- 1Research Center FONDAP IDEAL, Instituto de Acuicultura, Universidad Austral de Chile, Puerto Montt, Chile
- 2COPAS Sur-Austral, Centro de Investigación Oceanográfica en el Pacífico Sur-Oriental, Universidad de Concepción, Concepción, Chile
- 3Centro de Investigación en Ecosistemas de la Patagonia, Coyhaique, Chile
Some of the largest climate changes on Earth are projected for high-latitude polar and subpolar environments in the coming decades (IPCC, 2014). A number of simultaneous socio-environmental perturbations are affecting Patagonia (41–55∘S), a vast and complex ecosystem whose economic and societal well-being depends on coastal resources (∼80% of people live near-shore; the coastal economy provides>40,000 jobs). In this new scenario, overlapping natural variability (interannual – seasonal dynamics) and human-induced environmental changes (global change) are major drivers influencing the marine food web structure and functions. Evidence of large environmental changes has been detected in the Patagonia marine system through (a) regional-scale, climatic-oceanographic coupled anomalous events, (b) interannual hydrological changes (decreasing pattern) in freshwater river inputs (watersheds into fjords), and (c) more frequent coastal and oceanic microbial outbreaks (Harmful Algal Blooms). Those changes have highly uncertain effects for the basic functionalities, structure, and feedback responses of coastal systems and their coupling with hydrological (e.g., river streamflow) or biogeochemical (e.g., biological carbon pump) processes. The northern Patagonian fjords system is hypothesized to act as an indicator of large-scale climate change given strong environmental variability. This, combined with regional climatic-oceanographic events (e.g., El Niño Southern Oscillation, Southern Annular Mode) and effects of local anthropogenic activities (e.g., nutrient fluxes from extensive aquaculture, land change due to forestry) may impact the chemical and physical properties of the surface water, destabilizing biological productivity and chemical coastal processes. The present synthesis, which gives the results of systemic-sensitive chemical and biological variables, may be useful to managers and in preventing socio-environmental impacts from increasing natural and anthropogenic phenomena in Chile’s southern coastal system.
Introduction
The expansive Chilean Patagonia comprises a variety of marine and terrestrial forms: e.g., glaciers, the North and South Ice Fields, channels, bays, fjords, islands, lagoons, lakes, and straits. These create highly heterogeneous habitats for both native species and those (farmed and wild) introduced by aquaculture and agriculture. The terrestrial ecosystem is dominated by extensive mountain basins rich in native forests, rivers, pristine, and ultraoligotrophic lakes. However, the land has also been modified by historical colonization (human settlements led to changes in land use and forestation) and geological disturbances (e.g., volcanism, thermal vents). The topography of the Chilean Patagonia is irregular: steep mountain slopes (>1000 m above sea level) surround the fjords, penetrate the estuarine/marine system to depths ranging from 200 to 1000 m, and determine the importance of the forest – fjord interaction. Both terrestrial and aquatic ecosystems have high species richness, with birds, amphibians, and marine vertebrates and invertebrates, including several recently described cold-water hydrocoral species growing on vertical walls submerged at depths of 20 to 200 m (Häussermann and Försterra, 2007). Moreover, evidence of active geological processes (e.g., surface hydrothermal vents; Försterra et al., 2014) in Comau Fjord is relevant ecologically and biogeochemically (high methane and sulfide-enriched water), as these processes support trophic webs sustained by giant methanogenic bacteria (chemosynthetic communities) and diverse benthic species (Muñoz et al., 2014; Quiroga et al., 2016). Although the number of cold seeps in the fjord system remains unknown, patches of microbial mats, which obtain energy from the reduction of nitrate and sulfate, may play a key role in supporting a trophic link to invertebrate grazers (chitons, gastropods, mussels, and cold-water scleractinian corals) (Ugalde et al., 2013; Muñoz et al., 2014).
