- 1Department of Orthopaedic Surgery, Mie University Graduate School of Medicine, Tsu, Japan
- 2Department of Pathology & Matrix Biology, Mie University Graduate School of Medicine, Tsu, Japan
Tenascin-C (TNC) is a large multimodular glycoprotein of the extracellular matrix that consists of four distinct domains. Emerging evidence suggests that TNC may be involved in the pathogenesis of osteoarthritis (OA) and rheumatoid arthritis (RA). In this review, we summarize the current understanding of the role of TNC in cartilage and in synovial biology, across both OA and RA. TNC is expressed in association with the development of articular cartilage; the expression decreases during maturation of chondrocytes and disappears almost completely in adult articular cartilage. TNC expression is increased in diseased cartilage, synovium, and synovial fluid in OA and RA. In addition, elevated circulating TNC levels have been detected in the blood of RA patients. Thus, TNC could be used as a novel biochemical marker for OA and RA, although it has no specificity as a biochemical marker for these joint disorders. In a post-traumatic OA model of aged joints, TNC deficiency was shown to enhance cartilage degeneration. Treatment with TNC domains results in different, domain-specific effects, which are also dose-dependent. For instance, some TNC fragments including the fibrinogen-like globe domain might function as endogenous inducers of synovitis and cartilage matrix degradation through binding with toll-like receptor-4, while full-length TNC promotes cartilage repair and prevents the development of OA without exacerbating synovitis. The TNC peptide TNIIIA2 also prevents cartilage degeneration without causing synovial inflammation. The clinical significance of TNC effects on cartilage and synovium is unclear and understanding the clinical significance of TNC is not straightforward.
Introduction
Osteoarthritis (OA) is a well-known cause of disability, with an estimated global prevalence of more than 30% (1). Further, its prevalence is increasing because of rapid population aging. Risk factors for OA include person factors (age, sex, obesity, and genetics) and joint factors (deformity, malalignment, and injury) that interact in a complex manner (2). Rheumatoid arthritis (RA) is a chronic inflammatory disease that can cause joint destruction as well as disability (3). The global prevalence of RA is around 1%, and genetics are the principal risk factor for developing RA, while smoking is the main environmental risk factor. RA is most typically found in elderly women (3, 4). The pathogenesis of RA and periodontitis might be similar, with both diseases involving chronic inflammation and bone erosion (5).
Tenascin-C (TNC) is a non-structural extracellular matrix (ECM) protein that is highly expressed in morphogenesis and tissue remodeling, and has many effects on cellular responses (6). Emerging evidence suggests that TNC might be involved in the pathogenesis of OA and RA. In this review, we present the current understanding of the role of TNC in cartilage and in synovial biology, across both OA and RA.
Structure and Distribution of Tenascin-C
TNC is a hexameric glycoprotein component of the ECM. The TNC molecule is composed of large molecular weight subunits (220–400 kDa) consisting of four distinct domains, with each TNC subunit consisting of a tenascin assembly (TA) domain that forms a coil at the N-terminus, 14.5 epidermal growth factor-like (EGF-L) repeats, up to 17 fibronectin type III (FNIII) -like repeats, and a C-terminal fibrinogen-like globe (FBG) domain. The FNIII-like repeats undergo alternative splicing to bind different ECM proteins, such as fibronectin, syndecan-4, and integrins αVβ3 and α8β1 (7–10). Furthermore, the FNIII-like repeats bind to a number of growth factors including fibroblast growth factor (FGF), platelet-derived growth factor (PDGF), and the transforming growth factor-β (TGF-β) family. The FBG domain binds to αVβ3 integrin and receptor-type tyrosine-protein phosphatase zeta, and activates toll-like receptor-4 (TLR4) (9, 11). TNC can drive a range of processes including cell migration, attachment, proliferation, and synthesis of proteases and proinflammatory cytokines (9). Growth factors, such as TGF-β, FGF, and PDGF, can induce TNC expression. TNC is transiently expressed in the mesenchyme around developing organs, such as mammary glands, teeth, and kidneys. TNC is also expressed during embryo development in cartilage, ligament, tendon, periosteum, myotendinous junction, smooth muscle, and perichondrium. Expression of TNC is generally low in adult tissues, but is transiently elevated following tissue injury. Once the damaged tissue is repaired, TNC expression is inhibited. TNC shares a structural relationship with fibronectin (12); although fibronectin is adhesive in nature while TNC is only weakly adhesive (13). Functional inhibition of syndecan-4 suppresses TNC activity. In contrast, overexpression of syndecan-4 neutralizes the effect of TNC. Thus TNC and syndecan-4 work together to control fibroblast signaling and morphology, and to regulate the contraction of the matrix, including tissue repair (14, 15). TNC expression is regulated by mechanical stress, and is elevated in tissues that experience high tensile stress, such as smooth muscle, ligaments, and tendons (16).
