- 1Department of Immunology, IML and IdSSC, Hospital Clínico San Carlos, Madrid, Spain
- 2Department of Immunology, Ophthalmology and ENT, School of Medicine, Complutense University of Madrid, Madrid, Spain
- 3Immunodeficiency Interdepartmental Group (GIID), Madrid, Spain
- 4Clinical Analysis Department, Hospital Clínico San Carlos, Madrid, Spain
- 5Laboratory of Immunogenetics of Human Diseases, IdiPAZ Institute for Health Research, Madrid, Spain
Serum free light chain (sFLC) is a recently proposed biomarker for CVID diagnosis. Most CVID patients present low or undetectable sFLC up to 10-fold lower compared to other primary antibody deficiencies. Given that κ and λ light chains are normally secreted in excess with respect to immunoglobulins, this finding points to an intrinsic defect of B cell differentiation in CVID. sFLC levels were prospectively evaluated in a cohort of 100 primary immunodeficiency (PID) patients and in 49 patients with secondary immunodeficiency to haematological malignancy (SID). CVID patients had significantly lower κ and/or λ values (mean: κ: 1.39 ± 1.7 mg/L and λ: 1.97 ± 2.24 mg/L) compared to “other PIDs” (κ: 13.97 ± 5.88 mg/L and λ: 12.92 ± 7.4 mg/L, respectively, p < 0.001 both), and SID (κ 20.9 ± 22.8 mg/L and λ 12.8 ± 8.7 mg/L, respectively, p < 0.001 both). The sum of kappa and lambda (sum κ + λ) in CVID patients (7.25 ± 7.90 mg/L) was significantly lower respect to other PIDs (26.44 ± 13.25 mg/L, p < 0.0001), and to SID patients (28.25 ± 26.24 mg/L, p = 0.0002). ROC analysis of the sum κ + λ disclosed an area under the curve (AUC) of 0.894 for CVID diagnosis (SD 0.031; 95% CI: 0.83–0.95, p < 0.0001), with optimal cut-off of 16.7 mg/L, giving the highest combination of sensitivity (92%), specificity (75%) and NPV (98%). The Relative Risk (RR) for patients presenting a sum κ + λ below 16.7 mg/L was 20.35-fold higher (95%, CI: 5.630–75.93) for CVID than below this threshold. A similar behavior of the sFLC in our CVID cohort with respect to previously published studies was observed. We propose a cut-off of sum κ + λ 16.7 with diagnostic application in CVID patients, and discuss potential specific defects converging in low or undetectable sFLC.
Background: Immunoglobulin, The Master Key of Many Locks
Given the high clinical variability and immunological heterogeneity in clinical manifestations of common variable immunodeficiency (CVID), several researchers have proposed combinations of clinical and immunological biomarkers in order to refine the diagnosis and to provide more personalized follow-up and treatment strategies that may improve the prognosis of the individual patient (1–4). A recently proposed biomarker for CVID diagnosis is the quantification of serum free light chain (sFLC) (5–8).
The key-shaped structure of immunoglobulins (Ig) as originally described by Ehrlich (9), consists of four polypeptide chains, two pairwise identical copies of both heavy (H) and light (L) chains, the latter being named kappa (κ) or lambda (λ) chains (10). This “key” opens up a wide range of processes associated with innate and adaptive immunity. Among these processes stand out the direct neutralization of an almost unlimited number of antigens and toxins, autoantibodies, modulation of fas death receptor, binding to lectins, modulation of the complement cascade, regulation of monocytes/macrophages, activation of NK cells, regulatory T cell expansion, suppression of T and B cell activation, suppression of cytokines, neuroregulatory effects and increased sensitivity of steroids (10, 11). However, functions related to this “master key” are not completely known. L chains are incorporated into Ig molecules during B-cell development. Initially, large pre-B cells express a pre-BCR that is assembled from antibody μ H chains and surrogate L chain (VPREB1 and IGLL1). At the next stage, in small pre-B cells, bona fide L chains (κ and λ) undergo recombination and when this results in a productively recombined L chain, it is expressed together with μHC forming a BCR on the surface of pre B-cells (Figure 1) (12). Production in excess of L chains occurs throughout B-cell development till plasma cells, where they bind to H chains, excess L chains enter the bloodstream as FLCs. Secretion of L chains would reflect B cell activation (13, 14).
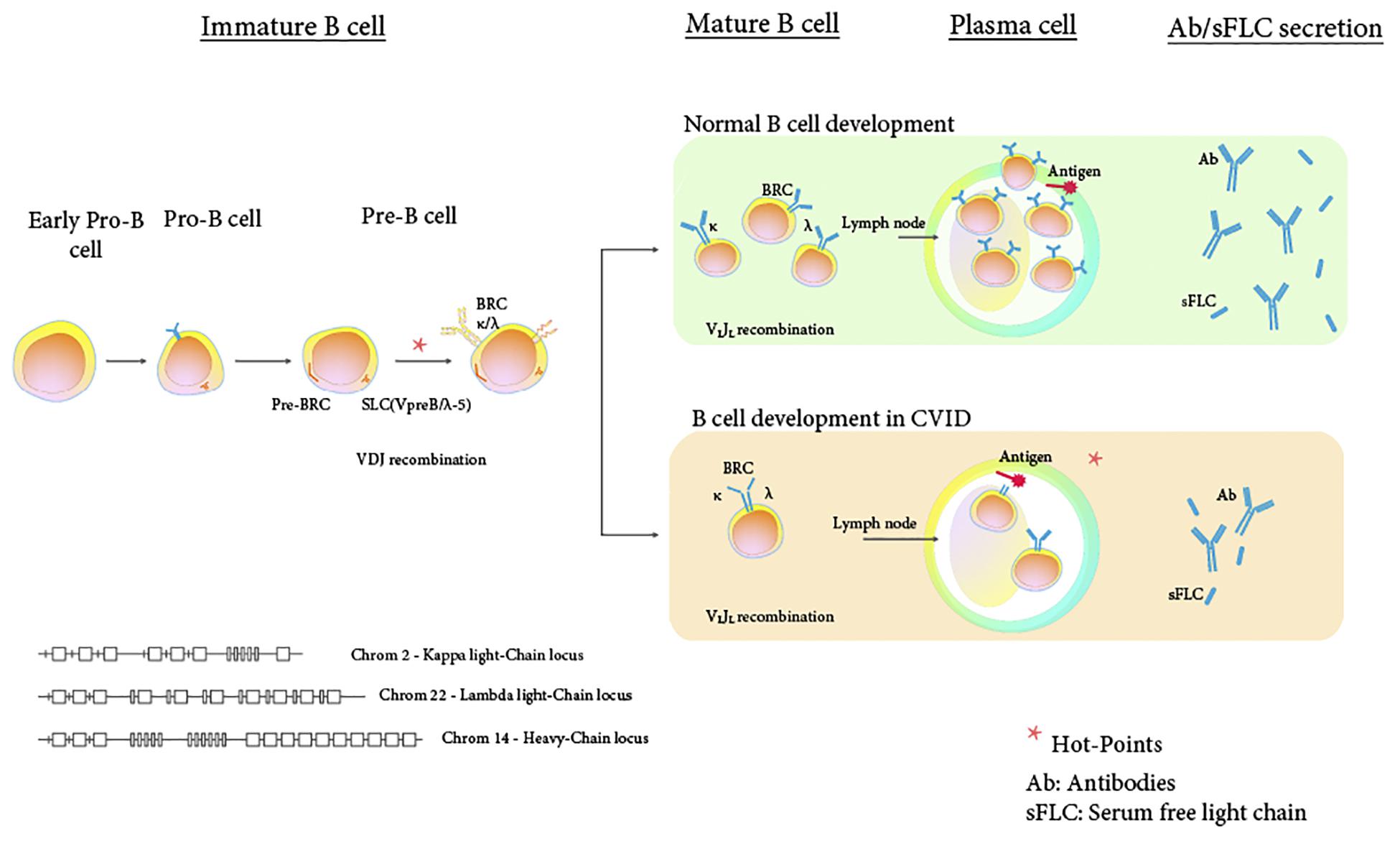
Figure 1. Potential hot-points in the development of sFLC synthesis, assembly and secretion. Scheme of the B cell maturation and differentiation, where an alteration in the rearrangement of the receptors of the pre-B cells, could condition the inadequate production of immunoglobulin light chains and, consequently, the defective expression of immunoglobulins. Modified from Winkler and Mårtensson (12).
