- 1Department of Dental Medicine, Karolinska Institutet, Huddinge, Sweden
- 2College of Dentistry, Prince Sattam Bin Abdulaziz University, Al-Kharj, Saudi Arabia
- 3Tenth People’s Hospital, Tongji University, Shanghai, China
- 4Pancreatic Surgery Unit, Division of Surgery, Department of Clinical Science, Intervention and Technology, Karolinska University Hospital, Huddinge, Sweden
- 5Division of Pathology, Department of Laboratory Medicine, Karolinska Institutet, Huddinge, Sweden
- 6Department of Clinical Pathology/Cytology, Karolinska University Hospital, Huddinge, Sweden
- 7Clinic of Endodontics and Periodontology, Eastman Institute Stockholm, Stockholm, Sweden
- 8Division of Surgical Oncology, Department of Surgery, University of Colorado, Aurora, CO, United States
- 9Division of Clinical Microbiology, Department of Laboratory Medicine, Karolinska Institutet, Huddinge, Sweden
Objectives: Intraductal papillary mucinous neoplasms (IPMNs) are cystic precursor lesions to pancreatic cancer. The presence of oral microbes in pancreatic tissue or cyst fluid has been associated with high-grade dysplasia (HGD) and cancer. The present study aims at investigating if humoral immunity to pancreas-associated oral microbes reflects IPMN severity.
Design: Paired plasma (n = 109) and saliva (n = 65) samples were obtained from IPMN pancreatic cystic tumor cases and controls, for anti-bacterial antibody analysis and DNA quantification by enzyme-linked immunosorbent assay (ELISA) and qPCR, respectively. Tumor severity was graded by histopathology, laboratory, and clinical data. Circulating plasma and salivary antibody reactivity to a pancreas-associated oral microbe panel were measured by ELISA and correlated to tumor severity.
Results: The patient group with high-risk cystic tumors (HGD and/or associated invasive cancer) shows ample circulating IgG reactivity to Fusobacterium nucleatum (F. nucleatum) but not to Granulicatella adiacens (G. adiacens), which is independent of the salivary bacteria DNA levels. This group also shows higher salivary IgA reactivity to F. nucleatum, Fap2 of F. nucleatum, and Streptococcus gordonii (S. gordonii) compared to low-risk IPMN and controls. The salivary antibody reactivity to F. nucleatum and Fap2 are found to be highly correlated, and cross-competition assays further confirm that these antibodies appear cross-reactive.
Conclusion: Our findings indicate that humoral reactivity against pancreas-associated oral microbes may reflect IPMN severity. These findings are beneficial for biomarker development.
Introduction
Pancreatic cancer (PC) is highly lethal, as the statistics of its incidence rate are closely similar to those of its mortality rate (1). It is the fourth most prevalent cause of cancer mortality in the United States (1), and approximately 330,400 die of PC each year worldwide (2). Yearly incidence rates of PC are dramatically increasing, and PC is expected to be the second top cancer killer before 2030 (3). Up to 90% of PC patients die within 5 years of diagnosis, and more than 50% of them die in the first 6 months after the diagnosis1,2. A substantial reason behind the high lethality of this disease lies in the obscure symptoms at its early stages, which result in late stage detection (4). Consequently, early detection of PC is a pivotal step in reducing the PC mortality rate, but much remains to be explored regarding the etiopathology as well as the diagnostic and therapeutic approaches to this cancer.
Pancreatic cystic neoplasms (PCN) became a common type of clinically detected lesion with the current advancement of cross-sectional imaging diagnostic modalities (5–7). One of the most common PCN types is intraductal papillary mucinous neoplasm (IPMN) (8, 9). IPMNs are epithelial neoplastic cysts in the ductal systems of the pancreas, distinguished by the papillary projection of proliferated epithelial cells and mucin secretion that leads to a dilated pancreatic duct (10). IPMNs are characterized by their association with malignancies, as it is believed that the dysplastic pattern of IPMN can progress from low-grade dysplasia (LGD) to high-grade dysplasia (HGD) with the potential to transform into invasive carcinoma (9, 11). The growing incidence of IPMNs and their tendency to progress toward invasive cancer focus great attention and efforts on detection of their benign forms, or LGD, which constitute the majority of cases and do not require surgical intervention (5, 8, 10). Preoperative diagnostic accuracy is still a major challenge that impacts the criteria for surgical resection; hence, the final diagnosis can only be confirmed histologically after operation (5, 10). This raises important issues with over- or under-treatment that could be better addressed by more accurate diagnostic measures.
Several commensal bacteria have been found to play oncogenic roles in different tumors (12), as microbial dysbiosis with markedly increased bacterial abundance is postulated to negatively impact immunity and foster pancreatic tumorigenesis (13). Distinct species of oral bacteria were found to be associated with distal tumor microenvironments in colorectal cancer and, recently, pancreatic neoplasms (14–16). Among them, Fusobacterium nucleatum—a non-motile, non-spore forming, gram-negative, opportunistic anaerobic periodontal bacterium—is garnering attention due to its overrepresentation in colon tumor tissues and has been proposed as a potential oncopathogen (14, 15, 17–19). Enrichment of oral commensals, including F. nucleatum, in pancreas is noted to correlate with malignancy in pancreas (16, 20). Presently, the role of oral bacteria in relation to pancreatic neoplasms remains unclear, but circulating antibodies against commensal oral bacteria appear elevated in PC and colorectal cancer (21–23). In contrast, relatively less is known about the salivary antibody responses to such bacteria in relation to the stage of PC development.
Consequently, to further investigate the link between F. nucleatum and IPMN, we measured the antibodies against F. nucleatum and other oral commensals using pre-operation plasma and saliva samples of IPMN patients. The relationship of cystic tumor severity to salivary bacteria levels was also analyzed. Specifically, the aim of this study is to characterize humoral immunity to oral bacteria previously identified in pancreatic microbiome (16), with a focus on F. nucleatum.
Materials and Methods
Study Population and Sample Collection
Patients with cystic lesions undergoing pancreatic surgery for suspected PC at Karolinska University Hospital, Stockholm, Sweden, were prospectively included in 2017–2019 as participants after signing informed consent forms. The final pancreas diagnosis confirmed by post-operative histology reports further divide the cohort into the following sub-groups: low-grade dysplasia IPMN (LGD-IPMN), high-grade dysplasia IPMN (HGD-IPMN), or IPMN with invasive cancer (Cancer); and non-IPMN. Clinicopathology dta were retrieved from medical journals by physicians. Blood samples were obtained from 109 participants at the day of the surgery, and 65 also donated saliva samples. Additional healthy donors without pancreas diagnoses (n = 8) were recruited for clinical oral health examination and saliva donation at the Karolinska University Hospital. Plasma was prepared after centrifugation in K2 EDTA tubes (BD Vacutainer) and Ficoll Paque PLUS (GE Life Sciences) density gradient according to manufacturers’ instructions, then immediately stored at −80°C. Saliva samples were collected the day before the pancreas surgery. The participants were asked to refrain from eating, drinking, smoking, or using oral hygiene products for at least 1 h prior to collection. Expectorated fresh stimulated saliva was collected in sterile 50 mL polyethylene tubes on ice, and thereafter immediately stored at −80°C in 1.5 mL aliquots.
