- 1Marine Department, Ecophysiology, Centre Scientifique de Monaco, Monaco City, Monaco
- 2Collège Doctoral, Sorbonne Université, Paris, France
Soft corals (Octocorallia) often constitute the second most abundant macrobenthic group on many tropical and temperate reefs. However, the gelatinous and leather-like nature of their tissue and their variable hydroskeleton entails a number of problems for tissue homogenization and data normalization. An easy and fast protocol for tissue homogenization, as well as a normalization metric that can be used to perform inter-studies or inter-species comparisons, are thus needed. In this study, we tested whether the tissue sample state before processing (frozen vs. freeze-dried samples) and the media used for tissue homogenization (0.2 μm filtered seawater; FSW vs. Milli-Q water; DI) affect the quantitative measurements of tissue descriptors (chlorophyll, protein, and Symbiodinium concentrations) in the model species Heteroxenia fuscescens. Furthermore, the suitability of dry weight (DW) and ash-free dry weight (AFDW) as size-normalizing metric was investigated across different soft coral species. Our results reveal that freeze-drying the samples and homogenizing them in DI water exhibited several benefits, namely enhancing chlorophyll and protein concentrations up to 50%, saving processing time and providing a more accurate determination of DW and AFDW. Overall, this optimized tissue processing protocol offers a more reliable quantification of tissue descriptors and reduces the chance of underestimating these parameters in soft corals. Finally, since the contribution of sclerites to the total DW of the colony can highly differ between species, we demonstrate that AFDW is a reliable metric for normalizing soft coral data, particularly when inter-species comparisons are made.
Introduction
Soft corals (Octocorallia: Alcyonacea) represent, after reef-building scleractinian corals, the second most abundant macrobenthic group on many tropical and temperate reefs (Fabricius and Alderslade, 2001). They notably become often dominant within disturbed reef systems (Inoue et al., 2013). High abundances of octocorals (soft corals or gorgonians) have thus been observed in Caribbean and Indo-Pacific eutrophicated reefs, where a reduced water quality does not favor the growth of scleractinian corals (Fabricius et al., 2005; Baum et al., 2016). Despite the fact that soft corals are increasingly recognized as key taxa on reefs, two major issues regarding physiological and ecological studies remain, however, to be clarified.
First, the coenenchyme (colonial tissue) of soft corals consists of thick gelatinous material containing fibers, amoeboid cells, scleroblast cells, and calcareous sclerites (Fabricius and Alderslade, 2001). Altogether, these leather-like tissue elements make cell layers difficult to break apart and tissue homogenization challenging. Therefore, measurements of tissue descriptors (Symbiodinium, chlorophyll, and protein concentrations) are difficult to obtain from frozen or fresh soft coral samples, and a reliable and easy tissue homogenization protocol remains to be implemented.
Second, a common normalization metric to perform inter-species comparisons of physiological and/or ecological processes is required. For example, apprehending functional processes that occur at the reef-ecosystem level (e.g., primary production) implies to understand how soft corals operate over space and time compared to scleractinian corals. Comparative measurements related to these sub-classes are therefore necessary, but are only enabled if they are normalized to the same metric. Normalization of tissue parameters or physiological measurements requires a metric that reflects the size of the organisms and is stable across variable environmental conditions. In scleractinian corals, skeletal surface area is the most commonly used metric for normalizing data to the size of the colony (Edmunds and Gates, 2002). However, soft corals lack a calcium carbonate skeleton and rely on hydrostatic pressure for body shape maintenance (Fabricius and Alderslade, 2001). Although this hydroskeleton can vary over time depending on environmental fluctuations (Fabricius, 1995; Hellström and Benzie, 2011), few studies have approximated soft coral surface area based on geometric body measurements (e.g., Bednarz et al., 2012, 2015; Kremien et al., 2013) or linear projections (Fabricius and Dommisse, 2000). Such normalization metric is critical when studying processes (e.g., photosynthesis) that strongly depend on a vectorial parameter (e.g., light) as it allows understanding the effectiveness of these processes (Kremien et al., 2013). For other measurements, such as the quantification of structural tissue descriptors or the production of metabolites, the variable size of the hydroskeleton excludes the possibility to accurately normalize to surface area at different time intervals (Haydon et al., 2018). Several other normalization metrics have thus been applied to soft corals and are summarized in Figure 1, Table 1, and Supplementary Table S1. Among these metrics, those involving wet weight and buoyant weight may also be sources of error as soft coral tissue withholds a lot of water and the density of a colony may be similar to that of seawater (Davies, 1989). For example, normalization of dimethylsulfoniopropionate (DMSP) production to soft coral fresh weight (Van Alstyne et al., 2006) led to significant lower values compared to the normalization to protein content, underestimating the functional role of soft compared to hard corals (Haydon et al., 2018). Coral biomass may therefore offer a greater potential to accurately reflect the size of a soft coral colony. Protein content has been widely used to normalize physiological data in corals. However, it can encounter great variability across environmental conditions, and may thus interfere with the intrinsic pattern of the parameter of interest (Edmunds and Gates, 2002). Ash-free dry weight (AFDW) is another common proxy for tissue biomass of many benthic reef organisms such as scleractinian corals, coralline algae, sponges (Kötter and Pernthaler, 2002; Schoepf et al., 2013; Comeau et al., 2014) and soft corals (Figure 1). AFDW quantification relies on a completely destructive method, whereas dry weight (DW) allows for potential analysis of tissue parameters before burning the sample. Although tissue energy reserves (i.e., lipid, protein, and carbohydrate) of soft corals have been determined from dried material (Ben-David-Zaslow and Benayahu, 1999), the possibility to obtain reliable measurements involving Symbiodinium (cell density and chlorophyll content) still needs to be tested.
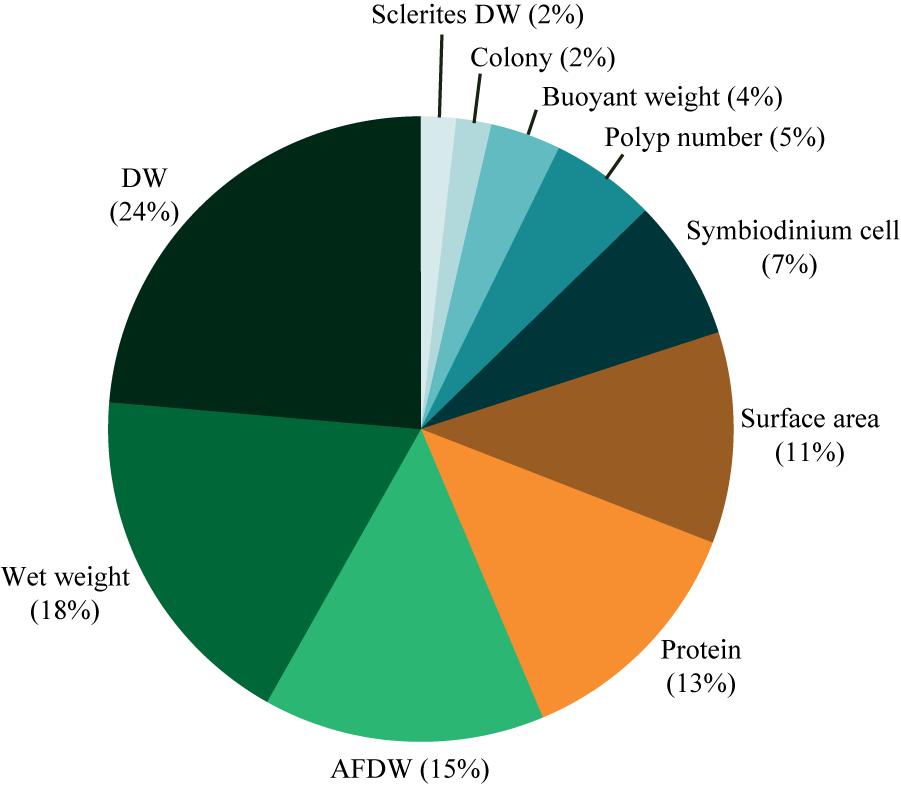
FIGURE 1. Normalization metrics used in physiological studies on soft corals. Percentages are based on the occurrence of the studies using the metric of interest. DW, dry weight; AFDW, ash-free dry weight.
