- 1Laboratório de Ficologia, Departamento de Botânica, Museu Nacional, Universidade Federal do Rio de Janeiro, Rio de Janeiro, Brazil
- 2Laboratório de Biologia Molecular, Universidade do Vale do Itajaí, Itajaí, Brazil
- 3Algal Resources Collection, MARBIONC, University of North Carolina Wilmington, Wilmington, DE, United States
A review of the dinoflagellate genus Alexandrium occurring in Brazilian coastal waters is presented based on both published information and new data. Seven Alexandrium species have been recorded from Brazil so far: Alexandrium catenella, Alexandrium fraterculus, Alexandrium gaardnerae, Alexandrium kutnerae, Alexandrium tamiyavanichi, Alexandrium tamutum, and Alexandrium sp. While A. gaardnerae and A. kutnerae were identified based only on morphological characteristics, phylogenetic analysis (ITS and LSU rDNA) were performed for the remaining species based on cultures and/or field populations. Monoclonal cultures of the analyzed species were isolated from field samples obtained from Bahia (A. tamiyavanichi, two strains), Rio de Janeiro (A. tamutum, three strains; Alexandrium sp., two strains), Santa Catarina (A. fraterculus, one strain), and Rio Grande do Sul (Alexandrium tamarense, three strains). This is the first record of A. tamutum for the South Atlantic. In addition, molecular data for Brazilian strains of A. fraterculus are presented for the first time, as well as sequences from the ITS region for A. catenella (previously reported as A. tamarense) from Brazilian coastal waters. The morphological characters of the three species corresponded to those typically recorded in the literature and their identification was confirmed by molecular analysis. Based on the LSU rDNA and ITS regions, the three strains of A. catenella showed a high degree of similarity with strains from Southern Chile and North America. The implications and limitations of these findings for the monitoring protocols within the global and regional context are discussed.
Introduction
The dinoflagellate genus Alexandrium Halim currently encompasses more than 30 species (Anderson et al., 2012), some of them known worldwide as the causative agents of blooms and/or production of neurotoxins associated to the Paralytic Shellfish Poisoning (PSP) syndrome (Wang, 2008; Etheridge, 2010). Recognized as the most geographically widespread algal-related shellfish poisoning syndrome, PSP constitutes a serious human illness caused by the ingestion of seafood contaminated with saxitoxin (STXs) and its derivatives (Deeds et al., 2008; Anderson et al., 2012). PSP symptoms include spreading numbness and tingling sensations, headache and nausea, and in more severe cases may cause paralysis of the muscles of the chest and abdomen leading to death (Etheridge, 2010). Besides the evident relevance to human health, accumulation of PSP toxins in filter-feeding bivalves constitutes an additional issue for the shellfish industry and fisheries activities (Etheridge, 2010; Rhodes and Munday, 2016).
Many factors have been suggested as the cause of the observed increase in the worldwide extension and frequency of Alexandrium blooms and other Harmful Algal Bloom (HAB) species, such as ocean currents, climate change, pollution, ballast water dispersal, and colonization of newly generated niches (Nagai et al., 2007; Matsuyama et al., 2010; Anderson et al., 2012, 2015; Anderson, 2017; Gobler et al., 2017). It has also been suggested that the higher number of blooms reported nowadays is a direct result of increased monitoring activities and the implementation of more sensitive tools to detect and prevent the negative effects of HABs worldwide, particularly in aquaculture areas (Anderson, 2007; Anderson et al., 2015). Both toxic and non-toxic blooms of Alexandrium species have been reported from distinct geographic regions, e.g., Japan (Kodama, 2010), Mediterranean Sea (Karydis and Kitsiou, 2012), China (Lu et al., 2014), Argentina Sea (Fabro et al., 2017), Australia (Ajani et al., 2017), and the Bering Sea (Natsuike et al., 2017).
In Brazil, records of HABs related to human intoxications in coastal areas increased in the last 20 years, becoming a national issue with socio-economic repercussions (Silva et al., 2013). Particularly, reports of Alexandrium species in the Brazilian coast have been intensifying (Figure 1). To date, five species were previously identified based on light microscopy: Alexandrium garderae Nguyen-Ngoc & Larsen [ = Alexandrium concavum (Gaarder) Balech], Alexandrium fraterculus (Balech) Balech, Alexandrium kutnerae (Balech) Balech, Alexandrium catenella (Whedon & Kofoid) Balech [ = Alexandrium tamarense (Lebour) Balech], and Alexandrium tamiyavanichi Balech (Balech, 1995; Persich et al., 2006; Menezes et al., 2010). STX production has been confirmed for isolates of A. catenella (Persich et al., 2006) and A. tamiyavanichi (Menezes et al., 2010). A toxic bloom of Alexandrium sp. (previously identified as Alexandrium minutum Halim by Menezes et al., 2007) and a non-toxic bloom of Alexandrium frateculus were recorded, respectively, in beaches at Rio de Janeiro (Menezes et al., 2007) and Santa Catarina (Omachi et al., 2007). Cysts of A. catenella and A. cf. minutum were additionally detected in sediments from Patos lagoon and Sepetiba bay, respectively (Persich and Garcia, 2003; Juliano and Garcia, 2006). Among these studies, only Persich et al. (2006) and Menezes et al. (2010) applied molecular techniques to study the phylogeny and biogeography of the identified species.
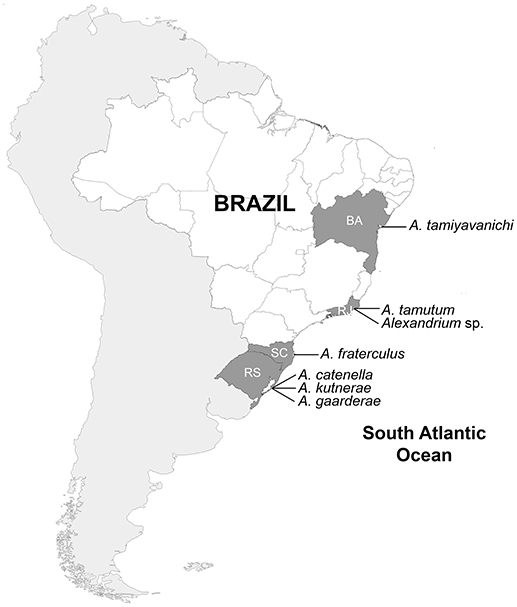
Figure 1. Distribution of Alexandrium species along the Brazilian coast. BA, Bahia; RJ, Rio de Janeiro; RS, Rio Grande do Sul; SC, Santa Catarina.
Here, we present a review of the dinoflagellate genus Alexandrium in Brazilian coastal waters, based both on available published information and new morphological and molecular data (28S rDNA e ITS) for A. fraterculus, A. catenella, Alexandrium sp., and Alexandrium tamutum Montresor, Beran & John (the latter being the first record for the South Atlantic).
Materials and Methods
This review was based on previously published information for A. garderae (Balech, 1995), A. kutnerae (Balech, 1995), A. tamyavanichi (Menezes et al., 2010), and A. catenella (formerly identified as A. tamarense by Persich et al., 2006). New morphological and molecular data were obtained from the analysis of monoclonal strains of A. tamutum and Alexandrium sp. (same species identified as A. minutum by Menezes et al., 2007) isolated from Rio de Janeiro, and A. fraterculus isolated from Santa Catarina (Figure 1). Cells were grown using in K medium at 16 and 24 PSU (A. tamutum), K medium at 30 PSU (Alexandrium sp.), and F/2 medium at 34 PSU (A. fraterculus). Cultures were maintained at 20–23°C with photon flux density of 70–136 μE m−2.s−1 and a 12:12-h light:dark photoperiod. We also performed the morphological characterization and ITS rDNA sequencing for three of the A. catenella strains (ATBR1A7, ATBR2E, ATBR3D) used by Persich et al. (2006), and obtained from the Woods Hole Oceanographic Institution. Finally, the morphology of Alexandrium sp. was further re-analyzed by scanning electron microscopy (SEM) using environmental Lugol fixed samples obtained from the bloom recorded in 2007 from Rio de Janeiro by Menezes et al. (2007). The precedence of all strains/samples used here is informed in Table 1.
Morphological Analysis
The tabulation of the species was characterized by the addition of calcofluor MR2 (10 ng ml−1) under an Olympus BX51 epifluorescence microscope (Fritz and Triemer, 1985). For SEM, cells were harvested by filtration on 0.45 μm pore acetate membranes (Millipore). Filters were immediately fixed for 2 h in 2.5% glutaraldehyde in 0.1 M sodium cacodylate buffer (pH 7.2). After that, they were washed three times (10 min) using the same buffer and postfixed in 1% osmium tetroxide in sodium cacodylate buffer (pH 7.2). Filters were finally dehydrated in an ascending ethanol series of 10 min each 35, 50, 70, 90, and 100%, followed by two washes for 10 min each in 100% ethanol, critical-point dried (CPD 020 Balzers Union). Dried filters were mounted on stubs, coated with gold (Balzers/Union FL-9496), and viewed with a JEOL 5310 scanning electron microscope (Akishima, Tokyo, Japan). The metric data that represented outliers for minimal and maximal cell dimensions were shown in parentheses.
