- 1Programa de Pós-Graduação em Genética, Departamento de Genética, Universidade Federal do Paraná, Curitiba, Brazil
- 2Department of Neurology, Weill Institute for Neurosciences, University of California, San Francisco, San Francisco, CA, United States
Regulation of NK cell activity is mediated through killer-cell immunoglobulin-like receptors (KIR) ability to recognize human leukocyte antigen (HLA) class I molecules as ligands. Interaction of KIR and HLA is implicated in viral infections, autoimmunity, and reproduction and there is growing evidence of the coevolution of these two independently segregating gene families. By leveraging KIR and HLA-C data from 1000 Genomes consortium we observed that the KIR2DL1 variant rs2304224*T is associated with lower expression of HLA-C in individuals carrying the ligand HLA-C2 (p = 0.0059). Using flow cytometry, we demonstrated that this variant is also associated with higher expression of KIR2DL1 on the NK cell surface (p = 0.0002). Next, we applied next generation sequencing to analyze KIR2DL1 sequence variation in 109 Euro and 75 Japanese descendants. Analyzing the extended haplotype homozygosity, we show signals of positive selection for rs4806553*G and rs687000*G, which are in linkage disequilibrium with rs2304224*T. Our results suggest that lower expression of HLA-C2 ligands might be compensated for higher expression of the receptor KIR2DL1 and bring new insights into the coevolution of KIR and HLA.
Introduction
The killer cell immunoglobulin-like receptor (KIR) genes on chromosome 19 encode receptors that interact with a subset of human leukocyte antigen (HLA) class I molecules, encoded by genes on chromosome 6, to regulate NK cell cytotoxicity against infected and neoplastic cells (1–3). In fact, combinations of variants of KIR and HLA have been repeatedly associated with autoimmune disease (4–6), cancer (7, 8), viral infections (9, 10), and are also implicated in reproduction (11–14). As a result, the interaction of KIR and HLA is relevant to fitness and survival and candidate for evolutionary studies (15).
KIR recognize subsets of HLA-A (A3, A11, and Bw4), HLA-B (Bw4 and Bw6), and HLA-C (C1 and C2) molecules (16). HLA-C appears to have had a great impact on KIR evolution, driving the expansion of lineage III KIR, which are the receptor lineage that recognize HLA-C (17, 18). The dimorphism in position 80 of HLA-C defines HLA-C1 (80Asn) and HLA-C2 (80Lys) and confers differential specificity to KIR. Among all ligands, the interaction between KIR2DL1 and HLA-C2 is responsible for the strongest regulatory signal and HLA-C seems to act as the main educator of NK cells (19, 20).
Worldwide studies demonstrate coordinated frequencies of KIR and HLA in populations. In a comprehensive study consisting of 30 populations, Single et al. (21) found that increasing frequencies of activating KIR are correlated with decreased frequencies of their respective HLA ligands. On the other hand, Hollenbach et al. (22) showed positive correlation between the presence of KIR2DL3 and the presence of HLA-C1 in 105 worldwide populations. A strong and negative correlation of KIR gene-content haplotype A and HLA-C2, a pair which is associated with increased risk of pre-eclampsia, was found in eight populations from European, African, and Asian ancestries (11). Moreover, there is extensive evidence of balancing selection maintaining diversity in KIR genes (23–25). KIR and HLA segregate independently and there are no reports of gametic association between these two gene families. Here, we show that a single nucleotide polymorphism (SNP) in KIR2DL1 is associated with expression levels of the KIR2DL1 receptor on the cell surface and also with HLA-C expression.
Results
KIR2DL1 Variant rs2304224*T Is Associated With Lower Expression Levels of HLA-C
To search for possible signals of coevolution between KIR and HLA, we evaluated if variants in inhibitory KIR that bind to HLA-C could be associated with HLA-C expression levels in global populations. We leveraged the public sequencing information available for all populations in the 1000 Genomes Project (1KGP) (26) and retrieved the genotypic data available for SNPs located within KIR2DL1 and KIR2DL23 (rs2304224, rs11673144, rs12982263, rs34721508, rs35719984, and rs35861855) in 955 individuals of various ancestries. We also obtained HLA genotyping data available for those individuals (27).
Subsequently, we used previously published data of HLA-C expression levels (28) and imputed the expression for each HLA-C genotype in the 1KGP cohort. The variant rs2304224*T was associated with lower HLA-C expression levels in individuals HLA-C1/C2 (p = 0.0420) and HLA-C2/C2 (p = 0.0059), but not in HLA-C1/C1 individuals (p = 0.0740; Figure 1A and Supplementary Figure 1). This variant is in position 13 of exon 1 and causes a phenylalanine to valine change in the KIR2DL1 signal peptide. We replicated these results by imputing the HLA expression in an independent panel of 308 Brazilians Euro-descendants for which HLA genotyping data was available, and we sequenced the first exon of KIR2DL1 to genotype rs2304224 (p = 0.0107; Figure 1B).