In healthy individuals, small amounts of both free L κ and λ chains can be found (κ = 3.3–19.4 mg/l, λ = 5.7–26.3 mg/l), with a normal κ/λ ratio ranging between 0.26 and 1.65 depending on the technical assay (5). These ranges were suggested using reference serum samples from 282 healthy donors between the ages of 21 and 90 years (15), based on the polyclonal Freelite assay.
sFLC quantification may indicate the presence of B cell clonality and is widely used in clinical practice for the diagnosis of B-cell lymphoproliferative disorders (B-CLPD), in particular the progression of monoclonal gammopathy of undetermined significance (MGUS) to multiple myeloma (MM), as well as a marker of neuroinflammation, for instance, in multiple sclerosis (16–23). Moreover, dysbalance in sFLC is used as a prognostic marker of various B-CLPD, such as chronic lymphocytic leukemia (CLL), B cell non-Hodgkin lymphomas (NHL), as well as for real-time monitoring of response to treatment and disease progression (5, 17, 24–27). Due to the inherent immunological alterations in a relevant proportion of PID patients, such as polyclonal B cell proliferation, it is particularly challenging to make an early diagnosis of B malignancy in these patients. Interestingly, alterations of sFLC in PID patients (κ/λ ratio), especially in CVID, correlate with clonal processes (6, 7, 13).
Here we sought to validate previous studies on the diagnostic and prognostic value of sFLC. Secondly, we suggest the sum κ + λ as a practical combined biomarker of CVID diagnosis and other potential applications for follow-up and prognosis. Finally, we present a hypothesis on the possible scenarios underlying very low sFLC in CVID and discuss potential experimental and clinical approximations.
Materials and Methods
sFLC levels were prospectively evaluated in a cohort of 100 primary immunodeficiency (PID) patients and in 49 patients diagnosed with a hematological malignancy referred to study of secondary immunodeficiency (SID) at the Clinical Immunology Dept., Hospital Clínico San Carlos of Madrid, Spain. All PID patients fulfilled the ESID registry diagnostic criteria (28).
sFLC κ and λ chains were quantified by nephelometry (FREELITE, The Binding Site Group Ltd., Birmingham, United Kingdom), according to the manufacturer’s instructions, using a BNII nephelometer (Siemens Healthcare Diagnostics, Camberley, Surrey, United Kingdom).
SPSS statistics software (Chicago, IL, United States) was used for descriptive and statistical data analysis. Pearson’s correlation coefficient was used to assess the correlation between variables. p < 0.05 was considered statistically significant. Receiver operating characteristic curve (ROC curve) and contingency analysis were performed using GraphPad Prism version 8.3.0 for Windows, GraphPad Software, La Jolla, CA, United States1.
Approval for the study was obtained from the hospital institutional Ethics Committee for PID and SID projects (19-284-E and 19/219-E), respectively. Written informed consent was obtained from all patients for inclusion in the study protocol.
Results
sFLC Discriminates CVID From Other Primary Immunodeficiencies
We studied sFLC in 100 patients with different PIDs (selective IgA deficiency n = 38, unclassified antibody deficiency n = 27, CVID n = 26, Good syndrome n = 2, 22q11.2 deletion syndrome n = 2, complement system deficiency n = 2, X-linked hypogammaglobulinemia n = 1, hyper IgM syndrome n = 1, and Kabuki syndrome n = 1) as part as routine immunological work-up. CVID patients showed significantly lower κ and/or λ values in comparison to other PIDs (mean: κ: 1.39 ± 1.7 mg/L and λ: 1.97 ± 2.24 mg/L versus κ: 13.97 ± 5.88 mg/L and λ: 12.92 ± 7.4 mg/L, respectively, p < 0.001 both) (Figure 2). The sum of kappa and lambda (sum κ + λ) in CVID patients was 7.25 ± 7.90 mg/L versus 26.44 ± 13.25 mg/L respect to other PIDs (p < 0.0001).
