- 1Centre for Integrative Ecology, Deakin University, Geelong, VIC, Australia
- 2University of Exeter, College of Life and Environmental Sciences, Hatherly Laboratories, Exeter, United Kingdom
Over 25 years ago the first satellite tracking studies of sea turtles were published. The technology and attachment methods have now come of age with long-term tracks over a year being commonplace and the ability to relay high resolution GPS locations via the Argos satellite system along with behavioral (e.g., diving and activity) and environmental (e.g., temperature) data. Early studies focused on breeding females because they come ashore to nest, allowing individuals to be restrained relatively easily for tag attachment. However, today the development of methods for the capture of turtles at sea are increasingly allowing studies on both adult male turtles as well as immature turtles as small as 11 cm carapace length. Here we review the extent of work after many thousands of individual turtles have been tracked. We consider the state-of-the-art equipment for satellite tracking turtles and how this technology is being used to tackle key questions. We highlight some of the emerging opportunities arising from improved spatial resolution of tracking, increased robustness and miniaturization of tags as well as increasing availability of environmental data. We highlight the huge potential for big-data studies to make use of the thousands of tracks that exist, although we discuss the long-standing challenges surrounding data accessibility.
Background: A Brief History of Marine Turtle Satellite Tracking
The movements of sea turtles have long inspired biologists with, for example, Charles Darwin marveling at how they find small islands after long migrations (Darwin, 1873). The group have also attracted long-standing conservation concerns as they have historically been hunted both for food and to make jewelery (Mcclenachan et al., 2006) and more recently have been suffering high levels of fishery bycatch (Lewison et al., 2014). Interest in tracking sea turtles has therefore been rooted in both understanding aspects of their biology as well as to aid conservation. Sea turtles are relatively easy to deploy tracking devices on, as adult females come ashore in key locations for an hour or more to lay eggs in sandy beaches, where they can be easily located and detained. Some of the first ever satellite tags (electronic devices that remotely transmit information that enables the turtle to be geolocated by satellite) were deployed on marine turtles, probably because turtles are relatively large, and because their carapace provided a good attachment surface, with the first results published more than 25 years ago (e.g., Stoneburner, 1982). Sea turtles are an unusual group of marine megafauna as they comprise just seven species (compared, for example, with ~18,000 species of marine teleost fish, 1,094 species of marine elasmobranchs and 88 species of cetaceans). This has meant that it has been possible, rather unusually, to track and gain an understanding of every species in the guild. Studies have proliferated to encompass different ocean basins and developmental stages. A key review in 2008 (Godley et al., 2008) showed that about 1,000 sea turtles were being actively tracked each year between 2003 and 2006, but the number of published studies was increasing exponentially year on year. Here we update this picture on the extent of sea turtle tracking work. Some of the research priorities for sea turtles, many involving satellite tracking, were outlined by Hamann et al. (2010) and then more recently by Rees et al. (2016). Furthermore, a large group of experts identified key questions in the area of marine megafauna movement ecology, which are relevant to sea turtles (Hays et al., 2016). In the present review, our objective is not to simply reiterate these questions. Rather we first review the volume of work being undertaken in satellite tracking sea turtles. We then consider the state-of-the-art for what data satellite tags can deliver and how improvements in tag design, attachment protocols and the satellite transmission bandwidth are contributing to increasing data volumes obtained from each deployment. We then discuss key remaining unresolved questions while highlighting some of the most significant recent breakthroughs toward addressing these questions. We draw on developments that have been made in both terrestrial tracking as well as tracking of other marine species, including how progress in data-analysis skills emerging from other disciplines, such as mathematics and physics, can be used to tackle important questions. We also review integration of tracking data with environmental conditions, as well as how tracking information is being used to inform on conservation management. Lastly, we detail that the huge volume of data that has been collected since 1982 on the horizontal and vertical movements of all seven species of sea turtles would, used together, reveal a suite of metrics that would be greater than the sum of their parts. In this way we hope this article provides a road-map for those involved in sea turtle tracking work, helping to maximize how tracking work both answers key questions about the biology of sea turtles as well as helping conservation planning.
The Extent of Sea Turtle Tracking Studies
We carried out a Google Scholar review of the tracking literature and estimate that, in total, at least 7,002 marine turtles have been satellite tracked up to the end of 2017 (Figure 1; Appendix 1). This includes 44% of transmitters on loggerhead turtles (the greatest proportion, n = 3,077), and 14.1% on green (and black) turtles, 4.3% on hawksbill turtles, 7.2% on Kemp's ridleys, 4.1% on olive ridleys, 5.0% on flatback turtles, and 21.2% on leatherback turtles. This is clearly a huge number of transmitters. In many cases, devices deployed on turtles transmit data for well over a year, but even if they lasted only 6 months each, over 3,500 years worth of tracking data have been collected. In total, the combined tracking data that has ever been collected for marine turtles covers all seven species, and the entire global oceans in which marine turtles are known to occur. There are no comparable tracking efforts across all species in a family for any other marine vertebrate that we are aware of. We also emphasize that Godley et al. (2008) suggested that much more tracking information may exist, but has not been published in peer reviewed scientific journals, may not have been uploaded to the major tracking data portals such as STAT (Coyne and Godley, 2005), Movebank (Kranstauber et al., 2011), or OBIS-SEAMAP (Halpin et al., 2006, 2009), or may not be identified in online searches.
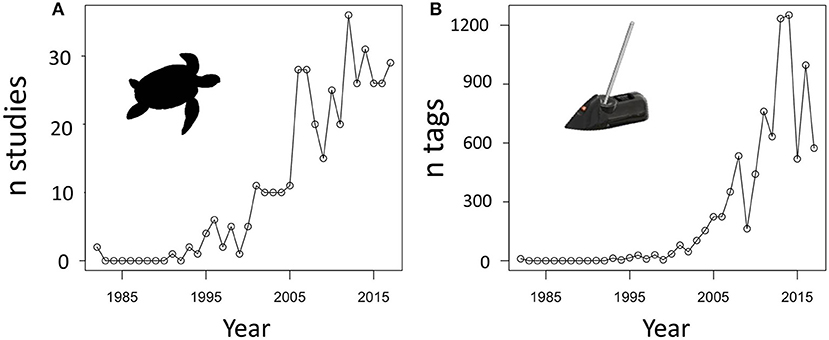
Figure 1. Plots showing the exponential increase in (A) the numbers of sea turtle tracking studies and (B) the number of unique satellite tags deployed on sea turtles since 1982. Data were collated using the search term “sea turtle satellite tracking” in Google Scholar, and excluded University theses, gray literature such as grant reports or conference proceedings (see Appendix 1 for database).
The Data That Satellite Tracking Can Deliver
The vast majority of satellite tracking is carried out via the Argos tracking network, which estimates locations using the Doppler effect in received signals as one or more of six satellites that carry Argos receivers approach, and then move away from, the satellite tag (www.argos-system.org). This system was established in 1978, mainly for vessel tracking, navigation and oceanography and provides locations whenever a transmitter (or satellite tag) is at the sea surface and a satellite is in view. It provides locations accurate to within a few hundreds of meters and up to several km from the true location of the transmitter (Witt et al., 2010), a resolution that may often suffice for describing broad scale migrations, but may be too poor to accurately describe localized movements, e.g., in and out of a port or small Marine Protected Area (Thomson et al., 2017). However, the advent of “Fastloc-GPS” has massively improved the accuracy of remote tracking, with tags acquiring GPS ephemeris data when a turtle surfaces and then relaying those data via the Argos system. Fastloc-GPS locations are typically accurate to within a few tens of meters of the true position (Dujon et al., 2014) allowing details of localized movements to be obtained (Christiansen et al., 2017). Argos satellite tags have proved to be an extremely useful data relay platform in this way, and other types of data collected on board a tag can now be remotely relayed, including dive profiles and water temperature (e.g., James et al., 2006).
As increasingly broad types of data are collected using remote biologging approaches, the bandwidth of the Argos system, the maximum amount of data that can be transferred per unit of time, remains a constraint. At present, the maximum is 256 bits of data per 15 second satellite uplink. In reality, since turtles are submerged for much of the time, the potential data transfer rate will be considerably less. Since advanced loggers now record thousands of data points per second (e.g., describing flipper beats or diving), the data transfer rate via the Argos system will be up to several orders of magnitude smaller than the rate at which data can be collected on board tags. Nevertheless, elegant techniques for compressing data prior to transmission (e.g., James et al., 2006) can ameliorate this problem to some extent, and detailed information can still be obtained remotely about not only where any animal is located but also what it is doing and what sort of environment it is in. For example >26,000 dive profiles were relayed for 12 leatherback turtles tracked moving around the North Atlantic for a combined tracking period of 9.6 years (Houghton et al., 2008). To date, studies involving extensive data relay via the Argos system represent only a fraction of the total tracking of marine turtles, not least because such devices usually cost considerably more than a standard “location only” transmitter and because analysis of the resultant data can be more challenging. The state of the art tracking studies are now deploying archival tracking devices (that have to be physically recovered in order to retrieve data), which can record three dimensional movements in sub-second resolution and provide startling insights not limited to locations at the sea surface (e.g., http://blog.arribada.org/2017/03/06/what-next-for-the-pit-stop-tag/). The use of accelerometry, for example, can enable “dead reckoning” (Bidder et al., 2012), which uses step lengths and directions to reconstruct the likely movement of an animal in obscure environments, in this case, underwater. Such innovative tracking approaches may be crucial to allow progress toward a comprehensive understanding of the ecology of marine turtles away from nesting beaches where they can be directly observed.
