- 1Department of Mathematics & Statistics, University of Strathclyde, Glasgow, United Kingdom
- 2Marine Laboratory, Marine Scotland Science, Aberdeen, United Kingdom
The zooplanktivorous schooling fish sandeels A. marinus comprise a quarter of North Sea fish biomass and are essential food for a number of marine mammals and birds. However, in recent decades there has been a decline in the abundance of older sandeels, with increased overwinter mortality proposed as a contributor. To survive winter animals require a minimum energy reserve which is determined by temperature, prey abundance, and possibly prey size. Here a new dynamic energy budget model is created to determine the influence of food and temperature on sandeel energy dynamics. The model hindcasts changes in energy and survival between 2000 and 2008, a period of pronounced stock decline in the northwestern North Sea. Overwinter starvation mortality was a large contributor toward a recent decline in sandeels in northern UK waters. Highest overwinter mortality rates were recorded for juveniles and not individuals aged 1 or over due to the effect of weight-specific metabolism. However, a sensitivity analysis of the model suggests that mortality rates are more sensitive to changes in copepod abundance in the build up to overwintering rather than temperature during overwintering. Further, projections show that temperature rises are negated by increases in large, but not small copepods. The conclusion is that food-driven size-selective starvation mortality contributed to the northwestern North Sea stock decline and that indirect food web effects of climate change are greater than direct physiological effects on sandeels.
Introduction
As an adaptation to low food availability many fish species build up energy reserves which are mobilized during periods of food scarcity (Biro et al., 2004; Tomiyama and Yanagibashi, 2004). Sandeels, a small shoaling fish, is an archetypal example. Aside from a spawning period in January–February (Régnier et al., 2018), these fish overwinter in the sediment between August and April subsisting on energy reserves acquired during a short feeding season (Winslade, 1974; Reeves, 1994). The sediment offers refuge from predation, which is huge (Reay, 1970), but sandeels require a minimum energy reserve to survive overwintering and reproduce, and therefore must balance a trade-off between foraging and remaining in the sediment (van Deurs et al., 2010).
Accounting for ~25% of North Sea fish biomass (Christensen et al., 2013), sandeels are crucial prey for a number of seabird species (Wanless et al., 1998, 2004; Lewis et al., 2001; Lahoz-Monfort et al., 2011). A. marinus, hereafter sandeels, is the most abundant sandeel species, comprising more than 90% of sandeel fishery catches (Macer, 1966; Goodlad and Napier, 1997). Sandeels begin life in winter as eggs on the seabed (Reay, 1970; Bergstad et al., 2001), and, after a larval stage, settle as juveniles in early summer. Individuals alternate between surface feeding and burying in the sediment (Ryland, 1964; Macer, 1966; van Deurs et al., 2013) before overwintering between spring and autumn, and the following spring (Reeves, 1994). A threshold fat content is required to begin overwintering (Winslade, 1974). Larger fatter individuals enter overwintering earlier than smaller individuals, with 0-group fish spending 1–2 months longer feeding, suggesting they need more time to acquire energy reserves for overwintering (Macer, 1966; Reeves, 1994; Kvist et al., 2001; Tomiyama and Yanagibashi, 2004).
Two selective pressures drive overwintering, starvation from exhausted reserves and predation mortality. The influence of these pressures varies according to sandeel size. Many 0-group fish use energy to grow rapidly to reduce predation pressure (Anthony et al., 2000), with some maintaining lipid levels just above the minimum required to survive, even when physiologically capable of allocating energy to reserves (Biro et al., 2005). Once a threshold size is reached, sandeels, like many other species, begin to store considerably more lipid (Robards et al., 1999; Post and Parkinson, 2001; Biro et al., 2005; Kooka and Yamamura, 2012). This threshold size corresponds to the minimum length of an overwintering sandeel (Holland et al., 2005), suggesting the onset of overwintering is determined by lipid stores (Robards et al., 1999; Holland et al., 2005).
Overwinter mortality caused by lipid reserve depletion is a huge contributor to overall mortality of 0-group fish (Hurst and Conover, 2003; Biro et al., 2004). Lipid depletion can occur in winter for several reasons. First, reserves are primarily determined by the quality and availability of prey during the feeding season. Sandeel growth rate, which likely correlates with reserve mass, may depend on prey size as well as biomass (van Deurs et al., 2014). This hypothesis is consistent with observed declines in copepod size (Reid et al., 1998, 2001; Beaugrand et al., 2002; Drinkwater et al., 2003; Reygondeau and Beaugrand, 2011), large copepod abundance (Reid et al., 2003) and sandeel growth rate (Wanless et al., 2004). Reserves are also sensitive to temperature rises, which accelerates lipid loss increasing the likelihood of starvation (van Deurs et al., 2011). Moreover, increased temperature accelerates sandeel oxygen consumption and also reduces oxygen concentration within the sediment (Benson and Krause, 1984), increasing mortality. Another mechanism for overwinter mortality is size-dependent reserve loss. Not only do smaller fish have fewer reserves than larger individuals, they burn reserves at a faster rate due to weight-specific metabolism (metabolism ~ body weightr; r < 1, Clarke and Johnston, 1999). Consequently, starvation risk increases with decreasing size (Oliver et al., 1979; Kirjasniemi and Valtonen, 1997; Schultz et al., 1998; Biro et al., 2004), making 0-group sandeels the most vulnerable age class. Zero-group overwinter starvation probability has likely increased in recent decades because of a decline in length (Wanless et al., 2004; van Deurs et al., 2011). This has been linked to declining sandeel abundance in an unfished region of the Northwestern North Sea (MacDonald et al., 2015, Figure 1). Since 2000, larval abundance has declined but 0-group abundance has been maintained (Heath et al., 2012a). In other words, the number of 0-group sandeels has not changed dramatically over time, while the number of older sandeels has continued to decline. Increasing starvation mortality of sandeels in their first winter can cause this pattern.
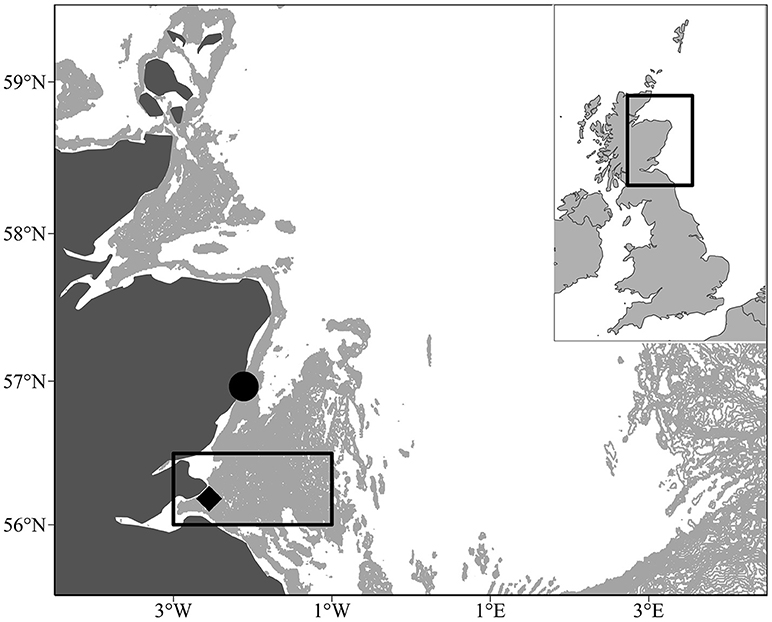
Figure 1. Location of the study area off the Scottish east coast (black box, 56° 00N and 56° 30N and longitudes 003° 00W and 001° 00W). The pale gray shaded area indicates water depths between 30 and 70 m. This covers the Wee Bankie/Marr Bank/Berwick's Bank sandbank complex, which is prime sandeel habitat (Proctor et al., 1998; Pedersen et al., 1999). The black diamond denotes the Isle of May seabird colony. The black circle denotes the plankton monitoring site at Stonehaven where food and temperature measurements were taken between 2000 and 2008 (56° 57.83'N, 002° 06.74'W).
Two main factors have been suggested as responsible for the decline in sandeel abundance, increases in overwinter and predation mortality (MacDonald et al., 2015). There is currently no evidence that fish predators have suppressed the sandeel stock off the Firth of Forth although this is a hypothesis worthy of investigation. While there is no evidence for increases in overwinter mortality, van Deurs et al. (2011) suggested the existence of a threshold size for overwinter survival. Therefore, evidence for declines in length of overwintering sandeels suggests an increased starvation risk. To address the hypothesis that overwinter starvation is a major contributor to a decline in abundance a new Dynamic Energy Budget (DEB) model is used to hindcast changes in sandeel energy and survival between 2000 and 2008. The model is a modified version of DEB models used to model salmonid growth (Broekhuizen et al., 1994; Jones et al., 2002). Overwinter mortality occurs when reserves are depleted, which are primarily determined by size, prey quality and availability, and temperature. DEB models are appropriate since they (1) describe the rates at which an individual organism acquires energy and uses it for growth or reproduction based on size and maturation status (Kooijman, 2010) and (2) allow a more direct measure of an individual's ability to withstand starvation (Broekhuizen et al., 1994).