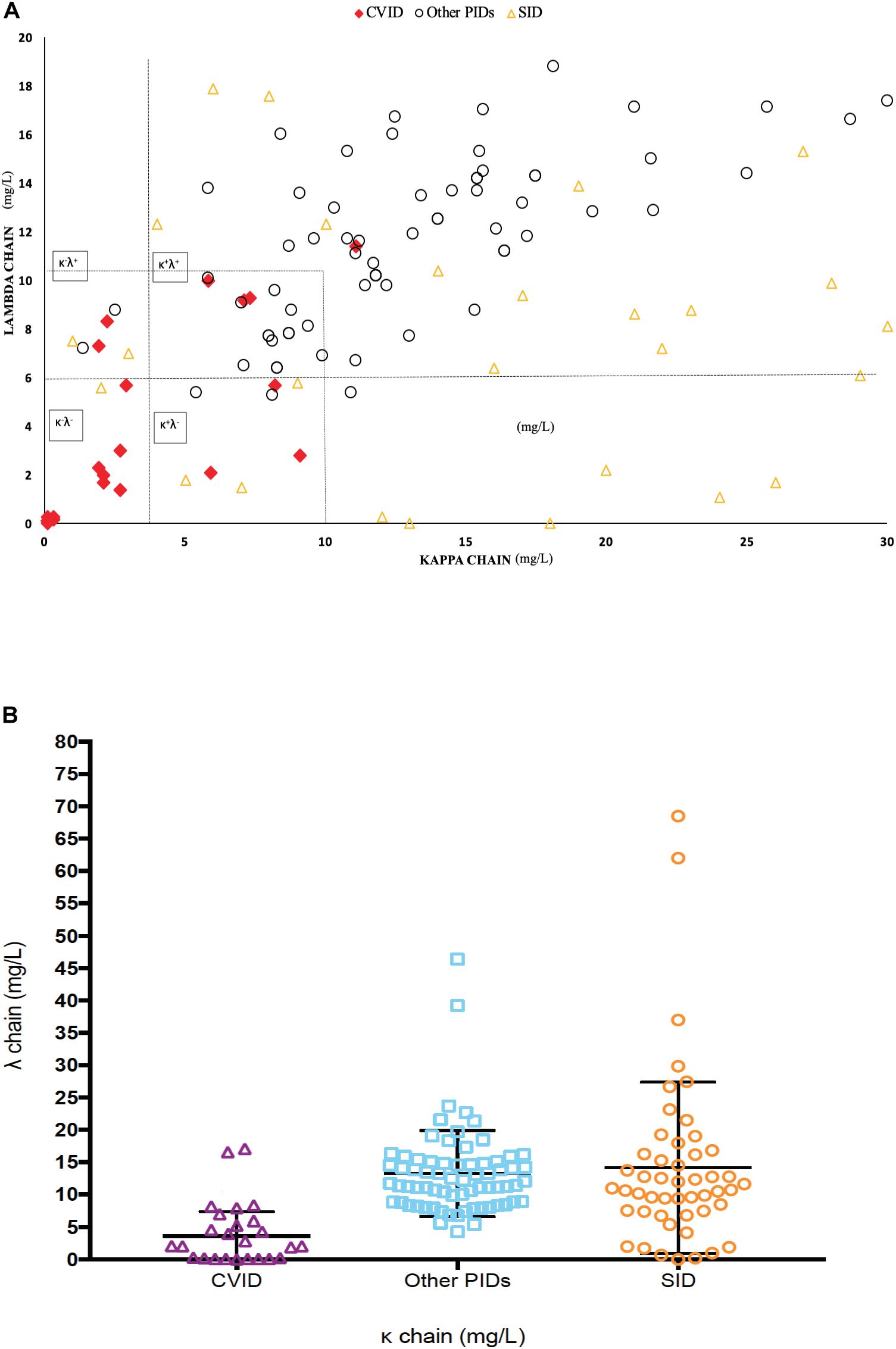
Figure 2. (A) Distribution of lambda and kappa chains among CVID, PID and SID patients (N = 100). (B) Distribution of sFLC concentration (sum κ + λ) in CVID versus other PIDs and SID.
When we analyzed the four previously described patterns of sFLCs in CVID (6–8, 29): 53.84% (14/26) disclosed the κ–λ– pattern; 30.76% (8/26) the κ+λ+ pattern; 7.69% (2/26) the κ–λ+ pattern; and 7.69% (2/26) κ+λ– pattern.
We then tested sFLC values in pure commercial gammaglobulin preparations, detecting mean levels of 4.15 mg/L of κ and 1.59 mg/L of λ, sum κ + λ 5.74 mg/L. However, it was insignificant when gammaglobulin was diluted 1:10, as found in normal plasma.
sFLCs May Aid in the Diagnosis of Secondary Immunodeficiencies
Regarding the comparison of sFLC expression in SID and CVID patients, we evaluated 49 patients with hematological malignancy: CLL (n = 12), NHL (n = 22), MGUS (n = 12) and MM (n = 3). CVID patients showed significantly lower kappa and/or lambda values than SID (mean: κ 1.39 ± 1.7 mg/L versus κ 20.9 ± 22.8 mg/L, p < 0.001; and λ 1.97 ± 2.24 mg/L versus λ 12.8 ± 8.7 mg/L, respectively, p < 0.001). When comparing the κ/λ ratio in both cohorts, CVID κ/λ ratio was significantly lower than SID (0.94 versus 1.91, p < 0.005). The sum κ + λ in CVID was also significantly lower than SID patients (7.25 ± 7.90 mg/L versus 28.25 ± 26.24 mg/L, p = 0.0002).
In our SID cohort to B-CLPD, 7 patients with CLL and NHL that showed very low or undetectable sFLC at diagnosis of malignancy were highly suspicious of an underlying CVID based on history of infections since childhood (n = 2) or suspicious family history of PID (n = 2), which were not diagnosed at the time, which could only be confirmed if PID-predisposing gene defect were found (30).
ROC Curves
The diagnostic performance of a CVID-pattern (i.e., diminished levels of free L κ and/or λ in respect to the reference range) was evaluated for the diagnosis of CVID with respect to other PID and SID, showing a sensitivity of 76.00%, specificity of 85.71%, positive predictive value (PPV) 52.78% and negative predictive value (NPV) of 94.4% for CVID diagnosis.
We then compared the diagnostic value of the sum of free L κ + λ levels in this setting. ROC analysis disclosed an area under the curve (AUC) of 0.894 (SD 0.031; 95% CI: 0.83–0.95, p < 0.0001), with optimal cut-off of 16.7 mg/L for the sum κ + λ giving the highest combination of sensitivity (92%) and specificity (75.6%), with NPV (97.8%). The Relative Risk (RR) found for CVID was of 20.35-fold (95% CI 5.630–75.93) for patients with sum κ + λ levels below 16.7 mg/L (Supplementary Figure S3). The frequency of patients below the recommended sum κ + λ cut-off of 16.7 mg/L was 92% of CVID patients; 10% of other PIDs; and 28% of SID (p < 0.0001, both).
Comparison of sFLC Values With Clinical Associations in CVID
We observed a significant correlation between κ–λ– pattern and CD27+IgD–IgM– switched-memory B cells (p < 0.05). We did not find statistical significance between specific sFLC patterns and age (p = 0.20), sex (p = 0.30), clinical onset (p = 0.15), CD21low B cells (p = 0.76) or Ig levels at diagnosis of CVID (p = 0.94). We then compared sFLC patterns (κ–λ+, κ+λ–, κ–λ–, κ+λ+) with clinical associations in CVID patients. We found that κ–λ– pattern was highly prevalent in CVID patients with enteropathy (66.66%, 4/6 patients, p = 0.01), splenomegaly (50.00%, 7/14 patients, p = 0.2) and bronchiectasis (68.75%, 11/16 patients, p = 0.1), compatible with the association described by other groups (6, 7, 29).