Tags have also become not just more sophisticated, but far more robust and reliable, with successful deployments of 2–3 years often now possible [e.g., Hawkes et al. (2012) tracked a hawksbill turtle for 1,302 days]. Important in this evolution of the technology has been identifying why satellite tags have failed in the past. For example, diagnostic data relayed via tags revealed that in the past a common reason for tag failure was the failure of the salt-water switch, which permits transmissions only when a turtle surfaces to breathe, because radio waves do not pass through seawater (Hays et al., 2007). The cause of failure of the salt-water switch appears to have been biofouling of the tag, so researchers are now increasingly aware of the need to paint tags with anti-biofouling compounds prior to deployment, as well as preparing tags prior to painting so that the paint stays attached to the tag for as long as possible rather than simply flaking off. Another common cause of tag failure appears to have been damage to the antenna as turtles often scrape their carapace, including the tag, on underwater rock ledges (Hays et al., 2007). Improvements to tag design mean that the antenna is now generally far more robust, for example being flexible and with the base in a sunken well, protected by raised baffles, which help prevent the antenna shearing off (Figure 2). Attachment systems have also improved, so that secure attachment of robust, low profile Argos tags providing minimum drag is now routine (Figure 2, Supplementary Video 1).
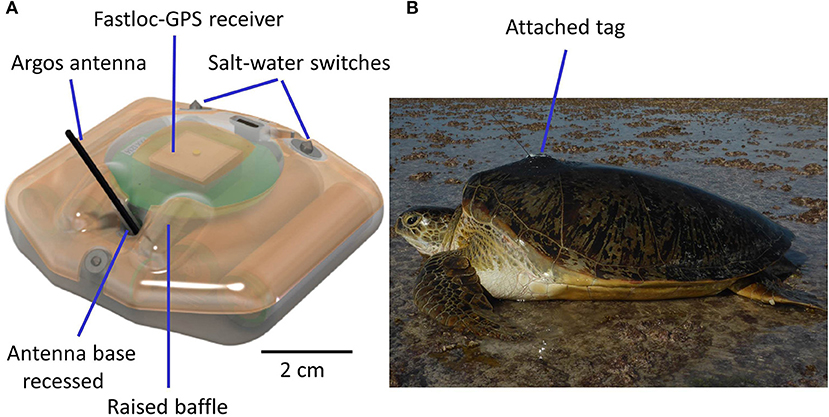
Figure 2. Secure attachment of robust, low profile Argos tags providing minimum drag is now routine (A). A schematic of a Fastloc-GPS Argos SPLASH manufactured by Wildlife Computers. Features of the tag which help prevent any damage if the turtle scrapes against rocks include the low profile of the tag, the flexible Argos antenna and the base of the antenna being in a depression protected by raised baffles. These features help reduce the likelihood of direct impacts to the base of the antenna and with any impacts the antenna simply bends rather than snaps (B). A photograph of the tag featured in (A) attached to a green turtle that had completed nesting (photo Graeme Hays). Note the low profile. The attachment has been painted with anti-fouling paint. For use of this tag see, for example, Hays et al. (2014b), Esteban et al. (2017), Thomson et al. (2017) and Christiansen et al. (2017). For a video of attachment see Supplementary Information.
Key Questions That Can be Addressed by Satellite Tracking
Satellite tracking is an ideal tool with which to address many of the key questions that have been raised and reviewed surrounding the biology and conservation of sea turtles (Hamann et al., 2007; Hays et al., 2016; Rees et al., 2016) and more broadly to marine megafauna in general, e.g., marine mammals, birds, and fish (Block et al., 2011). Below we detail key questions in italics, as detailed in the source review, and how satellite tracking can help.
What Are the Population Boundaries and Connections That Exist Among Rookeries and Foraging Grounds and Where Are These Key Foraging Habitats?
Tracking post-breeding individuals as they travel back to their foraging grounds, in combination with molecular approaches (e.g., Monzón-Argüello et al., 2010) can directly answer this research question. Indeed, this type of tracking work has been performed many times (Godley et al., 2008). Crucially here, the sample size needed to identify the full extent of foraging grounds used by turtles from a particular population is of interest. Should researchers plan to track 5, 10, 20 or more individuals? How many tags are enough? The answer to this question is site, species and population specific, and depends as well on the nature of the question being addressed and also the variability in behavior across individuals (Figure 3). For example while small sample sizes (< 10) may start to reveal the extent of migration, other studies using much larger sample sizes (>65 turtles e.g., Hawkes et al., 2011; Schofield et al., 2013a; Fossette et al., 2014) can estimate the proportion of the population traveling to different foraging areas.
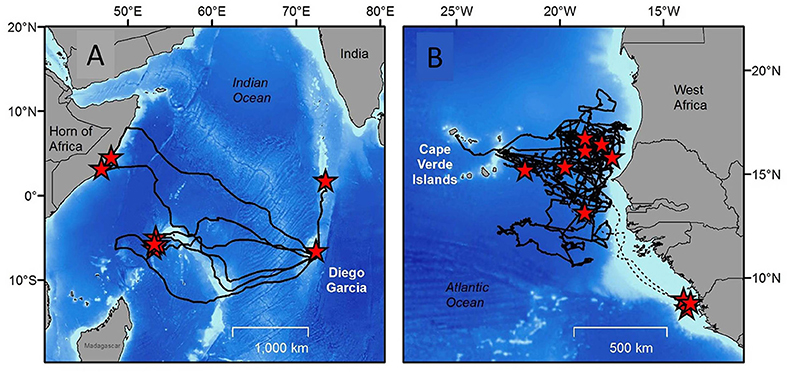
Figure 3. “How many tags are enough?” is an important question for any tracking study. The answer to this question depends on the nature of the question being addressed and also the variability in behavior across individuals (A). Tracks of eight green turtles equipped with satellite tags on nesting beaches on the Indian Ocean Island of Diego Garcia. Some migrate only a few 10s of km to the Great Chagos Bank, while others travel around 2,000 km to the Seychelles, and others still traveled almost 4,000 km to the coast of East Africa. The high variability in destinations means that more turtles will need to be tracked from this nesting area to identify the full extent of foraging ground destinations across the Indian Ocean (redrawn with permission from Hays et al., 2014b) (B). The tracks of 10 loggerhead turtles tracked from nesting beaches on the Cape Verde Islands (Atlantic Ocean). Seven out of ten individuals (solid lines) foraged across more than 500,000 km2 of oceanic areas without showing any focal behavior, while the remaining three (dashed lines) traveled to discrete foraging sites on the mainland coast of Africa. Here the rarer occurrence of travel to discrete foraging sites on mainland Africa might have been missed with a smaller sample size. Redrawn with permission from Hawkes et al. (2006).
How do Learning and Memory vs. Innate Behaviors Influence Movement Patterns, Including Ontogenetic Changes?
Hatchling sea turtles leave their nesting beaches and disperse into the ocean for a prolonged period, known as the “lost years” owing to the lack of precise knowledge of their movements during this period (Carr A. F Jr., 1987). Satellite tracking is increasingly being used to track small juvenile turtles (as small as 11 cm; Mansfield et al., 2014; Putman and Mansfield, 2015; Putman et al., 2016), facilitated by a miniaturization of satellite tags, principally for tracking birds. The development of new satellite tracking systems, such as ICARUS (https://icarusinitiative.org), may open up new opportunities for tracking even smaller turtles. However, because carapace growth rates are faster in juvenile than adult turtles, it is challenging to attach tags to very young turtles for long periods since the attachment contact point needs to expand as the turtle's carapace grows [see Mansfield et al. (2012) for attachment techniques that can cope]. It has been suggested that young turtles may drift passively around the oceans, only actively swimming if they find themselves in sub-optimum areas (Putman and Mansfield, 2015; Christiansen et al., 2016). It is thought that they imprint on suitable areas encountered during this oceanic traveling phase of their lives and return to those areas to forage as adults (Hays et al., 2010). Empirical support for this hypothesis comes from modeling studies that have shown that estimated hatchling drift patterns from nesting beaches correlate well with the migration directions of adult post-nesting turtles from those same sites (Shillinger et al., 2012; Scott et al., 2014). Tying down these ontogenetic links in movement will be aided by an ability to track hatchling turtles, which is not yet tractable. Furthermore, beginning from rather crude estimates made over 20 years ago of how ocean currents impact hatchling turtles (Hays and Marsh, 1997), there has been great progress made in modeling the effect of currents on hatchling turtle movements (e.g., Scott et al., 2014; Putman and Mansfield, 2015; Gaspar and Lalire, 2017), although ocean currents can be highly variably at sub-mesoscales (i.e., scales of tens of meters) meaning that modeled current data may still sometimes poorly reflect the actual currents experienced by a turtle (Briscoe et al., 2016; Putman et al., 2016). Further, developments (e.g., in situ recording of the ambient current where a turtle is located) are still needed to fully resolve the impact of currents on the movements of hatchings and juveniles. Finally, there are as yet no studies of learning or memory in sea turtles of any species to inform to what extent conscious cognition may play a role. Assessing the pattern of movement of young turtles will also help address the broader question:
What Parameters Influence the Biogeography of Sea Turtles in the Oceanic Realm?