Materials and Methods
The Model
The following sections offer an overview of the model. Key model elements are described in Table 1. For a full mathematical description of this model and its parameters, see Appendix F in Supplementary Material. Individual sandeels are modeled in terms of the energy content (kJ) of structure (S), reserves (R) and gonads (G). Sandeel condition (ρ) is an important component of the model. Condition influences models of energy allocation (allocating energy from reserves to structure and/or gonad tissue), starvation mortality and ingestion. Previous DEB models have used the reserve ratio, defined as the ratio of reserve energy to structural energy, as a proxy for condition (Broekhuizen et al., 1994; Jones et al., 2002). The ratio of reserve energy to structural and gonad energy () is used as a proxy for condition, and is referred to as the “reserve ratio,” or simply condition.
The metabolic cost of sustaining the life of an individual is prioritized over growth and reproduction. Therefore, energy allocation to gonad and structure should only occur after metabolic costs are covered. This is modeled using the following assumptions: 1. All assimilated energy enters reserves. 2. Metabolic costs are subtracted from reserves. 3. Once metabolic costs have been paid a fraction of remaining assimilated energy is allocated to structure and gonads. The rate of change of R, S, and G are modeled using three ordinary differential equations. The rate of change of reserve energy (kJ d−1) is given by:
where A is the rate at which an animal assimilates energy (kJ d−1) and M is the rate at which animals lose energy to metabolism (kJ d−1).
The rate of change of structural energy is given by:
where ρ is the sandeel reserve ratio and C(ρ, S) is the fraction of assimilated energy remaining after metabolism that is used to form structure (Appendix F in Supplementary Material). C(ρ, S) decreases with length and increases with the reserve ratio (Figure A1 in Supplementary Material). This is because (1) small sandeels appear to use much more energy to build structure than larger sandeels (Robards et al., 1999) meaning C(ρ, S) must be a decreasing function of length and (2) individuals with a high reserve ratio allocate more energy to structure than those with poor reserve ratios meaning C(ρ, S) must be an increasing function of the reserve ratio.
The rate of change of gonad energy is given by:
where G(ρ, S) is the fraction of reserve energy directed to gonads (Appendix F in Supplementary Material). Modeled gonad energy allocation occurs on 1st November and gonad formation efficiency is assumed to be 100%. Mean spawning day in the northwestern North Sea between 2000 and 2009 is 21st January (MacDonald, 2017).
Metabolism
Metabolism scales allometrically with body weight and increases with temperature. Metabolism scales with body weight to a power <1 meaning larger fish have lower weight-specific metabolism than smaller fish. Sandeel metabolism is higher during feeding season than overwintering due to activity associated with foraging. Foraging occurs during daylight (Ryland, 1964) and sandeels spend 2 times their standard metabolic rate when active (SMR) (van Deurs et al., 2015). To estimate the effect of temperature and body size on metabolism, respiration data from the closely related species, A. hexapterus, is used (Quinn and Schneider, 1991, see Appendix A in Supplementary Material).
Ingestion
Modeled ingestion rate scales allometrically with structural weight and increases with temperature. Further, ingestion rate increases in response to low condition and day length Ingestion rate also related to zooplankton community abundance and composition (Appendix B in Supplementary Material). Sandeels feed on copepods, crustacean larvae and annelids during daylight (Ryland, 1964; Macer, 1966; van Deurs et al., 2013). They appear to actively search for larger prey items, ignoring smaller ones (van Deurs et al., 2014). Copepod prey is therefore separated into large and small groups . To account for non-copepod prey, an extra constant background food concentration is assumed. Each of these food concentrations has an associated attack rate to reflect sandeels preference for each different prey group. Due to scarcity of data on A. marinus ingestion rate empirical data from the closely related species, A. tobianus, is used to inform the model (van Deurs et al., 2010, 2015) (Appendix B in Supplementary Material).
There is evidence that sandeels increase their ingestion rates when in poor condition. Animals grow extremely rapidly after going more than two-thirds of the year without feeding, taking only 2 months to double their energy content (Hislop et al., 1991). Modeled sandeels are able to increase their normal maximum ingestion rate when the reserve ratio decreases below a critical value τ.
Overwintering Timing
Sandeels must cease feeding and overwinter for a prolonged period. Sandeels emerge within a short window between the beginning of March and the end of April (Reeves, 1994). However, the causes of timing are not understood. Animals are therefore assumed to stop overwintering on April 1st.
In contrast to the overwintering end date, there is considerable variation in overwintering start dates, which can range between May and October (Reeves, 1994). Modeled sandeels overwinter when a reserve ratio threshold is reached. The timing of this overwintering threshold is size-specific; smaller sandeels require higher reserve ratios to overwinter than larger sandeels at length. This is because smaller animals have higher weight specific respiration rates and therefore deplete energy reserves at a relatively faster rate.
Mortality
Modeled sandeels incur two types of mortality: condition-dependent starvation mortality and a cohort-specific background mortality. Background mortality covers all types of mortality except starvation mortality. Starvation may start to occur once the reserve ratio falls below a critical value and is regarded as a probabilistic process, so a given fraction of the population is removed each day. Each cohort has an associated background mortality which is constant over its lifetime.
Relating Sandeel Energy Content to Length and Weight
Sandeel energy data is required to fit the model and estimate unknown parameters, however this data is not available. Instead, the model is validated by converting R, S, and G to length and weight, and comparing with observed length and weight estimates from survey data.
Reserve, structural and gonad energy is converted into wet weight using two steps. First, R, S, and G are converted to dry weight using dry weight energy densities. Then, reserve, structural, and gonad dry weight is converted into wet weight using conversion factors.
Rdry Sdry and Gdry is the ratio of wet weight to dry weight for reserve, structural and gonad tissue. Er, Es, Eg are energy densities for reserve, structural and gonad dry weight.
By definition, structure cannot decrease. Consequently, structure is a proxy for length. Several DEB models (Broekhuizen et al., 1994; Jones et al., 2002) assume this relationship takes the form:
where α and β are the length-structure scale and the length-structure exponent, respectively. Rdry, Sdry, Gdry, α and β are empirically derived (Appendix C in Supplementary Material).
Environmental Drivers
The model is driven by temperature T (°C), and prey energy concentration (kJ m−3). High-resolution food and temperature data were not available for the Firth of Forth. Therefore, food and temperature from a location ~60 km north of the center of the study region was used. Zooplankton samples and temperature readings were taken in Stonehaven (56° 57.83′N, 002° 06.74′W, Figure 1) between January 2000 and December 2008. For full details on sampling methods, see Bresnan et al. (2015). Temperature readings were taken using reversing thermometers and CTD probes. Surface and seabed temperature (45 m) is used in the model to account for temperature variation experienced by sandeels when they are feeding and overwintering, respectively. Copepod abundance was grouped by species and stage. Large copepods are defined as any of the advanced Calanus stages (Table A4 in Supplementary Material). Small copepods are defined as being any of the other major calanoid copepod species (Table A5 in Supplementary Material). Copepod energy content was estimated in the following way: First, copepod prosome length was converted to wet weight (van Deurs et al., 2015), then into energy content using energy densities for small (<1.3 mm) and large copepods (>1.3 mm) (van Deurs et al., 2013, Appendix H in Supplementary Material). Large and small copepod biomass concentration was estimated by fitting smooths (LOESS, span = 0.04) to the time series of copepod measurements (Figure A2 in Supplementary Material).
Initial Conditions of the Model
The model is run using reserve and structural energy of 0-group and age 1 fish in summer as initial conditions (Figure A3 in Supplementary Material). Each cohort consists of a group of individuals, each characterized by a unique structural and reserve energy, and abundance. Unfortunately, no observations of sandeel energy between 2000 and 2008 were available; only length and weight estimates are available from survey data. However, structural and reserve energy can be estimated by using empirically derived relationships between length, weight and energy content (section Relating Sandeel Energy Content to Length and Weight).
Parameter Estimation and Model Implementation
Parameter values (Table A2, Appendix D in Supplementary Material) are found using the following method. First, empirical data from the literature is used if available. If parameters are not available, they are derived using empirical data if possible. If a parameter is not available from the literature and cannot be derived, it is treated as a fitting parameter. Fitting parameters were found by fitting the model to length and weight data.