The oceanographic characteristics of southern Chile’s coastal marine system are heavily influenced by freshwater that precipitates (up to 7000 mm per year) directly over the water surface and enters through the mouths of tributary rivers (Dávila et al., 2002). Southern Chilean Patagonia (41 – 50°S) is characterized by a few large rivers (Petrohue: 41°S; Puelo: 41°S; Yelcho: 43°S; Palena: 43°S; Baker: 47°S; and Serrano: 48°S), numerous small river basins, many glaciers and icefields (i.e., South and North Icefields). In this vast region, high freshwater discharges (2470 – 3344 m3 s−1) into the eastern South Pacific Ocean are mainly observed between 42 and 50°S (Dávila et al., 2002). In these systems, high freshwater inflows (precipitation, glacier melting) generate strong vertical and horizontal density gradients, modulated mainly by a vertical salinity structure that determines a strong, permanent surface stratification – usually into two layers in coastal systems – of the water column (e.g., buoyancy frequency values of 20–60 × 10−3 s−2; Valle-Levinson et al., 2007; Castillo et al., 2016). Although the magnitude of fresh water flowing into the ocean varies along the South Pacific Ocean, the large spatial effect of lower salinity (33.0 – 33.8) along the oceanic margin of the Patagonian sector of the Pacific Ocean (Acha et al., 2004) could play a role in the modulation of physical (haline stratification), chemical (nutrient ratio: N:P:Si), and biological processes (primary production). On the other hand, the inner fjord region system that is influenced by heavy freshwater riverine and glacier-melt inflows is characterized by: (i) limited inorganic nutrient exchanges between the surface and deep layers of the water column due to haline stratification: tributaries contribute high concentrations of dissolved silicic acid (15 – 100 μM) to the surface layer, whereas Subantarctic waters deliver high concentrations of orthophosphate (1 – 2 μM) and nitrate (5 – 20 μM) to the deep layer, and (ii) both inorganic nutrients and radiation acting as the main seasonal limiting factors for seasonally high primary productivity (PP) (0.5 – 3 g C m−2 d−1), which is dominated mainly by diatoms (90%) (Iriarte et al., 2007; González et al., 2013; Jacob et al., 2014).
This article reports the main features and achievements obtained over more than 10 years of interdisciplinary research carried out in the marine system of Patagonia using approaches such as synoptic oceanographic cruises, micro-mesoscom experiments, and time-series autonomous bouys. The paper unites the fields of oceanography, hydrology, and climatology as a means of improving our understanding of ongoing natural and human perturbations and their possible impacts on ecosystem services.
Recent Threads in Patagonian Marine System
Should they come to bear, the potential impacts of human activities could certainly alter the structure and functioning of Patagonia’s terrestrial and aquatic ecosystems (Table 1). These impacts may include the addition of nutrients (e.g., nitrogen) from agriculture and aquaculture, the removal of inorganic (e.g., silicic acid) or organic (e.g., carbon sources) nutrients through dams, and global phenomena that may be remote (global warming), may occur on interannual (El Niño Southern Oscillation, ENSO; Southern Annular Mode, SAM) or decadal/centennial scales (volcanic eruptions), or may be climatic (increased radiation, wind stress reduction of West Wind Drift) (Garreaud, 2018; León-Muñoz et al., 2018).
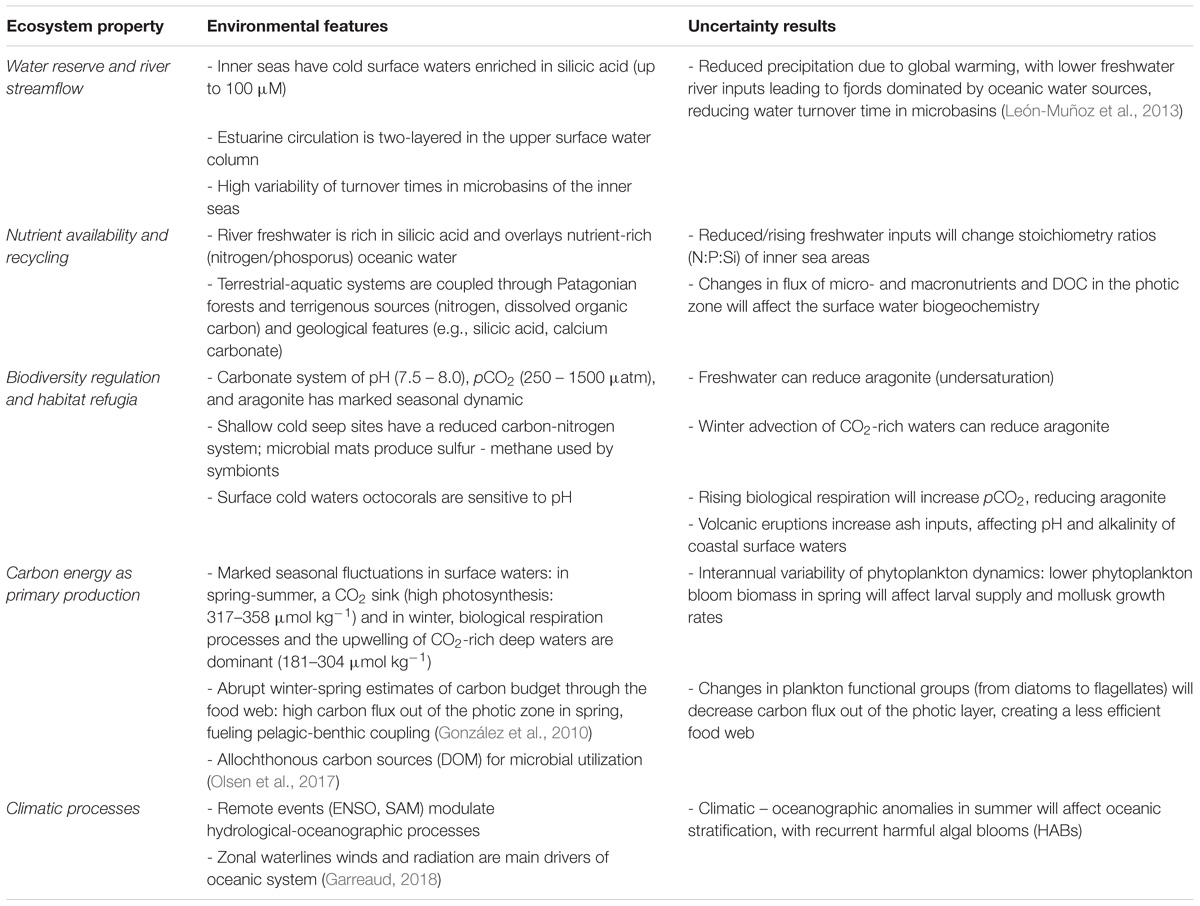
TABLE 1. Main properties (ecosystem scale) that may be supported by the principal climatic, hydrological, and oceanographic features considering current and future scenarios of uncertainty in the Patagonian marine ecosystem.
