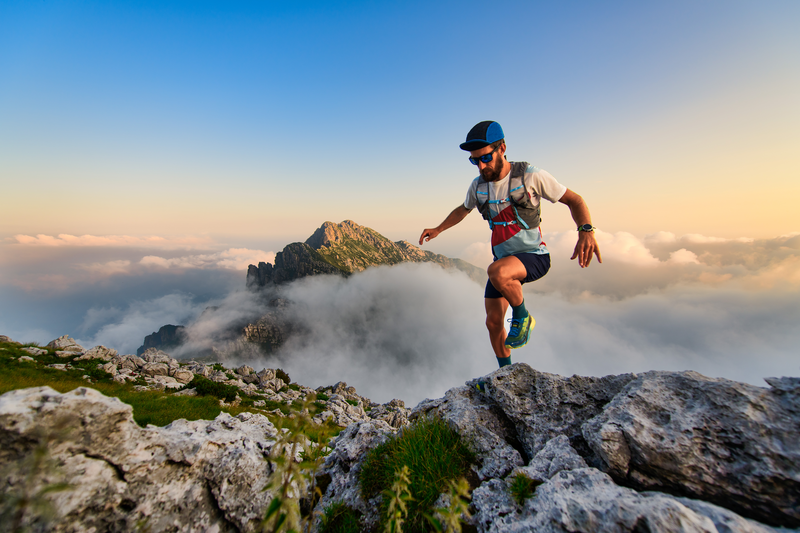
94% of researchers rate our articles as excellent or good
Learn more about the work of our research integrity team to safeguard the quality of each article we publish.
Find out more
ORIGINAL RESEARCH article
Front. Mar. Sci. , 10 October 2018
Sec. Marine Affairs and Policy
Volume 5 - 2018 | https://doi.org/10.3389/fmars.2018.00359
The concept of ecosystem services has gained traction as a means of linking societal benefits to the underlying ecology and functioning of ecosystems, and is now frequently included in decision-making and legislation. Moving the ecosystem service concept from theory into practice is now crucial. However, advancements in this area of research differ by ecosystem type, and marine systems lag significantly behind terrestrial counterparts in terms of understanding, implementation, and number of studies. In this paper we explore several reasons why ecosystem service research has been limited in marine systems and we outline the challenges that hinder progress. Marine systems suffer from a scarcity of spatial data relative to terrestrial counterparts. In terrestrial systems the spatial patterns of land-use/land-cover (LULC) are relatively straightforward to access via satellite and have been used as proxy indicators of service provisions. In contrast, remote sensing tools used to study the surface of the Earth are much less effective at capturing images of the seabed, and by extension marine habitats. Marine waters and their constituents are also frequently driven great distances by winds, tides, and currents. This creates a challenge for management as the identification and protection of areas where ecosystem services are exploited is not necessarily sufficient to ensure sustained service delivery. Further complications arise from the three-dimensional uses of marine systems, incorporating activities that use the sea surface, the water column and the benthic habitats below. Progress is being made as technological advancements are resulting in the acquisition of spatial data at faster rates and higher resolutions than previously possible. There is a growing capacity to map, model and value an increasing number of services with initiatives such as InVEST or principle-based modeling. We suggest that awareness is needed around the limited progress in marine systems as this could affect the way we value the biosphere and the relative proportion between biomes.
The importance of the natural environment for sustaining human life has been implicitly or explicitly recognized by civilisations for millennia. It has also been known for hundreds of years that the trajectories of population growth and resource exploitation cannot continue indefinitely (e.g., Malthus, 1798). Despite this knowledge, humans continue to degrade the ecological systems we depend upon (Hardin, 1968). The global population has now reached 7.2 billion people and the world continues to experience its highest rate of biodiversity loss (Diaz et al., 2006; Barnosky et al., 2011). To assist with urgent and necessary environmental stewardship, the concept of “ecosystem services” has rapidly gained traction. Ecosystem services provide a means of linking the range of benefits that humans derive to the underlying ecology and integrity of ecosystems (Daily et al., 1997; Millennium Ecosystem Assessmen, 2005; de Groot et al., 2012) and this includes less conspicuous benefits that have been easily ignored. There has been a recent drive to move ecosystem service theory beyond a compelling concept and into the realm of practical tools (Norgaard, 2009; Lele et al., 2013). However, if ecosystem service science progresses at different rates in different systems (e.g., terrestrial and marine; Figure 1), this could have the potential to alter the way we value the biosphere.
Figure 1. The occurrence of “ecosystem service” in publications titles, broken down by subject area, listed in ISI Web of Science from 2000 to 2015. “Ecosystem Service” was entered as a title search word, with year published used to demarcate time intervals. All papers abstracts were reviewed and assigned to one of three groups (“marine,” “terrestrial.” or “other”) and enumerated. Anomalies were removed including e.g., IT “digital service ecosystems,” articles retracted after publication and duplicated entries. “Marine” articles had a clear reference to, and a major focus on, only marine habitats (estuarine to deep-ocean). In keeping with (Costanza et al., 1997, 2014) Wetlands were classified as terrestrial studies but mangroves were classified as marine if they specifically related to fisheries. “Terrestrial” articles had a predominant focus on the earth's continents including forests, agroforestry, agriculture and grasslands, urban environments, lakes and rivers or a single or limited group of services predominating from terrestrial systems. “Other” articles referred to those that could not be classified as either marine or terrestrial. Some articles focused on specific services that referenced both marine and terrestrial systems i.e., climate focused. Other articles focused on the organization, valuation of or payment for, classification, frame-working, management or governance of ecosystem services without specifically relating to either marine or terrestrial systems.
Marine systems provide multiple and varied ecosystem services and are depended on by much of the world's population. Despite the global growth of the ecosystem service concept, progress in applying the concept to the marine realm has been relatively slow. For example, the TEEB initiatives began in 2008, but specific action in the marine sector had a significant lag (TEEB, 2012, Why Value the Oceans-A discussion paper). Publications in ecosystem service science are growing year on year, but this has been skewed toward terrestrial systems, and the gap between marine and terrestrial focused studies has grown over time (Figure 1). Marine studies represent a small proportion of the total ecosystem service literature (< 9% averaged over time), which is perplexing given the levels of human dependence on the coastal environment. Marine and coastal systems will be proximate to approximately 75% of the world's population by 2025 (UN 1992) and one third of coastal regions are now at a high risk of degradation from human activities (https://www.worldoceannetwork.org). Offshore our understanding of the marine environment becomes even more limited, with very few studies having explored deep-sea ecosystem services (e.g., Galparsoro et al., 2012; Jobstvogt et al., 2014a,b; Thurber et al., 2014). This is despite the deep sea being the largest ecosystem type on Earth (oceans cover >70% of Earth and have an average depth of ~4 km).
Fish are the most publicly recognized generators of marine ecosystem services (Holmlund and Hammer, 1999) and marine cultural services; notably tourism associated with coral reefs and sandy beaches, have been a primary focus (Barbier et al., 2008). However, too narrow an application of the ecosystem services concept serves to perpetuate single-sector, product-focused management, which may not necessarily enhance sustainability. An ecosystem services approach requires a broad consideration of multiple services to be effective, particularly supporting-habitat and regulating services that underpin healthy productive ecosystems (Guerry et al., 2012). Marine environments provide a wide array of benefits including global gas and climate regulation, nutrient regulation, carbon storage, waste treatment processing, coastal protection, genetic and medicinal resources, recreation opportunities, spiritual fulfillment, and cultural identity (Peterson and Lubchenco, 1997; Beaumont et al., 2007, 2008; Chan and Ruckelshaus, 2010; Guerry et al., 2011). Healthy, diverse marine ecosystems also provide more abstract benefits including the ecological resilience and resistance of communities to disturbances (Levin and Lubchenco, 2008) that ensure the delivery of services over time. If the benefits provided by marine systems are both diverse and essential, why is there not a more substantial body of work on marine ecosystem services? The simplest answer to this is that marine ecosystems continue to be among the most challenging on Earth to study, and observations and data are scarce in a vast majority of our planet's oceans.
In this paper we review some of the main challenges for applying the ecosystem services concept to marine systems: data scarcity; connectivity; scale; and social value complexities. These are not unique to marine systems, but we reason why they can disproportionally constrain application and limit the progress being made. We also examine some of the ways in which impediments can be, and are being, addressed. We do not consider this an exhaustive assessment and many challenges remain; however, this is a useful process for identifying gaps. We illustrate our points with examples from New Zealand marine ecosystems that we believe to be generally applicable elsewhere. We conclude by considering how limited progress of the ecosystem service approach in the marine realm may affect the perceived value of these systems.