The model is implemented in C and parameterized in R. Ordinary differential equations (Equations 1–3) were solved numerically using the Euler method with discrete daily time steps. To ensure the discrete differential equations converged to the continuous differential equations, model results using the Euler method and the ode45 method in the deSolve package (R Development Core Team, 2014) were compared. The model was fitted by selecting the set of unknown model parameters (Ω) that minimized the error between observed and modeled length, weight, reserve ratio, and abundance at survey date.
where Li, a, y, s denotes the ith individual at age a, year y, and survey time s.
This error was minimized by using simulated annealing in R (GenSA package).
This was done because there are many local minima due to the complexity of the model.
Model Experiments
The DEB model was used to unravel the relative influence of food and temperature on sandeel growth (see section Relative Influence of Food and Temperature on Sandeel Physiology). Modeled year-to-year changes in sandeel growth are dependent on the amount of food available during the short feeding period and/or the effect of temperature on metabolism during the long overwintering period. This was done by running the baseline model using climatological average temperature and food separately, and comparing length, weight, and energy predictions with estimates derived using observed food and temperature conditions. Large deviations from these estimates would imply large sensitivity to temperature or food. Vice-versa, small deviations from the best model fit would imply robustness to temperature or food.
A sensitivity analysis was undertaken to determine the relative influence of year-to-year variation in food and temperature on year-to-year changes in sandeel length, weight and energy content. The model was run using climatological average food and actual temperature, and then run again using climatological average temperature and actual food. Results were then compared to those expected using actual food and temperature.
The model was used to predict the effects of changes in temperature and small and large copepod abundance on sandeel abundance (see section Projecting Future Changes in Sandeel Size, Energy, and Survival). Temperature was varied between −5 and 5°C relative to the climatological average temperature between 2000 and 2008. Large and small copepod abundance was varied between −100 and 100% relative to the climatological average. Changes in length, weight, energy, and abundance of the average sandeel cohort during the study period were then estimated for each combination of temperature and food. To estimate the average cohort, the abundance of each sandeel 0-group was normalized and all initial length and weight estimates were combined, producing abundance-at-length-and-weight for the average sandeel cohort between 2000 and 2008 (Figure A4 in Supplementary Material). Changes in mean length, weight, energy content and overwinter survivorship of 0-group sandeels were tracked under different temperature and food conditions until the following July.
Results
Year-to-Year Changes in Energy Content
Given measurements of abundance at age, weight, and length for each cohort, the model was used to reconstruct past changes in energy and condition (Figures 2, 3). Figure 2 shows the ratio of reserve energy to structural energy. Reserve ratio shows a strong seasonal pattern, especially for cohorts born in 2000, 2001, and 2002. Minimum and maximum reserve ratios occurred in March and June, respectively.
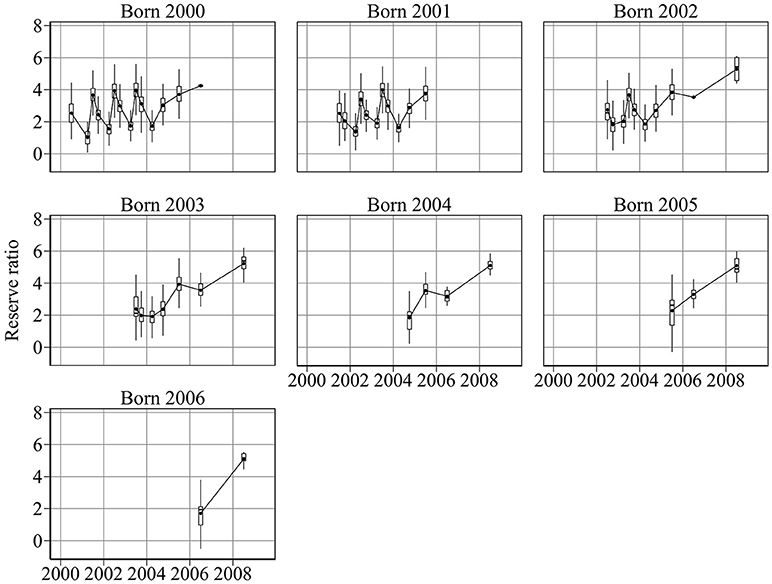
Figure 2. Year-to-year changes in cohort reserve ratio, the proxy for sandeel condition. Sandeel condition is highly seasonal, reaching a minimum in March and a maximum in June. Condition generally increases with age.
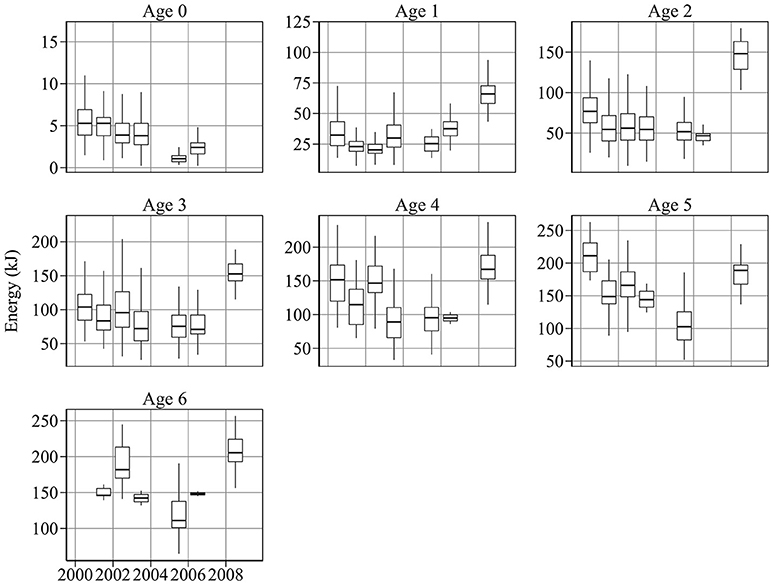
Figure 3. Year-to-year changes in energy content per sandeel. There was a general decline in energy content between 2000 and 2006 for most age groups. Energy per sandeel was relatively high in 2008.
Figure 3 shows year-to-year changes in sandeel energy content. There was considerable inter-annual variation in energy at age. 0-group energy content varied by a factor of 4 during the study period, ranging from 5.8 kJ in 2000, to 1.2 kJ in 2005 (Figure 3). A similar range was detected in age 1 fish, which had energy values of 16.7 and 73.2 kJ in 2002 and 2009, respectively.
Relative Influence of Food and Temperature on Sandeel Physiology
The model provides good fits to length, weight, reserve ratio, and abundance data (Figure 4). Large changes in modeled length, weight, and energy revealed that variation in food was more influential than variation in temperature (Figure 5). The climatological average food model produced large changes in length, weight, and energy, while the climatological average temperature model did not alter results demonstratively (Figure 5). For example, using observed and climatological average temperature in the model resulted in mean lengths of 10.09 and 10.14 cm for age 1 sandeels at the dates of the summer survey, respectively. However, using climatological average food in the model resulted in greater mean lengths of 11.65 cm.
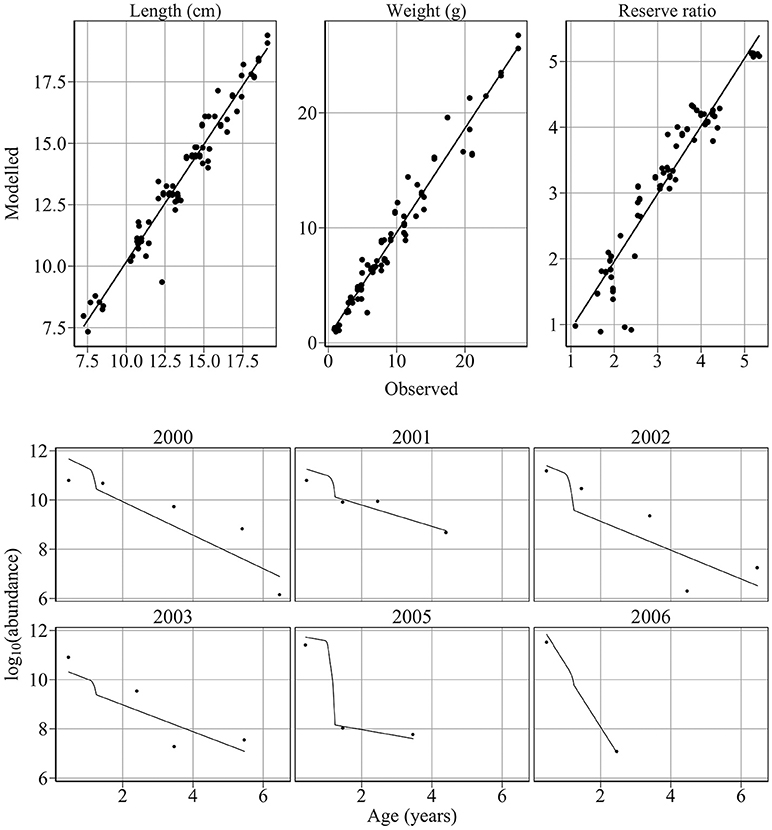
Figure 4. Comparison of modeled and observed weight, length, reserve ratio and abundance. Upper panel: Comparison of modeled and observed weight, length, reserve ratio. Points represent observed mean values at survey dates. Black lines represent linear fits through the data. The model provides excellent fits to weight (R2 = 0.96, n = 83, intercept = −0.224, slope = 1.063), length (R2 = 0.95, n = 83, intercept = 0.0292, slope = 0.996) and reserve ratio data (R2 = 0.91, n = 83, intercept = 0.394, slope = 0.88). Lower panel: comparison of modeled and observed abundance at age. Points represent observed abundance at age. Black lines represent modeled abundance at age. The model provides excellent fits to the 2000 (R2 = 0.71, p < 0.05, n = 5), 2001 (R2 = 0.87, p < 0.05), and 2005 (R2 = 0.996, p < 0.03, n = 3) cohorts and satisfactory fits to the 2002 (R2 = 0.67, p < 0.06), 2003 (R2 = 0.73, p < 0.1, n = 4), and 2006 (n = 2) cohorts. The model provides an excellent fit to sandeel abundance at age over the study period (R2 = 0.84, p < 1e-9, n = 23).