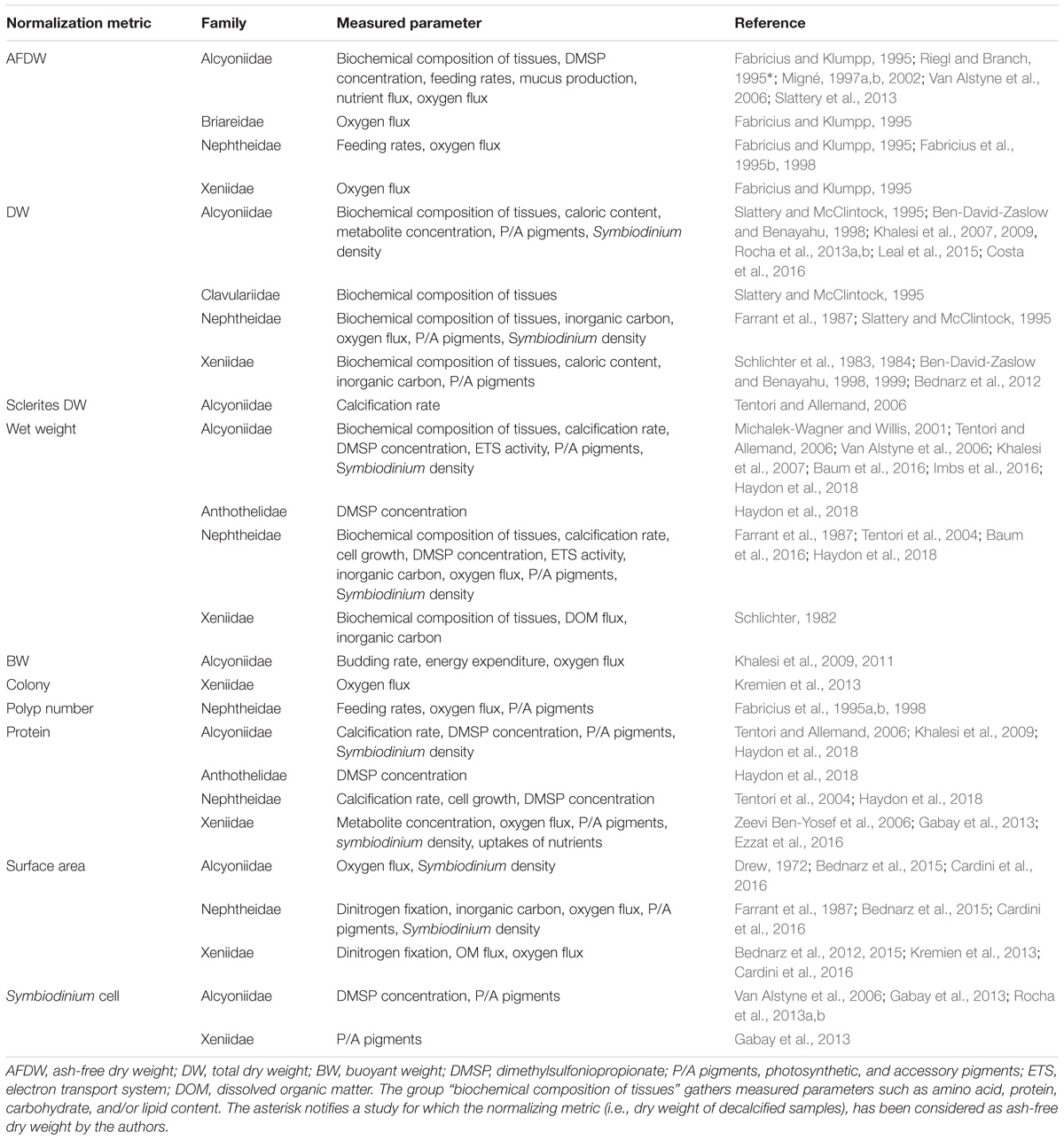
TABLE 1. Summary about the range of metrics used in previous studies to normalize physiological processes in soft corals.
Based on these issues the present study aimed (1) to optimize a protocol for soft coral tissue processing and (2) to find the normalization metric most relevant for physiological experiments involving interspecies comparisons. We tested whether the tissue sample state before processing (frozen vs. freeze-dried samples) and the media used for tissue homogenization (0.2 μm filtered seawater; FSW vs. Milli-Q water; DI) affect the quantitative measurements of tissue descriptors in the model species Heteroxenia fuscescens (Ehrenberg, 1834). Furthermore, the suitability of DW and AFDW as size-normalizing metric was investigated across different soft coral species (H. fuscescens, Dendronephthya sp. Kuekenthal, 1905), Litophyton arboreum (Forskål, 1775), and Rhytisma fulvum (Forskål, 1775), which are key components of Red Sea reefs. These species particularly exhibit different growth forms ranging from arborescent (Dendronephthya sp. and L. arboreum) to capitate (H. fuscescens) and encrusting (R. fulvum).
Materials and Methods
Biological Material
Nineteen colonies of H. fuscescens with 20 polyps and four colonies of Dendronephthya sp., L. arboreum, and R. fulvum, collected in the Red Sea were used for the experiments. They were equally allocated to five 20 L tanks and maintained under the same conditions for 8 weeks prior to starting the experiments. Tanks were continuously supplied with seawater, at a renewal rate of 10 Lh−1. HQI lamps (Tiger E40, Faeber, Italy) above the tanks provided photosynthetically active radiation (PAR) of 200 μmol photons m−2s−1.