One of the greatest challenges for marine ecosystem service applications is the scarcity of spatial data (Guerry et al., 2012; Liquete et al., 2013; Cabral et al., 2014; Townsend et al., 2014). This challenge is applicable across the natural sciences and to all biomes, but there is an argument that this disproportionally affects marine systems. In terrestrial systems the spatial patterns of land-use/land-cover (LULC) and habitats are easy to access via satellite e.g., Google Earth. Such measures (e.g., forest coverage, tree species) have been used as proxy indicators of service provisions (Riitters et al., 2000; Kareiva et al., 2011; Seddon et al., 2016). Successful applications of high resolution remote sensing have mapped marine habitats such as seagrass, kelp forests and coral reefs to depths of 40 m (Chauvaud et al., 1998; Poursanidis et al., 2018). However, most marine systems are deeper than the limits of remote sensing and coastal waters are frequently turbid, further reducing the potential application. Thus, for the majority of marine realm, there is a basic lack of knowledge on the distribution of habitats (analogous to terrestrial land cover) that can be used as a context for the provision and measurement of ecosystem services (Carr et al., 2003). The physical and highly dynamic nature of the oceans also results in a relatively greater need for both spatial and temporal measurements of ecosystem services. Advances are being made on a number of fronts.
New technology is facilitating the acquisition of spatial data at faster rates and higher resolutions than previously possible. Galparsoro et al. (2012) demonstrated how existing data can be applied in well studied European waters to map ecosystem services over vast areas. These authors produced ecosystem service maps for the North Eastern Atlantic Ocean, covering 1.7 million km2, ranging from the coast of Norway to south of the Canary Islands, and examined the contribution of 62 benthic habitats toward 12 ecosystem services. Even in well studied areas there are data gaps, so alternative ecosystem service approaches have utilized and integrated multiple forms of information. Traditional and expert knowledge has been used successfully in numerous studies along with structured communication techniques, such as the Delphi Method, that are able to generate consensus from groups of experts while reconciling different perspectives and experiences. Jobstvogt et al. (2014a,b) condensed expert knowledge at the fringes of current understanding for poorly understood deep-sea habitats with a consensus-based approach, and by doing so were able to decrease uncertainty.
Some ecosystem service modeling approaches have been developed with minimal data requirements including an “ecosystem principles approach” by Townsend et al. (2014). This was based on linking basic ecological constructs, “ecosystem principles,” to 12 services, using common biophysical parameters and a weighting structure to estimate variation in potential service provision. The Integrated Valuation of Ecosystem Services and Tradeoffs (InVEST) is now capable of mapping, modeling and valuing more services (Kareiva et al., 2011; Tallis and Polasky, 2011). Its expanded range for marine systems addresses eight recognized ES: renewable energy, food from fisheries and aquaculture, coastal protection, aesthetics, recreation, carbon storage and sequestration and water quality (Guerry et al., 2012). While the marine models are relatively basic (Tier 0-1 class; simple to slightly more complex models) compared with terrestrial counterparts (Tier 2 or 3 status; complex models), they have proved to be useful enough for applications in marine spatial management.
When basic data exist on marine habitats, matrix-based ecosystem service models have been used successfully to link different biota to a broad array of ecosystem services using scientific literature and expert opinion (Table 1) (Galparsoro et al., 2012; Cabral et al., 2014; Potts et al., 2014). Matrix models are populated with information on the contribution of each biota-type to the delivery of each service. Information is rarely present for all biota and a strength of a matrix approach in overcoming data scarcity is the integration of information from a range of sources and certainties (Jacobs et al., 2014). Matrix approaches have utility for addressing management challenges, for example, Cabral et al. (2014) combined a simple matrix model that linked 17 marine benthic habitats to the provisions of 11 ecosystem services (condensed into 3 broad groups) in the Normand-Breton Gulf, France. The additional novelty in this work was linking the matrix model to a vulnerability assessment, which focused on the susceptibility of benthic habitats to 11 human induced stressors. This demonstrated how different activities and scenarios of resource use could affect service delivery. However, they still rely heavily on spatial data of habitat types in order to be used.
Table 1. An example of an ecosystem service matrix utilizing expert information and scientific literature to evaluate the importance of marine habitats to specific services.
Techniques that allow multiple ecosystem services to be evaluated are necessary to move beyond single sector perspectives and facilitates discussions among stakeholders (Guerry et al., 2012), but their validity must be demonstrated before they are widely applied. The Box-Draper dictum rightly suggests that “all models are wrong; the practical question is how wrong do they have to be to not be useful.” The difficulty of validation is clearly not unique to marine systems and is applicable to all models. However, for certain multifaceted ecosystem services (e.g., nutrient recycling), validation in marine systems is particularly challenging due to the scarcity of data (Oreskes et al., 1994), a lack of understanding of the ecological processes and functioning that underpin service generation, and the associated difficulty in selecting meaningful indicators (Hattam et al., 2015). For example, benthic-pelagic coupling is frequently an important component of nutrient recycling processes (Graf, 1989) and measurements of this from oceanic systems, where the sediment is on average >3,600 m below the water surface, can be fundamentally challenging. Models have a range of assumptions and in marine systems this typically includes parametrisation from limited spatial and temporal measurements. There is a danger that models are assumed to be correct without due attention to caveats, generalizations or uncertainties and identifying the point at which a model stops being useful can easily be unclear in marine systems. Communicating model uncertainty to non-scientific audiences is an important area that needs to be addressed (Guerry et al., 2012). Uncertainty in ecosystem services maps is potentially dangerous as these can be used without due acknowledgment of the assumptions, formulations or the methods of data integration that underpin them (Cabral et al., 2014). Additionally, a range of participatory modeling methods have been developed that can integrate social values and knowledge of local systems into decision making frameworks while also reducing stakeholder uncertainty and risk by involving stakeholders in modeling (Davies et al., 2015). This is paramount for effective ecosystem-based management and marine spatial planning, to balance competing uses and resolve conflict.
Ecosystem services are not generated or delivered uniformly across seascapes. The seemingly simple exercise of allocating ecosystem services to particular map squares is complicated by unique features of marine ecosystems. Unlike terrestrial systems, where the land and habitats in a grid cell remain relatively stationary, marine waters and their constituents can be driven great distances by winds, tides, and currents. Even with a primary focus on seafloor habitats, the movements of water over them, transporting carbon, oxygen, nutrients, larvae, and contaminants adds significant complexity to marine service quantification and spatial allocation. In effect, the connectedness of grid cells to each other may depend more on the direction and speed of water movements than to proximity. Human uses of marine systems are also three dimensional, incorporating activities that use the sea surface (e.g., shipping), the water column (e.g., hook and line fishing) and the benthic habitats below (e.g., cable laying or mining). Thus, although challenging, a better understanding of the spatial interconnectivity of ecosystem processes and services is required to make advancements in marine systems.
Moreover, the identification and protection of areas where ecosystem services are valued or exploited is not necessarily sufficient to ensure sustained service delivery. Failure to integrate processes and locations important in the generation of a service, sensu “intermediate” services, may lead to impairment in the provision of the “final” service (Haines-Young and Potschin, 2010). For example, the preservation of harvestable fishing grounds may be insufficient to manage this provisioning service, if spawning and nursery grounds are not similarly protected. There are terrestrial equivalents to this e.g., migratory behaviors of bird species and the ramification for bird conservation, and many land-based species with large home-ranges (e.g., Ferguson et al., 1999; Mattisson et al., 2013). However, the basic lack of life history information for most marine species, coupled with fundamental difficulties of tracking marine organisms through fluid environments, means that the full extent of habitat usage and home range limits are often unknown. This puts marine systems at a significant disadvantage for understanding connectivity and the spatial requirements for ecosystem services. Many marine species demonstrate spatially separated ontogenesis which includes the use of both benthic and pelagic habitats. A vulnerability in marine ecosystem service approaches is their narrow focus on the benefits derived from particular services and locations, while neglecting interconnected processes and locations contributing to the generation. This is somewhat akin to single species fisheries management, as opposed to ecosystem-based management. Economists are wary of “double-counting” the value of components that contribute to a valued final service (Chan and Ruckelshaus, 2010; Bateman et al., 2011; Bartkowski et al., 2015), although this is only a problem where the concept of a “final service” is invoked as opposed to consideration of services as a whole. For management, understanding the connections and contributions of spatially arrayed ecosystem components is absolutely critical.