Cartilage
Articular cartilage is an aneural, avascular, alymphatic and viscoelastic tissue. It’s extremely low coefficient of friction contributes to the lubrication of joint movement. Water accounts for up to 85% of the wet weight of cartilage (17). By dry weight, the large aggregating proteoglycan aggrecan and type II collagen are the main ECM components of cartilage (18). Chondrocytes produce ECM with scant cell turnover (19). During the first stage of joint development, an interzone emerges at the presumptive joint site. Joint cavitation subsequently occurs at the center of the interzone, and cells within the interzone form the joint and cartilage (20). TNC participates in chondrogenesis and cartilage development (7, 8). At the early stage of articular cartilage formation the Indian hedgehog (Ihh), Erg, noggin, Wnt9a, and Gdf5 genes are expressed strongly. At the later stage, the expression of these early regulatory genes is downregulated, and the expression of structural genes, including proteoglycan (Prg) 4, type II collagen, CD44, and TNC, becomes preponderant (20, 21). In comparison, TGF-β, FGF18, and parathyroid hormone-related protein are continuously expressed during articular cartilage formation (20). In the newborn mouse knee, articular cartilage is a thin, dense tissue consisting of small, randomly oriented Prg4- and TNC-expressing cells (22). In mature cartilage, TNC is only present in the perichondrium (23), and disappears almost completely in adult articular cartilage (24). When the articular cartilage of TNC-knockout mice at a postnatal age of 8 weeks was compared to that of age-matched wild-type (WT) mice, the tangential/transitional zone was thicker and the density of chondrocytes was lower in WT mice than in the TNC-knockout mice. This observation in mice implies that TNC plays a role in increasing articular cartilage volume as well as producing ECM from birth to 2 months of age (25).
Cartilage Repair
Articular cartilage has a limited potential for repair and damaged cartilage is associated with the development of OA. Many strategies to repair cartilage have been proposed including bone marrow stimulation techniques, osteochondral graft, cell-based cartilage repair procedures, and the use of growth factors, such as TGF-β, bone morphogenetic protein-2, and FGF (26, 27). Autologous chondrocyte implantation offers great promise with good long-term results; however, its applicability for large defects is limited (28). Although local administration of growth factors could be easily implemented, this strategy has not been used in clinical trials (26). Notably, TNC appears to be capable of mediating repair in human OA cartilage in vitro (29). During in vivo cartilage repair, TNC expression was found at the early phase with expression disappearing at the late phase with cartilage maturation (30). In TNC-knockout BALB/c mice, cartilage repair was found to be significantly delayed compared to WT mice, and the deficiency of TNC accelerated degeneration of cartilage (30). When examining the effects of intra-articular TNC administration on the repair of full-thickness cartilage defects in rabbits using scaffolding matrices, full-length TNC (10 µg/mL) was found to promote the repair of cartilage in vivo (27). However, scaffold impregnated with a higher concentration (100 µg/mL) of TNC did not facilitate cartilage repair. In a BALB/c mouse model, full-length TNC (100 µg/mL) promoted cartilage repair in the absence of scaffold (31) (Table 1).
Osteoarthritis
Pathologic alterations in cartilage, bone, synovium, ligament, and meniscus are observed in OA, revealing OA to be a whole joint disease, with synovitis being one of the common features of OA. Synoviocytes synthesize hyaluronic acid and lubricin, which contribute to normal joint function (39). During progression of OA, the synovium can be a source of matrix metalloproteinase (MMP) and aggrecanase, which contributes to the degradation of the cartilage matrix. OA has historically been categorized as a non-inflammatory form of arthritis; however, the role of the development of synovitis in OA pathogenesis has been demonstrated (40–42). The synovium can produce soluble inflammatory mediators, including cytokines and chemokines, that are detected in joint tissues and synovial fluid in OA, and contribute to cartilage degeneration (40). Animal models of OA have been categorized into spontaneous and induced models, and the post-traumatic OA (PTOA) model is the most widely studied. Methods for inducing PTOA models include anterior cruciate ligament transection, medial collateral ligament transection, meniscectomy, and destabilization of the medial meniscus. In chemically induced models, sodium monoiodoacetate (MIA) is most commonly used to induce OA (43).
In clinical practice, the severity of OA is generally assessed with plain radiographs (44). Biochemical markers, however, provide an opportunity to better diagnose and stratify patients. Although cartilage oligomeric matrix protein in serum and C-terminal telopeptide of collagen type II in urine are the most widely investigated markers of tissue degradation, no marker has been well validated in clinical use for the diagnosis and monitoring of OA (39). It is not possible to determine the site of origin when biochemical markers are measured in serum or urine, but markers present in synovial fluid can provide insight into the damage in an individual joint (39). TNC levels in the synovial fluid were shown to be significantly increased in patients with knee OA (34, 45). In addition, TNC levels correlated with the radiographic grading levels (45), and TNC in the synovial fluid has been demonstrated to be a useful marker of OA progression (45). In a canine PTOA model, TNC levels increased markedly during the acute phase and then decreased over time, but remained elevated relative to the control group, even after 12 months (46).