Periodontal Self-Reported Questionnaire
The questionnaire to evaluate the oral health condition comprised a set of eight closed-ended, self-reported questions identified by the Centers for Disease Control and Prevention (CDC) in collaboration with the American Academy of Periodontology (AAP) (24, 25). These questions were formulated based on the CDC-AAP case definitions for surveillance of periodontitis (26) assessing gum and teeth health, dental treatment history, bone loss, loose teeth, teeth appearance, and usage of dental floss and mouthwash. The questionnaire was distributed prior to surgery to the participants. Data obtained were analyzed to compare the oral health condition between PCN groups.
Bacterial Strains, Handling, and Inactivation Methods
An array of clinical isolates related to previously reported pancreas microbiome were identified by Matrix-Assisted Laser Desorption/Ionization Time-of-Flight Mass Spectrometry (MALDI-TOF MS). F. nucleatum and Porphyromonas gingivalis were isolated from clinical blood cultures, identified as in standard clinical routine by MALDI-TOF MS, and were stored at −80°C. The isolates were then thawed and cultured on blood agar in anaerobic milieu 48 h at 37°C. Streptococcus gordonii, Streptococcus anginosus (S. anginosus), Granulicatella adiacens, and Escherichia coli (E. coli) strains were identified by MALDI-TOF MS after having been isolated from the pancreatic cyst fluid of the present patient cohort and then cultured on blood and hematin agar plates. The plates were incubated at 37°C and were examined after 24 h. Glycerol was then added to the liquid cultures (20% final concentration) prior to storage at −80°C. The bacterial pellets were inactivated for enzyme-linked immunosorbent assay (ELISA) analysis by heat inactivation. Isolated bacteria pelleted at 1 × 10E9 CFU were washed twice in PBS and heat-killed at 85°C for 1 h. The B cell mimotope peptide of F. nucleatum, Fap2 protein (Fap2), of the sequence TELAYKHYFGT described earlier by prediction analysis using the Immune Epitope Database (IEDB) Analysis Resource (22), was synthesized to a purity of 98% (Genscript, New York, United States). As an alternative to heat inactivation, bacterial pellets were inactivated by being resuspended in 0.5 mL Tris–buffered saline, fixed in 0.5% formalin-PBS overnight at 4°C, and washed three times with PBS.
ELISA Assays
Heat-inactivated bacteria were prepared as coating antigens to determine antibody reactivity by ELISA. P. gingivalis, S. gordonii, S. anginosus, and G. adiacens were used as oral bacterial controls and E. coli as a non-oral bacterial control. Indicated bacteria and peptide were resuspended in coating buffer (sodium carbonate buffer 50 mM, pH 9.6) to reach a concentration of 5 × 10E7 CFU/mL and 10 μg/mL, respectively. Afterward, 100 μL were added to each well of a Nunc MaxiSorp™ 96-well ELISA plate (Sigma-Aldrich Sweden AB, Stockholm, Sweden) for overnight coating at 4°C. Wells were washed three times with washing buffer [0.05% Tween-20 (VWR Chemicals, Spånga, Sweden) in PBS]. A buffer of 1% BSA and 2% goat serum (Sigma-Aldrich, G6767) in PBS was used as blocking and dilution buffer. Wells were incubated with the blocking buffer for 1 h at 37°C, and then washed 3 times with the washing buffer. After an initial assay optimization, plasma and saliva samples were diluted with the dilution buffer into 1:300 and 1:16, respectively, then incubated in duplicate wells for 1 h at 37°C on antigen-coated plates. For total IgG and IgA ELISA, samples were diluted 1:125 000 and 1:5000 for plasma and saliva, respectively. Plates were washed as described above and incubated with peroxidase antibody produced in goat anti-Human IgG/IgA (Sigma-Aldrich Sweden AB; diluted 1:10 000) for 1 h at 37°C. After washing, the wells were developed with tetramethylbenzidine substrate (R&D Systems, Minneapolis, Minnesota, United States) for 20 min and stopped immediately with 0.16 M sulfuric acid, and the absorbance was read at 450 nm (Multiskan MS, Thermo Labsystems, Vantaa, Finland). Internal controls consisting of a pool of high-respective low-reactive patient plasma or saliva from HG-IPMN + cancer resp. control group were included in each run. If the OD of internal controls deviated more than 10% from previous runs, the entire plate was repeated. The OD measurements of internal controls were used to calculate the inter/intra-assay coefficient of variability for all runs. Inter-assay coefficient of variability was 8.5 ± 4.5% and 10.4 ± 2.3%, respectively, for plasma and saliva assays, while the intra-assay coefficients of variability were 8 ± 2.3% and 8.7 ± 1%, respectively. The competitive ELISA assay was performed by coating plates with Fap2 peptide overnight. Saliva samples with or without pre-incubation with F. nucleatum, Fap2, or the E. coli control for 2 h at 37°C were added to the Fap2 peptide-coated plates and developed as above. Total levels of salivary IgA and plasma IgG antibodies were measured using Human IgA/IgG ELISA Kit (Novus Biologicals, Colorado, United States) according to the manufacturer’s instructions.
Salivary Bacterial DNA Isolation
Microbial DNA was isolated from 200 μL of bacterial culture or saliva using ZymoBIOMICS™ DNA Mini Kit (Zymo Research, Irvine, California, United States) according to the manufacturer’s instructions and eluted into 100 μL of RNAse free water. Isolated DNA was stored at −20°C until use.
qPCR Quantification of Microbial gDNA Levels
Saliva DNA isolated from 200 μL of saliva were analyzed by qPCR using 7500 Fast Real-Time PCR system (Applied Biosystems, Waltham, Massachusetts, United States). Bacterial gDNA of F. nucleatum and G. adiacens were used for both standard curves and positive controls, while nuclease-free water and blank PCR Master Mix were used for negative controls. Each sample for F. nucleatum analysis included, together with 5 μL of template DNA, 500 mM forward primer: 5′-AGGGTGAACGGCCACAAG-3′, 500 mM reverse primer: 5′-TCTCGGTCCATTGTCCAATATTCC-3′, 200 mM probe: 5′-FAM- ACACGGCCCTTACTCC -TAMRA-3′ (16, 27), and 10.0 μL TaqMan™ Fast Universal PCR Master Mix (ThermoFisher Scientific, Waltham, Massachusetts, United States) and RT-PCR grade water (Invitrogen, Waltham, Massachusetts, United States), for a total reaction volume of 20 μL. The composition of samples for G. adiacens analysis differed only in primers and probe: 5′-CAAGCTTCTGCTGATGGATGGA-3′ as forward, 5′-CTC AGGTCGGCTATGCATCAC-3′ as reverse primer, and 5′-FAM-GCTAGTTGGTGAGGTAACGGCTCA-TAMRA-3′ as probe (28). Primers for 16S gene analysis were: Forward 5′-TGGAGCATGTGGTTTAATTCGA-3, and reverse 5′-TGC GGGACTTAACCCAACA-3 primers, as 16S probe was 5′-FAM-CACGAGCTGACGACA[A/G]CCATGCA-TAMRA-3 (29). Samples were heated at 95°C for 10 min, followed by 40 cycles of 95°C for 15 s and 58°C for 30 s.
