- 1Sea Mammal Research Unit, Scottish Oceans Institute, University of St Andrews, St Andrews, United Kingdom
- 2BIOECOMAC Department of Animal Biology, Universidad de La Laguna, San Cristóbal de La Laguna, Spain
- 3Cascadia Research Collective, Olympia, WA, United States
- 4School of Biological Sciences, The University of Auckland, Auckland, New Zealand
- 5Bahamas Marine Mammal Research Organisation, Abaco, Bahamas
- 6Department of Biology, Dalhousie University, Halifax, NS, Canada
- 7Marine Ecology and Telemetry Research, Seabeck, WA, United States
Until the 1990s, beaked whales were one of the least understood groups of large mammals. Information on northern bottlenose whales (Hyperoodon ampullatus) and Baird’s beaked whales (Berardius bairdii) was available from data collected during whaling, however, little information existed on the smaller species other than occasional data gleaned from beach-cast animals. Recent research advances have been plentiful. Increasing global survey effort, together with morphometric and genetic analyses have shown at least 22 species in this group. Longitudinal field studies of at least four species (H. ampullatus, B. bairdii, Ziphius cavirostris, Mesoplodon densirostris) have become established over the last three decades. Several long-term studies support photo-identification catalogs providing insights into life history, social structure and population size. Tag-based efforts looking at diving, movements and acoustics have provided detail on individual behavior as well as population structure and ranges. Passive acoustic monitoring has allowed long-term and seasonal monitoring of populations. Genetic studies have uncovered cryptic species and revealed contrasting patterns of genetic diversity and connectivity amongst the few species examined. Conservation concern for these species was sparked by mass strandings coincident with military mid-frequency sonar use. Fat and gas emboli have been symptomatic indicators of mortalities related to sonar exposure, suggesting that their vulnerability stems from the physiological exertion of extreme diving for medium-sized whales. Behavioral response experiments have now shown that beaked whales appear to cease foraging and delay their return to foraging and/or leave the area in association with exposure to mid-frequency signals at low acoustic levels. Future priorities for these species will be to (1) continue field-studies to better understand smaller-scale habitat use, vital rates and social structure; (2) develop better detection methods for larger-scale survey work; (3) improve methodology for monitoring energetics, individual body condition and health; (4) develop tools to better understand physiology; (5) use recent genetic advances with improved sample databanks to re-examine global and local beaked whale relationships; (6) further quantify anthropogenic impacts (both sonar and other noise) and their population consequences (7) apply acquired data for realistic mitigation of sonar and other anthropogenic impacts for beaked whale conservation.
Introduction
Beaked whales (Ziphiidae) are a family of odontocete cetaceans (toothed whales) containing at least 22 species in 6 genera. Species range in size from 3 m and a few 100 kg to more than 10 m and 12 tones. All beaked whale species tend to live in deep, typically offshore, waters and, for those species for which dive data are available, all perform long, deep dives in search of their prey. Many species of beaked whales (particularly the genus Mesoplodon, which is the most numerous cetacean genus) have superficially similar external morphology, and so are often difficult to identify to species level in the wild, hampering field observations. Difficulties are enhanced by the variability of coloration of several species, with new patterns still being discovered (Aguilar de Soto et al., 2017). Therefore, while some species have detailed distribution records, many others have only a handful of scattered reports (MacLeod et al., 2006). The study of many of these species has therefore often relied on examination of beachcast animals (Ridgway and Harrison, 1989).
Several species have yet to be knowingly seen alive at sea, including six different species from the genus Mesoplodon (Dalebout et al., 2002). As a result, we still know comparatively little about many of these large mammal species. This scarcity of knowledge is a concern because beaked whales have been identified as particularly sensitive to some human activities, in particular those that generate high levels of underwater noise. Data are needed on physiology and behavior to understand the mechanisms of noise impacts, and how to mitigate them in order to guarantee beaked whale conservation. The lack of baseline data on distribution, population size and structure, and life history impedes assessment of potential population level impacts. Likewise, it is difficult to predict the consequences of climate change given the paucity of knowledge of prey or likely shifts in prey distribution, and the energetic and physiological impacts of these changes.
The purpose of this paper is to review current knowledge, recent advances and outline future directions in research for this diverse group of marine mammals. It is intended as a roadmap for future research on beaked whales, identifying pitfalls and shortcuts and accelerating progress toward key goals: a better understanding of their biology and ecology and the application of data for conservation and management.
History of Beaked Whale Studies
Whaling
Only two beaked whale species, northern bottlenose whales (Hyperoodon ampullatus) and Baird’s beaked whales (Berardius bairdii) were the targets of whaling operations. The northern bottlenose whale was hunted across the Northern Atlantic (e.g., Benjaminsen, 1972; Gray, 1882), while the Baird’s beaked whale continues to be hunted in Japan (e.g., Kasuya, 1977, 1986). Scientific research programs associated with the whaling operations provided reproductive and demographic information for these two species but information for most other beaked whales was sparse, often gleaned only occasionally from necropsies of stranded animals (Mead, 1984).
Field Studies
Long-term, non-lethal studies of wild populations began in the 1980s, with the study of northern bottlenose whales in the Gully, off the coast of eastern Canada (Table 1). Since then, several factors have led to a dramatic increase in research into beaked whale biology and ecology (Figure 1). An increase in number and diversity of studies has been partly driven by technological advances (Baird et al., 2006; Tyack et al., 2006; Schorr et al., 2009). However, there has also been an increased focus on beaked whales following evidence of a relationship between mid- and low-frequency sound production and mass strandings of at least some species (Frantzis, 1998; Cox et al., 2006), confirmed by common veterinary pathological findings (Fernandez et al., 2005). Today non-lethal long-term studies include research on single populations of northern bottlenose whales and Baird’s beaked whales, and multiple populations of Blainville’s beaked whales (Mesoplodon densirostris) and Cuvier’s beaked whales (Ziphius cavirostris) (Table 1).
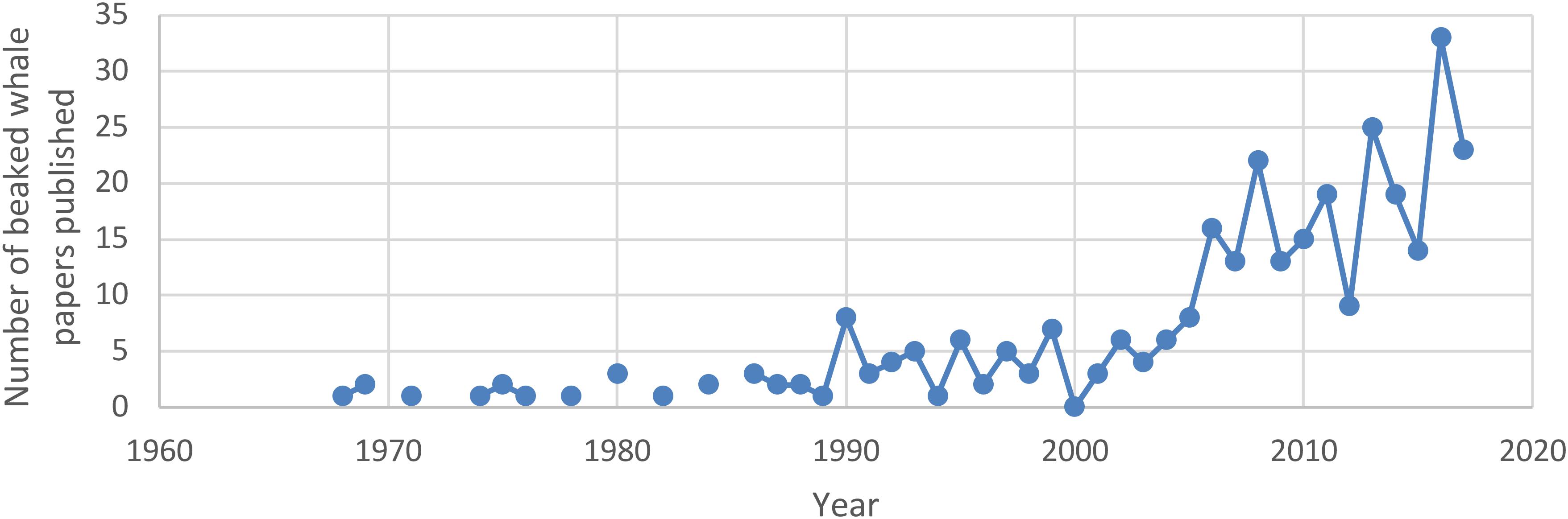
Figure 1. Web of science search results for papers with “beaked whale” in title through 2017. Increasing publication rate in the 1990s and again in the late 2000s is clearly visible.
Additional studies have also been more recently instigated. Some of these are currently becoming or have the potential to be extended into long-term studies1. Cuvier’s beaked whales have been regularly studied in the Mediterranean where there may be several key areas of whale abundance (MacLeod and Mitchell, 2006), with photo-identification studies in both the Genoa Canyon and the Ionian Sea (Canadas, 2012; Podesta et al., 2016). Cuvier’s beaked whales have also been the subject of intensive investigation for the last 6 years off North Carolina (Forney et al., 2017), an area with very high whale density (McLellan et al., 2018). Another Cuvier’s beaked whale population has been studied off Guadalupe Island, Mexico (Cárdenas-Hinojosa et al., 2015). A reliable population of northern bottlenose whales has been studied for several summer seasons off Jan Mayen, Norway (Miller et al., 2015, 2016). As interest has increased, opportunistic encounters with other beaked whale species have also been more widely reported. True’s beaked whales (Mesoplodon mirus) are regularly observed around the Azores and Canary Islands (Aguilar de Soto et al., 2017) and off George’s Bank in the western North Atlantic (DeAngelis et al., 2018). Sowerby’s beaked whales (Mesoplodon bidens) are regularly found in the Gully (Whitehead, 2013) and around the Azores (Silva et al., 2014).
Methods and Technology: Current Status
Species Identification
Molecular work, often in combination with morphological analysis, has proved vital to the discovery and identification of new species based on bones and tissues of dead individuals, using short fragments of mitochondrial (mtDNA) and nuclear DNA. For example, until recently one species, Longman’s beaked whale (Indopacetus pacificus), was identified only from two skulls in Australia and Somalia and it was not until 2003 that the ability to identify these animals in the wild was confirmed by combining morphological, photographic and molecular evidence (Dalebout et al., 2003). In 2004, a comprehensive molecular database of the 21 species recognized at the time was compiled using mtDNA and nuclear DNA markers that was validated using either skeletal material or some other form of documentation (Dalebout et al., 2004). This confirmed the monophyly of the beaked whale family and this and subsequent work produced insights into the speciose group. More recently, Deraniyagala’s beaked whale (Mesoplodon hotuala) (Dalebout et al., 2014) and a new species of Berardius (Morin et al., 2017) were identified by analyzing DNA-sequence data from specimens thought to be a smaller rare black-form of the species. If the black-form of Berardius is accepted (Morin et al., 2017), it will bring the species count to 23.
Beaked Whale Detection
Beaked whales, due to their long dives and often inconspicuous behavior at the surface, have particularly low detection rates compared to most other cetaceans (Barlow, 2015). However, like all odontocetes they are acoustic animals, and rely on echolocation clicks to navigate, forage and communicate. In key habitat areas they are found to click during their foraging dives made during both day and night (e.g., Baird et al., 2008; Stanistreet et al., 2017), being acoustically active some 20% of the time (Arranz et al., 2011). Beaked whale clicks are unique from other odontocetes in their temporal and spectral properties (Johnson et al., 2004, 2006) and, for those species that have been characterized, can be distinguished to the species level (albeit with some uncertainty due to off-axis effects and narrow acoustic beam) by their high frequency (peaks greater than 25 kHz), longer durations, consistent inter-click intervals, and characteristic frequency upsweep, using automated supervised detection systems (Baumann-Pickering et al., 2014).