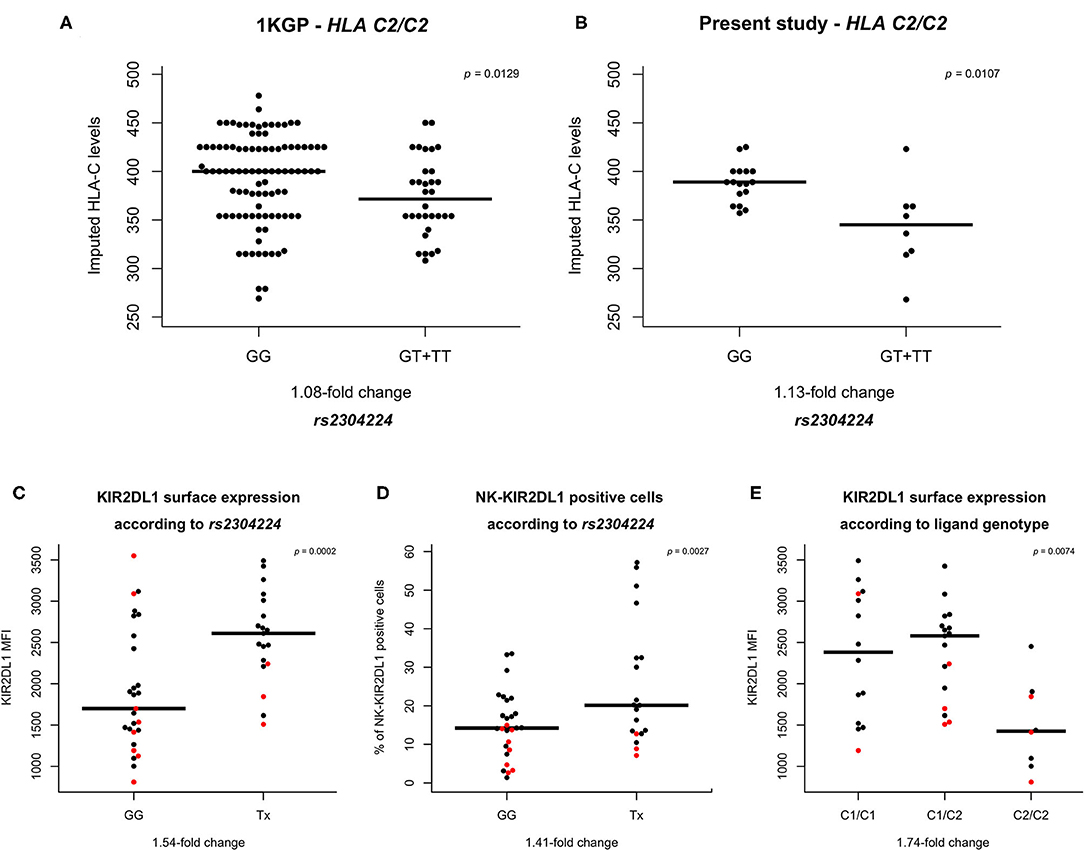
Figure 1. HLA-C and KIR2DL1 expression are associated with genetic variants. (A,B) rs2304224 in KIR2DL1 marks in silico HLA-C surface expression (28) in two different cohorts. The presence of allele rs2304224*T marks lower HLA-C expression in (A) 130 C2/C2 homozygotes out of 955 individuals from 1000 genomes consortium and (B) 25 C2/C2 homozygotes out of 308 Euro-Brazilians from Curitiba (present study). (C) Higher KIR2DL1 surface expression and (D) increased presence on NK cells are also associated with the variant rs2304224*T (p = 0.0002 and p = 0.0027, respectively). (E) HLA-C genotype is associated to KIR2DL1 surface expression (p = 0.0074). There is no difference in expression, however, between homozygotes C1/C1 and heterozygotes C1/C2 (p = 0.44). Homozygosity for C2/C2, on the other hand, is associated with lower KIR2DL1 surface expression than in C1/C1 (p = 0.0031) and C1/C2 (p = 0.0016). Each dot in the graphs represents one individual. Red dots indicate hemizygosity for KIR2DL1. Median values are shown in horizontal lines and statistical significance is indicated in the top right corners of each plot.
To demonstrate that our approach to impute the HLA-C expression is predictive of the cell surface expression in vivo, we measured the HLA-C surface levels of fresh CD3+ cells in 30 individuals using flow cytometry and compared to the imputed values. We found a correlation of r = 0.62, p < 0.0001 (Supplementary Figure 2).
rs2304224*T Is also Associated With Higher Surface Expression Levels of KIR2DL1
We sought to investigate if the variant rs2304224*T in KIR2DL1 was associated with KIR2DL1 surface expression. We used flow cytometry to quantify both the abundance of KIR2DL1 on the surface of NK cells (median fluorescence intensity, MFI) as well as the percentage of NK cells expressing KIR2DL1 on their surface (KIR2DL1+ NK cell), and also interrogated if copy number variation of KIR2DL1 affects surface expression. Although borderline, we did not find significant differences of expression levels in individuals carrying one copy (hemizygous) or two copies (homo- or heterozygous) of KIR2DL1+ (p = 0.0594; Supplementary Figure 3A). However, the number of KIR2DL1+ NK cells was 2.16-fold higher in individuals carrying two copies (p = 0.0001; Supplementary Figure 3B). For all KIR2DL1 expression analyses, we used copy number of KIR2DL1 as covariant in the regression model.