Statistical Analysis
All statistical analyses were performed with GraphPad Prism (version 8.00 for Windows, GraphPad Software, La Jolla, CA, United States). Pairwise statistical comparisons between each group were made using Kruskal–Wallis test with Dunn’s multiple comparisons correction for quantitative parameters and Fisher’s exact test for qualitative values. The difference in antibody responses between the groups was analyzed by using Kolmogorov–Smirnov test. The correlation between plasma IgG and salivary IgA reactivity against F. nucleatum was examined with Pearson analysis. For the competition assay analysis, Wilcoxon test pairwise statistics was used. The ELISA cut-off values of saliva samples were computed based on the formula described by Frey et al. on healthy controls (30). Values higher than the cut-off were regarded as reactive while those that were lower as non-reactive.
Results
Characteristics of the Study Participants
A total of 109 participants with clinical and radiographically confirmed PCN with suspicion of cancer undergoing surgery during 2017–2019 were recruited for the study. Based on final postoperative histopathology reports, the cases were sub-grouped into: HGD-IPMN + Cancer (IPMN with invasive cancer; n = 46), LGD-IPMN (n = 45), and non-IPMN (n = 18; Table 1). This gives a pre-operative diagnosis accuracy at 52, 0, 65% for non-IPMN, LGD-IPMN, and HGD-IPMN + Cancer, respectively, based on comparisons of the pre-operation diagnosis. Moreover, the non-IPMN cases were often younger, and fewer were male or had diabetes. Elevated serum CA19-9 level was found in 55.6% in HGD-IPMN + Cancer group, and 6.7–16.7% in the other groups.
Plasma Reactivities to Oral Commensals Associated With IPMN Cancer Signature
Plasma samples were tested for binding reactivity to F. nucleatum, G. adiacens, S. gordonii, S. anginosus, and a Fap2 mimotope of F. nucleatum by indirect IgG and IgA ELISA. E. coli was included as a non-oral commensal control. In Figure 1, as compared by the absorbance readings, the IgG binding reactivities to F. nucleatum in the HGD-IPMN + Cancer group appeared higher than non-IPMN (control group) but not LGD-IPMN group. Reactivities to the Fap2 mimotope of F. nucleatum, S. anginosus, S. gordonii, or E. coli did not differ between the groups (Figures 1B–F). But reactivities to G. adiacens were noted to be weaker in HGD-IPMN + Cancer than other groups (Figure 1C). Moreover, no significant difference was found when the total plasma IgG levels were compared (Figure 1G). Of note, plasma IgA reactivities were weak in general; no significant differences were noted between the groups, irrespectively of the antigen tested (data not shown).
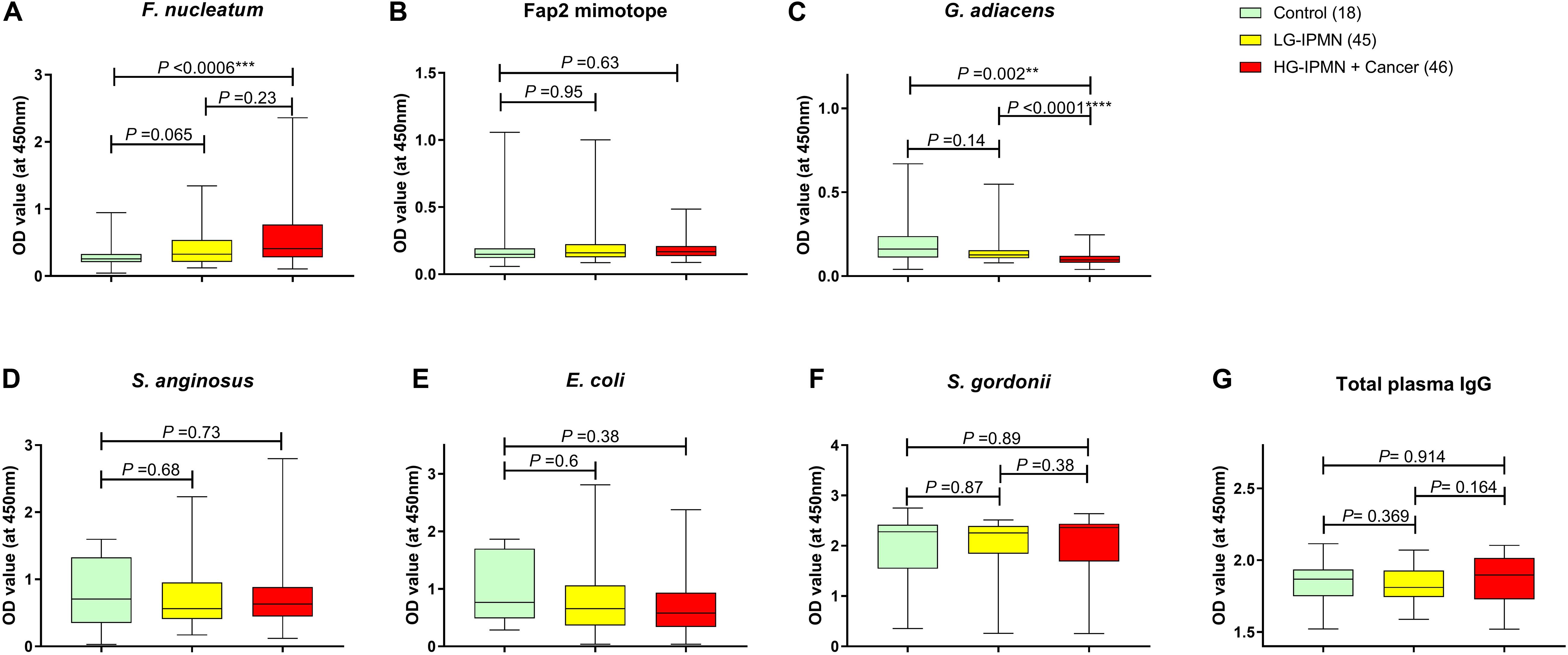
Figure 1. Circulating plasma antibody reactivity to oral commensals identified in pancreatic tumor lesions. Plasma diluent (1/300) from patients with histology verified pancreas tissues: IPMN with low grade dysplasia (LGD-IPMN), IPMN with high grade dysplasia or with invasive cancer (HGD-IPMN + Cancer), or benign tumors/non-IPMN (Control) was tested in duplicates in ELISA plates coated with indicated antigen in (A–F) (bacteria: 5 × 10E7 CFU/ml, peptide 10 μg/mL). The total plasma IgG level is shown in (G). Internal control Mean OD absorbance values of duplicates are shown. Statistical analysis was performed using the Kolmogorov–Smirnov test, **p < 0.01, ***p < 0.001, and ****p < 0.0001.
Salivary Reactivity to Oral Commensals Associated With IPMN Cancer Signature
Because bacteria of interest here mostly colonize the oral cavity, saliva samples donated by the patient cohort above were tested. To provide a better baseline for saliva tests, orally healthy participants (n = 8) without pancreas diagnosis were included in the control group (Table 2). From the total of 65 saliva donors tested, salivary IgA appeared to dominate over IgG (data not shown). In Figure 2, the results indicate that salivary IgA reactivity to F. nucleatum in the HGD-IPMN + Cancer group was higher than both LGD-IPMN and control groups. This was shown also for the Fap2 mimotope of F. nucleatum, as well as for S. gordonii (Figures 2A–F), but not for the other bacteria tested in the panel, and is independent of the total salivary IgA which appear comparable in all three groups. As noted in oral health data, these groups report similar frequency of gum bleeding or untreated gum disease and bone loss. These two groups’ perceptions of own dental health and other oral parameters are also similar (Supplementary Table S1). Moreover, the Fap2-mimotope- or whole bacteria-binding antibody (OD readings) levels when expressed as percentage of reactive or non-reactive saliva samples suggest that 52.4% of HG-IPMN + Cancer group had Fap2 mimotope reactivity in saliva, whilst up to 61.9% had salivary reactivity to any Fap2-mimotope, F. nucleatum, or S. gordonii antigen (Supplementary Table S2).