PCR Amplification and DNA Sequencing
DNA from A. tamutum and Alexandrium sp. was extracted from exponentially growing cultures using NucleoSpin® Plant II extraction kit (Macherey-Nagel GmbH & Co., KG, Germany) following the manufacturer's protocol. The ITS (including 5.8S rDNA gene) and LSU (D1–D3) regions of rDNA were amplified using primers ITSA/ITSB (Sato et al., 2011) and D3F/D1R (Scholin et al., 1994; Litaker et al., 2003), respectively. The PCR mix (25 μl final volume) contained 1 U GoTaq® DNA polymerase (Promega, Madison, WI, USA), 1X GoTaq® Flexi buffer (Promega), 1.25 mM MgCl2 solution (Promega), 0.16 mM dNTPs (Thermo Scientific Inc., USA), 8 pmol of each primer, 0.2 μg of Bovine Serum Albumin (BSA) (New England Biolabs Inc.), and ~15 ng genomic DNA. The PCR protocol was as follows: initial denaturation for 5 min at 94°C, followed by 35 cycles of 1 min denaturation at 94°C, 1 min annealing at 50 and 55°C (ITS and LSU rDNA, respectively), and 1 min extension at 72°C, plus a final extension of 5 min at 72°C. The PCR products were purified and sequenced by Macrogen (Seoul, Korea) in both directions using the PCR primers. The sequences obtained were deposited in GenBank (Supplementary Tables S1, S2).
DNA extraction from A. fraterculus strain was performed using a modified CTAB protocol (Lebret et al., 2012), whereas DNA extracts from fixed samples of A. catenella strains were obtained using a modified guanidinium isothiocyanate protocol (Alves-de-Souza et al., 2011). Both ITS and LSU (D1–D3) regions of rDNA were obtained for A. fraterculum whereas only ITS rDNA sequences were obtained for A. catenella using the same primer mentioned above. For both species, the PCR mix (15 mL final volume) contained 1–6 mL of the DNA extract, 330 mM of each deoxynucleoside triphosphate (dNTP), 2.5 mM of MgCl2, 1.25 U of GoTaq1 DNA polymerase (Promega Corporation), 0.17 mM of both primers, 1X reaction buffer (Promega Corporation). PCR protocol included an initial denaturating step at 95°C for 5 min, 35 cycles at 95°C for 1 min, annealing at 55°C for 45 s, extension at 72°C for 1 min 15 s, with a final extension step at 72°C for 7 min. PCR products were purified using the ExoSAP-IT kit (USB) following the manufacturer's recommendations and directly sequenced on an ABI Prism 3100 automatic sequencer (Applied Biosystems).
Phylogenetic analyses were performed for each rDNA region (ITS and LSU) individually. Alexandrium sequences obtained from this study and from GenBank (Supplementary Tables S1, S2) were aligned using the online package MAFFT version 7 (https://mafft.cbrc.jp/alignment/server/) followed by manual editing. The Maximum Likelihood (ML) analyses were conducted using the software MEGA 7.0 (Kumar et al., 2016). The evolutionary models used were the Hasegawa-Kishino-Yano model (HKY) for ITS rDNA and the Tamura-Nei model (TN93) for LSU rDNA, both with gamma distribution. These models were the best available for the tree of the rDNA region data (ITS and LSU). All positions containing gaps and missing data were eliminated. The robustness of the inferred topology was tested by bootstrap resampling (1,000 replicates). Bayesian inferences (BI) were obtained with the software MrBayes 3.2 (Ronquist et al., 2012). Rather than selecting a nucleotide substitution model by a priori model selection, we used the “lset nst = mixed” Markov Chain Monte Carlo procedure, consisting of two independent trials with four chains. The chains were run for 5,000,000 generations and sampled every 100th cycle. Posterior probability (PP) values for the resulting 50% majority rule consensus tree were estimated after discarding the first 25% of the trees as burn-in. All trees were rooted using Alexandrium lee and Alexandrium diversaporum sequences as outgroup.
Results
Based on both published information and the new data, seven Alexandrium species have been identified from Brazilian coastal waters so far (Figure 1, Table 1). Except for A. catenella and A. fraterculus, which were reported for different locations in Rio Grande do Sul and Santa Catarina (southern Brazil), the other five species were recorded only once for the northeast (A. tamiyavanichi), southeast (Alexandrium sp., A. tamutum), and south (Alexandrium gaardnerae and A. kutnerae) parts of the country. This is the first report of A. tamutum for the South Atlantic.
Morphology
Alexandrium catenella (Figures 2A,B)
Cells isodiametric (40–50 μm long, 30–50 μm wide). The first apical plate (1′) was irregularly rhomboidal connected direct or indirectly to the apical pore (Po). The third apical plate (3′) was asymmetrical and the ventral pore (v.p.) was always located along the margin between plate 1′ and plate 4′. The posterior sulcal plate (s.p.) was always pentagonal without a connecting pore.
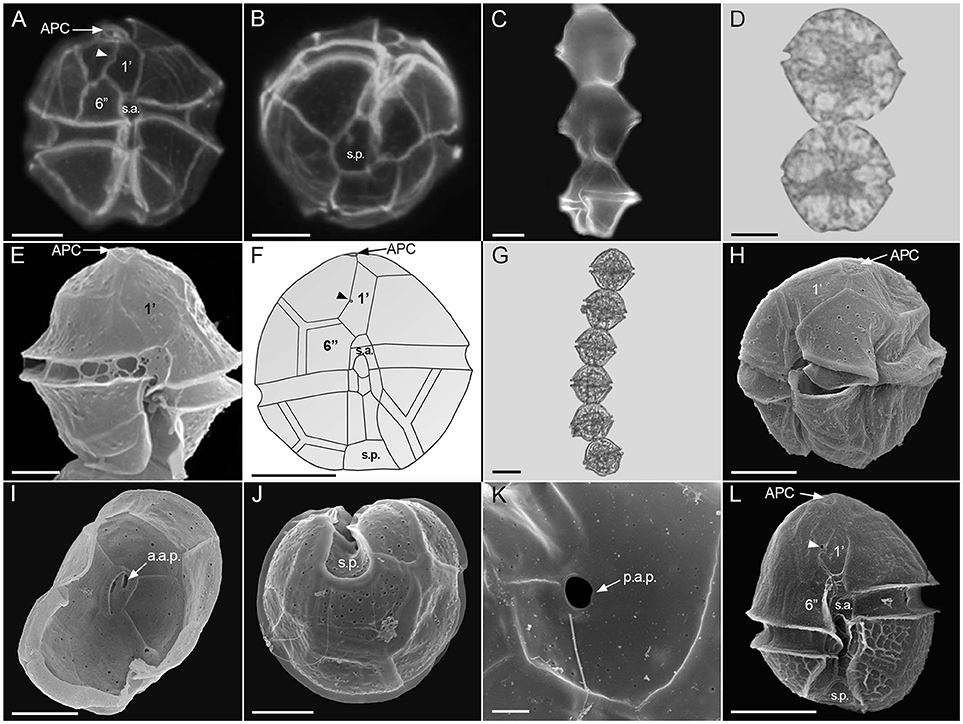
Figure 2. Alexandrium species recorded from Brazil. Epifluorescence micrographs (A–C), light micrographs (D,G), scanning electron micrographs (E,H–L), and drawing (F). A. catenella in ventral view showing ventral pore (arrowhead) (A) and antapical view (B) A. fraterculus chains, (C,D) and cell in ventral view (E), A. kutnerae in ventral view showing ventral pore (arrowhead) (F), A. tamiyavanichi chain (G), latero-ventral view (H), epitheca inside showing apical pore complex with anterior attachment pore (a.a.p.) (I), antapical view (J), posterior sulcal plate with a large posterior attachment pore (p.a.p.) (K), Alexandrium sp. in ventral view showing ventral pore (arrowhead) (L). Scale = 10 μm (A,B,E,F,H–J,L), 20 μm (C,D,G), 2 μm (K). APC, apical pore complex; 1′, first apical plate; 6′′, sixth precingular plate; s.a., anterior sulcal plate; s.p., posterior sulcal plate; a.a.p., anterior attachment pore; p.a.p, posterior attachment pore. The drawing of A. kutnerae was based on Balech (1995).