We observed that the allele rs2304224*T, associated with decreased HLA-C expression, was also associated with 1.54-fold increase of the KIR2DL1 surface expression (p = 0.0002) and a 1.41-fold increase of KIR2DL1+ NK cells (p = 0.03; Figures 1C,D). The median expression of each KIR allotype is shown in Supplementary Figure 4. We also observed that KIR2DL1 expression was decreased in individuals homozygous for the presence of the C2 ligand (C2/C2, p = 0.007; Figure 1E).
Signals of Positive Selection for KIR2DL1 Variants in Linkage Disequilibrium With rs2304224
We next analyzed the entire KIR2DL1 gene in a subset of 109 Euro-descendants and 75 Japanese descendants sequenced using our custom next generation sequencing method (29). In Euro descendants, we observed low correlation but strong linkage disequilibrium (LD) between rs2304224 and three other variants (Supplementary Figures 5A,B). The first variant is at position −406 upstream of the KIR2DL1 gene (rs4806553, D′ = 0.99, r2 = 0.18, p < 10−8). The other variants are located within the coding region, in exon 4 (rs687000, D′ = 0.99, r2 = 0.52, p < 10−12) and exon 7 (rs34721508, D′ = 0.99, r2 = 0.24, p < 10−3). Weaker LD was observed for the same variants in Japanese descendants (Supplementary Figures 5C,D). Frequencies for all SNPs in both populations are given in Supplementary Table 1. Moreover, the frequency of HLA-C2 in our Japanese-descendant cohort was 10.3% while in Euro-descendants it was 40.9%.
We searched for signals of population specific selection, for both Euro and Japanese descendants, by estimating the extended haplotype homozygosity (EHH) using rs2304224 and also variants in significant LD with it as focal SNPs. The bifurcation patterns are consistent with positive selection increasing frequencies of the haplotype more rapidly than they could be broken by genetic recombination. Signals of positive selection were observed for the derived allele rs4806553*G in Japanese but not in Euro-descendants (Figures 2A,C). Strong signals of positive selection were also observed for the derived allele rs687000*G in both Euro and Japanese descendants (Figures 2B,D).
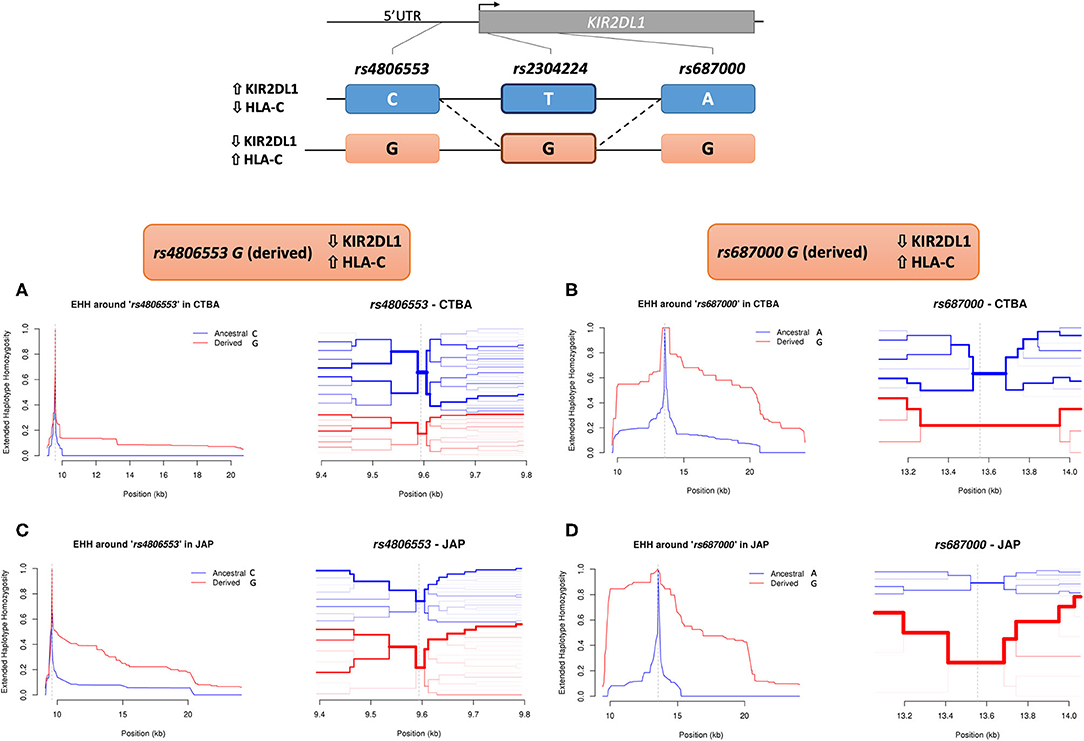
Figure 2. Extended haplotype homozygosity (EHH) in KIR2DL1. The extended homozygosity analysis is based on the premise that advantageous alleles increase in frequency at a higher pace than the local recombination rate breaks down the haplotypes in which these alleles are located. Therefore, alleles marking regions with elevated extended homozygosity are possibly under recent positive selection. Here we identify extended haplotypes surrounding KIR2DL1 variants rs4806553 and rs687000. The possible haplotypes of rs4806553 and rs687000 in relation to rs2304224 are represented at the top of the image. The continuous line represents the most common configuration between two variants, and the dashed line represent less frequent configurations. On the left of each haplotype, arrows indicate higher or lower expression of KIR2DL1 and HLA-C, as associated with rs2304224 alleles G or T. A representation of the genomic organization of KIR2DL1 with the indicated location of the three variants is represented above. (A) EHH graph of decay in homozygosity (left) and furcation plot (right) for rs4806553 in Euro-Brazilians. The graph shows little to no difference between ancestral rs4806553*C (blue) and derived rs4806553*G (red) alleles in Euro-Brazilians. (C) EHH graph of decay in homozygosity (left) and furcation plot (right) for rs4806553 in Japanese. In Japanese, elevated homozygosity is associated with derived allele rs4806553*G (red). (B) EHH graph of decay in homozygosity (left) and furcation plot (right) for rs687000 in Brazilians with European ancestry and (D) Brazilians with Japanese ancestry. Elevated homozygosity associated with derived allele rs687000*G (red) is consistent with the selective sweep model, in which recent positive selection sweeps the diversity on nearby loci. Vertical dotted lines indicate the position of the core SNP. The thickness of each branch in the furcation plot is determined by haplotype frequency.