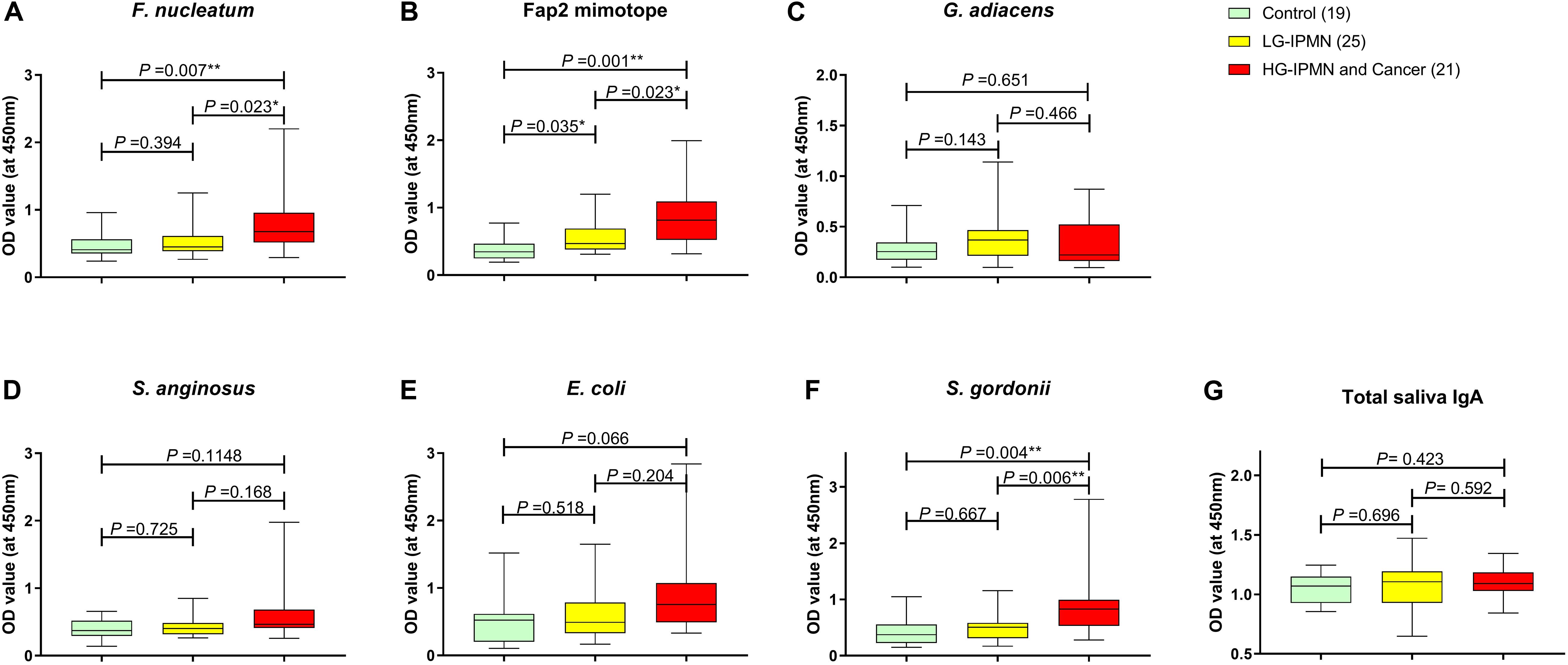
Figure 2. Salivary IgA antibody reactivity to oral commensals quantified by ELISA using saliva diluent (1/16) from indicated patient group. Salivary IgA reactivity to F. nucleatum, the Fap2 mimotope, and S. gordonii (A,B,F) in HG-IPMN/Cancer patients is significantly increased compared to other groups. The differences in antibody levels against bacterial antigens of G. adiacens, S. anginosus, and E. coli (C,D,E) were insignificant between groups. Total plasma IgA level did not differ between the groups (G). Statistical analysis was performed using the Kolmogorov–Smirnov test, *p < 0.05 and **p < 0.01.
Correlation of F. nucleatum Binding to Fap2 Mimotope in Plasma and Saliva, and Cross-Reactivity Validation
Because the increased salivary IgA reactivity to F. nucleatum and the Fap2 mimotope were noted separately, subsequent correlation analysis was carried out. It could confirm that the two IgA specificities in saliva are clearly correlated unlike that of plasma IgG (Figures 3A,B). The plasma and salivary antibody reactivities to F. nucleatum, but not to Fap2, are also significantly correlated (Figures 3C,D). To further examine the specificity interactions of salivary IgA between F. nucleatum- and Fap2 mimotope-binding antibodies, a competition ELISA utilizing the mimotope coated plates was performed. A pre-absorption for 2 h at 37°C, either with F. nucleatum or the Fap2 mimotope, was enough to reduce the Fap2 mimotope binding efficiency significantly by 24 ± 31% and 40 ± 20%, respectively, but not with E. coli (1.7 ± 25%; Figure 3E). Taken together, these results confirm that antibodies to the Fap2 mimotope in saliva cross-react with F. nucleatum and may interfere with the antigen binding sites.