Regarding clinical associations in CVID patients using the sum κ + λ below 16.7 versus above this cut-off, 28.57% (6/21 patients) presented enteropathy versus 0% (p = 0.1), 57.14% (12/21) splenomegaly versus 40% (p = 0.4) and 66.66% (14/21) bronchiectasis versus 40% (p = 0.2).
Discussion
sFLC as a Diagnostic Tool in Primary Immunodeficiencies
Compatible with previous studies, a similar distribution of normal sFLC levels among all PID was observed, except for CVIDs, with significantly lower κ and/or λ values than other PIDs (p < 0.001). To better define the most optimal cutoff level for sFLC in our population, we used the sum κ + λ of 16.7 through standard statistical ROC analyses for discriminating CVID from other PID and SID, AUC of 0.894 (p < 0.0001, for both). The sum κ + λ provided a high sensitivity, specificity and NPV for CVID diagnosis.
Several groups have pioneered a greater understanding on the role of sFLCs in the clinical scenario of PIDs (6–8, 29). While many primary antibody deficiency (PAD) share a common profile of hypogammaglobulinemia, it has not been fully elucidated why only CVID presents a characteristic profile of low or undetectable sFLC (6–8, 29). Unsworth et al. described very low sFLCs in 18 out of 20 PID cases, CVID being the commonest diagnosis (16/20), followed by X-linked agammaglobulinemia (XLA, 2/20). Hyper-IgM syndrome (HIGM, 1/20) and non-HIGM with raised polyclonal IgM (1/20) showed normal sFLC values. The common denominator for all 20 patients was antibody immunodeficiency and associated increased frequency of bacterial infections (8). Scarpa et al. have recently described significant variations in sFLCs values in a wide cohort of PID. CVID patients showed decreased or undetectable values of sFLCs compared to normal values in patients with unclassified antibody defects (p < 0.0001) (7). Their findings were similar to those described in other studies with different PIDs cohorts (6–8, 29, 31, 32). Regarding XLA, there are contradictory results in the different cohorts described, ranging from low to normal sFLC, which may depend on the genetic defect (33).
In the present study, we used nephelometry with the same test, reagents, and platforms described by Scarpa et al. and Unsworth et al. in PIDs cohorts (7, 8), which reduces the variability among results. The technique used has shown the highest sensitivity for sFLC, with detection up to 0.1 mg/L for both κ and λ as low reference levels (5). However, it could be interesting to design comparative studies between the different techniques currently available for measuring sFLC in the PID setting.
The marked decrease in sFLCs could reflect a profound damage, both quantitatively and functional of the BCR during lymphocyte differentiation in CVID. Moreover, a dysfunctional BCR might be at the origin of a lymphoproliferative or self-reactive status in this patients’ population (12). In general, the production of both L chains increases during infections or inflammatory states, with higher absolute concentrations but without change in κ/λ ratio (5, 6, 8). Infection and inflammation are very common and define the clinical phenotypes “cytopenia,” “polyclonal lymphocytic infiltration,” “unexplained enteropathy,” and “no disease-related complications (only infections)” of CVID patients (3). We could infer that CVID patients with chronic inflammatory phenotype would present with high levels in a κ+λ+ pattern. Scarpa et al. analyzed the association of sFLCs patterns (κ–λ+, κ+λ–, κ–λ–, κ+λ+) and CVID clinical phenotypes. Counterintuitively, infectious and inflammatory phenotypes were more frequently observed in CVID patients with low or absent levels of sFLC (κ–λ–) (6, 7, 29). Likewise, we found that enteropathy, splenomegaly and bronchiectasis were more prevalent in the κ–λ– pattern in our cohort, although the non-significance in splenomegaly and bronchiectasis could be due to the small sample size (Supplementary Figure S1). Our findings did not demonstrate statistical significance between specific sFLC patterns and age, sex, clinical onset or Ig levels at diagnosis of CVID. In the study of Compagno et al., κ–λ+ pattern was the most represented in CVID (21 out of 46 patients, 46%) with higher risk of mortality derived from autoimmune cytopenias, lymphoproliferation and enteropathy (12/21 patients, 57%), followed by κ–λ– pattern (15/46 patients, 33%) with a trend to present splenomegaly (6/15 patients, 40%) and malignancy (5/15 patients, 33%) (6). Scarpa et al. hypothesized that low sFLC levels may be an epiphenomenon of a higher degree of impairment in B cell differentiation, with reduced B cell class-switch affecting immunoglobulins’ production (7). Altogether, these findings support that diminished sFLC values observed in CVID are associated with this pathology and can be used as an accessory diagnostic tool to support CVID diagnosis. However, the clinical significance of these patterns is still under study and needs further validation.
Comparison of sFLC Patterns With Other CVID Biomarkers
There is no clear correlation between sFLC with all serum Ig levels at diagnosis in the different PIDs groups studied (7, 8, 29). Scarpa et al. described a direct association of IgA and IgM with serum κ and λ chain concentrations in CVID but not in control groups, while no association between sFLC and serum IgG neither in CVID or control groups (7). Unsworth et al. did not find correlation between IgG and IgA values with sFLC concentrations in their PID cohort (8). Hanitsch et al. described significantly lower IgG levels in κ–λ– CVID, although IgA and IgM levels were not different (29).
There are controversial results regarding sFLC with B cell phenotype in CVID patients. We observed a significant correlation between κ–λ– pattern and class-switch CD27+IgD–IgM– memory B cells (p < 0.05), without association between CD21low B cells and sFLC patterns. In contrast, Compagno et al. described a significant decrease in numbers of switched memory, marginal zone, CD21low B cells in the κ-λ- pattern, and a marked decrease of the subsets linked to B-cell activation and Ig production, while no correlation with transitional B cells (6). IN contrast, Scarpa et al. showed the highest frequency of CD21low B cells in κ-λ- group (7). The clinical association derived from these results warrants further study.