Discussion
Previous results show that cis polymorphisms associated with HLA-C expression do not associate with NK cell activity (30), despite the compelling evidence that KIR-HLA are coevolving as an integrated system (11, 16, 21, 22). Here, we show evidence of coevolution of KIR and HLA by identifying a variant in KIR2DL1 that was associated with surface expression of the ligand HLA-C2 in worldwide populations. The allele rs2304224*T was associated with lower expression of imputed HLA-C surface expression in 995 individuals from 1KGP and also in an independent cohort of 308 Brazilian Euro-descendants. The association was only observed in individuals carrying at least one copy of HLA-C2, which suggests an orchestrated and refined evolution between these two systems. Although the antibody used in this study (DT9) cross reacts with HLA-E, it has been demonstrated that its binding represents the surface expression of HLA-C (28, 31) and also is correlated with mRNA expression levels of HLA-C measured by quantitative PCR (32). Therefore, our direct measurement of HLA-C expression in 30 individuals demonstrates that imputing HLA expression based on previously published data is predictive of the expression observed on the surface of fresh blood cells.
It is also interesting that the same allele rs2304224*T is associated with higher expression of the receptor KIR2DL1 in NK cells and also present in the high expressing KIR2DL1*002. The SNP rs2304224 in exon 1 causes a non-synonymous substitution of valine (allele G) to phenylalanine (allele T) in the signal peptide. The hydrophobicity of the signal peptide can influence protein retention in the cytosol (33). According to the Wimley-White interfacial hydrophobicity scale (34), valine has a free energy of transfer of 0.07 ΔG from water to bilayer, and the free energy of phenylalanine is −1.13 ΔG. The lower and negative value of phenylalanine indicates this transference is more favorable, and therefore, rs2304224*T may increase protein availability in the membrane. This could explain the increased KIR2DL1 expression associated with rs2304224*T.
The patterns that we observed for the expression of KIR2DL1 allotypes (Supplementary Figure 4) are consistent with previous studies (20, 35–37). Our results showing that copy number of KIR2DL1 affects the quantity of KIR2DL1+ NK cells corroborate those by Béziat et al. (37). On the other hand, the lack of significant association that we observed between KIR2DL1 copy number and the abundance of expression on the cell surface reinforces the idea that copy number does not affect levels of KIR2DL1 as strongly as it affects the proportion of cells expressing the receptor (37). The presence of HLA-C2 was associated with lower expression of surface KIR2DL1, according to our results and of others (35, 38, 39). However, differently from the observations from Le Luduec et al. (38), who observed that the expression of KIR2DL1 is associated to the presence of C2 in a dose dependent manner, we found association only in individuals carrying two copies of C2.