In addition to transporting hatchling and juvenile turtles, currents may continue to influence the adult movements of oceanic species such as the leatherback turtle (Luschi et al., 2006), olive ridley (Plot et al., 2015; Dawson et al., 2017; Peavey et al., 2017) and some oceanic sub-populations of loggerhead (Hatase et al., 2002; Hawkes et al., 2006) and green turtles (Seminoff et al., 2008). For these oceanic foragers, ocean currents may influence the distribution of prey. For example, satellite tracking has highlighted that pelagic foragers often target the edges of mesoscale features (Doyle et al., 2008) or the boundary area between different ocean currents (Chambault et al., 2017), presumably because of locally high prey concentrations. For the majority, however, adult cheloniid turtles are relatively site faithful to small, benthic foraging areas. Thus, water depth and temperature appear to play the most significant role in determining the spatio-temporal occurrence of sea turtles in studies to date (Broderick et al., 2007; Hawkes et al., 2007, 2011; Schofield et al., 2008; Pikesley et al., 2014; Varo-Cruz et al., 2016).
What Will be the Impacts of Climate Change on Sea Turtles and How Can These Be Mitigated?
Satellite tracking allows many important demographic components of a sea turtles' life to be recorded, many of which may be impacted by climate change (Hamann et al., 2007; Hawkes et al., 2009; Poloczanska et al., 2009). Among these components are the range occupied, which may be influenced by sea temperature (McMahon and Hays, 2006), seasonal movements into cooler waters to feed (Hawkes et al., 2007), patterns of inactivity/winter dormancy (Broderick et al., 2007; Hawkes et al., 2007; Hochscheid et al., 2007) and the timing of migration (Schofield et al., 2013b). The biggest step forward in understanding the effect of large scale, long-term climatic change on sea turtle movement would be to amass the entire body of tracking data of all marine turtles for a particular ocean basin. Amongst the noise of thousands of individuals making seasonal movements between breeding and foraging grounds over decades would be signals of response to the onset of spring and to atypical climatic conditions [e.g., record warm summers or cold winters, Stott (2016)]. Such data would also allow a “then-and-now” comparison between past decades and the present, and would be invaluable in forecasting the effects of climate change to marine turtles at sea, which has been relatively poorly studied compared to the terrestrial breeding phase (Hamann et al., 2007; Hawkes et al., 2009; Poloczanska et al., 2009). Finally, strategies to mitigate the impact of climate change on turtles at sea have not been tackled in any meaningful way to date owing to this lack of knowledge.
What Are the Most Reliable Methods for Estimating Demographic Parameters?
There have been some exciting recent developments in the use of satellite tracking to identify where, when and how often sea turtles nest during a single season. This sort of information is often impossible to collect without telemetry because of the logistical difficulties of patrolling long sections of beach by foot, or if nesting densities are particularly high (Shanker et al., 2004; Marco et al., 2011; Valverde et al., 2012). Historically satellite tags tended to be attached to nesting females toward the end of the nesting season to track their post-breeding migrations, however, tags can instead be attached at the start of a nesting season to record each time a turtle comes ashore to nest. Several studies have now used satellite tags in this way and have revealed that turtles lay more clutches within a season than previously suspected (Tucker, 2010; Weber et al., 2013; Esteban et al., 2017; Tucker et al., 2018). The implications of this finding are important. Often census information for the status of populations is obtained by counting tracks on nesting beaches and assuming some nominal mean clutch frequency per individual. So then the total number of nesting females in a season equals the total number of nesting tracks divided by the assumed nominal mean clutch frequency. So the finding that turtles lay far more clutches than previously suspected, means that the number of nesting turtles may be far fewer that previously calculated (Esteban et al., 2017). This finding means that the conservation value of each nesting turtle, in terms of their contribution of eggs to a population, is far more than previously assumed. Satellite tags also reveal, with high resolution, where sea turtles nest and so identify their nesting beach (and site) fidelity. In this way satellite tagging can complement assessments of nest locations made by foot patrols (Patricio et al., 2017).
In addition to resolving clutch frequency (see above), the biology of adult male turtles, which are understudied compared to adult females, can be investigated using satellite tracking (Arendt et al., 2012; Pajuelo et al., 2012; Casale et al., 2013). Unlike females, male turtles do not come ashore to nest and so have to be captured at sea or surveyed using drones (Schofield et al., 2017). Importantly, tracking work has shown that the interval between breeding seasons (the remigration interval) is generally shorter for males than females, which makes sense since males likely make a smaller energetic investment in breeding than female turtles (Hays et al., 2014a). The implications of this finding are important in that the female skewed hatchling sex ratios that have been widely reported around the globe (Hawkes et al., 2009) likely translate into more balanced operational sex ratios (i.e., the adult sex ratio on the breeding grounds) (Wright et al., 2012; Hays et al., 2014a). Tracking data are also revealing how the extent of adult movements in the breeding period may influence the incidence of multiple paternity in clutches by impacting male-female encounter rates (Lee and Hays, 2004; Zbinden et al., 2007; Uller and Olsson, 2008; Wright et al., 2012; Lee et al., 2018). For example, leatherback turtles tend to travel extensively during the breeding season in order to forage (Hitipeuw et al., 2007; Witt et al., 2008; Fossette et al., 2009), which tends to reduce male-female encounters (Lee et al., 2018) and hence produce a low incidence of multiple paternity in clutches. Conversely, for example, limited movements by green turtles in the breeding season (Craig et al., 2004; Fuller et al., 2008) can contribute to a much high incidence of multiple paternity (Lee et al., 2018).
In some cases satellite tracking may also be used to estimate mortality rates in sea turtles (Hays et al., 2003a). In these cases the end point of satellite tracking has been indicative of the death of the tracked turtle. For example, the information relayed may indicate that the tag has come out of the water and it starts to move like a vessel, suggesting the turtle has been fished; or a tag may come out of the water and into a coastal village, suggesting capture and mortality; or a tag may move ashore on the coast, indicative of a turtle dying and drifting ashore. Where on-site visits have been possible, then mortality inferred in this way from tag data has often been confirmed by local inspection (Hays et al., 2003a). However, there are multiple reasons for why tags may fail, including exhaustion of batteries, biofouling and loss of the tag antenna and distinguishing the reasons for tag failure is not always simple (Hays et al., 2007). However, we urge people to examine the data received immediately prior to a tag failing in order to try and diagnose the reasons for the cessation of transmissions. In addition, satellite tags that pop off and float to the surface may be used to indicate mortality of pelagic turtles. In this case tags may be programmed to detach from the turtle once, (a) a certain depth is exceeded or (b) the tag remains at a constant depth for several days, with both these conditions set to values which would not occur in a living animal (de Quevedo et al., 2013).
How Can Movement Data Be Used to Support Conservation and Management?
While satellite tracking can be an important step toward informed conservation planning–if we know where animals live then we know where to protect them–conservation planning is often much more complex and involves competing issues from different stakeholders (Wilson et al., 2007; Engel et al., 2008; Jenkins et al., 2012; Hussey et al., 2015). At the same time, conservation policy may too little rely on solid, empirical biodiversity data, such as that provided by tracking, instead being based on intuition and anecdotes to set spatial boundaries, temporal limits and moderate the level of anthropogenic activity (Ferraro and Pattanayak, 2006). An important challenge therefore is how to make better use of tracking data in terms of translation into policy (e.g., Hooker et al., 2011) and there has only been one assessment to date of the translation of tracking data into conservation practice for marine turtles (Jeffers and Godley, 2016). Perhaps surprisingly, only 19% of marine turtle tracking studies cited “conservation issues” as a main reason for tracking, and the review was only able to find 12 instances where tracking had led to real-world changes in conservation practice. Clearly, there is much room to do better in future studies, including the re-use of the huge body of tracking data that has already been collected.
How do Animals Navigate and Orientate in the Open Sea?
Sea turtles have long been considered highly accomplished navigators because they can travel hundreds of kilometers to small, remote island targets, or with high fidelity to repeated areas between years, for example to breed (Luschi et al., 1998; Åkesson et al., 2003; Broderick et al., 2007). Laboratory experiments have gone some way to help explain how these journeys are successfully completed—sea turtles possess a compass sense (Lohmann et al., 2008) and may use air-borne olfactory cues in navigation (Hays et al., 2003b). This role of air-borne cues is likewise thought to be important for seabirds finding small islands (Gagliardo et al., 2013; Pollonara et al., 2015) with, for example, birds that are experimentally prevented from using their sense of smell, i.e., that were anosmic, often struggling to locate island targets. In addition, elegant laboratory studies in which components of the ambient magnetic inclination and magnetic intensity have been manipulated have suggested that sea turtles may have a crude magnetic map of the areas they will occupy throughout their lives as juveniles and adults (Lohmann et al., 2001), implying that they may not use the Earth's geomagnetic field for pinpoint navigation, but rather geomagnetic signposts to broadly orientate themselves. Taken together, it can be predicted that adult turtles traveling long distances to remote island targets will tend to travel directly to the general vicinity of their island target using geomagnetic cues, but may then need local cues (e.g., winds) for the final island approach. However, to date most satellite tracking has been for post-breeding sea turtles traveling away from breeding sites, so future studies tracking individuals toward their small island targets will be key.
What Areas can be Considered Hotspots for Multiple Species on a Global Scale?