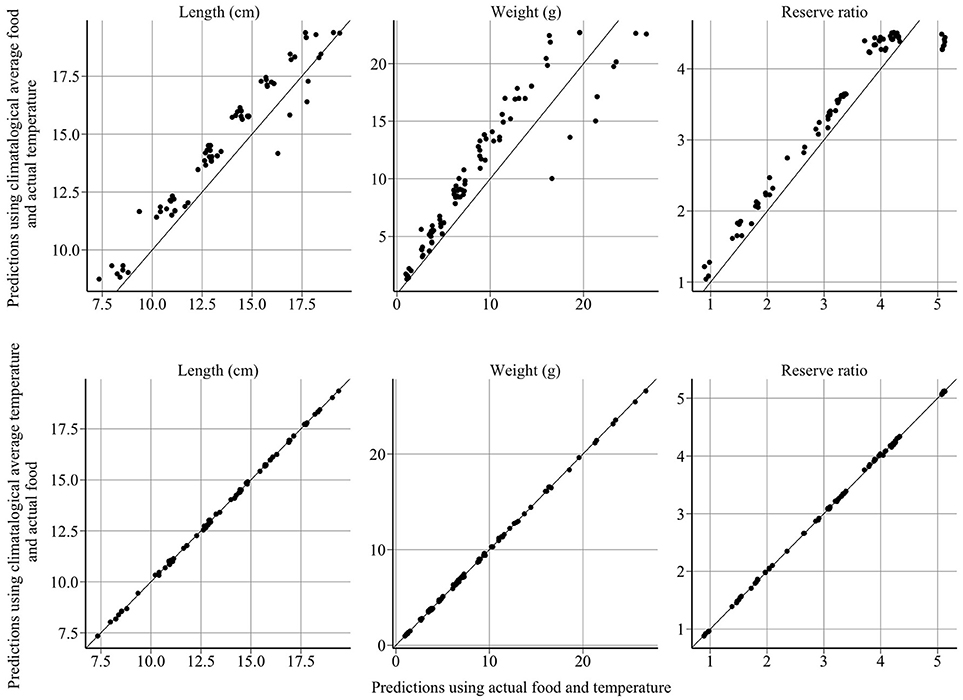
Figure 5. Model sensitivity of length, weight and reserve ratio to food and temperature. The x-axis represents the model predictions and the y-axis represents predictions using climatological averages. Year-to-year changes in sandeel size and energy are driven by variation in food, not temperature.
Year-to-year changes in food were also the most important driver of variation in annual survival rates (Figure 6). Figure 6 shows first year survival of 0-group sandeels under observed environmental conditions (red line), and climatological averaged food and temperature (blue and black lines). First year survivorship under observed environmental conditions ranged from ~0.03% in 2005/06 to 11.69% in 2003/04 (Figure 6). The magnitude of starvation mortality varied year-to-year. 68.5% of sandeels born in 2005 succumbed to starvation mortality compared to 2.6% of sandeels born in 2006. Modeled survivorship was markedly higher when using a climatological average for food, and ranged from 0.45% in 2006/07 to 47.29% in 2005/06 (Figure 6, Table 2).
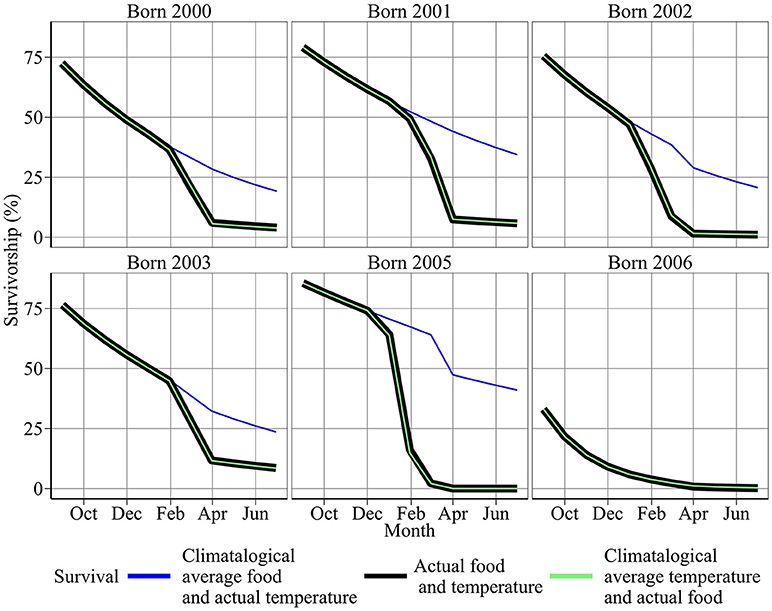
Figure 6. Sensitivity of survival to food and temperature. Red points denote results using the best model fit. Blue and black points denote results using climatological average food and temperature between 2000 and 2008, respectively.
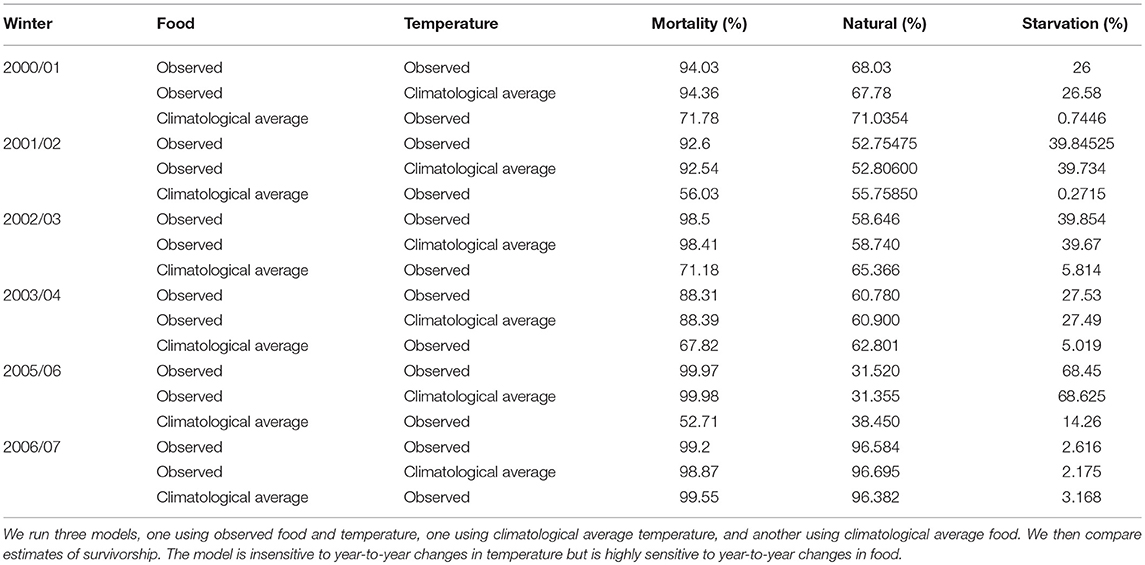
Table 2. Sensitivity of modeled 0-group survivorship (%) between June and the following April to food and temperature.