We found three SNPs in LD with rs2304224 (D′ = 0.99, 0.18≤ r2 ≤0.51). The low correlation coefficient is explained by difference in the allele frequencies among them. The frequency of the variant rs2304224*T is 0.26 in Euro-Brazilians, while the frequency of rs4806553*C is 0.67; rs687000*A is 0.57; and rs34721508*C is 0.86. From the three variants in LD with rs2304224, only rs34721508, in exon 7, has been previously associated with differential expression levels of KIR2DL1 in transfected cell lines (36). That study showed that cells expressing allotypes with 245Cys have reduced protein stability and are more susceptible to ligand mediated expression down-regulation in comparison to those with 245Arg. Interestingly, this variant was also present in the 1KGP dataset, and we did not observe association of rs34721508 genotypes with HLA-C imputed expression levels (p = 0.28). We also demonstrated that there is an additive effect of rs2304224*T and rs34721508*C on KIR2DL1 expression, which indicates that each has independent effect on the expression of KIR2DL1 (Supplementary Figure 6), despite the fact that both these variants are present in the high expressing KIR2DL1*002 (Supplementary Table 1). This observation argues in favor of our approach to expand the analysis of individual SNPs rather than solely analyzing the common combinations of SNPs present in the most frequent KIR2DL1 alleles.
We applied extended haplotype homozygosity (EHH) analysis to all SNPs in LD with rs2304224, using the next generation sequencing data that we generated for a subset of Euro and Japanese descendants. Homozygosity surrounding the derived allele rs4806553*G was prominent in the Japanese population, suggesting this allele has been under recent positive selection. Japanese populations are especially interesting because they exhibit the lowest frequency of the HLA-C2 allotype (only 8%) (40) and, accordingly, we report low frequency of C2 also in the Brazilians of Japanese ancestry (10.3%). The low frequency of HLA-C2 could be driving the evolution of KIR2DL1 in the Japanese population.
The SNP rs4806553 is located 406 kbp upstream of the KIR2DL1 gene, in the sequence corresponding to its intermediate promoter (Pro-I), suggested to control protein expression in mature NK cells (41). Moreover, it has been shown that the Pro-I sequence containing allele rs4806553*C binds to the transcription factor activator protein-1 (AP1), while rs4806553*G abrogates this binding (42). This could potentially explain the higher expression of KIR2DL1*002, which contains allele rs4806553*C, in comparison to other KIR2DL1 alleles carrying the variant rs4806553*G, such as KIR2DL1*004 and KIR2DL1*006 (Supplementary Figure 4 and Supplementary Table 1). Our data suggests that the attenuation of NK inhibition mediated by KIR2DL1 represents an evolutionary advantage and is being favored by positive selection in the Japanese population.
Strong signals of positive selection were observed toward the derived allele rs687000*G in both our cohorts. This variant is a synonymous change in exon 4, without apparent impact on regulation of KIR2DL1 expression. One hypothesis is that rs687000*G rose in frequency due to hitchhiking with a nearby variation that was positively selected and eventually fixed. We did not observe signals of positive selection for rs2304224 and rs34721508, which strongly associate with KIR2DL1 expression levels. One possibility is that selection could be favoring specific KIR2DL1 alleles that carry these variants. In fact, the combination of rs2304224*G (neutral), rs687000*G (positively selected), and rs34721508*C (neutral) defines KIR2DL1*003, the most frequent allele across all populations worldwide (43).
Coevolution of KIR and HLA is mostly driven by HLA-C (20, 44), which encodes a strong educator for KIR+ NK cells (45, 46). A fine tuning mechanism of NK cell regulation through the cell-specific promoter NK-Pro (47) was recently proposed, in which expression levels of HLA-C during NK cell education combines with expression levels and interaction strength of KIR and HLA in mature NK cells to modulate their selectivity and cytotoxicity (48). KIR2DL1 is the receptor with the highest affinity and avidity to HLA-C, and mediates the strongest NK response (19, 20, 49). Therefore, it is plausible that variation in KIR2DL1 could be under selection and also that KIR2DL1 and HLA-C are coevolving. Here, we show a KIR2DL1 variant that is associated with lower expression of KIR2DL1 and inversely associated with higher HLA-C expression in HLA-C2/C2 individuals. This could be an indication that higher levels of the ligand are being compensated by lower expression of the receptor. We also observed evidence of positive selection on KIR2DL1. Our data show that much remains to be understood regarding the mechanisms of the KIR-HLA recognition and evolution. They also bring insights into the evolution of these two systems and suggest that more questions will emerge as we explore more deeply KIR-HLA diversity at high resolution.