Immunohistochemical analysis of TNC expression revealed that TNC staining intensity increased with the degeneration of cartilage in comparison with normal cartilage (29). TNC staining is observed on the OA cartilage surface overlying chondroitin sulfate (CS)-positive areas (29), and enhanced TNC staining is associated with clusters of chondrocytes (47). These results suggest that the distribution of TNC is correlated with CS production and chondrocyte proliferation in OA cartilage. In cultured human OA chondrocytes, treatment with TNC induced chondrocyte proliferation and increased aggrecan levels (29). Tumor necrosis factor (TNF)-α stimulates TNC expression through nuclear factor-κB signaling with RelA subunit activation, which could affect cell proliferation. TNC is reported to have a potential role in remodeling of cartilage (47); however, elevated levels of TNC could induce inflammatory mediators and promote degradation of matrix in OA cartilage (34). TNC plays dual roles in synovial fluid, where it not only acts as a marker of joint damage, but also stimulates joint degradation (46). These two opposing roles of TNC in synovial fluid may stem from the versatile nature of this glycoprotein (48). Full-length TNC prevented cartilage degeneration in a PTOA model using BALB/c mice (36). In contrast, recombinant TNC fragments induced aggrecanase activity and mediated cartilage degeneration. The EGF-L and FNIII-like domains 3–8 of TNC showed high aggrecan-degrading activity, which was not observed with either full-length TNC or other TNC domains (32) (Table 1, Figure 1). TNIIIA2 is a 22-mer peptide of TNC that induces β1 integrin activation through syndecan-4, and intra-articular injection of TNIIIA2 prevented degeneration of articular cartilage in a PTOA model using BALB/c mice (33). The inflammatory effects of TNC were identified through binding with TLR4, and integrins α9β1 and αVβ3 (49), but further work is required to clarify whether TNC contributes to OA pathogenesis via integrins. In cultured human and bovine chondrocytes, treatment with TNC upregulated interleukin (IL)-6, prostaglandin E2, nitrate release, and disintegrin and metalloproteinase with thrombospondin motifs-4 (34). Treatment with TNC decreased the amount of proteoglycan present in cartilage explants (32, 34). The FBG domain was reported to be an endogenous inducer of cartilage matrix degradation (32), while full-length TNC did not cause the severe inflammation observed with the FBG domain (27, 31, 36). Animal OA models using TNC-knockout mice have generated mixed results. Intra-articular injection of TNC induced synovitis in a TLR4-dependent manner using 129/SV mice (35) (Table 1). TLR4 activates the Fcγ receptor, and regulate the early onset of joint inflammation and cartilage damage during immune complex-mediated arthritis (50). TNC is not involved in the early onset of joint inflammation but is required for maintenance of inflammatory processes (35). The damage-associated molecular patterns CD14 ligand appears to contribute to cartilage repair in OA (51), although CD14 is a modulator of innate inflammatory signaling that acts as a receptor for bacterial lipopolysaccharide with TLR4 (52). There are reports of beneficial effects for joint tissues (31, 36) in BALB/c mice, while deleterious effects for joint tissues were found in a different mouse species (129/SV).
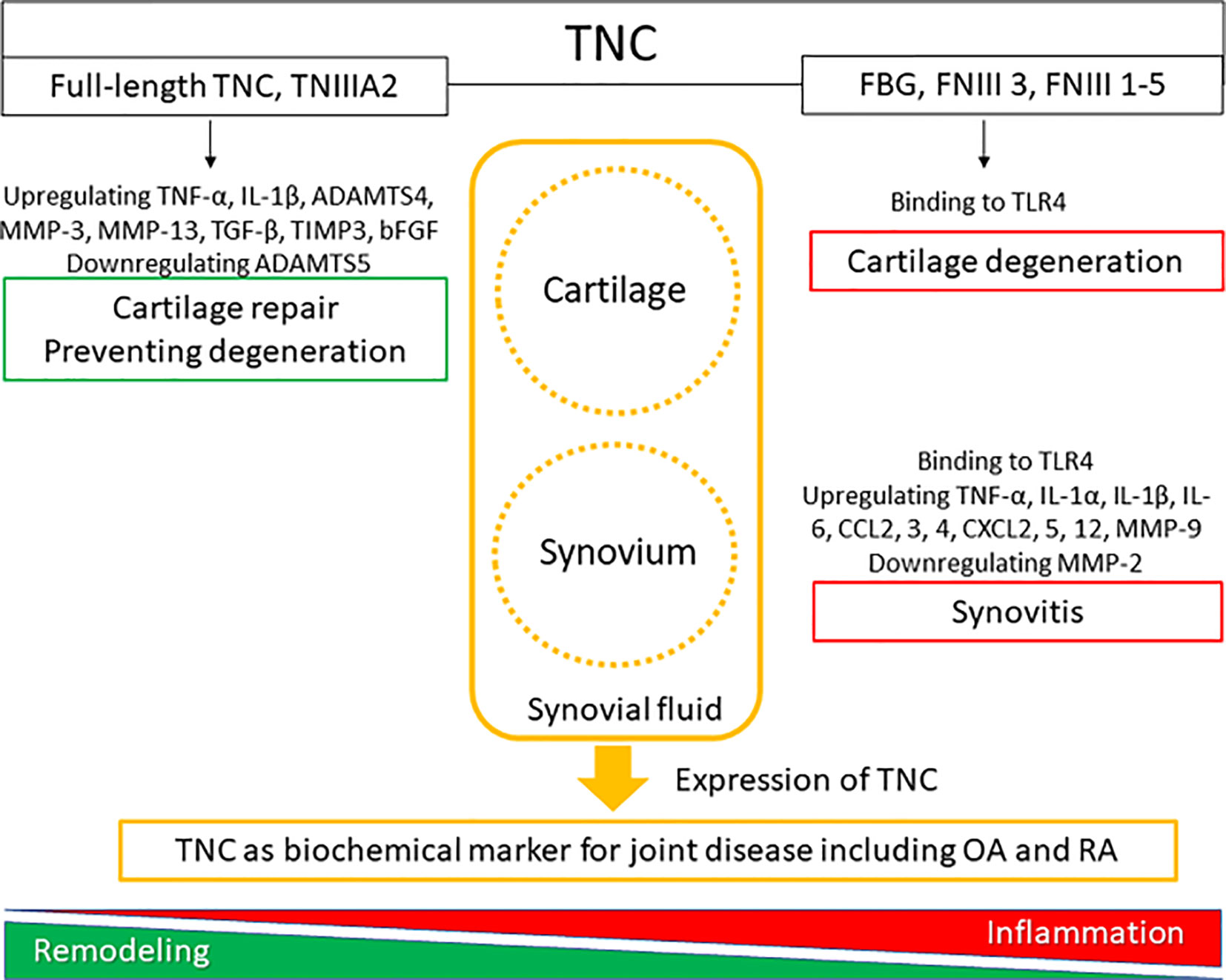
Figure 1 The roles of tenascin-C (TNC) in synovial joint biology. TNC expression is upregulated in degenerated cartilage and inflamed synovial tissue.