Projecting Future Changes in Sandeel Size, Energy, and Survival
Due to the positive effect of temperature on growth in the model a 5°C rise increases energy, weight and length by 32, 31, and 9% from baseline values, respectively. Energy increased from 45 to 59.5 kJ, weight from 6.4 to 8.4 g and length from 12.2 to 13.3 cm. However, a 5°C temperature rise also leads to higher overwinter mortality, with the baseline biomass value declining by 65%.
Figure 7 shows the modeled changes in sandeel abundance in relation to deviations from the climatological average food and temperature. If large copepod abundance is equivalent to the climatological average, changes in sandeel abundance are driven primarily by changes in temperature, not changes in small copepod abundance (left panel, Figure 7). In contrast, if small copepod abundance is equivalent to the climatological average, changes in sandeel abundance are driven by a combination of temperature changes and changes in large copepod abundance (right panel, Figure 7).
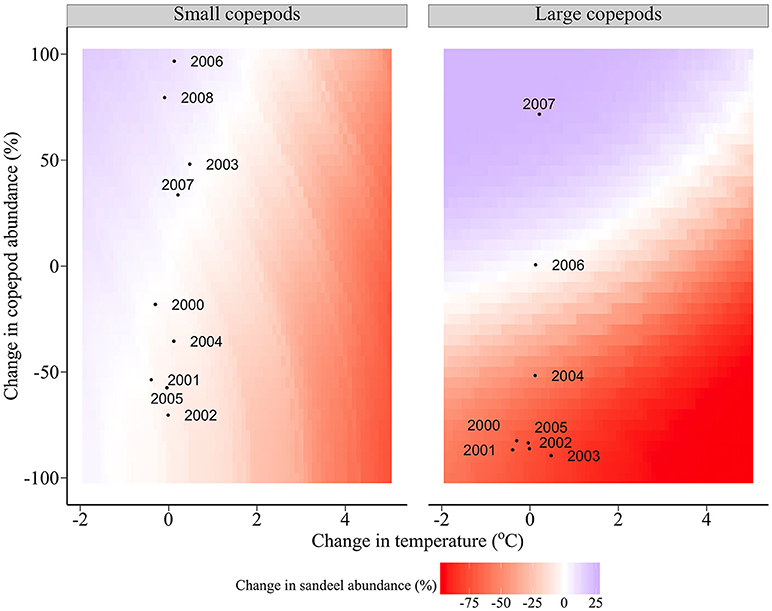
Figure 7. Changes in abundance of the average sandeel cohort in relation to deviations from the climatological average food and temperature between 2000 and 2008. Points represent actual food (between Julian days 145 and 212) and temperature measurements (annual). The change in large copepod abundance for 2008 is not shown (change = 498%).
Modeled Prey Composition of Sandeels
Individuals displayed a preference for large copepods. Half-saturation constants were 0.0607 and 0.253 g WW m−3 for 6 cm sandeels feeding on large and small copepods, respectively. Figure 8 shows mean monthly prey composition of a 6 cm sandeel between April and July. Perhaps surprisingly, non-copepod food was the most important prey (~57% of mean energy intake), more so than large (~33% of mean energy intake) or small (~10% of mean energy intake) copepods between 2000 and 2008.
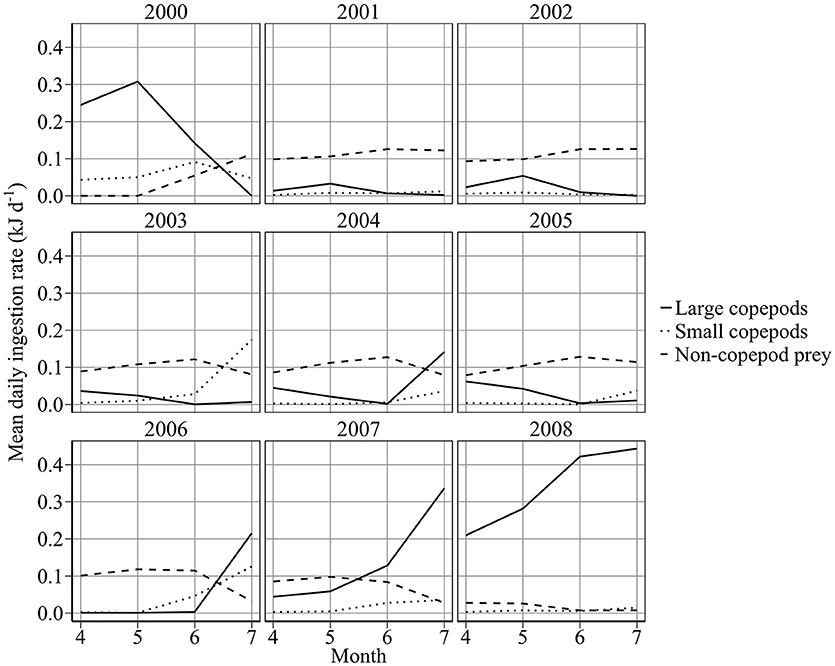
Figure 8. Modeled mean daily ingestion rate (kJ d−1) of a 6 cm sandeel feeding on large, small, and non-copepod prey.
Discussion
Recent changes in sandeel growth and mortality reflected changes in copepod biomass concentration, rather than variation in temperature (Figures 6, 7). Overwinter starvation was a key component of 1st year mortality and contributed to declines in abundance (Figure 6). The importance of lipid reserves for 0-group fish survival and the magnitude of starvation have been highlighted before for rainbow trout Onchorhynchus mykiss (Biro et al., 2004). Modeled mortality was consistent with published estimates for A. marinus, with mortality >90% for 0-group fish (Reay, 1973; Cook, 2004). It is not the increase in metabolic costs associated with increased temperature, which uses up sandeel energy reserves faster, that causes the overwinter mortality; it is the fact that these reserves are smaller because of the poorer feeding conditions. Sandeels are bottom-up limited by phytoplankton (Frederiksen et al., 2006; Eliasen et al., 2011) and zooplankton (Frederiksen et al., 2006), and spatial variation in sandeel productivity may reflect regional differences in copepod abundance (Heath et al., 2012b; O'Brien et al., 2013). Sandeels off the Firth of Forth may have the fewest foraging opportunities in the North Sea since the spring bloom is shorter than anywhere else (Fransz et al., 1991) and copepod abundance is typically lower than in central and southern areas (Edwards et al., 2010). This may be why field data on sandeel stomach content off the Firth of Forth show that appendicularians are the main prey (García et al., 2012), and explain why no relationship exists between Calanus spp. abundance and the proportion of sandeels in seabird diets (Howells et al., 2017). Modeled sandeels mostly consumed non-copepod prey (Figure 8), reflecting these points.
North Sea sea surface temperature may rise by more than 3°C by 2100 if emissions continue at similar rates (IPCC, 2007). Under current levels of prey abundance and community composition, a 5°C rise slightly increases sandeel growth but leads to a considerable increase in overwinter starvation due to accelerated reserve loss under higher temperatures. Negative impacts of climate warming on sandeel physiology are negated by an increase in large copepod abundance, but not by the abundance of small copepods. For example, a 1°C rise is negated by an increase in large copepod abundance of 11%, however an increase of small copepods by 41% is required to maintain sandeel abundance at current levels (Figure 7). Short term (<10 years) variation in sandeel abundance is driven by changes in large copepods. Under current temperatures, a 10% decrease in large and small copepods reduces sandeel abundance by 6 and 1%, respectively (Figure 7). Copepod size is expected to decrease at a given latitude (Reid et al., 1998, 2001; Beaugrand et al., 2002; Drinkwater et al., 2003; Reygondeau and Beaugrand, 2011), therefore, sandeels, which are highly residential, will feed on smaller prey, reducing growth and survival (van Deurs et al., 2014, this study). Mitigating negative effects of smaller prey by extending the foraging season is unlikely if the optimal feeding period occurs in late spring/early summer and fitness is enhanced by remaining in the sediment (van Deurs et al., 2010).
Ocean warming is largely predicted to result in decreases in fish size (Baudron et al., 2014; van Rijn et al., 2017). Our model is inconsistent with this prediction, showing that a 5°C rise leads to a slight increase in sandeel growth. However, recent experimental work shows that A. marinus somatic energy content will not be significantly affected by a 5°C temperature rise (Wright et al., 2017). While the importance of direct temperature effects on sandeel population dynamics cannot be conclusively ruled out, this work is not the first to suggest they have a weak effect on growth and mortality (Buckley et al., 1984; Pitois et al., 2012). This can explain why sandeel populations have not declined in areas that have undergone most warming, for example the Southern North Sea.
Sandeel energy content at age varied markedly between years (Figure 3). For instance, seabirds feeding on 0-group sandeels in 2000 ingested almost 400% more energy per fish than in 2005 (Figure 3). Such extreme variation in prey energy content can impact breeding success of single prey loading species (Wanless et al., 2005). Although spatial variation in energy content has been demonstrated across the North Sea and its implications on single prey loading seabirds discussed (Rindorf et al., 2016), this study is the first to provide a long-term account of variation in energy content.
