- Respiratory Infections Section, St Mary’s Campus, National Heart and Lung Institute, Imperial College London, London, United Kingdom
Infections with respiratory syncytial virus (RSV) occurs repeatedly throughout life because sustained, protective memory responses fail to develop. Why this occurs is not known. During RSV infection the recognition of the virus via the cytosolic RIG-I like receptors and signaling via the adaptor protein MAVS is crucial for mounting an innate immune response. However, if this signaling pathway is important for T cell responses during primary infection and during re-infection is not fully elucidated. We describe a second peak of pro-inflammatory mediators during the primary immune response to RSV that coincides with the arrival of T cells into the lung. This second peak of cytokines/chemokines is regulated differently than the early peak and is largely independent of signaling via MAVS. This was concurrent with Mavs−/− mice mounting a strong T cell response to primary RSV infection, with robust IFN-γ; and Granzyme B production. However, after RSV re-infection, Mavs−/− mice showed fewer CD4+ and CD8+ short term memory T cells and their capacity to produce IFN-γ; and Granzyme B, was decreased. In sum, cytosolic recognition of RSV is important not only for initiating innate anti-viral responses but also for generating or maintaining efficient, short term T cell memory responses.
Introduction
Respiratory syncytial virus (RSV) is the most common cause of lower respiratory tract infections (LRTI) in children, resulting in bronchiolitis and viral pneumonia and often leading to hospitalization (1, 2). Currently, no vaccines or effective treatments against RSV replication and induced disease are available with a pressing need to understand the underlying mechanisms for development of disease and how to obtain protection against the infection. Innate immune responses are important for an efficient, early anti-viral response (2, 3). Type I interferons (IFNs) are essential mediators of the innate immune response and their protective role during RSV infection has been demonstrated in animal models (4–7). Importantly, RSV disease severity has been linked to polymorphisms in genes that control the IFN system (8–10). Alveolar macrophages (AMs) are the primary source of type I IFNs during RSV infection and these type I IFNs lead to both cell intrinsic viral control and cell extrinsic anti-viral effects (5). One of the major cell extrinsic effect of type I IFNs is to trigger CCL2-induced recruitment of inflammatory monocytes, which contribute to the control of the virus (5). RSV infection of mice deficient in retinoic acid inducible gene I receptors (RLR) adaptor protein mitochondrial antiviral-signaling protein (MAVS; Mavs−/−) revealed a severe deficiency in type I IFNs, pro-inflammatory cytokines and chemokines and inflammatory monocyte recruitment during RSV infection with associated increased illness and viral burden (5, 6, 11). Early anti-viral cytokines are not only important to contain virus replication and spread, they also play an essential role for the induction and regulation of protective adaptive immune responses (12). T cell responses are important for the final clearance of RSV. However, the T cells also contribute to illness and disease severity during RSV infection (2, 13, 14). In the absence of most innate immune responses several reports suggest that Mavs−/− mice are perfectly capable of inducing RSV-specific T cell responses (5, 6, 11). However, how viral sensing through the RLR-MAVS pathway influences short term T cell memory responses during RSV infection is not fully elucidated. Upon primary infection with a pathogen a population of long-lived memory T cells are maintained in the tissue and circulation in order to respond rapidly to potential secondary encounter with the same pathogen (15). In this study, we investigated the influence of innate immune responses on the initiation and effector function of T cells during the acute and memory phase of immune response to RSV infection. Following primary challenge with RSV, Mavs−/− mice displayed cytokine/chemokine production as well as infiltration of immune cells including an increased T cell recruitment into the lung tissue and airways with heightened T cell effector functions compared to wild type (wt) mice. However, after re-infection, Mavs−/− mice failed to mount a CD4+ or CD8+ T cell response to levels detected in wt mice. In addition, fewer of the memory T cells in the Mavs−/− mice produced Granzyme B and IFN-γ. Our study demonstrates a dependency on the early innate immune responses for the presence of RSV-specific memory T cells during RSV re-infection.
Materials and Methods
Mice
Wt mice and mice deficient in MAVS (Mavs−/−), obtained from S. Akira [World Premier International Immunology Frontier Research Center, Osaka University, Osaka, Japan, (16)] were bred and maintained in specific pathogen-free conditions. Both strains were Ifna6 gfp/+ but since Ifna6 expression was not a primary readout the mice are designated as wildtype (wt) and Mavs−/− mice. The mice were gender- and age-matched (7–12 weeks) in each experiment. All animal experiments were reviewed and approved by Animal Welfare and Ethical Review Board (AWERB) within Imperial College London and approved by the UK Home Office in accordance with the Animals (Scientific Procedures) Act 1986 and the ARRIVE guidelines were followed.
Virus and Infection
Plaque-purified human RSV (hRSV herein referred to as RSV) A2 (originally from ATCC) was grown in HEp-2 cells with DMEM supplemented with 2% fetal calf serum (FCS) and 2mM L-glutamine. Mice were lightly anesthetized and infected intranasally (i.n.) with 1.8x106 FFU of RSV in 100 μl.
Isolation of Cells From Airway (BAL) and Lung
Mice were sacrificed at different time points after infection. For some mice as indicated in figure legends, an intravenous injection of 2 μg anti-CD45-BUV394 (Biolegend, Cambridge, UK) in 200 μl was performed 10 min before euthanasia. Bronchoalveolar lavage (BAL) was performed by flushing the lungs 3 times with 1 ml phosphate-buffered saline (PBS) containing 0.5 mM EDTA (Life Technology, Paisley, UK). The obtained lavage was spun at 3,500 x g for 5 min to separate the BAL fluid from the cells. Lungs were perfused with PBS. To obtain single cell suspension, lungs were collected into C-Tubes (Milteny Biotech, Surrey, UK) containing complete DMEM (cDMEM; supplemented with 10% FCS, 2mM L-glutamine, 100 U/ml penicillin and 100 μg/ml streptomycin), 1 mg/ml Collagenase D (Roche, Welwyn Garden City, UK) and 30 μg/ml DNase I (Sigma Aldridge, Dorset UK) and processed with gentleMACS dissociator according to manufacturer’s protocol. After incubation for 30 min at 37°C the lungs were processed in the gentleMACS dissociator again. The red blood cells in BAL cells and lung single cell suspension were lysed using ACK buffer.
Flow Cytometry
Lung or BAL cells were incubated for 20 min at 4°C with purified rat IgG2b anti-mouse CD16/CD32 receptor antibody and then stained with fluorochrome-conjugated antibodies against CD11c (HL3, PE-CF594), CD11b (M1/70, AF700), CD45 (30-F11, eFluor780 or BV605), Ly6C (HK1.4, eFluor450), CD103 (2E7, PerCP-Cy5.5), Siglec-H (eBio440c, eFluor660), B220 (RA3-6B2, eFluor450), Siglec-F (E50-2450, PE), CD64 (X54-5/7.1, APC), Ly6G (1A8, BV570), CD3 (17A2, AF700), CD4 (GK1.5, PE), CD8 (53-6.7, eFluor780), CD19 (6D5, FITC), CD44 (IM7, PE-Cy7), and CD62L (MEL-14, BV421) in PBS containing 1% BSA, 5 mM EDTA and 0.05% NaN3 for 25 min at 4°C. This was followed by an incubation with fixable live-dead Aqua dye (Invitrogen, Paisly, UK) for 30 min at 4°C before fixing the cells with fixation buffer (Biolegend, Cambridge, UK). Alexa Fluor 647-conjugated M187-195 tetramers (H-2Db/NAITNAKII) were obtained from the NIH Tetramer Core Facility (Emory University Atlanta, GA, USA). M Tetramer staining was performed following Fc-block and prior to surface staining for 30 min at RT in PBS containing 1% BSA, 5 mM EDTA, and 0.05% NaN3.
For intracellular staining the cells were re-stimulated with 5 μg/ml RSV M187-195-peptide for 1 h at 37°C. After addition of Golgi Plug (BD Biosciences) the samples were incubated for another 3 h, stained for surface marker expression as described above and fixed in fixation buffer (Biolegend). Cells were stained with fluorochrome-conjugated antibodies against granzyme B (GB11, PE-CF594) and IFN-γ (XMG1.2, BV711) in the presence of purified rat IgG2b anti-mouse CD16/CD32 receptor antibody in permeabilization buffer (Biolgend) for 60 min. Samples were measured on a Becton Dickinson Fortessa LSR-SORP equipped with 20 mW 355 nm, 50 mW 405 nm, 50 mW 488 nm, 50 mW 561 nm, 20 mW 633 nm lasers and a ND1.0 filter in front of the FSC photodiode. Acquisition was set to 250,000 single, live cells. PMT voltages were adjusted after standardized CST checks minimizing the spectral overlap to increase data precision. All antibodies were purchased from BD, eBioscience or Biolegend. Data were analyzed using FlowJo software (Treestar, Ashland, OR, USA).