The morphology of the three isolates agreed with the description reported by Balech (1995) for the morphospecies A. tamarense. No morphological variability regarding presence/absence of the both v.p. and s.p. connecting pore. Although previously identified as A. tamarense (Persich et al., 2006), these three isolates along with others from South and North America (A. tamarense complex group I, sensu John et al., 2014) should be currently named as A. catenella (Prud'homme van Reine, 2017). Besides the three isolates analyzed in this study, Persich et al. (2006) analyzed the toxin content of ten more strains isolated from the same locality (Patos Lagoon estuary) (Table 1). These isolates showed toxicity from 7.07 to 65.92 pg STX eq cell−1 and total toxin content ranged from 42 to 199 fmol cell−1. Toxin C1,2 was predominant in most of the strains, comprising up to 78.4% of the total toxins (Persich et al., 2006). More recently, high concentrations of PSP toxins (601.13 to 3.199.6 μg STX eq.kg−1) were detected in bivalves (Perna perna and Crassostera gigas) on October 2017 from several coastal locations in Santa Catarina (http://www.cidasc.sc.gov.br/defesasanitariaanimal/). These PSP levels were associated with the presence of A. tamarense morphospecies complex in the plankton (7,350–11,750 cells L−1) and lead to the closure of all mariculture farms in the area for ~2 months. Although several toxic Alexandrium species have been previously reported in Brazil, this is the first record of high PSP toxins in shellfish associated to a species of this genus.
Alexandrium fraterculus (Figures 2C–E)
Chains formed by of 2–8 isodiametric, pentagonal cells (30–40 μm long, 30–40 μm wide). The first apical plate (1′) was directly attached to the Po. The ventral pore (v.p.) was located in the middle of the right margin, in the suture with the fourth apical plate (4′). The posterior sulcal plate (s.p.) was isodiametric, rhomboidal with a large connecting pore located in the center of the plate. The anterior sulcal plate (s.a.) was slightly longer than it was wide, with a deep posterior sinus.
No toxicity was found in the analyzed strain (L.A.O. Proença, personal communication).
Alexandrium gaarderae
This species was reported by Balech (1995) based on environmental samples from the Brazilian coast as A. concavum (locality not informed), along with a description but no illustrations. Cells were 50–81 μm long, 45–64.5 μm wide. The first apical plate (1′) was rhomboidal, connected indirectly or directly to the apical pore (Po). A ventral pore (v.p.) occurred enclosed within the right margin of 1′. The posterior sulcal plate (s.p.) was longer than wide, symmetrical.
Alexandrium kutnerae (Figure 2F)
This species was described by Balech (1995) as having rounded cells, 51–65 long, 41–57 wide. The first apical plate (1′) is connected directly or indirectly to the apical pore (Po). A ventral pore (v.p.) occurs inside of the first apical plate (1′). The anterior sulcal plate (s.a.) had a quadrangular or hexagonal precingular part (p.pr.). The posterior sulcal plate (s.p.) was longer than wide, symmetrical, without an attachment pore.
Alexandrium tamiyavanichi (Figures 2G–K)
This species was characterized by Menezes et al. (2010) as having small and rounded cells, sometimes slightly wider than long, 31–41 μm long and 26–35 μm width. A small ventral pore (v.p.) occurred within the right margin on the first apical plate (1′). The anterior sulcal plate (s.a.) showed a triangular precingular part (p.pr). The posterior sulcal plate (s.p.) was longer than wide, symmetrical, with an attachment pore.
Six PSP toxins have been identified for one A. tamiyavanichi strain (A1PSA): STX, Neo-STX, GTX4, GTX3, dcGTX2, and dcGTX3. STX was the main toxin (16.85 fmol. cell−1), accounting for 67.06%) of the overall cell toxin content (Menezes et al., 2010).
Alexandrium tamutum (Figures 3A–J)
Cells were ovate to spherical, 20.1–35.0 μm long, and 19.2–33.6 μm wide. The thecae surface was smooth and very thin. The first apical plate (1′) was rhomboidal with a direct connection to the APC and posterior margin straight. A ventral pore was present on the anterior right margin of the 1′ plate. The sixth precingular (6′′) plate was pentagonal, longer than wide or wider than long. Apical pore complex (APC) with comma-shaped. The anterior sulcal plate (s.a.) was longer than wide with anterior margin straight and posterior margin concave, often extending to the epitheca. The posterior sulcal (s.p.) plate was wider than long, asymmetrical, and without a posterior attachment pore.
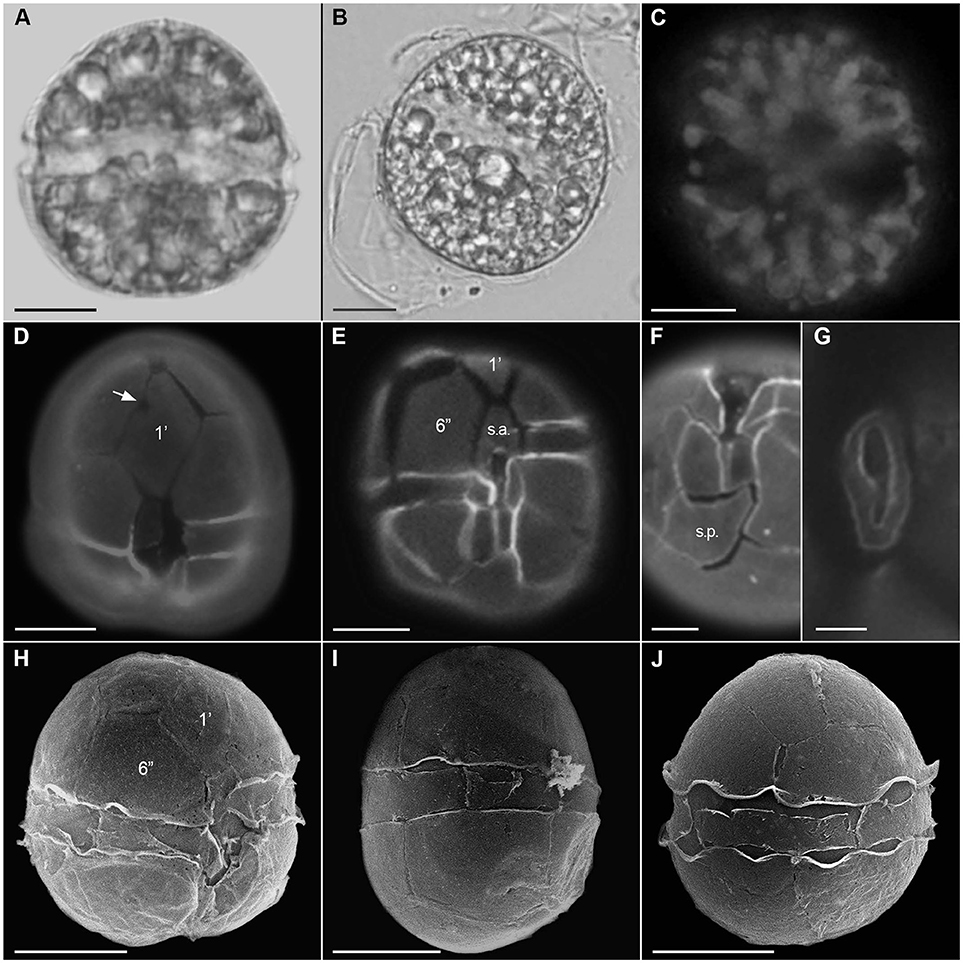
Figure 3. Alexandrium tamutum. Light micrographs (A,B), epifluorescence micrographs (C–G), and scanning electron micrographs (H–J). Live cell (A), cyst (B), chloroplast autofluorescence (C), ventral view (D,E), showing the firth apical plate (1′) with ventral pore (arrow), sixth precingular plate (6′′), and anterior sulcal plate (s.a.), antapical view showing posterior sulcal plate (s.p.) (F), apical pore complex (G), ventral view showing the firth apical plate (1′) and large sixth precingular plate (6′′); lateral view (I), and dorsal view (J). Scale = 10 μm (A–E,H–J), 5 μm (F), 2 μm (G).
The three analyzed strains presented cell morphology matching with the original description of the species (Montresor et al., 2004).
Alexandrium sp. (Figure 2L)
Cells were usually solitary, sometimes forming short chains of up to four ovate to irregularly elliptical cells (17.1)19–31.2(32.3) μm long, (15.2)17.1–31.2(32.3) μm wide. The sixth precingular (6′′) plate was generally pentagonal, longer than wide, sometimes as long as wide. The first apical plate (1′) showed a clear ventral pore and connected directly or through a channel with the Po plate. Epitheca surface was smooth whereas hypotheca surface showed strong reticulation.
This species was at first identified as A. minutum by Menezes et al. (2007) based on samples obtained from a bloom in 2007 from the Rio de Janeiro coast. However, DNA sequencing (see Phylogenetic Analysis section) of strains recently isolated from Guanabara bay depicting the same unusual theca ornamentation pattern (epitheca smooth and hypotheca strongly reticulated) indicated that both populations belong to a new Alexandrium species, currently under description. Four analogous of STX have been found in the cells from the 2007's bloom: Neo-STX, STX, GTX4, and GTX2 (Menezes et al., 2007). Neo-STX was the dominant toxin, accounting to 83.47% of STX equivalents (Menezes et al., 2007).