The marine system of northern Patagonia (41 – 44°S) recently experienced a strong interannual change in chlorophyll-a dynamics. Specifically, in two consecutive years (2009 – 2010), the magnitude of spring blooms was lower (less than 2 mg Chlorophyll-a m−3). This shift was associated with the cooling of the ocean surface and heavily disrupted the mollusk larval supply, mainly in the Inner Sea of Chiloé, from 2010 to 2011 (Lara et al., 2016). These strong seasonal changes vary on an interannual scale and are probably forced by regional oceanographic-climate changes such as ENSO and SAM. Furthermore, recurrent harmful algal blooms (HABs) were observed in coastal and oceanic areas in 2016–2017. These coincided with remote phenomena (ENSO, SAM) that led to very dry conditions, higher than normal solar radiation reaching the surface, and high sea surface temperatures. Specifically, results showed that anomalies created by ENSO and SAM led to drought on land and oceanographic conditions that set the stage for an unusual “super bloom” of Pseudochattonella sp. (León-Muñoz et al., 2018). Due to global climate change, marine phytoplankton in the southermost fjords region will likely experience increasing temperatures, constant glacial melting, and probably increased nitrogen (e.g., zooplankton excretion rates) in the future. We expect the interactive effects of these processes to heighten both the growth rates and toxicity of harmful algal species. Several of the toxic algal species favored by these environmental features – including Pseudochattonella spp. (dictyophytes), Alexandrium spp. and Karenia spp. (dinoflagellates), and Pseudo-nitzschia spp. (diatoms) – have been observed in the inshore and oceanic waters off Patagonia during the summer months of 2016 – 2017.
The oceanographic characteristics of the Patagonian fjord marine ecosystem favor the cultivation of aquatic species, explaining why more than 90% of aquaculture biomass (salmon, blue mussels, and macroalgae) are found in the inner seas of Patagonia. Optimal ranges of key variables (e.g., temperature, salinity, and dissolved oxygen) for the smoltification, growth, and physiological processes of fish and invertebrates, together with highly transparent river waters and the importance of physical forcings (e.g., tidal currents as a process of particle dispersion) make these systems ideal for the development of aquaculture. In this scenario, aquaculture “uses” the surface layer of the sea (∼25 m deep) for farming organisms, utilizing a variety of feeding strategies (pellets for salmon, natural phytoplankton for mytilids). However, the surface layer is precisely where the greatest physical (thermocline, pycnocline, mixed layer, and photic layer), chemical (halocline, nutricline, and oxycline), and biological gradients (biomass, primary production, and oxygen consumption) occur, making this thin oceanic layer highly vulnerable to external disturbances. Specifically, the addition and removal of inorganic and organic substances at the surface from anthropogenic activities (e.g., aquaculture, agriculture, forestry, and hydroelectricity) could represent, in the near future, a new critical state for the Patagonian marine system. For example, the stoichiometric ratio for three inorganic nutrients (nitrogen:phosphorus:silica, 16:1:1) in the fjord surface layer is expected to deviate under three environmental regimes: (i) lower flow from large Patagonian rivers (freshwater enriched in silicic acid) (León-Muñoz et al., 2013), (ii) frequent advection of deep oceanic waters with high orthophosphate, nitrogen, and CO2 levels (Torres et al., 2014), and (iii) the addition of nitrogen (ammonia) through the excrement of fish and mollusks farmed in the coastal waters off Patagonia (Soto and Norambuena, 2004).