Most of our patients were on Ig replacement therapy (IgRT), and serum testing at CVID diagnosis and pre-infusion. Commercial gammaglobulin preparations of pooled normal IgG did contain detectable κ and λ sFLCs, similar to previously published data (8), thus it seems unlikely that they may affect the results, since all patients had normal renal function and the half-life of sFLC in the circulation is 2–6 h (5). IgG infusions are typically repeated every 3–4 weeks so that pre-infusion concentrations measured are likely to only contain sFLC produced by the patient’s immune system. Likewise, the multi-time measurement of sFLC in order to determine intra-individual variability showed no difference in our patients (data not shown).
sFLC and the Dilema Between Primary and Secondary Immunodeficiencies
There is a cancer-immune paradox in PID described by some authors (34). The type of malignancy seems to be highly dependent on the specific PID, the age of the patient, and chronic infectious stimuli or dysbiosis involving complex pathogenic mechanisms (35). Cancer is 1.4 to 5-fold higher in registry-based PID studies respect to general population (36, 37), from which 70% corresponds to lymphoid malignancy (38, 39). Individuals with CVID are at 5 to 10-fold higher risk of developing hematological malignancies (36, 37, 40), while unexpectedly lower incidence on most common cancers than general population has been described (41, 42). Cancer is a leading cause of mortality in PID, and thus early diagnosis and treatment of malignancy is a priority (22, 43). When comparing the κ/λ ratio in both cohorts, SID showed significantly higher κ/λ ratio (p < 0.005), as expected (7, 29, 43). In CVID patients, the κ/λ ratio is usually normal. In CVID, a B-CLPD can be the first and only clinical manifestation and thus the diagnosis of PID versus SID represents a difficult clinical dilemma. Low κ and/or λ values at hematological malignancy diagnosis might be pointing an underlying CVID. There are no data on the “potential PID patients” in the whole pool of patients with B-CLPD, which may justify to investigate an underlying PID as cancer predisposing factor (35, 40, 41, 44). Also, κ/λ ratio could be important in the follow-up of CVID patients, and hence an altered κ/λ ratio or a sudden increase in sFLC values may be an indicator for further investigation (blood smear, LDH, serum β2-microglobulin, PET-TAC, etc.) that allows appropriate and timely strategies. We consider that the κ/λ ratio behaves like a more reliable marker than the isolated determination of the sFLC when comparing both cohorts.
The Key to CVID: Distinctive Light Chain Defect
The extremely low sFLC in CVID might be explained by different reasons: (i) the lowest the plasma cells numbers, the lowest secretion of sFLC; (ii) increased elimination of sFLC; (iii) altered rearrangement, assembly or secretion of LC during B cell ontogeny; (iv) an intrinsic defect of plasma cells secretion of light chains. To address precisely the first argument, we should quantify plasma cells in the bone marrow, which is not feasible. Indirect measures of total peripheral B cells, class-switched B cells or total serum IgG did not explain sFLC (Supplementary Figure S2). We discarded the second argument, since none of the patients had renal or other protein loss. The two last hypothesis imply that the low levels of sFLC in CVID patients may reflect an intrinsic alteration affecting normal production, assembly or secretion of L chains into Ig molecules, which points either to specific defects in plasma cell differentiation (45), or stretches the way back to a critical early event during B cell differentiation. Ig genes are first rearranged in early B cell development through the V(D)J recombination in the liver and then bone marrow and then further modified upon antigenic encounter through the somatic hypermutation (SHM) process in germinal centers of lymphoid nodes. We hypothesize that different underlying mechanisms might correlate with different sFLC patterns, which we discuss below according to clinical and immunological observations and experimental published data:
1. Early B cell defect: Particularly in cases where sFLC are undetectable, L chains should be more profoundly affected than H chains, for which a revision of genetic and molecular processes in the generation of Ig diversity is required. L chains of Ig are encoded in different multigene families from H chains and in different chromosomes: κ in chromosome 2p11.2, λ in chromosome 22q11.2 and H in chromosome 14q32.2, Several potential genetic variations might occur during this process (10, 31), affecting rearrangement of H and L chains in CVID (germline polymorphisms, allelic variants, insertion, deletion, etc.) (14, 46). At pre-B cells stage, first phase (antigen-independent) rearrangement of the H chain occurs, which reacts with light chain-like molecule called surrogate L (SL) chains by allelic exclusion (14, 46, 47). During the cell division cycles, the composition of the pre-B cell receptors (BCR) in the daughter cells engender successful production of a complete κ or λ light chain and further allows the expression of IgM on B cell surface (10).
The pre-BCR is a heterodimer composed of a H chain covalently associated with a surrogate light chains (SL) chain, a temporary common light chain composed by two non-covalently associated proteins, namely lambda-5 (λ5) and V-preB, which together have structural homology with conventional L chains (48). VDJ recombination of the H chain (pre-B cell) precedes pairing with SL chains, proliferation of large pre-B cells and subsequently L chain rearrangement (Figure 2). At this stage (at pre-BI to pre-BII or at pre-BII cell to immature B cells for pre-BCR), any transcriptional error in the SL chains, involving the region of the interchain bond during pre-B cells, would affect the correct linkage of SL to H chains and results in lack of LC. Conley reported the first patient with autosomal recessive mutation in the λ5 gene causing severe B cell deficiency and agammaglobulinaemia (33). The mutant λ5 resulted in impaired protein folding and secretion of Ig. It is conceivable that alteration of the players of this highly coordinated process at the pre-BCR stage may result in complete lack of secretion of L chains and undetectable sFLC. Isolated lack of single L chains occurs lately during this sequence. Complete absence of κ chain with normal Ig concentrations has been reported in a patient due to a heterozygous point mutation (1288 GG) that generated an amino acid substitution from Cys to Gly in the protein sequencing, causing an abnormal folding of the polypeptidic constant region of κ chain (31). In this patient, lack of κ chains determined a reduced antibody repertoire despite normal Ig concentrations, associated to recurrent bacterial infections. A correct class switch of H chains requires that the functional CH genes, located at one end of the rearranged H chain, are activated (10, 47). This synergy of mechanisms might explain in part why, in CVID patients, the decrease in sFLC is frequently associated with the inability to generate CD27+IgD–IgM– switched memory B cells. Additionally, an aberrant recombination of the L chain gene repertoire might favor autoreactive phenomena (49), while altered DNA repair controlling this recombination process would entail an increased pool of aberrant protein involves in B-cell oncogenesis (50, 51).
2. Plasma cell defect: acquired or genetic functional B cell defects have been described after TLR, CD40 and BCR-mediated NFκB signaling pathways that account for altered memory B cell phenotype in CVID patients and low Ig (52, 53). Deep sequencing of IgH locus demonstrated restriction of characteristic patterns of IgHV and IgHJ usage depending on the B cell stimulus (54). Specific defects in the terminal plasma cell differentiation were shown in a subgroup of CVID patients at germinal center responses, with diverse mechanisms converging into the block of final step of plasma cell differentiation (45). In addition, gene lesions in MSH5 has been related to a few CVID patients, with defective S region junctions between LC and HC that would take place after antigen encounter (55). Altogether, these diverse mechanisms result in reduced and modulated class-switched B cells and Ig, which may relate to L chains’ restriction and low levels of sFLC secretion (Figure 1).