Phylogenetic Analyses
Maximum-likelihood (ML) and BI analyses based on ITS and LSU rDNA resulted in phylogenetic trees with similar topologies (Figures 4, 5). All phylogenies exhibited two main clades: (i) a clade composed of the A. tamarense complex (e.g., A. catenella, Alexandrium fundyense, Alexandrium mediterraneum, A. tamarense, Alexandrium australiense, Alexandrium pacificum), A. tamiyavanichi, A. fraterculus, and Alexandrium affine sequences (ML = 86% and BI = 1.0 for ITS rDNA; ML = 100% and BI = 1.0 for LSU rDNA), and (ii) a clade composed of Alexandrium insuetum, A. minutum, A. ostenfeldii, A. tamutum, and Alexandrium sp. sequences (ML = 72% and BI = 0.98 for ITS rDNA; ML and BI < 0.5 for LSU rDNA).
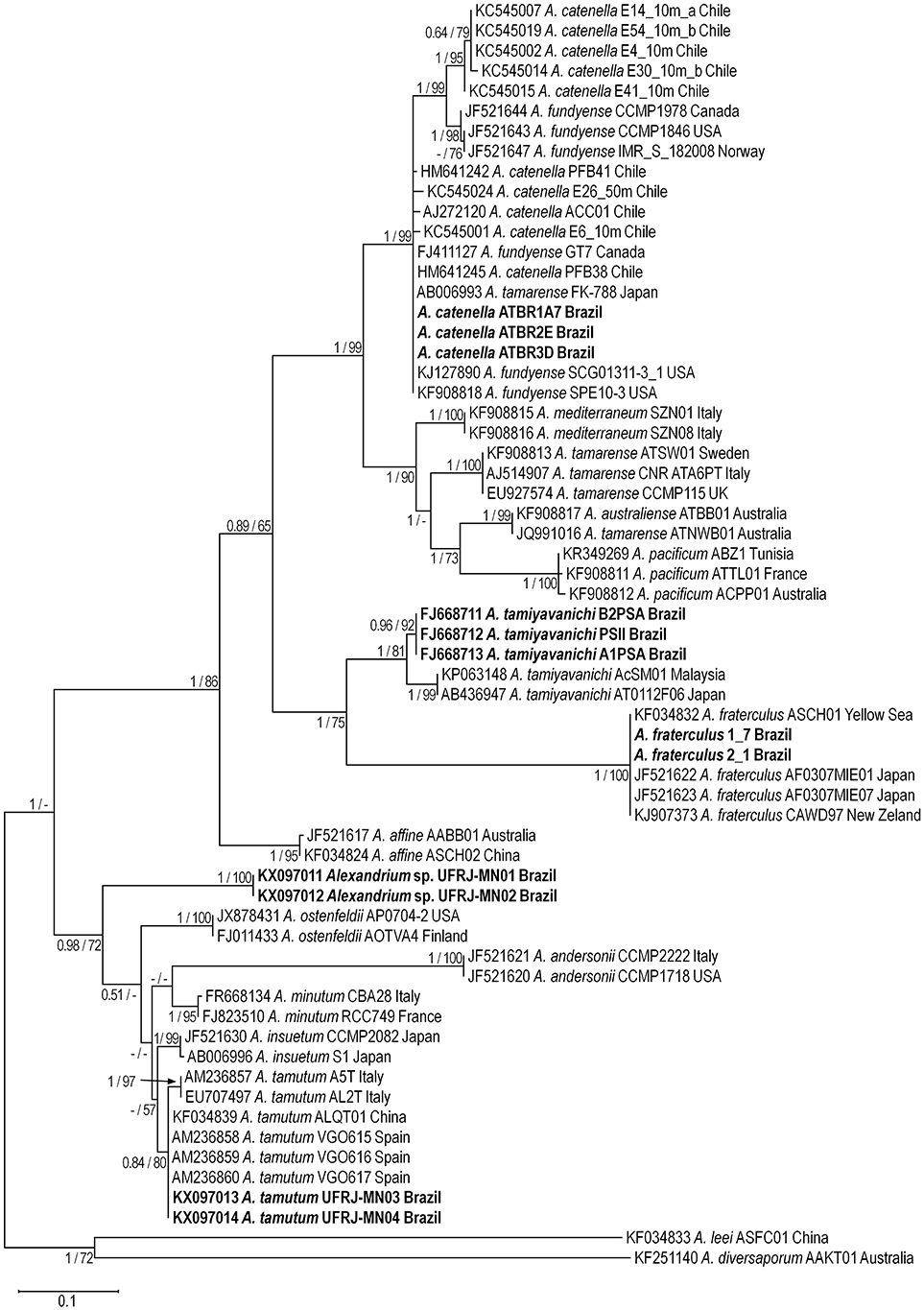
Figure 4. Maximum Likelihood tree based on ITS rDNA sequences. Alexandrium sequences from Brazil are shown in bold. Numbers at nodes are bootstrap and posterior probabilities values for Maximum Likelihood and Bayesian Inference analysis, respectively. Only values >50% (ML) and 0.50 (BI) are shown.
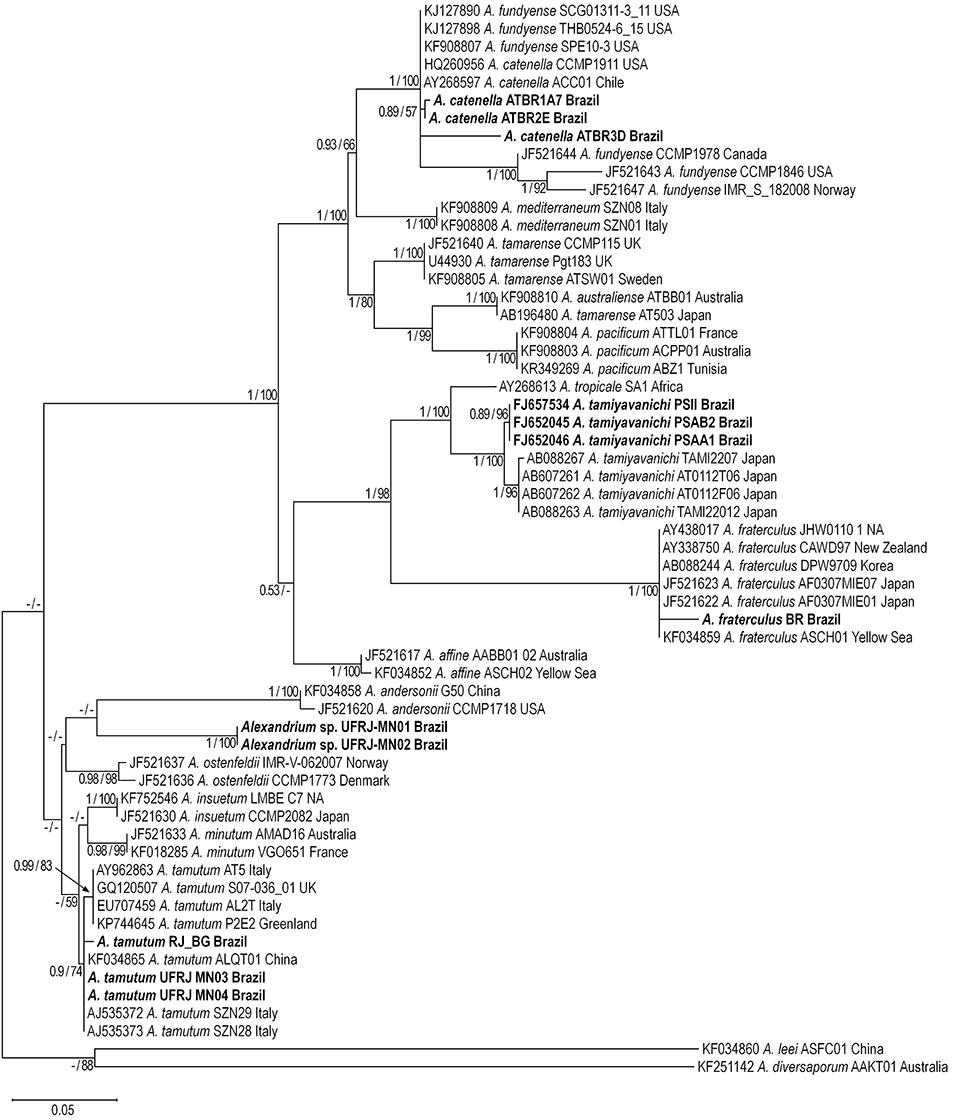
Figure 5. Maximum Likelihood tree based on LSU rDNA sequences. Alexandrium sequences from Brazil are shown in bold. Numbers at nodes are bootstrap and posterior probabilities values for Maximum Likelihood and Bayesian Inference analysis, respectively Only values >50% (ML) and 0.50 (BI) are shown.
The A. tamarense complex formed a large monophyletic group made up by sequences of A. catenella, A. mediterraneum, A. tamarense, A. australiense, and A. pacificum (ML = 99% and BI = 1.0 for ITS; ML = 100% and BI = 1.0 for LSU rDNA). Alexandrium catenella sequences from Brazil grouped with sequences of this species from USA and Chile, North America (USA and Canada), and Japan (ML = 99% and BI = 1.0 for ITS rDNA; ML = 100% and BI = 1.0 for LSU rDNA). Two subclades were formed inside the A. catenella clade in trees based on ITS rDNA: a clade composed only by sequences from Chile (ML = 99% and BI = 1.0) and a clade composed with sequences from Canada, USA and Norway (ML = 98% and BI = 1.0). The latter subclade was also recognized inside the A. catenella clade formed in the trees based on LSU rDNA (ML = 100% and BI = 1.0).