Results from recent microcosm experiments performed in the Patagonian fjords and considering scenarios of ammonium addition by aquaculture (Olsen et al., 2014, 2017) have indicated that this nitrogen compound is highly “bioavailable” in the surface layer, mainly for biological assimilation processes by phytoplankton and bacteria. One of the main ecological implications of these mesocosm results is the possibility that an excessive increase in surface ammonium concentrations (ammonium flux higher than 1 μmol N L−1 d−1) could, in the near future, trigger rapid and sustained growth of the autotrophic biomass, associated with increased bacterial production rates (i.e., higher respiration rates and seawater pCO2). This would eventually lead to sporadic, low-oxygen events in the photic layer, which would have negative consequences at higher trophic levels (fish and mollusk mortality). Finally, an important change in the components of the food web due to frequent pulses of nutrients (e.g., ammonium, silicic acid) or dissolved matter (e.g., dissolved carbon substances) from external sources could modify the trophic state of the ecosystem, shifting it from predominantly autotrophic (diatoms) to predominantly heterotrophic (flagellates) (Thingstad et al., 2010).
Recently, studies of northern Patagonian fjords influenced by rivers have shown strong changes in the seasonal dynamics of the carbonate system variables in the surface layer. High-frequency pH/pCO2 measurements taken during a seasonal cycle in a northern fjord showed that riverine freshwater inputs and phytoplankton productivity affected the chemistry of the fjord, enhancing the shift from CO2 sink in summer–autumn (high primary production) to CO2 source in winter (high community respiration, CO2-rich oceanic water advection). Given the high PP of the northern Patagonian fjords in spring–summer, with strong seasonality in the air–sea carbon flow, the dynamics of the carbonate system indicate that the atmospheric–oceanic CO2 exchange creates a CO2 sink system in the far southern fjords under spring–summer conditions (Torres et al., 2011). However, seasonal pCO2 flux data showed that a river-influenced northern fjord (Reloncavi fjord) was a strong CO2 sink in the austral spring–summer and a CO2 source in winter, resulting in an annual net efflux (0.71 mol CO2 m−2 yr−1). This result seems to suggest that the net uptake of CO2 by phytoplankton in spring–summer is offset by heterotrophic respiration in winter. The high PP in the coastal waters off Patagonia creates an efficient flow of matter (carbon) and energy through higher trophic levels such as zooplankton (Patagonian krill), mollusks (bivalves), cold water corals, pelagic fish (sardines), and whales.
The Subantarctic water chemistry in the top 50 m of the inner seas of Patagonia varies markedly by season (spring–winter) in terms of pH, alkalinity, and concentrations of aragonite, as well as nutrient ratios (N:P:Si). Specifically, aragonite is an indicator of the saturation level of the form of CaCO3 in seawater available for use by the mollusk larvae that require CaCO3 for shell formation. In winter, the state of CaCO3 saturation in Patagonian surface waters is close to undersaturated for calcium carbonate in the form of aragonite (Ω < 1) (Torres et al., 2011; Alarcón et al., 2015), suggesting that increased freshening and acidification may undersaturate surface waters in the short term. Furthermore, an alteration in the winter–spring dynamics of aragonite saturation would have implications for the survival of larval stages of benthic shelled mollusks. For example, a delay in undersaturated aragonite values (Ω < 1) toward the spring months could negatively affect survival of early stage mollusk larvae during this period, ultimately affecting their growth rates (Ekstrom et al., 2015).
A different pattern can be expected in the southernmost region, particularly the Subantarctic Magellan ecosystem (50–55°S), where most glacier tongues (e.g., Marinelli, Ventisquero, and Santa Inés) have experienced some degree of retreat (Bown et al., 2014) as well as increased ice melting processes and higher volume inputs of freshwater to the fjord system. Whatever their causes (atmospheric warming, less precipitation, and Calvin cycle), these effects are expected to continue along with important atmosphere–ocean interactions that may profoundly influence biological processes in marine fjords. In general, when freshwater enters a fjord, it creates a “front”; this low-salinity layer provides vertical stability (deepening the mixed layer) and nutrient-enriched surface waters. Surface waters within the Strait of Magellan system are mainly derived from mixing between oceanic Subantarctic Surface Water (SAAW) and nitrate-poor, continental freshwater (Modified Subantarctic Water; Sievers and Silva, 2008; Torres et al., 2014). Here, the main phytoplankton blooms occur in spring–summer along the coast, when relatively high rates of primary production (1 mg C m−2 d−1) may be supported by the shallowing of the mixed and euphotic layers, high near-surface light levels, and abundant macro- and micronutrients (Iriarte et al., 2001; Lutz et al., 2016). Given ongoing global changes and their direct effect on glaciers, it remains difficult to predict how high freshwater fluxes and strong stratification will impact glacial–fjord systems, especially in terms of the efficiency of the biological carbon pump (Figure 1).