Optimized Protocol for Symbiodinium, Chlorophyll, and Protein Determination
A first experiment, performed with H. fuscescens, aimed to compare Symbiodinium, chlorophyll, and protein concentrations in frozen or freeze-dried samples, homogenized in FSW or DI. Four combinations were thus considered, with five replicates per condition: (i) freeze-dried samples homogenized in FSW, (ii) freeze-dried samples homogenized in DI, (iii) frozen samples homogenized in FSW, and (iv) frozen samples homogenized in DI. For this purpose, 20 tubes were prepared, each containing one freshly cut polyp of 10 colonies of H. fuscescens randomly chosen in the tanks. All tubes were flash-frozen in liquid nitrogen. Half of them were processed directly whereas the other half was freeze-dried (Alpha 2-4 LDplus freeze-dryer, Martin Christ, Germany), until obtaining a dried powder. Frozen and freeze-dried samples were ground with a potter tissue grinder and solubilized in 10 mL FSW or DI. This procedure was rapid for the freeze-dried samples (5 min) but took more than 30 min for the frozen samples. From each homogenate, 500 μL was collected for total protein measurement (see following section). The remaining homogenate was centrifuged for 10 min (at 11000 g at 4°C) to pellet the Symbiodinium. Light microscopy confirmed the total removal of Symbiodinium from the supernatant (i.e., host fraction), which was freeze-dried again. This was done to determine the host DW and to subsequently compare the tissue parameters of frozen and freeze-dried samples normalized to the same metric. Symbiodinium pellets were rinsed twice to eliminate any remaining host cells (Tremblay et al., 2012), and re-suspended in 10 mL FSW or DI. After mixing, 100 μL and 0.5 mL were subsampled for the determination of Symbiodinium density and total chlorophyll concentration, respectively (see following section).
Tissue Parameter Measurements
Proteins were extracted from the 500 μL subsamples in a sodium hydroxide solution (0.5 M) for 5 h at 60°C. Thereafter, protein content was measured using a BC assay kit (Interchim, France) (Smith et al., 1985), and protein standards were prepared using bovine serum albumin (Interchim, France). Absorbance of subsamples was measured at 562 nm by an UVmc2 spectrophotometer (SAFAS, Monaco). Symbiodinium density was quantified microscopically via eight replicate haemocytometer counts in the 100 μL subsamples (Neubauer haemocytometer, Marienfeld, Germany). For chlorophyll analysis, the 5 mL subsamples were centrifuged for 10 min (at 8000 g at 4°C). The supernatant was discarded and 5 mL of acetone (100%) amended with magnesium chloride (Sigma-Aldrich, Germany) were added to the Symbiodinium pellet to extract the chlorophyll over 24 h in the dark (at 4°C). After centrifugation at 11000 g for 15 min at 4°C, absorbances were measured at 750, 663, and 630 nm using a Xenius spectrophotometer (SAFAS, Monaco) and chlorophyll concentrations calculated according to Jeffrey and Humphrey (1975).
Normalization Parameters
An experiment was performed with H. fuscescens to highlight any correlation between biomass (DW), protein content, and colony’s body volume. The body volume of five colonies with different polyp numbers was estimated using the water displacement technique (Benayahu and Loya, 1986), before colonies were freeze-dried and homogenized in 10 mL DI. A subsample of 500 μL was used for protein content determination. We also compared the DW and ash weight (AW) of the four different coral species listed above. For this purpose, colonies of each species were freeze-dried, crushed, and split in five powder heaps of different weights (DW). They were combusted at 450°C for 4 h in a muffle furnace (Thermolyne 62700, Thermo Fisher Scientific, United States). Such combustion process avoids losses of sclerites (Harvell and Fenical, 1989) and allows estimating the DW of sclerites.
Statistical Analyses
Analyses were performed using R software (R Foundation for Statistical Computing) and assumptions of normality and homoscedasticity of variance were evaluated through Shapiro’s and Bartlett’s tests along with graphical analyses of residuals. Protein, Symbiodinium, and chlorophyll concentrations were analyzed using a two-way ANOVA, in which the sample state (frozen vs. freeze-dried) and type of water (FSW vs. DI) were fixed effects. Regression equations were established between colony DW and protein content, volume or AW. Pearson’s product moment correlation coefficients were calculated to test the strength of the linear associations.
Results and Discussion
This study shows that both the sample state (i.e., frozen, freeze-dried) and the homogenization media (i.e., DI and FSW) significantly influence the quantification of tissue parameters in soft corals, while no effect was observed for Symbiodinium counts (Figure 2 and Table 2). Sample state mainly affects chlorophyll determinations with twofold higher concentrations in freeze-dried as compared to frozen samples (Figure 2B). A previous work on reef sands also demonstrated a 27 to 39% increased chlorophyll concentration in freeze-dried over frozen samples, likely due to the removal of mucous matrices surrounding the cells and a better exposure of pigments to the solvent in the former (Hannides et al., 2014). Here, the reduced chlorophyll concentration in frozen samples likely results from pigment damage due to the longer homogenization period of frozen fragments (30 min) as compared to the freeze-dried fragments (5 min). Although samples were kept on ice during homogenization, this caution did not prevent tissue slurry from mechanic heating with the Potter grinder. Similarly, Hannides et al. (2014) highlighted that longer sonication periods caused a significant decline in chlorophyll content, due to pigment degradation (Metaxatos and Ignatiades, 2002). Variations in the length of the homogenization procedure can occur for coral samples with different tissue thickness, and may amplify variance in the chlorophyll measurements. Future studies on corals should thus take into account such tissue processing flaws and compare the efficacy of the usual tissue extraction procedure with the freeze-drying one. Care must also be taken when comparing studies that use photosynthetic pigments as normalization metric for other parameters (e.g., coral productivity). As highlighted in this study, a 50% reduced photosynthetic pigment extraction in frozen as compared to freeze-dried samples can lead to a significant overestimation of the productivity in the former, an issue that can be extended to scleractinian corals too. Finally, saving time is another benefit when processing freeze-dried over frozen samples, which is important in experiments involving numerous samples. Although the homogenization media has an overall low effect on tissue processing, the use of DI compared to FSW significantly increases the protein content measurable in tissue samples (Figure 2C and Table 2). This could have important implications when comparing physiological processes across different coral species and environmental conditions. The use of DI also reduces the amount of salts in homogenates, thereby providing a higher accuracy for the determination of absolute host and Symbiodinium DW as well as avoiding salt crystals entering instruments such as mass spectrometers (Baker et al., 2015).