Alexandrium tamiyavanichi formed a monophyletic clade consisting of two different subclades. The first subclade was formed only by sequences from Brazil (ML = 92% and BI = 0.96 for ITS rDNA; ML = 96% and BI = 0.89 for LSU rDNA rDNA) whereas the second subclade included sequences from Japan and Malaysia (ML = 99% and BI = 1.0 for ITS rDNA; ML = 96% and BI = 1.0 for LSU rDNA). Alexandrium tropicale formed a sister group of A. tamiyavanichi in the trees based on LSU rDNA (ML = 100% and BI = 1.0). The A. fraterculus clade grouped with the A. tamiyavanichi clade (ML = 75% and BI = 1.0 ITS rDNA trees; ML = 98% and BI = 1.0 for LSU rDNA) and included sequences from Brazil, New Zealand, Yellow Sea, Japan, and Korea (ML = 100% and BI = 1.0 for ITS and LSU rDNA).
Alexandrium tamutum sequences formed a monophyletic group (ML = 80% and BI = 0.84 for ITS; ML = 74% and BI = 0.9 for LSU). A subclade with a small branch composed of sequences from Italy was observed inside the A. tamutum clade in trees based on ITS rDNA (ML = 97% and BI = 1.0) whereas Brazilian strains grouped with sequences from Italy, Greenland, and United Kingdom (ML = 99% and BI = 0.83). Alexandrium sp. sequences formed a monophyletic group (ML = 99% and BI = 1.0 for ITS; ML = 100% and BI = 1.0 for LSU) clearly separated from other Alexandrium clades.
Discussion
Alexandrium species reported from Brazilian coastal waters so far have been identified based mostly on traditional morphological features, such as plate patterns, cell size and shape, presence and localization of the ventral pore, presence of a sulcal pore, and chain formation. These morphological traits, however, are widely variable often with overlapping or transitional forms between species and therefore are shown to be less robust for species discrimination (e.g. Lilly et al., 2005; Anderson et al., 2012).
Alexandrium gaarderae and A. kutnerae have been reported only once from Brazilian coastal waters (Balech, 1995). In addition to Brazil, A. gaarderae has been recorded from Argentina, Gulf of Mexico, the Caribbean Sea, and Vietnam (Balech, 1995; Larsen and Nguyen-Ngoc, 2004), whereas A. kutnerae has records for Argentina and Western Mediterranean (Balech, 1995; Bravo et al., 2006). While A. gaarderae has a distinctive cell shape characterized by a conical epitheca (Larsen and Nguyen-Ngoc, 2004), the general cell appearance of A. kutnerae (Figure 2F) reminds as observed in the A. tamarense complex. The presence of a pre-cingular part in the sulcal plate (s.p.) in A. kutnerae is the main morphological characteristic separating this species from those of the A. tamarense complex. However, it is not clear if this feature is discriminative enough to keep the former apart from representatives of the A. tamarense complex. As a matter of fact, phylogenetic analysis including ITS sequences of an isolate of A. kutnerae from the Catalan Sea (Spain) indicated this species belongs to the A. pacificum clade (Group IV, sensu John et al., 2014), with a high support value (Gu, 2011).
Taxonomic and phylogenetic reviews of the genus Alexandrium revealed several cryptic species in this genus, not only invalidating some of the described species in the literature but also precluding the application of a morphospecies concept for the species circumscriptions (Lilly et al., 2007; Miranda et al., 2012; John et al., 2014; Wang et al., 2014). An example of that was observed in the A. tamarense complex (formed by the morphospecies A. tamarense, A. catenella, and A. fundyense), where species were segregated into five genetic distinct clades, each one corresponding to a different cryptic species: A. catenella (Group I), A. mediterraneum (Group II), A. tamarense (Group III), A. pacificum (Group IV), and A. australiense (Group V) (Lilly et al., 2007; Miranda et al., 2012; John et al., 2014; Wang et al., 2014).
In our morphological analysis, strains of A. catenella (Group I sensu John et al., 2014; formerly identified as the morphospecies A. tamarense) presented cells always with a ventral pore, which is consistent with the A. tamarense morphospecies description provided by Balech (1995). According to this author, the presence of a ventral pore in the morphospecies A. tamarense constitutes a diacritic character separating this morphospecies from A. catenella and A. fundyense (the latter two morphospecies lacking this feature). Stability in the presence of a ventral pore has been observed in strains of the morphospecies A. tamarense from Australia (MacKenzie et al., 2004), Europe (Thau Lagoon) (Genovesi et al., 2011), and from western Greenland (Tillmann et al., 2016), the former one corresponding to Group IV (now A. pacificum) and the latter two to Group III (now A. tamarense). In contrast, many studies based on wild populations and cultures have demonstrated intermediate morphology between A. catenella, A. fundyense, and A. tamarense morphospecies, regarding the presence/absence of a ventral pore and/or chain formation (for more details see Orlova et al., 2007; Wang et al., 2008, 2014; John et al., 2014).
Phenotypic plasticity and genetic variability has been reported for A. catenella strains (Group I) from Chile, Mediterranean Sea, and Japan (Penna et al., 2005; Cruzat et al., 2018). Alpermann et al. (2009) reported phenotypic and genetic variability in A. tamarense isolates (Group III) from the North Sea coast of Scotland that were interpreted, respectively, as having strong potential for adaptability of the population and evidence for a lack of strong selective pressure on respective phenotypic traits at the time the population was sampled. Logares et al. (2007, 2009) reported almost identical DNA sequences for the dinoflagellate morphospecies Peridinium aciculiferum and Scripsiella hangoei, which are well-differentiated based on morphology (Logares et al., 2007, 2009). Similar results were reported for P. aciculiferum, Peridinium euryceps, and Peridinium baicalense by Annenkova et al. (2015). These authors hypothesized that identical sequences do not necessarily mean the same microbial species, and these taxa are not a case of phenotypic plasticity but originated via recent rapid diversification (radiation) into several species followed by dispersion to environments with different ecological conditions (Logares et al., 2007, 2009; Annenkova et al., 2015).
Phenotypic plasticity is a single genotype's ability to produce variable phenotypes in response to environmental conditions thereby enabling it to buy additional time for adaptation to occur (Sunday et al., 2014) and provide a mechanism for adaptation to occur rapidly (Pigliucci et al., 2006; Ghalambor et al., 2015). Although it is recognized that phenotypic plasticity can increase organism survival under specific conditions, there is no consensus on its effects on the organisms: whether they drive the evolution of novel traits and promote taxonomic diversity, or whether they act more on acceleration or evolutionary changes (Pfennig and Pfennig, 2010). Little is known about the extent of the phenotypic plasticity in Alexandrium. Studies on the phenotypic plasticity on this genus should be addressed aiming to respond to questions such as: Could this phenotypic plasticity be a pre-adaptation or genetic shift after an invasive process of this genus to different localities? Could the acclimatization process cause definitive change in morphology?
The phylogenetic analysis of ITS and LSU rDNA sequences corroborated that the isolates of A. catenella from southern Brazil belong to the clade formed by A. catenella (Group I, sensu John et al., 2014), along with other isolates from Chile, Japan, Norway and North America, as already pointed out by Persich et al. (2006). The phylogeny resulted from ITS rDNA sequences also confirmed the existence of genetic diversity within this clade (Cruzat et al., 2018) with a subclade formed by some isolates from Chile and a second subclade formed by isolates from the United States, Canada and Norway. The presence of the Group I in South America has been hypothesized as being a result of ballast water introduction (invasive species) or natural dispersion (see Lilly et al., 2007).
According to John et al. (2003), the ancestral population of Alexandrium was globally distributed, diverging into the ancestors of Groups I and II that occurred in waters off North America and in the tropical Atlantic. Posteriorly, Group II evolved in the tropical Atlantic, becoming distinct from the Group I populations and later colonizing the Mediterranean (John et al., 2003). There is evidence that Group I populations were transported to the west coast of South America by a natural dispersal mechanism during a period in which the weather was substantially cooler than nowadays, e.g., as in one of the recent ice ages (Persich et al., 2006; Lilly et al., 2007). However, the presence of Group I on the east Atlantic coast of South America is hypothesized to have resulted recently from Chilean populations, given the similarity of the LSU rDAN sequences (D1–D2) among the isolates from both sites (Persich et al., 2006; Lilly et al., 2007), as corroborated by the our phylogeny results based on ITS rDNA. Although Persich et al. (2006) suggested the possibility that A. catenella (Group I) is spreading northward by current ocean circulation patterns, environmental changes due to global warming would likely explain the recent spread of A. catenella to the east coast of South America (Lilly et al., 2007).