Passive acoustic monitoring has allowed long-term datasets to be collected in specific regions to assess presence/absence over multiple seasons/years (e.g., Moretti et al., 2006; Baumann-Pickering et al., 2014; Kowarski et al., 2018). These have highlighted the presence of beaked whales despite lack of characterization to species-level (Baumann-Pickering et al., 2014). In some locations, real-time acoustic monitoring has been possible (primarily on Navy acoustic ranges, Moretti et al., 2016), which has helped to direct on-water research effort (Tyack et al., 2011).
Population Monitoring
Long-term beaked whale photo-identification (photo-ID) studies (Table 1), have provided critical information on population structure, population size and vital rates. Many beaked whale individuals show unique markings – either via nicks and notches in the dorsal fin, or via scars or rake marks on the back and sides – and so individuals can be identified photographically and detected repeatedly over time (e.g., Gowans and Whitehead, 2001; McSweeney et al., 2007; Fedutin et al., 2015). Many of these long-term studies have shown small and site-specific populations (Table 1). In some cases these photo-ID studies have been conducted in conjunction with satellite tagging to help further elucidate population range and habitat use (e.g., Schorr et al., 2009; Baird et al., 2011).
Passive acoustic monitoring has also provided detail on the seasonal and long-term usage of specific areas by beaked whale species. Bottom-mounted autonomous acoustic recorders, variously called Ecological Acoustic Recorders (EARs) or High-frequency Acoustic Recording Packages (HARPs), or Autonomous Multichannel Acoustic Recorders (AMARs), have been deployed across several sea-floor locations in the Pacific where they showed little seasonal influence on beaked whale detection (Baumann-Pickering et al., 2014; but see also Stanistreet et al., 2017; Kowarski et al., 2018). However, bottom-mounted archival hydrophones have traced seasonal, spatial and inter-annual variation in northern bottlenose whale abundance in the Gully area, and more broadly over all eastern Canadian waters (Stanistreet et al., 2017).
Population Structure and Social Organization
Long-term photo-ID work has allowed animals to be identified individually and has demonstrated persistent and resident populations, often with little connectivity between nearby locations (Table 1). Many beaked whales can be identified photographically in terms of their age/sex status. Mature males can be differentiated from females morphologically in most beaked whales (Ridgway and Harrison, 1989). In tropical areas scars from cookie-cutter shark bites (e.g., Hawai‘i, McSweeney et al., 2007) can aid assessment of age, while in other areas other types of accumulated scars (e.g., linear scars caused by male-male contact) or changes in pigmentation patterns (e.g., Gowans et al., 2000a; Coomber et al., 2016) have been combined with re-sighting information to assess this (Gowans et al., 2001; Baird, 2019).
Individual association patterns are variable between species. Northern bottlenose whales have been found to show stronger male-male associations than other age-sex class associations (Gowans et al., 2001). A similar pattern was found in Baird’s beaked whales in which more scarred (old and/or male) animals were most likely to form stable associations (Fedutin et al., 2015). Cuvier’s and Blainville’s beaked whales appear to be much less social, often found either as lone individuals or in small groups (Claridge, 2006; McSweeney et al., 2007; Baird, 2019). For both species, females with small calves seem to preferentially associate in larger groups containing multiple adult females (Baird, 2019). Larger groups of Cuvier’s beaked whales often have more than one adult male present, while for Blainville’s beaked whales typically only one adult male is present, suggesting female defense polygyny (Claridge, 2006; McSweeney et al., 2007; Baird, 2019). Studies of association patterns of Blainville’s beaked whales suggest that male and female associations may last for at least a year (Dunn, 2015). At-sea behavioral observations can also provide detail on animal social interactions, such as the head-butting behavior observed for male bottlenose whales (Gowans and Rendell, 1999).
Since the early 2000s molecular methods have been used to assess population structure in beaked whales, showing different species have contrasting patterns of genetic diversity and connectivity. Cuvier’s are the only globally distributed beaked whale for which a study of genetic diversity has been conducted, and the species showed significant differentiation between ocean basins (Dalebout et al., 2005). In contrast, high genetic diversity and no genetic structure was found for Gray’s beaked whales (Mesoplodon grayii) around New Zealand where morphological differences have been noted between the east and west sides of the country (Thompson et al., 2014). The work also suggested that Gray’s beaked whales underwent a population expansion with abundant habitat, limited geographic barriers, and no directed exploitation by humans (Thompson et al., 2016). Gray’s beaked whales are unique among ziphiids in that when they strand, it is often in large groups (e.g., 28 in a mass stranding). Patel et al. (2017) used microsatellites and mtDNA haplotypes from 113 stranded animals to show that both male and female dispersal is likely in the species and that the whales strand in unrelated groups. Regional work in the North Atlantic has shown low genetic diversity and significant population structure between Gully and Labrador stocks of northern bottlenose whales based on mtDNA control region and microsatellite markers (Dalebout et al., 2001, 2006).
Diving and Foraging
Much information on beaked whale diet has been derived from studies of prey contents found in the stomachs of stranded or whaled animals (Ridgway and Harrison, 1989; Fernandez et al., 2014; West et al., 2017). However, some chemical sampling methods (stable isotope and fatty acid analysis) can also provide detail about prey types (Hooker et al., 2001). Different beaked whale species appear to specialize to differing extents on squid and fish (Riccialdelli et al., 2017). Studies of prey can also help to infer recent movements of animals. For instance, the presence of warm-water prey has been used to suggest that northern bottlenose whales stranded in the northeast Atlantic had probably recently traveled northward (Fernandez et al., 2014).
Passive acoustic recorders and hydrophone networks can be used to detect the foraging clicks of beaked whales, and are an excellent tool to examine temporal variability in foraging behavior. In the Northern Main Hawaiian Islands (Ni’iahu and Kaua’i) Blainville’s beaked whales showed similar rates of foraging day and night, with a slight decrease during crepuscular periods (Henderson et al., 2016). Tagging studies of both Blainville’s and Cuvier’s beaked whales have also shown similar rates of foraging dives during both day and night (Baird et al., 2008).
The field of biotelemetry has advanced rapidly in the last two decades, providing a more direct way to assess diving and foraging in beaked whales. Tagging studies have been conducted on beaked whales since 1997 (Hooker and Baird, 1999). These have shown the very deep-diving capabilities common across all beaked whale species studied (e.g., Johnson et al., 2004; Tyack et al., 2006; Minamikawa et al., 2007; Baird et al., 2008). Importantly, some of these tags have recorded sound (Johnson and Tyack, 2003). Depth and acoustic data combined have highlighted the unique foraging behavior of beaked whales (Tyack et al., 2006), with whales feeding during long and deep dives to over 800 m depth performed typically each 1–1.5 h, after which there may be one to several silent recovery dives that can exceed 400 m depth and 30 min long. These tags have also described some of the social sounds made by beaked whales (Aguilar de Soto et al., 2012; Dunn et al., 2013).
Most tagging efforts initially focused on high-resolution but short-term suction cup-attached tags. These are currently still limited to average deployments of less than 1 day, and maximum of a few days (Shorter et al., 2014). New research into both the material properties of suction cups (Shorter et al., 2014), and physical properties of the cups, e.g., adding texture, may help to increase attachment durations. Parallel efforts have developed longer-duration animal-attached tags by using penetrating darts on small cetaceans, including beaked whales (e.g., Schorr et al., 2009). Current dart-attached tags in beaked whales have been demonstrated to last as long as 120 days (Schorr et al., 2014), although most attachments are on the order of a month. These longer-term diving records have provided important insights into the range of behaviors these whales are capable of, in addition to regional and individual variability within species (Schorr et al., 2014). The recent addition of Fastloc global positioning systems (GPS) into dart-attached LIMPET tags (Wildlife Computers) has increased the ability to collect high-resolution spatial data in addition to summarized dive data (R. D. Andrews, unpublished data).
Attaching tags to smaller cetaceans with a tendency to avoid boats, such as beaked whales, has required the use of light-weight carbon fiber poles allowing tag placement from 5–8 m distance. Suction-cup Dtags have been attached to bottlenose whales using the ARTS pneumatic launching system, which increased effective tagging ranges to 12–15 m (Miller et al., 2016). Small dart-attached tags, such as LIMPET tags, have also been deployed this way. As the precision of pneumatic launchers increases, researchers will be able to deploy larger tags from greater distances, though orientation to the animal continues to be a challenge, particularly for dart-attached tags. The ethics of deploying larger tags to relatively small whales with a thin blubber layer has also been raised as a concern (Moore and Zerbini, 2017).
Insights into how beaked whales can conduct such long and deep dives have also been gained by analysis of the morphology and anatomy of stranded whales (e.g., Velten et al., 2013; Pabst et al., 2016). Mesoplodon beaked whales have been found to differ from other deep-diving cetaceans in their locomotor muscle design, with the fast glycolytic muscle fibers of a sprinter. It is hypothesized that these fibers may be a metabolically inexpensive oxygen store, recruited later on ascent to aerobically power swimming. This may help to explain accelerometer-derived observations of a change in movement patterns (type of stroke) during ascent (Lopez et al., 2015).
Behavioral Response to Anthropogenic Impacts
It is now accepted that beaked whales can strand due to exposure to naval sonar, though the precise mechanism by which this occurs remains poorly understood. Fat and gas emboli have been symptomatic indicators of mortalities related to sonar exposure, suggesting that their vulnerability stems from the physiological exertion of extreme diving for medium-sized whales (Cox et al., 2006; Hooker et al., 2012). Spatial and temporal correlations between sonar activity and beaked whale strandings suggest that bathymetric topography may also be a factor (Filadelfo et al., 2009). A comprehensive review of beaked whale mass strandings (D’amico et al., 2009) suggested that some strandings might also be associated with other source events such as seismic surveys, although evidence for these is less comprehensive.
Increasing conservation concerns about strandings associated with military low- and mid-frequency sonar use have led to many recent studies looking into reactions of beaked whales to noise. These found similar behavioral responses to simulated sonar signals among the small number of beaked whales tested, with responses to starting at low received levels (100–130 dB re. 1 μPa; Table 2). Beaked whale species appear to respond similarly, typically showing a combination of avoidance and cessation of click-production associated with foraging (Table 2 and references therein). These types of behavioral changes were also documented in work monitoring vocal activity using navy range hydrophones (Tyack et al., 2011; Moretti et al., 2014; Henderson et al., 2016), response to intense ship noise (Aguilar de Soto et al., 2006) and as a reaction to active echosounder activity (Cholewiak et al., 2017).
Future Directions
Future directions for beaked whale research are likely to come both from methodological and technological improvements, and most importantly from continuation and further development of long-term population monitoring datasets to provide increasing detail on habitat-use, social structure and fundamental natural history data such as survival, age at sexual maturity, pregnancy rate, and natural infant mortality (Figure 2). Improvements in technological tools will support development of acoustic and DNA detection methods, and methods such as drone technologies will enable monitoring of body condition and physiology. Better understanding of smaller-scale habitat use and social interactions will be gained as increasing numbers of long-term studies come to fruition and other sources of information are incorporated (e.g., citizen science, genetics, medium duration high-resolution multi-sensor archival tags). Increasing availability of global datasets and databanks such as GenBank will allow examination of beaked whale relationships and differences. In terms of understanding anthropogenic threats, larger sample sizes of exposure to real sonar and increased attention to nuances of whale behavior will allow better determination of the causal factors leading to detrimental responses. We collate these into seven main research directions which will further develop our understanding of beaked whale biology, ecology and conservation.