Due to a lack of empirical data on A. marinus experimental data on closely related species was used. This decision is justified by similarity in behavior, body shape and length-weight relationships of sandeel species (Table A3, Appendix E in Supplementary Material). Future work should determine if sandeel ingestion rate is strongly dependent on prey size (van Deurs et al., 2014), and if non-copepod prey form a large proportion of the diet (García et al., 2012). This will require sampling sandeel stomach content, zooplankton size, and concentration in the field. Another model assumption was that density dependent effects on growth were negligible. However, density dependence is likely limited to the egg and larval stages, while only post-metamorphic sandeels are modeled. Cannibalism on post-metamorphic individuals is low (Ritzau Eigaard et al., 2014) and growth suppression of small fish by larger individuals is unlikely since sandeels usually aggregate according to size (Johnsen et al., 2009; Jensen et al., 2011).
Due to an absence of zooplankton abundance data from the Firth of Forth, a time series of weekly measurements of copepod concentration from another monitoring site was used. It is known that C. finmarchicus are transported into the North Sea from waters beyond the European shelf, so arrive later in Southern areas (Heath et al., 1999). The implication is that copepod phenology is earlier than it should be in the model. However, this effect is likely minimal due to the close proximity of the two monitoring sites. It was also assumed that copepod energy content remained fixed in time, however, this may show seasonal and annual variation (Miller et al., 2000; McKinstry et al., 2013).
The cause of the sandeel stock decline in the northwestern North Sea was addressed by considering only direct physiological and lifecycle changes to the sandeel. The role of predation mortality was not analyzed, and this is undoubtedly an important driver of stock dynamics. However, piscivorous fish capable of suppressing the sandeel stock (Hislop, 1997; Greenstreet et al., 1998) did not increase off the Firth of Forth (Greenstreet et al., 2010). Further, increased predation by fish that prey on the larval sandeels (Frederiksen et al., 2007) appears unlikely since larval survival has increased (Heath et al., 2012a). The sandeel stock may be suppressed by other pelagic fish. For example, the abundance of mackerel, which prey on sandeels and compete for food (Jones, 1983), has increased substantially in the North Sea since 2000 (Engelhard et al., 2008; Jansen, 2016).
Author Contributions
AM, MH, and DS designed the manuscript. AM performed the analysis and wrote most of the manuscript. All authors contributed to the interpretation of results and the writing of the manuscript.
Funding
This work received funding from the MASTS pooling initiative (The Marine Alliance for Science and Technology for Scotland) and Marine Scotland Science whose support is gratefully acknowledged. MASTS is funded by the Scottish Funding Council (grant reference HR09011) and contributing institutions.
Conflict of Interest Statement
The authors declare that the research was conducted in the absence of any commercial or financial relationships that could be construed as a potential conflict of interest.
Acknowledgments
Many thanks to Robert Wilson, Neil Banas, Graeme Ruxton, and Phil Boulcott for data and fruitful discussions. Thanks to Mikael van Deurs who kindly provided sandeel size and energy data. The long-term commitment to sampling at Stonehaven and off the Firth of Forth was provided by the Scottish Government. We gratefully acknowledge the assistance of the crew members of the survey vessels Shuna and Temora at Stonehaven, and Clupea and Alba Na Mara off the Firth of Forth.
Supplementary Material
The Supplementary Material for this article can be found online at: https://www.frontiersin.org/articles/10.3389/fmars.2018.00339/full#supplementary-material
References
Anthony, J. A., Roby, D. D., and Turco, K. R. (2000). Lipid content and energy density of forage fishes from the northern Gulf of Alaska. J. Exp. Mar. Biol. Ecol. 248, 53–78. doi: 10.1016/S0022-0981(00)00159-3
Baudron, A. R., Needle, C. L., Rijnsdorp, A. D., and Marshall, C. T. (2014). Warming temperatures and smaller body sizes: synchronous changes in growth of North Sea fishes. Glob. Chang. Biol. 20, 1023–1031. doi: 10.1111/gcb.12514
Beaugrand, G., Reid, P. C., Iba-ez, F., Lindley, J. A., and Edwards, M. (2002). Reorganization of North Atlantic Marine Copepod Biodiversity and climate. Science 296, 1692–1694. doi: 10.1126/science.1071329
Benson, B. B., and Krause, D. Jr. (1984). The concentration and isotopic fraction of oxygen dissolved in freshwater and seawater in equilibrium with the atmosphere. Limnol. Oceanogr. 29, 620–632. doi: 10.4319/lo.1984.29.3.0620
Bergstad, O. A., Høines, Å. S., and Krüger-Johnsen, E. M. (2001). Spawning time, age and size at maturity, and fecundity of sandeel, Ammodytes marinus, in the north-eastern North Sea and in unfished coastal waters off Norway. Aquat. Living Resour. 14, 293–301. doi: 10.1016/S0990-7440(01)01134-2
Biro, P. A., Morton, A. E., Post, J. R., and Parkinson, E. A. (2004). Over-winter lipid depletion and mortality of age-0 rainbow trout (Oncorhynchus mykiss). Can. J. Fish. Aquat. Sci. 61, 1513–1519. doi: 10.1139/f04-083
Biro, P. A., Post, J. R., and Abrahams, M. V. (2005). Ontogeny of energy allocation reveals selective pressure promoting risk taking behaviour in young fish cohorts. Proc. R. Soc. Lond. B Biol. Sci. 272, 1443–1448. doi: 10.1098/rspb.2005.3096
Bresnan, E., Cook, K. B., Hughes, S. L., Hay, S. J., Smith, K., Walsham, P., et al. (2015). Seasonality of the plankton community at an east and west coast monitoring site in Scottish waters. J. Sea Res. 105, 16–29. doi: 10.1016/j.seares.2015.06.009
Broekhuizen, N., Gurney, W. S. C., Jones, A., and Bryant, A. D. (1994). Modelling compensatory growth. Funct. Ecol. 8, 770–782. doi: 10.2307/2390237
Buckley, L. J., Turner, S. I., Halavik, T. A., Smigielski, A. S., Drew, S. M., and Laurence, G. C. (1984). Effects of temperature and food availability on growth, survival, and RNA-DNA ratio of larval sand lance (Ammodytes americanus). Mar. Ecol. Prog. Ser. 15, 91–97. doi: 10.3354/meps015091
Christensen, A., Butenschön, M., Gürkan, Z., and Allen, I. J. (2013). Towards an integrated forecasting system for fisheries on habitat-bound stocks. Ocean Sci. 9, 261–279. doi: 10.5194/os-9-261-2013
Clarke, A., and Johnston, N. M. (1999). Scaling of metabolic rate with body mass and temperature in teleost fish. J. Anim. Ecol. 68, 893–905. doi: 10.1046/j.1365-2656.1999.00337.x
Cook, R. M. (2004). Estimation of the age-specific rate of natural mortality for Shetland sandeels. ICES J. Mar. Sci. 61, 159–164. doi: 10.1016/j.icesjms.2003.11.005
Drinkwater, K. F., Belgrano, A., Borja, A., Conversi, A., Edwards, M., Greene, C. H., et al. (2003). The response of marine ecosystems to climate variability associated with the North Atlantic Oscillation. Geophys. Monogr. Ser. 134, 211–234. doi: 10.1029/134GM10
Edwards, M., Heath, M., and McQuatters-Gollop, A. (2010). “Plankton,” in MCCIP Annual Report Card 2010-2011, MCCIP Science Review, 10.
Eliasen, K., Reinert, J., Gaard, E., Hansen, B., Jacobsen, J. A., Grønkjær, P., et al. (2011). Sandeel as a link between primary production and higher trophic levels on the Faroe shelf. Mar. Ecol. Prog. Ser. 438, 185–194. doi: 10.3354/meps09301
Engelhard, G. H., Van Der Kooij, J., Bell, E. D., Pinnegar, J. K., Blanchard, J. L., Mackinson, S., et al. (2008). Fishing mortality versus natural predation on diurnally migrating sandeels Ammodytes marinus. Mar. Ecol. Prog. Ser. 369, 213–227. doi: 10.3354/meps07575
Fransz, H. G., Colebrook, J. M., Gamble, J. C., and Krause, M. (1991). The zooplankton of the North Sea. J. Sea Res. 28, 1–52. doi: 10.1016/0077-7579(91)90003-J
Frederiksen, M., Edwards, M., Richardson, A. J., Halliday, N. C., and Wanless, S. (2006). From plankton to top predators: bottom-up control of a marine food web across four trophic levels. J. Anim. Ecol. 75, 1259–1268. doi: 10.1111/j.1365-2656.2006.01148.x
Frederiksen, M., Furness, R. W., and Wanless, S. (2007). Regional variation in the role of bottom-up and top-down processes in controlling sandeel abundance in the North Sea. Mar. Ecol. Prog. Ser. 337, 279–286. doi: 10.3354/meps337279
García, G. G., Demain, D., Drewery, H., and Gallego, A. (2012). Scottish Marine and Freshwater Science Report Vol 3 No 4 The Distribution of Zooplankton Prey of Forage Fish in The Firth of Forth Area. East Coast of Scotland.