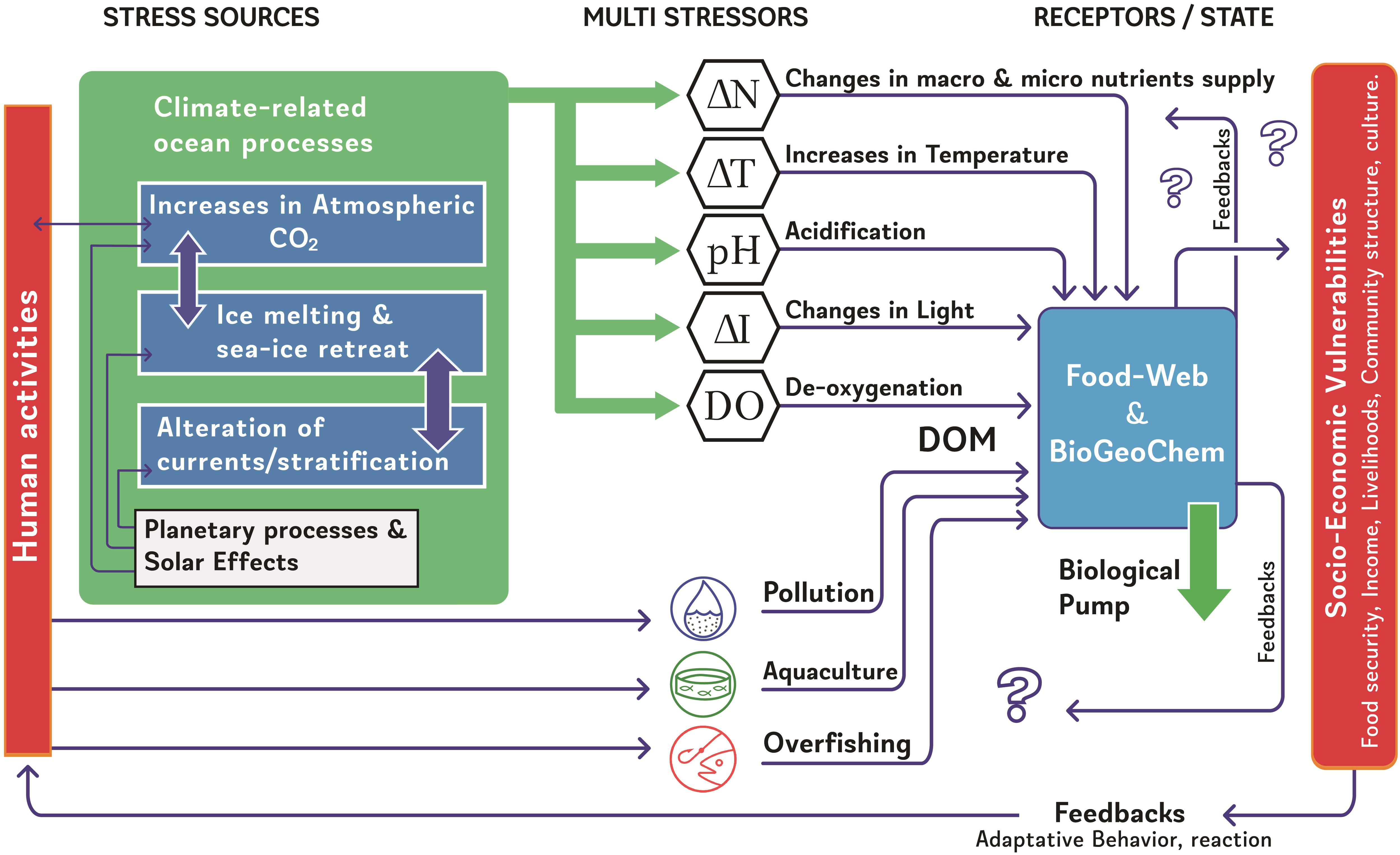
FIGURE 1. Conceptual flow diagram of a coastal marine ecosystem, showing complex atmosphere–ocean interactions through: (i) a variety of different actual stress sources from Human, Atmosphere, Cryosphere, and Ocean main processes, (ii) the individual and combined effects of environmental stressors, and (iii) their main changes in outputs acting on the Biological Carbon Pump and marine food web chains. In the Subantarctic Patagonian system, most of the actual and potential stressors are acting in a thin, two-layer water column that is heavily stratified by salinity near the surface. Changes in the food web compartment can lead to decreased or increased efficiency of the Biological Pump, a key aspect on which efforts must be focused in the near future. The diagram includes a series of climatic and non-climatic stressors (e.g., overfishing, aquaculture) that might eventually affect the food web and, subsequently, the Biological Pump; however, the concomitant positive/negative feedbacks to societal coastal related processes remain unknown (Main conceptual figure from the Ocean Certain EU Program, with permission of Dr. Murat V. Ardelan).