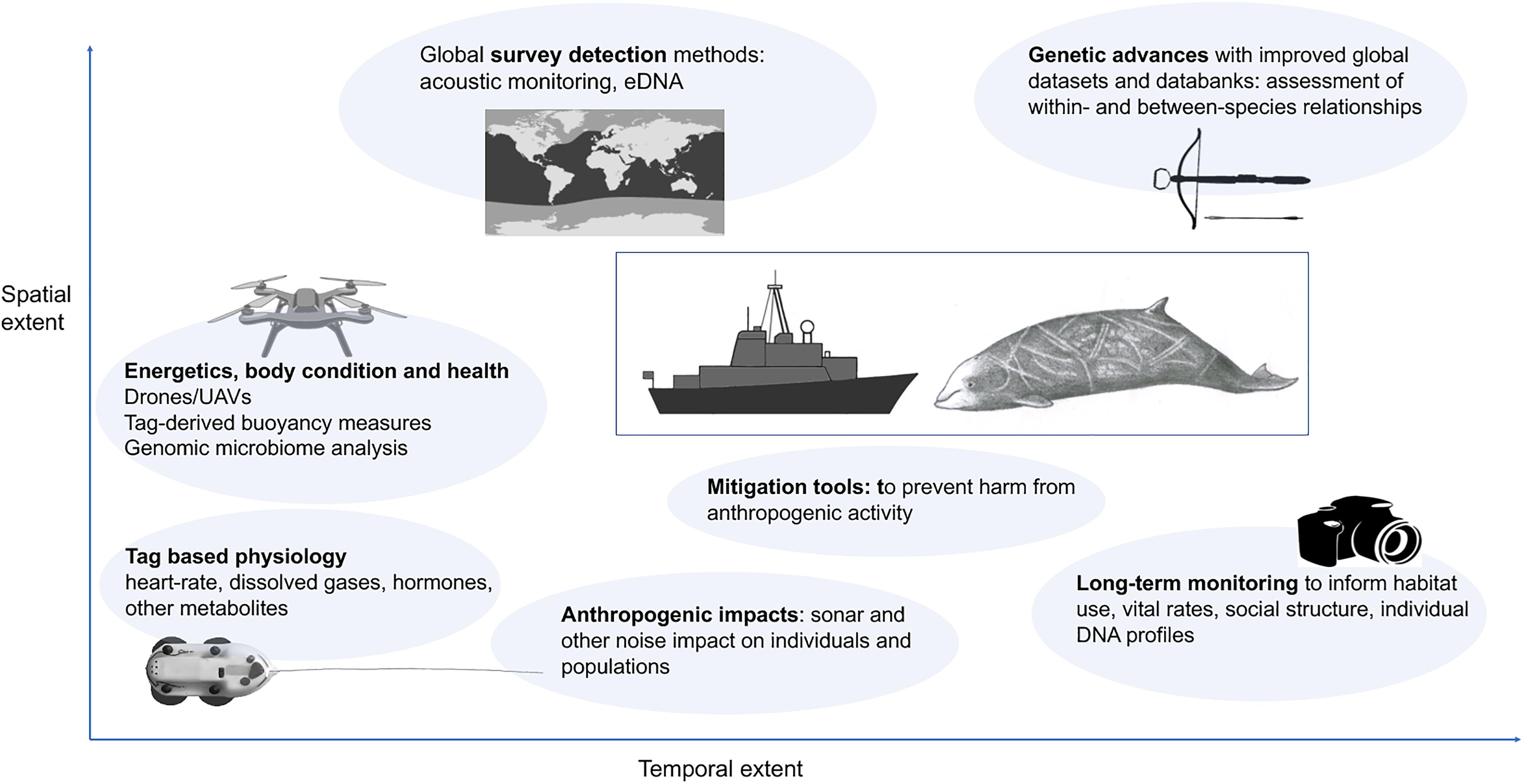
Figure 2. Suggested future directions for beaked whale research vary in terms of both spatial and temporal extent. See text for more information on each.
Continue Field-Studies to Better Understand Individuals and Populations
One of the limiting factors for beaked whale studies is sample size, given the often low density of animals in an area and the even lower sighting probabilities. In some oceanic archipelagos with deep water near the coast, beaked whale research is aided by whale watching data (e.g., Hawai‘i, Canary Islands). Here, citizen science is playing a role, with contributions of photos that can help address distribution (e.g., Aguilar de Soto et al., 2017), demographics, vital rates and social structure (e.g., Baird, 2019) by filling in temporal gaps in sampling, and in general improving sample size. With increasing numbers of people on the water with high resolution cameras, it is likely that such citizen science will increase its role over time.
The ability to follow individuals over longer periods of time than just one or a few surfacing bouts is also a limiting factor, and boat-based researchers working in concert with land-based observers (e.g., Canary Islands, Arranz et al., 2014), or tracking tagged animals in areas that combine photo-identification with tagging (e.g., Hawai‘i, North Carolina, Canary Islands), allow for longer-term focal follows that can help address small-scale habitat use and/or social structure questions. Satellite tagging studies also have the potential to inform understanding of social structure as well as fine scale habitat use, the latter in particular when Fastloc-GPS tags are being used. Even with the relatively low resolution of Argos-derived locations, combining satellite tag data with oceanographic data provides a powerful approach to assessing what factors may drive spatial use (e.g., Abecassis et al., 2015), though data on the deep-water oceanography where these species forage remains sparse and difficult to collect.
When multiple tags are deployed on individuals in the same group (or on individuals in different groups at the same time), the distance between individuals (in relation to social structure) as well as coordination of diving behavior can be assessed (Aguilar de Soto et al., 2006). In such cases, tags may provide medium-term information on how long individuals remain together in the same group (particularly with Fastloc-GPS enabled tags), or how often individuals join and split. Commercially available radio direction finder systems to track Argos tags (e.g., the Argos goniometer) also allow for re-locating satellite tagged individuals potentially over periods of days to weeks, allowing assessment of association patterns.
The ability to assign individuals to an age class is important for estimation of past and future vital rates, as well as population-level responses to abiotic (e.g., climate) and biotic (e.g., prey resources) factors. For at least some species of beaked whales, scars accumulate and remain visible thus allowing estimation of age and contributing to studies of vital rates and demography. The development of molecular age biomarkers, or measurable changes in DNA or RNA abundance or sequence change with time, provides a new way to estimate chronological age (Jarman et al., 2015). Such tools could be validated using both known-age animals from long-term study sites and from stranded animals aged using skeletal materials or natural chemical markers (Polanowski et al., 2014; Laptikhovsky et al., 2018).
Acoustic records can also provide insight. Creaks/buzzes (clicks with short inter-click intervals) may be used to infer prey capture, and thus give a measure of foraging activity (Madsen et al., 2005). Recordings of simultaneous or coordinated click trains can indicate associations, and give insight into behavior and social structure. And, because noise is seen as a primary threat to beaked whales (Cox et al., 2006; Cholewiak et al., 2017), the simultaneous recording of anthropogenic sounds (generated by ships, echosounders, sonars, seismic, military, and other sources) can allow us to measure how the distribution, abundance, foraging, and sociality of beaked whales are affected by human activities.
Genetic and genomic methods can be used to identify and track individuals and their kin over time and provide a way of assessing relatedness and social structure. Genetic profiles that uniquely identify individuals can be generated using DNA from skin biopsy samples, sloughed skin from living whales, and tissue samples from dead whales (Carroll et al., 2018). By tracking individuals across space and time, genetic monitoring with DNA profiles has been used to estimate demographic parameters like abundance, growth rate and population expansion in cetacean populations (e.g., Carroll et al., 2013). Additionally, social structure, dispersal patterns and mating systems can be inferred from patterns of relatedness and paternity (e.g., Patel et al., 2017). Given the difficulty in directly observing mating and social interactions between beaked whales, genetic information provides insight into social structure that is important for conservation and management (e.g., showing that Gray’s beaked whales strand in unrelated groups, Patel et al., 2017). Genetic methods could be used in future, alone or in combination with photo-ID data, to determine whether small beaked whale populations are genetically and demographically isolated.
Develop Better Detection Methods for Larger-Scale Survey Work
Acoustics have become an effective way to map the temporal and spatial distributions of beaked whale populations. Hydrophones mounted on the sea floor (either “pop-up” archival arrangements, or cabled systems), towed behind boats, or from autonomous underwater or surface vehicles, such as “gliders” (Suberg et al., 2014) or “drifters” (Griffiths and Barlow, 2016), are giving high-resolution but broad-scale data on when and where different species are present (e.g., Klinck et al., 2012; Stanistreet et al., 2017). These acoustic records have the potential to be used for abundance estimation, either using modified “Distance” methods in which source directionality is typically estimated using some form of array (Marques et al., 2013) or simpler one-dimensional estimates that use data from single non-directional hydrophones (Horrocks et al., 2011). Both methods need information on typical group sizes within a particular area, as well as estimates of the click-rates of individuals over a variety of time scales. The latter can come from acoustic-tag recordings (e.g., Tyack et al., 2006), but may vary in groups of different sizes.
The burgeoning field of environmental DNA (eDNA) research may provide an opportunity to develop better detection methods for beaked whales. The simple premise is that animals in the environment shed cells and DNA in their wake, leaving a trail that can be detected using molecular methods. Evidence suggests that eDNA is a reliable detection method (Rees et al., 2014), although this depends on the system under investigation (Foote et al., 2012). So far, applications to marine mammal science have been few, although it has been shown to detect harbor porpoises (Phocoena phocoena) in both a controlled and natural environment (Foote et al., 2012). The duration of detection of killer whales (Orcinus orca) with eDNA was estimated to be 2 h in the Puget Sound, although more work is needed to validate this and to determine detection probabilities across species and marine systems (Baker et al., 2018). One key factor required for a successful eDNA study is molecular information to design and validate species identification assays. The extensive molecular phylogeny work conducted on beaked whales provides such information (e.g., Dalebout et al., 2004). In fact, the parallels between eDNA and DNA from bone, such as degradation of DNA into short fragments and the relative abundance of mtDNA compared with nuclear DNA, mean that previous molecular work conducted on beaked whales lends itself to eDNA work and puts the taxonomic group in an excellent position for future eDNA studies. The ability of genomic methods to categorically identify individuals to species level is also particularly useful when sightings of ‘unidentified’ beaked whales are made, or to ground-truth acoustic monitoring.
Monitoring Energetics, Individual Body Condition, and Health
Little is known about the food or metabolic requirements of beaked whales as temporary capture (or maintenance in captivity) for physiological measurements has rarely been attempted (Lynn and Reiss, 1992). Recent advances in the use of animal-attached tags have made it possible to measure indicators of prey-consumption from echolocation buzzes produced during prey-capture attempts (Johnson et al., 2004) and locomotion effort using accelerometers to measure stroking (Williams et al., 2017). Breathing patterns can also be used to investigate field metabolic rates and energy expenditure (Isojunno et al., 2018), although assumptions about constant tidal volume and rate of oxygen extraction may cloud this approach. That said, these metrics have been useful as energy indicators, or proxies to identify potential effects of disturbance (Miller et al., 2015).
Aerial photogrammetry and the use of drones has been developed to assess body shape changes to infer reproductive status, nutritive condition and growth in cetaceans (Miller et al., 2012; Fearnbach et al., 2018). Lactation imposes high energetic demands on female cetaceans and aerial photogrammetry has demonstrated that body condition is compromised in the early months of lactation when whales may have to rely on endogenous nutrient reserves to support increased energy expenditure (Christiansen et al., 2016). However, full ground truthing against measured hormones (e.g., from blubber or blow, Hunt et al., 2013) would help to establish efficacy for its use as an indicator of nutritional stress (e.g., using cortisol) and pregnancy (e.g., using progesterone). Ultimately individual-based annual changes in body condition could provide an indicator of longer-term nutritional stress. The potential utility of aerial photogrammetry for assessing body condition and predicting pregnancy in Blainville’s beaked whales at the Abaco, Bahamas study site has been demonstrated (J. W. Durban, unpublished data). Aerial images indicated variability in individuals’ body shapes likely associated with nutrition and pregnancy, notably lean females with dependent calves and the anomalous width profile of a pregnant female. Aerial photographs of Blainville’s can be readily matched to established photo-identification catalogs, allowing photogrammetric measurements to be linked to known individuals, providing the ability to monitor changes in body condition and growth over time. Even if there is no long-term sighting history, images can be assigned to state-specific and sex classes based on dentition and scarring (albeit with some uncertainty between females and subadult males in areas with few cookie cutter sharks; see Claridge, 2013). Such aerial imagery in combination with long-term field studies will allow measurement of the length and impact of the weaning period, and ultimately beaked whale reproductive potential.
