase and glutathione S-transferase in the reef-building coral Orbicella faveolata exposed to anthracene. Dis. Aquat. Organ. 119, 153–161. doi: 10.3354/dao02980
Mora, C. (2008). A clear human footprint in the coral reefs of the Caribbean. Proc. R. Soc. Lond. B Biol. Sci. 275, 767–773. doi: 10.1098/rspb.2007.1472
Mumby, P. J. (2009). Phase shifts and the stability of macroalgal communities on Caribbean coral reefs. Coral Reefs 28, 761–773. doi: 10.1007/s00338-009-0506-8
Mumby, P. J., Steneck, R. S., Edwards, A. J., Ferrari, R., Coleman, R., Harborne, A. R., et al. (2012). Fishing down a Caribbean food web relaxes trophic cascades. Mar. Ecol. Prog. Ser. 445, 13–24. doi: 10.3354/meps09450
Norström, A., Nyström, M., Lokrantz, J., and Folke, C. (2009). Alternative states on coral reefs: beyond coral-macroalgal phase shifts. Mar. Ecol. Prog. Ser. 376, 295–306. doi: 10.3354/meps07815
Orr, J. C., Fabry, V. J., Aumont, O., Bopp, L., Doney, S. C., Feely, R. A., et al. (2005). Anthropogenic ocean acidification over the twenty-first century and its impact on calcifying organisms. Nature 437, 681–686. doi: 10.1038/nature04095
Paddack, M. J., Reynolds, J. D., Aguilar, C., Appeldoorn, R. S., Beets, J., Burkett, E. W., et al. (2009). Recent region-wide declines in Caribbean reef fish abundance. Curr. Biol. 19, 590–595. doi: 10.1016/j.cub.2009.02.041
Pandolfi, J. M., and Lovelock, C. E. (2014). Novelty trumps loss in global biodiversity. Science 344, 266–267. doi: 10.1126/science.1252963
Pawlik, J. R., Burkepile, D. E., and Thurber, R. V. (2016). A vicious circle? Altered carbon and nutrient cycling may explain the low resilience of caribbean coral reefs. BioScience 66, 470–476. doi: 10.1093/biosci/biw047
Pendleton, L. H., Hoegh-Guldberg, O., Langdon, C., and Comte, A. (2016). Multiple stressors and ecological complexity require a new approach to coral reef research. Front. Mar. Sci. 3:36. doi: 10.3389/fmars.2016.00036
Perry, C. T., Alvarez-Filip, L., Graham, N. A. J., Mumby, P. J., Wilson, S. K., Kench, P. S., et al. (2018). Loss of coral reef growth capacity to track future increases in sea level. Nature 558, 396–400. doi: 10.1038/s41586-018-0194-z
Perry, C. T., Murphy, G. N., Kench, P. S., Smithers, S. G., Edinger, E. N., Steneck, R. S., et al. (2013). Caribbean-wide decline in carbonate production threatens coral reef growth. Nat. Commun. 4:1402. doi: 10.1038/ncomms2409
Prada, C., Weil, E., and Yoshioka, P. M. (2010). Octocoral bleaching during unusual thermal stress. Coral Reefs 29, 41–45. doi: 10.1007/s00338-009-0547-z
Prada, M., Castro, E., Puello, E., Pomare, M., Penaloza, G., James, L., et al. (2007). Threats to the grouper population due to fishing during reproductive seasons in the san andres and providencia archipelago, Colombia. Proc. Gulf Caribbean Fish. Inst. 58, 270–275.