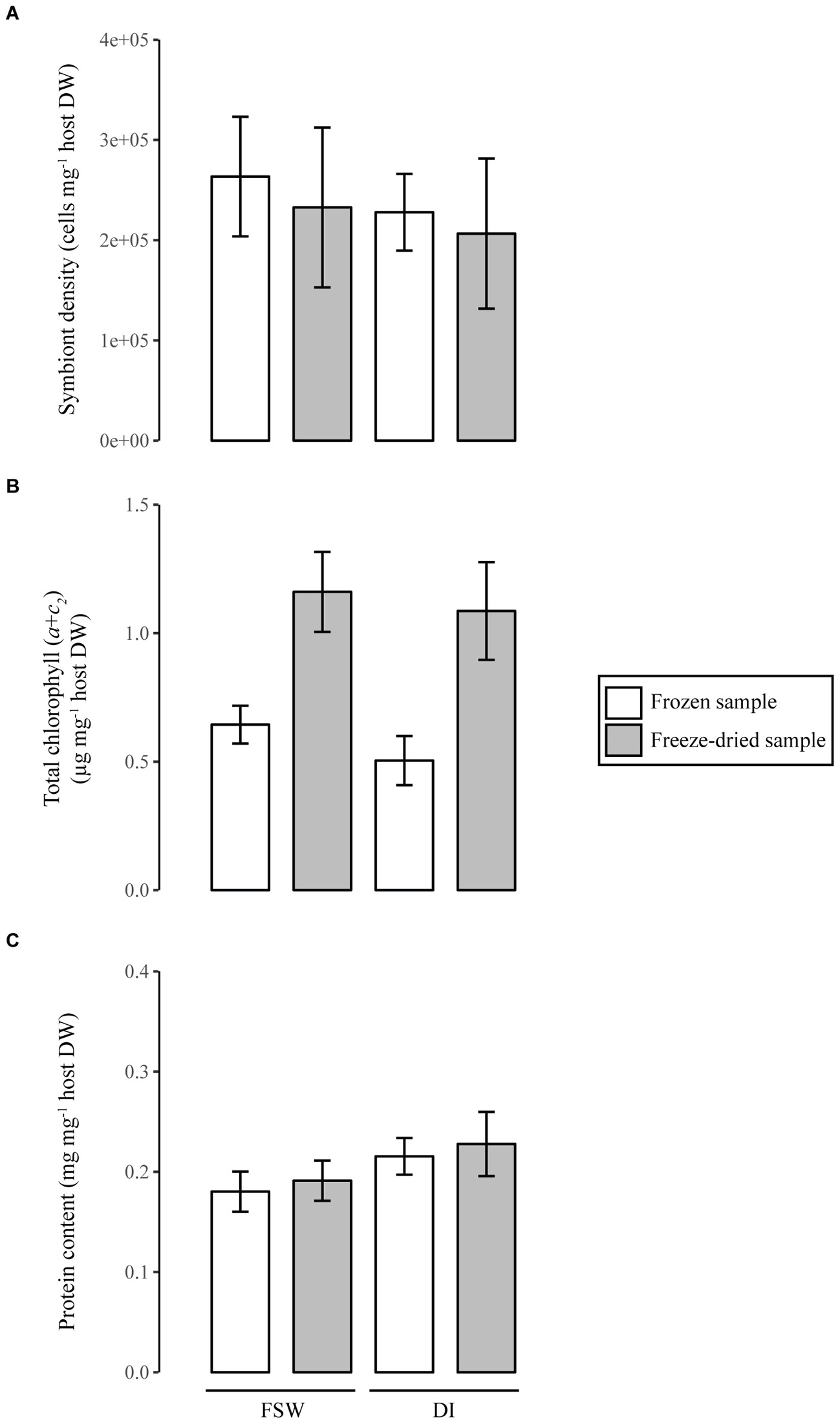
FIGURE 2. Tissue parameter quantification in soft corals using different sample processing techniques. Samples were either processed frozen or after freeze-drying and homogenized in filtered seawater (FSW) or Milli-Q water (DI). All tissue parameters (Symbiodinium density, A; chlorophyll, B; and protein content, C), are normalized to host dry weight. Error bars represent standard deviation of five samples.
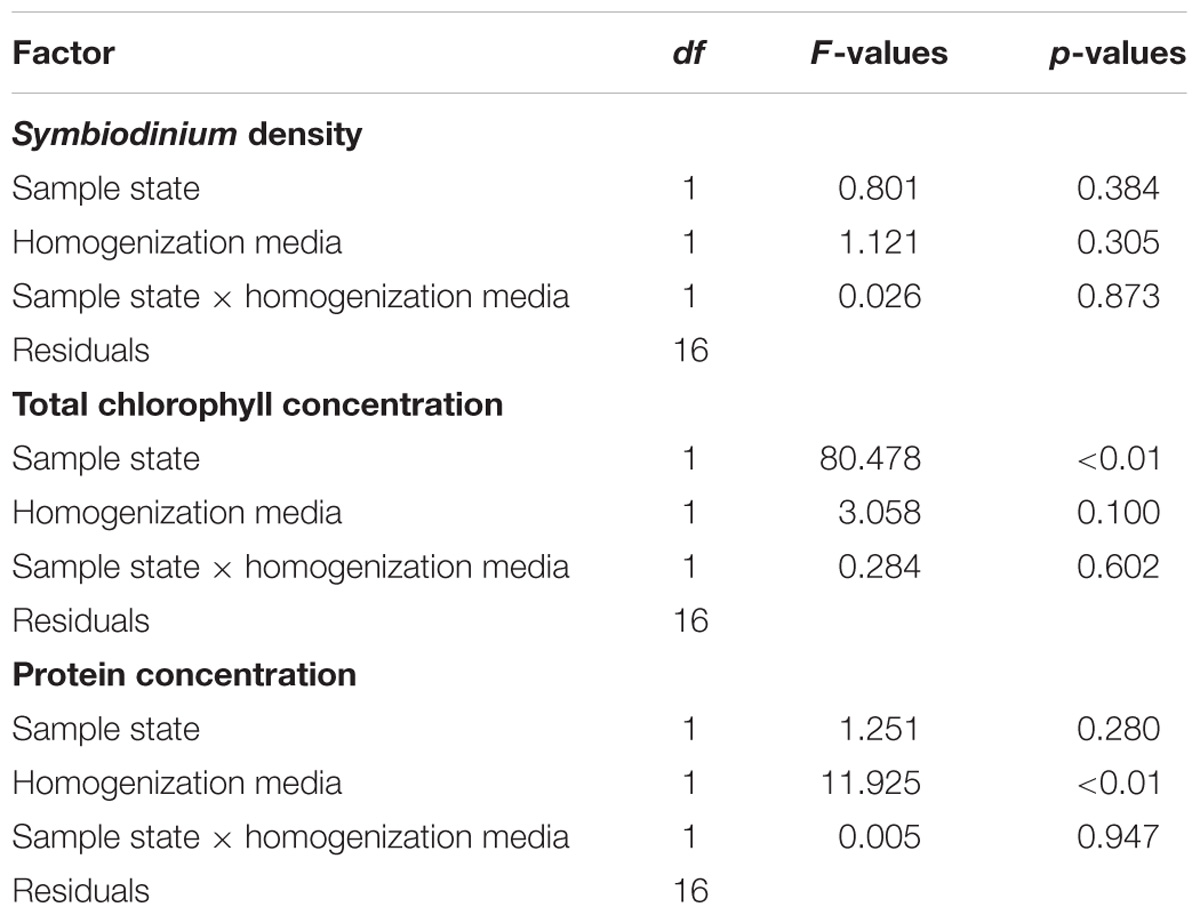
TABLE 2. Statistical results about the effect of sample state, and homogenization media on Symbiodinium density, total chlorophyll, and protein concentrations measured in Heteroxenia fuscescens.