Chemokine, Cytokine, and RSV-Specific Ig Detection
BAL fluid was assessed using a granzyme B and IFN-γ ELISA kit (R&D Systems, Minneapolis, MN, USA) according to manufacturer’s instructions. Detection limits were 31 pg/ml and 16 pg/ml respectively.
To quantify RSV-specific IFN-γ levels from lung cells, 4x105 lungs cells/well were stimulated with either medium, RSV (MOI 1) or 5, 0.5, and 0.05 ng/ml RSV M187-195 (H-2Db/NAITNAKII) peptide or as control, 5 ng/ml RSV M282-90 (H-2Kd/SYIGSINNI) in cDMEM for 72 h at 37°C. Supernatants were assessed using the IFN-γ ELISA kit (R&D Systems) according to manufacturer’s instructions.
To quantify chemokines and cytokines in the lung tissue, lungs were homogenized using a tissue lyser (Qiagen, Crawley, UK) in PBS containing protease inhibitor according to manufacturer’s instructions (Roche). After spinning for 10 min at 10,000 × g supernatants were analyzed using a Magnetic Cytokine 20-plex panel for Luminex (Life Technologies) according to manufacturer’s instructions. The data were obtained from a Bioplex 200 system (Bio-Rad laboratories).
To quantify RSV-specific Ig, in serum and BAL, ELISA was used. Briefly, ELISA plates were coated with RSV or HEp-2 control antigens in the same concentration and incubated overnight at 4°C. After blocking with 1% BSA in PBS for 1 h at 37°C, serum or BAL fluid was added in serial dilutions. After a further incubation for 2 h at 37°C HRP-conjugated detection antibodies were added; anti-total Ig (0.25µg/ml, Bio-Rad, Hertfordshire, UK) or anti- IgA antibodies (0.5µg/ml, Bio-Rad). The plates were incubated for 2 h at 37°C before adding TMB substrate (Life-Tech) and after achieving proper color development, the reaction was stopped using 2 M H2SO4. The plates were analyzed using a spectrophotometry (SoftMax Pro v5.2; Molecular Devices) and optical density was read at 450 nm. The data are plotted as absorbance (Abs) 450 of RSV-specific Ig minus HEp-2 specific Ig.
RNA Isolation and Quantitative RT-PCR
Lungs were homogenized using a TissueLyser LT (Qiagen), and total RNA was extracted using RNeasy Mini kit including DNA removal as described by the manufacturer (Qiagen). 1 μg RNA was reverse transcribed using High Capacity RNA-to-cDNA kit (Applied Biosystems, Paisley, UK) according to manufacturer’s instructions. To quantify RNA levels in lung tissue, quantitative RT-PCR reaction for Tnfa, Ifng, and RSV L gene was performed using primers and probes as previously described (4) in QuantiTect Probe PCR Master Mix (Qiagen). For absolute quantification, the exact number of copies of the gene of interest was calculated using a plasmid standard, and the results were normalized to the housekeeping gene Gapdh (Applied Biosystems). The relative expression of Il1b, Il6, and Cxcl1 (all from Applied Biosystems) to the housekeeping gene Gapdh was determined. First, the ΔCt (Ct=cycle threshold) between the target gene and Gapdh for each sample was calculated, following the calculation of 2-ΔCt. Analysis was performed using 7500 Fast System SDS Software (Applied Biosystems).
Statistical Analysis
Statistical analysis of data was performed using GraphPad Prism 6 (GraphPad Software Inc., La Jolla, CA, USA). Two-group comparisons were performed using unpaired, two-tailed Student’s t test. One-way ANOVA with Tukey’s post hoc test was used to compare multiple groups. For all tests a value of P<0.05 was considered as significant. *p<0.05; **p<0.01; ***p<0.001; ND, not detectable.
Results
Inflammatory Cytokines and Recruitment of Innate Immune Cells During the Adaptive Response Is Independent of MAVS Signaling
RSV infections are characterized by the rapid (18–24 h following infection) and transient induction of early innate immune mediators such as type I IFNs, IL-6, and TNF-α (3, 5, 6, 17). The production of these cytokines are dependent on signaling via MAVS, as previously published (5, 6). To monitor cytokine responses during the later course of primary RSV infection, cytokines and chemokines were measured in lung samples of RSV infected wild type (wt) and Mavs−/− mice, starting 4 days post infection (p.i.) up to day 9 p.i. The relative gene expression of Tnfa, Il6, and Il1b, and the protein expression of chemokine CCL2 were all detected on days 5 to 7 p.i. in both wt and Mavs−/− mice (Figures 1A, B). Notably, this second peak of mediators (days 5–7 p.i.) was smaller than the first peak (18–24 h p.i.) identified in RSV infected wt mice [Figure 1 and (5, 6)]. The neutrophil chemoattractant CXCL1, was induced more prominently in Mavs−/− mice than wt mice during the second peak (days 4–5; Figure 1B). This differential expression of the various cytokines and chemokines raised the question about the dynamics of pulmonary innate cells during RSV disease. Therefore, lung cell populations were assessed by flow cytometry from day 4 up to day 9 post RSV infection. Gating was performed as previously described (5). Equivalent to the cytokine and chemokine responses the majority of the identified cell populations (CD103+ dendritic cells (DCs), plasmacytoid DCs (pDCs), inflammatory monocytes (CD64+ infMo) and neutrophils) showed recruitment into the lung of RSV infected wt and Mavs−/− mice from day 4 to day 9 p.i. (Figures 1C–G). CD11b+ DCs were recruited slightly later in Mavs−/− mice and compared to wt mice (Figure 1C). CD103+ DCs, pDCs and infMo were recruited in both Mavs−/− and wt mice but a slightly higher number of these cells was observed in Mavs−/− mice at the later time points compared to wt mice (Figures 1E, F). Neutrophil numbers in Mavs−/− mice were increased over baseline for most time points while wt mice showed neutrophil recruitment on day 8 p.i. (Figure 1G). In summary, late responses during RSV infection (days 4–9), including cytokine expression and immune cell recruitment depend on mechanisms other than MAVS signaling and are differently regulated than in the early response (days 0–3) where the cytokine and chemokine responses are mostly dependent on MAVS signaling (5).