To assess global hotspots across sea turtle species, the entire body (or at least a large part) of tracking data for all sea turtle species would ideally be combined. This has been achieved for some other taxa (e.g., Block et al., 2011) but here a key issue surrounds data sharing and data availability (see section below on “Future challenges”). Another challenge surrounds the biases in deployment sites, with many more tags deployed from North America, for example, than Africa (see Figure 3 in Godley et al., 2008) and more tags deployed from nesting sites than foraging sites. In the future, this bias could be tackled by weighting analyses of tracking from different areas, but ultimately may require additional deployments in under-represented areas (Godley et al., 2008).
Are There Simple Rules Underlying Seemingly Complex Movement Patterns and, Hence, Common Drivers for Movement Across Species?
Addressing this question again requires large data-sets and so data sharing and accessibility is extremely important. Underlying this question is the fact that different sea turtle species may face common issues in terms of finding resources such as food, mates and refuges from predation. For example, prey may be patchily distributed necessitating search to locate resources, such that similar search patterns might be expected across species (e.g., Humphries et al., 2010). Additionally sea turtles may themselves denude foraging patches and so face choices as patch quality declines in terms of when they leave to search for new patches. Again there may be commonalities in how the different species respond to this changing patch quality. Alternatively turtles may move within a well-defined home-range where their prey distribution is better known (e.g., Hawkes et al., 2011; Wood et al., 2016; Christiansen et al., 2017) but where the risk of predation also varies in some predictable manner so that animals need to trade-off competing pressures of feeding but also avoiding predators (Hammerschlag et al., 2015, 2016). Again there may be common solutions to these competing pressures. To address these questions common data analysis procedures need to be applied across tracking data from multiple taxa. Here there have been important developments in how to decompose animal tracks into their component parts such as step lengths between turns (Bidder et al., 2012, 2015; Sequeira et al., 2018). Here turtle biologists can benefit from the developments made from studies involving other taxa such as fish and birds (Humphries et al., 2010; Hussey et al., 2015; Kays et al., 2015).
Future Challenges
Tag Cost and Data Capture
A major challenge for the future of tag development for sea turtles is the huge cost of developing, refining and miniaturizing technology. Although tracking tags may appear initially expensive, the person-hours required to develop each tag are disproportionately high in relation to the market opportunity to which they can be sold. By contrast, the sports wearable, home consumer and medical markets represent billions of dollars of opportunity (e.g., the global wearables market was worth $4.8 billion in the second quarter of 2018; www.idc.com), which thus funds remarkable advances in sensor design and miniaturization. It has been largely impossible to translate these gains to wildlife—devices designed for human use are rarely depth proof, and are usually designed to either relay data to a highly powered data station, such as a smart phone, or to collect data for a just a few hours to days before needing recharging via USB. There are some developments in this area with cheap (< approx. US$100) GPS logging tags allowing marine animals to be tracked over short periods of just a few days with high resolution (e.g., Allan et al., 2013, the Arribada Initiative: https://www.raspberrypi.org/blog/sea-turtles/). These cheap devices work well when the logger can be recovered, such as with foraging seabirds returning to provision a chick or between sea turtle nesting attempts, but their application to migrating animals is more challenging as the period over which data can be collected is usually restricted to between a few days to a week. In these cases GPS logging tags may be a more viable option than Argos satellite tags (e.g., Schofield et al., 2007). There are projects underway to develop cheap satellite tags that remotely relay information to bespoke receivers, such as the ICARUS initiative (https://icarusinitiative.org). However, there are generally few engineers available to develop such tags for the conservation sector, and despite more than a decade of encouragement for scientists to work across disciplines, insufficient work of this type has been funded.
Another useful approach is to supplement results from satellite tracking by using other approaches. In some cases stable isotope (SI) analysis has been effectively combined with satellite tracking. For example, post-nesting turtles have been satellite tracked and at the same time tissue samples collected for SI analysis (Zbinden et al., 2011). SI ratios may vary between individuals foraging in different areas and, when this is the case, then SI analysis alone can be used to infer the destination for post-nesting turtles (Zbinden et al., 2011). Molecular analysis of turtles captured on foraging grounds may also be used to assess their natal nesting area (e.g., Rees et al., 2017). This use of a combination of methods to assess patterns of movement (satellite tracking, stable isotopes, molecular analysis) is a useful approach to bolster the sample sizes achieved with satellite tracking alone.
The limited bandwidth of the Argos system (256 bits transmitted maximally every 15 s) also limits the data transfer volume so that some information (e.g., extended video) cannot be relayed. While on board data compression tactics, such as reducing dive profiles to key points of inflection (e.g., Doyle et al., 2008), help recover large amounts of data despite this limited bandwidth, ultimately the gigabytes of data (e.g., video, accelerometry) that latest projects seek to collect cannot be transmitted remotely without supplementary battery power. Instead, archival, retrieved tag systems are far better suited to such study aims, dedicating battery life to data acquisition and storage.
Data
The issue of data sharing and availability pervades through many of the key questions surrounding the movement ecology of sea turtles (see above). In many cases the data has been collected to address key questions, but is not accessible. Given this wealth of information from satellite tracking, we echo the sentiments of Godley et al. (2008) 10 years ago in suggesting that to provide robust metrics for real conservation protection of marine turtles, tracking data should be collated into broad, multi-species and population datasets to produce a single meta-dataset. This would reveal a suite of metrics from the sum of the tracking, which could be greater than any of the parts. For example, animated by day of year, this dataset could reveal the seasonal movements of temperate marine turtles between summer foraging and wintering grounds, highlighting differences between ocean basins, species and regional populations. Likewise, overlaid with oceanographic variables en masse, it would be possible to quantify the extent to which changes in temperature, depth and currents influence marine turtle movements, and how these differ by body size and by species. By collating huge numbers of individuals encountering the same conditions through time and space, statistical outliers could be identified and the consequences of the decisions made by atypical turtles examined. Animated over the 35 years over which marine turtle tracking has taken place to date, responses of turtles to large-scale climatic fluctuations could be analyzed—revealing insights into the potential impact of climate change on the total distribution of marine turtles.
This issue of data availability pervades through many different areas of science and in some cases it has been successfully addressed. For example, a wide range of satellite remote sensed environmental data is freely available, such as sea surface temperature, bathymetry and ocean color and these data have underpinned many studies that examine the influence of ocean conditions on the distribution of marine animals (Hussey et al., 2015). There are also some pioneering examples of huge amounts of animal tracking data that are now freely available, such as Ocean Biogeographic Information System—Spatial Ecological Analysis of Megavertebrate Populations (OBIS SEAMAP) (Halpin et al., 2006, 2009). How, then, might it be possible to combine and make available sea turtle tracking data? A major barrier to such an endeavor remains the ownership of these expensive data. Satellite tracking data are expensive and difficult to obtain and so field biologists may, understandably, be reluctant to simply hand over their hard-won data to others to publish. This is an extremely difficult challenge to tackle, heightened by the competitive nature of academic publishing, appointments and grant funding. In some of these cases mentioned above, data availability was a condition of initial funding from a centralized grant or funder, and this same constraint applies in many nationally funded marine observation programs such as Australia's Integrated Marine Observing System (IMOS) (Hoenner et al., 2018). However, most sea turtle tracking projects are funded through individually won grants from a huge variety of public and private sources, and it is thus far harder to instigate a policy where data availability is a condition of funding, nor to retrospectively apply this to past data. Another development is the increasing requirement for authors to make data open access at publication in academic journals, although summaries of tracking data can be posted, rather than original raw location data. The most likely route to data sharing thus remains collaborative studies, which has worked well with a growing volume of data and growing number of data providers. It is vital that these barriers of data availability be overcome for the sake of marine turtle conservation, which should be the ultimate goal for deployment of the overwhelming majority of satellite transmitters on turtles, as well as the reason for monitoring nesting activity on beaches.
There are encouraging examples of the benefit of collaborative tracking studies where data are shared. For example, several groups that had satellite tracked leatherback turtles came together to compare the pattern of movement for individuals tracked in the Pacific vs. Atlantic (Bailey et al., 2012) (Figure 4). This study revealed markedly different patterns of movement between these ocean basins. Individuals in the Atlantic often traveled very slowly, indicative of high foraging success in prey patches, but this movement behavior almost never occurred in the Pacific. This poor foraging environment in the Pacific may be closely linked to the low reproductive output and poor conservation outlook for leatherbacks in that ocean basin (Saba et al., 2008). More recently data from a range of studies have been pooled to compare 106 leatherback tracks from the North and South Atlantic to assess key sites of interaction with pelagic longlines fisheries (Fossette et al., 2014) with similar research gains to be made. The value of collaborative studies has recently been embraced by several international programmes. For example, as part of the Marine Megafauna Movement Analytical Program (MMMAP), Sequeira et al. (2018) analyzed ~2.8 million locations from >2,600 tracked individuals spanning 50 marine vertebrate species, including sea turtles. MMMAP continue to expand this data-set and welcome further collaborative partners (https://mmmap.wordpress.com/). Another international programme, MiCO is likewise assembling marine animal tracking data to address conservation questions (https://mgel.env.duke.edu/mico/). There is hence an encouraging outlook for data-sharing, with the animal tracking community increasingly becoming aware that there are huge benefits from being associated with these collaborative programmes. Published papers comprising dozens of authors are a positive sign that the field is learning to collaborate for the best possible conservation outcomes for sea turtles.