An illustration of ecosystem service connectivity and the potential for spatial separation between intermediate and final marine ecosystem services comes from the Hauraki Gulf, New Zealand (Figure 2), where fishing for snapper is a highly valued commercial and recreational pursuit. On a typical summer day, there can be more than 1,000 recreational boats on the water being used for rod and line fishing, with snapper making up ~80% of the landed catch. Aerial surveys and boat ramp interviews conducted by the Ministry of Primary Industries (Hartill et al., 2012, 2013) have been used to pinpoint the areas of highest fishing intensity. Many of these areas are adjacent to harbors and estuaries, but often not within them (http://www.nabis.govt.nz) (Figure 2). This is despite the fact that structured habitats within estuaries, such as intertidal and shallow subtidal seagrass beds, are known to be nursery grounds for juvenile snapper (Thrush et al., 2002). Moreover, a proportion of Mahurangi Harbor's productivity is exported to the adjacent coast (i.e., more chlorophyll a exits from the estuary on outgoing tides than enters on incoming tides and the shallow waters have higher rates of productivity; AML, unpublished), so a logical hypothesis is that the high productivity of the shallow estuarine habitats is linked to secondary productivity outside the harbor and the populations of harvestable snapper (Figure 2). This illustrates why coastal resource managers need to consider estuarine health and functioning and the wider processes (i.e., intermediate services) in order to preserve the more readily identifiable “final” services (in this case, the provision of wild caught seafood). A number of stressors have been impacting the health of Mahurangi Harbor, most notably terrigenous sediments which affects productivity and structure forming organisms (Thrush et al., 2004); ecosystem service connectivity suggests that the consequences of estuarine stressors may extend to the adjacent coast.
Figure 2(A). Map of New Zealand with the positions of Mahurangi Harbor (B,C) and Kaikoura Peninsula (D) indicated. Panel B presents the bathymetry of Mahurangi Harbor where the most productive waters are intertidal (orange-red) and shallow subtidal (yellow) and located in the main arms and upper reaches of the harbor. The centers of productivity contrast sharply with the areas of highest fishing intensity which are outside of the harbor mouth and in the main harbor channel, shown in Panel C from aerial surveys of recreational fishing effort (NABIS, http://www.nabis.govt.nz). Panel D shows the Kaikoura Peninsula (red circle) and the topography of the region with deep trenches south of the peninsula where cetaceans congregate.
For the highly migratory species, assigning specific services to certain places can have associated problems. Kaikoura, on the South Island of New Zealand, is a coastal town that has been transformed by whale and dolphin based eco-tourism (Figure 2). Multiple whale-watching trips are scheduled each day, there are opportunities to observe whales, swim with dolphins, and fur seal and sea-bird colonies are accessible (Orams, 2002; Curtin, 2003; Cloke and Perkins, 2005). Sites responsible for generating ecotourism income are well known, the value of tourism in economic terms is estimable (Simmons and Fairweather, 1998), and it is possible to identify specific human beneficiaries (e.g., boat operators, hoteliers, tourists). Local management agencies can control and limit the interactions of tour operators with marine mammals to prevent harassment and insure local protection. However, many of the key species are migratory either annually or throughout their lifecycles. Adolescent male sperm whales are resident in Kaikoura where they utilize the productive upwelling waters to feed and grow, before migrating to tropical waters later in life to reproduce. There are many other transient species including humpback, minke, blue, fin, sei, beaked, and pilot whales which pass through Kaikoura and contribute to its international reputation as an area for nature observation. Several of these species are targeted by Japanese fishing efforts which extend outside of Japanese territorial waters. Although benefits may be concentrated spatially in Kaikoura, they are still dependent on activities in locations external to the immediate environment; including those outside of New Zealand national legislation and control (Marine Mammals Protection Act, 1978). Simple links can be drawn between the delivery of specific services and benefits, but it is clear there are complex spatial underpinnings. Additionally, these megafauna contribute to a range of other values that cannot be easily monetised. Management must ensure that the interactions between different ecosystem components (water column, seafloor, sedentary, and migratory species), uses (tourism, fishing, mineral extraction), and values (indigenous, local, national), both near and far, are not overlooked.
We suggest that, rather than dividing services into “intermediate” and “final” components and focussing on service quantity, studies should be explicit about (i) locations where a service is generated, (ii) locations where a service is realized, (iii) how these locations are connected, and (iv) who the beneficiaries are (Figure 2). Determining who benefits and who loses in marine ecosystem service assessments requires explicit consideration of social, cultural, and economic system components, and a commitment to participatory processes that guide the determination of values. We argue that this type of explicitness is important as we transition the ecosystem services concept into a more practical and useful tool. Nevertheless, for some types of marine ES, this type of explicitness is difficult to put into practice.
Spatial scale and spatial requirements are recurrent themes for services delivery from marine systems. A single marine habitat or organism type can be linked to the provision of multiple services, and these services typically operate over a range of spatial scales (Raffaelli and White, 2013). As a result there are complex relationships that require clarity and explicitness to avoid confusion; namely which habitat, which ecosystem service and who is benefiting. This is challenging because the aspirations of many ecosystem services applications are to provide broad information relevant to management e.g. covering multiple services, focusing on areas that contain multiple coastal or estuarine habitats, and where beneficiaries have not necessarily been defined.
Using an example, mangroves are woody vascular plants occupying semi-submerged coastal shoreline in many parts of the world (Morrisey et al., 2010). Mangrove forests are well-known as a significant contributor to the marine environment (Spalding et al., 2010; Donato et al., 2011), providing essential habitat for fish and invertebrate species, raw material, fuel and trapping sediments and wastes (Lugo and Snedaker, 1974; Granek et al., 2009; Buelow and Sheaves, 2015). Mangroves are highlighted for their role in coastal protection as the trunks and complex root and branch architecture dampens wind and waves (Alongi, 2008; Christensen et al., 2008; Narayan et al., 2016), which can protect life and property during storms (Othman, 1994; Phan et al., 2015). In the context of coastal protection, only a few hectares may be sufficient to yield significant benefits to a local population. Similarly, relatively small areas of mangrove forest may be critical to support a local subsistence fishery as juvenile nursey ground or for building material (Sandilyan and Kathiresan, 2012); whilst the economic benefits of maintaining mangroves in terms of avoided losses from tropical storms can also be quantified at the local level (Barbier, 2006). However, in the context of more global services e.g., climate regulation, we may need to consider mangroves at the scale of the tropics, where they cover hundreds of square kilometers, or at larger scales before the area becomes meaningful to service delivery. Regardless, high spatial variation in rates of carbon cycling and storage makes it challenging to extrapolate the contributions of mangroves patches to global carbon budgets (Bouillon et al., 2008; Lovelock, 2008). Thus, there is no single scale from which all the benefits of mangroves, or other marine habitats, can be reasonably or sufficiently captured. The concept of “service providing unit” (SPU, Luck et al., 2003, 2009) and “Ecosystem Service Providers” (Kremen, 2005) is clearly habitat-, service-, and context-specific.
Services themselves are context specific, where a thick band of mangroves in front of an uninhabited coastline may have comparatively low or no value for coastal protection services compared to the same benefits generated in front of a highly populated coastline. Context specificity is a common occurrence in ecology (Cardinale et al., 2000; Duffy et al., 2005; Needham et al., 2011; Chamberlain et al., 2014) and is crucial to the assessment of social and economic values, but has not always been adequately addressed in ecosystem service applications. For example, the service of biodiversity enhancement and the contribution of mangroves to fisheries production and tourism values varies substantially from place to place. Most documentation of this comes from tropical ecosystems (Halpern, 2004), whereas the benefits at higher latitudes are much more limited (Morrisey et al., 2010). In New Zealand, the only native mangrove species, Avicennia marina, typically is submerged for less than two h per day, and depths at high tide in most New Zealand mangrove forests are less than 50 cm, preventing equivalent use by fish compared to their tropical counterparts. This has not prevented New Zealand mangroves from being possibly overvalued in economic studies that have extrapolated economic values from tropical literature that include this fishery nursery value (van den Belt and Cole, 2014).
Historically, ecosystem services have been measured in biophysical or economic terms and utilized to increase interest and investment in environmental management (Daily et al., 2009). Analyses of marine ecosystem services are dominated by modeling tools, scenarios and trade-offs that have either economic or biophysical focus (Guerry et al., 2012). However, these approaches do not fully account for the many ways that humans benefit from or value nature. Values are beliefs about desirable states or modes of conduct that guide behavior and prioritization (Schwartz, 1994). Values are not static; they can shift as a result of learning or changes in society, situation, or self-conception (Rokeach, 1973). Cultural services and cultural values are terms that are used widely and loosely in the ecosystem services literature, yet these differ between generations, ethnicities, religions, countries of origin, income level, sectors of society, and location of residence (Hofstede, 1991; Hebel, 1999). Social values can be difficult to parameterise/metricise and the degree to which they can be meaningfully integrated with other fundamentally disparate value types has been disputed (Bryan et al., 2010; Chan et al., 2012; Daniel et al., 2012; Harmsworth and Awatere, 2014). Attempts to identify multiple values should consider social values in addition to environmental and economic components, and the interactions among them (Raymond et al., 2009; Reyers et al., 2013).