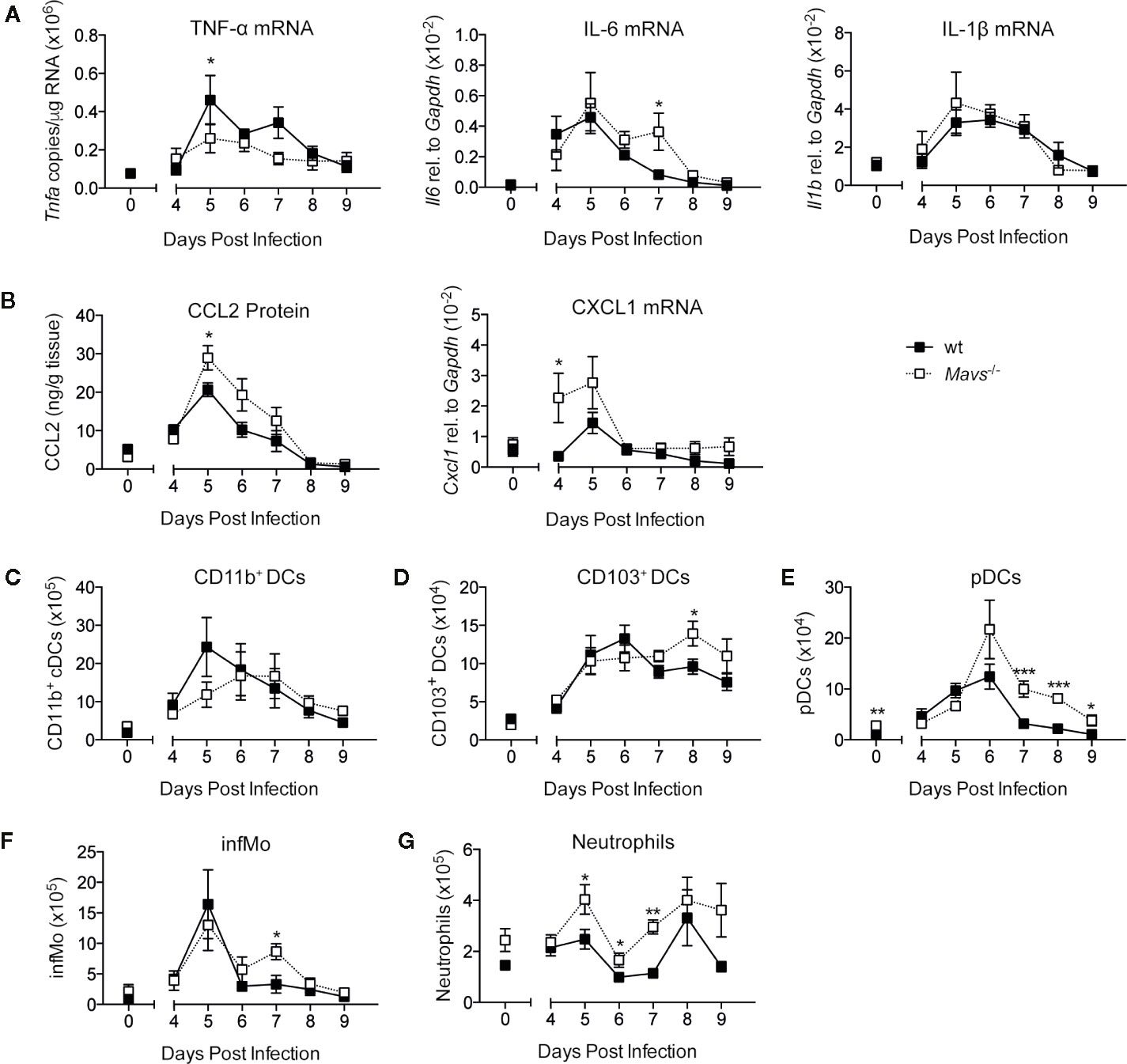
Figure 1 The induction of cytokines, chemokines and cell recruitment on days 4 to 9 of primary RSV infection. (A) Gene expression analysis of Tnfa, Il6, Il1b, and (B) CCL2 protein levels and Cxcl1 mRNA were determined at indicated time points in lung tissue from RSV-infected or mock infected (t=0) wt and Mavs−/− mice. Total numbers of (C) CD11b+ DCs, (D) CD103+ DCs, (E) plasmacytoid DCs (pDCs), (F) inflammatory monocytes (infMo), and (G) neutrophils in the lungs were determined by flow cytometry at indicated time points. Data are represented as mean ± SEM. Data in (A, B) are pooled from two independent experiments, n = 5–10. Data in (C–G) are representative of at least two experiments with four to five mice per group. Statistical significances of differences between indicated genotypes at each time point was determined by unpaired Student’s t test. *P < 0.05; **P < 0.01; ***P < 0.001.
T Cells Are Increased in the Lung of Mavs−/− Mice During Primary RSV Infection
The induction of appropriate adaptive immune responses and the recruitment of RSV-specific T cells play an essential role in viral clearance, regulating inflammatory responses and disease pathology (2, 13). In previous reports we and others have shown that wt and Mavs−/− mice recruit CD4+ and CD8+ T cells into the lung (5, 6, 11). A quantitative and qualitative analysis of differences in airway and lung T cells during experimental RSV infection and the possible impact of innate immune responses remained elusive. Time course analysis (days 4–9) in the RSV infection model revealed an induction of IFN-γ mRNA from day 5 p.i. in lungs from both wt and Mavs−/− mice albeit a more prominent increase was seen in wt mice at day 5 p.i. (Figure 2A). T cells are recruited into inflamed tissue by chemokines such as CXCL9 or CXCL10, which are induced by IFNs (18). Both CXCL9 and CXCL10 protein was detected in the lungs of wt and Mavs−/− mice and the levels of these chemokines were slightly higher at days 7 to 9 in the Mavs−/− mice compared to wt mice (Figure 2B). For analysis of T cell responses during RSV infection, wt and Mavs−/− mice were sacrificed on day 8 p.i., representing the peak of the T cells response (19, 20). Cells from the lungs and the airways (bronchoalveolar lavage; BAL) were isolated and stained for cell surface markers to phenotypically distinguish different T cell subsets (gating shown in Supplemental Figure S1A). Numbers of total CD4+ and CD8+ T cells were significantly increased in the lung tissue of Mavs−/− mice compared to wt mice (Figure 2C). However, the recruitment of T cells into the airways showed no differences between Mavs−/− and wt mice (Figure 2D). RSV-specific CD8+ T cells, determined by tetramer staining (gating shown in Supplemental Figure S1B) were represented in equal percentages and numbers (Figures 2E, F) in the lung tissue of wt and Mavs−/− mice, consistent with previous reports from our group and others (5, 6). However, viral clearance was delayed in Mavs−/− mice with more RSV L gene copies detected at day 8 p.i. [Figure 2G (5, 11)]. This is most likely a result of the absence of a complete innate immune response (5, 6, 11) and an increased viral load might contribute to the heightened recruitment of CD4+ and CD8+ T cells observed in Mavs−/− mice.
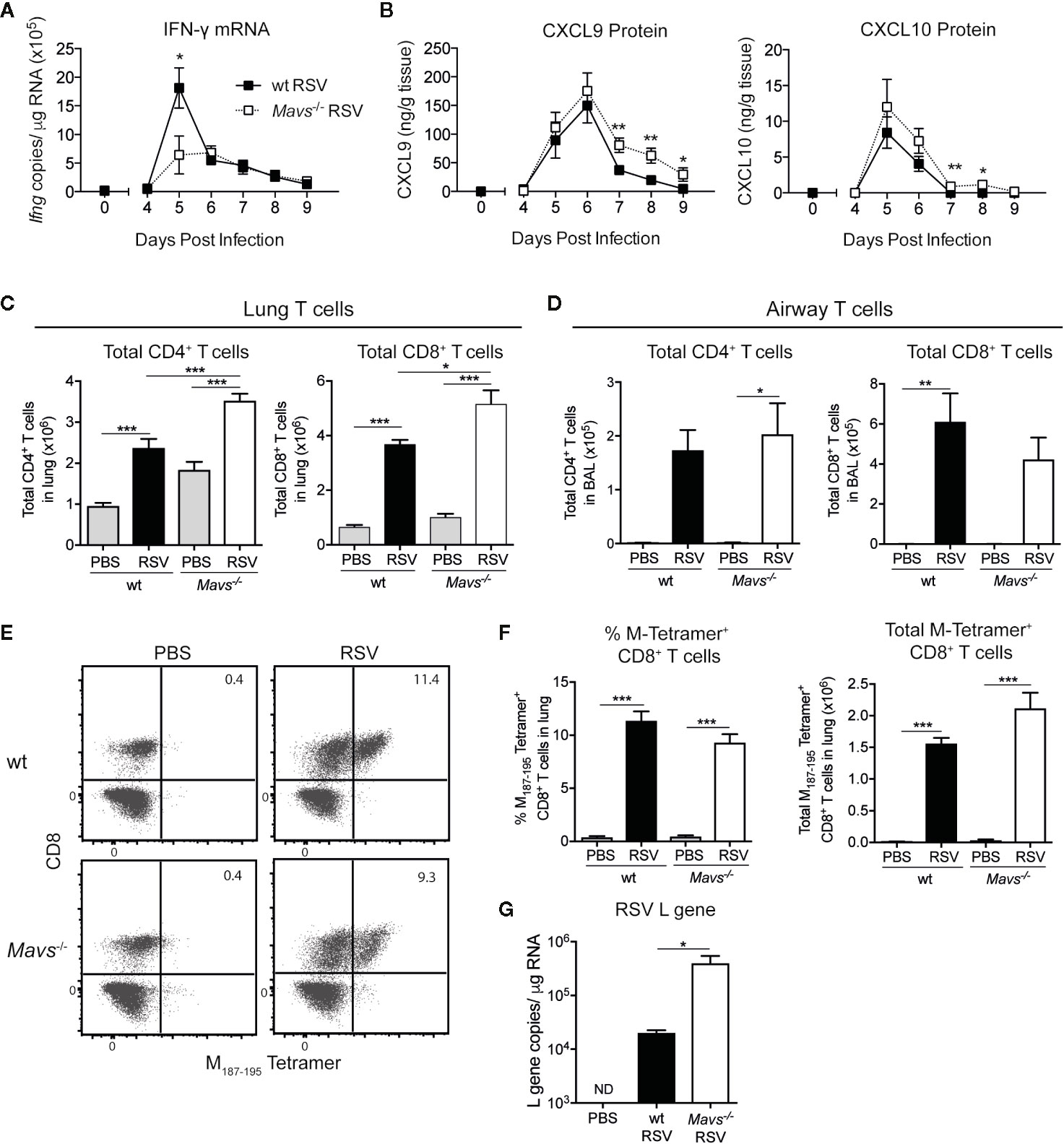
Figure 2 More CD8+ and CD4+ T cells are detected in lungs of Mavs−/− mice compared to wt mice during primary RSV infection. (A) Gene expression analysis of Ifng was determined by quantitative PCR at indicated time points in lung tissue from RSV-infected or mock-infected (t=0) wt and Mavs−/− mice. (B) CXCL9 and CXCL10 protein levels were determined by Luminex of lung homogenate at indicated time points. (C) Total numbers of CD4+ and CD8+ T cells in the lung tissue and (D) airways of RSV infected or mock-infected (PBS) wt and Mavs−/− mice, on day 8 p.i. (E) Representative plots of lung tissue M187-195 tetramer positive CD8+ T cells in wt and Mavs−/− mice, on day 8 p.i. Numbers in quadrants represent the percentage of M187-195 tetramer positive CD3+CD8+ T cells in total lung. (F) Quantification of frequencies and total M187-195 tetramer positive CD8+ T cells in lungs, on day 8 p.i. (G) Gene expression analysis of RSV L gene in lung tissue was determined by quantitative PCR, on day 8 p.i. Data are represented as mean ± SEM and pooled from two independent experiments, n = 5–10). In (A, B) statistical significances of differences between indicated genotypes at each time point was determined by unpaired Student’s t test. In (C, D, F, G) statistical significances of differences between groups was determined by one-way ANOVA with Tukey’s post hoc test. *P < 0.05; **P < 0.01; ***P < 0.001; ND, not detectable.