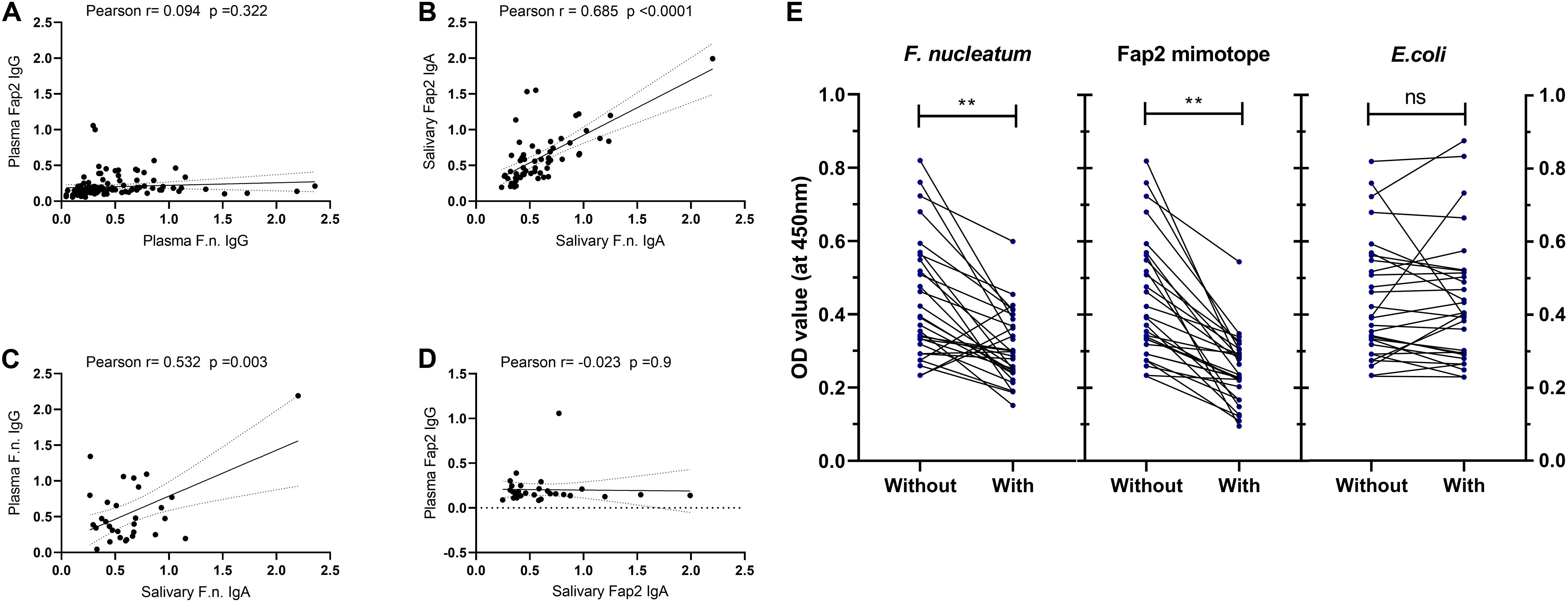
Figure 3. Correlation between circulating plasma (A) or salivary (B) antibody reactivity to F. nucleatum and Fap2 mimotope, or between circulating plasma antibody and salivary antibodies to either of antigen (C,D). Competitive ELISA (E) with saliva samples with or without antigen pre-absorption as indicated for 2 h, then subjected to Fap2 mimotope ELISA test. Statistical analysis was performed using two-tailed Pearson correlation test and Wilcoxon test for two related samples. **p < 0.01, ns = not significant.
Salivary Bacteria Copy Numbers in Relation to Pancreas Diagnosis
To determine whether F. nucleatum and G. adiacens salivary antibody levels are attributed to the salivary bacteria, bacterial DNA copy levels were measured by targeted qPCR Taqman assays. Interestingly, the levels of F. nucleatum and G. adiacens DNA appear to be relatively comparable in all three groups, regardless of the method used to express them (i.e., as percentage of total salivary 16S copy level or genome copy number per volume saliva; Figures 4A–D). Total salivary bacterial DNA as assessed by universal 16S gene copy levels showed comparable levels among the three groups (Figure 4E). Additionally, correlation analyses showed no significant association between bacterial DNA copy levels to either bacteria antibody reactivity, smoking, or antibiotic use in the past 3 months prior to sample collection (data not shown).
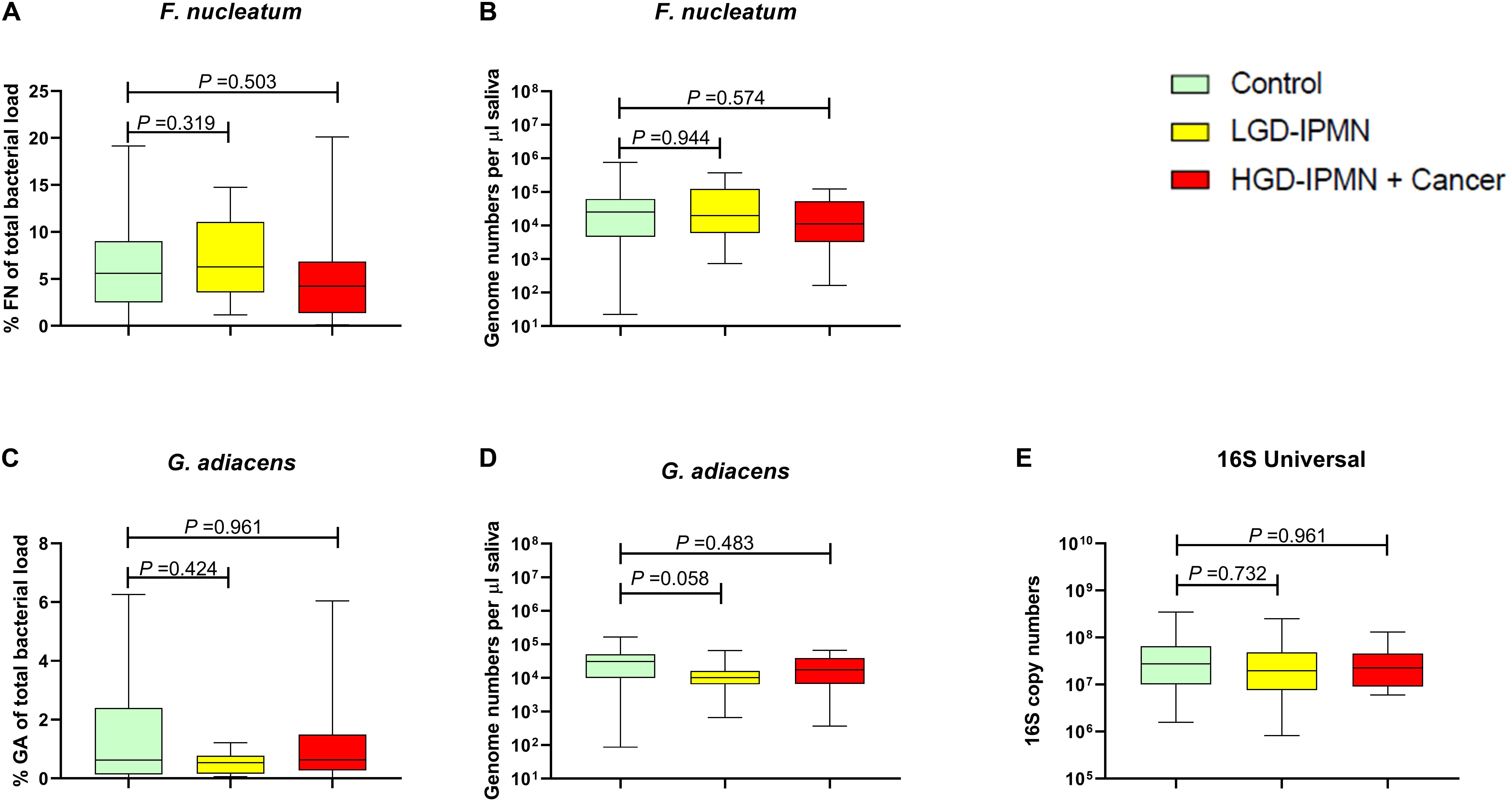
Figure 4. Quantification of F. nucleatum and G. adiancens genome counts in saliva in patients of indicated diagnose group, expressed as relative of total salivary 16S copy count of the sample (A,C), or as per μl of sample (B,D). Total salivary bacterial 16S genome counts in indicated group (E). Statistical analysis was performed using Mann–Whitney U test.