Another approach to quantify energy balances of free-ranging animals is to assess the quantity of their lipid-store from how it influences their buoyancy. Lipids are less dense than other body tissues, and so the buoyancy of marine divers has been used as an indicator of body condition (Biuw et al., 2003). There may be two difficulties in using this for beaked whales. (1) Beaked whales are considered to be income-breeders, and are not thought to go through prolonged fasting cycles like capital-breeding seals and many baleen whales. However, their medium body size implies a potential for lipid energy stores to be an important indicator of their body condition, particularly related to the energy and lipid demands for lactation and successful weaning of offspring (New et al., 2013). (2) Beaked whales have a rather unique blubber makeup in that their blubber is dominated by wax esters rather than triacylglycerol (Koopman, 2007). Wax esters have a lower density and their metabolization is less efficient than triacylglycerol. Nevertheless, the presence of more or less blubber would be expected to relate (albeit perhaps imperfectly) to accessible energy stores and body condition. Recent work has shown that northern bottlenose whales tagged with archival suction cup tags near Jan Mayen tended to have a lower body density, indicating larger proportional lipid stores, than those tagged in the Gully (Miller et al., 2016).
Future work on body condition (as measured either via photogrammetry or buoyancy models) would benefit from increased understanding of the function of wax-ester dominated blubber (which appears to be related to deep-diving; Koopman, 2007; Koopman and Westgate, 2012). The ability to measure lipid-stores over longer-time scales would assist ground-truthing its utility as an index of body condition and improve our understanding of how stores might fluctuate in response to metabolic requirements of the animals. These might include the need to lactate, or long-distance movements during which opportunities for feeding are likely to be more limited.
Genomic methods can also increase our understanding of beaked whale health and diet. For example, DNA diet studies provide a non-invasive method for assessing trophic interactions of marine mammals (Hardy et al., 2017). Additionally, genomic sequencing of microbial communities living on or in multicellular organisms or “hosts,” termed microbiomes, are becoming a way of monitoring the health of marine mammals (Apprill et al., 2017). This is because the microbiome can impact host fitness and health by direct (e.g., stimulating immunity) or indirect (e.g., modifying metabolism) mechanisms (Acevedo-Whitehouse et al., 2010). Monitoring of the southern resident killer whale population revealed antibiotic-resistant bacteria in the respiratory microbiome of apparently healthy individuals (Raverty et al., 2017). Monitoring over time could also indicate changes in the environment: characteristics of the microbiome have been shown to change with the quality of the social or broader environment in some species (e.g., Amato et al., 2013).
Develop Tag-Based Tools to Better Understand Physiology
Understanding the potentially dangerous responses of cetaceans to noise is a two-stage process: (1) understanding the noise required to cause the behavioral modification and (2) understanding the physiological mechanism by which that behavioral modification causes harm to the animal. At present, almost all research has focused on the first of these, i.e., work evaluating response to playbacks. Beyond efforts using models of nitrogen concentration (e.g., Hooker et al., 2009; Fahlman et al., 2014), almost nothing is known about the mechanism via which the response leads to harm (i.e., decompression stress).
Beaked whales are particularly remarkable for their astounding diving abilities and therefore present ideal subjects for further research on the physiological mechanisms underlying diving to great depth. Tag-based developments to incorporate heart-rate loggers in suction-cup attached tags are underway although to date these have only been deployed on captive harbor porpoises (McDonald et al., 2018). Remote deployment on free-ranging beaked whales would allow the collection of data on natural and exposure-driven physiological dive responses, providing additional insight into the causes of beaked whales’ adverse reaction to sonar.
It is also possible that additional sensors could be incorporated into the penetrating portions of a dart-attached longer-term tag. This would potentially allow collection of data on dissolved gasses, hormones or other metabolites which would also greatly improve our understanding of diving physiology (Hooker et al., 2012). However, ongoing concerns about a rigid tag implanted into semi-rigid blubber and the potential for muscle shear to cause tag-tip cavitation require further examination (Moore and Zerbini, 2017). There may be the potential for use of materials which more closely mimic the biomechanics of the tissue structure, and to increase the flexibility of the link between any external and internal tag components to help increase attachment duration, minimize tissue disruption and improve wound healing.
Use Recent Genetic Advances With Improved Sample Databanks
International collaboration is key to future genetic and genomic investigations of beaked whales as many species span ocean-basins and some are global. Many countries now have schemes that store samples from stranded whales in national archives (e.g., New Zealand’s Cetacean Tissue Archive: Thompson et al., 2013), often with photographs or other morphological meta-data. Museum collections also provide a valuable resource for older samples and type specimens. When brought together, such resources are the starting material for comparative studies of population structure and phylogenetics of beaked whales. Initiatives that bring these datasets together should be supported, despite the difficulties associated with moving samples between laboratories while complying with the Convention on the International Trade in Endangered Species (CITES), and other national and international permitting frameworks.
Genetic methods have a strong history of identifying and resolving species identification questions in beaked whales. The continuing development of more cost-effective and high throughput genomic sequencing techniques means that this tradition is likely to continue with more powerful tools at our disposal. Much of the work done to date has been based on short fragments of the mtDNA control region, but targeted capture techniques mean that it is possible to cost-effectively select and sequence the whole mtDNA genome from multiple individuals (Hancock-Hanser et al., 2013). The increased data of the whole mtDNA genome provides more genetic variation and resolution of both phylogenetic relationships and population structure. For example, preliminary work using whole mitochondrial genomes has suggested complex evolutionary histories and/or patterns of contemporary gene flow in Blainville’s, Gervais’ (M. europaeus) and Cuvier’s beaked whales (Morin et al., 2012).
The focus on mtDNA in much of beaked whale phylogeny has been due to necessity, in that it is easier to obtain from bone samples than nuclear DNA markers, as well as usefulness: the higher rate of genetic drift in mtDNA means that recently diverged species may be monophyletic for mtDNA haplotypes but not nuclear DNA loci (see Discussion in Dalebout et al., 2004). However, nuclear DNA markers are necessary to investigate hybridization and introgression at the species level, and for assessing bi-parental gene flow, kinship and, ideally, structure at the population level. Whole genome sequencing is becoming increasingly cost-effective and technological developments mean it is possible to get genome level data from well preserved bone samples, for example, type or ancient specimens (e.g., Dalebout et al., 2003).
Methods of studying genomic-level information in a species or population include reduced representation approaches and whole genome resequencing projects (see Cammen et al., 2016). The usefulness of these methods is highlighted by a pilot study in Blainville’s and Cuvier’s that produced 10,000 variable loci using a restriction site associated DNA sequencing approach (Peterson et al., 2012; Carroll et al., 2016). In addition to questions on species identity, genetic diversity, demographic history and population structure, such data will permit the investigation of key questions in beaked whale evolution, such as the genomic architecture of adaptation to deep diving (e.g., using genome scan methods, de Villemereuil et al., 2014). Understanding patterns of species radiation and population expansion in relation to historical climate processes using genomic methods could also allow predictions of changes in beaked whale populations under future climate change scenarios.
Additionally, once a beaked whale reference genome is available, more cost-effective and reproducible methods of producing DNA profiles can be developed, including single nucleotide polymorphism (SNP) genotyping assays and targeted capture approaches (see Carroll et al., 2018). This would allow effective long-term genetic monitoring projects to determine the relatedness and connectivity of individuals within and between study sites in a consistent and comparable way.
Further Quantify Impact of Sound
Understanding how beaked whales are affected by underwater noise in general is vital, as human activity within beaked whale habitats is likely to continue to increase, particularly in high-latitude habitats (Reeves et al., 2014). A number of recent behavioral response studies (see Southall et al., 2016; Harris et al., 2018) have indicated the generally strong responses of beaked whales to sonar, typically showing a combination of avoidance and cessation of feeding, with these responses to simulated sonar starting at low received levels. However, variation in responses between and within individuals and populations suggests that context may play a role in modulating the dose-response relationship (Harris et al., 2018). Indeed, Cuvier’s beaked whales responses were more pronounced for lower-source level dipping helicopter-based military sonar than for the higher-power ship sonar, suggesting that sonar type may be more important than sound levels (Falcone et al., 2017).
Most of the information on beaked whale responses to sonar has been collected in areas on, or adjacent to, US Naval facilities. Animals resident in those areas may not be typical of beaked whales in the rest of the world’s oceans. A single experiment with northern bottlenose whales in the relatively pristine water off Jan Mayen showed a clear and strong behavioral response of extended avoidance and cessation of foraging by a tagged whale and other whales nearby (Miller et al., 2015; Table 2). While the overall characteristics of the response was highly similar, the severity of the response observed appeared stronger than has been reported for on-range experiments on other beaked whales, possibly indicating that beaked whales near navy ranges have learned to tolerate these sounds.
Compared to the level of information about effects of sonar on beaked whales, there are few studies of the effect of other noise sources (but see - Aguilar de Soto et al., 2006; Cholewiak et al., 2017). Seismic surveys occur frequently in many parts of the world and seismic pulses are a persistent component of background noise in some ocean basins (Nieukirk et al., 2012). Additional observations, experiments, and evaluation of how beaked whales respond to a wider range of noise sources in diverse habitats would further improve our understanding of the impacts of this form of habitat degradation.
The observed reaction of beaked whales to stressors such as noise (Table 2) highlights the potential for anthropogenic impact on individuals. However, the population level impact of these stressors is less clear. There has been a good deal of work on this issue (for recent review, see Pirotta et al., 2018). Bioenergetics models suggest that beaked whales require relatively high-quality habitat to meet their energy requirements (New et al., 2013), and that regular displacement from preferred feeding habitats (such as caused by exposure to anthropogenic sound sources) could impact survival and reproduction through compromised body condition.
The specificity of beaked whale target prey may also affect energetic costs when they are displaced and move toward new prey patches. The energy landscapes that animals are confronted with (Shepard et al., 2013) may detrimentally impact animals that are startled into routes they may not otherwise choose to take. This may be of particular concern for pregnant and lactating females that have increased energetic demands and may have to rely on endogenous nutrient reserves to support any increased energy expenditure (Christiansen et al., 2016). Recent observations of low reproductive success of M. densirostris at the Bahamas AUTEC range may indicate the population consequence of repeated disturbances (McCarthy et al., 2011; Tyack et al., 2011; Claridge, 2013). The collection of empirical data on female body condition, growth of calves and natural calf mortality is vital, and would allow a direct assessment of whether nutritional stress from disturbance is a realistic mechanism for explaining apparent population responses.
Develop Mitigation for Anthropogenic Impacts
Beaked whales face significant threats. Their atypical mass-strandings in the face of sonar exposure have showcased the vulnerability of these species, and have focused attention on anthropogenic threats such as noise, fishery interactions and shipping. These types of threats can be thought of as discrete events in space and time, the reduction of which will reduce the potential for harm to animals. To mitigate these, increased understanding of temporal and spatial distribution of beaked whales will enable more effective planning for noise-generating activities to occur where and when fewer sensitive animals are present.
For known resident populations that are not regularly exposed to sonar, exclusion of naval sonars may be the best option. In the Canary Islands, after several mass-strandings coinciding with naval activity, a moratorium was established in 2004 excluding naval sonar operation within 50 nm of the islands (Aguilar de Soto et al., 2016). This has resulted in no further atypical mass strandings, and should be considered for other known beaked-whale hotspots.