Some recent volcanic eruptions (Chaiten in 2008, Puyehue-Cordón Caulle in 2011, and Calbuco in 2015) could have temporarily impacted both terrestrial and aquatic habitats and, therefore, the structure and functioning of the biotic (including human) communities that inhabit and use the coastal area of far southern Chile. Specifically, the precipitation of volcanic ash (rich in silica and Fe; Torres et al., 2014; Browning et al., 2015) into the system of basins and tributary rivers flowing into estuarine/marine fjord systems altered several aspects of the water chemistry: suspended material content (mainly dissolved inorganic, organic carbon and particulate carbon), light penetration through the short photic layer, and the properties of the carbonate system (pH, alkalinity). For example, after the eruption of the Calbuco volcano (May, 2015), pH dropped to 7.2 in the first 4 m of depth, returning to normal (7.8) after 10 days (Vergara et al., 2016). One major biogeochemical implication was the capacity of the marine system for resilience – mainly when facing changes in pH – due to its great buffering potential. On the other hand, trends (e.g., lower freshwater flows) observed on some rivers in northern Patagonia (Rio Puelo; León-Muñoz et al., 2013) and strong river–fjord–ocean interactions could have complex biogeochemical implications due mainly to changes in alkalinity, oxygen concentrations, and dissolved matter in coastal and marine environments.
Conclusion
At present, Chile’s southern Patagonia region is experiencing unprecedented environmental impacts from climate change (e.g., warming, ice melting, and glacial retreat), such that aquatic organisms (including humans) are expected to respond and adapt in their surroundings during the near future. Environmental changes will affect the basic functionality and feeback responses of coastal systems (Figure 1) as well as couplings with hydrological (e.g., river streamflow, glacier melting) and biogeochemical (e.g., carbonate system) processes. This initial qualitative analysis of some potential effects of disturbances – both natural and anthropogenic in origin – on Patagonia’s coastal marine system helps identify near-future mitigation actions and mechanisms. For example: Lowering nutrient loading in coastal waters would reduce hypoxia in the surface sediments and deep water column as well as the frequency of HABs. In turn, encouraging the farming or extraction of diverse new autocthonous fishes and invertebrates species (as opposed to the monospecific strategies that presently focus mostly on salmonid and mussel species) would reduce social vulnerability to environmental impacts. Finally, the availability of information to science through aquaculture-related industry-research partnerships, offshore systems that autonomously monitor new biogeochemical variables (pH, aragonite, ammonium), and longer time-series of the main variables (chlorophyll-a, sea surface temperature, dissolved oxygen) all serve to support interannual and seasonal variability of metabolic functions of marine species as well as continually verify the “health” of the system.
Author Contributions
JI conceived the idea, integrated the information, and wrote the paper.
Funding
The general view (“socio-environmental” perspective) for Subantarctic Patagonian fjords was inspired by Ocean Certain (Program EU-FP7 603773) and by the FONDAP IDEAL Center (Program 15150003). The author wishes to acknowledge the Hydrographic and Oceanographic Service of the Chilean Navy (SHOA) and the National Oceanographic Committee (CONA) for their support through the marine research program, CIMAR FIORDOS (CONA-C15F 09-12 and CONA-C18F- 2012).
Conflict of Interest Statement
The author declares that the research was conducted in the absence of any commercial or financial relationships that could be construed as a potential conflict of interest.
Acknowledgments
The author grateful to Dr. M. Ardelan, who provided Figure 1, and to Andrea Navarro, Journalist, who pushed for a translation of the oceanographic concepts into language that would be accessible, interesting, and applicable to the general public. The information presented in Table 1 stems from valuable questions and comments discussed with my graduate students: Jorge León-Muñoz, Carlos Lara, Maximiliano Vergara, Marco Pinto, and Jurleys Vellojin.
References
Acha, E. M., Mianzan, H. W., Guerrero, R., Favero, M., and Bava, J. (2004). Marine fronts at the continental shelve of austral South America physical and ecological processes. J. Mar. Syst. 44, 83–105. doi: 10.1016/j.jmarsys.2003.09.005
Alarcón, E., Valdés, N., and Torres, R. (2015). Calcium carbonate saturation state in an area of mussels culture in the Reloncaví Sound, northern Patagonia. Chile. Latin Am. J. Aquat. Res. 43, 277–281. doi: 10.3856/vol43-issue2-fulltext-1
Bown, F., Rivera, A., Zenteno, P., Bravo, C., and Cawkwell, F. (2014). “First glacier inventory and recent glacier variation on isla grande de tierra del fuego and adjacent islands in southern chile,” in Global Land Ice Measurements from Space, eds J. Kargel, G. Leonard, M. Bishop, A. Kääb, and B. Raup (Berlin: Springer).