Expression of GzmB in CD8+ T Cells Is Enhanced in Mavs−/− Mice During Primary Infection With RSV
During acute viral infection, effector CD8+ T cells engage several mechanisms that mediate killing of infected cells and influence antiviral immune responses. For example, the production of cytotoxins, such as granzyme B (GzmB) and perforin, or the expression of surface proteins, like FasL induces programmed-cell death in target cells (14). Additionally, the release of IFN-γ and TNF−α have pleiotropic and immune stimulatory effects (21, 22). We assessed the effector function of lung and airway CD8+ T cells after primary encounter with RSV by stimulating them ex vivo with RSV M187-195 peptide and evaluated their capacity to produce GzmB and IFN-γ, or both, by intracellular staining and flow cytometry analysis (gating shown in Supplemental Figure S1B). In the airway and lungs of Mavs−/− mice, 40% to 50% of CD8+ T cells expressed GzmB, compared to wt mice where 30% to 40% of CD8+ T cells stained positive for GzmB (Figures 3A, B). Also, total numbers of CD8+ GzmB+ T cells in the lung tissue was significantly higher in Mavs−/− mice at day 8 during primary infection (Figure 3A). In terms of the capacity of CD8+ T cells to produce IFN-γ, wt and Mavs−/− mice displayed no significant differences (Figures 3A, B). The same accounted for multifunctional CD8+ T cells capable of producing both GzmB and IFN-γ (Supplemental Figures S2A, B). This suggested that Mavs−/− mice were able to recruit highly activated effector CD8+ T cells into the infection site and a large fraction of them were able to secrete effector mediators. Of note, the peptide stimulation did not allow for stimulation of CD4+ T cells, which could also be potent producers of IFN-γ. Furthermore, to detect GzmB and IFN-γ; levels in vivo, BAL fluids were analyzed. The overall GzmB and IFN-γ; responses were significantly increased in Mavs−/− mice at day 8 p.i. compared to wt mice (Figures 3C, D). Altogether, our data show that CD4+ and CD8+ T cell numbers and CD8+ T cell effector functions are elevated in the absence of MAVS signaling during primary RSV infection.
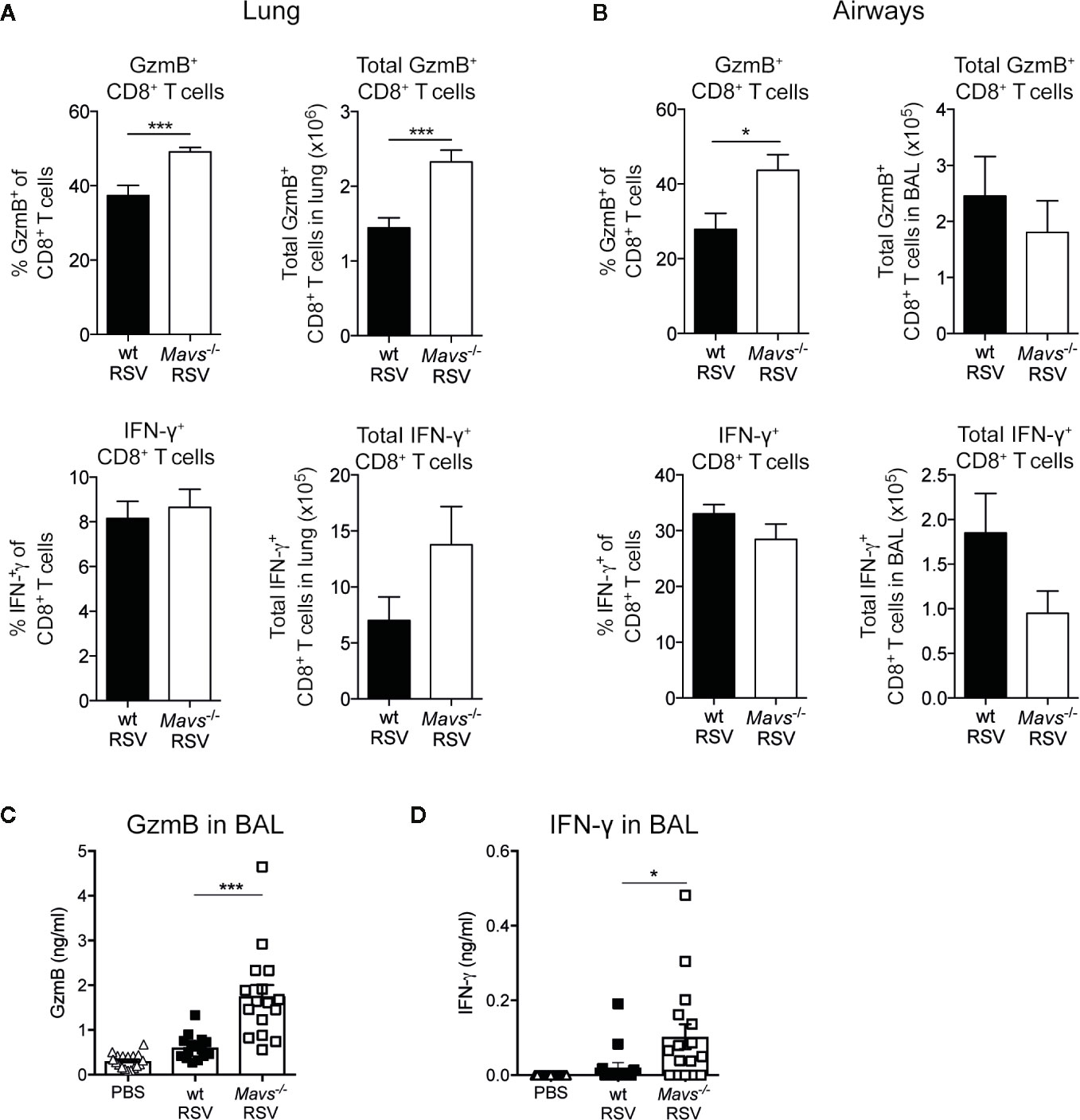
Figure 3 GzmB and IFN-γ producing T cells are increased in Mavs−/− mice during primary RSV infection. Percentage and total number of granzyme B (GzmB) and/or IFN-γ producing CD8+ T cells in (A) lung and (B) airways on day 8 in RSV-infected wt and Mavs−/− mice, was determined by flow cytometry. (C) GzmB and (D) IFN-γ levels in BAL fluid of RSV-infected and mock-infected (PBS) mice were determined by ELISA. Data are represented as mean ± SEM. Data in (A, B) are pooled from two independent experiments with four to five mice per group (n = 9–10). Statistical significances of differences between indicated genotypes at each time point was determined by unpaired Student’s t test. Data in (C, D) are pooled from three independent experiments with three to five mice per group (n = 14–15). Each symbol represents one individual mouse. Statistical significances of differences between groups were determined by one-way ANOVA with Tukey’s post hoc test. *P < 0.05; ***P < 0.001.