Incorporating human values into coastal and marine ecosystem service approaches is complicated by a wide range of often conflicting values to consider, and the complex use and governance systems in place in these areas. Conflicts in value can be exacerbated by marine resources being primarily publicly owned. This “shared” responsibility invokes strong feelings of personal responsibility and custodianship but also the feeling of inherent rights to engage in certain activities. Sentiments of collective ownership result in a high proportion of social and cultural importance; and increase the focus and value of customary rights (Thompson, 1991). The multiplicity of uses and values associated with relatively constrained coastal areas can lead to conflict among, for example, anthropocentric (human well-being focused) and biocentric (environmental and all species well-being focused) worldviews (Mace, 2014). This friction can be seen in debates about issues such as designating marine protected areas (MPAs): while many argue that MPAs impinge on local fishing rights, others assert that some areas should be set aside for the protection of fish and other species without regard to human interests (Agardy, 2003). Even in offshore marine environments, where most people never go and uses are generally limited to commercial fishing and industrial interests such as mining or oil and gas exploration, high social value is increasingly placed on keeping these areas “untouched” or “wild” (Karlõševa et al., 2016).
The arguments are becoming increasingly nuanced as the dynamic relationships that exist between people and the environment are more broadly recognized, and holistic approaches based on social-ecological systems begin to emerge (Carpenter et al., 2009). However, while some sectors may move rapidly to embrace social-ecological systems approaches, others are likely to lag far behind. For example, coastal and marine governance arrangements that dictate use and access rights have been developed to manage common pool resources such as fish stocks within the confines of particular cultural contexts and constraints (Ostrom, 1990). In New Zealand, customary rights and title regarding coastal and marine areas must be carefully negotiated without preventing existing rights and uses related to fishing, aquaculture, or public access [Marine and Coastal Area (Takutai Moana) Act, 2011]. Disrupting these systems to produce more adaptable and resilient ones requires certain institutional components to be in place over a range of temporal and spatial scales. These challenges all highlight the need to carefully assess whose values are given priority when it comes to defining the value of marine ecosystem services.
As the importance of incorporating social dynamics and human values into ecosystem services valuation processes has become more obvious to decision makers and researchers alike, efforts to address these gaps have gained momentum. For example, non-market valuation methods such as revealed preference and stated preference methods have been developed to capture the value of services that are not traded in markets (National Research Council, 2004), and have been applied extensively to coastal and marine environments (Hanley et al., 2015; Torres and Hanley, 2016). Stated preference methods have also been used to estimate the values of biodiversity conservation of deep sea areas in Scotland and Norway (Jobstvogt et al., 2014a,b; Aanesen et al., 2015). Spatial measures of social values and preferences have been made using stated preference surveys and ecosystem service maps, and have increasingly included participatory elements (Brown, 2005; Raymond et al., 2009; Bryan et al., 2010; Klain and Chan, 2012). A participatory mapping study conducted with a diverse range of coastal stakeholders in New Zealand's Manukau Harbor revealed that participants associate more of their values with seascapes than landscapes, a finding which may suggest that land-based decision-making approaches that do not explicitly consider coastal goods and services are perpetuating poor-decision making and conflict in coastal areas (Davies, 2015). Meanwhile, a recent critique of participatory processes associated with ecosystem services found a disturbing lack of consideration of power relationships inherent in these methods, and highlighted the urgent need for more work in this area (Davies et al., 2015). Further exciting work is being guided by indigenous researchers, who are restructuring the ecosystem services framework to more appropriately reflect their worldviews (Pert et al., 2015), and the possibility of refocusing frameworks on “relational values,” defined as preferences, principles, and virtues associated with relationships, both interpersonal and as articulated by policies and social norms, has also been suggested as a way forward (Chan et al., 2016).
Not surprisingly given these concerns, cultural service valuation in marine spaces has proven to be highly contentious to undertake. Cultural services provide immaterial benefits such as spiritual fulfillment, and a lack of cultural services are often central to public dissatisfaction with environmental decision making. A wide range of methods have been utilized to account for individual cultural services, but few provide satisfactory integrated results (Daniel et al., 2012). For example, while cultural services such as the ecotourism of coral reefs are relatively easy to quantify, services that are harder to incorporate, such as the spiritual value associated with watching the sun set over the sea, are frequently overlooked in ecosystem service assessments, and the overlap between these two services is not considered. While it can seem useful to determine the monetary value associated with ecosystem services for decision making purposes, few markets currently exist for these marine and coastal ecosystem services (Drake et al., 2013), which makes these values primarily useful on a local scale rather than in a national or global context and, therefore, easily ignored in largescale, long-term decision-making contexts.
The challenges of implementing the ecosystem services concept in the marine environment also extend to subsequent economic valuation. Economic valuation is similarly hampered by the scarcity of spatial data needed to underpin service quantification and valuation, the complexities of spatial scale and the scale at which services are valued, and the struggle to capture, articulate, and include a sufficient range of human values. We posit that a failure to sufficiently progress the ecosystem service approach in marine systems could lead to significant undervaluation, rather than overvaluation of ecosystem services. This may occur through a focus of valuation on single or simple services at the exclusion of others (Sathirathai and Barbier, 2001; Barbier et al., 2008; Guerry et al., 2012), generating a bias toward valuing locations where data are available and failure to capture services that operate at large scales. When data are sparse, it is important to recognize that “the absence of evidence is not evidence of absence” with respect to the generation of ecosystem services or their value. There is a risk that marine ecosystems are perceived as being less valuable simply because their actual value has not been sufficiently quantified. There may also be a failure to adequately record the losses of value associated with environmental damage. For example, as already highlighted, the issues of data scarcity indicates that for much of the world's oceans, there is little information on the habitats and biota present. There are established links between marine bivalves and services such as carbon sequestration and water filtration (Filgueira et al., 2015), between bioturbators and nutrient recycling and other ecosystem regulatory processes (Lohrer et al., 2004, 2015), and between biodiversity and benthic community resilience (Stachowicz et al., 2007). There are also robust empirical studies that demonstrate broad-scale and long term change to benthic communities from destructive fishing practices (Thrush and Dayton, 2002; Thrush et al., 2015). Changes in value have been illustrated by Costanza et al. (1997) and 2014, where marine systems shift from contributing 63 to 39.8% of the total value of services across the biosphere. Over this period, they reported a median increase of 59.9% across marine biomes, but a 464.5% increase across terrestrial/freshwater biomes. Pendleton et al. (2016) analyzed and critiqued the updated valuation based on double-counting, selection summary statistics and other issues. Pendleton et al. (2016) noted that 95% of the estimated change in value was attributable to coral reefs. Thus, relative stasis in the economic value of marine ecosystem services, with the exception of coral reefs, does not correspond with the observation that one third of coastal regions are now at a high risk of degradation from human activities (Thrush et al., 1998).
The ecosystem services concept is an attempt to create a visible and useful link between ecosystems and humans. The fundamental challenge is to provide explicit descriptions and adequate assessment of the links between structure and functioning of the natural system and the economic benefits we draw form it (Barbier, 2012). As the functioning of an ecosystem underpins the services it provides, many of the issues that form ecological theory are important for measuring and mapping ecosystem services. In marine systems, issues of connectivity and scale are particularly important and are still being grappled with in a number of research areas. However, in ecosystem service research these issues are only just emerging and techniques to incorporate connectivity, in particular, are generally not available. Context-dependency is also a present area of research in all ecosystems and this means not only that the biophysical measure of a service may differ depending on environmental, stressor, ecological community and historical context, but also on the social dynamics and cultural and economic values present.
At present, ecosystem services are predominantly visualized as a management tool, supporting impact scenarios of human activities and trade-offs between actions. This makes them inherently location dependent, with a requirement to understand spatial and temporal variation. In marine systems this emphasizes the problems of present data scarcity and the difficulties and expense of collecting data to fill gaps. These problems also often result in habitat-specific information (e.g., coral reefs and mangroves) or on single rather than multiple ecosystem services. While models are increasingly being developed to fill gaps, these are frequently based on non-ecological data, and it is important to understand the limitations and simplifications required for the models and the caveats on the measures produced. In particular, validation at a variety of scales and locations is required, which in its turn would benefit from some development of effective and cheap sampling techniques and standardized metrics/indicators of marine ecosystem services (Seppelt et al., 2011; Burkhard et al., 2012; Hattam et al., 2015).