As highlighted in the introduction, a literature review identified 36 physiological studies on soft corals, which display a wide variety of normalization metrics (Figure 1, Table 1, and Supplementary Table S1). Although the choice of the metrics certainly depends on the process under study and the information provided, a consensual normalization metric is important to allow better comparability of results among studies. For a given soft coral species maintained under a constant environmental condition, all metrics can be proxies for biomass and colony size. Results obtained with H. fuscescens indeed exhibit significant linear relationships between total DW and body volume or protein concentration (Figure 3A and Table 3). Other studies have also shown significant correlations between AFDW or DW and polyp number, colony height, or carbon and nitrogen content (Migné, 1993; Fabricius et al., 1995b; Migné et al., 1996). However, the use of several metrics listed above can be unsatisfactory when comparing processes between different species or different environmental conditions. We demonstrate that DW does not provide a reliable normalization metric to compare tissue parameters across coral species (Figure 3B and Table 3), although dried samples enable better tissue processing in soft corals. Linear regression analyses between DW and AW of different soft coral species show species-specific linear relationships; this is due to the presence of sclerites, which can differ in size, shape, and abundance within a colony and between species (Van Alstyne et al., 1992). Sclerites are frequently used as taxonomic indicator, and species-specific differences likely depend on the necessity to accumulate sclerites for structural defense against predators and/or skeletal support against wave action (Van Alstyne et al., 1992). Overall, our results show that the contribution of sclerites to the total DW of the colony can highly differ between species, and thus, we recommend the use of AFDW over DW for normalizing physiological processes in soft corals, particularly when inter-species comparisons are made.
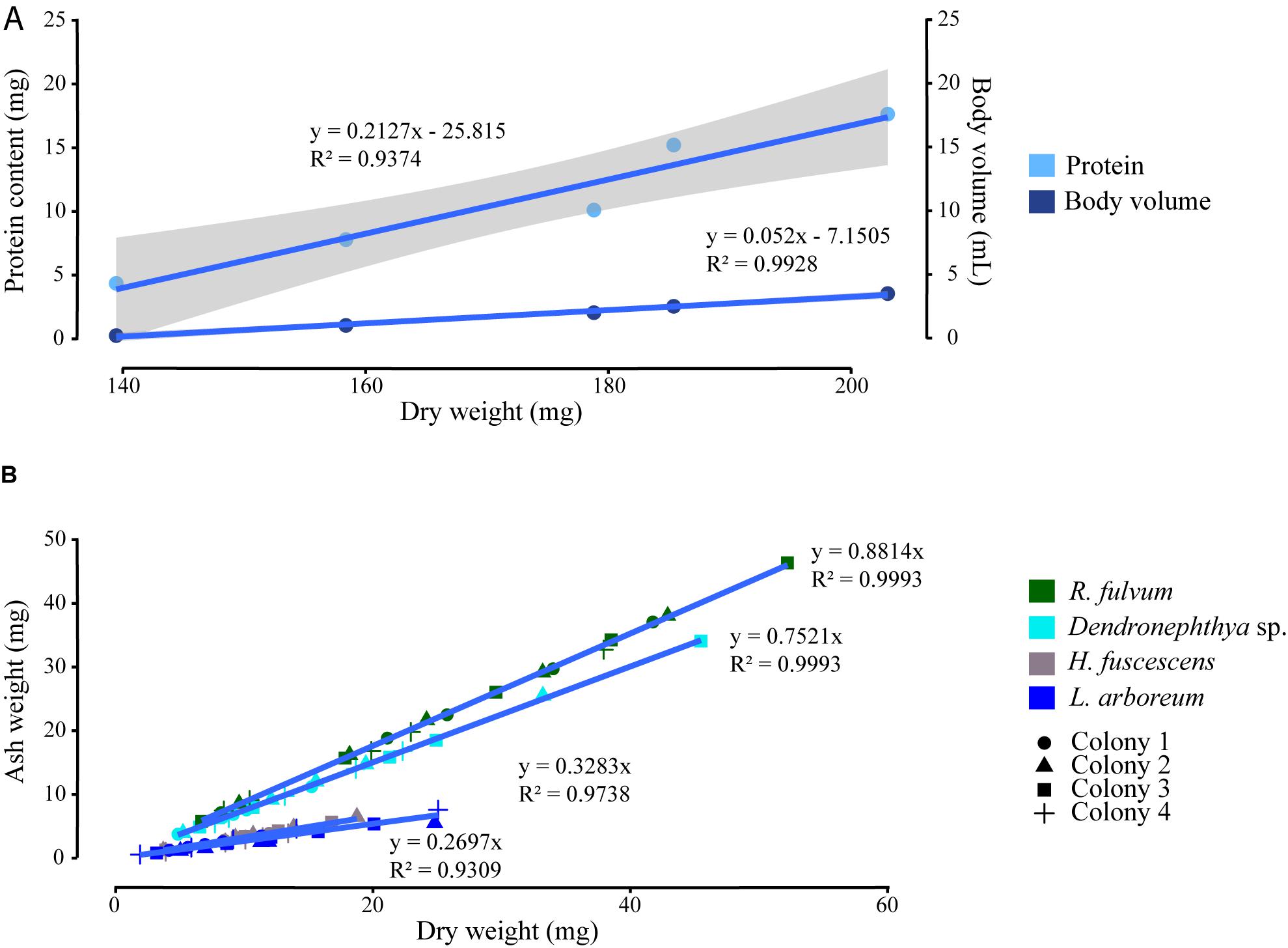
FIGURE 3. Correlations between the normalizing metrics. (A) Total dry weight with protein content and body volume of five Heteroxenia fuscescens colonies. (B) Linear regressions between total dry weight and ash weight of four colonies of Rhytisma fulvum, Dendronephthya sp., H. fuscescens, and Litophyton arboreum. Gray areas display confidence interval of 0.95 around the regression lines.
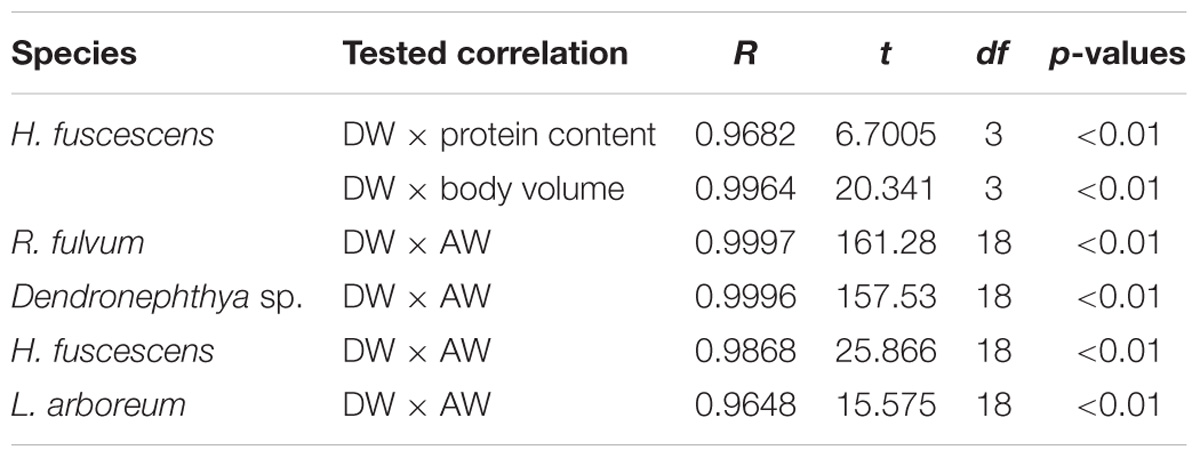
TABLE 3. Significant correlations between dry weight (DW), protein content, body volume, and ash weight (AW), of Heteroxenia fuscescens, Rhytisma fulvum, Dendronephthya sp., and Litophyton arboreum.