Fewer T Cell in the Lungs After RSV Re-Infection of Mavs−/− Mice
During secondary encounter with a pathogen memory T cells within the tissue or recruited from circulation are rapidly activated for defense of the host tissue (15). We hypothesized that the loss of innate immune activation in Mavs−/− mice (5, 6, 11) impacts on the quality of the short-term memory responses to RSV. The virus is cleared in both wt and Mavs−/− mice after 8 to 9 days post infection (6, 23) so to study short-term memory responses in Mavs−/− mice, we re-infected wt and Mavs−/− mice 21 days after primary infection with RSV and determined the T cell responses 4 days post re-challenge by flow cytometry (gating strategy see Supplemental Figure S1A). To note, in vivo labelling of CD45+ cells were performed in wt mice at day 4 post re-infection to distinguish T cells residing in the vasculature versus in the lung parenchyma and a majority (70–80%) of the CD4+ and CD8+ T cells were shown to reside in the lung parenchyma (Supplemental Figure 3).
Overall, lung CD4+ and CD8+ T cells were increased in RSV re-challenged mice compared to mice 25 days post RSV primary infection (Figure 4A). Between the re-challenged groups, the absolute numbers of CD4+ and CD8+ T cell in the lung tissue were higher in wt mice compared to Mavs−/− mice (Figure 4A). Although, the expansion/recruitment of T cells was similar in the airways of both re-infected strains (Figure 4B) and the T cell-attracting chemokine CXCL9 was detectable in the BAL fluid of both, wt and Mavs−/− mice. Albeit, Mavs−/− mice had a two- to three-fold higher level of CXCL9 than wt mice (Figure 4C).
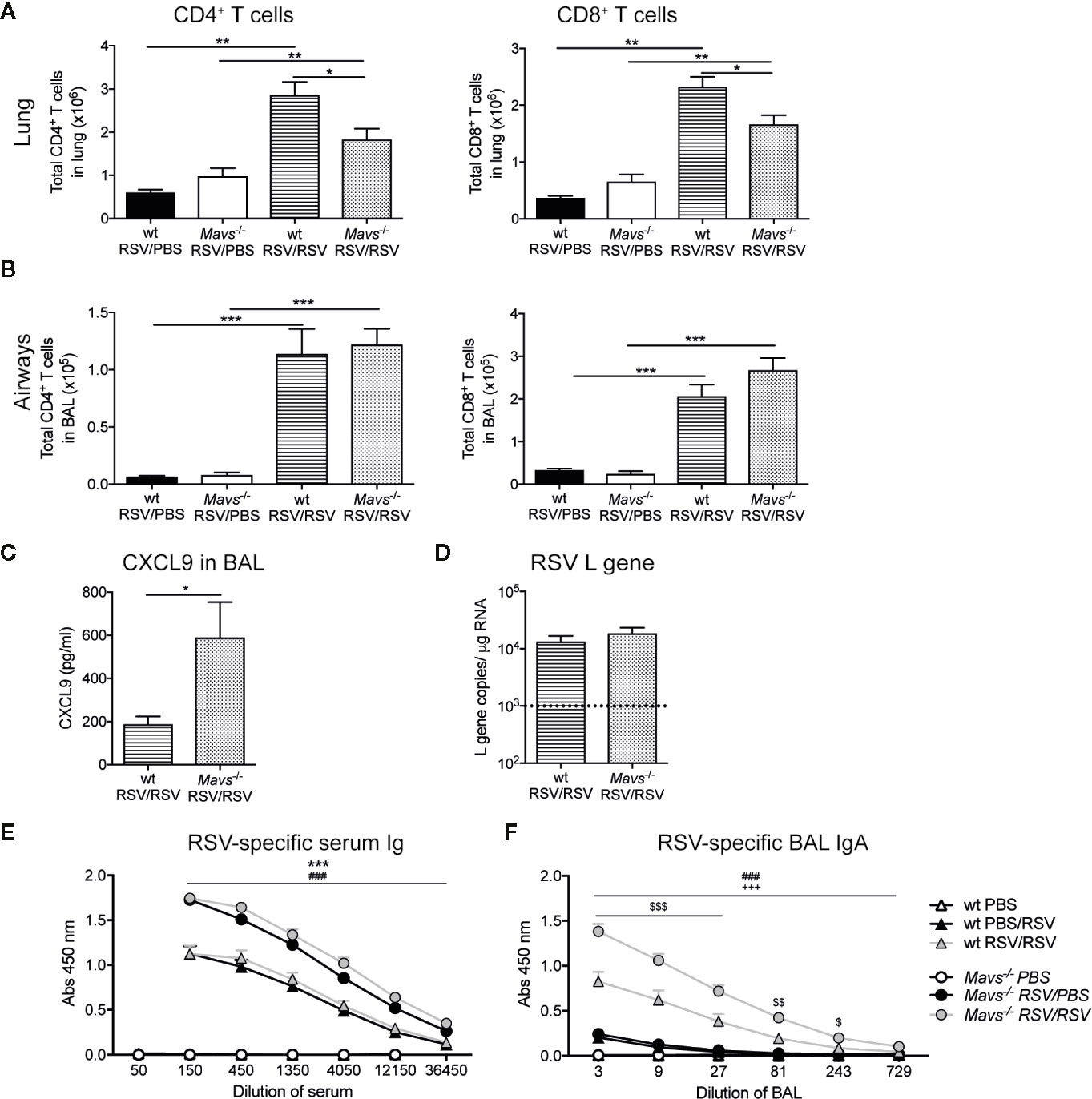
Figure 4 Reduced T cell numbers in the lung of Mavs−/− mice after re-infection with RSV. Wt and Mavs−/− mice were re-infected with RSV (RSV/RSV) or mock-infected (RSV/PBS) 21 days after primary RSV infection. Four days after secondary infection mice were sacrificed. Total number of CD4+ and CD8+ T cells in the (A) lung and (B) airways of wt and Mavs−/− mice were determined by flow cytometry. (C) Levels of CXCL9 in the BAL fluid of re-challenged mice were determined by Luminex analysis. (D) Gene expression analysis of RSV L gene in lung tissue was determined by quantitative PCR, on day 4 post RSV re-infection. Dotted line represents the detection limit. (E) RSV-specific serum Ig and (F) BAL IgA were determined by ELISA. (A–C) Data are represented as mean ± SEM and pooled from two independent experiments, n = 5–10. (D–F) Data are represented as mean ± SEM and pooled from three independent experiments with up to five mice per group (PBS n = 7–8 and RSV n = 14–15). Statistical significances of differences between groups were determined by (A, B) one-way ANOVA with Tukey’s post hoc test or (C, D) unpaired Student’s t test. (E, F) Statistical significance of differences between groups at defined dilutions was determined by one-way ANOVA with Turkey’s post hoc test. “#” represents statistical significance between wt RSV/RSV and Mavs−/− RSV/RSV mice, “*” represents statistical significance between wt RSV/PBS with Mavs−/− RSV/PBS mice, “$” represents statistical significance between wt RSV/RSV and wt RSV/PBS, “+” represents statistical significance between Mavs−/− RSV/RSV and Mavs−/− RSV/PBS mice. One symbol p ≤ 0.05; two symbols p ≤ 0.01, three symbols p ≤ 0.001.
To elucidate if this was due to an altered viral load, the number of RSV L gene copies was determined on day 4 after re-infection with RSV. The amount of detectable RSV L gene was low but no differences in lungs from wt and Mavs−/− mice were detected (Figure 4D). This is probably due to effective neutralization of RSV virions by RSV-specific antibodies (24) as both wt and Mavs−/− mice induce neutralizing RSV-specific antibodies. In our study, Mavs−/− mice showed higher levels of RSV-specific Ig compared to wt mice (Figures 4E, F) while a previous study showed an impaired RSV-specific Ig response in Mavs−/− mice (6). Overall, these data suggest a defect in the accumulation of lung memory T cells in the Mavs−/− mice.
Defect in Effector Function of RSV-Specific Memory CD8+ T Cells in Mavs−/− Mice During Secondary RSV Infection
To evaluate the RSV-specific CD8+ T cell response three strategies were employed. First, tetramer staining was performed to quantify the frequency and total number of RSV-specific CD8+ T cells. Mavs−/− mice had fewer lung M-tetramer positive cells compared to wt mice (Figure 5A). To confirm these results and quantify total CD8+ T cell responses, lung single-cell suspensions were re-stimulated with RSV M187-195 peptide at different concentrations (5, 0.5, and 0.05 ng/ml), control peptide RSV M282-90 (5 ng/ml) and RSV (MOI 1) for 3 days and the supernatants assessed for IFN-γ (Figure 5B). These data confirmed that Mavs−/− mice have fewer RSV-specific CD8+ T cells as their lung cells secreted less IFN-γ upon CD8+ T cell peptide stimulation than wt lung cells (Figure 5B).