Discussion
We examined humoral responses to selected oral pathogens that have been previously identified intratumorally in PCN microbiota. Our findings indicate that IPMN cases with high-grade dysplasia or that progressed into invasive cancer have ample antibody reactivities to these oral microbes. In particular, F. nucleatum bacteria are recognized by circulating IgG as well as salivary IgA. In this group, salivary IgA reactivity to F. nucleatum was found to extend to the Fap2 immunodominant region of F. nucleatum. The notable positive correlation between F. nucleatum and Fap2 salivary IgA reactivities indicates that the antibodies exist within the same individuals’ saliva. Our data also suggest that they are not only cross-reactive at antigen level but also appear to antagonize, as confirmed by the cross-competition assay. Thus, they should be able to bind and attach to salivary F. nucleatum in the oral cavity, where the bacteria normally reside. It is relevant given that Fap2 is the bacterial outer membrane adhesin employed by F. nucleatum to bind itself to its target, in the tumor microenvironment it has been shown to impair antitumoral NK cell functions and induce lymphocytic apoptosis through TIGIT and CEACAM1 activation. Moreover, Fap2 is also involved in mediating tumor cells attachment by binding to Gal-GalNAc lectin sugar moiety that is overexpressed on tumor cells (31–34). Although secreted mucosal IgA plays a critical role in host defense against pathogenic bacteria, a recent interesting study suggests that gut microbiota may also utilize IgA for mucosal colonization (35), a mechanism that seems to exist in vivo allowing commensal species to exploit IgA specifically to promote its establishment. Surface capsule modified mutants of human commensal Bacteroides fragilis were shown to have this attribute and supported host-microbial symbiosis in experimentally mono-colonized mouse models.
Of note, considering that clinical fusobacteria strains naturally lacking Fap2 may exist and Fap2 expression could be abolished by transposon modifications (31, 34), the differential Fap2 salivary IgA reactivities seen here are therefore intriguing. Further investigation into oral colonization of Fap2-overexpressing strains could perhaps shed more light on this. Moreover, in spite of the correlation between plasma and salivary antibody to F. nucleatum, the plasma antibody reactivity to Fap2 detected in the HGD-IPMN + Cancer group appears as low as other groups. It is not clear if it may block a Fap2-mediated attachment of F. nucleatum in the case of a systemic dissimilation. Furthermore, the HGD-IPMN + Cancer group shows an increased antibody reactivity to S. gordonii in saliva, along with a limited antibody reactivity to G. adiacens in circulation. Although these bacteria were isolated from pancreas, their potential as an oncopathogen has not been described. However, S. gordonii cross-feeding mechanism driving mutualism with F. nucleatum (36) could potentially foster a co-existence and perhaps support immunogenicity of S. gordonii.
In colorectal cancer, circulating IgG antibodies to F. nucleatum and the Fap2 mimotope were recently reported to be increased (22, 23), but a recent European population study questions the diagnostic potential of F. nucleatum proteins in pre-diagnostic serum samples (37). Whether salivary antibody studies could contribute would be interesting to explore. As in colorectal cancer, the oral commensal F. nucleatum in pancreatic cystic fluid has been found to associate with pancreatic malignancy (16, 20). Although F. nucleatum is more renowned as a periodontal pathogen (38) and periodontal disease might increase the risk for PC (39), patients under periodontal treatment seem not to have elevated F. nucleatum antibody levels as compared to periodontally healthy controls (40, 41). In our study, the majority of HGD-IPMN + Cancer patients disclosed no more periodontal conditions than the LGD-IPMN patient group. In spite of this, it is likely that past periodontal insults or dysbiosis are equally important in regulation of immune memory to commensal bacteria. Additional areas to explore include immune memory and tissue resident memory cells (42, 43) that should help gain more understanding into the complex dynamics of immune recognition of cancer-associated commensals in individuals at risk of pancreas malignancies.
Generally, antigens trigger the immune response to secreted antibodies. These humoral antibodies have been widely used in the diagnosis and tumor screening of many diseases. F. nucleatum humoral antibodies have been reported in several studies to be significantly increased in patients with Alzheimer’s disease (44), aortic atherosclerosis (45), chronic bronchitis (46), and colorectal cancer (23). Intriguingly, in line with our observations, plasma antibody responses to F. nucleatum were not significantly showing a difference between pre-diagnosed PC and matched controls (21). The potential of a saliva IgA assay could therefore be further investigated.
Fusobacterium nucleatum has long been considered an opportunistic pathogen, given its frequent isolation and identification in anaerobic samples from patients with different infections. Recently, F. nucleatum has garnered much attention in colorectal cancer microbiome studies (47), and an association between the presence of F. nucleatum and human colorectal cancer has emerged across the tumor stages. Although well known to oral and medical microbiologists and new studies are revealing the intricate ways in which a bacterium can contribute to the development and spread of colorectal cancer and induce resistance to chemotherapy (48–50), the clinical relevance of F. nucleatum in development and prognosis of PC remains to be studied.
Currently, the majority of PCs are diagnosed at a late stage of their natural history when they are symptomatic. Individuals with cystic lesions represent high-risk cohorts which should be entered into surveillance programs, despite the fact that only a subset of them develop PC. Determination of cancer risk biomarkers will require multiple input parameters such as polygenic risk score, BMI, smoking history, as well as other variables (51). Surveillance and diagnosis of asymptomatic PC in longitudinally monitored high-risk cohorts will require biomarkers with discriminating sensitivity and specificity to avoid the risks of under- or over-diagnosis.
The main limitations of this study were the small sample size and having only single blood and saliva measure at one time point. Saliva sampling is presently not a routine element in pancreas oncology programs; therefore, not all patients could donate saliva in this study, though it could be integrated in the near future. However, here the patient subgroups were properly matched with histologically validated LGD-IPMN vs. HGD-IPMN + Cancer cases, which are the two most challenging groups to differentiate in the clinic. Efforts were taken to include an additional baseline control group with bona fide benign pancreas tumors, including paired saliva from orally healthy individuals. Although our results are interesting, and have not been reported earlier to our knowledge, we did not compare the cellular immunity. Intracellular replication is a feature of fusobacteria (52), and this possibility may occur in pancreas tissues; thus, it could be a target for immune killing also in the tumors. Another limitation of this study was the lack of investigation on whether drug use (e.g., antibiotics or NSAID use) may have influenced bacteria antibody levels.
Although our work is restricted to the present microbiota antigen modalities and patient cohort, we believe our results are attractive for the future development of a non-invasive and affordable diagnostic biomarker for patients at risk of PC, for accurate identification and selection of a suitable intervention to approach a cancer cure.
Data Availability Statement
The raw data supporting the conclusions of this article will be made available by the authors, without undue reservation.
Ethics Statement
The studies involving human participants were reviewed and approved by Regionala Etikprövningsnämnden i Stockholm. The patients/participants provided their written informed consent to participate in this study.
Author Contributions
MSC, VÖ, MD, and HA: study design. HA, LL, and GG: sample collection and processing. AH, ZA, CF, HA, and LJ: clinical data collection and interpretation. HA, GG, LL, and VÖ: laboratory work. HA, GG, MJS, and MSC: data analysis and statistics. HA, MSC, GG, MJS, and HD: manuscript preparation. HA, LL, GG, AH, ZA, MJS, HD, CF, LJ, MD, VÖ, and MSC: approval of final draft submission. All authors contributed to the article and approved the submitted version.
Funding
This work was supported by the Swedish Cancer Society (CAN2016/731 and 19 0495 Pj 01 H to MC; CAN 2017/409 and CAN 2017/419 to MD), Karolinska Institutet funds, SOF Strategic Odontological Research, Radiumhemmets forskningsfonder to MC, and ALF SLL20150113 to MD.
Conflict of Interest
The authors declare that the research was conducted in the absence of any commercial or financial relationships that could be construed as a potential conflict of interest.