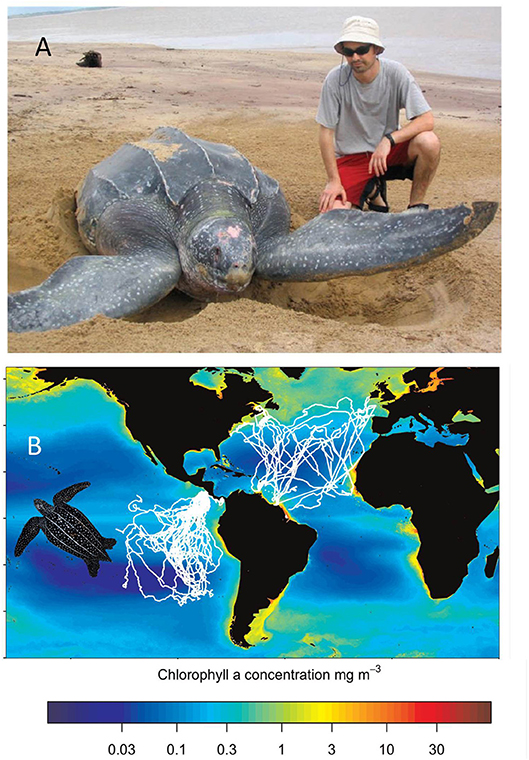
Figure 4. The value of collaborative studies that pool tracking data (A). The leatherback turtle (Dermochelys coriacea) is the largest species of sea turtle, nesting on tropical and sub-tropical beaches but then traveling extensively across ocean basins in search of its jellyfish prey. In the eastern Pacific leatherback turtles are listed as “Critically Endangered” (i.e., on the verge of extinction) while in the North Atlantic their conservation status is listed as “Least Concern” (http://www.iucnredlist.org) (photo credit and informed consent: Tom Doyle) (B). The tracks of 77 leatherback turtles tracked in the eastern Pacific and North Atlantic. Different movement patterns between these two ocean basins indicate higher foraging success for individuals in the Atlantic, which may be linked to their higher reproductive output and healthier conservation status. Modified with permission from Bailey et al. (2012).
Concluding Remarks
The use of satellite tracking as a tool for studying sea turtles continues to expand and tracking data are providing answers to more and more of the key questions surrounding the biology and conservation of sea turtles. Added to this encouraging outlook, perhaps the most important development in this area in recent years has been an international move for sharing of tracking data, not just for sea turtles but more widely for marine animals in general. Support for these initiatives is growing, pointing to a new era of studies using big data to address unresolved questions and the widespread realization of tracking data being used for conservation benefit.
Author Contributions
All authors listed have made a substantial, direct and intellectual contribution to the work, and approved it for publication.
Funding
GH was supported by the Bertarelli Foundation as part of the Bertarelli Programme in Marine Science. LH thanks Jess Rudd for help digitizing turtle tracking records.
Conflict of Interest Statement
The authors declare that the research was conducted in the absence of any commercial or financial relationships that could be construed as a potential conflict of interest.
Acknowledgments
We thank all those who have been part of our turtle tracking studies over the last 25 years.
Supplementary Material
The Supplementary Material for this article can be found online at: https://www.frontiersin.org/articles/10.3389/fmars.2018.00432/full#supplementary-material
Video S1. Attaching satellite tags to sea turtles. For hard-shelled turtles, current attachment procedures typically involve first removing any fouling biota (e.g., barnacles or algae) from the carapace and then to sand-paper and wipe the carapace with alcohol or acetone, removing any grease and leaving a very clean, dry carapace which has a good surface for glue to bond to. Next a strong two-part epoxy is usually used to attach the transmitter to the carapace. For example the Hays Lab uses Pure-2 K (Powers Fastening Innovations) also known in some countries as Pure 150-PRO (DeWalt). The epoxy is usually smoothed to provide a streamlined shape (e.g., using silicon baking spatulas) and the attachment is painted with a marine antifouling paint (e.g.,Trilux 33, International). Care is taken to ensure that upon surfacing, water can easily drain from around the salt-water switches, e.g., there is nowhere for water to pool around the switches.
References
Åkesson, S., Broderick, A. C., Glen, F., Godley, B. J., Luschi, P., Papi, F., et al. (2003). Navigation by green turtles: which strategy do displaced adults use to find Ascension Island? Oikos 103, 363–372. doi: 10.1034/j.1600-0706.2003.12207.x
Allan, B. M., Arnould, J. P. Y., Martin, J. K., and Ritchie, E. G. (2013). A cost-effective and informative method of GPS tracking wildlife. Wildl. Res. 40, 345–348. doi: 10.1071/WR13069
Arendt, M. D., Segars, A. L., Byrd, J. I., Boyton, J., Schwenter, J. A., Whitaker, J. D., et al. (2012). Migration, distribution, and diving behavior of adult male loggerhead sea turtles (Caretta caretta) following dispersal from a major breeding aggregation in the Western North Atlantic. Mar. Biol. 159, 113–125. doi: 10.1007/s00227-011-1826-0
Bailey, H., Fossette, S., Bograd, S. J., Shillinger, G. L., Swithenbank, A. M., Georges, J.-Y., et al. (2012). Movement patterns for a critically endangered species, the leatherback turtle (Dermochelys coriacea), linked to foraging success and population status. PLoS ONE 7:e36401. doi: 10.1371/journal.pone.0036401
Bidder, O. R., Soresina, M., Shepard, E. L. C., Halsey, L. G., Quintana, F., Gómez-Laich, A., et al. (2012). The need for speed: testing acceleration for estimating animal travel rates in terrestrial dead-reckoning systems. Zoology 115, 58–64. doi: 10.1016/j.zool.2011.09.003
Bidder, O. R., Walker, J. S., Jones, M. W., Holton, M. D., Urge, P., Scantlebury, D. M., et al. (2015). Step by step: reconstruction of terrestrial animal movement paths by dead-reckoning. Mov. Ecol. 3:23. doi: 10.1186/s40462-015-0055-4
Block, B. A., Jonsen, I. D., Jorgensen, S. J., Winship, A. J., Shaffer, S. A., Bograd, S. J., et al. (2011). Tracking apex marine predator movements in a dynamic ocean. Nature 475:86. doi: 10.1038/nature10082
Briscoe, D. K., Parker, D. M., Balazs, G. H., Kurita, M., Saito, T., Okamoto, H., et al. (2016). Active dispersal in loggerhead sea turtles (Caretta caretta) during the ‘lost years'. Proc. Roy. Soc. Lond. B 283:20160690. doi: 10.1098/rspb.2016.0690
Broderick, A. C., Coyne, M. S., Fuller, W. J., Glen, F., and Godley, B. J. (2007). Fidelity and over-wintering of sea turtles. Proc. Roy. Soc. Lond. B 274, 1533–1538. doi: 10.1098/rspb.2007.0211
Carr A. F Jr. (1987). New perspectives on the pelagic stage of sea turtle development. Cons. Biol. 1, 103–121. doi: 10.1111/j.1523-1739.1987.tb00020.x
Casale, P., Freggi, D., Cinà, A., and Rocco, M. (2013). Spatio-temporal distribution and migration of adult male loggerhead sea turtles (Caretta caretta) in the Mediterranean Sea: further evidence of the importance of neritic habitats off North Africa. Mar. Biol. 160, 703–718. doi: 10.1007/s00227-012-2125-0
Chambault, P., Roquet, F., Benhamou, S., Baudena, A., Pauthenet, E., De Thoisy, B., et al. (2017). The Gulf Stream frontal system: a key oceanographic feature in the habitat selection of the leatherback turtle? Deep Sea Res. I 123, 35–47. doi: 10.1016/j.dsr.2017.03.003
Christiansen, F., Esteban, N., Mortimer, J. A., Dujon, A. M., and Hays, G. C. (2017). Diel and seasonal patterns in activity and home range size of green turtles on their foraging grounds revealed by extended Fastloc-GPS tracking. Mar. Biol. 164:10. doi: 10.1007/s00227-016-3048-y
Christiansen, F., Putman, N. F., Farman, R., Parker, D. M., Rice, M. R., Polovina, J. J., et al. (2016). Spatial variation in directional swimming enables juvenile sea turtles to reach and remain in productive waters. Mar. Ecol. Prog. Ser. 557, 247–259. doi: 10.3354/meps11874
Coyne, M. S., and Godley, B. J. (2005). Satellite tracking and analysis tool (STAT): an integrated system for archiving, analyzing and mapping animal tracking data. Mar. Ecol. Prog. Ser. 301, 1–7. doi: 10.3354/meps301001
Craig, P., Parker, D. M., Brainard, R., Rice, M., and Balazs, G. H. (2004). Migrations of green turtles in the central South Pacific. Biol. Cons. 116, 433–438. doi: 10.1016/S0006-3207(03)00217-9
Dawson, T. M., Formia, A., Agamboué, P. D., Asseko, G. M., Boussamba, F., Cardiec, F., et al. (2017). Informing marine protected area designation and management for nesting olive ridley sea turtles using satellite tracking. Front. Mar. Sci. 4:312. doi: 10.3389/fmars.2017.00312
de Quevedo, I. A., San Felix, M., and Cardona, L. (2013). Mortality rates in by-caught loggerhead turtle Caretta caretta in the Mediterranean Sea and implications for the Atlantic populations. Mar. Ecol. Prog. Ser. 489, 225–234. doi: 10.3354/meps10411