Materials and Methods
Samples
We analyzed a cohort of 308 individuals of predominantly European ancestry and 75 individuals of Japanese ancestry from Curitiba, Brazil. About 80% of the population from Curitiba self-reported as Euro-descendant (50), which is in accordance with previous genetic studies (51). For the Japanese descendants, we only included individuals who had two parents or four grandparents born in Japan, with no history of admixture with non-Japanese ancestries. In order to measure KIR2DL1 expression levels, we analyzed fresh blood cells from a subset of 48 Euro-descendants. A subset of 30 of these individuals were included in the HLA-C expression assay. Detailed information about the study design is given in Supplementary Figure 7. All individuals were living in Curitiba, Brazil, at the time of blood collection. Median age in the group was 26 years (ranging from 20 to 64) and the male/female ratio was 0.37.
For expression assays, we collected 8 mL of peripheral blood samples and isolated PBMC (peripheral blood mononuclear cells) using LeucosepTM tubes (Greiner Bio-One, Austria), which have a selective membrane for density-based lymphocyte separation, and Ficoll Hypaque (Sigma Aldrich, MO). Isolated PBMC were counted in a Neubauer chamber under an optical microscope. A total of 0.5 × 106 cells were incubated with specific antibodies for KIR2DL1 and HLA-C and analyzed by flow cytometry. Detailed description and gate strategy are shown in Supplementary Figure 8.
KIR2DL1 and HLA-C Genotyping
We initially sequenced exons 1, 4, 5, 7, and 9 to distinguish the main KIR2DL1 allele groups using the Sanger method (52) in the 48 Euro-descendants included in the expression assay (Supplementary Figure 9). The sequences obtained were aligned with reference sequences from IPD-KIR database (43), using the software Mutation Surveyor® (SoftGenetics, PA) and identified manually. Additionally, we sequenced only the exon 1 (containing the variant rs2304224) in extra 260 Euro-descendant individuals to increase statistical power for the analysis of rs2304224.
We applied quantitative PCR to determine copy number of KIR2DL1 compared to KIR3DL3, which is present in virtually all haplotypes. KIR2DL1 was amplified in triplicate using one set of primers and the reference gene KIR3DL3 was amplified using other three sets of primers, each in triplicate, in a total of 12 (4 × 3) reactions per sample. The sequence of all primers used for amplification, sequencing and copy number assay, including those designed in this study as well as those described previously (53–57) are given in Supplementary Table 2.
We also sequenced the entire KIR2DL1 gene in 109 Euro-descendants and 75 Japanese descendants from Curitiba, Brazil. These samples were sequenced using the previously published method for next generation sequencing of KIR and HLA genes (29) using Illumina platform.
Data Analysis
Normality of variables was tested using Kolmogorov-Smirnov test, in R package nortest (58). Difference in HLA-C expression between KIR2DL1 SNP genotypes was tested via the Kruskal-Wallis test, using stats R (59). Post-hoc analysis of Dunn was applied to Kruskal-Wallis results in order to identify pair-wise significant differences between genotypes, in R package dunn.test (60). Median HLA-C expression by allele, as defined by Apps et al. (28), was imputed for each allele in an individual, and then summed. The imputation was performed in all 308 Brazilians of European ancestry sequenced for rs2304224 and 1KGP individuals. Correlation analysis between expected HLA-C expression in CD3+ cells and in vivo HLA-C expression in CD3+ cells was calculated with R package Hmisc (61). Difference in KIR2DL1 expression according to copy number was tested using Mann-Whitney, in stats R (59). Association of KIR2DL1 expression with allotype and rs2304224 was tested through logistic regression using copy number as a covariate, also in stats R. Linkage disequilibrium was estimated using LD function from R package genetics (62) and plotted with a modified version of R package LDheatmap (63). Median expression graphs were plotted using base and beeswarm R packages (59, 64).
KIR2DL1 SNPs obtained from genomic sequence data were phased using fastPHASE, with modified parameters (-T10 -H200). The phased data was used for estimation of extended haplotype homozygosity (EHH) (65) using R package rehh (66). Ancestral and derived alleles were defined according to the Database of Single Nucleotide Polymorphisms (dbSNP) (67).
Data Availability Statement
All datasets generated for this study are included in the article/Supplementary Material.
Ethics Statement
The studies involving human participants were reviewed and approved by Brazilian National Human Research Ethics Committee (CONEP), Protocol No. CAAE 02727412.4.0000.0096, in accordance to the Brazilian Federal laws. The patients/participants provided their written informed consent to participate in this study.
Author Contributions
DA designed the study. LV, RD, VC-S, LA, and HI performed Sanger sequencing and genotyping. LV, DA, and BH performed next generation sequencing. LV, RD, and DA performed flow cytometry analysis. LV, DA, and WM analyzed the data. MP-E, JH, and DA contributed with samples and/or reagents. LV and DA drafted the manuscript. All authors contributed to the article and approved the submitted version.
Funding
This work was supported by grants and scholarships from the Coordenação de Aperfeiçoamento de Pessoal de Nível Superior—Brasil (CAPES)—Finance Code 001, Conselho Nacional de Desenvolvimento Científico e Tecnológico (CNPq), Fundação Araucária and NIH grant U19NS095774.