Conclusion
In conclusion, we recommend to dry the biological material for biochemical and tissue parameter analyses and to use a subset of the dried powder for AFDW determination as final normalization metric (Figure 4). AFDW stands for a useful metric to normalize tissue parameters in soft corals, benefiting of the aforementioned advantages owed from its freeze-dried state and allowing best comparability among species. Other non-destructive normalization metrics, such as surface area, can, however, be used in parallel to provide a different information on the physiological processes studied.
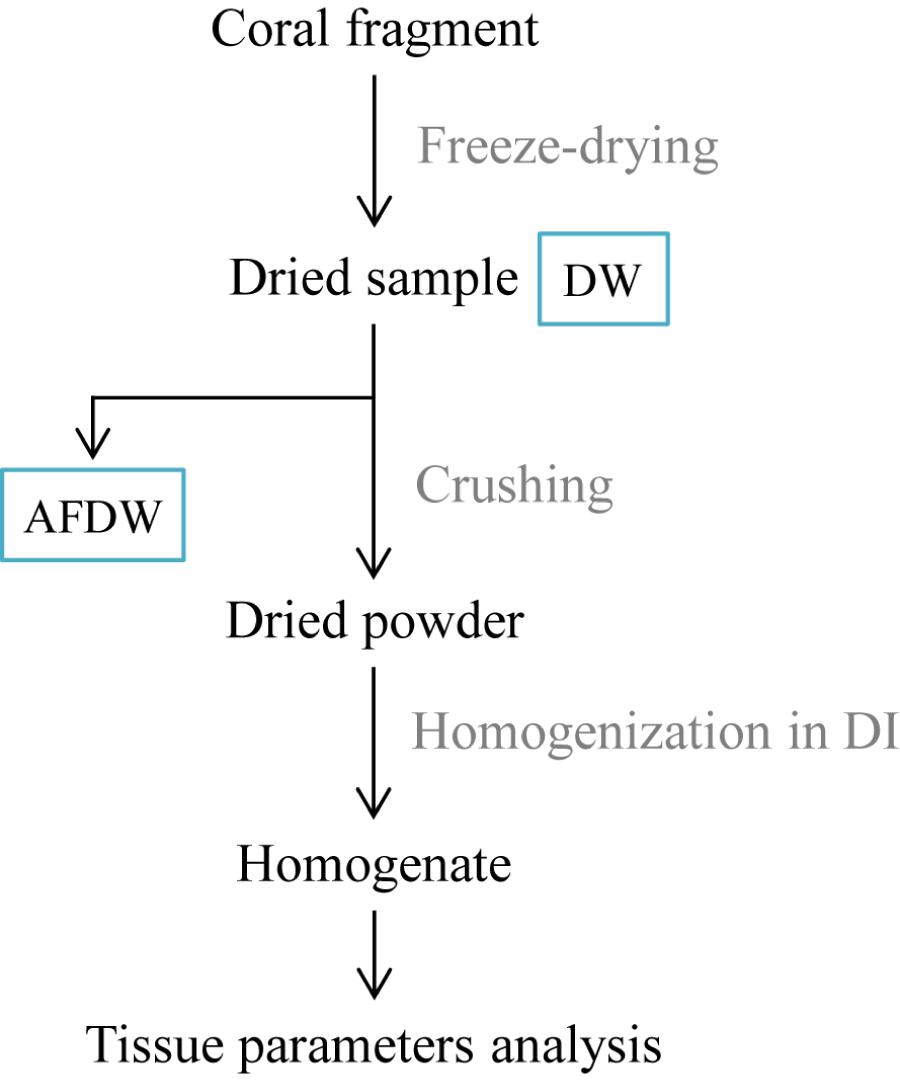
FIGURE 4. Optimized workflow for processing soft coral tissue samples. DW, dry weight; AFDW, ash-free dry weight; DI, Milli-Q water.
Ethics Statement
Animals involved in the subject are not listed in CITES and were cultured in the laboratory for experimental purpose.
Author Contributions
CP, VB, and CF-P designed the study, wrote and reviewed the manuscript. CP and VB performed the experimental works. CP analyzed the data.
Funding
This study was funded by the Centre Scientifique de Monaco.
Conflict of Interest Statement
The authors declare that the research was conducted in the absence of any commercial or financial relationships that could be construed as a potential conflict of interest.
Acknowledgments
We thank D. Allemand for scientific support, M. Boussion and C. Rottier for their contribution in sample processing, and the ecophysiology team for useful comments. Two reviewers greatly contributed to improve this manuscript.
Supplementary Material
The Supplementary Material for this article can be found online at: https://www.frontiersin.org/articles/10.3389/fmars.2018.00348/full#supplementary-material
References
Baker, D. M., Freeman, C. J., Knowlton, N., Thacker, R. W., Kim, K., and Fogel, M. L. (2015). Productivity links morphology, symbiont specificity and bleaching in the evolution of Caribbean octocoral symbioses. ISME J. 9, 2620–2629. doi: 10.1038/ismej.2015.71
Baum, G., Januar, I., Ferse, S. C. A., Wild, C., and Kunzmann, A. (2016). Abundance and physiology of dominant soft corals linked to water quality in Jakarta Bay. Indonesia. PeerJ 4:e2625. doi: 10.7717/peerj.2625
Bednarz, V. N., Cardini, U., Van Hoytema, N., Al-Rshaidat, M. M. D., and Wild, C. (2015). Seasonal variation in dinitrogen fixation and oxygen fluxes associated with two dominant zooxanthellate soft corals from the northern Red Sea. Mar. Ecol. Prog. Ser. 519, 141–152. doi: 10.3354/meps11091
Bednarz, V. N., Naumann, M. S., Niggl, W., and Wild, C. (2012). Inorganic nutrient availability affects organic matter fluxes and metabolic activity in the soft coral genus Xenia. J. Exp. Biol. 215, 3672–3679. doi: 10.1242/jeb.072884
Benayahu, Y., and Loya, Y. (1986). Sexual reproduction of a soft coral: synchronous and brief annual spawning of Sarcophyton glaucum (Quoy and Gaimard, 1833). Biol. Bull. 170, 32–42. doi: 10.2307/1541378
Ben-David-Zaslow, R., and Benayahu, Y. (1998). Competence and longevity in planulae of several species of soft corals. Mar. Ecol. Prog. Ser. 163, 235–243. doi: 10.3354/meps171235
Ben-David-Zaslow, R., and Benayahu, Y. (1999). Temporal variation in lipid, protein and carbohydrate content in the Red Sea soft coral Heteroxenia fuscescens. J. Mar. Biol. Assoc. 79, 1001–1006. doi: 10.1017/S002531549900123X
Cardini, U., Bednarz, V. N., van Hoytema, N., Rovere, A., Naumann, M. S., Al-Rshaidat, M. M. D., et al. (2016). Budget of primary production and dinitrogen fixation in a highly seasonal Red Sea coral reef. Ecosystems 19, 771–785. doi: 10.1007/s10021-016-9966-1
Comeau, S., Edmunds, P. J., Spindel, N. B., and Carpenter, R. C. (2014). Fast coral reef calcifiers are more sensitive to ocean acidification in short-term laboratory incubations. Limnol. Ocean. 59, 1081–1091. doi: 10.4319/lo.2014.59.3.1081
Costa, A. P. L., Calado, R., Marques, B., Lillebø, A. I., Serôdio, J., Soares, A. M. V. M., et al. (2016). The effect of mixotrophy in the ex situ culture of the soft coral Sarcophyton cf. glaucum. Aquaculture 452, 151–159. doi: 10.1016/j.aquaculture.2015.10.032
Davies, P. S. (1989). Short-term growth measurements of corals using an accurate buoyant weighing technique. Mar Biol. 395, 389–395. doi: 10.1007/BF00428135
Drew, E. A. (1972). The biology and physiology of alga-invertabrate symbiosis. 2. The density of symbiotic algal cells in a number of hermatypic corals and Alcyonarians from various depths. J. Exp. Mar. Biol. Ecol. 9, 71–75. doi: 10.1016/0022-0981(72)90008-1
Edmunds, P., and Gates, R. (2002). Normalizing physiological data for scleractinian corals. Coral Reefs 21, 193–197. doi: 10.1007/s00338-002-0214-0
Ezzat, L., Maguer, J. F., Grover, R., and Ferrier-Pagès, C. (2016). Limited phosphorus availability is the Achilles heel of tropical reef corals in a warming ocean. Sci. Rep. 6:31768. doi: 10.1038/srep31768
Fabricius, K., De’ath, G., McCook, L., Turak, E., and Williams, D. M. B. (2005). Changes in algal, coral and fish assemblages along water quality gradients on the inshore great Barrier Reef. Mar. Pollut. Bull. 51, 384–398. doi: 10.1016/j.marpolbul.2004.10.041