Doyle, T. K., Houghton, J. D. R., O'súilleabháin, P. F., Hobson, V. J., Marnell, F., Davenport, J., et al. (2008). Leatherback turtles satellite tagged in European waters. Endangered Species Res. 4, 23–31. doi: 10.3354/esr00076
Dujon, A. M., Lindstrom, R. T., and Hays, G. C. (2014). The accuracy of Fastloc-GPS locations and implications for animal tracking. Methods Ecol. Evol. 5, 1162–1169. doi: 10.1111/2041-210X.12286
Engel, S., Pagiola, S., and Wunder, S. (2008). Designing payments for environmental services in theory and practice: an overview of the issues. Ecol. Econom. 65, 663–674. doi: 10.1016/j.ecolecon.2008.03.011
Esteban, N., Mortimer, J. A., and Hays, G. C. (2017). How numbers of nesting sea turtles can be over-estimated by nearly a factor of two. Proc. Roy. Soc. Lond. B 284:20162581. doi: 10.1098/rspb.2016.2581
Ferraro, P. J., and Pattanayak, S. K. (2006). Money for nothing? A call for empirical evaluation of biodiversity conservation investments. PLoS Biol. 4:e105. doi: 10.1371/journal.pbio.0040105
Fossette, S., Girard, C., Bastian, T., Calmettes, B., Ferraroli, S., Vendeville, P., et al. (2009). Thermal and trophic habitats of the leatherback turtle during the nesting season in French Guiana. J. Exp. Mar. Biol. Ecol. 378, 8–14. doi: 10.1016/j.jembe.2009.06.021
Fossette, S., Witt, M. J., Miller, P., Nalovic, M. A., Albareda, D., Almeida, A. P., et al. (2014). Pan-Atlantic analysis of the overlap of a highly migratory species, the leatherback turtle, with pelagic longline fisheries. Proc. Roy. Soc. Lond. B 281:20133065. doi: 10.1098/rspb.2013.3065
Fuller, W. J., Broderick, A. C., Phillips, R. A., Silk, J. R. D., and Godley, B. J. (2008). Utility of geolocating light loggers for indicating at-sea movements in sea turtles. Endangered Species Res. 4, 139–146. doi: 10.3354/esr00048
Gagliardo, A., Bried, J., Lambardi, P., Luschi, P., Wikelski, M., and Bonadonna, F. (2013). Oceanic navigation in Cory's shearwaters: evidence for a crucial role of olfactory cues for homing after displacement. J. Exp. Biol. 216, 2798–2805. doi: 10.1242/jeb.085738
Gaspar, P., and Lalire, M. (2017). A model for simulating the active dispersal of juvenile sea turtles with a case study on western Pacific leatherback turtles. PLoS ONE 12:e0181595. doi: 10.1371/journal.pone.0181595
Godley, B. J., Blumenthal, J. M., Broderick, A. C., Coyne, M. S., Godfrey, M. H., Hawkes, L. A., et al. (2008). Satellite tracking of sea turtles: where have we been and where do we go next? Endangered Species Res. 4, 3–22. doi: 10.3354/esr00060
Halpin, P. N., Read, A. J., Best, B. D., Hyrenbach, K. D., Fujioka, E., Coyne, M. S., et al. (2006). OBIS-SEAMAP developing a biogeographic research data commons for the ecological studies of marine mammals, seabirds, and sea turtles. Mar. Ecol. Prog. Ser. 316, 239–246. doi: 10.3354/meps316239
Halpin, P. N., Read, A. J., Fujioka, E. I., Best, B. D., Donnelly, B. E. N., Hazen, L. J., et al. (2009). OBIS-SEAMAP: the world data center for marine mammal, sea bird, and sea turtle distributions. Oceanography 22, 104–115. doi: 10.5670/oceanog.2009.42
Hamann, M., Godfrey, M. H., Seminoff, J. A., Arthur, K., Barata, P. C. R., Bjorndal, K. A., et al. (2010). Global research priorities for sea turtles: informing management and conservation in the 21st century. Endangered Species Res. 11, 245–269. doi: 10.3354/esr00279
Hamann, M., Limpus, C. J., and Read, M. A. (2007). “Vulnerability of marine reptiles in the Great Barrier Reef to climate change,” in Climate change and the Great Barrier Reef: A Vulnerability Assessment, eds J. E. Johnson, and P. A. Marshall (Hobart: Great Barrier Reef Marine Park Authority and Australia), 465–496.
Hammerschlag, N., Bell, I., Fitzpatrick, R., Gallagher, A. J., Hawkes, L. A., Meekan, M. G., et al. (2016). Behavioral evidence suggests facultative scavenging by a marine apex predator during a food pulse. Behavioral Ecol. Sociobiol. 70, 1777–1788. doi: 10.1007/s00265-016-2183-2
Hammerschlag, N., Broderick, A. C., Coker, J. W., Coyne, M. S., Dodd, M., Frick, M. G., et al. (2015). Evaluating the landscape of fear between apex predatory sharks and mobile sea turtles across a large dynamic seascape. Ecology 96, 2117–2126. doi: 10.1890/14-2113.1
Hatase, H., Takai, N., Matsuzawa, Y., Sakamoto, W., Omuta, K., Goto, K., et al. (2002). Size-related differences in feeding habitat use of adult female loggerhead turtles Caretta caretta around Japan determined by stable isotope analyses and satellite telemetry. Mar. Ecol. Prog. Ser. 233, 273–281. doi: 10.3354/meps233273
Hawkes, L. A., Broderick, A. C., Coyne, M. S., Godfrey, M. H., and Godley, B. J. (2007). Only some like it hot-quantifying the environmental niche of the loggerhead sea turtle. Divers. Distrib. 13, 447–457. doi: 10.1111/j.1472-4642.2007.00354.x
Hawkes, L. A., Broderick, A. C., Coyne, M. S., Godfrey, M. H., Lopez-Jurado, L. F., Lopez-Suarez, P., et al. (2006). Phenotypically linked dichotomy in sea turtle foraging requires multiple conservation approaches. Curr. Biol. 16:990–995. doi: 10.1016/j.cub.2006.03.063
Hawkes, L. A., Broderick, A. C., Godfrey, M. H., and Godley, B. J. (2009). Climate change and marine turtles. Endangered Species Res. 7, 137–154. doi: 10.3354/esr00198
Hawkes, L. A., Tomas, J., Revuelta, O., Leon, Y. M., Blumenthal, J. M., Broderick, A. C., et al. (2012). Migratory patterns in hawksbill turtles described by satellite tracking. Mar. Ecol. Prog. Ser. 461, 223–232. doi: 10.3354/meps09778
Hawkes, L. A., Witt, M. J., Broderick, A. C., Coker, J. W., Coyne, M. S., Dodd, M., et al. (2011). Home on the range: spatial ecology of loggerhead turtles in Atlantic waters of the USA. Divers. Distribut. 17, 624–640. doi: 10.1111/j.1472-4642.2011.00768.x
Hays, G. C., Åkesson, S., Broderick, A. C., Glen, F., Godley, B. J., Papi, F., et al. (2003b). Island-finding ability of marine turtles. Proc. Roy. Soc. B. 270, S5–S7. doi: 10.1098/rsbl.2003.0022
Hays, G. C., Bradshaw, C. J. A., James, M. C., Lovell, P., and Sims, D. W. (2007). Why do Argos satellite tags deployed on marine animals stop transmitting? J. Exp. Mar. Biol. Ecol. 349, 52–60. doi: 10.1016/j.jembe.2007.04.016
Hays, G. C., Broderick, A. C., Godley, B. J., Luschi, P., and Nichols, W. J. (2003a). Satellite telemetry suggests high levels of fishing induced mortality for marine turtles. Mar. Ecol. Prog. Ser. 262, 305–309. doi: 10.3354/meps262305
Hays, G. C., Ferreira, L. C., Sequeira, A. M. M., Meekan, M. G., Duarte, C. M., Bailey, H., et al. (2016). Key questions in marine megafauna movement ecology. Trends Ecol. Evol. 6, 463–475. doi: 10.1016/j.tree.2016.02.015
Hays, G. C., Fossette, S., Katselidis, K. A., Mariani, P., and Schofield, G. (2010). Ontogenetic development of migration: lagrangian drift trajectories suggest a new paradigm for sea turtles. J. Royal Soc. Interface 7, 1319–1327. doi: 10.1098/rsif.2010.0009
Hays, G. C., and Marsh, R. (1997). Estimating the age of juvenile loggerhead sea turtles in the North Atlantic. Can. J. Zool. 75, 40–46. doi: 10.1139/z97-005
Hays, G. C., Mazaris, A. D., and Schofield, G. (2014a). Different male vs. female breeding periodicity helps mitigate offspring sex ratio skews in sea turtles. Front. Mar. Sci. 1:43. doi: 10.3389/fmars.2014.00043
Hays, G. C., Mortimer, J. A., Ierodiaconou, D., and Esteban, N. (2014b). Use of long-distance migration patterns of an endangered species to inform conservation planning for the world's largest marine protected area. Cons. Biol. 28, 1636–1644. doi: 10.1111/cobi.12325
Hitipeuw, C., Dutton, P. H., Benson, S. R., Thebu, J., and Bakarbessy, J. (2007). Population status and internesting movement of leatherback turtles, Dermochelys coriacea, nesting on the northwest coast of Papua, Indonesia. Chelon. Conserv. Biol. 6, 28–36. doi: 10.2744/1071-8443