Acknowledgments
We are thankful to all participants for making this study possible. We would also like to thank Maura Krook at Karolinska University Hospital for organizing patient recruitment.
Supplementary Material
The Supplementary Material for this article can be found online at: https://www.frontiersin.org/articles/10.3389/fimmu.2020.02003/full#supplementary-material
References
1. Siegel RL, Miller KD, Jemal A. Cancer statistics, 2017. CA Cancer J Clin. (2017) 67:7–30. doi: 10.3322/caac.21387
2. Torre LA, Bray F, Siegel RL, Ferlay J, Lortet-Tieulent J, Jemal A. Global cancer statistics, 2012. CA Cancer J Clin. (2015) 65:87–108. doi: 10.3322/caac.21262
3. Rahib L, Smith BD, Aizenberg R, Rosenzweig AB, Fleshman JM, Matrisian LM. Projecting cancer incidence and deaths to 2030: the unexpected burden of thyroid, liver, and pancreas cancers in the united states. Cancer Res. (2014) 74:2913–21.
4. Kamisawa T, Wood LD, Itoi T, Takaori K. Pancreatic cancer. Lancet. (2016) 388:73–85. doi: 10.1016/S0140-6736(16)00141-0
5. Del Chiaro M, Besselink MG, Scholten L, Bruno MJ, Cahen DL, Gress TM, et al. European evidence-based guidelines on pancreatic cystic neoplasms. Gut. (2018) 67:789–804. doi: 10.1136/gutjnl-2018-316027
6. de Jong K, Nio CY, Hermans JJ, Dijkgraaf MG, Gouma DJ, van Eijck CHJ, et al. High prevalence of pancreatic cysts detected by screening magnetic resonance imaging examinations. Clin Gastroenterol Hepatol. (2010) 8:806–11. doi: 10.1016/j.cgh.2010.05.017
7. Laffan TA, Horton KM, Klein AP, Berlanstein B, Siegelman SS, Kawamoto S, et al. Prevalence of unsuspected pancreatic cysts on MDCT. Am J Roentgenol. (2008) 191:802–7.
8. Valsangkar NP, Morales-Oyarvide V, Thayer SP, Ferrone CR, Wargo JA, Warshaw AL, et al. 851 resected cystic tumors of the pancreas: a 33-year experience at the Massachusetts General Hospital. Surgery. (2012) 152(3 Suppl.):4–12.
9. Aronsson L, Andersson R, Ansari D. Intraductal papillary mucinous neoplasm of the pancreas–epidemiology, risk factors, diagnosis, and management. Scand J Gastroenterol. (2017) 52:803–15.
10. Tanaka M, Fernández-Del Castillo C, Adsay V, Chari S, Falconi M, Jang JY, et al. International consensus guidelines 2012 for the management of IPMN and MCN of the pancreas. Pancreatology. (2012) 12:183–97. doi: 10.1016/j.pan.2012.04.004
11. Dumlu EG, Karakoç D, Özdemir A. Intraductal papillary mucinous neoplasm of the pancreas: current perspectives. Int Surg. (2015) 100:1060–8.
13. Pushalkar S, Hundeyin M, Daley D, Zambirinis CP, Kurz E, Mishra A, et al. The pancreatic cancer microbiome promotes oncogenesis by induction of innate and adaptive immune suppression. Cancer Discov. (2018) 8:403–16. doi: 10.1158/2159-8290.CD-17-1134
14. Fukugaiti MH, Ignacio A, Fernandes MR, Ribeiro U, Nakano V, Avila-Campos MJ. High occurrence of Fusobacterium nucleatum and clostridium difficile in the intestinal microbiota of colorectal carcinoma patients. Braz J Microbiol. (2015) 46:1135–40.
15. Warren RL, Freeman DJ, Pleasance S, Watson P, Moore RA, Cochrane K, et al. Co-occurrence of anaerobic bacteria in colorectal carcinomas. Microbiome. (2013) 1:16. doi: 10.1186/2049-2618-1-16
16. Gaiser RA, Halimi A, Alkharaan H, Lu L, Davanian H, Healy K, et al. Enrichment of oral microbiota in early cystic precursors to invasive pancreatic cancer. Gut. (2019) 68:2186–94. doi: 10.1136/gutjnl-2018-317458
17. Kostic AD, Gevers D, Pedamallu CS, Michaud M, Duke F, Earl AM, et al. Genomic analysis identifies association of Fusobacterium with colorectal carcinoma. Genome Res. (2012) 22:292–8.
18. Chen S, Su T, Zhang Y, Lee A, He J, Ge Q, et al. Fusobacterium nucleatum promotes colorectal cancer metastasis by modulating KRT7-AS/KRT7. Gut Microbes. (2020) 11:511–25. doi: 10.1080/19490976.2019.1695494
19. Wong SH, Yu J. Gut microbiota in colorectal cancer: mechanisms of action and clinical applications. Nat Rev Gastroenterol Hepatol. (2019) 16:690–704. doi: 10.1038/s41575-019-0209-8
20. Mitsuhashi K, Nosho K, Sukawa Y, Matsunaga Y, Ito M, Kurihara H, et al. Association of Fusobacterium species in pancreatic cancer tissues with molecular features and prognosis. Oncotarget. (2015) 6:7209–20.
21. Michaud DS, Izard J, Wilhelm-Benartzi CS, You DH, Grote VA, Tjønneland A, et al. Plasma antibodies to oral bacteria and risk of pancreatic cancer in a large European prospective cohort study. Gut. (2013) 62:1764–70.
22. Guevarra LA, Afable ACF, Belza PJO, Dy KJS, Lee SJQ, Sy-Ortin TT, et al. Immunogenicity of a Fap2 peptide mimotope of Fusobacterium nucleatum and its potential use in the diagnosis of colorectal cancer. Infect Agent Cancer. (2018) 13:1–6.
23. Wang HF, Li LF, Guo SH, Zeng QY, Ning F, Liu WL, et al. Evaluation of antibody level against Fusobacterium nucleatum in the serological diagnosis of colorectal cancer. Sci Rep. (2016) 6:1–10. doi: 10.1038/srep33440
24. Eke PI, Thornton-Evans G, Dye B, Genco R. Advances in surveillance of periodontitis: the centers for disease control and prevention periodontal disease surveillance project. J Periodontol. (2012) 83:1337–42. doi: 10.1902/jop.2012.110676
25. Eke PI, Dye BA, Wei L, Slade GD, Thornton-Evans GO, Beck JD, et al. Self-reported measures for surveillance of periodontitis. J Dent Res. (2013) 92:1041–7. doi: 10.1177/0022034513505621
26. Eke PI, Dye BA, Wei L, Thornton-Evans GO, Genco RJ. Prevalence of periodontitis in adults in the united states: 2009 and 2010. J Dent Res. (2012) 91:914–20.
27. Pessi T, Karhunen V, Karjalainen PP, Ylitalo A, Airaksinen JK, Niemi M, et al. Bacterial signatures in thrombus aspirates of patients with myocardial infarction. Circulation. (2013) 127:1219–28.