Fabricius, K., Yahel, G., and Genin, A. (1998). In situ depletion of phytoplankton by an azooxanthellate soft coral. Limnol. Oceanogr. 43, 354–356. doi: 10.4319/lo.1998.43.2.0354
Fabricius, K. E. (1995). Slow population turnover in the soft coral genera Sinularia and Sarcophyton on mid- and outer-shelf reefs of the great barrier Reef. Mar. Ecol. Prog. Ser. 126, 145–152. doi: 10.3354/meps126145
Fabricius, K. E., and Alderslade, P. P. (2001). Soft Corals and Sea Fans: a Comprehensive Guide to the Tropical Shallow Water Genera of the Central-West Pacific, the Indian Ocean and the Red Sea. Townsville, QLD: Australian Institute of Marine Science.
Fabricius, K. E., Benayahu, Y., and Genin, A. (1995a). Herbivory in asymbiotic soft corals. Science 268, 90–92. doi: 10.1126/science.268.5207.90
Fabricius, K. E., Genin, A., and Benayahu, Y. (1995b). Flow-dependent herbivory and growth in zooxanthellae-free soft corals. Limnol. Oceanogr. 40, 1290–1301. doi: 10.4319/lo.1995.40.7.1290
Fabricius, K. E., and Dommisse, M. (2000). Depletion of suspended particulate matter over coastal reef communities dominated by zooxanthellate soft corals. Mar. Ecol. Prog. Ser. 196, 157–167. doi: 10.3354/meps196157
Fabricius, K. E., and Klumpp, D. W. (1995). Widespread mixotrophy in reef-inhabiting soft corals: the influence of depth, and colony expansion and contraction on photosynthesis. Mar. Ecol. Prog. Ser. 125, 195–204. doi: 10.3354/meps125195
Farrant, P. A., Borowitzka, M. A., Hinde, R., and King, R. J. (1987). Nutrition of the temperate Australian soft coral Capnella gaboensis I. Photosynthesis and carbon fixation. Mar Biol 95, 565–574. doi: 10.1007/BF00393100
Gabay, Y., Benayahu, Y., and Fine, M. (2013). Does elevated pCO2 affect reef octocorals? Ecol. Evol. 3, 465–473. doi: 10.1002/ece3.351
Hannides, A. K., Glazer, B. T., and Sansone, F. J. (2014). Extraction and quantification of microphytobenthic Chl a within calcareous reef sands. Limnol. Oceanogr. Methods 12, 126–138. doi: 10.4319/lom.2014.12.126
Harvell, C. D., and Fenical, W. (1989). Chemical and structural defenses of Caribbean gorgonians (Pseudopterogorgia spp.): intercolony localization of defense. Limnol. Oceanogr. 34, 382–389. doi: 10.4319/lo.1989.34.2.0382
Haydon, T. D., Seymour, J. R., and Suggett, D. J. (2018). Soft corals are significant DMSP producers in tropical and temperate reefs. Mar. Biol. 165, 109. doi: 10.1007/s00227-018-3367-2
Hellström, M., and Benzie, J. A. H. (2011). Robustness of size measurement in soft corals. Coral Reefs 30, 787–790. doi: 10.1007/s00338-011-0760-4
Imbs, A. B., Demidkova, D. A., and Dautova, T. N. (2016). Lipids and fatty acids of cold-water soft corals and hydrocorals: a comparison with tropical species and implications for coral nutrition. Mar. Biol. 163, 1–12. doi: 10.1007/s00227-016-2974-z
Inoue, S., Kayanne, H., Yamamoto, S., and Kurihara, H. (2013). Spatial community shift from hard to soft corals in acidified water. Nat. Clim. Chang. 3, 683–687. doi: 10.1038/nclimate1855
Jeffrey, S. W., and Humphrey, G. F. (1975). New spectrophotometric equations for determining chlorophylls a, b, c1 and c2 in higher plants, algae and natural phytoplankton. Biochem. Physiol. Pflanz 167, 191–194. doi: 10.1016/S0015-3796(17)30778-3
Khalesi, M. K., Beeftink, H. H., and Wijffels, R. H. (2007). Flow-dependent growth in the zooxanthellate soft coral Sinularia flexibilis. J. Exp. Mar. Bio. Ecol. 351, 106–113. doi: 10.1016/j.jembe.2007.06.007
Khalesi, M. K., Beeftink, H. H., and Wijffels, R. H. (2009). Light-dependency of growth and secondary metabolite production in the captive zooxanthellate soft coral Sinularia flexibilis. Mar. Biotechnol. 11, 488–494. doi: 10.1007/s10126-008-9164-z
Khalesi, M. K., Beeftink, H. H., and Wijffels, R. H. (2011). Energy budget for the cultured, zooxanthellate Octocoral Sinularia flexibilis. Mar. Biotechnol. 13, 1092–1098. doi: 10.1007/s10126-011-9373-8
Kötter, I., and Pernthaler, J. (2002). “In situ feeding rates of obligate and facultative coelobite (cavity-dwelling) sponges in a Caribbean coral reef,” in Proceedings of the Ninth International Coral Reef Symposium, Bali,
Kremien, M., Shavit, U., Mass, T., and Genin, A. (2013). Benefit of pulsation in soft corals. Proc. Natl. Acad. Sci. U.S.A. 110, 8978–8983. doi: 10.1073/pnas.1301826110
Leal, M. C., Jesus, B., Ezequiel, J., Calado, R., Rocha, R. J. M., Cartaxana, P., et al. (2015). Concurrent imaging of chlorophyll fluorescence, Chlorophyll a content and green fluorescent proteins-like proteins of symbiotic cnidarians. Mar. Ecol. 36, 572–584. doi: 10.1111/maec.12164
Metaxatos, A., and Ignatiades, L. (2002). Seasonality of algal pigments in the sea water and interstitial water/sediment system of an eastern Mediterranean coastal area. Estuar. Coast. Shelf Sci. 55, 415–426. doi: 10.1006/ecss.2001.0915
Michalek-Wagner, K., and Willis, B. L. (2001). Impacts of bleaching on the soft coral Lobophytum compactum. II. Biochemical changes in adults and their eggs. Coral Reefs 19, 240–246. doi: 10.1007/PL00006959
Migné, A., and Davoult, D. (1993). Relations « taille-poids » chez quelques Cnidaires coloniaux. Cah. Biol. Mar. 34, 103–110.