Hochscheid, S., Bentivegna, F., Bradhai, M. N., and Hays, G. C. (2007). Overwintering behaviour in sea turtles: dormancy is optional. Mar. Ecol. Prog. Ser. 340, 287–298. doi: 10.3354/meps340287
Hoenner, X., Huveneers, C., Steckenreuter, A., Simpfendorfer, C. A., Tattersall, K., Jaine, F., et al. (2018). Australia's continental-scale acoustic tracking database and its automated quality control process. Sci. Data 5:180206. doi: 10.1038/sdata.2017.206
Hooker, S. K., Cañadas, A., Hyrenbach, K. D., Corrigan, C., Polovina, J. J., and Reeves, R. R. (2011). Making protected area networks effective for marine top predators. Endangered Species Res. 13, 203–218. doi: 10.3354/esr00322
Houghton, J. D. R., Doyle, T. K., Davenport, J., Wilson, R. P., and Hays, G. C. (2008). The role of infrequent and extraordinary deep dives in leatherback turtles (Dermochelys coriacea). J. Exp. Biol. 211, 2566–2575. doi: 10.1242/jeb.020065
Humphries, N. E., Queiroz, N., Dyer, J. R. M., Pade, N. G., Musyl, M. K., Schaefer, K. M., et al. (2010). Environmental context explains Lévy and Brownian movement patterns of marine predators. Nature 465, 1066–1069. doi: 10.1038/nature09116
Hussey, N. E., Kessel, S. T., Aarestrup, K., Cooke, S. J., Cowley, P. D., Fisk, A. T., et al. (2015). Aquatic animal telemetry: a panoramic window into the underwater world. Science 348:1255642. doi: 10.1126/science.1255642
James, M. C., Davenport, J., and Hays, G. C. (2006). Expanded thermal niche for a diving vertebrate: a leatherback turtle diving into near-freezing water. J. Exp. Mar. Biol. Ecol. 335, 221–226. doi: 10.1016/j.jembe.2006.03.013
Jeffers, V. F., and Godley, B. J. (2016). Satellite tracking in sea turtles: how do we find our way to the conservation dividends? Biol. Cons. 199, 172–184. doi: 10.1016/j.biocon.2016.04.032
Jenkins, L. D., Maxwell, S. M., and Fisher, E. (2012). Increasing conservation impact and policy relevance of research through embedded experiences. Cons. Biol. 26, 740–742. doi: 10.1111/j.1523-1739.2012.01878.x
Kays, R., Crofoot, M. C., Jetz, W., and Wikelski, M. (2015). Terrestrial animal tracking as an eye on life and planet. Science 348:aaa2478. doi: 10.1126/science.aaa2478
Kranstauber, B., Cameron, A., Weinzerl, R., Fountain, T., Tilak, S., Wikelski, M., et al. (2011). The Movebank data model for animal tracking. Environ. Model. Soft. 26, 834–835. doi: 10.1016/j.envsoft.2010.12.005
Lee, P. L. M., and Hays, G. C. (2004). Polyandry in a marine turtle: females make the best of a bad job. Proc. Natl. Acad. Sci. U.S.A. 101, 6530–6535. doi: 10.1073/pnas.0307982101
Lee, P. L. M., Schofield, G., Haughey, R. I., Mazaris, A. D., and Hays, G. C. (2018). A review of patterns of multiple paternity across sea turtle rookeries. Adv. Marine Biol. 79, 1–31. doi: 10.1016/bs.amb.2017.09.004
Lewison, R. L., Crowder, L. B., Wallace, B. P., Moore, J. E., Cox, T., Zydelis, R., et al. (2014). Global patterns of marine mammal, seabird, and sea turtle bycatch reveal taxa-specific and cumulative megafauna hotspots. Proc. Natl. Acad. Sci. U.S.A. 111, 5271–5276. doi: 10.1073/pnas.1318960111
Lohmann, K. J., Cain, S. D., Dodge, S. A., and Lohmann, C. M. F. (2001). Regional magnetic fields as navigational markers for sea turtles. Science 294, 364–366. doi: 10.1126/science.1064557
Lohmann, K. J., Luschi, P., and Hays, G. C. (2008). Goal navigation and island-finding in sea turtles. J. Exp. Mar. Biol. Ecol. 356, 83–95. doi: 10.1016/j.jembe.2007.12.017
Luschi, P., Hays, G. C., Del Seppia, C., Marsh, R., and Papi, F. (1998). The navigational feats of green sea turtles migrating from Ascension Island investigated by satellite telemetry. Proc. Roy. Soc. Lond. B 265, 2279–2284. doi: 10.1098/rspb.1998.0571
Luschi, P., Lutjeharms, J. R. E., Lambardi, R., Mencacci, R., Hughes, G. R., and Hays, G. C. (2006). A review of migratory behaviour of sea turtles off Southeastern Africa. South African J. Sci. 102, 51–58.
Mansfield, K. L., Wyneken, J., Porter, W. P., and Luo, J. G. (2014). First satellite tracks of neonate sea turtles redefine the 'lost years' oceanic niche. Proc. Roy. Soc. Lond. B 281:20133039. doi: 10.1098/rspb.2013.3039
Mansfield, K. L., Wyneken, J., Rittschoff, D., Walsh, M., Lim, C. W., and Richards, P. (2012). Satellite tag attachment methods for tracking neonate sea turtles. Mar. Ecol. Prog. Ser. 457 181–192. doi: 10.3354/meps09485
Marco, A., Abella-Perez, E., Monzón-Argüello, C., Martins, S., Araujo, S., and Lopez-Jurado, L. F. (2011). The international importance of the archipelago of Cape Verde for marine turtles, in particular the loggerhead turtle Caretta caretta. Zool. Caboverd. 2, 1–11.
Mcclenachan, L., Jackson, J. B. C., and Newman, M. J. H. (2006). Conservation implications of historic sea turtle nesting beach loss. Front. Ecol. Environ. 4, 290–296. doi: 10.1890/1540-9295
McMahon, C. R., and Hays, G. C. (2006). Thermal niche, large scale movements and implications of climate change for a critically endangered marine vertebrate. Glob. Change Biol. 12, 1330–1338. doi: 10.1111/j.1365-2486.2006.01174.x
Monzón-Argüello, C., López-Jurado, L. F., Rico, C., Marco, A., López, P., Hays, G. C., et al. (2010). Evidence from genetic and Lagrangian drifter data for transatlantic transport of small juvenile green turtles. J. Biogeog. 37, 1752–1766. doi: 10.1111/j.1365-2699.2010.02326.x
Pajuelo, M., Bjorndal, K. A., Reich, K. J., Arendt, M. D., and Bolten, A. B. (2012). Distribution of foraging habitats of male loggerhead turtles (Caretta caretta) as revealed by stable isotopes and satellite telemetry. Mar. Biol. 159, 1255–1267. doi: 10.1007/s00227-012-1906-9
Patricio, A. R., Varela, M. R., Barbosa, C., Broderick, A. C., Ferreira Airaud, M. B., Godley, B. J., et al. (2017). Nest site selection repeatability of green turtles, Chelonia mydas, and consequences for offspring. Anim. Behav. 139, 91–102. doi: 10.1016/j.anbehav.2018.03.006
Peavey, L. E., Popp, B. N., Pitman, R. L., Gaines, S. D., Arthur, K. E., Kelez, S., and Seminoff, J. A., (2017). Opportunism on the high seas: foraging ecology of olive ridley turtles in the Eastern Pacific Ocean. Front. Mar. Sci. 4:348. doi: 10.3389/fmars.2017.00348
Pikesley, S. K., Broderick, A. C., Cejudo, D., Coyne, M. S., Godfrey, M. H., Godley, B. J., et al. (2014). Modelling the niche for a marine vertebrate: a case study incorporating behavioural plasticity, proximate threats and climate change. Ecography 38, 803–812. doi: 10.1111/ecog.01245
Plot, V., De Thoisy, B., and Georges, J. Y. (2015). Dispersal and dive patterns during the post-nesting migration of olive ridley turtles from French Guiana. Endangered Species Res. 26, 221–234. doi: 10.3354/esr00625
Pollonara, E., Luschi, P., Guilford, T., Wikelski, M., Bonadonna, F., and Gagliardo, A. (2015). Olfaction and topography, but not magnetic cues, control navigation in a pelagic seabird: displacements with shearwaters in the Mediterranean Sea. Sci. Rep. 5:16486. doi: 10.1038/srep16486
Poloczanska, E. S., Limpus, C. J., and Hays, G. C. (2009). Vulnerability of marine turtles to climate change. Adv. Marine Biol. 56, 151–211. doi: 10.1016/S0065-2881(09)56002-6
Putman, N. F., Lumpkin, R., Sacco, A. E., and Mansfield, K. L. (2016). Passive drift or active swimming in marine organisms? Proc. Roy. Soc. Lond. B 283:20161689. doi: 10.1098/rspb.2016.1689
Putman, N. F., and Mansfield, K. L. (2015). Direct evidence of swimming demonstrates active dispersal in the sea turtle ‘lost years'. Curr. Biol. 25, 1221–1227. doi: 10.1016/j.cub.2015.03.014
Rees, A. F., Alfaro-Shigueto, J., Barata, P. C. R., Bjorndal, K. A., Bolten, A. B., Bourjea, J., et al. (2016). Are we working toward global research priorities for management and conservation of sea turtles? Endangered Species Res. 31, 337–382. doi: 10.3354/esr00801