The prevailing approach to ecosystem services and their value has thus far been anthropocentric–which is to say that ecosystem services have value in so far as they contribute to human well-being. There are other, more holistic approaches to valuation that could be utilized; for example, the biocentric approach weights other species and their well-being on par with that of humans, but this approach has proven difficult to implement (Goulder and Kennedy, 2011). This tension has highlighted the importance of considering how and who determines ecosystem service values, and this continues to be an area of active research and negotiation. Attempts to create a more holistic value structure have led to the inclusion of resilience and biodiversity in the value of supporting services. Interactions between different components of an ecosystem means that consideration of an impact on a single service are likely to incorrectly predict responses and that non-linearity and threshold effects are most likely as connections break and effects cascade (van der Heide et al., 2012; Thrush et al., 2014). In marine systems regime shifts and threshold effects have been demonstrated in response to a number of pressures (Levin and Möllmann, 2015; Hewitt et al., 2016).
In summary, marine ecosystem service research still faces several challenges: data scarcity and difficulty in collecting data; the degree to which processes are spatially and temporally dynamic; differences in where services may be generated and where they may be valued; and the degree of importance of social dynamics and cultural values in publicly owned spaces. In response to these challenges some useful techniques are developing. For example, models to predict multiple ecosystem services, public participation in spatial planning exercises allowing non-monetary values as well as economic values to be considered. We would also suggest three other “best practice” points. Firstly, location mismatches between service generation and delivery are not always elucidated by concepts of “intermediate,” “supporting,” and “final” services. Instead, explicitly discussing locations and scales of connectivity between generation and delivery is likely to be more useful. In a simple form, this could be communicating the ways in which estuaries underpin the benefits realized elsewhere in the coastal marine environment or how certain habitats and locations support local fisheries. Secondly, ecosystem services should be more explicitly linked to social and cultural values and research is needed in this area. It is the connection to value, where preferences can be understood and behaviors toward the environment influenced (Hicks et al., 2015). Thirdly, a more holistic view of ecosystems and the services they provide needs to be encouraged and studied. In New Zealand this aligns more closely with the indigenous cultural view, where Māori consider the environment in its entirety from “the mountains to the sea” in a concept referred to as Ki uta ki tai (Tipa et al., 2016). Interconnections between ecosystem services requires studying multiple services, and both this and the concept that the ecosystem is more than the sum of its services, are not aided by the fear of “double counting.” Finally, a sound ecological foundation is needed if we hope to measure and use the ecosystem services concept in a way that will support human well-being now and throughout future generations.
MT and AL conceived the present idea, structured the manuscript, and contributed to all sections. CL, KD, and NH, contributed to sections Scale and Context-Specificity, Social dynamics and Value Complexities, Valuing Marine Ecosystem Services, respectively. JH helped structure the manuscript and contributed to section Making Progress in Marine Systems.
The authors declare that the research was conducted in the absence of any commercial or financial relationships that could be construed as a potential conflict of interest.
This work was supported by the Ministry for Business, Innovation and Employment (MBIE) Contract No. C01X1227 (Environmental futures in New Zealand's marine ecosystems: a dynamic approach toward managing for resilience), MBIE Contract No. C01X1515 (Sustainable Seas National Science Challenge project 2.1.3), and NIWA Coasts and Oceans Research Programme 5 (SCI 2015/16).
Aanesen, M., Armstrong, C., Czajkowski, M., Falk-Petersen, J., Hanley, N., and Navrud, S. (2015). Willingness to pay for unfamiliar public goods: preserving cold-water corals in Norway. Ecol. Econ. 112, 53–67. doi: 10.1016/j.ecolecon.2015.02.007
Agardy, T. (2003). “An environmentalist's perspective on responsible fisheries: the need for holistic approaches,” in Responsible Fisheries in the Marine Ecosystem, eds M. Sinclair and G. Valdimarsson. (Wallingford; Oxon; Cambridge, MA: CABI Publishing), 65–85.
Alongi, D. M. (2008). Mangrove forests: resilience, protection from tsunamis, and responses to global climate change. Estuar. Coast Shelf S. 76, 1–13. doi: 10.1016/j.ecss.2007.08.024
Barbier, E. B. (2006). Natural barriers to natural disasters: replanting mangroves after the tsunami. Front. Ecol. Environ. 4, 124–131. doi: 10.1890/1540-9295(2006)004[0124:NBTNDR]2.0.CO;2
Barbier, E. B. (2012). Ecosystem Services and Wealth Accounting Chapter 9. in UNU-IHDP and UNEP. Inclusive Wealth Report 2012. Measuring Progress Towards Sustainability. Cambridge: Cambridge University Press.
Barbier, E. B., Koch, E. W., Silliman, B. R., Hacker, S. D., Wolanski, E., Primavera, J., et al. (2008). Coastal ecosystem-based management with nonlinear ecological functions and values. Science 319, 321–323. doi: 10.1126/science.1150349
Barnosky, A. D., Matzke, N., Tomiya, S., Wogan, G. O. U., Swartz, B., Quental, T. B., et al. (2011). Has the earth's sixth mass extinction already arrived? Nature 471, 51–57. doi: 10.1038/nature09678
Bartkowski, B., Lienhoop, N., and Hansjürgens, B. (2015). Capturing the complexity of biodiversity: a critical review of economic valuation studies of biological diversity. Ecol. Econ. 113, 1–14. doi: 10.1016/j.ecolecon.2015.02.023
Bateman, I., Mace, G., Fezzi, C., Atkinson, G., and Turner, K. (2011). Economic analysis for ecosystem service assessments. Environ. Resour. Econ. 48, 177–218. doi: 10.1007/s10640-010-9418-x
Beaumont, N. J., Austen, M. C., Atkins, J. P., Burdon, D., Degraer, S., Dentinho, T. P., et al. (2007). Identification, definition and quantification of goods and services provided by marine biodiversity: implications for the ecosystem approach. Mar. Pollut. Bull. 54, 253–265. doi: 10.1016/j.marpolbul.2006.12.003
Beaumont, N. J., Austen, M. C., Mangi, S. C., and Townsend, M. (2008). Economic valuation for the conservation of marine biodiversity. Mar. Pollut. Bull. 56, 386–396. doi: 10.1016/j.marpolbul.2007.11.013
Bouillon, S., Borges, A. V., Castaneda-Moya, E., Diele, K., Dittmar, T., Duke, N. C., et al. (2008). Mangrove production and carbon sinks: a revision of global budget estimates. Glob. Biogeochem. 22:GB2013. doi: 10.1029/2007GB003052
Brown, G. (2005). Mapping spatial attributes in survey research for natural resource management: methods and applications. Soc. Natur. Resour. 18, 17–39. doi: 10.1080/08941920590881853
Bryan, B. A., Raymond, C. M., Crossman, N. D., and Hatton Macdonald, D. (2010). Targeting the management of ecosystem services based on social values: Where, what, and how? Landscape Urban Plan. 97, 111–122. doi: 10.1016/j.landurbplan.2010.05.002
Buelow, C., and Sheaves, M. (2015). A birds-eye view of biological connectivity in mangrove systems. Estuar. Coast. Shelf Sci. 152, 33–43. doi: 10.1016/j.ecss.2014.10.014
Burkhard, B., Kroll, F., Nedkov, S., and Müller, F. (2012). Mapping ecosystem service supply, demand and budgets. Ecol. Indic. 21, 17–29. doi: 10.1016/j.ecolind.2011.06.019
Cabral, P., Levrel, H., Schoenn, J., Thiébaut, E., Le Mao, P., Mongruel, R., et al. (2014). Marine habitats ecosystem service potential: a vulnerability approach in the Normand-Breton (Saint Malo) Gulf, France. Ecosyst. Serv. 16, 306–318. doi: 10.1016/j.ecoser.2014.09.007
Cardinale, B. J., Nelson, K., and Palmer, M. A. (2000). Linking species diversity to the functioning of ecosystems: on the importance of environmental context. Oikos 91, 175–183. doi: 10.1034/j.1600-0706.2000.910117.x
Carpenter, S. R., Mooney, H. A., Agard, J., Capistrano, D., DeFries, R. S., Diaz, S., et al. (2009). Science for managing ecosystem services: beyond the millennium ecosystem assessment. Proc. Natl. Acad. Sci. U.S.A. 106, 1305–1312. doi: 10.1073/pnas.0808772106
Carr, M., Neigel, J., Estes, J. A., Andelman, S., Warner, R. R., and Largier, J. (2003). Comparing marine and terrestrial ecosystems: implications for the design of coastal marine reserves. Ecol. App. 13, S90–S107. doi: 10.1890/1051-0761(2003)013[0090:CMATEI]2.0.CO;2
Chamberlain, S. A., Bronstein, J. L., and Rudgers, J. A. (2014). How context dependent are species interactions? Ecol. Lett. 17, 881–890. doi: 10.1111/ele.12279
Chan, K. M. A., Balvanera, P., Benessaiah, K., Chapman, M., Díaz, S., Gómez-Baggethun, E., et al. (2016). Why protect nature? rethinking values and the environment. Proc. Natl. Acad. Sci. U.S.A. 113, 1462–1465. doi: 10.1073/pnas.1525002113
Chan, K. M. A., and Ruckelshaus, M. (2010). Characterizing changes in marine ecosystem services. F1000 Biol. Rep. 2:54. doi: 10.3410/B2-54
Chan, K. M. A., Satterfield, T., and Goldstein, J. (2012). Rethinking ecosystem services to better address and navigate cultural values. Ecol. Econ. 74, 8–18. doi: 10.1016/j.ecolecon.2011.11.011
Chauvaud, S., Bouchon, C., and Maniére, R. (1998). Remote sensing techniques adapted to high resolution mapping of tropical coastal marine ecosystems (coral reefs, seagrass beds and mangrove). Int. J. Remote Sens. 19, 3625–3639.