Concluding Remarks and Further Perspectives
Our data validates previous studies emphasizing the relevance of sFLC quantification in the diagnosis and follow-up of CVID patients. sFLC behaves as a promising biomarker in the differential diagnosis of CVID with other PID and SID, and κ/λ ratio as a prognostic biomarker associated with specific clinical phenotypes. A cutoff level κ + λ < 16.7 mg/L supports CVID diagnosis. Moreover, κ/λ ratio alteration or a sudden increase in sFLC values may alert lymphoid malignancy and prompt appropriate and timely diagnostic work-out and therapy, a major concern in this patients’ population that impacts the survival. Reference values and cut-off points must be validated for each technique and then compare the different available immunoassays to come up with a reference range for each assay in different populations.
We hypothesize that decreased levels of sFLC in CVID patients may reflect an intrinsic early defect at a critical common step of B cell differentiation in the bone marrow affecting SL or L chain assembly or secretion that would affect memory B cell phenotype. Work is ongoing to check the hypothesis rooting this phenomenon by discarding gene defects during early B cell ontology, or intrinsic alterations in the terminal plasma cell differentiation, by in vitro differentiation of plasma cells from CVID patients after stimulation with subsequent determination of sFLC production and secretion. Altogether, we provide new evidence that this biologic phenomenon of low κ and λ provides a common feature of CVID, and leaves entirely open the question of whether would it be necessary to revisit the classification of CVID according to it.
Data Availability Statement
The raw data supporting the conclusions of this article will be made available by the authors, without undue reservation.
Ethics Statement
The studies involving human participants were reviewed and approved by the ethical committee of the San Carlos Clinical Hospital, Madrid, Spain. The patients/participants provided their written informed consent to participate in this study.
Author Contributions
KG-H and SS-R designed the study, integrity and analysis of data, and writing of the manuscript. RP, JO-G, and MF-A contributed to the analysis of data. KG-H contributed to the design of the images and figures. MC contributed to the measurement of the serum-free light chain and the analysis of data. All authors reviewed and approved the final version of the manuscript.
Conflict of Interest
The authors declare that the research was conducted in the absence of any commercial or financial relationships that could be construed as a potential conflict of interest.
Acknowledgments
We sincerely thank all our patients. We are grateful to Silvia Largacha, Luisa Campos, Nuno Barbosa, and Yolanda R. Carrasco for valuable discussions. Figures were created by Marina Lorne.
Supplementary Material
The Supplementary Material for this article can be found online at: https://www.frontiersin.org/articles/10.3389/fimmu.2020.02004/full#supplementary-material
FIGURE S1 | Bar chart comparing sFLCs patterns (κ–λ+, κ+λ–, κ–λ–, κ+λ+) with infectious and inflammatory phenotypes (Enteropathy, splenomegaly, bronchiectasis and cytopenia’s).
FIGURE S2 | Scatter plots comparing Kappa light chain concentration against: (A) Serum IgG at diagnosis; (B) Class-switched Memory B cells (CD19 + IgD-IgM-CD27 +); (C) B cells CD19%.
FIGURE S3 | Receiver operating characteristic (ROC) curves for CVID diagnosis with the sum κ + λ testing. The area under the curve (AUC) for CVID was 0.894 (P = 0.001). The use of sum κ + λ testing identified 24 of 26 cases of CVID, for a sensitivity of 92% [95% confidence interval (CI), 0.83–0.95 (p < 0.0001)].
Footnotes
References
1. Wehr C, Kivioja T, Schmitt C, Ferry B, Witte T, Eren E, et al. The EUROclass trial: defining subgroups in common variable immunodeficiency. Blood. (2008) 111:77–85. doi: 10.1182/blood-2007-06-091744
2. Bonilla FA, Barlan I, Chapel H, Costa-Carvalho BT, Cunningham-Rundles C, de la Morena MT, et al. International consensus document (ICON): common variable immunodeficiency disorders. J Allergy Clin Immunol Pract. (2016) 4:38–59. doi: 10.1016/j.jaip.2015.07.025
3. Chapel H, Lucas M, Patel S, Lee M, Cunningham-Rundles C, Resnick E, et al. Confirmation and improvement of criteria for clinical phenotyping in common variable immunodeficiency disorders in replicate cohorts. J Allergy Clin Immunol. (2012) 130:1197–8.e9. doi: 10.1016/j.jaci.2012.05.046
4. Bryant A, Calver NC, Toubi E, Webster AD, Farrant J. Classification of patients with common variable immunodeficiency by B cell secretion of IgM and IgG in response to anti-IgM and interleukin-2. Clin Immunol Immunopathol. (1990) 56:239–48. doi: 10.1016/0090-1229(90)90145-g
5. Bhole MV, Sadler R, Ramasamy K. Serum-free light-chain assay: clinical utility and limitations. Ann Clin Biochem. (2014) 51:528–42. doi: 10.1177/0004563213518758
6. Compagno N, Cinetto F, Boscaro E, Semenzato G, Agostini C. Serum free light chains in the differential diagnosis and prognosis of primary and secondary hypogammaglobulinemia. J Allergy Clin Immunol. (2015) 135:1075–7.e6. doi: 10.1016/j.jaci.2014.10.003
7. Scarpa R, Pulvirenti F, Pecoraro A, Vultaggio A, Marasco C, Ria R, et al. Serum free light chains in common variable immunodeficiency disorders: role in differential diagnosis and association with clinical phenotype. Front Immunol. (2020) 11:319. doi: 10.3389/fimmu.2020.00319
8. Unsworth DJ, Wallage MJ, Sarkar E, Lock RJ. Abnormalities of serum-free light chain in patients with primary antibody deficiency in the absence of B lymphocyte clonality. J Clin Pathol. (2012) 65:1128–31. doi: 10.1136/jclinpath-2012-201044
9. Ehrlich P. Croonian lecture.–on immunity with special reference to cell life. Proc R Soc London. (1900) 66:424–48. doi: 10.1098/rspl.1899.0121
10. Schroeder HW, Cavacini L. Structure and function of immunoglobulins. J Allergy Clin Immunol. (2010) 125:S41–52. doi: 10.1016/j.jaci.2009.09.046
11. Ramos-Medina R, Corbí AL, Sánchez-Ramón S. Inmunoglobulinas intravenosas: llave inmunomoduladora del sistema inmunológico. Med Clín. (2012) 139:112–7. doi: 10.1016/j.medcli.2011.11.022
12. Winkler TH, Mårtensson I-L. The role of the Pre-B cell receptor in B cell development, repertoire selection, and tolerance. Front Immunol. (2018) 9:2423. doi: 10.3389/fimmu.2018.02423
13. Andersen P. Deficiency of somatic hypermutation of the antibody light chain is associated with increased frequency of severe respiratory tract infection in common variable immunodeficiency. Blood. (2005) 105:511–7. doi: 10.1182/blood-2003-12-4359
14. Watson CT, Breden F. The immunoglobulin heavy chain locus: genetic variation, missing data, and implications for human disease. Genes Immunity. (2012) 13:363–73. doi: 10.1038/gene.2012.12
15. Katzmann JA, Clark RJ, Abraham RS, Bryant S, Lymp JF, Bradwell AR, et al. Serum reference intervals and diagnostic ranges for free kappa and free lambda immunoglobulin light chains: relative sensitivity for detection of monoclonal light chains. Clin Chem. (2002) 48:1437–44.