Privitera-Johnson, K., Lenz, E. A., and Edmunds, P. J. (2015). Density-associated recruitment in octocoral communities in St. John, US Virgin Islands. J. Exp. Mar. Biol. Ecol. 473, 103–109. doi: 10.1016/j.jembe.2015.08.006
Ramirez, L. F. (2016). Marine protected areas in Colombia: advances in conservation and barriers for effective governance. Ocean Coast. Manage. 125, 49–62. doi: 10.1016/j.ocecoaman.2016.03.005
Restrepo, J. D., Zapata, P., Díaz, J. M., Garzón-Ferreira, J., and García, C. B. (2006). Fluvial fluxes into the Caribbean Sea and their impact on coastal ecosystems: the magdalena river, Colombia. Glob. Planet. Change 50, 33–49. doi: 10.1016/j.gloplacha.2005.09.002
Roberts, C. M., McClean, C. J., Veron, J. E., Hawkins, J. P., Allen, G. R., McAllister, D. E., et al. (2002). Marine biodiversity hotspots and conservation priorities for tropical reefs. Science 295, 1280–1284. doi: 10.1126/science.1067728
Rowley, S. J. (2018). Environmental gradients structure gorgonian assemblages on coral reefs in SE Sulawesi, Indonesia. Coral Reefs 37, 609–630. doi: 10.1007/s00338-018-1685-y
Sánchez, J. A. (2016). “Diversity and evolution of octocoral animal forests at both sides of tropical america,” in Marine Animal Forests, eds S. Rossi, L. Bramanti, A. Gori, and C. Orejas Saco del Valle (Cham: Springer International Publishing), 1–33.
Sánchez, J. A., Díaz, J. M., and Zea, S. (1997a). Gorgonian communities in two contrasting environments on oceanic atolls of the southwestern Caribbean. Bull. Mar. Sci. 61, 453–465.
Sánchez, J. A., Zea, S., and Diaz, J. M. (1997b). Gorgonian communities of two contrasting environments from oceanic Caribbean atolls. Bull. Mar. Sci. 61, 61–72.
Sánchez, J. A., Dueñas, L. F., Rowley, S. J., González, F. L., Vergara, D. C., Montaño-Salazar, S. M., et al. (2019). “Gorgonian corals (39),” in Mesophotic Coral Ecosystems Coral Reefs of the World, eds Y. Loya et al. (Basel:Springer Nature), 727–745. doi: 10.1007/978-3-319-92735-0_39
Sánchez, J. A., Herrera, S., Navas-Camacho, R., Rodríguez-Ramírez, A., Herron, P., Pizarro, V., et al. (2010). White plague-like coral disease in remote reefs of the Western Caribbean. Rev. Biol. Trop. 58, 145–154. doi: 10.15517/rbt.v58i1.20031
Sánchez, J. A., Pizarro, V., Acosta-De-Sanchez, A. R., Castillo, P. A., Herron, P., Martinez, J. C., et al. (2005). Evaluating coral reef benthic communities in remote atolls (quitasueno, serrana, and roncador banks) to recommend marine-protected areas for the seaflower biosphere reserve. Atoll Res. Bull. 531, 1–66. doi: 10.5479/si.00775630.531.1
Sánchez, J. A., and Wirshing, H. H. (2005). A field key to the identification of tropical western Atlantic zooxanthellate octocorals (Octocorallia?: Cnidaria). Caribb. J. Sci. 41, 508–522.
Sánchez, J. A., Zea, S., and Díaz, J. M. (1998). Patterns of octocoral and black coral distribution in the oceanic barrier reef-complex of providencia Island, Southwestern Caribbean. Caribb. J. Sci. 34, 250–264.
Schneider, C. A., Rasband, W. S., and Eliceiri, K. W. (2012). NIH Image to ImageJ: 25 years of image analysis. Nat. Methods 9:671. doi: 10.1038/nmeth.2089
Schönberg, C. H. L., Fang, J. K. H., Carreiro-Silva, M., Tribollet, A., and Wisshak, M. (2017). Bioerosion: the other ocean acidification problem. ICES J. Mar. Sci. 74, 895–925. doi: 10.1093/icesjms/fsw254
Schrope, M. (2008). Conservation: providential outcome. Nat. News 451, 122–123. doi: 10.1038/451122a
Sherman, C., Schmidt, W., Appeldoorn, R., Hutchinson, Y., Ruiz, H., Nemeth, M., et al. (2016). Sediment dynamics and their potential influence on insular-slope mesophotic coral ecosystems. Cont. Shelf Res. 129, 1–9. doi: 10.1016/j.csr.2016.09.012
Slattery, M., and Lesser, M. P. (2014). Allelopathy in the tropical alga Lobophora variegata (Phaeophyceae): mechanistic basis for a phase shift on mesophotic coral reefs? J. Phycol. 50, 493–505. doi: 10.1111/jpy.12160
Spalding, M., Burke, L., Wood, S. A., Ashpole, J., Hutchison, J., and zu Ermgassen, P. (2017). Mapping the global value and distribution of coral reef tourism. Mar. Policy 82, 104–113. doi: 10.1016/j.marpol.2017.05.014
Taylor, E., Baine, M., Killmer, A., and Howard, M. (2013). Seaflower marine protected area: governance for sustainable development. Mar. Policy 41, 57–64. doi: 10.1016/j.marpol.2012.12.023
Triffleman, N. J., Hallock, P., and Hine, A. C. (1992). Morphology, sediments, and depositional environments of a small carbonate platform; Serranilla Bank, Nicaraguan Rise, Southwest Caribbean Sea. J. Sediment Res. 62, 591–606. doi: 10.1306/D426796A-2B26-11D7-8648000102C1865D
Valderrama, D., and Zea, S. (2003). Esquemas de distribución de esponjas arrecifales (Porifera) del noroccidente del golfo de Urabá, Caribe sur, Colombia. Bol. Investig. Mar. Costeras 32, 37–56.