Gilman, S. (1994). An energy budget for northern sand lance, Ammodytes dubius, on Georges bank, 1977–1986. Fish. Bull. 92, 647–654.
Goodlad, D., and Napier, I. (1997). Assessment of the Shetland Sandeel Fishery – 1996. Ph.D. thesis, Fisheries Development Note, North Atlantic Fisheries College, Scalloway.
Greenstreet, S., Fraser, H., Amrstrong, E., and Gibb, I. (2010). Monitoring the Consequences of the Northwestern North Sea Sandeel Fishery Closure. Vol. 1. Scottish Marine and Freshwater Science. Edinburgh: Scottish Government.
Greenstreet, S. P. R., McMillan, J. A., and Armstrong, E. (1998). Seasonal variation in the importance of pelagic fish in the diet of piscivorous fish in the Moray Firth, NE Scotland: a response to variation in prey abundance? ICES J. Mar. Sci. 55, 121–133.
Heath, M. R., Backhaus, J. O., Richardson, K., McKenzie, E., Slagstad, D., Beare, D., et al. (1999). Climate fluctuations and the spring invasion of the North Sea by Calanus finmarchicus. Fish. Oceanogr. 8, 163–176. doi: 10.1046/j.1365-2419.1999.00008.x
Heath, M. R., Neat, F. C., Pinnegar, J. K., Reid, D. G., Sims, D. W., and Wright, P. J. (2012b). Review of climate change impacts on marine fish and shellfish around the UK and Ireland. Aquat. Conserv. 22, 337–367. doi: 10.1002/aqc.2244
Heath, M. R., Rasmussen, J., Bailey, M. C., Dunn, J., Fraser, J., Gallego, A., et al. (2012a). Larval mortality rates and population dynamics of Lesser Sandeel (Ammodytes marinus) in the northwestern North Sea. J. Mar. Syst. 93, 47–57. doi: 10.1016/j.jmarsys.2011.08.010
Hislop, J., Harris, M., and Smith, J. (1991). Variation in the calorific value and total energy content of the lesser sandeel (Ammodytes marinus) and other fish preyed on by seabirds. J. Zool. 224, 501–517. doi: 10.1111/j.1469-7998.1991.tb06039.x
Hislop, J. R. G. (1997). Database Report of the Stomach Sampling Project 1991. International Council for the Exploration of the Sea, Cooperative Research Report No. 219.
Holland, G., Greenstreet, S., Gibb, I., Fraser, H., and Robertson, M. (2005). Identifying sandeel Ammodytes marinus sediment habitat preferences in the marine environment. Mar. Ecol. Prog. Ser. 303, 269–282. doi: 10.3354/meps303269
Howells, R. J., Burthe, S. J., Green, J. A., Harris, M. P., Newell, M. A., Butler, A., et al. (2017). From days to decades: short- and long-term variation in environmental conditions affect offspring diet composition of a marine top predator. Mar. Ecol. Prog. Ser. 583, 227–242. doi: 10.3354/meps12343
Hurst, T. P., and Conover, D. O. (2003). Seasonal and Interannual Variation in the allometry of energy allocation in juvenile striped bass. Ecology 84, 3360–3369. doi: 10.1890/02-0562
IPCC (2007). “Climate change 2007: the physical science basis,” in Contribution of Working Group I to the Fourth Assessment Report of the Intergovernmental Panel on Climate Change, eds S. Solomon, D. Qin, M. Manning, Z. Chen, M. Marquis, K. B. Averyt, M. H. Tignor, and L. Miller (Cambridge: Cambridge University Press), 996.
Jansen, T. (2016). First-year survival of North East Atlantic mackerel (Scomber scombrus) from 1998 to 2012 appears to be driven by availability of Calanus, a preferred copepod prey. Fish. Oceanogr. 25, 457–469. doi: 10.1111/fog.12165
Jensen, H., Rindorf, A., Wright, P. J., and Mosegaard, H. (2011). Inferring the location and scale of mixing between habitat areas of lesser sandeel through information from the fishery. ICES J. Mar. Sci. 68, 43–51. doi: 10.1093/icesjms/fsq154
Johnsen, E., Pedersen, R., and Ona, E. (2009). Size-dependent frequency response of sandeel schools. ICES J. Mar. Sci. 66, 1100–1105. doi: 10.1093/icesjms/fsp091
Jones, R. (1983). “The decline in herring and mackerel and the associated increase in other species in the North Sea,” in Proceedings of the Expert consultation to Examine Changes in Abundance and Species Composition of Neritic Fish Resources, eds G. D. Sharp and J. Csirke (Rome: FAO), 507–520.
Jones, W., Gurney, W. S. C., Speirs, D. C., Bacon, P. J., and Youngson, A. F. (2002). Seasonal patterns of growth, expenditure and assimilation in juvenile Atlantic salmon. J. Anim. Ecol. 71, 916–924. doi: 10.1046/j.1365-2656.2002.00679.x
Kirjasniemi, M., and Valtonen, T. (1997). Size-dependent over-winter mortality of young-of-the-year roach, Rutilus rutilus. Environ. Biol. Fishes 50, 451–456. doi: 10.1023/A:1007302931943
Kooijman, S. A. L. M. (2010). Dynamic Energy Budget Theory for Metabolic Organisation. Cambridge: Cambridge University Press.
Kooka, K., and Yamamura, O. (2012). Winter energy allocation and deficit of juvenile walleye pollock Theragra chalcogramma in the Doto area, northern Japan. Environ. Biol. Fishes 94, 389–402. doi: 10.1007/s10641-011-9957-1
Kvist, T., Gislason, H., and Thyregod, P. (2001). Sources of variation in the age composition of sandeel landings. ICES J. Mar. Sci. 58, 842–851. doi: 10.1006/jmsc.2001.1075
Lahoz-Monfort, J. J., Morgan, B. J. T., Harris, M. P., Wanless, S., and Freeman, S. N. (2011). A capture–recapture model for exploring multi-species synchrony in survival. Methods Ecol. Evol. 2, 116–124. doi: 10.1111/j.2041-210X.2010.00050.x
Lewis, S., Wanless, S., Wright, P. J., Harris, M. P., Bull, J., and Elston, D. A. (2001). Diet and breeding performance of black-legged kittiwakes Rissa tridactyla at a North Sea colony. Mar. Ecol. Prog. Ser. 221, 277–284. doi: 10.3354/meps221277
MacDonald, A. (2017). Modelling the Impact of Environmental Change on the Physiology and Ecology of Sandeels. Ph.D thesis. University of Strathclyde, Glasgow.
MacDonald, A., Heath, M. R., Edwards, M., Furness, R. W., Pinnegar, J. K., Wanless, S., et al. (2015). Climate- driven trophic cascades affecting seabirds around the British Isles. Oceanogr. Mar. Biol. Annu. Rev. 53, 55–80. doi: 10.1201/b18733-3
Macer, C. T. (1966). Sand eels (Ammodytidae) in the south-western North Sea; their biology and fishery. Fish. Invest. 24, 1–55.
McKinstry, C. A. E., Westgate, A. J., and Koopman, H. N. (2013). Annual variation in the nutritional value of stage V Calanus finmarchicus: implications for right whales and other copepod predators. Endanger. Species Res. 20, 195–204. doi: 10.3354/esr00497
Miller, C. B., Crain, J. A., and Morgan, C. A. (2000). Oil storage variability in Calanus finmarchicus. ICES J. Mar. Sci. 57, 1786–1799. doi: 10.1006/jmsc.2000.0975
O'Brien, T. D., Wiebe, P. H., and Falkenhaug, T. (Eds) (2013). ICES Zooplankton Status Report 2010/2011. ICES Cooperative Research Report No. 318. 208.