Alexandrium tamiyavanichi from northeastern Brazilian coast was included in a distinct clade along with isolates from Japan and other Asian locations with medium support value, with the Brazilian population diverging earlier (Menezes et al., 2010). One of the hypotheses that seems plausible to explain the presence of the species in Brazil is due to an invasion event that could not be recent or occurred several times (Menezes et al., 2010). Alexandrium tamiyavanichi is a tropical species but it also occurs in warm-temperate regions such as Japan, Baja California, and South Africa (Sierra-Beltrán et al., 1998; Ruiz-Sebastián et al., 2005; Nagai et al., 2011). The origin of this species in Brazil could be explain by closure of Tethys Ocean and the emergence of the Isthmus of Panama that formed a land bridge between the two American continents and its final closure when there was a final interruption of the Atlantic and Pacific oceans' connection disrupting the flow of genes of many species (Hou and Li, 2018). On the other hand, after the closure of Tethys Ocean the dispersal between the Atlantic and Indo-Pacific could have occurred via southern Africa during interglacial periods (Bowen et al., 2006). These same hypotheses could be applied to A. fraterculus that segregated with isolates from Japan, Korea, China, and New Zealand, forming a sister clade of A. tamiyavanichi.
Data on the geographical distribution of A. tamutum are still scarce. Previously recorded as Alexandrium sp. for the northwest Pacific (Yoshida, 2002), A. tamutum was formally described based on material isolated from the Mediterranean (Montresor et al., 2004). Since then, the species has been reported in morphological studies of populations from Russia, Malaysia, and Scotland (Selina and Morozova, 2005; Hii et al., 2012; Swan and Davidson, 2014) and in morphological and genetic studies of populations from Ireland, Shetland, China, Malaysia, Greenland (Touzet et al., 2008; Brown et al., 2010; Gu et al., 2013; Kon et al., 2013; Tillmann et al., 2016). Our results demonstrated a genetic variability within the A. tamutum clade, which was formed by some subclades, including the Brazilian subclade. Genetic variability between isolates from different geographical areas of A. tamutum based on LSU and ITS rDNA has been reported by Montresor et al. (2004), Penna et al. (2008) and Tillmann et al. (2016). However, more sequences from distinct locations are necessary to confirm the occurrence of a geographical partitioning of A. tamutum species. The two isolates of Alexandrium sp. formed a monophyletic clade, however it is necessary for more sequences to make any inference about its geographical distribution. These populations have been found in Guanabara Bay and constitute a new species for the science (currently under description), related to A. minutum and A. tamutum.
This review constitutes the first approach on the occurrence of the Alexandrium genus in Brazilian coastal waters. The number of species recorded was very incipient considering the 7,491 km of the Brazilian coast, with the new record of A. tamutum and the discovery of a new species (Alexandrium sp.) indicating a wide gap in knowledge on the genus diversity in the country. Brazil has a Program for the National Sanitary Control of Bivalve Molluscs (PNCMB) established in 2011 designed to monitor for the presence of toxins in seafood and the presence of harmful algae in the coastal area. Although it is a national program, until now it has been implemented only in Santa Catarina. The presence of toxic Alexandrium strains in other areas on the country points out to the need of expand the current coverage of the PNCMB and improve our ability to identify causative species of HABs through training courses and international technical and research collaborations.
Author Contributions
All authors listed have made a substantial, direct and intellectual contribution to the work, and approved it for publication.
Funding
This study was supported by Conselho Nacional de Desenvolvimento Científico e Tecnológico (CNPq, No. 503443/2012-3 and 471303/2013-5), by Coordenação de Aperfeiçoamento de Pessoal de Nível Superior (CAPES, No. 562283/2010-2), and Marbionc Program at UNC Wilmington (State of North Carolina, USA).
Conflict of Interest Statement
The authors declare that the research was conducted in the absence of any commercial or financial relationships that could be construed as a potential conflict of interest.
Acknowledgments
To Dr. Don Anderson (Woods Hole Oceanographic Institution) who kindly provided the three A. catenella strains used in this study. To the Institute of Biophysics Carlos Chagas Filho/UFRJ for facilitating access to their scanning electron microscope.
Supplementary Material
The Supplementary Material for this article can be found online at: https://www.frontiersin.org/articles/10.3389/fmars.2018.00421/full#supplementary-material
References
Ajani, P., Harwood, D. T., and Murray, S. A. (2017). Recent trends in marine phycotoxins from Australian coastal waters. Mar.Drugs 15, 1–33. doi: 10.3390/md15020033
Alpermann, T. J., Tillmann, U., Beszteri, B., Cembella, A. D., and John, U. (2009). Phenotypic variation and genotypic diversity in a planktonic population of the toxigenic marine dinoflagellate Alexandrium tamarense (Dinophyceae) 1. J. Phycol. 46, 18–32. doi: 10.1111/j.1529-8817.2009.00767.x
Alves-de-Souza, C., Cornet, C., Nowaczyk, A., Gasparini, S., and Guillou, L. (2011). Blastodinium spp. infect copepods in the ultra-oligotrophic marine waters of the Mediterranean sea. Biogeosci. Disc. 8, 1–12. doi: 10.5194/bgd-8-2563-2011
Anderson, C. R., Moore, S. K., Tomlinson, M. C., Silke, J., and Cusack, C. K. (2015). “Living with harmful algal blooms in a changing world: strategies for modeling and mitigating their effects in coastal marine ecosystems,” in The Coastal and Marine Hazards, Risks, and Disasters, eds J. F. Shroder, J. T. Ellis, and D. J. Sherman (Amsterdan: Elsevier), 495–561.
Anderson, D. M. (2007). The Ecology and Oceanography of Harmful Algal Blooms: Multidisciplinar Approaches to Research and Management. IOC Bruun Memorial Lectures, Technical Series 10. Paris: UNESCO.
Anderson, D. M. (2017). “Harmful algal blooms”, in Harmful Algal Blooms (HABs) and Desalination: A Guide to Impacts, Monitoring and Management, IOC Manuals and Guides No. 78, eds D. M. Anderson, S. F. E. Boerlage, and M. Dixon (Paris: Intergovernmental Oceanographic Commission of UNESCO), 17–52.
Anderson, D. M., Cembella, A. D., and Hallegraeff, G. M. (2012). Progress in understanding harmful algal blooms: paradigm shifts and new technologies for research, monitoring, and management. Annu. Rev. Mar. Sci. 4, 143–176. doi: 10.1146/annurev-marine-120308-081121
Annenkova, N. V., Hansen, G., Moestrup, Ø., and Rengefors, K. (2015). Recent radiation in a marine and freshwater dinoflagellate species flock. The ISME J. 9, 1821–1834. doi: 10.1038/ismej.2014.267
Balech, E. (1995). The Genus Alexandrium Halim (Dinoflagellata). Cork: Sherkin Island Marine Station.
Bowen, B. W., Muss, A., Rocha, L. A., and Grant, W. S. (2006). Shallow mtDNA coalescence in Atlantic pygmy angelfishes (genus Centropyge) indicates a recent invasion from the Indian Ocean. J. Hered. 97, 1–12. doi: 10.1093/jhered/esj006
Bravo, I., Garcés, E., Diogène, J., Fraga, S., Sampedro, N., and Figueroa, R. I. (2006). Resting cysts of the toxigenic dinoflagellate genus Alexandrium in recent sediments from the Western Mediterranean coast, including the first description of cysts of A. kutnerae and A. peruvianum. Eur. J. Phycol. 41, 293–302. doi: 10.1080/09670260600810360
Brown, L., Bresnan, E., Graham, J., Lacaze, J. P., Turrell, E., and Collins, C. (2010). Distribution, diversity and toxin composition of the genus Alexandrium (Dinophyceae) in Scottish waters. Eur. J. Phycol. 45, 375–393. doi: 10.1080/09670262.2010.495164
Cruzat, F. A., Muñoz, C., González-Saldía, R. R., Inostroza, A., and Andree, K. B. (2018). High genetic variability of Alexandrium catenella directly detected in environmental samples from the Southern Austral Ecosystem of Chile. Mar. Pollut. Bull. 127, 437–444. doi: 10.1016/j.marpolbul.2017.12.022
Deeds, J., Landsberg, J., Etheridge, S., Pitcher, G., and Longan, S. (2008). Non-traditional vectors for paralytic shellfish poisoning. Mar. Drugs 6, 308–348. doi: 10.3390/md6020308
Etheridge, S. M. (2010). Paralytic shellfish poisoning: seafood safety and human health perspectives. Toxicon 56, 108–122. doi: 10.1016/j.toxicon.2009.12.013
Fabro, E., Almandoz, G. O., Ferrario, M., John, U., Tillmann, U., Toebe, K., et al. (2017). Morphological, molecular, and toxin analysis of field populations of Alexandrium genus from the Argentine Sea. J. Phycol. 53, 1206–1222. doi: 10.1111/jpy.12574
Fritz, L., and Triemer, R. E. (1985). A rapid simple technique utilizing Calcofluor white M2R for the visualization of dinoflagellate thecal plates. J. Phycol. 21, 662–664. doi: 10.1111/j.0022-3646.1985.00662.x
Genovesi, B., Shin-Grzebyk, M.-S., Grzebyk, D., Laabir, M., Gagnaire, P.-A., Vaquer, A., et al. (2011). Assessment of cryptic species diversity within blooms and cyst bank of the Alexandrium tamarense complex (Dinophyceae) in a Mediterranean lagoon facilitated by semi-multiplex PCR. J. Plank. Res. 33, 405–414. doi: 10.1093/plankt/fbq127
Ghalambor, C. K., Hoke, K. L., Ruell, E. W., Fischer, E. K., Reznick, D. N., and Hughes, K. A. (2015). Non-adaptive plasticity potentiates rapid adaptive evolution of gene expression in nature. Nature 525, 372–375. doi: 10.1038/nature15256
Gobler, C. J., Doherty, O. M., Hattenrath-Lehmann, T. K., Griffith, A. W., Kang, Y., and Litaker, R. W. (2017). Ocean warming since 1982 has expanded the niche of toxic algal blooms in the North Atlantic and North Pacific oceans. Proc. Natl. Acad. Sci. U.S.A. 114, 4975–4980. doi: 10.1073/pnas.1619575114
Gu, H. (2011). Morphology, phylogenetic position, and ecophysiology of Alexandrium ostenfeldii (Dinophyceae) from the Bohai Sea, China. J. Syst. Evol. 49, 606–616. doi: 10.1111/j.1759-6831.2011.00160.x
Gu, H., Zeng, N., Liu, T., Yang, W., Müller, A., and Krock, B. (2013). Morphology, toxicity, and phylogeny of Alexandrium (Dinophyceae) species along the coast of China. Harmful Algae 27, 68–81. doi: 10.1016/j.hal.2013.05.008
Hii, K.-S., Tan, T. H., Leaw, C. P., Chai, H. C., Takata, Y., and Lim, P. T. (2012). “Occurence of three Alexandrium species, A. affine, A. tamutum and A. tamiyavanichii in kuching waters,” in Sustaining Tropical Natural Resources Through Innovations, Technologies and Practice, Proceedings NTrop4, eds M. E. Wasli, H. Sani, F. B. Ahmed, S. L. P. Mohamad Teen, L. K. Soon, and M. Sidi (Sarawaka), 165–171.