Velásquez, J., López-Angarita, J., and Sánchez, J. A. (2011). Evaluation of the FORAM index in a case of conservation. Biodivers. Conserv. 20, 3591–3603. doi: 10.1007/s10531-011-0152-7
Velásquez, J., and Sánchez, J. A. (2015). Octocoral species assembly and coexistence in Caribbean coral reefs. PLoS One 10:e0129609. doi: 10.1371/journal.pone.0129609
Weil, E. (2004). “Coral reef diseases in the wider Caribbean,” in Coral Health and Disease, eds E. Rosenberg and Y. Loya (Berlin: Springer), 35–68. doi: 10.1007/978-3-662-06414-6_2
Wiedenmann, J., D’Angelo, C., Smith, E. G., Hunt, A. N., Legiret, F.-E., Postle, A. D., et al. (2013). Nutrient enrichment can increase the susceptibility of reef corals to bleaching. Nat. Clim. Change 3, 160–164. doi: 10.1038/nclimate1661
Wilkinson, C., and Souter, D. (2008). Status of Caribbean Coral Reefs after Bleaching and Hurricanes in 2005. Townsville: Global Coral Reef Monitoring Network, and Reef and Rainforest Research Center.
Keywords: coral reefs, coral decline, Caribbean, algae cover, FORAM index, octocoral community, atoll, SeaFlower
Citation: Sánchez JA, Gómez-Corrales M, Gutierrez-Cala L, Vergara DC, Roa P, González-Zapata FL, Gnecco M, Puerto N, Neira L and Sarmiento A (2019) Steady Decline of Corals and Other Benthic Organisms in the SeaFlower Biosphere Reserve (Southwestern Caribbean). Front. Mar. Sci. 6:73. doi: 10.3389/fmars.2019.00073
Received: 31 July 2018; Accepted: 08 February 2019;
Published: 26 February 2019.
Edited by:
Rochelle Diane Seitz, College of William & Mary, United StatesReviewed by:
Carlos Jimenez, Enalia Physis Environmental Research Centre (ENALIA), CyprusCopyright © 2019 Sánchez, Gómez-Corrales, Gutierrez-Cala, Vergara, Roa, González-Zapata, Gnecco, Puerto, Neira and Sarmiento. This is an open-access article distributed under the terms of the Creative Commons Attribution License (CC BY). The use, distribution or reproduction in other forums is permitted, provided the original author(s) and the copyright owner(s) are credited and that the original publication in this journal is cited, in accordance with accepted academic practice. No use, distribution or reproduction is permitted which does not comply with these terms.
*Correspondence: Juan Armando Sánchez, anVhbnNhbmNAdW5pYW5kZXMuZWR1LmNv
†These authors have contributed equally to this work
Disclaimer: All claims expressed in this article are solely those of the authors and do not necessarily represent those of their affiliated organizations, or those of the publisher, the editors and the reviewers. Any product that may be evaluated in this article or claim that may be made by its manufacturer is not guaranteed or endorsed by the publisher.
Research integrity at Frontiers
Learn more about the work of our research integrity team to safeguard the quality of each article we publish.