In other areas, better real-time detection mechanisms for animals may help, so that noise sources can be reduced or stopped when in the presence of animals likely to be detrimentally affected. However, this is particularly difficult for beaked whales which have a low availability for visual detection. Although beaked whales are only vocal for 20% of the time (Arranz et al., 2011; Barlow et al., 2013), they are vocally active for two or three times as long as they are visually detectable (Aguilar de Soto et al., 2012), meaning that passive acoustic monitoring may be the best way forward for beaked whale detection and subsequent mitigation efforts. The characterization of beaked whale clicks (Johnson et al., 2004; Zimmer et al., 2005; Gillespie et al., 2009; Baumann-Pickering et al., 2013) and advances in automated classification methods to identify such clicks to species level, may help (Aguilar de Soto et al., 2016).
However, many other threats are more global in nature – including climate change, fisheries entanglement, ocean contaminants, and plastic ingestion. Climate change is likely to cause shifts in prey ranges either latitudinally or vertically within the water column resulting in physiological impacts on these whales. Entanglement with fishing gear has been noted in longline fisheries and monofiliament line (Gowans et al., 2000b; Garrison, 2007), but the scale of this issue is not known. Given their teuthivorous diet, it is also likely that beaked whales would ingest marine plastics (Lusher et al., 2015) and face risks from these, as well as from other ocean contaminants (Hooker et al., 2008). Mitigation for these global threats is far more problematic and is likely to require high-level governmental policy and/or societal change in order to reduce impacts at source. Our lack of knowledge about beaked whale populations means that even monitoring the effects of these individual factors is difficult. Yet threats are rarely isolated, and consideration of cumulative impacts is a challenging new research priority (National Academies of Sciences Engineering and Medicine, 2017).
We hope to see many of these research topics pursued in the next decades. With multiple studies taking place for different species and for populations in different ocean basins and in different habitats (e.g., insular, pelagic and continental in the case of Cuvier’s beaked whales), comparative studies will also begin to play an important role. These investigations will enhance our understanding of beaked whales generally, and by inference the behavior of less-well studied species. This collaborative effort is critical if we are to understand how changing oceans will impact the distribution of beaked whales and their prey.
Author Contributions
All authors listed have made a substantial, direct and intellectual contribution to the work, and approved it for publication.
Conflict of Interest Statement
The authors declare that the research was conducted in the absence of any commercial or financial relationships that could be construed as a potential conflict of interest.
Footnotes
- ^ This was the case for example in Hawai‘i, where Dan McSweeney began taking opportunistic photos of both Blainville’s and Cuvier’s beaked whales in the late 1980s, although a photo-identification catalog was not actually established until the early 2000s as other research on those populations began in the area. The use of these opportunistic photos effectively added 15 years to the photo-identification study.
References
Abecassis, M., Polovina, J., Baird, R. W., Copeland, A., Drazen, J. C., Domokos, R., et al. (2015). Characterizing a foraging hotspot for short-finned pilot whales and Blainville’s beaked whales located off the west side of Hawai’i Island by using tagging and oceanographic data. PLoS One 10:22. doi: 10.1371/journal.pone.0142628
Acevedo-Whitehouse, K., Rocha-Gosselin, A., and Gendron, D. (2010). A novel non-invasive tool for disease surveillance of free-ranging whales and its relevance to conservation programs. Anim. Conserv. 13, 217–225. doi: 10.1111/j.1469-1795.2009.00326.x
Aguilar de Soto, N., Gkikopoulou, K. C., Hooker, S., Isojunno, S., Johnson, M., Miller, P., et al. (2016). From physiology to policy: a review of physiological noise effects on marine fauna with implications for mitigation. Proc. Meet. Acoust. 27:040008. doi: 10.1121/2.0000299
Aguilar de Soto, N., Johnson, M., Madsen, P. T., Tyack, P. L., Bocconcelli, A., and Borsani, J. F. (2006). Does intense ship noise disrupt foraging in deep-diving Cuvier’s beaked whales (Ziphius cavirostris)? Mar. Mamm. Sci. 22, 690–699. doi: 10.1111/j.1748-7692.2006.00044.x
Aguilar de Soto, N., Madsen, P. T., Tyack, P., Arranz, P., Marrero, J., Fais, A., et al. (2012). No shallow talk: cryptic strategy in the vocal communication of Blainville’s beaked whales. Mar. Mamm. Sci. 28, E75–E92. doi: 10.1111/j.1748-7692.2011.00495.x
Aguilar de Soto, N., Martin, V., Silva, M., Edler, R., Reyes, C., Carrillo, M., et al. (2017). True’s beaked whale (Mesoplodon mirus) in Macaronesia. PeerJ 5:22. doi: 10.7717/peerj.3059
Amato, K. R., Yeoman, C. J., Kent, A., Righini, N., Carbonero, F., Estrada, A., et al. (2013). Habitat degradation impacts black howler monkey (Alouatta pigra) gastrointestinal microbiomes. ISME J. 7, 1344–1353. doi: 10.1038/ismej.2013.16
Apprill, A., Miller, C. A., Moore, M. J., Durban, J. W., Fearnbach, H., and Barrett-Lennard, L. G. (2017). Extensive core microbiome in drone-captured whale blow supports a framework for health monitoring. mSystems 2:15. doi: 10.1128/mSystems.00119-17
Arranz, P., Borchers, D. L., De Soto, N. A., Johnson, M. P., and Cox, M. J. (2014). A new method to study inshore whale cue distribution from land-based observations. Mar. Mamm. Sci. 30, 810–818. doi: 10.1111/mms.12077
Arranz, P., De Soto, N. A., Madsen, P. T., Brito, A., Bordes, F., and Johnson, M. P. (2011). Following a foraging fish-finder: diel habitat use of Blainville’s beaked whales revealed by echolocation. PLoS One 6:10. doi: 10.1371/journal.pone.0028353
Baird, R. W. (2019). “Behavior and ecology of not-so-social odontocetes: cuvier’s and blainville’s beaked whales,” in Ethology and Behavioral Ecology of Toothed Whales and Dolphins, the Odontocetes, ed. B. Wursig (Berlin: Springer).
Baird, R. W., Schorr, G. S., Webster, D. L., Mahaffy, S. D., Mcsweeney, D. J., Hanson, M. B., et al. (2011). Open-ocean movements of a satellite-tagged Blainville’s beaked whale (Mesoplodon densirostris): evidence for an offshore population in Hawai’i? Aquat. Mamm. 37, 506–511. doi: 10.1578/AM.37.4.2011.506
Baird, R. W., Webster, D. L., Mcsweeney, D. J., Ligon, A. D., Schorr, G. S., and Barlow, J. (2006). Diving behaviour of Cuvier’s (Ziphius cavirostris) and Blainville’s (Mesoplodon densirostris) beaked whales in Hawai’i. Can. J. Zool. 84, 1120–1128. doi: 10.1139/z06-095
Baird, R. W., Webster, D. L., Schorr, G. S., Mcsweeney, D. J., and Barlow, J. (2008). Diel variation in beaked whale diving behavior. Mar. Mamm. Sci. 24, 630–642. doi: 10.1371/journal.pone.0092633
Baker, C. S., Steel, D., Nieukirk, S., and Klinck, H. (2018). Environmental DNA (eDNA) from the wake of the whales: droplet digital PCR for detection and species identification. Front. Mar. Sci. 5:133. doi: 10.3389/fmars.2018.00133
Barlow, J. (2015). Inferring trackline detection probabilities, g(0), for cetaceans from apparent densities in different survey conditions. Mar. Mamm. Sci. 31, 923–943. doi: 10.1111/mms.12205
Barlow, J., Tyack, P. L., Johnson, M. P., Baird, R. W., Schorr, G. S., Andrews, R. D., et al. (2013). Trackline and point detection probabilities for acoustic surveys of Cuvier’s and Blainville’s beaked whales. J. Acoust. Soc. Am. 134, 2486–2496. doi: 10.1121/1.4816573
Baumann-Pickering, S., Mcdonald, M. A., Simonis, A. E., Berga, A. S., Merkens, K. P. B., Oleson, E. M., et al. (2013). Species-specific beaked whale echolocation signals. J. Acoust. Soc. Am. 134, 2293–2301. doi: 10.1121/1.4817832
Baumann-Pickering, S., Roch, M. A., Brownell, R. L., Simonis, A. E., Mcdonald, M. A., Solsona-Berga, A., et al. (2014). Spatio-temporal patterns of beaked whale echolocation signals in the North Pacific. PLoS One 9:17. doi: 10.1371/journal.pone.0086072
Benjaminsen, T. (1972). On the biology of the bottlenose whale, Hyperoodon ampullatus (Forster). Norwegian J. Zool. 20, 233–241.
Biuw, M., Mcconnell, B. J., Bradshaw, C. J. A., Burton, H., and Fedak, M. (2003). Blubber and buoyancy: monitoring the body condition of free-ranging seals using simple dive characteristics. J. Exp. Biol. 206, 3405–3423. doi: 10.1242/jeb.00583
Cammen, K. M., Andrews, K. R., Carroll, E. L., Foote, A. D., Humble, E., Khudyakov, J. I., et al. (2016). Genomic methods take the plunge: recent advances in high-throughput sequencing of marine mammals. J. Heredity 107, 481–495. doi: 10.1093/jhered/esw044
Canadas, A. (2012). Ziphius cavirostris (Mediterranean subpopulation), Cuvier’s Beaked Whale. Gland: The IUCN Red List of Threatened Species.
Cárdenas-Hinojosa, G., Hoyos-Padilla, M., and Rojas-Bracho, L. (2015). Occurrence of Cuvier’s beaked whales (Ziphius cavirostris) at Guadalupe Island, Mexico, from 2006 through 2009. Latin Am. J. Aquat. Mamm. 10, 38–47. doi: 10.5597/lajam00192
Carroll, E. L., Bruford, M. W., Dewoody, J. A., Leroy, G., Strand, A., Waits, L., et al. (2018). Genetic and genomic monitoring with minimally invasive sampling methods. Evol Appl. 11:7. doi: 10.1111/eva.12600
Carroll, E. L., Childerhouse, S. J., Fewster, R. M., Patenaude, N. J., Steel, D., Dunshea, G., et al. (2013). Accounting for female reproductive cycles in a superpopulation capture-recapture framework. Ecol. Appl. 23, 1677–1690. doi: 10.1890/12-1657.1
Carroll, E. L., Reyes, C., Gaggiotti, O. E., Olsen, M. T., Maaholm, D. J., and Rosso, M. (2016). “Pilot study to assess the utility of ddRAD sequencing in identifying species-specific and shared SNPs among Blainville’s (Mesoplodon densirostris) and Cuvier’s (Ziphius cavirostris) beaked whales,” in Report SC/66b/DNA/03 Presented to the Scientific Committee, (London: International Whaling Commission).
Cholewiak, D., Deangelis, A. I., Palka, D., Corkeron, P. J., and Van Parijs, S. M. (2017). Beaked whales demonstrate a marked acoustic response to the use of shipboard echosounders. R. Soc. Open Sci. 4:15. doi: 10.1098/rsos.170940
Christiansen, F., Dujon, A. M., Sprogis, K. R., Arnould, J. P. Y., and Bejder, L. (2016). Noninvasive unmanned aerial vehicle provides estimates of the energetic cost of reproduction in humpback whales. Ecosphere 7:18. doi: 10.1002/ecs2.1468
Claridge, D. E. (2006). Fine-Scale Distribution and Habitat Selection of Beaked Whales. Master thesis, Aberdeen University, Aberdeen.
Claridge, D. E. (2013). Population Ecology of Blainville’s Beaked Whales (Mesoplodon densirostris). Ph.D. thesis, University of St Andrews, St Andrews.