Migné, A., and Davoult, D. (1997a). Ammonium excretion in two benthic cnidarians: Alcyonium digitatum (Linnaeus, 1758) and Urticina felina (Linnaeus, 1767). J. Sea Res. 37, 101–107. doi: 10.1016/S1385-1101(97)00002-6
Migné, A., and Davoult, D. (1997b). “Oxygen Consumption in two Benthic Cnidarians: Alcyonium digitatum (Linnaeus, 1758) and Urticina felina (Linnaeus, 1767),” in Proceedings of the 6th International Conference Coelenterate Biology, Vol. 1995, (Dordrecht: Leiden), 321–328.
Migné, A., and Davoult, D. (2002). Experimental nutrition in the soft coral Alcyonium digitatum (Cnidaria: Octocorallia): removal rate of phytoplankton and zooplankton. Cah. Biol. Mar. 43, 9–16.
Migné, A., Davoult, D., Janquin, M. A., and Kupka, A. (1996). Biometrics, carbon and nitrogen content in two Cnidarians: urticina felina and alcyonium digitatum. J. Mar. Biol. Assoc. U. K. 76:595. doi: 10.1017/S0025315400031301
Riegl, B., and Branch, G. M. (1995). Effects of sediment on the energy budgets of four scleractinian (Bourne 1900) and five alcyonacean (Lamouroux 1816) corals. J. Exp. Mar. Biol. Ecol. 186, 259–275. doi: 10.1016/0022-0981(94)00164-9
Rocha, R. J. M., Calado, R., Cartaxana, P., Furtado, J., and Serôdio, J. (2013a). Photobiology and growth of leather coral Sarcophyton cf. glaucum fragments stocked under low light in a recirculated system. Aquaculture 414–415, 235–242. doi: 10.1016/j.aquaculture.2013.08.018
Rocha, R. J. M., Serôdio, J., Costa, M., Cartaxana, P., and Calado, R. (2013b). Effect of light intensity on post-fragmentation photobiological performance of the soft coral Sinularia flexibilis. Aquaculture 391, 24–29. doi: 10.1016/j.aquaculture.2013.01.013
Schlichter, D. (1982). Nutritional strategies of cnidarian: the absorption, translocation and utilization of dissolved nutrients by Heteroxenia fuscecens. Am. Zool. 22, 659–669. doi: 10.1093/icb/22.3.659
Schlichter, D., Kremer, B. P., and Svoboda, A. (1984). Zooxanthellae providing assimilatory power for the incorporation of exogenous acetate in Heteroxenia fuscescens (Cnidaria: Alcyonaria). Mar. Biol. 83, 277–286. doi: 10.1007/BF00397460
Schlichter, D., Svoboda, A., and Kremer, B. P. (1983). Functional autotrophy of Heteroxenia fuscescens (Anthozoa: Alcyonaria): carbon assimilation and translocation of photosynthates from symbionts to host. Mar. Biol. 78, 29–38. doi: 10.1007/BF00392968
Schoepf, V., Grottoli, A. G., Warner, M. E., Cai, W. J., Melman, T. F., Hoadley, K. D., et al. (2013). Coral energy reserves and calcification in a high-CO2 world at two temperatures. PLoS One 8:e75049. doi: 10.1371/journal.pone.0075049
Slattery, M., and McClintock, J. B. (1995). Population structure and feeding deterrence in three shallow-water antartic soft corals. Mar. Biol. 122, 461–470. doi: 10.1007/BF00350880
Slattery, M., Renegar, D. A., and Gochfeld, D. J. (2013). Direct and indirect effects of a new disease of alcyonacean soft corals. Coral Reefs 32, 879–889. doi: 10.1007/s00338-013-1035-z
Smith, P. K., Krohn, R. I., Hermanson, G. T., Mallia, A. K., Gartner, F. H., Provenzano, M. D., et al. (1985). Measurement of protein using bicinchoninic acid. Anal. Biochem. 150, 76–85. doi: 10.1016/0003-2697(85)90442-7
Tentori, E., and Allemand, D. (2006). Light-enhanced calcification and dark decalcification in isolates of the soft coral Cladellia sp. during tissue recovery. Biol. Bull. 211, 193–202.
Tentori, E., Allemand, D., and Shepherd, R. (2004). Cell growth and calcification result from uncoupled physiological processes in the soft coral Litophyton arboreum. Mar. Ecol. Prog. Ser. 276, 85–92. doi: 10.3354/meps276085
Tremblay, P., Grover, R., Maguer, J. F., Legendre, L., and Ferrier-Pagès, C. (2012). Autotrophic carbon budget in coral tissue: a new 13C-based model of photosynthate translocation. J. Exp. Biol. 215, 1384–1393. doi: 10.1242/jeb.065201
Van Alstyne, K. L., Schupp, P., and Slattery, M. (2006). The distribution of dimethylsulfoniopropionate in tropical Pacific coral reef invertebrates. Coral Reefs 25, 321–327. doi: 10.1007/s00338-006-0114-9
Van Alstyne, K. L., Wylie, C. R., Paul, V. J., and Meyer, K. (1992). Antipredator defenses in tropical Pacific soft corals (Coelenterata: Alcyonacea). I. Sclerites as defenses against generalist carnivorous fishes. Biol. Bull. 182, 231–240. doi: 10.1016/0022-0981(94)90222-4
Keywords: soft corals, tissue descriptors, normalization, ash-free dry weight, freeze-drying
Citation: Pupier CA, Bednarz VN and Ferrier-Pagès C (2018) Studies With Soft Corals – Recommendations on Sample Processing and Normalization Metrics. Front. Mar. Sci. 5:348. doi: 10.3389/fmars.2018.00348
Received: 13 April 2018; Accepted: 07 September 2018;
Published: 27 September 2018.
Edited by:
Juan Armando Sanchez, University of Los Andes, ColombiaReviewed by:
David Suggett, University of Technology Sydney, AustraliaSusana Enríquez, Universidad Nacional Autónoma de México, Mexico
Copyright © 2018 Pupier, Bednarz and Ferrier-Pagès. This is an open-access article distributed under the terms of the Creative Commons Attribution License (CC BY). The use, distribution or reproduction in other forums is permitted, provided the original author(s) and the copyright owner(s) are credited and that the original publication in this journal is cited, in accordance with accepted academic practice. No use, distribution or reproduction is permitted which does not comply with these terms.
*Correspondence: Chloé A. Pupier, cpupier@centrescientifique.mc; chloe.pupier@gmail.com