Hou, Z., and Li, S. (2018). Tethyan changes shaped aquatic diversification. Biol. Rev. 93, 874–896. doi: 10.1111/brv.12376
John, U., Fensome, R. A., and Medlin, L. K. (2003). The application of a molecular clock based on molecular sequences and the fossil record to explain biogeographic distributions within the Alexandrium tamarense “species complex”(Dinophyceae). Mol. Biol. Evol. 20, 1015–1027. doi: 10.1093/molbev/msg105
John, U., Litaker, R. W., Montresor, M., Murray, S., Brosnahan, M. L., and Anderson, D. M. (2014). Formal revision of the Alexandrium tamarense species complex (Dinophyceae) taxonomy: the introduction of five species with emphasis on molecular-based (rDNA) classification. Protist 165, 779–804. doi: 10.1016/j.protis.2014.10.001
Juliano, V. B., and Garcia, V. M. T. (2006). Cysts of potentially harmful dinoflagellates, with emphasis of the genus Alexandrium, in sepetiba bay (Brazil) during a port survey of Globallast. Afr. J. Mar. Sci. 28, 299–303. doi: 10.2989/18142320609504166
Karydis, M., and Kitsiou, D. (2012). Eutrophication and environmental policy in the Mediterranean Sea: a review. Environ. Monit. Assess. 184, 4931–4984. doi: 10.1007/s10661-011-2313-2
Kodama, M. (2010). Paralytic shellfish poisoning toxins: biochemistry and origin. Aqua Biosci. Monogr. 3, 1–38. doi: 10.5047/absm.2010.00301.0001
Kon, N. F., Hii, K. S., Tan, T. H., Yek, L. H., Lim, P. T., and Leaw, C. P. (2013). First record of marine dinoflagellate, Alexandrium tamutum (Dinophyceae) from Malaysia. Malays. J. Sci. 32, 81–88. doi: 10.22452/mjs.vol32no3.7
Kumar, S., Stecher, G., and Tamura, K. (2016). MEGA7: molecular evolutionary genetics analysis version 7.0 for bigger datasets. Mol. Biol. Evol. 33, 1870–1874. doi: 10.1093/molbev/msw054
Larsen, J., and Nguyen-Ngoc, L. (2004). Potentially toxic macroalgae of Vietnamese waters. Opera Bot. 140, 5–216.
Lebret, K., Kritzberg, E. S., Figueroa, R., and Rengefors, K. (2012). Genetic diversity within and genetic differentiation between blooms of a microalgal species. Environ. Microbiol. 14, 2395–2404. doi: 10.1111/j.1462-2920.2012.02769.x
Lilly, E. L., Halanych, K. M., and Anderson, D. M. (2005). Phylogeny, biogeography, and species boundaries within the Alexandrium minutum group. Harmful Algae 4, 1004–1020. doi: 10.1016/j.hal.2005.02.001
Lilly, E. L., Halanych, K. M., and Anderson, D. M. (2007). Species boundaries and global biogeography of the Alexandrium tamarense complex (Dinophyceae). J. Phycol. 43, 1329–1338. doi: 10.1111/j.1529-8817.2007.00420.x
Litaker, R. W., Vandersea, M. W., Kibler, S. R., Reece, K. S., Stokes, N. A., Steidinger, K. A., et al. (2003). Identification of Pfiesteria piscicida (Dinophyceae) and Pfiesteria-like organisms using internal transcribed spacer-specific PCR assays. J. Phycol. 39, 754–761. doi: 10.1046/j.1529-8817.2003.02112.x
Logares, R., Bråte, J., Bertilsson, S., Clasen, J. L., Shalchian-Tabrizi, K., and Rengefors, K. (2009). Infrequent marine-freshwater transitions in the microbial world. Trends Microbiol. 17, 414–422. doi: 10.1016/j.tim.2009.05.010
Logares, R., Rengefors, K., Kremp, A., Shalchian-Tabrizi, K., Boltovskoy, A., Tengs, T., et al. (2007). Phenotypically different microalgal morphospecies with identical ribosomal DNA: a case of rapid adaptive evolution. Microb. Ecol. 53, 549–561. doi: 10.1007/s00248-006-9088-y
Lu, D., Qi, Y., Gu, H., Dai, X., Wang, H., Gao, Y., et al. (2014). Causative species of harmful algal blooms in Chinese coastal waters. Algol. Stud. 145, 145–168. doi: 10.1127/1864-1318/2014/0161
MacKenzie, L., de Salas, M., Adamson, J., and Beuzenberg, V. (2004). The dinoflagellate genus Alexandrium (Halim) in New Zealand coastal waters: comparative morphology, toxicity and molecular genetics. Harmful Algae 3, 71–92. doi: 10.1016/j.hal.2003.09.001
Matsuyama, Y., Nishitani, G., and Nagai, S. (2010). “Direct detection of harmful algae from the oyster spat and live fish transporting trailers,” in Proceedings of XIII International Conference on Harmful Algae, eds M. Kin-Chung Ho, J. Zhou and Y. Z. Qi (Hong Kong), 185–189.
Menezes, M., Branco, S., Proença, L. A. O., and Schramm, M. A. (2007). Bloom of Alexandrium minutum Halim on Rio de Janeiro coast: occurrence and toxicity. Harmful Algae News 34, 7–9.
Menezes, M., Varela, D., de Oliveira Proença, L. A., da Silva Tamanaha, M., and Paredes, J. (2010). Identification of the toxic alga Alexandrium tamiyavanichi (Dinophyceae) from Northeastern Brazil: acombined morphological and rDNA sequence (partial lsu and its) approach. J. Phycol. 46, 1239–1251. doi: 10.1111/j.1529-8817.2010.00918.x
Miranda, L. N., Zhuang, Y., Zhang, H., and Lin, S. (2012). Phylogenetic analysis guided by intragenomic SSU rDNA polymorphism refines classification of “Alexandrium tamarense” species complex. Harmful Algae 16, 35–48. doi: 10.1016/j.hal.2012.01.002
Montresor, M., John, U., Beran, A., and Medlin, L. K. (2004). Alexandrium tamutum sp. nov. (Dinophyceae): a new nontoxic species in the genus Alexandrium. J. Phycol. 40, 398–341. doi: 10.1111/j.1529-8817.2004.03060.x
Nagai, S., Lian, C. L., Yamaguchi, S., Hamaguchi, M., Matsuyama, Y., Itakura, S., et al. (2007). Microsatellite markers reveal population genetic structure of the toxic dinoflagellate Alexandrium tamarense (Dinophyceae) in Japanese coastal waters. J. Phycol. 43, 43–54. doi: 10.1111/j.1529-8817.2006.00304.x
Nagai, S., Yoshida, G., and Tarutani, K. (2011). “Changes of species composition and distribution of algae in coastal waters of western Japan,” in Global Warming Impacts - Case Studies on the Economy, Human Health, and on Urban and Natural Environments, ed S. Casalegno (Rijeka: InTech-Open Access Publisher), 209–235.