Coomber, F., Moulins, A., Tepsich, P., and Rosso, M. (2016). Sexing free-ranging adult Cuvier’s beaked whales (Ziphius cavirostris) using natural marking thresholds and pigmentation patterns. J. Mammal. 97, 879–890. doi: 10.1093/jmammal/gyw033
Cox, T. M., Ragen, T. J., Read, A. J., Vos, E., Baird, R. W., Balcomb, K., et al. (2006). Understanding the impacts of anthropogenic sound on beaked whales. J. Cetacean Res. Manag. 7, 177–187.
Dalebout, M. L., Baker, C. S., Mead, J. G., Cockcroft, V. G., and Yamada, T. K. (2004). A comprehensive and validated molecular taxonomy of beaked whales, family Ziphiidae. J. Heredity 95, 459–473. doi: 10.1093/jhered/esh054
Dalebout, M. L., Baker, C. S., Steel, D., Thompson, K., Robertson, K. M., Chivers, S. J., et al. (2014). Resurrection of Mesoplodon hotaula deraniyagala 1963: a new species of beaked whale in the tropical Indo-Pacific. Mar. Mamm. Sci. 30, 1081–1108. doi: 10.1111/mms.12113
Dalebout, M. L., Hooker, S. K., and Christensen, I. (2001). Genetic diversity and population structure among northern bottlenose whales, Hyperoodon ampullatus, in the western North Atlantic Ocean. Can. J. Zool. 79, 478–484. doi: 10.1139/z01-005
Dalebout, M. L., Mead, J. G., Baker, C. S., Baker, A. N., and Van Helden, A. L. (2002). A new species of beaked whale Mesoplodon perrini sp. N. (Cetacea: Ziphiidae) discovered through phylogenetic analyses of mitochondrial DNA sequences. Mar. Mamm. Sci. 18, 577–608. doi: 10.1111/j.1748-7692.2002.tb01061.x
Dalebout, M. L., Robertson, K. M., Frantzis, A., Engelhaupt, D., Mignucci-Giannoni, A. A., Rosario-Delestre, R. J., et al. (2005). Worldwide structure of mtDNA diversity among Cuvier’s beaked whales (Ziphius cavirostris): implications for threatened populations. Mol. Ecol. 14, 3353–3371. doi: 10.1111/j.1365-294X.2005.02676.x
Dalebout, M. L., Ross, G. J. B., Baker, C. S., Anderson, R. C., Best, P. B., Cockcroft, V. G., et al. (2003). Appearance, distribution, and genetic distinctiveness of Longman’s beaked whale, Indopacetus pacificus. Mar. Mamm. Sci. 19, 421–461. doi: 10.1111/j.1748-7692.2003.tb01314.x
Dalebout, M. L., Ruzzante, D. E., Whitehead, H., and Oien, N. I. (2006). Nuclear and mitochondrial markers reveal distinctiveness of a small population of bottlenose whales (Hyperoodon ampullatus) in the western North Atlantic. Mol. Ecol. 15, 3115–3129. doi: 10.1111/j.1365-294X.2006.03004.x
D’amico, A., Gisiner, R. C., Ketten, D. R., Hammock, J. A., Johnson, C., Tyack, P. L., et al. (2009). Beaked whale strandings and naval exercises. Aquat. Mamm. 35, 452–472. doi: 10.1578/AM.35.4.2009.452
de Villemereuil, P., Frichot, E., Bazin, E., Francois, O., and Gaggiotti, O. E. (2014). Genome scan methods against more complex models: when and how much should we trust them? Mol. Ecol. 23, 2006–2019. doi: 10.1111/mec.12705
DeAngelis, A. I., Stanistreet, J. E., Baumann-Pickering, S., and Cholewiak, D. M. (2018). A description of echolocation clicks recorded in the presence of True’s beaked whale (Mesoplodon mirus). J. Acoust. Soc. Am. 144, 2691–2700. doi: 10.1121/1.5067379
DeRuiter, S. L., Southall, B. L., Calambokidis, J., Zimmer, W. M. X., Sadykova, D., Falcone, E. A., et al. (2013). First direct measurements of behavioural responses by Cuvier’s beaked whales to mid-frequency active sonar. Biol. Lett. 9, 1–5. doi: 10.1098/rsbl.2013.0223
Dunn, B. P. (2015). Insights into Blainville’s Beaked Whale (Mesoplodon densirostris) Communication. Ph.D. thesis, University of St Andrews, St. Andrews.
Dunn, C., Claridge, D., Durban, J., Shaffer, J., Moretti, D., Tyack, P., et al. (2017). Insights into Blainville’s beaked whale (Mesoplodon densirostris) echolocation ontogeny from recordings of mother-calf pairs. Mar. Mamm. Sci. 33, 356–364. doi: 10.1111/mms.12351
Dunn, C., Hickmott, L., Talbot, D., Boyd, I., and Rendell, L. (2013). Mid-frequency broadband sounds of Blainville’s beaked whales. Bioacoustics 22, 153–163. doi: 10.1080/09524622.2012.757540
Fahlman, A., Tyack, P. L., Miller, P. J. O., and Kvadsheim, P. H. (2014). How man-made interference might cause gas bubble emboli in deep diving whales. Front. Physiol. 5:13. doi: 10.3389/fphys.2014.00013
Falcone, E. A., Schorr, G. S., Douglas, A. B., Calambokidis, J., Henderson, E., Mckenna, M. F., et al. (2009). Sighting characteristics and photo-identification of Cuvier’s beaked whales (Ziphius cavirostris) near San Clemente Island, California: a key area for beaked whales and the military? Mar. Biol. 156, 2631–2640. doi: 10.1007/s00227-009-1289-8
Falcone, E. A., Schorr, G. S., Watwood, S. L., Deruiter, S. L., Zerbini, A. N., Andrews, R. D., et al. (2017). Diving behaviour of Cuvier’s beaked whales exposed to two types of military sonar. R. Soc. Open Sci. 4:21. doi: 10.1098/rsos.170629
Fearnbach, H., Durban, J. W., Ellifrit, D. K., and Balcomb, K. C. (2018). Using aerial photogrammetry to detect changes in body condition of endangered southern resident killer whales. Endanger. Species Res. 35, 175–180. doi: 10.3354/esr00883
Fedutin, I. D., Filatova, O. A., Mamaev, E. G., Burdin, A. M., and Hoyt, E. (2015). Occurrence and social structure of Baird’s beaked whales, Berardius bairdii, in the Commander Islands, Russia. Mar. Mamm. Sci. 31, 853–865. doi: 10.1111/mms.12204
Fernandez, A., Edwards, J. F., Rodriguez, F., Espinosa De Los Monteros, A., Herraez, P., Castro, P., et al. (2005). “Gas and fat embolic syndrome” involving a mass stranding of beaked whales (family Ziphiidae) exposed to anthropogenic sonar signals. Vet. Pathol. 42, 446–457. doi: 10.1354/vp.42-4-446
Fernandez, R., Pierce, G. J., Macleod, C. D., Brownlow, A., Reid, R. J., Rogan, E., et al. (2014). Strandings of northern bottlenose whales, Hyperoodon ampullatus, in the north-east Atlantic: seasonality and diet. J. Mar. Biol. Assoc. U.K. 94, 1109–1116. doi: 10.1017/S002531541300180X
Filadelfo, R., Mintz, J., Michlovich, E., D’amico, A., Tyack, P. L., and Ketten, D. R. (2009). Correlating military sonar use with beaked whale mass strandings: what do the historical data show? Aquat. Mamm. 35, 435–444. doi: 10.1578/AM.35.4.2009.435
Foote, A. D., Thomsen, P. F., Sveegaard, S., Wahlberg, M., Kielgast, J., Kyhn, L. A., et al. (2012). Investigating the potential use of environmental DNA (eDNA) for genetic monitoring of marine mammals. PLoS One 7:6. doi: 10.1371/journal.pone.0041781
Forney, K. A., Southall, B. L., Slooten, E., Dawson, S., Read, A. J., Baird, R. W., et al. (2017). Nowhere to go: noise impact assessments for marine mammal populations with high site fidelity. Endanger. Species Res. 32, 391–413. doi: 10.3354/esr00820
Garrison, L. P. (2007). Interactions between marine mammals and pelagic longline fishing gear in the U.S. Atlantic Ocean between 1992 and 2004. Fish. Bull. 105, 408–417.
Gillespie, D., Dunn, C., Gordon, J., Claridge, D., Embling, C., and Boyd, I. (2009). Field recordings of Gervais’ beaked whales Mesoplodon europaeus from the Bahamas. J. Acoust. Soc. Am. 125, 3428–3433. doi: 10.1121/1.3110832
Gowans, S., Dalebout, M. L., Hooker, S. K., and Whitehead, H. (2000a). Reliability of photographic and molecular techniques for sexing northern bottlenose whales (Hyperoodon ampullatus). Can. J. Zool. 78, 1224–1229. doi: 10.1139/z00-062
Gowans, S., Whitehead, H., Arch, J. K., and Hooker, S. K. (2000b). Population size and residency patterns of northern bottlenose whales (Hyperoodon ampullatus) using the Gully, Nova Scotia. J. Cetacean Res. Manage. 2, 201–210.
Gowans, S., and Rendell, L. (1999). Head-butting in northern bottlenose whales (Hyperoodon ampullatus): a possible function for big heads? Mar. Mamm. Sci. 15, 1342–1350. doi: 10.1111/j.1748-7692.1999.tb00896.x
Gowans, S., and Whitehead, H. (2001). Photographic identification of northern bottlenose whales (Hyperoodon ampullatus): sources of heterogeneity from natural marks. Mar. Mamm. Sci. 17, 76–93. doi: 10.1111/j.1748-7692.2001.tb00981.x
Gowans, S., Whitehead, H., and Hooker, S. K. (2001). Social organization in northern bottlenose whales (Hyperoodon ampullatus): not driven by deep-water foraging. Anim. Behav. 62, 369–377. doi: 10.1006/anbe.2001.1756
Gray, D. (1882). Notes on the characteristics and habits of the bottlenose whale (Hyperoodon rostratus). Proc. Endanger. Species Res. Zool. Soc. Lond. 1882, 726–731.