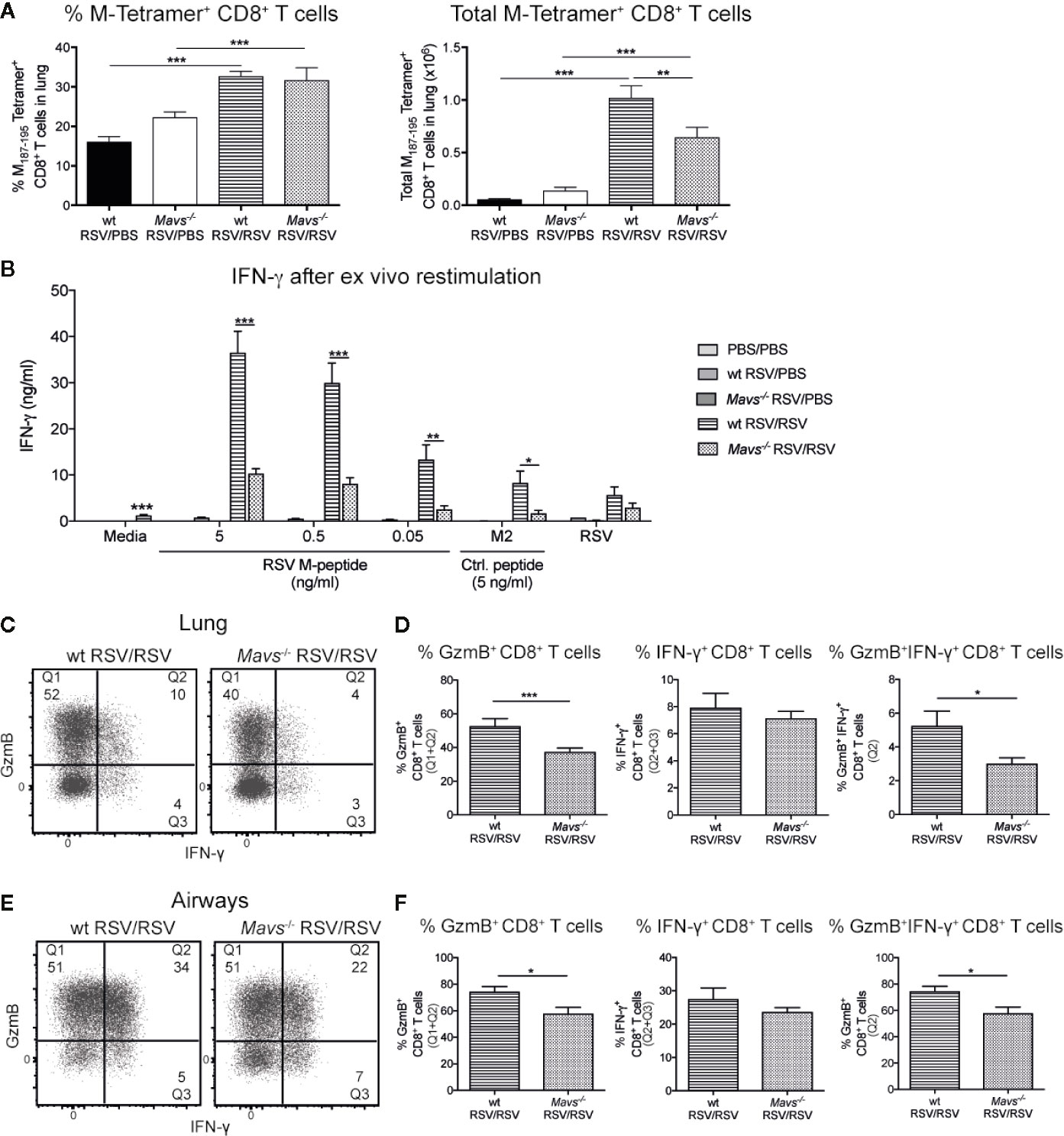
Figure 5 Less potent RSV-specific GzmB and IFN-γ/GzmB producing CD8+ T cells in Mavs−/− mice during secondary RSV infection. CD8+ T cells contribute significantly to IFN-γ; and Granzyme B (GzmB) production during secondary infection with RSV. (A) Quantification of frequencies and total M187-195 tetramer positive CD8+ T cells in lungs, on day 4 post RSV re-infection. (B) IFN-γ produced by lung cells after ex vivo stimulation with the immunodominant RSV peptide CD8+ T cell M187-195, the irrelevant peptide M282-90 as control (Ctrl) and RSV (MOI 1). IFN-γ protein levels were determined by ELISA after 72 h. (C) Representative flow cytometry plots of CD8+ T cells expressing GzmB and/or IFN-γ in the lung tissue of wt and Mavs−/− mice re-challenged with RSV (RSV/RSV). The frequencies of GzmB and/or IFN-γ producing cells of the CD8+ T cells in the lung are shown in the quadrants and quantified in (D). (E) Representative flow cytometry plots of CD8+ T cells expressing GzmB and/or IFN-γ in the airways of wt and Mavs−/− mice re-challenged with RSV (RSV/RSV). The numbers in the quadrants represent the frequencies of GzmB and/or IFN-γ producing cells of the CD8+ T cells in the airways and this is quantified in (F). Data are represented as mean ± SEM and pooled from two independent experiments with four to five mice per group (n = 5–10). In addition, in (B) each data point was determined as a mean of duplicate wells. Statistical significances of differences between groups were determined by unpaired Student’s t test. In (B) only difference between RSV re-infected groups are shown. *P < 0.05; **P < 0.01; ***P < 0.001.
We also speculated that, in addition to fewer RSV-specific CD8+ T cells, Mavs−/− mice additionally exhibit functional defects in the RSV-specific CD8+ T cell responses. After a short peptide restimulation, frequency and number of CD8+ GzmB+ and multifunctional CD8+ T cells (IFN-γ and GzmB) in both lung tissue and airways, were significantly reduced in Mavs−/− mice compared to wt mice (Figures 5C–F). In absolute numbers, a decrease in GzmB+ and GzmB+IFN-γ;+ cells were noted in the lung (Supplemental Figure 4). Taken together, our data show that numbers and full functionality of short-term memory CD8+ T cells are dependent on signaling via MAVS.
Discussion
RSV infection induces a strong innate immune response via the RLR-MAVS pathway with type I IFNs and subsequent IFNAR-signaling identified as central mediators of early pro-inflammatory responses and monocyte recruitment (4–6). However, the effects of the innate immune responses have on the subsequent T cell responses during RSV infection are not fully elucidated. Whether the lack of MAVS signaling influence the immune responses in the later stages of primary RSV infection or if MAVS signaling influence T cell responses during RSV infection was investigated in this study.
Type I IFN secretion comprises the imminent response triggered in infected cells with robust antiviral and immunomodulatory response to contain virus replication and spread (25). During RSV infection, type I IFNs derived from alveolar macrophages (AMs) regulate the early inflammatory and antiviral activity of different lung cell populations (4, 5, 17). This response is completely dependent on MAVS signaling since type I IFNs and many other early mediators are completely absent in Mavs−/− mice after RSV infection (5, 6). Also, deficiency in MAVS signaling and/or deficiency in type I IFNs receptor signaling promote susceptibility to RSV-induced disease (4, 5). However, the cytokine and chemokine expression in the lung of wt mice after infection with RSV is characterized by a bi-phasic induction resulting in two peaks of leukocyte infiltration and cytokines and chemokine secretion in the lungs (previously described (26–29) and confirmed in our study). A second peak (days 4–9 p.i.) was detected for the chemokines CCL2, CXCL1, CXCL9 and CXCL10, the pro-inflammatory cytokines TNF-α, IL-6, IL-1β, and IFN-γ; and DCs, CD64+ inflammatory monocytes and neutrophils. These observations resembles experimental RSV challenge studies in humans, where a transient induction of the neutrophil chemokine CXCL8 (mouse homologue CXCL1) shortly after inoculation was followed by an additional CXCL8 peak during RSV-induced illness and viral shedding (30). Additionally, IL-8, CCL2, CCL3, and CCL5 were elevated in nasal secretions during symptomatic illness (30). Importantly, this second wave of cytokine and chemokine gene upregulation with cellular infiltration was initiated by mechanism/s independent of the RLR-MAVS pathway. In the later stages of primary RSV infection, apoptotic epithelial cells and immune cells are markedly increased in the lungs (31). It is therefore possible that signaling of endosomal TLR3 and TLR7 in phagocytic cells that clear apoptotic cells containing viral antigen or cells expressing DAMP receptors become increasingly important.