Conflict of Interest
The authors declare that the research was conducted in the absence of any commercial or financial relationships that could be construed as a potential conflict of interest.
Acknowledgments
We would like to thank all the volunteers who participated in this study and all the members of the Human Molecular Laboratory of the Federal University of Paraná, Brazil, for their unconditional support.
Supplementary Material
The Supplementary Material for this article can be found online at: https://www.frontiersin.org/articles/10.3389/fimmu.2020.01881/full#supplementary-material
References
1. Kiessling R, Klein E, Pross H, Wigzell H. “Natural” killer cells in the mouse. II. Cytotoxic cells with specificity for mouse moloney leukemia cells. Characteristics of the killer cell. Eur J Immunol. (1975) 5:117–21. doi: 10.1002/eji.1830050209
2. Waggoner SN, Reighard SD, Gyurova IE, Cranert SA, Mahl SE, Karmele EP, et al. Roles of natural killer cells in antiviral immunity. Curr Opin Virol. (2016) 16:15–23. doi: 10.1016/j.coviro.2015.10.008
3. Ciccone E, Pende D, Viale O, Di Donate C, Tripodi G, Orengo AM, et al. Evidence of a natural killer (NK) cell repertoire for (allo) antigen recognition: definition of five distinct NK-determined allospecificities in humans. J Exp Med. (1992) 175:709–18. doi: 10.1084/jem.175.3.709
4. van der Slik AR, Koeleman BPC, Verduijn W, Bruining GJ, Roep BO, Giphart MJ. KIR in type 1 diabetes: disparate distribution of activating and inhibitory natural killer cell receptors in patients versus HLA-matched control subjects. Diabetes. (2003) 52:2639–42. doi: 10.2337/diabetes.52.10.2639
5. Augusto DG, Lobo-Alves SC, Melo MF, Pereira NF, Petzl-Erler ML. Activating KIR and HLA Bw4 ligands are associated to decreased susceptibility to pemphigus foliaceus, an autoimmune blistering skin disease. PLoS ONE. (2012) 7:e39991. doi: 10.1371/journal.pone.0039991
6. Nelson GW, Martin MP, Gladman D, Wade J, Trowsdale J, Carrington M. Cutting edge: heterozygote advantage in autoimmune disease: hierarchy of protection/susceptibility conferred by HLA and killer Ig-like receptor combinations in psoriatic arthritis. J Immunol. (2004) 173:4273–6. doi: 10.4049/jimmunol.173.7.4273
7. Jobim MR, Jobim M, Salim PH, Portela P, Jobim LF, Leistner-Segal S, et al. Analysis of KIR gene frequencies and HLA class I genotypes in breast cancer and control group. Hum Immunol. (2013) 74:1130–3. doi: 10.1016/j.humimm.2013.06.021
8. Middleton D, Diler AS, Meenagh A, Sleator C, Gourraud PA. Killer immunoglobulin-like receptors (KIR2DL2 and/or KIR2DS2) in presence of their ligand (HLA-C1 group) protect against chronic myeloid leukaemia. Tissue Antigens. (2009) 73:553–60. doi: 10.1111/j.1399-0039.2009.01235.x
9. Martin MP, Qi Y, Gao X, Yamada E, Martin JN, Pereyra F, et al. Innate partnership of HLA-B and KIR3DL1 subtypes against HIV-1. Nat Genet. (2007) 39:733–40. doi: 10.1038/ng2035
10. Khakoo SI, Thio CL, Martin MP, Brooks CR, Gao X, Astemborski J, et al. HLA and NK cell inhibitory receptor genes in resolving hepatitis C virus infection. Science. (2004) 305:872–4. doi: 10.1126/science.1097670
11. Hiby SE, Walker JJ, O'Shaughnessy KM, Redman CWG, Carrington M, Trowsdale J, et al. Combinations of maternal KIR and fetal HLA-C genes influence the risk of preeclampsia and reproductive success. J Exp Med. (2004) 200:957–65. doi: 10.1084/jem.20041214
12. Trowsdale J, Moffett A. NK receptor interactions with MHC class I molecules in pregnancy. Semin Immunol. (2008) 20:317–20. doi: 10.1016/j.smim.2008.06.002
13. Nakimuli A, Chazara O, Hiby SE, Farrell L, Tukwasibwe S, Jayaraman J, et al. A KIR B centromeric region present in Africans but not Europeans protects pregnant women from pre-eclampsia. Proc Natl Acad Sci USA. (2015) 112:845–50. doi: 10.1073/pnas.1413453112
14. Bulmer JN, Lash GE. The role of uterine NK cells in normal reproduction and reproductive disorders. Adv Exp Med Biol. (2015) 868:95–126. doi: 10.1007/978-3-319-18881-2_5