Griffiths, E. T., and Barlow, J. (2016). Cetacean acoustic detections from free-floating vertical hydrophone arrays in the southern California Current. J. Acoust. Soc. Am. 140:EL399. doi: 10.1121/1.4967012
Hancock-Hanser, B. L., Frey, A., Leslie, M. S., Dutton, P. H., Archer, F. I., and Morin, P. A. (2013). Targeted multiplex next-generation sequencing: advances in techniques of mitochondrial and nuclear DNA sequencing for population genomics. Mol. Ecol. Resour. 13, 254–268. doi: 10.1111/1755-0998.12059
Hardy, N., Berry, T., Kelaher, B. P., Goldsworthy, S. D., Bunce, M., Coleman, M. A., et al. (2017). Assessing the trophic ecology of top predators across a recolonisation frontier using DNA metabarcoding of diets. Mar. Ecol. Prog. Ser. 573, 237–254. doi: 10.3354/meps12165
Harris, C. M., Thomas, L., Falcone, E. A., Hildebrand, J., Houser, D., Kvadsheim, P. H., et al. (2018). Marine mammals and sonar: dose-response studies, the risk-disturbance hypothesis and the role of exposure context. J. Appl. Ecol. 55, 396–404. doi: 10.1111/1365-2664.12955
Henderson, E. E., Martin, S. W., Manzano-Roth, R., and Matsuyama, B. M. (2016). Occurrence and habitat use of foraging Blainville’s beaked whales (Mesoplodon densirostris) on a US Navy Range in Hawaii. Aquat. Mamm. 42, 549–562. doi: 10.1578/AM.42.4.2016.549
Hooker, S. K., and Baird, R. W. (1999). Deep-diving behaviour of the northern bottlenose whale, Hyperoodon ampullatus (Cetacea: Ziphiidae). Proc. R. Soc. B Biol. Sci. 266, 671–676. doi: 10.1098/rspb.1999.0688
Hooker, S. K., Baird, R. W., and Fahlman, A. (2009). Could beaked whales get the bends? Effect of diving behaviour and physiology on modelled gas exchange for three species: Ziphius cavirostris, Mesoplodon densirostris and Hyperoodon ampullatus. Respir. Physiol. Neurobiol. 167, 235–246. doi: 10.1016/j.resp.2009.04.023
Hooker, S. K., Fahlman, A., Moore, M. J., De Soto, N. A., De Quiros, Y. B., Brubakk, A. O., et al. (2012). Deadly diving? Physiological and behavioural management of decompression stress in diving mammals. Proc. R. Soc. B Biol. Sci. 279, 1041–1050. doi: 10.1098/rspb.2011.2088
Hooker, S. K., Iverson, S. J., Ostrom, P., and Smith, S. C. (2001). Diet of northern bottlenose whales inferred from fatty-acid and stable-isotope analyses of biopsy samples. Can. J. Zool. 79, 1442–1454. doi: 10.1139/z01-096
Hooker, S. K., Metcalfe, T. L., Metcalfe, C. D., Angell, C. M., Wilson, J. Y., Moore, M. J., et al. (2008). Changes in persistent contaminant concentration and CYP1A1 protein expression in biopsy samples from northern bottlenose whales, Hyperoodon ampullatus, following the onset of nearby oil and gas development. Environ. Pollut. 152, 205–216. doi: 10.1016/j.envpol.2007.05.027
Hooker, S. K., and Whitehead, H. (2002). Click characteristics of northern bottlenose whales (Hyperoodon ampullatus). Mar. Mamm. Sci. 18, 69–80. doi: 10.1111/j.1748-7692.2002.tb01019.x
Horrocks, J., Hamilton, D. C., and Whitehead, H. (2011). A likelihood approach to estimating animal density from binary acoustic transects. Biometrics 67, 681–690. doi: 10.1111/j.1541-0420.2010.01496.x
Hunt, K. E., Moore, M. J., Rolland, R. M., Kellar, N. M., Hall, A. J., Kershaw, J., et al. (2013). Overcoming the challenges of studying conservation physiology in large whales: a review of available methods. Conserv. Physiol. 1:cot006. doi: 10.1093/conphys/cot006
Isojunno, S., Aoki, K., Curé, C., Kvadsheim, P. H., and Miller, P. J. O. M. (2018). Breathing patterns indicate cost of exercise during diving and response to experimental sound exposures in long-finned pilot whales. Front. Physiol. 9:1462. doi: 10.3389/fphys.2018.01462
Jarman, S. N., Polanowski, A. M., Faux, C. E., Robbins, J., De Paoli-Iseppi, R., Bravington, M., et al. (2015). Molecular biomarkers for chronological age in animal ecology. Mol. Ecol. 24, 4826–4847. doi: 10.1111/mec.13357
Johnson, M., Madsen, P. T., Zimmer, W. M. X., Aguilar De Soto, N., and Tyack, P. L. (2004). Beaked whales echolocate on prey. Proc. R. Soc. Lond. B Biol.Sci. 271, S383–S386. doi: 10.1098/rsbl.2004.0208
Johnson, M., Madsen, P. T., Zimmer, W. M. X., De Soto, N. A., and Tyack, P. L. (2006). Foraging Blainville’s beaked whales (Mesoplodon densirostris) produce distinct click types matched to different phases of echolocation. J. Exp. Biol. 209, 5038–5050. doi: 10.1242/jeb.02596
Johnson, M., and Tyack, P. L. (2003). A digital acoustic recording tag for measuring the response of wild marine mammals to sound. IEEE J. Ocean. Eng. 28, 3–12. doi: 10.1109/JOE.2002.808212
Joyce, T. W., Durban, J. W., Claridge, D. E., Dunn, C. A., Fearnbach, H., Parsons, K. M., et al. (2017). Physiological, morphological, and ecological tradeoffs influence vertical habitat use of deep-diving toothed-whales in the Bahamas. PLoS One 12:27. doi: 10.1371/journal.pone.0185113
Joyce, T. W., Durban, J. W., Fearnbach, H., Claridge, D., and Ballance, L. T. (2016). Use of time-at-temperature data to describe dive behavior in five species of sympatric deep-diving toothed whales. Mar. Mamm. Sci. 32, 1044–1071. doi: 10.1111/mms.12323
Kasuya, T. (1977). Age determination and growth of the Baird’s beaked whale with a comment on the fetal growth rate. Sci. Rep. Whales Res. Inst. 29, 1–20.
Kasuya, T. (1986). Distribution and behaviour of Baird’s beaked whales off the Pacific coast of Japan. Sci. Rep. Whales Res. Inst. 37, 61–83.
Klinck, H., Mellinger, D. K., Klinck, K., Bogue, N. M., Luby, J. C., Jump, W. A., et al. (2012). Near-real-time acoustic monitoring of beaked whales and other cetaceans using a seaglider (TM). PLoS One 7:8. doi: 10.1371/journal.pone.0036128
Koopman, H. N. (2007). Phylogenetic, ecological, and ontogenetic factors influencing the biochemical structure of the blubber of odontocetes. Mar. Biol. 151, 277–291. doi: 10.1007/s00227-006-0489-8
Koopman, H. N., and Westgate, A. J. (2012). Solubility of nitrogen in marine mammal blubber depends on its lipid composition. J. Exp. Biol. 215, 3856–3863. doi: 10.1242/jeb.074443
Kowarski, K., Delarue, J., Martin, B., O’brien, J., Meade, R., Cadhla, O. O., et al. (2018). Signals from the deep: spatial and temporal acoustic occurrence of beaked whales off western Ireland. PLoS One 13:e0199431. doi: 10.1371/journal.pone.0199431
Laptikhovsky, V. V., Barrett, C. J., and Hollyman, P. R. (2018). From coral reefs to whale teeth: estimating mortality from natural accumulations of skeletal materials. Mar. Ecol. Prog. Ser. 598, 273–291. doi: 10.3354/meps12260
Lopez, L. M. M., Miller, P. J. O., De Soto, N. A., and Johnson, M. (2015). Gait switches in deep-diving beaked whales: biomechanical strategies for long-duration dives. J. Exp. Biol. 218, 1325–1338. doi: 10.1242/jeb.106013
Lusher, A. L., Hernandez-Milian, G., O’brien, J., Berrow, S., O’connor, I., and Officer, R. (2015). Microplastic and macroplastic ingestion by a deep diving, oceanic cetacean: The True’s beaked whale Mesoplodon mirus. Environ. Pollut. 199, 185–191. doi: 10.1016/j.envpol.2015.01.023
Lynn, S. K., and Reiss, D. L. (1992). Pulse sequence and whistle production by two captive beaked whales, Mesoplodon species. Mar. Mamm. Sci. 8, 299–305. doi: 10.1111/j.1748-7692.1992.tb00413.x
MacLeod, C. D., and Mitchell, G. (2006). Key areas for beaked whales worldwide. J. Cetacean Res. Manage. 7, 309–322.
MacLeod, C. D., Perrin, W. F., Pitman, R., Barlow, J., Ballance, L., D’amico, A., et al. (2006). Known and inferred distributions of beaked whale species (Cetacea: Ziphiidae). J. Cetacean Res. Manage. 7, 271–286.
Madsen, P. T., Johnson, M., Aguilar De Soto, N., Zimmer, W. M. X., and Tyack, P. (2005). Biosonar performance of foraging beaked whales (Mesoplodon densirostris). J. Exp. Biol. 208, 181–194. doi: 10.1242/jeb.01327
Marques, T. A., Thomas, L., Martin, S. W., Mellinger, D. K., Ward, J. A., Moretti, D. J., et al. (2013). Estimating animal population density using passive acoustics. Biol. Rev. 88, 287–309. doi: 10.1111/brv.12001
McCarthy, E., Moretti, D., Thomas, L., Dimarzio, N., Morrissey, R., Jarvis, S., et al. (2011). Changes in spatial and temporal distribution and vocal behavior of Blainville’s beaked whales (Mesoplodon densirostris) during multiship exercises with mid-frequency sonar. Mar. Mamm. Sci. 27, E206–E226. doi: 10.1111/j.1748-7692.2010.00457.x
McDonald, B. I., Johnson, M., and Madsen, P. T. (2018). Dive heart rate in harbour porpoises is influenced by exercise and expectations. J. Exp. Biol. 221:9. doi: 10.1242/jeb.168740
McLellan, W. A., Mcalarney, R. J., Cummings, E. W., Read, A. J., Paxton, C. G. M., Bell, J. T., et al. (2018). Distribution and abundance of beaked whales (Family Ziphiidae) off Cape Hatteras, North Carolina, U.S.A. Mar. Mamm. Sci. 34, 997–1017. doi: 10.1111/mms.12500
McSweeney, D. J., Baird, R. W., and Mahaffy, S. D. (2007). Site fidelity, associations, and movements of Cuvier’s (Ziphius cavirostris) and Blainville’s (Mesoplodon densirostris) beaked whales off the island of Hawai’i. Mar. Mamm. Sci. 23, 666–687. doi: 10.1111/j.1748-7692.2007.00135.x
Mead, J. G. (1984). Survey of Reproductive Data for the Beaked Whales (Ziphiidae). London: International Whaling Commission, 91–96.
Miller, C. A., Best, P. B., Perryman, W. L., Baumgartner, M. F., and Moore, M. J. (2012). Body shape changes associated with reproductive status, nutritive condition and growth in right whales Eubalaena glacialis and E. australis. Mar. Ecol. Prog. Ser. 459, 135–156. doi: 10.3354/meps09675
Miller, P., Narazaki, T., Isojunno, S., Aoki, K., Smout, S., and Sato, K. (2016). Body density and diving gas volume of the northern bottlenose whale (Hyperoodon ampullatus). J. Exp. Biol. 219, 2458–2468. doi: 10.1242/jeb.137349
Miller, P. J. O., Kvadsheim, P. H., Lam, F. P. A., Tyack, P. L., Cure, C., Deruiter, S. L., et al. (2015). First indications that northern bottlenose whales are sensitive to behavioural disturbance from anthropogenic noise. R. Soc. Open Sci. 2:140484. doi: 10.1098/rsos.140484
Minamikawa, S., Iwasaki, T., and Kishiro, T. (2007). Diving behaviour of a Baird’s beaked whale, Berardius bairdii, in the slope water region of the western North Pacific: first dive records using a data logger. Fish. Oceanogr. 16, 573–577. doi: 10.1111/j.1365-2419.2007.00456.x
Moore, M. J., and Zerbini, A. N. (2017). Dolphin blubber/axial muscle shear: implications for rigid transdermal intramuscular tracking tag trauma in whales. J. Exp. Biol. 220, 3717–3723. doi: 10.1242/jeb.165282
Moretti, D., Dimarzio, N., Morrissey, R., Ward, J., and Jarvis, S. (2006). “Estimating the density of Blainville’s beaked whale (Mesoplodon densirostris) in the Tongue of the Ocean (TOTO) using passive acoustics,” in Proceedings of the IEEE Conference Proceedings: Oceans 2006, Boston. doi: 10.1109/OCEANS.2006.307083
Moretti, D., Morrissey, R., Jarvis, S., and Shaffer, J. (2016). “Findings from US navy hydrophone ranges,” in Listening in the Ocean: New Discoveries and Insights on Marine Life from Autonomous Passive Acoustic Recorders, eds W. W. L. Au and M. O. Lammers (New York, NY: Springer), 239–256.
Moretti, D., Thomas, L., Marques, T., Harwood, J., Dilley, A., Neales, B., et al. (2014). A risk function for behavioral disruption of Blainville’s beaked whales (Mesoplodon densirostris) from mid-frequency active sonar. PLoS One 9:e85064. doi: 10.1371/journal.pone.0085064
Morin, P., Duchene, S., Lee, N., Durban, J., and Claridge, D. (2012). “Preliminary analysis of mitochondrial genome phylogeography of Blainville’s, Cuvier’s and Gervais’ beaked whales,” in Report SC/64/SM14 presented to the Scientific Committee of the International Whaling Commission, (Cambridge: International Whaling Commission).