Christensen, S. M., Tarp, P., and Hjortsø, C. N (2008). Mangrove forest management planning in coastal buffer and conservation zones, Vietnam: a multi-methodological approach incorporating multiple stakeholders. Ocean Coast Manage. 51, 712–726. doi: 10.1016/j.ocecoaman.2008.06.014
Cloke, P., and Perkins, C. (2005). Cetacean performance and tourism in Kaikoura, New Zealand. Environ. Plann. D. 23, 903–924. doi: 10.1068/d57j
Costanza, R., Arge, D., Groot, D., Farber, S., Grasso, M., Hannon, B., et al. (1997). The value of ecosystem service and nature capital in the world. Nature. 387, 235–260.
Costanza, R., de Groot, R., Sutton, P., van der Ploeg, S., Anderson, S. J., Kubiszewskia, I., et al. (2014). Changes in the global value of ecosystem services. Glob. Environ. Chang. 26, 152–158. doi: 10.1016/j.gloenvcha.2014.04.002
Curtin, S. (2003). Whale-watching in Kaikoura: sustainable destination development? J. Ecotour. 2, 173–195. doi: 10.1080/14724040308668143
Daily, G. C., Alexander, S., Ehrlich, P. R., Goulder, L., Lubchenco, J., Matson, P. A., et al. (1997). Ecosystem Services: Benefits Supplied to Human Societies by Natural Ecosystems. Washington, DC: Ecological Society of America.
Daily, G. C., Polasky, S., Goldstein, J., Kareiva, P. M., Mooney, H. A., Pejchar, L., et al. (2009). Ecosystem services in decision making: time to deliver. Front. Ecol. Environ. 7, 21–28. doi: 10.1890/080025
Daniel, T. C., Muhar, A., Arnberger, A., Aznar, O., Boyd, J. W., Chan, K. M. A., et al. (2012). Contributions of cultural services to the ecosystem services agenda. Proc. Natl. Acad. Sci. U.S.A. 109, 8812–8819. doi: 10.1073/pnas.1114773109
Davies, K. (2015). Many Voices of the Manukau: Participatory Modelling, Ecosystem Services and Decision Making in New Zealand. Doctor of Philosophy. Auckland: The University of Auckland. Available online at: https://researchspace.auckland.ac.nz/handle/2292/25931
Davies, K., Fisher, K., Dickson, M., Thrush, S. F., and LeHeron, R. (2015). Improving ecosystem service frameworks to address wicked problems. Ecol. Soc. 20:37. doi: 10.5751/ES-07581-200237
de Groot, R., Brander, L., van der Ploeg, S., Costanza, R., Bernard, F., Braat, L., et al. (2012). Global estimates of the value of ecosystems and their services in monetary units. Ecosyst. Serv. 1, 50–61. doi: 10.1016/j.ecoser.2012.07.005
Diaz, S., Fargione, J., Chapin, F. S., and Tilman, D. (2006). Biodiversity loss threatens human well-being. PLoS Biol. 4:e277. doi: 10.1371/journal.pbio.0040277
Donato, D. C., Kauffman, J. B., Murdiyarso, D., Kurnianto, S., Stidham, M., and Kanninen, M. (2011). Mangroves among the most carbon-rich forests in the tropics. Nat. Geosci. 4, 293–297. doi: 10.1038/ngeo1123
Drake, B., Smart, J. C. R., Termansen, M., and Hubacek, K. (2013). Public preferences for production of local and global ecosystem services. Reg. Environ. Change.13, 649–659. doi: 10.1007/s10113-011-0252-7
Duffy, J. E., Richardson, J. P., and France, K. E. (2005). Ecosystem consequences of diversity depend on food chain length in estuarine vegetation. Ecol. Lett. 8, 301–309. doi: 10.1111/j.1461-0248.2005.00725.x
Ferguson, S. H., Taylor, M. K., Born, E. W., Rosing-Asvid, A., and Messier, F. (1999). Determinants of home range size for polar bears (Ursus maritimus). Ecol. Lett. 2, 311–318.
Filgueira, R., Byron, C. J., Comeau, L. A., Costa-Pierce, B., Cranford, P. J., Ferreira, J. G., et al. (2015). An integrated ecosystem approach for assessing the potential role of cultivated bivalve shells as part of the carbon trading system. Mar. Ecol. Prog. Ser. 518, 281–287. doi: 10.3354/meps11048
Galparsoro, I., Connor, D. W., Borja, A., Aish, A., Amorim, P., Bajjouk, T., et al. (2012). Using EUNIS habitat classification for benthic mapping in European seas: present concerns and future needs. Mar. Pollut. Bull. 64, 2630–2638. doi: 10.1016/j.marpolbul.2012.10.010
Goulder, L., and Kennedy, D. (2011). “Interpreting and estimating the value of ecosystem services,” in Natural Capital: Theory and Practice of Mapping Ecosystem Services, eds P. Kareiva, H. Tallis, T. H. Ricketts, G. C. Daily, and S. Polasky (Oxford: Oxford University Press), 34–53.
Granek, E. F., Compton, J. E., and Phillips, D. L. (2009). Mangrove-exported nutrient incorporation by sessile coral reef invertebrates. Ecosystems. 12, 462–472. doi: 10.1007/s10021-009-9235-7
Guerry, A. D., Plummer, M. L., Ruckelshaus, M. H., and Harvey, C. (2011). “Ecosystem service assessments for marine conservation,” in Natural Capital: Theory and Practice of Mapping Ecosystem Services, eds P. Kareiva, H. Tallis, T. H. Ricketts, G. C. Daily, and S. Polasky (Oxford: Oxford University Press), 296–322.
Guerry, A. D., Ruckelshaus, M. H., Arkema, K. K., Bernhardt, J. R., Guannel, G., Kim, C., et al. (2012). Modeling benefits from nature: using ecosystem services to inform coastal and marine spatial planning. Int. J. Biodivers. Sci. Ecosyst. Serv. Manage. 8, 107–121. doi: 10.1080/21513732.2011.647835
Haines-Young, R., and Potschin, M. (2010). “The links between biodiversity, ecosystem services and human well-being,” in Ecosystem Ecology, eds D. G. Raffaelli and C. L. J. Frid (Cambridge: A New SynthesisCambridge University Press, British Ecological Society), 110–139.
Halpern, B. S. (2004). Are mangroves a limiting resource for two coral reef fishes? Mar. Ecol. Prog. Ser. 272, 93–98. doi: 10.3354/meps272093
Hanley, N., Hynes, S., Jobstvogt, N., and Paterson, D. (2015). Economic valuation of marine and coastal ecosystems: is it currently fit for purpose?. J Ocean Coast Econ. 2, 1–24. doi: 10.15351/2373-8456.1014
Harmsworth, G. R., and Awatere, S. (2014). “Indigenous Māori knowledge and perspectives of ecosystems,” in Ecosystem Services in New Zealand–Condition and Trends, ed J. Dymond (Lincoln, OR: Manaaki Whenua Press), 274–286.
Hartill, B., Bian, R., Rush, N., and Armiger, H. (2013). Aerial-Access Recreational Harvest Estimates For Snapper, Kahawai, Red Gurnard, Tarakihi And Trevally In FMA 1 in 2011–12. New Zealand Fisheries Assessment Report 2013/70. 44 p.
Hartill, B. W., Cryer, M., Lyle, J. M., Rees, E. B., Ryan, K. L., Steffe, A. S., et al. (2012). Scale-and context-dependent selection of recreational harvest estimation methods: the Australasian experience. N. Am. J. Fish. Manage. 32, 109–123. doi: 10.1080/02755947.2012.661387
Hattam, C., Atkins, J. P., Beaumont, N., Börger, T., Böhnke-Henrichs, A., Burdon, D., et al. (2015). Marine ecosystem services: linking indicators to their classification. Ecol. Indic. 49, 61–75. doi: 10.1016/j.ecolind.2014.09.026
Hebel, M. (1999). World-view as the emergent property of human value system. Syst. Res. Behav. Sci. 16, 253–261.