Browning, T. J., Stone, K., Bouman, H. A., Mather, T. A., Pyle, D. M., and Moore, C. M. (2015). Volcanic ash supply to the surface ocean-remote sensing of biological responses and their wider biogeochemical significance. Front. Mar. Sci. 2:14. doi: 10.3389/fmars.2015.00014
Castillo, M. I., Cifuentes, U., Pizarro, O., Djurfeldt, L., and Caceres, M. (2016). Seasonal hydrography and surface outflow in a fjord with a deep sill: the Reloncaví fjord. Chile. Ocean Sci. 12, 533–534. doi: 10.5194/os-12-533-2016
Dávila, P. M., Figueroa, D., and Müller, E. (2002). Freshwater input into the coastal ocean and its relation with the salinity distribution off austral Chile (35–55°S). Cont. Shelf Res. 22, 521–534. doi: 10.1016/S0278-4343(01)00072-3
Ekstrom, J. A., Suatoni, L., Cooley, S. R., Pendleton, L. H., Waldbusser, G. G., Cinner, J. E., et al. (2015). Vulnerability and adaptation of US shellfisheries to ocean acidification. Nat. Climate Change 5, 207–214. doi: 10.1038/nclimate2508
Försterra, G., Häussermann, V., Laudien, J., Jantzen, C., Sellanes, J., and Muñoz, P. (2014). Mass die-off of the cold-water coral Desmophyllum dianthus in the Chilean Patagonian region. Bull. Mar. Sci. 90, 895–899. doi: 10.5343/bms.2013.1064
Garreaud, R. D. (2018). A plausible atmospheric trigger for the 2017 coastal El Niño. Int. J. Clim. 38, e1296–e1302. doi: 10.1002/joc.5426
González, H. E., Calderón, M. J., Castro, L., Clement, A., Cuevas, L. A., Daneri, G., et al. (2010). Primary production and plankton dynamics in the Reloncavi Fjord and the Interior Sea of Chiloé, Northern Patagonia, Chile. Mar. Ecol. Prog. Ser. 402, 13–30. doi: 10.3354/meps08360
González, H. E., Castro, L. R., Danieri, G., Iriarte, J. L., Silva, N., Tapia, F., et al. (2013). Land-ocean gradient in haline stratification and its effects on plankton dynamics and trophic carbon fluxes in Chilean Patagonian fjords (47–50°S). Prog. Oceanogr. 119, 32–47. doi: 10.1016/j.pocean.2013.06.003
Häussermann, V., and Försterra, G. (2007). Extraordinary abundance of hydrocorals (Cnidaria, Hydrozoa, Stylasteridae) in shallow water of the Patagonian fjord region. Polar Biol. 30, 487–492. doi: 10.1007/s00300-006-0207-5
IPCC (2014). Climate Change 2014: Synthesis Report. Contributions of Working Groups I, II and III to the Fifth Assessment Report of the Intergovernmental Panel on Climate Change, eds R. K. Pachauri and L. A. Meyer (Geneva: IPCC), 151.
Iriarte, J. L., González, H. E., Liu, K. K., Rivas, C., and Valenzuela, C. (2007). Spatial and temporal variability of chlorophyll and primary productivity in surface waters of southern Chile (41.5–43°S). Est. Coast. Shelf Sci. 74, 471–480. doi: 10.1016/j.ecss.2007.05.015
Iriarte, J. L., Kusch, A., Osses, J., and Ruiz, R. (2001). Phytoplankton biomass in the sub-antarctic area of the Strait of Magellan (53°S), Chile during spring-summer 1997/1998. Polar Biol. 24, 154–162. doi: 10.1007/s003000000189
Jacob, B., Quiñones, R. A., Iriarte, J. L., Daneri, G., Tapia, F., Montero, P., et al. (2014). Springtime size-fractionated primary production across hydrographic and PAR-light gradients in Chilean Patagonia (41–50°S). Prog. Oceanogr. 129, 75–84.