Natsuike, M., Saito, R., Fujiwara, A., Matsuno, K., Yamaguchi, A., Shiga, N., et al. (2017). Evidence of increased toxic Alexandrium tamarense dinoflagellate blooms in the eastern Bering Sea in the summers of 2004 and 2005. PLoS ONE 12:e0188565. doi: 10.1371/journal.pone.0188565
Odebrecht, C., Mendéz, S., and Garcia, V. M. T. (1997). “Oceanographic processes and HAB in the subtropical southwestern Atlantic (28–368 S),” in Abstracts of the 8th International Conference on Harmful Algae, eds B. Reguera, J. Blanco, M. L. Fernández, and T. Wyatt (Vigo), 152.
Omachi, C. Y., Tamanaha, M. D. S., and Proença, L. A. O. (2007). Bloom of Alexandrium fraterculus in coastal waters off Itajaí, SC, Southern Brazil. Braz. J. Oceanog. 55, 57–61. doi: 10.1590/S1679-87592007000100007
Orlova, T. Y., Selina, M. S., Lilly, E. L., Kulis, D. M., and Anderson, D. M. (2007). Morphogenetic and toxin composition variability of Alexandrium tamarense (Dinophyceae) from the east coast of Russia. Phycologia 46, 534–548. doi: 10.2216/06-17.1
Penna, A., Fraga, S., Masa, M., Giacobbe, M. G., Bravo, I., Vila, M., et al. (2008). Phylogenetic relationships among the Mediterranean Alexandrium (Dinophyceae) species based on sequences of 5.8S gene and internal transcribed spacers of the rRNA operon. Eur. J. Phycol. 43, 163–178. doi: 10.1080/09670260701783730
Penna, A., Garcés, E., Vila, M., Giacobbe, M. G., Fraga, S., Lugli,è, A., et al. (2005). Alexandrium catenella (Dinophyceae), a toxic ribotype expanding in the NW Mediterranean Sea. Mar. Biol. 148, 13–23. doi: 10.1007/s00227-005-0067-5
Persich, G. R., and Garcia, V. M. (2003). Ocorrência de cistos de dinoflagelados, com ênfase em espécies potencialmente nocivas, no sedimento próximo à desembocadura da Laguna dos Patos (RS). Atlântica (Rio Grande) 25, 123–133.
Persich, G. R., Kulis, D. M., Lilly, E. L., Anderson, D. M., and Garcia, V. M. (2006). Probable origin and toxin profile of Alexandrium tamarense (Lebour) Balech from southern Brazil. Harmful Algae 5, 36–44. doi: 10.1016/j.hal.2005.04.002
Pfennig, D. W., and Pfennig, K. S. (2010). Character displacement and the origins of diversity. Am. Nat. 176(Suppl. 1), S26–44. doi: 10.1086/657056
Pigliucci, M., Murren, C. J., and Schlichting, C. D. (2006). Phenotypic plasticity and evolution by genetic assimilation. J. Exp. Biol. 209, 2362–2367. doi: 10.1242/jeb.02070
Prud'homme van Reine, W. F. (2017). Report of the nomenclature committee for algae: 15. Taxon 66, 191–192. doi: 10.12705/661.16
Rhodes, L., and Munday, R. (2016). “Harmful algae and their commercial implications,” in Algae Biotechnology, eds F. Bux and Y. Chisti (Cham: Springer), 301–315. doi: 10.1007/978-3-319-12334-9_15
Ronquist, F., Teslenko, M., Mark, P., Ayres, D. L., Darling, A., Höhna, S., et al. (2012). MrBayes 3.2: efficient Bayesian phylogenetic inference and model choice across a large model space. Syst. Biol. 61, 539–542. doi: 10.1093/sysbio/sys029
Ruiz-Sebastián, C., Etheridge, S. M., Cook, P. A., Oryan, C., and Pitcher, G. C. (2005). Phylogenetic analysis of toxic Alexandrium (Dinophyceae) isolates from South Africa: implications for the global phylogeography of the Alexandrium tamarense species complex. Phycologia 44, 49–60. doi: 10.2216/0031-8884(2005)44[49:PAOTAD]2.0.CO;2
Sato, S., Nishimura, T., Uehara, K., Sakanari, H., Tawong, W., et al. (2011). Phylogeography of Ostreopsis along West Pacific coast, with special reference to a novel clade from Japan. PLoS ONE 6:e27983. doi: 10.1371/journal.pone.0027983
Scholin, C. A., Herzog, M., Sogin, M., and Anderson, D. M. (1994). Identification of group- and strain-specific genetic markers from globally distributed Alexandrium (Dinophyceae). II. Sequence analysis of fragments of the LSU rRNA gene. J. Phycol. 30, 999–1011. doi: 10.1111/j.0022-3646.1994.00999.x
Selina, M. S., and Morozova, T. V. (2005). First records of dinoflagellates Alexandrium margalefi Balech, 1994 and A. tamutum Montresor, Beran et John, 2004 in the seas of the Russian Far East. Russ. J. Mar. Biol. 31, 187–191. doi: 10.1007/s11179-005-0066-x
Sierra-Beltrán, A. P., Cruz-Villacorta, A., Núñez-Vázquez, Del Villar, L. M., Cerecero, J. J., and Ochoa, J. L. (1998). An overview of the marine food-poisoning in Mexico. Toxicon 36, 1493–1502. doi: 10.1016/S0041-0101(98)00139-1
Silva, N. J., Tang, K. W., and Lopes, R. M. (2013). Effects of microalgal exudates and intact cells on subtropical marine zooplankton. J. Plank. Res. 35, 855–865. doi: 10.1093/plankt/fbt026
Sunday, J. M., Calosi, P., Dupont, S., Munday, P. L., Stillman, J. H., and Reusch, T. B. H. (2014). Evolution in an acidifying ocean. Trends Ecol. Evol. 29, 117–125. doi: 10.1016/j.tree.2013.11.001
Swan, S. C., and Davidson, K. (2014). “Alexandrium and risk management within the Scottish phytoplankton monitoring programme,” in: Marine and Freshwater Harmful Algae 2014. Proceedings of the 16th International Conference on Harmful. Algae, eds A. Lincoln MacKenzie [Cawthron Institute, Nelson, New Zealand and the International Society for the Study of Harmful Algae (ISSHA)].
Tillmann, U., Krock, B., Alpermann, T. J., and Cembella, A. (2016). Bioactive compounds of marine dinoflagellate isolates from western Greenland and their phylogenetic association within the genus Alexandrium. Harmful Algae 51, 67–80. doi: 10.1016/j.hal.2015.11.004
Touzet, N., Franco, J. M., and Raine, R. (2008). Morphogenetic diversity and biotoxin composition of Alexandrium (Dinophyceae) in Irish coastal waters. Harmful Algae 7, 782–797. doi: 10.1016/j.hal.2008.04.001
Wang, D.-Z. (2008). Neurotoxins from marine dinoflagellates: a brief review. Mar. Drugs 6, 349–371. doi: 10.3390/md6020349
Wang, D.-Z., Lin, L., Gu, H.-F., Chan, L. L., and Hong, H.-S. (2008). Comparative studies on morphology, ITS sequence and protein profile of Alexandrium tamarense and A. catenella isolated from the China Sea. Harmful Algae 7, 106–113. doi: 10.1016/j.hal.2007.06.002
Wang, L., Zhuang, Y., Zhang, H., Lin, X., and Lin, S. (2014). DNA barcoding species in Alexandrium tamarense complex using ITS and proposing designation of five species. Harmful Algae 31, 100–113. doi: 10.1016/j.hal.2013.10.013
Keywords: Alexandrium catenella (group I), Alexandrium tamutum, phenotypic plasticity, taxonomy, phylogeny, paralytic shellfish poisoning
Citation: Menezes M, Branco S, Miotto MC and Alves-de-Souza C (2018) The Genus Alexandrium (Dinophyceae, Dinophyta) in Brazilian Coastal Waters. Front. Mar. Sci. 5:421. doi: 10.3389/fmars.2018.00421
Received: 01 August 2018; Accepted: 23 October 2018;
Published: 15 November 2018.
Edited by:
Jorge I. Mardones, Instituto de Fomento Pesquero (IFOP), ChileReviewed by:
Satoshi Nagai, National Research Institute of Fisheries Science, Japan Fisheries Research and Education Agency, JapanF. Leonardo Guzmán, Instituto de Fomento Pesquero (IFOP), Chile
Copyright © 2018 Menezes, Branco, Miotto and Alves-de-Souza. This is an open-access article distributed under the terms of the Creative Commons Attribution License (CC BY). The use, distribution or reproduction in other forums is permitted, provided the original author(s) and the copyright owner(s) are credited and that the original publication in this journal is cited, in accordance with accepted academic practice. No use, distribution or reproduction is permitted which does not comply with these terms.
*Correspondence: Mariângela Menezes, menezes.mariangela@gmail.com