Interestingly, Mavs−/− mice showed significant increased influx of CD103+ DCs, pDCs, infMo and neutrophils in the respiratory tract [our data and (11)]. Notably, the viral load in the lung of Mavs−/− mice was higher than in wt mice (5, 6, 11) and this could drive the increased recruitment of these cell types to the lung. Similarly, influx of monocytes and neutrophils was abundant in autopsy material from infants who died from RSV-induced LRTI (31).
The second peak of cytokines and cellular infiltration in the lung coincides with T cells infiltrating the lungs and also RSV-induced illness characterized by increased airway hyperresponsiveness, airway resistance and weight loss in mice (17, 26). In most infections the activation of the adaptive immune responses and their potent effector functions is required for the final elimination of invasive pathogens. It is commonly believed that the initiation of the adaptive immune system requires innate cytokines, such as type I IFNs (12). Accordingly, it was very surprising to discover similar or slightly increased T cell responses in Mavs−/− mice during primary infection [day 8 p.i.; data presented here and (5, 6, 11)]. There are several possible explanations for this. Firstly, the viral load in the lung of Mavs−/− mice is higher than in wt mice (5, 6, 11) and this could be driving stronger T cell responses. It is important to note that similar levels of RSV specific CD8+ T cells were detected in wt and Mavs−/− mice, which could suggest a heightened recruitment of CD8+ T cells with other TCR specificities. Second, a MAVS independent mechanism could drive the maturation and antigen presentation capacity of DCs. We have previously shown that mice deficient in MyD88/TRIF and MAVS can mount T cell responses albeit to a reduced level compared to wt mice (32). In addition, Bhoj et al. described a migratory DC subset in RSV-infected Mavs−/− mice suggested to be involved in cross-presentation of exogenous antigen to CD8+ T cells in the draining lymph nodes (6). A specific characterization of this particular DC subset and the specific molecular signals that lead to priming of T cells by DCs remain unclear.
After two to three weeks the majority of activated T cells undergo apoptosis and only a small pool of virus-specific memory T cells are maintained (15, 33). Upon secondary encounter with a pathogen, activation and clonal expansion of the memory T cell pool is crucial for a rapid host defense and survival. We show that activation and/or expansion of short-term memory cells was compromised in the lungs of mice lacking MAVS signaling, which led to a significant impairment in the production of the T cell effector molecules GzmB and IFN-γ. To note, this was observed in the lung but not in the airways. This was not due to a difference in viral load or RSV-specific antibody titers. Interestingly, the Mavs−/− mice showed higher RSV-specific Ig responses. The deficiency of short term memory T cells could be the result of a defect in the priming phase of the T cell response that leads to a problem in establishing or retaining a memory T cell pool. Alternatively, MAVS signaling could be necessary during the re-activation of memory T cell in the lungs during RSV secondary infection. To fully re-activate memory T cells, cognate and non-cognate signals (such as type I IFNs, IL-15, or IL-18) are required to rapidly acquire effector functions (34–36). Kohlmeier et al. showed that signaling of type I IFNs through the type I IFN receptor (IFNAR) on memory CD8+ T cells enhances GzmB production and cytolytic activity in recall responses to influenza virus (34). Therefore, it is possible that the memory T cells formed during primary RSV infection in Mavs−/− mice cannot be re-activated due to a lack of essential inflammatory cytokines. Also, the type of memory cells in the lung will be important to evaluate. Resident memory T cells have been shown to be important during RSV infection (37, 38) and the formation and function of these cells in MAVS deficient mice will be important to elucidate in future studies.
In conclusion, our work emphasizes a role for MAVS signaling beyond the initiation of early anti-viral responses that protect from RSV infection. In particular, we identified a critical role for the RLR-MAVS pathway in the numbers and effector functions of the short term memory T cell pool during secondary RSV infection. RSV elicits a short-lived memory response in humans and our data suggest that a weak innate response results in an impaired short-term T cell memory response, especially in the CD8+ T cells, which highlights the importance of a balanced immune response to achieve an efficient and long-lasting anti-viral immune response important both during infection and vaccination.
Data Availability Statement
All datasets presented in this study are included in the article/Supplementary Material.
Ethics Statement
The animal study was reviewed and approved by Imperial College AWERB.
Author Contributions
MiP designed, performed and analyzed the experiments and wrote the paper. NP, AV, and FK performed specific experiments and reviewed the paper. MaP helped in designing the flow cytometry panel. CJ supervised the project, designed and analyzed the experiments, and wrote the paper. All authors contributed to the article and approved the submitted version.
Funding
CJ was supported by a Career Development Award from the Medical Research Council (Grant G0800311) and a grant from the Rosetrees Trust and the Stoneygate Trust (M370). MiP was supported by a PhD Fellowship from the National Heart and Lung Institute Foundation (registered charity number 1048073). FK was supported by a Wellcome Trust grant (109058/Z/15/Z) and AV by European Respiratory Society and the Asociación Latinoamericana de Tórax joint long-term Research Fellowship 2019 (Number: LTRF 201901-00546).
Conflict of Interest
The authors declare that the research was conducted in the absence of any commercial or financial relationships that could be construed as a potential conflict of interest.
Acknowledgments
We thank S. Akira and Y. Kumagai (World Premier International Immunology Frontier Research Center, Osaka University, Osaka, Japan) for providing Mavs−/− mice. We thank the NIH Tetramer Core Facility for RSV M187-195 tetramer (H-2Db/NAITNAKII) and staff of the St Mary’s flow cytometry facility and the animal facility for their assistance.
Supplementary Material
The Supplementary Material for this article can be found online at: https://www.frontiersin.org/articles/10.3389/fimmu.2020.572747/full#supplementary-material
References
1. Russell CD, Unger SA, Walton M, Schwarze J. The Human Immune Response to Respiratory Syncytial Virus Infection. Clin Microbiol Rev (2017) 30:481–502. doi: 10.1128/CMR.00090-16
2. Openshaw PJM, Chiu C, Culley FJ, Johansson C. Protective and Harmful Immunity to RSV Infection. Annu Rev Immunol (2017) 35:501–32. doi: 10.1146/annurev-immunol-051116-052206
3. Johansson C. Respiratory syncytial virus infection: an innate perspective. F1000Res (2016) 5:2898. doi: 10.12688/f1000research.9637.1
4. Goritzka M, Durant LR, Pereira C, Salek-Ardakani S, Openshaw PJM, Johansson C. Interferon-α/β receptor signaling amplifies early pro-inflammatory cytokine production in the lung during Respiratory Syncytial Virus infection. J Virol (2014) 88:6128–36. doi: 10.1128/JVI.00333-14
5. Goritzka M, Makris S, Kausar F, Durant LR, Pereira C, Kumagai Y, et al. Alveolar macrophage-derived type I interferons orchestrate innate immunity to RSV through recruitment of antiviral monocytes. J Exp Med (2015) 212:699–714. doi: 10.1084/jem.20140825
6. Bhoj VG, Sun Q, Bhoj EJ, Somers C, Chen X, Torres JP, et al. MAVS and MyD88 are essential for innate immunity but not cytotoxic T lymphocyte response against respiratory syncytial virus. Proc Natl Acad Sci USA (2008) 105:14046–51. doi: 10.1073/pnas.0804717105
7. Guerrero-Plata A, Baron S, Poast JS, Adegboyega PA, Casola A, Garofalo RP. Activity and regulation of alpha interferon in respiratory syncytial virus and human metapneumovirus experimental infections. J Virol (2005) 79:10190–9. doi: 10.1128/JVI.79.16.10190-10199.2005