Lara, C., Saldías, G. S., Tapia, F. J., Iriarte, J. L., and Broitman, B. R. (2016). Interannual variability in temporal patterns of chlorophyll-a and their potential influence on the supply of mussel larvae to inner waters in northern Patagonia (41-44°S). J. Mar. Syst. 155, 11–18. doi: 10.1016/j.jmarsys.2015.10.01
León-Muñoz, J., Marcé, R., and Iriarte, J. L. (2013). Influence of hydrological regime of an Andean river on salinity, temperature and oxygen in a Patagonian fjord, Chile. New Zeal. J. Mar. Fresh. Res. 47, 515–528. doi: 10.1080/00288330.2013.802700
León-Muñoz, J., Urbina, M. A., Garreaud, R., and Iriarte, J. L. (2018). Hydroclimatic conditions trigger record harmful algal bloom in western Patagonia (summer 2016). Sci. Rep. 8:1330. doi: 10.1038/s41598-018-19461-4
Lutz, V., Frouin, R., Negri, R., Silva, R., Pompeu, M., Rudorff, N., et al. (2016). Bio-optical characteristics along the Straits of Magallanes. Cont. Shelf Res. 119, 56–67. doi: 10.1016/j.csr.2016.03.008
Muñoz, P., Sellanes, J., Villalobos, K., Zapata-Hernández, G., and Mayr, C. (2014). Geochemistry of reduced fluids from shallow cold vents hosting chemosynthetic communities (Comau Fjord, Chilean Patagonia, 42°S). Prog. Oceanogr. 129, 159–169. doi: 10.1016/j.pocean.2014.10.003
Olsen, L., Hernández, K., Ardelan, M., Iriarte, J. L., Sánchez, N., González, H., et al. (2014). Responses in the food web structure to increased rate of nutrient supply in a temperate fjord in southern Chile: possible implications from cage aquaculture. Aquat. Environ. Int. 6, 11–27. doi: 10.3354/aei00114
Olsen, L. M., Hernandez, K. L., Ardelan, M., Iriarte, J. L., Bizsel, K. C., and Olsen, Y. (2017). Responses in bacterial community structure to waste nutrients from aquaculture: an in situ microcosm experiment in a Chilean fjord. Aquat. Environ. Int. 9, 21–32. doi: 10.3354/aei00212
Quiroga, E., Ortiz, P., González-Saldías, R., Reid, B., Tapia, F. J., Pérez-Santos, I., et al. (2016). Seasonal benthic patterns in a glacial Patagonia fjord: the role of suspended sediment and terrestrial organic matter. Mar. Ecol. Prog. Ser. 561, 31–50. doi: 10.3354/meps11903
Sievers, H., and Silva, N. (2008). “Water masses and circulation in austral Chilean channels and fjords progress,” in The Oceanographic Knowledge of Chilean Interior Waters, from puerto Montt to Cape Horn, eds N. Silva and S. Palma (Valparaíso, CL: omité Oceanográfico Nacional - Pontificia Universidad Católica de Valparaíso).
Soto, D., and Norambuena, F. (2004). Evaluation of salmon farming effects on marine systems in the inner seas of southern Chile: a large-scale mensurative experiments. J. Appl. Ichthyol. 20, 493–501. doi: 10.1111/j.1439-0426.2004.00602.x
Thingstad, T. F., Strand, E., and Larsen, A. (2010). Stepwise building of plankton functional type (PFT) models: a feasible route to complex models. Prog. Oceanogr. 84, 6–15. doi: 10.1016/j.pocean.2009.09.001
Torres, R., Pantoja, S., Harada, N., Gonzalez, H., Daneri, G., Frangopulos, M., et al. (2011). Air-sea CO2 fluxes along the coast of Chile: from CO2 outgassing in central-northern upwelling waters to CO2 uptake in southern Patagonian fjords. J. Geophys. Res. 116:C09006. doi: 10.1029/2010JC006344
Torres, R., Silva, N., Reid, B., and Frangopulos, M. (2014). Silicic acid enrichment of sub Antarctic surface water from continental inputs along the Patagonian archipelago interior sea (41–56°S). Prog. Oceanogr. 129, 50–61. doi: 10.1016/j.pocean.2014.09.008
Ugalde, J. A., Gallardo, M. J., Belmar, C., Muñoz, P., Ruiz-Tagle, N., Ferrada-Fuentes, S., et al. (2013). Microbial Life in a fjord: Metagenomic analysis of a microbial mat in Chilean Patagonia. PLoS One 8:e71952. doi: 10.1371/journal.pone.0071952
Valle-Levinson, A., Sarkar, N., Sanay, R., Soto, D., and León, J. (2007). Spatial structure of hydrography and flow in a Chilean fjord. Estuario Reloncaví. Est. Coasts 30, 113–126.
Vergara, M. J., Torres, R., Alarcón, E., Beatty, C., Degrandpre, M., Cuevas, et al. (2016). “Strong-short term effects of a volcanic eruption on the carbonate system of a highly stratified fjord in the northern chilean Patagonia,” in Proceedings of the 4th International Symposium on the Ocean in a High-CO2 World, Hobart, TAS.
Keywords: Patagonian fjords system, marine biogeochemistry, environmental – harmful algae coupling, ecosystem functions – stressors, salinity-driven system
Citation: Iriarte JL (2018) Natural and Human Influences on Marine Processes in Patagonian Subantarctic Coastal Waters. Front. Mar. Sci. 5:360. doi: 10.3389/fmars.2018.00360
Received: 09 May 2018; Accepted: 19 September 2018;
Published: 08 October 2018.
Edited by:
Simone Libralato, Istituto Nazionale di Oceanografia e di Geofisica Sperimentale (OGS), ItalyReviewed by:
Carlos Rosas, Universidad Nacional Autónoma de México, MexicoValeria Mamouridis, Independent Researcher, Rome, Italy
Copyright © 2018 Iriarte. This is an open-access article distributed under the terms of the Creative Commons Attribution License (CC BY). The use, distribution or reproduction in other forums is permitted, provided the original author(s) and the copyright owner(s) are credited and that the original publication in this journal is cited, in accordance with accepted academic practice. No use, distribution or reproduction is permitted which does not comply with these terms.
*Correspondence: José L. Iriarte, amlyaWFydGVAdWFjaC5jbA==