Genetic variations might play a role in the differential responses in cartilage and synovium. For example, the MRL/MpJ strain mouse has an impaired inflammatory response and no susceptibility to OA (53). Moreover, MRL/MpJ mice showed lower levels of IL-1 and higher levels of IL-4 and IL-10 compared with C57BL/6 mice in a PTOA model (53). STR/ort mice are highly susceptible to OA, while C57BL/6J mice are only moderately susceptible (54).
Rheumatoid Arthritis
RA is characterized by swelling, tenderness, and destruction of joints due to synovitis, inflammation, and autoantibodies, particularly to rheumatoid factor and citrullinated peptide, which can cause cartilage and bone damage and consequent disability (3, 4). The 2010 American College of Rheumatology/European League Against Rheumatism RA classification criteria are widely used for clinical diagnosis (55). The increased risk for RA in patients with the shared epitope is associated with seropositivity for autoantibodies against rheumatoid factor, which are autoantibodies against IgG and citrullinated peptide (ACPA). The inflammatory milieu in the synovial joint is regulated by a complex cytokine and chemokine network. TNF-α and IL-6 are essential to the process, whereas IL-1 and various chemokines may be less important (3, 56). Serum biomarkers are routinely used in monitoring of disease progression including erythrocyte sedimentation rate (ESR), C-reactive protein (CRP), and MMP-3 (57). Interaction of TNC and α9β1 integrin induced the expression of IL-6 and MMPs in synovial fibroblasts, and IL-1β and TNF-α in synovial macrophages (58).
Zymosan can be used to induce acute synovitis in 129/SV mice, where synovitis and cartilage proteoglycan loss were observed at 4 days in WT mice. In contrast, TNC-knockout mice did not exhibit any synovitis or loss of cartilage proteoglycan (35) (Table 1). TNC induced the synthesis of proinflammatory cytokines via endogenous activation of TLR4 (35). Using 129/SV mice, intra-articular injection of 1 or 3 µg FBG induced synovitis, inflammatory cell infiltration, pannus formation, and loss of cartilage proteoglycan; in contrast, 100 ng FBG injection did not induce any inflammation or proteoglycan loss (35) (Table 1). However, intra-articular administration of full-length TNC to BALB/c mice induced similar synovial inflammation compared to no TNC administration (31, 36). In a PTOA model using BALB/c mice, low-grade synovitis occurred at 2 weeks, but these changes improved at 4 weeks in mice with and without intra-articular injection of TNIIIA2 (33) (Table 1). While the FBG domain of TNC could be a critical driver of synovial inflammation in 129/SV mice, administration of full-length TNC and TNIIIA2 had no role in synovial inflammation in BALB/c mice (Figure 1). The capacity of TNC to exert both beneficial and deleterious effects on joint tissues is fascinating, and the mechanism underlying the dual nature of TNC remains poorly understood. Moreover, the dose of TNC administered appears to be an important factor in determining the in vivo effects of TNC.
TNC plays an important role in physiological tissue repair but also drives pathological inflammation and fibrosis (49). Intense TNC immunoreactivity was found in the RA synovium with strong chronic inflammation and fibrosis (59). Elevated circulating TNC levels have been detected in the blood of RA patients, and patients with late stage RA have higher TNC levels compared to those with early stage RA (60). TNC levels in blood and synovial fluid were not reported to correlate with CRP (59, 60). Moreover, blood levels of TNC did not correlate with other biomarkers, such as ESR and ACPA (60). However, TNC levels in blood correlated positively with erosion scores determined by ultrasound in early stage RA (60). TNC concentrations in synovial fluid were reported to be fourfold higher in RA compared with OA (59). Multiple citrullination sites exist in the FBG domain of TNC, and citrullinated TNC (cTNC) 5 was recognized as a biochemical marker that can be detected years before the onset of RA (61). Periodontitis is a risk factor for RA, and antibodies to cytokeratin 13 were found to correlate with anti-cTNC5 (62). The TN64 antibody is directed against the FNIII-like repeats 1-5 (TNfnIII 1-5) of TNC, and has been observed to prevent fibroblast-mediated cartilage destruction. The TN64 antibody was evaluated in collagen-induced arthritis in BALB/c mice, and TN64 was found to prevent the induction of arthritis by downregulation of TNF-α, IL-6, IL-10, IL-12, and IFN-γ (38). Both full-length TNC and the FBG domain induced synthesis of IL-6 in synovial fibroblasts (35). Mapping the active domain within TNC demonstrated a unique structural FBG epitope, essential for binding to and activating TLR4 (63). Monoclonal antibodies recognizing the FBG domain of TNC inhibited release of TNF-α, IL-6, and IL-8 by RA synovium (64). Blocking inflammatory signals from the ECM including TNC domains represents a potential novel therapeutic strategy for treating RA that may avoid global immune suppression.