Morin, P. A., Baker, C. S., Brewer, R. S., Burdin, A. M., Dalebout, M. L., Dines, J. P., et al. (2017). Genetic structure of the beaked whale genus Berardius in the North Pacific, with genetic evidence for a new species. Mar. Mamm. Sci. 33, 96–111. doi: 10.1111/mms.12345
National Academies of Sciences Engineering and Medicine (2017). Approaches to Understanding the Cumulative Effects of Stressors on Marine Mammals. Washington, DC: The National Academies Press.
New, L. F., Moretti, D. J., Hooker, S. K., Costa, D. P., and Simmons, S. E. (2013). Using energetic models to investigate the survival and reproduction of beaked whales (family Ziphiidae). PLoS One 8:14. doi: 10.1371/journal.pone.0068725
Nieukirk, S. L., Mellinger, D. K., Moore, S. E., Klinck, K., Dziak, R. P., and Goslin, J. (2012). Sounds from airguns and fin whales recorded in the mid-Atlantic Ocean, 1999–2009. J. Acoust. Soc. Am. 131, 1102–1112. doi: 10.1121/1.3672648
O’Brien, K., and Whitehead, H. (2013). Population analysis of endangered northern bottlenose whales on the scotian shelf seven years after the establishment of a marine protected area. Endanger. Species Res. 21, 273–284. doi: 10.3354/esr00533
Pabst, D. A., Mclellan, W. A., and Rommel, S. (2016). How to build a deep-diver: the extreme morphology of mesoplodonts. Integr. Comp. Biol. 56, 1337–1348. doi: 10.1093/icb/icw126
Patel, S., Thompson, K. F., Santure, A. W., Constantine, R., and Millar, C. D. (2017). Genetic kinship analyses reveal that Gray’s beaked whales strand in unrelated groups. J. Heredity 108, 456–461. doi: 10.1093/jhered/esx021
Peterson, B. K., Weber, J. N., Kay, E. H., Fisher, H. S., and Hoekstra, H. E. (2012). Double digest RADseq: an inexpensive method for de novo SNP discovery and genotyping in model and non-model species. PLoS One 7:e37135. doi: 10.1371/journal.pone.0037135
Pirotta, E., Booth, C. G., Costa, D. P., Fleishman, E., Kraus, S. D., Lusseau, D., et al. (2018). Understanding the population consequences of disturbance. Ecol. Evol. 8, 9934–9946. doi: 10.1002/ece3.4458
Podesta, M., Azzellino, A., Canadas, A., Frantzis, A., Moulins, A., Rosso, M., et al. (2016). “Cuvier’s beaked whale, Ziphius cavirostris, distribution and occurrence in the Mediterranean Sea: high-use areas and conservation threats,” in Advances in Marine Biology, Vol 75: Mediterranean Marine Mammal Ecology and Conservation, eds G. N. Disciara, M. Podesta, and B. E. Curry (San Diego, CA: Elsevier Academic Press Inc.), 103–140.
Polanowski, A. M., Robbins, J., Chandler, D., and Jarman, S. N. (2014). Epigenetic estimation of age in humpback whales. Mol. Ecol. Resour. 14, 976–987. doi: 10.1111/1755-0998.12247
Raverty, S. A., Rhodes, L. D., Zabek, E., Eshghi, A., Cameron, C. E., Hanson, M. B., et al. (2017). Respiratory microbiome of endangered southern resident killer whales and microbiota of surrounding sea surface microlayer in the Eastern North Pacific. Sci. Rep. 7:12. doi: 10.1038/s41598-017-00457-5
Rees, H. C., Maddison, B. C., Middleditch, D. J., Patmore, J. R. M., and Gough, K. C. (2014). Review the detection of aquatic animal species using environmental DNA – a review of eDNA as a survey tool in ecology. J. Appl. Ecol. 51, 1450–1459. doi: 10.1111/1365-2664.12306
Reeves, R. R., Ewins, P. J., Agbayani, S., Heide-Jorgensen, M. P., Kovacs, K. M., Lydersen, C., et al. (2014). Distribution of endemic cetaceans in relation to hydrocarbon development and commercial shipping in a warming Arctic. Mar. Pol. 44, 375–389. doi: 10.1016/j.marpol.2013.10.005
Riccialdelli, L., Viola, M. N. P., Panarello, H. O., and Goodall, R. N. P. (2017). Evaluating the isotopic niche of beaked whales from the southwestern South Atlantic and Southern Oceans. Mar. Ecol. Prog. Ser. 581, 183–198. doi: 10.3354/meps12296
Ridgway, S. H., and Harrison, R. (1989). Handbook of Marine Mammals Vol 4: River Dolphins and the Larger Toothed Whales. London: Academic Press.
Rosso, M., Ballardini, M., Moulins, A., and Wurtz, M. (2011). Natural markings of Cuvier’s beaked whale Ziphius cavirostris in the Mediterranean Sea. Afr. J. Mar. Sci. 33, 45–57. doi: 10.2989/1814232X.2011.572336
Schorr, G. S., Baird, R. W., Hanson, M. B., Webster, D. L., Mcsweeney, D. J., and Andrews, R. D. (2009). Movements of satellite-tagged Blainville’s beaked whales off the island of Hawai‘i. Endanger. Species Res. 10, 203–213. doi: 10.3354/esr00229
Schorr, G. S., Falcone, E. A., Moretti, D. J., and Andrews, R. D. (2014). First long-term behavioral records from Cuvier’s beaked whales (Ziphius cavirostris) reveal record-breaking dives. PLoS One 9:10. doi: 10.1371/journal.pone.0092633
Shepard, E. L. C., Wilson, R. P., Rees, W. G., Grundy, E., Lambertucci, S. A., and Vosper, S. B. (2013). Energy landscapes shape animal movement ecology. Am. Nat. 182, 298–312. doi: 10.1086/671257
Shorter, K. A., Murray, M. M., Johnson, M., Moore, M., and Howle, L. E. (2014). Drag of suction cup tags on swimming animals: modeling and measurement. Mar. Mamm. Sci. 30, 726–746. doi: 10.1111/mms.12083
Silva, M. A., Prieto, R., Cascao, I., Seabra, M. I., Machete, M., Baumgartner, M. F., et al. (2014). Spatial and temporal distribution of cetaceans in the mid-Atlantic waters around the Azores. Mar. Biol. Res. 10, 123–137. doi: 10.1080/17451000.2013.793814
Southall, B. L., Nowacek, D. P., Miller, P. J. O., and Tyack, P. L. (2016). Experimental field studies to measure behavioral responses of cetaceans to sonar. Endanger. Species Res. 31, 293–315. doi: 10.3354/esr00764
Stanistreet, J. E., Nowacek, D. P., Baumann-Pickering, S., Bell, J. T., Cholewiak, D. M., Hildebrand, J. A., et al. (2017). Using passive acoustic monitoring to document the distribution of beaked whale species in the western North Atlantic Ocean. Can. J. Fish. Aquat. Sci. 74, 2098–2109. doi: 10.1139/cjfas-2016-0503
Stimpert, A. K., Deruiter, S. L., Southall, B. L., Moretti, D. J., Falcone, E. A., Goldbogen, J. A., et al. (2014). Acoustic and foraging behavior of a Baird’s beaked whale. Berardius bairdii, exposed to simulated sonar. Sci. Rep. 4:7031. doi: 10.1038/srep07031
Suberg, L., Wynn, R. B., Van Der Kooij, J., Fernand, L., Fielding, S., Guihen, D., et al. (2014). Assessing the potential of autonomous submarine gliders for ecosystem monitoring across multiple trophic levels (plankton to cetaceans) and pollutants in shallow shelf seas. Methods Oceanogr. 10, 70–89. doi: 10.1016/j.mio.2014.06.002
Thompson, K. F., Millar, C. D., Baker, C. S., Dalebout, M., Steel, D., Van Helden, A. L., et al. (2013). A novel conservation approach provides insights into the management of rare cetaceans. Biol. Conserv. 157, 331–340. doi: 10.1016/j.biocon.2012.07.017
Thompson, K. F., Patel, S., Baker, C. S., Constantine, R., and Millar, C. D. (2016). Bucking the trend: genetic analysis reveals high diversity, large population size and low differentiation in a deep ocean cetacean. Heredity 116, 277–285. doi: 10.1038/hdy.2015.99
Thompson, K. F., Ruggiero, K., Millar, C. D., Constantine, R., and Van Helden, A. L. (2014). Large-scale multivariate analysis reveals sexual dimorphism and geographic differences in the Gray’s beaked whale. J. Zool. 294, 13–21. doi: 10.1111/jzo.12151
Tyack, P. L., Johnson, M., Aguilar Soto, N., Sturlese, A., and Madsen, P. T. (2006). Extreme diving of beaked whales. J. Exp. Biol. 209, 4238–4253. doi: 10.1242/jeb.02505
Tyack, P. L., Zimmer, W. M. X., Moretti, D., Southall, B. L., Claridge, D. E., Durban, J. W., et al. (2011). Beaked whales respond to simulated and actual navy sonar. PLoS One 6:e17009. doi: 10.1371/journal.pone.0017009
Velten, B. P., Dillaman, R. M., Kinsey, S. T., Mclellan, W. A., and Pabst, D. A. (2013). Novel locomotor muscle design in extreme deep-diving whales. J. Exp. Biol. 216, 1862–1871. doi: 10.1242/jeb.081323
West, K. L., Walker, W. A., Baird, R. W., Mead, J. G., and Collins, P. W. (2017). Diet of Cuvier’s beaked whales Ziphius cavirostris from the North Pacific and a comparison with their diet world-wide. Mar. Ecol. Prog. Ser. 574, 227–242. doi: 10.3354/meps12214
Whitehead, H. (2013). Trends in cetacean abundance in the Gully submarine canyon, 1988-2011, highlight a 21% per year increase in Sowerby’s beaked whales (Mesoplodon bidens). Can. J. Zool. 91, 141–148. doi: 10.1139/cjz-2012-0293
Williams, T. M., Kendall, T. L., Richter, B. P., Ribeiro-French, C. R., John, J. S., Odell, K. L., et al. (2017). Swimming and diving energetics in dolphins: a stroke-by-stroke analysis for predicting the cost of flight responses in wild odontocetes. J. Exp. Biol. 220, 1135–1145. doi: 10.1242/jeb.154245
Keywords: beaked whale, Hyperoodon,Mesoplodon, Ziphius, Berardius
Citation: Hooker SK, De Soto NA, Baird RW, Carroll EL, Claridge D, Feyrer L, Miller PJO, Onoufriou A, Schorr G, Siegal E and Whitehead H (2019) Future Directions in Research on Beaked Whales. Front. Mar. Sci. 5:514. doi: 10.3389/fmars.2018.00514
Received: 16 August 2018; Accepted: 21 December 2018;
Published: 25 January 2019.
Edited by:
Lars Bejder, University of Hawai‘i at Manoa, United StatesReviewed by:
Whitlow W. L. Au, University of Hawai‘i, United StatesElizabeth McHuron, University of California, Santa Cruz, United States
Copyright © 2019 Hooker, De Soto, Baird, Carroll, Claridge, Feyrer, Miller, Onoufriou, Schorr, Siegal and Whitehead. This is an open-access article distributed under the terms of the Creative Commons Attribution License (CC BY). The use, distribution or reproduction in other forums is permitted, provided the original author(s) and the copyright owner(s) are credited and that the original publication in this journal is cited, in accordance with accepted academic practice. No use, distribution or reproduction is permitted which does not comply with these terms.
*Correspondence: Sascha K. Hooker, cy5ob29rZXJAc3QtYW5kcmV3cy5hYy51aw==