28. Farrell JJ, Zhang L, Zhou H, Chia D, Elashoff D, Akin D, et al. Variations of oral microbiota are associated with pancreatic diseases including pancreatic cancer. Gut. (2012) 61:582–8. doi: 10.1136/gutjnl-2011-300784
29. Yang S, Lin S, Kelen DG, Quinn CT, Dick DJ, Gaydos AC, et al. Quantitative multiprobe PCR assay for the simultaneous detection and species identification of bacterial pathogens. J Clin Microbiol. (2002) 40:3449–54.
30. Frey A, Di J, Zurakowski D. A statistically defined endpoint titer determination method for immunoassays. J Immunol Methods. (1998) 221:35–41. doi: 10.1016/s0022-1759(98)00170-7
31. Coppenhagen-Glazer S, Sol A, Abed J, Naor R, Zhang X, Han YW, et al. Fap2 of Fusobacterium nucleatum is a galactose-inhibitable adhesin involved in coaggregation, cell adhesion, and preterm birth. Infect Immun. (2015) 83:1104–13.
32. Gur C, Ibrahim Y, Isaacson B, Yamin R, Abed J, Gamliel M, et al. Binding of the Fap2 protein of Fusobacterium nucleatum to human inhibitory receptor TIGIT protects tumors from immune cell attack. Immunity. (2015) 42:344–55.
33. Gur C, Maalouf N, Shhadeh A, Berhani O, Singer BB, Bachrach G, et al. Fusobacterium nucleatum supresses anti-tumor immunity by activating CEACAM1. Oncoimmunology. (2019) 8:1–6. doi: 10.1080/2162402X.2019.1581531
34. Abed J, Emgård JEM, Zamir G, Faroja M, Almogy G, Grenov A, et al. Fap2 Mediates Fusobacterium nucleatum colorectal adenocarcinoma enrichment by binding to tumor-expressed Gal-GalNAc. Cell Host Microbe. (2016) 20:215–25.
35. Donaldson GP, Ladinsky MS, Yu KB, Sanders JG, Yoo BB, Conner ME, et al. Gut microbiota utilize immunoglobulin a for mucosal colonization. Science. (2018) 360:795–800.
36. Sakanaka A, Kuboniwa M, Takeuchi H, Hashino E, Amano A. Arginine-ornithine antiporter ArcD controls arginine metabolism and interspecies biofilm development of Streptococcus gordonii. J Biol Chem. (2015) 290: 21185–98.
37. Butt J, Jenab M, Pawlita M, Overvad K, Tjonneland A, Olsen A, et al. Antibody responses to Fusobacterium nucleatum proteins in prediagnostic blood samples are not associated with risk of developing colorectal cancer. Cancer Epidemiol Biomarkers Prev. (2019) 28: 1552–5.
38. Bolstad AI, Jensen HB, Bakken V. Taxonomy, biology, and periodontal aspects of Fusobacterium nucleatum. Clin Microbiol Rev. (1996) 9:55–71.
39. Michaud DS, Joshipura K, Giovannucci E, Fuchs CS. A prospective study of periodontal disease and pancreatic cancer in US male health professionals. J Natl Cancer Inst. (2007) 99:171–5.
40. Doty SL, Lopatin DE, Syed SA, Smith FN. Humoral immune response to oral microorganisms in periodontitis. Infect Immun. (1982) 37: 499–505.
41. Shin J, Kho SA, Choi YS, Kim YC, Rhyu IC, Choi Y. Antibody and T cell responses to Fusobacterium nucleatum and treponema denticola in health and chronic periodontitis. PLoS One. (2013) 8:e53703. doi: 10.1371/journal.pone.0053703
42. Sorini C, Cardoso RF, Gagliani N, Villablanca EJ. Commensal bacteria-specific CD4+T cell responses in health and disease. Front Immunol. (2018) 9:2667. doi: 10.3389/fimmu.2018.02667
43. Hegazy AN, West NR, Stubbington MJT, Wendt E, Suijker KIM, Datsi A, et al. Circulating and tissue-resident CD4+ T cells with reactivity to intestinal microbiota are abundant in healthy individuals and function is altered during inflammation. Gastroenterology. (2017) 153:1320– 37.e16.
44. Sparks Stein P, Steffen MJ, Smith C, Jicha G, Ebersole JL, Abner E, et al. Serum antibodies to periodontal pathogens are a risk factor for Alzheimer’s disease. Alzheimers Dement. (2012) 8:196–203.
45. Velsko IM, Chukkapalli SS, Rivera-Kweh MF, Chen H, Zheng D, Bhattacharyya I, et al. Fusobacterium nucleatum alters atherosclerosis risk factors and enhances inflammatory markers with an atheroprotective immune response in ApoE null mice. PLoS One. (2015) 10:e0129795. doi: 10.1371/journal.pone.0129795
46. Brook I, Frazier EH. Immune response to Fusobacterium nucleatum and prevotella intermedia in the sputum of patients with acute exacerbation of chronic bronchitis. Chest. (2003) 124:832–3. doi: 10.1378/chest.124.3.832
47. Brennan CA, Garrett WS. Fusobacterium nucleatum – symbiont, opportunist and oncobacterium. Nat Rev Microbiol. (2019) 17:156–66. doi: 10.1038/s41579-018-0129-6
48. Zhang S, Wang Q, Zhou C, Chen K, Chang H, Xiao W, et al. Colorectal cancer, radiotherapy and gut microbiota. Chinese J Cancer Res. (2019) 31:212–22.
49. Chen J, Pitmon E, Wang K. Microbiome, inflammation and colorectal cancer. Semin Immunol. (2017) 32:43–53.
50. Helmink BA, Khan MAW, Hermann A, Gopalakrishnan V, Wargo JA. The microbiome, cancer, and cancer therapy. Nat Med. (2019) 25:377–88.
51. Singhi AD, Koay EJ, Chari ST, Maitra A. Early detection of pancreatic cancer: opportunities and challenges. Gastroenterology. (2019) 156:2024–40. doi: 10.1053/j.gastro.2019.01.259
Keywords: pancreatic cancer, pancreatic cyst neoplasm, IPMN, oral commensals, humoral response
Citation: Alkharaan H, Lu L, Gabarrini G, Halimi A, Ateeb Z, Sobkowiak MJ, Davanian H, Fernández Moro C, Jansson L, Del Chiaro M, Özenci V and Sällberg Chen M (2020) Circulating and Salivary Antibodies to Fusobacterium nucleatum Are Associated With Cystic Pancreatic Neoplasm Malignancy. Front. Immunol. 11:2003. doi: 10.3389/fimmu.2020.02003
Received: 15 April 2020; Accepted: 24 July 2020;
Published: 28 August 2020.
Edited by:
Andrea Facciabene, University of Pennsylvania, United StatesReviewed by:
Bo Shen, The University of Texas Southwestern Medical Center, United StatesKarl O. A. Yu, University at Buffalo, United States
Copyright © 2020 Alkharaan, Lu, Gabarrini, Halimi, Ateeb, Sobkowiak, Davanian, Fernández Moro, Jansson, Del Chiaro, Özenci and Sällberg Chen. This is an open-access article distributed under the terms of the Creative Commons Attribution License (CC BY). The use, distribution or reproduction in other forums is permitted, provided the original author(s) and the copyright owner(s) are credited and that the original publication in this journal is cited, in accordance with accepted academic practice. No use, distribution or reproduction is permitted which does not comply with these terms.
*Correspondence: Margaret Sällberg Chen, TWFyZ2FyZXQuQ2hlbkBraS5zZQ==