It is not clear how the different domains in TNC interact with each other at a functional level and knowledge of different ligand binding modes of TNC is also limited (49). Moreover, it remains unclear whether activation of TLR4 by the FBG domain occurs in isolation from or in synergy with other TNC domains. Similarly, little is known about how tissue-specific responses to TNC are mediated (64). The clinical significance of TNC effects on cartilage and synovium is unclear and understanding the clinical significance of TNC is not straightforward, as it appears to contribute to both beneficial and deleterious effects in a context-dependent manner (65). However, TNC is clearly an important molecule involved in controlling cellular activity during tissue remodeling and inflammation (Figure 1). TNC does not have any specificity as a biochemical marker for OA and RA but has been shown to be a potential marker for other diseases. Elevated TNC expression was reported to predict poor prognosis among patients with various cancers, and TNC can be a serum biochemical marker for cancer (66). Serum TNC levels in patients with asthma was associated with clinical features of asthma, suggesting that serum TNC can be a biochemical for asthma (67). Circulating TNC levels were also elevated in patients with ankylosing spondylitis, systemic lupus erythematosus, psoriatic arthritis, ulcerative colitis, and Crohn’s disease (60, 68).
Conclusion
TNC is a key molecule in tissue remodeling and is associated with OA and RA. TNC could be used as a novel biochemical marker for OA and RA, although it has no specificity as a biochemical marker for these joint disorders. Administration of TNC showed both beneficial and deleterious effects across different joint tissues and different TNC domains, and it’s in vivo effects were clearly dose-dependent. Full-length TNC and TNIIIA2 prevented the development of OA in a PTOA model using BALB/c mice, suggesting that TNC domains and animal species might influence the type and nature of the responses obtained in pre-clinical studies. Thus, the clinical significance of TNC effects on cartilage and synovium awaits further clarification.
Author Contributions
MH and TY designed and reviewed the paper and contributed in drafting the manuscript. AS reviewed the manuscript. All authors contributed to the article and approved the submitted version.
Conflict of Interest
The authors declare that the research was conducted in the absence of any commercial or financial relationships that could be construed as a potential conflict of interest.
References
1. Vina ER, Kwoh CK. Epidemiology of osteoarthritis: literature update. Curr Opin Rheumatol (2018) 30:160–7. doi: 10.1097/BOR.0000000000000479
2. Palazzo C, Nguyen C, Lefevre-Colau MM, Rannou F, Poiraudeau S. Risk factors and burden of osteoarthritis. Ann Phys Rehabil Med (2016) 59:134–8. doi: 10.1016/j.rehab.2016.01.006
3. Smolen JS, Aletaha D, McInnes IB. Rheumatoid arthritis. Lancet (2016) 388:2023–38. doi: 10.1016/S0140-6736(16)30173-8
4. Scott DL, Wolfe F, Huizinga TW. Rheumatoid arthritis. Lancet (2010) 376:1094–108. doi: 10.1016/S0140-6736(10)60826-4
5. Potempa J, Mydel P, Koziel J. The case for periodontitis in the pathogenesis of rheumatoid arthritis. Nat Rev Rheumatol (2017) 13:606–20. doi: 10.1038/nrrheum.2017.132
6. Imanaka-Yoshida K, Aoki H. Tenascin-C and mechanotransduction in the development and diseases of cardiovascular system. Front Physiol (2014) 5:283. doi: 10.3389/fphys.2014.00283
7. Chiquet M, Fambrough DM. Chick myotendinous antigen. I. A monoclonal antibody as a marker for tendon and muscle morphogenesis. J Cell Biol (1984) 98:1926–36. doi: 10.1083/jcb.98.6.1926
8. Chiquet M, Fambrough DM. Chick myotendinous antigen. II. A novel extracellular glycoprotein complex consisting of large disulfide-linked subunits. J Cell Biol (1984) 98:1937–46. doi: 10.1083/jcb.98.6.1937
9. Giblin SP, Midwood KS. Tenascin-C: Form versus function. Cell Adh. Migr (2015) 9:48–82. doi: 10.4161/19336918.2014.987587
10. Tucker RP, Chiquet-Ehrismann R. Tenascin-C: Its functions as an integrin ligand. Int J Biochem Cell Biol (2015) 65:165–8. doi: 10.1016/j.biocel.2015.06.003
11. Yoshida T, Akatsuka T, Imanaka-Yoshida K. Tenascin-C and integrins in cancer. Cell Adh Migr (2015) 9:96–104. doi: 10.1080/19336918.2015.1008332
12. Halper J, Kjaer M. Basic components of connective tissues and extracellular matrix: elastin, fibrillin, fibulins, fibrinogen, fibronectin, laminin, tenascins and thrombospondins. Adv Exp Med Biol (2014) 802:31–47. doi: 10.1007/978-94-007-7893-1_3
13. Chiquet-Ehrismann R, Kalla P, Pearson CA, Beck K, Chiquet M. Tenascin interferes with fibronectin action. Cell (1988) 53:383–90. doi: 10.1016/0092-8674(88)90158-4
14. Huang W, Chiquet-Ehrismann R, Moyano JV, Garcia-Pardo A, Orend G. Interference of tenascin-C with syndecan-4 binding to fibronectin blocks cell adhesion and stimulates tumor cell proliferation. Cancer Res (2001) 61(23):8586–94.