16. Dispenzieri A, Kyle R, Merlini G, Miguel JS, Ludwig H, Hajek R, et al. International myeloma working group guidelines for serum-free light chain analysis in multiple myeloma and related disorders. Leukemia. (2009) 23:215–24. doi: 10.1038/leu.2008.307
17. Hussong JW, Arber DA, Bradley KT, Brown MS, Chang C-C, de Baca ME, et al. Protocol for the examination of specimens from patients with non-hodgkin lymphoma/lymphoid neoplasms. Arch Pathol Lab Med. (2010) 134:e40–7. doi: 10.1043/1543-2165-134.6.e40
18. Maurer MJ, Micallef INM, Cerhan JR, Katzmann JA, Link BK, Colgan JP, et al. Elevated serum free light chains are associated with event-free and overall survival in two independent cohorts of patients with diffuse large B-cell lymphoma. J Clin Oncol. (2011) 29:1620–6. doi: 10.1200/JCO.2010.29.4413
19. Landgren O, Goedert JJ, Rabkin CS, Wilson WH, Dunleavy K, Kyle RA, et al. Circulating serum free light chains as predictive markers of AIDS-related lymphoma. J Clin Oncol. (2010) 28:773–9. doi: 10.1200/JCO.2009.25.1322
20. Comenzo RL, Reece D, Palladini G, Seldin D, Sanchorawala V, Landau H, et al. Consensus guidelines for the conduct and reporting of clinical trials in systemic light-chain amyloidosis. Leukemia. (2012) 26:2317–25. doi: 10.1038/leu.2012.100
21. Konen FF, Wurster U, Witte T, Jendretzky KF, Gingele S, Tumani H, et al. The impact of immunomodulatory treatment on kappa free light chains as biomarker in neuroinflammation. Cells. (2020) 9:842. doi: 10.3390/cells9040842
22. Lopez-Anglada L, Cueto-Felgueroso C, Rosiñol L, Oriol A, Teruel AI, Lopez de la Guia A, et al. Prognostic utility of serum free light chain ratios and heavy-light chain ratios in multiple myeloma in three PETHEMA/GEM phase III clinical trials. PLoS One. (2018) 13:e0203392. doi: 10.1371/journal.pone.0203392
23. Kaplan B, Ganelin-Cohen E, Golderman S, Livneh A. Diagnostic utility of kappa free light chains in multiple sclerosis. Expert Rev Mol Diagn. (2019) 19:277–9. doi: 10.1080/14737159.2019.1586535
24. Sabatino R, Perrone A, Cuomo M, Liotti S, Barchiesi V, Cantile M, et al. Analytical criticalities associated to different immunological methods for serum free light chain detection in plasma cell dyscrasias: a description of particular clinical cases. Int J Mol Sci. (2017) 18:804. doi: 10.3390/ijms18040804
25. Clay-Gilmour AI, Rishi AR, Goldin LR, Greenberg-Worisek AJ, Achenbach SJ, Rabe KG, et al. Association of elevated serumfree light chains with chronic lymphocytic leukemia and monoclonal B-cell lymphocytosis. Blood Cancer J. (2019) 9:59.
26. Morabito F, De Filippi R, Laurenti L, Zirlik K, Recchia AG, Gentile M, et al. The cumulative amount of serum-free light chain is a strong prognosticator in chronic lymphocytic leukemia. Blood. (2011) 118:6353–61.
27. Han X, Wang J, Zhang N, Yao J, Feng Y, Li D, et al. The prognostic utility and the association of serum light chains (free and total) and absolute lymphocyte count in patients with newly diagnosed diffuse large B-cell lymphoma. Leuk Res. (2014) 38:1291–8. doi: 10.1016/j.leukres.2014.09.006
28. ESID (European Society for Immunodeficiencies)Registry Working Party Diagnosis criteria. (2020). Available online at: https://esid.org/Working-Parties/Registry-Working-Party/Diagnosis-criteria (accessed June 3, 2020).
29. Hanitsch LG, Sotzny F, Volk H-D, Scheibenbogen C, Wittke K. Serum free light chains in CVID-a marker for differential diagnosis. J Clin Immunol. (2018) 38:163–5. doi: 10.1007/s10875-018-0478-y
30. Ochoa-Grullón J, Benavente Cuesta C, Pérez López C, Peña Cortijo A, Rodríguez de la Peña A, Álvarez Carmona A, et al. Evaluation of polysaccharide typhim Vi antibody response as a predictor of humoral immunodeficiency in haematological malignancies. Clin Immunol. (2020) 210:108307. doi: 10.1016/j.clim.2019.108307
31. Sala P, Colatutto A, Fabbro D, Mariuzzi L, Marzinotto S, Toffoletto B, et al. Immunoglobulin K light chain deficiency: a rare, but probably underestimated, humoral immune defect. Eur J Med Genet. (2016) 59:219–22. doi: 10.1016/j.ejmg.2016.02.003
32. Bousfiha A, Jeddane L, Picard C, Ailal F, Bobby Gaspar H, Al-Herz W, et al. The 2017 IUIS phenotypic classification for primary immunodeficiencies. J Clin Immunol. (2018) 38:129–43. doi: 10.1007/s10875-017-0465-8
33. Minegishi Y, Coustan-Smith E, Wang YH, Cooper MD, Campana D, Conley ME. Mutations in the human lambda5/14.1 gene result in B cell deficiency and agammaglobulinemia. J Exp Med. (1998) 187:71–7. doi: 10.1084/jem.187.1.71
34. Bomken S, van der Werff Ten Bosch J, Attarbaschi A, Bacon CM, Borkhardt A, Boztug K, et al. Current understanding and future research priorities in malignancy associated with inborn errors of immunity and DNA repair disorders: the perspective of an interdisciplinary working group. Front Immunol. (2018) 9:2912. doi: 10.3389/fimmu.2018.02912
35. Mortaz E, Tabarsi P, Mansouri D, Khosravi A, Garssen J, Velayati A, et al. Cancers related to immunodeficiencies: update and perspectives. Front Immunol. (2016) 7:365. doi: 10.3389/fimmu.2016.00365
36. Haas OA. Primary immunodeficiency and cancer predisposition revisited: embedding two closely related concepts into an integrative conceptual framework. Front Immunol. (2019) 9:3136. doi: 10.3389/fimmu.2018.03136
37. Mayor P, Eng K, Singel KL, Abrams SI, Odunsi K, Moysich K, et al. Cancer in primary immunodeficiency diseases: cancer incidence in the United States immune deficiency network registry. J Allergy Clin Immunol. (2017) 141:1028–35. doi: 10.1016/j.jaci.2017.05.024
38. Kersey JH, Shapiro RS, Filipovich AH. Relationship of immunodeficiency to lymphoid malignancy. Pediatr Infect Dis J. (1988) 7:S10–2.