Rees, A. F., Carreras, C., Broderick, A. C., Margaritoulis, D., Stringell, T. B., and Godley, B. J. (2017). Linking loggerhead locations: using multiple methods to determine the origin of sea turtles in feeding grounds. Mar. Biol. 164:30. doi: 10.1007/s00227-016-3055-z
Saba, V. S., Spotila, J. R., Chavez, F. P., and Musick, J. A. (2008). Bottom-up and climatic forcing on the worldwide population of leatherback turtles. Ecology 89, 1414–1427. doi: 10.1890/07-0364.1
Schofield, G., Bishop, C. M., Katselidis, K. A., Dimopoulos, P., Pantis, J. D., and Hays, G. C. (2008). Microhabitat selection by sea turtles in a dynamic thermal marine environment. J. Anim. Ecol. 78, 14–21. doi: 10.1111/j.1365-2656.2008.01454.x
Schofield, G., Bishop, C. M., MacLeon, G., Brown, P., Baker, M., Katselidis, K. A., et al. (2007). Novel GPS tracking of sea turtles as a tool for conservation management. J. Exp. Mar. Biol. Ecol. 347, 58–68. doi: 10.1016/j.jembe.2007.03.009
Schofield, G., Dimadi, A., Fossette, S., Katselidis, K. A., Koutsoubas, D., Lilley, M. K. S., et al. (2013a). Satellite tracking large numbers of individuals to infer population level dispersal and core areas for the protection of an endangered species. Divers. Distribut. 19, 834–844. doi: 10.1111/ddi.12077
Schofield, G., Katselidis, K. A., Lilley, M. K. S., Reina, R. D., and Hays, G. C. (2017). Detecting elusive aspects of wildlife ecology using drones: new insights on the mating dynamics and operational sex ratios of sea turtles. Funct. Ecol. 31, 2310–2319. doi: 10.1111/1365-2435.12930
Schofield, G., Scott, R., Dimadi, A., Fossette, S., Katselidis, K. A., Koutsoubas, D., et al. (2013b). Evidence-based marine protected area planning for a highly mobile endangered marine vertebrate. Biol. Cons. 161, 101–109. doi: 10.1016/j.biocon.2013.03.004
Scott, R., Marsh, R., and Hays, G. C. (2014). Ontogeny of long distance migration. Ecology 95, 2840–2850. doi: 10.1890/13-2164.1
Seminoff, J. A., Zárate, P., Coyne, M. S., Foley, D. G., Parker, D. M., Lyon, B., et al. (2008). Post-nesting migrations of Galapagos green turtles, Chelonia mydas, in relation to oceanographic conditions of the eastern tropical Pacific Ocean: integrating satellite telemetry with remotely-sensed ocean data. Endangered Species Res. 4, 57–72. doi: 10.3354/esr00066
Sequeira, A. M. M., Rodríguez, J. P., Eguíluz, V. M., Harcourt, R., Hindell, M., Sims, D. W., et al. (2018). Convergence of marine megafauna movement patterns in coastal and open oceans. Proc. Natl. Acad. Sci. U.S.A. 12, 3072–3077. doi: 10.1073/pnas.1716137115
Shanker, K., Pandav, B., and Choudhury, B. C. (2004). An assessment of the olive ridley turtle (Lepidochelys olivacea) nesting population in Orissa, India. Biol. Conserv. 115, 149–160. doi: 10.1016/S0006-3207(03)00104-6
Shillinger, G. L., Di Lorenzo, E., Luo, H., Bograd, S. J., Hazen, E. L., Bailey, H., et al. (2012). On the dispersal of leatherback turtle hatchlings from Mesoamerican nesting beaches. Proc. Roy. Soc. Lond. B 279, 2392–2395. doi: 10.1098/rspb.2011.2348
Stoneburner, D. L. (1982). Satellite telemetry of loggerhead sea turtle movement on the Georgia Bight. Copeia 1982, 400–408. doi: 10.2307/1444621
Stott, P. (2016). How climate change affects extreme weather events. Science 352, 1517–1518. doi: 10.1126/science.aaf7271
Thomson, J. A., Börger, L., Christianen, M. J. A., Esteban, N., Laloë, J. O., and Hays, G. C. (2017). Implications of location accuracy and data volume for home range estimation and fine-scale movement analysis: comparing Argos and Fastloc-GPS tracking data. Mar. Biol. 164:204. doi: 10.1007/s00227-017-3225-7
Tucker, A. D. (2010). Nest site fidelity and clutch frequency of loggerhead turtles are better elucidated by satellite telemetry than by nocturnal tagging efforts: implications for stock estimation. J. Exp. Mar. Biol. Ecol. 383, 48–55. doi: 10.1016/j.jembe.2009.11.009
Tucker, A. D., Baldwin, R. F., Willson, A., Kiyumi, A. A., Harthi, S. A., Schroeder, B., et al. (2018). Revised clutch frequency estimates for Masirah Island loggerhead turtles (Caretta caretta). Herpetol. Conserv. Biol. 13, 158–166.
Uller, T., and Olsson, M. (2008). Multiple paternity in reptiles: patterns and processes. Mol. Ecol. 17, 2566–2580. doi: 10.1111/j.1365-294X.2008.03772.x
Valverde, R. A., Orrego, C. M., Tordoir, M. T., Gómez, F. M., Solís, D. S., Hernández, R. A., et al. (2012). Olive Ridley mass nesting ecology and egg harvest at Ostional Beach, Costa Rica. Chelon. Conserv. Biol. 11, 1–11. doi: 10.2744/CCB-0959.1
Varo-Cruz, N., Bermejo, J. A., Calabuig, P., Cejudo, D., Godley, B. J., López-Jurado, L. F., et al. (2016). New findings about the spatial and temporal use of the Eastern Atlantic Ocean by large juvenile loggerhead turtles. Divers. Distribut. 22, 481–492. doi: 10.1111/ddi.12413
Weber, N., Weber, S. B., Godley, B. J., Ellick, J., Witt, M. J., and Broderick, A. C. (2013). Telemetry as a tool for improving estimates of marine turtle abundance. Biol. Cons. 167, 90–96. doi: 10.1016/j.biocon.2013.07.030
Wilson, K. A., Underwood, E. C., Morrison, S. A., Klausmeyer, K. R., Murdoch, W. W., Reyers, B., et al. (2007). Conserving biodiversity efficiently: what to do, where, and when. PLoS Biol. 5:e223. doi: 10.1371/journal.pbio.0050223
Witt, M. J., Åkesson, S., Broderick, A. C., Coyne, M. S., Ellick, J., Formia, A., et al. (2010). Assessing accuracy and utility of satellite tracking data using Argos-linked Fastloc-GPS. Anim. Behav. 80, 571–581. doi: 10.1016/j.anbehav.2010.05.022
Witt, M. J., Broderick, A. C., Coyne, M. S., Formia, A., Ngouessono, S., Parnell, R. J., et al. (2008). Satellite tracking highlights difficulties in the design of effective protected areas for critically endangered leatherback turtles Dermochelys coriacea during the inter-nesting period. Oryx 42, 296–300. doi: 10.1017/S0030605308006947
Wood, L. D., Brunnick, B., and Milton, S. L. (2016). Home range and movement patterns of subadult hawksbill sea Turtles in Southeast Florida. J. Herpet. 51, 58–67. doi: 10.1670/15-133
Wright, L. I., Stokes, K. L., Fuller, W. J., Godley, B. J., Mcgowan, A., Snape, R., et al. (2012). Turtle mating patterns buffer against disruptive effects of climate change. Proc. Roy. Soc. B 279, 2122–2127. doi: 10.1098/rspb.2011.2285
Zbinden, J. A., Bearhop, S., Bradshaw, P., Gill, B., Margaritoulis, D., Newton, J., et al. (2011). Migratory dichotomy and associated phenotypic variation in marine turtles revealed by satellite tracking and stable isotope analysis. Mar. Ecol. Progr. Ser. 421, 291–302. doi: 10.3354/meps08871
Keywords: fastloc, migration, megafauna, conservation, ICARUS, MMMAP, MiCO, movement
Citation: Hays GC and Hawkes LA (2018) Satellite Tracking Sea Turtles: Opportunities and Challenges to Address Key Questions. Front. Mar. Sci. 5:432. doi: 10.3389/fmars.2018.00432
Received: 10 August 2018; Accepted: 29 October 2018;
Published: 20 November 2018.
Edited by:
Michael Paul Jensen, Southwest Fisheries Science Center (NOAA), United StatesReviewed by:
Luis Cardona, University of Barcelona, SpainJérôme Bourjea, Institut Français de Recherche pour l'Exploitation de la Mer (IFREMER), France
Copyright © 2018 Hays and Hawkes. This is an open-access article distributed under the terms of the Creative Commons Attribution License (CC BY). The use, distribution or reproduction in other forums is permitted, provided the original author(s) and the copyright owner(s) are credited and that the original publication in this journal is cited, in accordance with accepted academic practice. No use, distribution or reproduction is permitted which does not comply with these terms.
*Correspondence: Graeme C. Hays, g.hays@deakin.edu.au
†These authors have contributed equally to this work