15. Midwood KS, Valenick LV, Hsia HC, Schwarzbauer JE. Coregulation of fibronectin signaling and matrix contraction by tenascin-C and syndecan-4. Mol Biol Cell (2004) 15:5670–7. doi: 10.1091/mbc.e04-08-0759
16. Kreja L, Liedert A, Schlenker H, Brenner RE, Fiedler J, Friemert B, et al. Effects of mechanical strain on human mesenchymal stem cells and ligament fibroblasts in a textured poly(l-lactide) scaffold for ligament tissue engineering. J Mater Sci Mater Med (2012) 23:2575–82. doi: 10.1007/s10856-012-4710-7
17. Krishnan Y, Grodzinsky AJ. Cartilage diseases. Matrix Biol (2018) 71-72:51–69. doi: 10.1016/j.matbio.2018.05.005
18. Tian J, Zhang FJ, Lei GH. Role of integrins and their ligands in osteoarthritic cartilage. Rheumatol Int (2015) 35:787–98. doi: 10.1007/s00296-014-3137-5
19. Decker RS. Articular cartilage and joint development from embryogenesis to adulthood. Semin Cell Dev Biol (2017) 62:50–6. doi: 10.1016/j.semcdb.2016.10.005
20. Chijimatsu R, Saito T. Mechanisms of synovial joint and articular cartilage development. Cell Mol Life Sci (2019) 76:3939–52. doi: 10.1007/s00018-019-03191-5
21. Koyama E, Shibukawa Y, Nagayama M, Sugito H, Young B, Yuasa T, et al. A distinct cohort of progenitor cells participates in synovial joint and articular cartilage formation during mouse limb skeletogenesis. Dev Biol (2008) 316:62–73. doi: 10.1016/j.ydbio.2008.01.012
22. Decker RS, Um HB, Dyment NA, Cottingham N, Usami Y, Enomoto-Iwamoto M, et al. Cell origin, volume and arrangement are drivers of articular cartilage formation, morphogenesis and response to injury in mouse limbs. Dev Biol (2017) 426:56–68. doi: 10.1016/j.ydbio.2017.04.006
23. Mackie EJ, Thesleff I, Chiquet-Ehrismann R. Tenascin is associated with chondrogenic and osteogenic differentiation in vivo and promotes chondrogenesis in vitro. J Cell Biol (1987) 105:2569–79. doi: 10.1083/jcb.105.6.2569
24. Chevalier X, Groult N, Larget-Piet B, Zardi L, Hornebeck W. Tenascin distribution in articular cartilage from normal subjects and from patients with osteoarthritis and rheumatoid arthritis. Arthritis Rheumatol (1994) 37:1013–22. doi: 10.1002/art.1780370706
25. Gruber BL, Mienaltowski MJ, MacLeod JN, Schittny J, Kasper S, Flück M. Tenascin-C expression controls the maturation of articular cartilage in mice. BMC Res Notes (2020) 13:78. doi: 10.1186/s13104-020-4906-8
26. Goldberg A, Mitchell K, Soans J, Kim L, Zaidi R. The use of mesenchymal stem cells for cartilage repair and regeneration: a systematic review. J Orthop Surg Res (2017) 12:39. doi: 10.1186/s13018-017-0534-y
27. Ikemura S, Hasegawa M, Iino T, Miyamoto K, Imanaka-Yoshida K, Yoshida T, et al. Effect of tenascin-C on the repair of full-thickness osteochondral defects of articular cartilage in rabbits. J Orthop Res (2015r) 33:563–71. doi: 10.1002/jor.22794
28. Peterson L, Vasiliadis HS, Brittberg M, Lindahl A. Autologous chondrocyte implantation: a long-term follow-up. Am J Sports Med (2010) 38:1117–24. doi: 10.1177/0363546509357915
29. Nakoshi Y, Hasegawa M, Akeda K, Iino T, Sudo A, Yoshida T, et al. Distribution and role of tenascin-C in human osteoarthritic cartilage. J Orthop Sci (2010) 15:666–73. doi: 10.1007/s00776-010-1513-x
30. Okamura N, Hasegawa M, Nakoshi Y, Iino T, Sudo A, Imanaka- Yoshida K, et al. Deficiency of tenascin-C delays articular cartilage repair in mice. Osteoarthritis Cartilage (2010) 18:839–48. doi: 10.1016/j.joca.2009.08.013
31. Unno H, Hasegawa M, Suzuki Y, Iino T, Imanaka-Yoshida K, Yoshida T, et al. Tenascin-C promotes the repair of cartilage defects in mice. J Orthop Sci (2020) 25:324–30. doi: 10.1016/j.jos.2019.03.013
32. Sofat N, Robertson SD, Hermansson M, Jones J, Mitchell P, Wait R. Tenascin-C fragments are endogenous inducers of cartilage matrix degradation. Rheumatol Int (2012) 32:2809–17. doi: 10.1007/s00296-011-2067-8
33. Hattori T, Hasegawa M, Unno H, Iino T, Fukai F, Yoshida T, et al. TNIIIA2, The Peptide of Tenascin-C, as a Candidate for Preventing Articular Cartilage Degeneration. Cartilage (2020). doi: 10.1177/1947603520912300. Online ahead of print.