Hewitt, J. E., Ellis, J. I., and Thrush, S. F. (2016). Multiple stressors, nonlinear effects and the implications of climate change impacts on marine coastal ecosystems. Glob. Chang. Biol. 22, 2665–2675. doi: 10.1111/gcb.13176
Hicks, C. C., Cinner, J. E., Stoeckl, N., and McClanahan, T. R. (2015). Linking ecosystem services and human-values theory. Conserv. Biol. 29, 1471–1480. doi: 10.1111/cobi.12550
Hofstede, G. (1991). Cultures and Organisations: Software of the Mind. London: McGraw-Hill International Limited.
Holmlund, C. M., and Hammer, M. (1999). Ecosystem services generated by fish populations. Ecol. Econ. 29, 253–268.
Jacobs, S., Burkhard, B., Van Daele, T., Staes, J., and Schneiders, A. (2014). ‘The Matrix Reloaded': a review of expert knowledge use for mapping ecosystem services. Ecol. Model. 295, 21–30. doi: 10.1016/j.ecolmodel.2014.08.024
Jobstvogt, N., Hanley, N., Hynes, S., Kenter, J., and Witte, U. (2014a). Twenty thousand sterling under the sea: estimating the economic value of protecting deep sea biodiversity. Ecol. Econ. 97, 10–19. doi: 10.1016/j.ecolecon.2013.10.019
Jobstvogt, N., Townsend, M., Witte, U., and Hanley, N. (2014b). How can we identify and communication the ecological value of deep-sea ecosystem services? PLoS ONE 9:e100646. doi: 10.1371/journal.pone.0100646
Kareiva, P., Tallis, H., Ricketts, T., Daily, G., and Polasky, S. (2011). Natural Capital: Theory and Practice of Mapping Ecosystem Services. Oxford: Oxford University Press.
Karlõševa, A., Nõmmann, S., Nõmmann, T., Urbel-Piirsalu, E., Budzinski, W., Czajkowski, M., et al. (2016). Marine trade-offs: comparing the benefits of off-shore wind farms and marine protected areas. Energ. Econ. 55, 127–134. doi: 10.1016/j.eneco.2015.12.022.
Klain, S. C., and Chan, K. M. A. (2012). Navigating coastal values: participatory mapping of ecosystem services for spatial planning. Ecol. Econ. 82, 104–113. doi: 10.1016/j.ecolecon.2012.07.008
Kremen, C. (2005). Managing ecosystem services: what do we need to know about their ecology? Ecol. Lett. 8, 468–479. doi: 10.1111/j.1461-0248.2005.00751.x
Lele, S., Springate-Baginski, O., Lakerveld, R., Deb, D., and Dash, P. (2013). Ecosystem services: origins, contributions, pitfalls and alternatives. Conserv. Soc. 11, 343–358. doi: 10.4103/0972-4923.125752
Levin, P. S., and Möllmann, C. (2015). Marine ecosystem regime shifts: challenges and opportunities for ecosystem-based management. Phil. Trans. R. Soc. B. 370:20130275. doi: 10.1098/rstb.2013.0275
Levin, S. A., and Lubchenco, J. (2008). Resilience, robustness, and marine ecosystem-based management. Bioscience 58, 27–32. doi: 10.1641/B580107
Liquete, C., Piroddi, C., Drakou, E. G., Gurney, L., Katsanevakis, S., Charef, A., et al. (2013). Current status and future prospects for the assessment of marine and coastal ecosystem services: a systematic review. PLoS ONE 8:e67737. doi: 10.1371/journal.pone.0067737
Lohrer, A. M., Thrush, S. F., and Gibbs, M. M. (2004). Bioturbators enhance ecosystem function through complex biogeochemical interactions. Nature. 431, 1092–1095. doi: 10.1038/nature03042
Lohrer, A. M., Thrush, S. F., Hewitt, J. E., and Kraan, C. (2015). The up-scaling of ecosystem functions in a heterogeneous world. Sci Rep. 5:10439. doi: 10.1038/srep10349
Lovelock, C. E. (2008). Soil respiration and belowground carbon allocation in mangrove forests. Ecosystems 11, 342–354. doi: 10.1007/s10021-008-9125-4
Luck, G. W., Daily, G. C., and Ehrlich, P. R. (2003). Population diversity and ecosystem services. Trends Ecol. Evol. 18, 331–336. doi: 10.1016/S0169-5347(03)00100-9
Luck, G. W., Harrington, R., Harrison, P. A., Kremen, C., Berry, P. M., Bugter, R., et al. (2009). Quantifying the contribution of organisms to the provision of ecosystem services. Bioscience 59, 223–235. doi: 10.1525/bio.2009.59.3.7
Malthus, T. R. (1798). An Essay on the Principle of Population. London: Electronic Scholarly Publishing Project. Available online at: http://www.esp.org/books/malthus/population/malthus.pdf
Marine Mammals Protection Act (1978). Available online at: http://www.legislation.govt.nz/act/public/1978/0080/latest/DLM25115.html
Marine Coastal Area (Takutai Moana) Act (2011). Available online at: http://www.legislation.govt.nz/act/public/2011/0003/latest/DLM3213131.html
Mattisson, J., Sand, H., Wabakken, P., Gervasi, V., Liberg, O., Linnell, J. D., et al. (2013). Home range size variation in a recovering wolf population: evaluating the effect of environmental, demographic, and social factors. Oecologia 173, 813–825. doi: 10.1007/s00442-013-2668-x
Millennium Ecosystem Assessment (2005). Ecosystem and Human Well-Being: Current State and Trends. Washington, DC: Island Press.
Morrisey, D. J., Swales, A. W., Dittmann, S., Morrison, M. A., Lovelock, C. E., and Beard, C. M. (2010). The ecology and management of temperate mangroves. Oceanogr. Mar. Bio. 48, 43–160. doi: 10.1201/EBK1439821169-c2
Narayan, S., Beck, M. W., Reguero, B. G., Losada, I. J., van Wesenbeeck, B., Pontee, N., et al. (2016).The effectiveness, costs and coastal protection benefits of natural and nature-based defences. PLoS ONE 11:e0154735. doi: 10.1371/journal.pone.0154735
National Research Council (2004). Valuing Ecosystem Services: Toward Better Environmental Decision-Making. Washington, D.C. Available online at: http://www.nap.edu/openbook.php?record_id=11139
Needham, H. R., Pilditch, C. A., Lohrer, A. M., and Thrush, S. F. (2011). Context-specific bioturbation mediates changes to ecosystem functioning. Ecosystems. 14, 1096–1109. doi: 10.1007/s10021-011-9468-0
Norgaard, R. B. (2009). Ecosystem services: from eye-opening metaphor to complexity blinder. Ecol. Econ. 69, 1219–1227. doi: 10.1016/j.ecolecon.2009.11.009
Orams, M. (2002). Marine ecotourism as a potential agent for sustainable development in Kaikoura, New Zealand. Int. J. Sust. Dev. 5, 338–352. doi: 10.1504/IJSD.2002.003757
Oreskes, N., Shraderfrechette, K., and Belitz, K. (1994). Verification, validation, and confirmation of numerical- models in the earth-sciences. Science 263, 641–646.
Ostrom, E. (1990). Governing the Commons: The Evolution of Institutions for Collective Action. Cambridge: Cambridge University Press.
Pendleton, L. H., Thebaud, O., Mongruel, R. C., and Levrel, H. (2016). Has the value of global marine and coastal ecosystem services changed? Mar. Pol. 64, 156–158. doi: 10.1016/j.marpol.2015.11.018
Pert, P. L., Hill, R., Maclean, K., Dale, A., Rist, P., Schmider, J., et al. (2015). Mapping cultural ecosystem services with rainforest aboriginal peoples: integrating biocultural diversity, governance and social variation. Ecosys. Serv. 16, 41–56. doi: 10.1016/j.ecoser.2014.10.012
Peterson, C. H., and Lubchenco, J. (1997). “Marine ecosystem services,” in Nature's Services: Societal Dependence on Natural Ecosystems, ed G. Daily (Washington, DC: Island Press), 177–194.