8. Janssen R, Bont L, Siezen CLE, Hodemaekers HM, Ermers MJ, Doornbos G, et al. Genetic susceptibility to respiratory syncytial virus bronchiolitis is predominantly associated with innate immune genes. J Infect Dis (2007) 196:826–34. doi: 10.1086/520886
9. Siezen CLE, Bont L, Hodemaekers HM, Ermers MJ, Doornbos G, Van’t Slot R, et al. Genetic susceptibility to respiratory syncytial virus bronchiolitis in preterm children is associated with airway remodeling genes and innate immune genes. Pediatr Infect Dis J (2009) 28:333–5. doi: 10.1097/INF.0b013e31818e2aa9
10. Tulic MK, Hurrelbrink RJ, Prêle CM, Laing IA, Upham JW, Le Souef P, et al. TLR4 polymorphisms mediate impaired responses to respiratory syncytial virus and lipopolysaccharide. J Immunol (2007) 179:132–40. doi: 10.4049/jimmunol.179.1.132
11. Demoor T, Petersen BC, Morris S, Mukherjee S, Ptaschinski C, De Almeida Nagata DE, et al. IPS-1 Signaling Has a Nonredundant Role in Mediating Antiviral Responses and the Clearance of Respiratory Syncytial Virus. J Immunol (2012) 189:5942–53. doi: 10.4049/jimmunol.1201763
12. Crouse J, Kalinke U, Oxenius A. Regulation of antiviral T cell responses by type I interferons. Nat Rev Immunol (2015) 15:231–42. doi: 10.1038/nri3806
13. Openshaw PJ, Chiu C. Protective and dysregulated T cell immunity in RSV infection. Curr Opin Virol (2013) 3:468–74. doi: 10.1016/j.coviro.2013.05.005
14. Chiu C, Openshaw PJ. Antiviral B cell and T cell immunity in the lungs. Nat Immunol (2015) 16:18–26. doi: 10.1038/ni.3056
15. Schenkel JM, Masopust D. Tissue-resident memory T cells. Immunity (2014) 41:886–97. doi: 10.1016/j.immuni.2014.12.007
16. Kumagai Y, Takeuchi O, Kato H, Kumar H, Matsui K, Morii E, et al. Alveolar macrophages are the primary interferon-alpha producer in pulmonary infection with RNA viruses. Immunity (2007) 27:240–52. doi: 10.1016/j.immuni.2007.07.013
17. Pribul PK, Harker J, Wang B, Wang H, Tregoning JS, Schwarze J, et al. Alveolar macrophages are a major determinant of early responses to viral lung infection but do not influence subsequent disease development. J Virol (2008) 82:4441–8. doi: 10.1128/JVI.02541-07
18. Sokol CL, Luster AD. The Chemokine System in Innate Immunity. Cold Spring Harb Perspect Biol (2015) 7(5):a016303. doi: 10.1101/cshperspect.a016303
19. Schmidt ME, Varga SM. The CD8 T Cell Response to Respiratory Virus Infections. Front Immunol (2018) 9:678. doi: 10.3389/fimmu.2018.00678
20. Chang J, Srikiatkhachorn A, Braciale TJ. Visualization and characterization of respiratory syncytial virus F-specific CD8(+) T cells during experimental virus infection. J Immunol (2001) 167:4254–60. doi: 10.4049/jimmunol.167.8.4254
21. Schroder K, Hertzog PJ, Ravasi T, Hume DA. Interferon-gamma: an overview of signals, mechanisms and functions. J Leukoc Biol (2004) 75:163–89. doi: 10.1189/jlb.0603252
22. Croft M. The role of TNF superfamily members in T-cell function and diseases. Nat Rev Immunol (2009) 9:271–85. doi: 10.1038/nri2526
23. Loebbermann J, Thornton H, Durant L, Sparwasser T, Webster KE, Sprent J, et al. Regulatory T cells expressing granzyme B play a critical role in controlling lung inflammation during acute viral infection. Mucosal Immunol (2012) 5:161–72. doi: 10.1038/mi.2011.62
24. Tregoning JS, Wang BL, McDonald JU, Yamaguchi Y, Harker JA, Goritzka M, et al. Neonatal antibody responses are attenuated by interferon-γ produced by NK and T cells during RSV infection. Proc Natl Acad Sci USA (2013) 110:5576–81. doi: 10.1073/pnas.1214247110
25. McNab F, Mayer-Barber K, Sher A, Wack A, O’Garra A. Type I interferons in infectious disease. Nat Rev Immunol (2015) 15:87–103. doi: 10.1038/nri3787
26. Chávez-Bueno S, Mejias A, Gomez AM, Olsen KD, Ríos AM, Fonseca-Aten M, et al. Respiratory syncytial virus-induced acute and chronic airway disease is independent of genetic background: an experimental murine model. Virol J (2005) 2:46. doi: 10.1186/1743-422X-2-46
27. Jafri HS, Chávez-Bueno S, Mejias A, Gomez AM, Ríos AM, Nassi SS, et al. Respiratory syncytial virus induces pneumonia, cytokine response, airway obstruction, and chronic inflammatory infiltrates associated with long-term airway hyperresponsiveness in mice. J Infect Dis (2004) 189:1856–65. doi: 10.1086/386372
28. Miller AL, Bowlin TL, Lukacs NW. Respiratory syncytial virus-induced chemokine production: linking viral replication to chemokine production in vitro and in vivo. J Infect Dis (2004) 189:1419–30. doi: 10.1086/382958
29. Tregoning JS, Pribul PK, Pennycook AMJ, Hussell T, Wang B, Lukacs N, et al. The chemokine MIP1alpha/CCL3 determines pathology in primary RSV infection by regulating the balance of T cell populations in the murine lung. PloS One (2010) 5:e9381. doi: 10.1371/journal.pone.0009381
30. Noah TL, Becker S. Chemokines in nasal secretions of normal adults experimentally infected with respiratory syncytial virus. Clin Immunol (2000) 97:43–9. doi: 10.1006/clim.2000.4914
31. Welliver TP, Reed JL, Welliver RC. Respiratory syncytial virus and influenza virus infections: observations from tissues of fatal infant cases. Pediatr Infect Dis J (2008) 27:S92–6. doi: 10.1097/INF.0b013e318168b706
32. Goritzka M, Pereira C, Makris S, Durant LR, Johansson C. T cell responses are elicited against Respiratory Syncytial Virus in the absence of signalling through TLRs, RLRs and IL-1R/IL-18R. Sci Rep (2015) 5:18533. doi: 10.1038/srep18533
33. Cauley LS, Lefrançois L. Guarding the perimeter: protection of the mucosa by tissue-resident memory T cells. Mucosal Immunol (2013) 6:14–23. doi: 10.1038/mi.2012.96
34. Kohlmeier JE, Cookenham T, Roberts AD, Miller SC, Woodland DL. Type I interferons regulate cytolytic activity of memory CD8(+) T cells in the lung airways during respiratory virus challenge. Immunity (2010) 33:96–105. doi: 10.1016/j.immuni.2010.06.016
35. Soudja SM, Ruiz AL, Marie JC, Lauvau G. Inflammatory monocytes activate memory CD8(+) T and innate NK lymphocytes independent of cognate antigen during microbial pathogen invasion. Immunity (2012) 37:549–62. doi: 10.1016/j.immuni.2012.05.029
36. Kupz A, Guarda G, Gebhardt T, Sander LE, Short KR, Diavatopoulos DA, et al. NLRC4 inflammasomes in dendritic cells regulate noncognate effector function by memory CD8⁺ T cells. Nat Immunol (2012) 13:162–9. doi: 10.1038/ni.2195
37. Kinnear E, Lambert L, McDonald JU, Cheeseman HM, Caproni LJ, Tregoning JS. Airway T cells protect against RSV infection in the absence of antibody. Mucosal Immunol (2017) 11:249–56. doi: 10.1038/mi.2017.46
Keywords: memory T cells, cytokines, respiratory viral infection, lung, innate immunity
Citation: Paulsen M, Varese A, Pinpathomrat N, Kirsebom FCM, Paulsen M and Johansson C (2020) MAVS Deficiency Is Associated With a Reduced T Cell Response Upon Secondary RSV Infection in Mice. Front. Immunol. 11:572747. doi: 10.3389/fimmu.2020.572747
Received: 17 June 2020; Accepted: 16 September 2020;
Published: 06 October 2020.
Edited by:
Ramon Arens, Leiden University Medical Center, NetherlandsReviewed by:
Steven M. Varga, The University of Iowa, United StatesXavier Saelens, Ghent University, Belgium
Copyright © 2020 Paulsen, Varese, Pinpathomrat, Kirsebom, Paulsen and Johansson. This is an open-access article distributed under the terms of the Creative Commons Attribution License (CC BY). The use, distribution or reproduction in other forums is permitted, provided the original author(s) and the copyright owner(s) are credited and that the original publication in this journal is cited, in accordance with accepted academic practice. No use, distribution or reproduction is permitted which does not comply with these terms.
*Correspondence: Cecilia Johansson, c.johansson@imperial.ac.uk
†Present address: Michelle Paulsen, Translational Lung Research Centre Heidelberg (TLRC), Member of the German Centre for Lung Research (DZL), University of Heidelberg, Heidelberg, Germany
Nawamin Pinpathomrat, Department of Biomedical Sciences, Faculty of Medicine, Prince of Songkla University, Hat Yai, Songkhla, Thailand
Malte Paulsen, European Molecular Biology Laboratory (EMBL), Heidelberg, Germany