34. Patel L, Sun W, Glasson SS, Morris EA, Flannery CR, Chockalingam PS. Tenascin-C induces inflammatory mediators and matrix degradation in osteoarthritic cartilage. BMC Musculoskelet Disord (2011) 12:164. doi: 10.1186/1471-2474-12-164
35. Midwood K, Sacre S, Piccinini AM, Inglis J, Trebaul A, Chan E, et al. Tenascin-C is an endogenous activator of Toll-like receptor 4 that is essential for maintaining inflammation in arthritic joint disease. Nat Med (2009) 15:774–80. doi: 10.1038/nm.1987
36. Matsui Y, Hasegawa M, Iino T, Imanaka-Yoshida K, Yoshida T, Sudo A. Tenascin-C Prevents Articular cartilage degeneration in murine osteoarthritis models. Cartilage (2018) 9:80–8. doi: 10.1177/1947603516681134
37. Kanayama M, Kurotaki D, Morimoto J, Asano T, Matsui Y, Nakayama Y, et al. Alpha9 integrin and its ligands constitute critical joint microenvironments for development of autoimmune arthritis. J Immunol (2009) 182(12):8015–25. doi: 10.4049/jimmunol.0900725
38. Mehta BB, Tiwari A, Sharma S, Shukla A, Sharma M, Vasishta RK, et al. Amelioration of collagen antibody induced arthritis in mice by an antibody directed against the fibronectin type III repeats of tenascin-C: Targeting fibronectin type III repeats of tenascin-C in rheumatoid arthritis. Int Immunopharmacol (2018) 58:15–23. doi: 10.1016/j.intimp.2018.02.022
39. Glyn-Jones S, Palmer AJ, Agricola R, Price AJ, Vincent TL, Weinans H, et al. Osteoarthritis. Lancet (2015) 386:376–87. doi: 10.1016/S0140-6736(14)60802-3
40. Scanzello CR, Goldring SR. The role of synovitis in osteoarthritis pathogenesis. Bone (2012) 51:249–57. doi: 10.1016/j.bone.2012.02.012
41. Oehler S, Neureiter D, Meyer-Scholten C, Aigner T. Subtyping of osteoarthritic synoviopathy. Clin Exp Rheumatol (2002) 20:633–40.
42. Krenn V, Morawietz L, Burmester GR, Kinne RW, Mueller-Ladner U, Muller B, et al. Synovitis score: discrimination between chronic low-grade and high-grade synovitis. Histopathology (2006) 49:358–64. doi: 10.1111/j.1365-2559.2006.02508.x
43. Kuyinu EL, Narayanan G, Nair LS, Laurencin CT. Animal models of osteoarthritis: classification, update, and measurement of outcomes. J Orthop Surg Res (2016) 11:19. doi: 10.1186/s13018-016-0346-5
44. Kellgren JH, Lawrence JS. Radiological assessment of osteoarthrosis. Ann Rheum Dis (1957) 16:494–502. doi: 10.1136/ard.16.4.494
45. Hasegawa M, Hirata H, Sudo A, Kato K, Kawase D, Kinoshita N, et al. Tenascin-C concentration in synovial fluid correlates with radiographic progression of knee osteoarthritis. J Rheumatol (2004) 31:2021–6.
46. Chockalingam PS, Glasson SS, Lohmander LS. Tenascin-C levels in synovial fluid are elevated after injury to the human and canine joint and correlate with markers of inflammation and matrix degradation. Osteoarthritis Cartilage (2013) 21:339–45. doi: 10.1016/j.joca.2012.10.016
47. Nakoshi Y, Hasegawa M, Sudo A, Yoshida T, Uchida A. Regulation of tenascin-C expression by tumor necrosis factor-alpha in cultured human osteoarthritis chondrocytes. J Rheumatol (2008) 35:147–52.
48. Spring J, Beck K, Chiquet-Ehrisman R. Two contrary functions of tenascin: dissection of the active sites by recombinant tenascin fragments. Cell (1989) 59:325–34. doi: 10.1016/0092-8674(89)90294-8
49. Marzeda AM, Midwood KS. Internal Affairs: Tenascin-C as a Clinically Relevant, Endogenous Driver of Innate Immunity. J Histochem Cytochem (2018) 66:289–304. doi: 10.1369/0022155418757443
50. van Lent PL, Blom AB, Grevers L, Sloetjes A, van den Berg WB. Toll-like receptor 4 induced FcgammaR expression potentiates early onset of joint inflammation and cartilage destruction during immune complex arthritis: Toll-like receptor 4 largely regulates FcgammaR expression by interleukin 10. Ann Rheum Dis (2007) 66:334–40. doi: 10.1136/ard.2006.057471
51. Liu-Bryan R, Terkeltaub R. The growing array of innate inflammatory ignition switches in osteoarthritis. Arthritis Rheumatol (2012) 64:2055–8. doi: 10.1002/art.34492
52. Lévêque M, Simonin-Le Jeune K, Jouneau S, Moulis S, Desrues B, Belleguic C, et al. Soluble CD14 acts as a DAMP in human macrophages: origin and involvement in inflammatory cytokine/chemokine production. FASEB J (2017) 31:1891–902. doi: 10.1096/fj.201600772R
53. Ward BD, Furman BD, Huebner JL, Kraus VB, Guilak F, Olson SA. Absence of posttraumatic arthritis following intraarticular fracture in the MRL/MpJ mouse. Arthritis Rheumatol (2008) 58:744–53. doi: 10.1002/art.23288