Phan, L. K., Vries, V. T. D., and Stive, M. J. F. (2015). Coastal mangrove squeeze in the mekong Delta. J Coast Res. 31, 233–243. doi: 10.2112/JCOASTRES-D-14-00049.1
Potts, T., Burdon, D., Jackson, J., Atkins, J., Hastings, E., and Langmead, O. (2014). Do marine protected areas deliver flows of ecosystem services to support human welfare? Mar. Policy 44, 139–148. doi: 10.1016/j.marpol.2013.08.011
Poursanidis, D., Topouzelis, K., and Chrysoulakis, N. (2018). Mapping coastal marine habitats and delineating the deep limits of the Neptune's seagrass meadows using very high resolution Earth observation data. Int. J. Remote Sens. doi: 10.1080/01431161.2018.1490974
Raffaelli, D. G., and White, P. C. L. (2013). Ecosystems and their services in a changing world: an ecological perspective. Adv. Ecol. Res. 48, 1–70. doi: 10.1016/B978-0-12-417199-2.00001-X
Raymond, C. M., Bryan, B. A., Hatton Macdonald, D., Cast, A., Strathearn, S., Grandgirard, A., et al. (2009). Mapping community values for natural capital and ecosystem services. Ecol. Econ. 68, 1301–1315. doi: 10.1016/j.ecolecon.2008.12.006
Reyers, B., Biggs, R., Cumming, G. S., Elmqvist, T., Hejnowicz, A. P., and Polasky, S. (2013). Getting the measure of ecosystem services: a social-ecological approach. Front. Ecol. Environ. 11, 268–273. doi: 10.1890/120144
Riitters, K. H., Wickham, J. D., Vogelmann, J. E., and Jones, K. B. (2000). National land-cover pattern data. Ecology 81:604. doi: 10.1890/0012-9658(2000)081[0604:NLCPD]2.0.CO;2
Sandilyan, S., and Kathiresan, K. (2012). Mangrove conservation: a global perspective. Biodivers. Conserv. 21, 3523–3542. doi: 10.1007/s10531-012-0388-x
Sathirathai, S., and Barbier, E. B. (2001). Valuing mangrove conservation, Southern Thailand. Contemp. Econ. Pol. 19, 109–122. doi: 10.1093/cep/19.2.109
Schwartz, S. H. (1994). Are there universal aspects in the structure and contents of human values? J. Soc. Iss. 50, 19–45.
Seddon, A. W. A., Macias-Fauria, M., Long, P. R., Benz, D., and Willis, K. J. (2016). Sensitivity of global terrestrial ecosystems to climate variability. Nature 531, 229–232. doi: 10.1038/nature16986
Seppelt, R., Dormann, C. F., Eppink, F. V., Lautenbach, S., and Schmidt, S. (2011). A quantitative review of ecosystem service studies: approaches, shortcomings and the road ahead. J. Appl. Ecol. 48, 630–636. doi: 10.1111/j.1365-2664.2010.01952.x
Simmons, D. G., and Fairweather, J. R. (1998). Towards a Tourism Plan for Kaikoura. Kaikoura Case Study., Tourism Research and Education Centre (TREC). Lincoln University Report No. 10.
Stachowicz, J. J., Bruno, J. F., and Duffy, J. E. (2007). Consequences of biodiversity for marine communities and ecosystems. Ann. Rev. Ecol. Evol. Syst. 38, 739–766. doi: 10.1146/annurev.ecolsys.38.091206.095659
Tallis, H., and Polasky, S. (2011). “Assessing multiple ecosystem services: an integrated tool for the real world,” in Natural Capital: Theory and Practice of Mapping Ecosystem Services, eds P. Kareiva, H. Tallis, T. H. Ricketts, G. C. Daily, and S. Polasky (Oxford: Oxford University Press), 34–50.
TEEB (2012). Why Value the Oceans-A Discussion Paper. Available online at: http://www.teebweb.org/media/2013/10/2013-Why-Value-the-Oceans-Discussion-Paper.pdf
Thompson, E. P. (1991). Customs in Common: Studies in Traditional Popular Culture. London: Merlin Press.
Thrush, S. F., and Dayton, P. K. (2002). Disturbance to marine benthic habitats by trawling and dredging: implications for marine biodiversity. Annu. Rev. Ecol. Syst. 33, 449–473. doi: 10.1146/annurev.ecolsys.33.010802.150515
Thrush, S. F., Ellingsen, K. E., and Davies, K. (2015). Implications of fisheries impacts to seabed biodiversity and ecosystem-based management. ICES J. Mar. Sci. 73, i44–i50. doi: 10.1093/icesjms/fsv114
Thrush, S. F., Hewitt, J. E., Cummings, V. J., Dayton, P. K., Cryer, M., Turner, S. J., et al. (1998). Disturbance of the marine benthic habitat by commercial fishing: impacts at the scale of the fishery. Ecol. App. 8, 866–879.
Thrush, S. F., Hewitt, J. E., Cummings, V. J., Ellis, J. I., Hatton, C., Lohrer, A. M., et al. (2004). Muddy waters: elevating sediment input to coastal and estuarine habitats. Front. Ecol. Environ. 2, 299–306. doi: 10.1890/1540-9295(2004)002[0299:MWESIT]2.0.CO;2
Thrush, S. F., Hewitt, J. E., Parkes, S., Lohrer, A. M., Pilditch, C., Woodin, S. A., et al. (2014). Experimenting with ecosystem interaction networks in search of threshold potentials in real-world marine ecosystems. Ecology 95, 1451–1457. doi: 10.1890/13-1879.1
Thrush, S. F., Schultz, D., Hewitt, J. E., and Talley, D. (2002). Habitat structure in soft-sediment environments and abundance of juvenile snapper Pagrus auratus. Mar. Ecol. Prog. Ser. 245, 273–280. doi: 10.3354/meps245273
Thurber, A. R., Sweetman, A. K., Narayanaswamy, B. E., Jone, D. O. B., Ingels, J., and Hansman, R. L. (2014). Ecosystem function and services provided by the deep sea. Biogeosciences 11, 3941–3963. doi: 10.5194/bg-11-3941-2014
Tipa, G., Harmsworth, G. R., Williams, E., and Kitson, J. C. (2016). “Integrating mātauranga Māori into freshwater management, planning and decision making,” in Advances in New Zealand Freshwater Science, eds P. G. Jellyman, T. J. A. Davie, C. P. Pearson, and J. S. Harding (Wellington: New Zealand Freshwater Sciences Society and New Zealand Hydrological Society), 613–638.
Torres, C., and Hanley, N. (2016). Economic Valuation of Coastal and Marine Ecosystem Services in the 21st century: An Overview From a Management Perspective. Working Papers in Environmental Economics. Report No.2016-1. University of St Andrews. Available online at: http://www.st-andrews.ac.uk/gsd/research/envecon/eediscus/
Townsend, M., Thrush, S. F., Lohrer, A. M., Hewitt, J. E., Lundquist, C. J., Carbines, M., et al. (2014). Overcoming the challenges of data scarcity in mapping marine ecosystem service potential. Ecosyst. Serv. 8, 44–55. doi: 10.1016/j.ecoser.2014.02.002
van den Belt, M., and Cole, A. (2014). Ecosystem Goods and Services in Marine Protected Areas (MPA)s. Science for Conservation. Wellington: Department of Conservation (DOC).
Keywords: connectivity, data scarcity, ecosystem services, mapping, marine, measuring, valuation
Citation: Townsend M, Davies K, Hanley N, Hewitt JE, Lundquist CJ and Lohrer AM (2018) The Challenge of Implementing the Marine Ecosystem Service Concept. Front. Mar. Sci. 5:359. doi: 10.3389/fmars.2018.00359
Received: 14 June 2018; Accepted: 18 September 2018;
Published: 10 October 2018.
Edited by:
Lyne Morissette, M–Expertise Marine, CanadaReviewed by:
Dimitris Poursanidis, Foundation for Research and Technology Hellas, GreeceCopyright © 2018 Townsend, Davies, Hanley, Hewitt, Lundquist and Lohrer. This is an open-access article distributed under the terms of the Creative Commons Attribution License (CC BY). The use, distribution or reproduction in other forums is permitted, provided the original author(s) and the copyright owner(s) are credited and that the original publication in this journal is cited, in accordance with accepted academic practice. No use, distribution or reproduction is permitted which does not comply with these terms.
*Correspondence: Michael Townsend, TWljaGFlbC50b3duc2VuZEB3YWlrYXRvcmVnaW9uLmdvdnQubno=
† Present Address: Michael Townsend, Waikato Regional Council, Hamilton, New Zealand
Disclaimer: All claims expressed in this article are solely those of the authors and do not necessarily represent those of their affiliated organizations, or those of the publisher, the editors and the reviewers. Any product that may be evaluated in this article or claim that may be made by its manufacturer is not guaranteed or endorsed by the publisher.
Research integrity at Frontiers
Learn more about the work of our research integrity team to safeguard the quality of each article we publish.