39. Riaz IB, Faridi W, Patnaik MM, Abraham RS. A Systematic review on predisposition to lymphoid (B and T cell) neoplasias in patients with primary immunodeficiencies and immune dysregulatory disorders (Inborn Errors of Immunity). Front Immunol. (2019) 10:777. doi: 10.3389/fimmu.2019.00777
40. Quinti I, Agostini C, Tabolli S, Brunetti G, Cinetto F, Pecoraro A, et al. Malignancies are the major cause of death in patients with adult onset common variable immunodeficiency. Blood. (2012) 120:1953–4. doi: 10.1182/blood-2012-05-431064
41. Pulvirenti F, Quinti I. Malignancies as the main cause of death in common variable immunodeficiency: an italian multicentre study. Eur Med J. (2018) 3:79–80.
42. Gangemi S, Allegra A, Musolino C. Lymphoproliferative disease and cancer among patients with common variable immunodeficiency. Leuk Res. (2015) 39:389–96. doi: 10.1016/j.leukres.2015.02.002
43. Singh G. Serum free light chain assay and κ/λ ratio: performance in patients with monoclonal gammopathy-high false negative rate for κ/λ ratio. J Clin Med Res. (2017) 9:46–57. doi: 10.14740/jocmr2802w
44. Leone P, Vacca A, Dammacco F, Racanelli V. Common variable immunodeficiency and gastric malignancies. Int J Mol Sci. (2018) 19:451. doi: 10.3390/ijms19020451
45. Taubenheim N, von Hornung M, Durandy A, Warnatz K, Corcoran L, Peter H-H, et al. Defined blocks in terminal plasma cell differentiation of common variable immunodeficiency patients. J Immunol. (2005) 175:5498–503. doi: 10.4049/jimmunol.175.8.5498
46. Collins AM, Watson CT. Immunoglobulin light chain gene rearrangements, receptor editing and the development of a self-tolerant antibody repertoire. Front Immunol. (2018) 9:2249. doi: 10.3389/fimmu.2018.02249
47. Lucas AH. Immunoglobulin gene construction: human. eLS. (2003). Available online at: https://onlinelibrary.wiley.com/doi/full/10.1038/npg.els.0001172 (accessed April 15, 2020),Google Scholar
48. Mårtensson I-L, Ceredig R. Role of the surrogate light chain and the pre-B-cell receptor in mouse B-cell development. Immunology. (2000) 101:435–41. doi: 10.1046/j.1365-2567.2000.00151.x
49. Gay D, Saunders T, Camper S, Weigert M. Receptor editing: an approach by autoreactive B cells to escape tolerance. J Exp Med. (1993) 177:999–1008. doi: 10.1084/jem.177.4.999
50. Bassing CH, Swat W, Alt FW. The mechanism and regulation of chromosomal V(D)J recombination. Cell. (2002) 109(Suppl.):S45–55. doi: 10.1016/s0092-8674(02)00675-x
51. Liao MJ, Van Dyke T. Critical role for Atm in suppressing V(D)J recombination-driven thymic lymphoma. Genes Dev. (1999) 13:1246–50. doi: 10.1101/gad.13.10.1246
52. Yu JE, Knight AK, Radigan L, Marron TU, Zhang L, Sanchez-Ramón S, et al. Toll-like receptor 7 and 9 defects in common variable immunodeficiency. J Allergy Clin Immunol. (2009) 124:349–56.e1–3. doi: 10.1016/j.jaci.2009.05.019
53. Keller B, Cseresnyes Z, Stumpf I, Wehr C, Fliegauf M, Bulashevska A, et al. Disturbed canonical nuclear factor of κ light chain signaling in B cells of patients with common variable immunodeficiency. J Allergy Clin Immunol. (2017) 139:220–31.e8. doi: 10.1016/j.jaci.2016.04.043
54. Simchoni N, Cunningham-Rundles C. TLR7- and TLR9-responsive human B cells share phenotypic and genetic characteristics. J Immunol. (2015) 194:3035–44. doi: 10.4049/jimmunol.1402690
Keywords: common variable immunodeficiency, serum-free immunoglobulins light chains, diagnostic tool, prognostic biomarkers, primary immunodeficiencies
Citation: Guevara-Hoyer K, Ochoa-Grullón J, Fernández-Arquero M, Cárdenas M, Pérez de Diego R and Sánchez-Ramón S (2020) Serum Free Immunoglobulins Light Chains: A Common Feature of Common Variable Immunodeficiency? Front. Immunol. 11:2004. doi: 10.3389/fimmu.2020.02004
Received: 11 May 2020; Accepted: 24 July 2020;
Published: 11 August 2020.
Edited by:
Guzide Aksu, Ege University, TurkeyReviewed by:
Isabella Quinti, Sapienza University of Rome, ItalyJennifer Heaney, University of Birmingham, United Kingdom
Copyright © 2020 Guevara-Hoyer, Ochoa-Grullón, Fernández-Arquero, Cárdenas, Pérez de Diego and Sánchez-Ramón. This is an open-access article distributed under the terms of the Creative Commons Attribution License (CC BY). The use, distribution or reproduction in other forums is permitted, provided the original author(s) and the copyright owner(s) are credited and that the original publication in this journal is cited, in accordance with accepted academic practice. No use, distribution or reproduction is permitted which does not comply with these terms.
*Correspondence: Silvia Sánchez-Ramón, c3NyYW1vbkBzYWx1ZC5tYWRyaWQub3Jn