54. Sebastian A, Chang JC, Mendez ME, Murugesh DK, Hatsell S, Economides AN, et al. Comparative Transcriptomics Identifies Novel Genes and Pathways Involved in Post-Traumatic Osteoarthritis Development and Progression. Int J Mol Sci (2018) 19:pii: E2657. doi: 10.3390/ijms19092657
55. Kay J, Upchurch KS. ACR/EULAR 2010 rheumatoid arthritis classification criteria. Rheumatol (Oxford) (2012) 51(Suppl 6):vi5–9. doi: 10.1093/rheumatology/kes279
56. Feldmann M, Maini SR. Role of cytokines in rheumatoid arthritis: an education in pathophysiology and therapeutics. Immunol Rev (2008) 223:7–19. doi: 10.1111/j.1600-065X.2008.00626.x
57. Miossec P, Verweij CL, Klareskog L, Pitzalis C, Barton A, Lekkerkerker F, et al. Biomarkers and personalised medicine in rheumatoid arthritis: a proposal for interactions between academia, industry and regulatory bodies. Ann Rheum Dis (2011) 70:1713–8. doi: 10.1136/ard.2011.154252
58. Asano T, Iwasaki N, Kon S, Kanayama M, Morimoto J, Minami A, et al. α9β1 integrin acts as a critical intrinsic regulator of human rheumatoid arthritis. Rheumatol (Oxford) (2014) 53:415–24. doi: 10.1093/rheumatology/ket371
59. Hasegawa M, Nakoshi Y, Muraki M, Sudo A, Kinoshita N, Yoshida T, et al. Expression of large tenascin-C splice variants in synovial fluid of patients with rheumatoid arthritis. J Orthop Res (2007) 25:563–8. doi: 10.1002/jor.20366
60. Page TH, Charles PJ, Piccinini AM, Nicolaidou V, Taylor PC, Midwood KS. Raised circulating tenascin-C in rheumatoid arthritis. Arthritis Res Ther (2012) 14:R260. doi: 10.1186/ar4105
61. Schwenzer A, Jiang X, Mikuls TR, Payne JB, Sayles HR, Quirke AM, et al. Identification of an immunodominant peptide from citrullinated tenascin-C as a major target for autoantibodies in rheumatoid arthritis. Ann Rheum Dis (2016) 75:1876–83. doi: 10.1136/annrheumdis-2015-208495
62. Schwenzer A, Quirke AM, Marzeda AM, Wong A, Montgomery AB, Sayles HR, et al. Association of distinct fine specificities of anti-citrullinated peptide antibodies with elevated immune responses to Prevotella intermedia in a Subgroup of patients with rheumatoid arthritis and periodontitis. Arthritis Rheumatol (2017) 69:2303–13. doi: 10.1002/art.40227
63. Zuliani-Alvarez L, Marzeda AM, Deligne C, Schwenzer A, McCann FE, Marsden BD, et al. Mapping tenascin-C interaction with toll-like receptor 4 reveals a new subset of endogenous inflammatory triggers. Nat Commun (2017) 8:1595. doi: 10.1038/s41467-017-01718-7
64. Aungier SR, Cartwright AJ, Schwenzer A, Marshall JL, Dyson MR, Slavny P, et al. Targeting early changes in the synovial microenvironment: a new class of immunomodulatory therapy? Ann Rheum Dis (2019) 78:186–91. doi: 10.1136/annrheumdis-2018-214294
65. Imanaka-Yoshida K, Yoshida T, Miyagawa-Tomita S. Tenascin-C in development and disease of blood vessels. Anat Rec (Hoboken) (2014) 297:1747–57. doi: 10.1002/ar.22985
66. Ming X, Qiu S, Liu X, Li S, Wang Y, Zhu M, et al. Prognostic Role of Tenascin-C for Cancer Outcome: A Meta-Analysis. Technol Cancer Res Treat (2019) 18. doi: 10.1177/1533033818821106
67. Yasuda M, Harada N, Harada S, Ishimori A, Katsura Y, Itoigawa Y, et al. Characterization of tenascin-C as a novel biomarker for asthma: utility of tenascin-C in combination with periostin or immunoglobulin E. Allergy Asthma Clin Immunol (2018) 14:72. doi: 10.1186/s13223-018-0300-7
Keywords: tenascin-C, osteoarthritis, rheumatoid arthritis, cartilage, repair, synovitis, animal model
Citation: Hasegawa M, Yoshida T and Sudo A (2020) Tenascin-C in Osteoarthritis and Rheumatoid Arthritis. Front. Immunol. 11:577015. doi: 10.3389/fimmu.2020.577015
Received: 28 June 2020; Accepted: 15 September 2020;
Published: 30 September 2020.
Edited by:
Kim Midwood, University of Oxford, United KingdomReviewed by:
Ali Mobasheri, State Research Institute Center for Innovative Medicine, LithuaniaYasser Mohamed El-Sherbiny, Nottingham Trent University, United Kingdom
Copyright © 2020 Hasegawa, Yoshida and Sudo. This is an open-access article distributed under the terms of the Creative Commons Attribution License (CC BY). The use, distribution or reproduction in other forums is permitted, provided the original author(s) and the copyright owner(s) are credited and that the original publication in this journal is cited, in accordance with accepted academic practice. No use, distribution or reproduction is permitted which does not comply with these terms.
*Correspondence: Masahiro Hasegawa, bWFzYWhhc2VAY2xpbi5tZWRpYy5taWUtdS5hYy5qcA==