Oliver, J. D., Holeton, G. F., and Chua, K. E. (1979). Overwinter mortality of fingerling smallmouth bass in relation to size, relative energy stores, and environmental temperature. Trans. Am. Fish. Soc. 108, 130–136. doi: 10.1577/1548-8659(1979)108<130:OMOFSB>2.0.CO;2
Pedersen, S. A., Lewy, P., and Wright, P. (1999) Assessments of the lesser sandeel (Ammodytes marinus) in the North Sea based on revised stock divisions. Fish. Res. 41 221–241. doi: 10.1016/S0165-7836(99)00026-0
Pitois, S. G., Lynam, C. P., Jansen, T., Halliday, N., and Edwards, M. (2012). Bottom-up effects of climate on fish populations: data from the Continuous Plankton Recorder. Mar. Ecol. Prog. Ser. 456, 169–186. doi: 10.3354/meps09710
Post, J. R., and Parkinson, E. (2001). Energy allocation strategy in young fish: allometry and survival. Ecology 82, 1040–1051. doi: 10.1890/0012-9658(2001)082[1040:EASIYF]2.0.CO;2
Proctor, R., Wright, P. J., and Everitt, A. (1998). Modelling the transport of larval sandeels on the north-west European shelf. Fish. Oceanogr. 7, 347–354. doi: 10.1046/j.1365-2419.1998.00077.x
Quinn, T., and Schneider, D. E. (1991). Respiration of the teleost fish Ammodytes hexapterus in relation to its burrowing behavior. Comp. Biochem. Physiol.Part A Physiol. 98, 71–75. doi: 10.1016/0300-9629(91)90580-6
R Development Core Team (2014). R: A Language and Environment for Statistical Computing. Vienna: R Foundation for Statistical Computing. Available online at: http://www.R-project.org
Reay, P. J. (1970). Synopsis of biological data on North Atlantic sand eels of the genus Ammodytes (A. tobianus, A. dubius, A. americanus and A. marinus). FAO Fish. Synop. 82, 1–51.
Reay, P. J. (1973). Some aspects of the biology of the sandeel, Ammodytes tobianus L., in Langstone Harbour, Hampshire. J.Mar. Biol. Assoc. U. K. 53, 325–346. doi: 10.1017/S002531540002230X
Reeves, S. A. (1994). Seasonal and Annual Variation in Catchability of Sandeels at Shetland. Copenhagen: ICES CM. D:19.
Régnier, T., Gibb, F. M., and Wright, P. J. (2018). Temperature effects on egg development and larval condition in the lesser sandeel, Ammodytes marinus. J. Sea Res. 134, 34–41. doi: 10.1016/j.seares.2018.01.003
Reid, P. C., Borges, M. D. F., and Svendsen, E. (2001). A regime shift in the North Sea circa 1988 linked to changes in the North Sea horse mackerel fishery. Fish. Res. 50, 163–171. doi: 10.1016/S0165-7836(00)00249-6
Reid, P. C., Edwards, M., Beaugrand, G., Skogen, M., and Stevens, D. (2003). Periodic changes in the zooplankton of the North Sea during the twentieth century linked to oceanic inflow. Fish. Oceanogr. 12, 260–269. doi: 10.1046/j.1365-2419.2003.00252.x
Reid, P. C., Edwards, M., Hunt, H. G., and Warner, A. J. (1998). Phytoplankton change in the North Atlantic. Nature 391:546. doi: 10.1038/35290
Reygondeau, G., and Beaugrand, G. (2011). Future climate-driven shifts in distribution of Calanus finmarchicus. Glob. Chang. Biol. 17, 756–766. doi: 10.1111/j.1365-2486.2010.02310.x
Rindorf, A., Wright, P. J., Jensen, H., and Maar, M. (2016). Spatial differences in growth of lesser sandeel in the North Sea. J. Exp. Mar. Biol. Ecol. 479, 9–19. doi: 10.1016/j.jembe.2016.02.007
Ritzau Eigaard, O., van Deurs, M., Behrens, J. W., Bekkevold, D., Brander, K., Plambech, M., et al. (2014). Prey or predator - expanding the food web role of sandeel Ammodytes marinus. Mar. Ecol. Prog. Ser. 516, 267–273. doi: 10.3354/meps11064
Robards, M. D., Anthony, J. A., Rose, G. A., and Piatt, J. F. (1999). Changes in proximate composition and somatic energy content for Pacific sand lance (Ammodytes hexapterus) from Kachemak Bay, Alaska relative to maturity and season. J. Exp. Mar. Biol. Ecol. 242, 245–258. doi: 10.1016/S0022-0981(99)00102-1
Ryland, J. S. (1964). The feeding of plaice and sandeel larvae in the southern North Sea. J. Mar. Biol. Assoc. U. K. 44, 343–364. doi: 10.1017/S0025315400024875
Schultz, E. T., Conover, D. O., and Ehtisham, A. (1998). The dead of winter: size-dependent variation and genetic differences in seasonal mortality among Atlantic silverside (Atherinidae: Menidia menidia) from different latitudes. Can. J. Fish. Aquat. Sci. 55, 1149–1157. doi: 10.1139/f97-320
Tomiyama, M., and Yanagibashi, S. (2004). Effect of temperature, age class, and growth on induction of aestivation in Japanese sandeel (Ammodytes personatus) in Ise Bay, central Japan. Fish. Oceanogr. 13, 81–90. doi: 10.1046/j.1365-2419.2003.00272.x
van Deurs, M., Christensen, A., Frisk, C., and Mosegaard, H. (2010). Overwintering strategy of sandeel ecotypes from an energy/predation trade-off perspective. Mar. Ecol. Prog. Ser. 416, 201–214. doi: 10.3354/meps08763
van Deurs, M., Christensen, A., and Rindorf, A. (2013). Patchy zooplankton grazing and high energy conversion efficiency: ecological implications of sandeel behavior and strategy. Mar. Ecol. Prog. Ser. 487, 123–133. doi: 10.3354/meps10390
van Deurs, M., Hartvig, M., and Steffensen, J. F. (2011). Critical threshold size for overwintering sandeels (Ammodytes marinus). Mar. Biol. 158, 2755–2764. doi: 10.1007/s00227-011-1774-8
van Deurs, M., Jørgensen, C., and Fiksen, Ø. (2015). Effects of copepod size on fish growth: a model based on data for North Sea sandeel. Mar. Ecol. Prog. Ser. 520, 235–243. doi: 10.3354/meps11092
van Deurs, M., Koski, M., and Rindorf, A. (2014). Does copepod size determine food consumption of particulate feeding fish? ICES J. Mar. Sci. 71, 35–43. doi: 10.1093/icesjms/fst090
van Rijn, I., Buba, Y., DeLong, J., Kiflawi, M., and Belmaker, J. (2017). Large but uneven reduction in fish size across species in relation to changing sea temperatures. Glob. Chang. Biol. 23, 3667–3674. doi: 10.1111/gcb.13688
Wanless, S., Harris, M. P., and Greenstreet, S. P. R. (1998). Summer sandeel consumption by seabirds breeding in the Firth of Forth, south-east Scotland. ICES J. Mar. Sci. 55, 1141–1151. doi: 10.1006/jmsc.1998.0372
Wanless, S., Harris, M. P., Redman, P., and Speakman, J. R. (2005). Low energy values of fish as a probable cause of a major seabird breeding failure in the North Sea. Mar. Ecol. Prog. Ser. 294, 1–8. doi: 10.3354/meps294001
Wanless, S., Wright, P. J., Harris, M. P., and Elston, D. A. (2004). Evidence for decrease in size of lesser sandeels Ammodytes marinus in a North Sea aggregation over a 30-yr period. Mar. Ecol. Prog. Ser. 279, 237–246. doi: 10.3354/meps279237
Winslade, P. (1974). Behavioural studies on the lesser sandeel Ammodytes marinus (Raitt) III. The effect of temperature on activity and the environmental control of the annual cycle of activity. J. Fish Biol. 6, 587–599. doi: 10.1111/j.1095-8649.1974.tb05102.x
Keywords: growth, model, reserve, energy, A. marinus, copepod
Citation: MacDonald A, Speirs DC, Greenstreet SPR and Heath MR (2018) Exploring the Influence of Food and Temperature on North Sea Sandeels Using a New Dynamic Energy Budget Model. Front. Mar. Sci. 5:339. doi: 10.3389/fmars.2018.00339
Received: 03 July 2018; Accepted: 04 September 2018;
Published: 25 September 2018.
Edited by:
Lyne Morissette, M – Expertise Marine, CanadaReviewed by:
Khor Waiho, Shantou University, ChinaValeria Mamouridis, Independent Researcher, Rome, Italy
Copyright © 2018 MacDonald, Speirs, Greenstreet and Heath. This is an open-access article distributed under the terms of the Creative Commons Attribution License (CC BY). The use, distribution or reproduction in other forums is permitted, provided the original author(s) and the copyright owner(s) are credited and that the original publication in this journal is cited, in accordance with accepted academic practice. No use, distribution or reproduction is permitted which does not comply with these terms.
*Correspondence: Alan MacDonald, YWxhbi5tYWNkb25hbGRAc3RyYXRoLmFjLnVr