15. Parham P. MHC class I molecules and KIRS in human history, health and survival. Nat Rev Immunol. (2005) 5:201–14. doi: 10.1038/nri1570
16. Augusto DG, Petzl-Erler ML. KIR and HLA under pressure: evidences of coevolution across worldwide populations. Hum Genet. (2015) 134:929–40. doi: 10.1007/s00439-015-1579-9
17. Older Aguilar AM, Guethlein LA, Adams EJ, Abi-Rached L, Moesta AK, Parham P. Coevolution of killer cell Ig-like receptors with HLA-C to become the major variable regulators of human NK cells. J Immunol. (2010) 185:4238–51. doi: 10.4049/jimmunol.1001494
18. Parham P, Guethlein LA. Genetics of natural killer cells in human health, disease, and survival. Annu Rev Immunol. (2018) 36:519–48. doi: 10.1146/annurev-immunol-042617-053149
19. Stewart CA, Laugier-Anfossi F, Vely F, Saulquin X, Riedmuller J, Tisserant A, et al. Recognition of peptide-MHC class I complexes by activating killer immunoglobulin-like receptors. Proc Natl Acad Sci USA. (2005) 102:13224–9. doi: 10.1073/pnas.0503594102
20. Hilton HG, Guethlein LA, Goyos A, Nemat-Gorgani N, Bushnell DA, Norman PJ, et al. Polymorphic HLA-C receptors balance the functional characteristics of KIR haplotypes. J Immunol. (2015) 195:3160–70. doi: 10.4049/jimmunol.1501358
21. Single RM, Martin MP, Gao X, Meyer D, Yeager M, Kidd JR, et al. Global diversity and evidence for coevolution of KIR and HLA. Nat Genet. (2007) 39:1114–9. doi: 10.1038/ng2077
22. Hollenbach JA, Augusto DG, Alaez C, Bubnova L, Fae I, Fischer G, et al. 16th IHIW: population global distribution of killer immunoglobulin-like receptor (KIR) and ligands. Int J Immunogenet. (2013) 40:39–45. doi: 10.1111/iji.12028
23. Augusto DG, Norman PJ, Dandekar R, Hollenbach JA. Fluctuating and geographically specific selection characterize rapid evolution of the human KIR region. Front Immunol. (2019) 10:989. doi: 10.3389/fimmu.2019.00989
24. Gendzekhadze K, Norman PJ, Abi-Rached L, Layrisse Z, Parham P. High KIR diversity in Amerindians is maintained using few gene-content haplotypes. Immunogenetics. (2006) 58(5–6):474–80. doi: 10.1007/s00251-006-0108-3
25. Nemat-Gorgani N, Edinur HA, Hollenbach JA, Traherne JA, Dunn PPJ, Chambers GK, et al. KIR diversity in Māori and polynesians: populations in which HLA-B is not a significant KIR ligand. Immunogenetics. (2014) 66:597–611. doi: 10.1007/s00251-014-0794-1
26. The 1000Genomes Project Consortium. A global reference for human genetic variation. Nature. (2015) 526:68–74. doi: 10.1038/nature15393
27. Gourraud PA, Khankhanian P, Cereb N, Yang SY, Feolo M, Maiers M, et al. HLA diversity in the 1000 genomes dataset. PLoS ONE. (2014) 9:e97282. doi: 10.1371/journal.pone.0097282
28. Apps R, Qi Y, Carlson JM, Chen H, Gao X, Thomas R, et al. Influence of HLA-C expression level on HIV control. Science. (2013) 340:87–91. doi: 10.1126/science.1232685
29. Norman PJ, Hollenbach JA, Nemat-Gorgani N, Marin WM, Norberg SJ, Ashouri E, et al. Defining KIR and HLA class I genotypes at highest resolution via high-throughput sequencing. Am J Hum Genet. (2016) 99:375–91. doi: 10.1016/j.ajhg.2016.06.023
30. Charoudeh HN, Schmied L, Gonzalez A, Terszowski G, Czaja K, Schmitter K, et al. Quantity of HLA-C surface expression and licensing of KIR2DL+ natural killer cells. Immunogenetics. (2012) 64:739–45. doi: 10.1007/s00251-012-0633-1
31. Thomas R, Apps R, Qi Y, Gao X, Male V, O'Huigin C, et al. HLA-C cell surface expression and control of HIV/AIDS correlate with a variant upstream of HLA-C. Nat Genet. (2009) 41:1290–4. doi: 10.1038/ng.486
32. Kulkarni S, Qi Y, O'hUigin C, Pereyra F, Ramsuran V, McLaren P, et al. Genetic interplay between HLA-C and MIR148A in HIV control and Crohn disease. Proc Natl Acad Sci USA. (2013) 110:20705–10. doi: 10.1073/pnas.1312237110
33. Zhang L, Leng Q, Mixson AJ. Alteration in the IL-2 signal peptide affects secretion of proteins in vitro and in vivo. J Gene Med. (2005) 7:354–65. doi: 10.1002/jgm.677
34. Wimley WC, White SH. Experimentally determined hydrophobicity scale for proteins at membrane interfaces. Nat Struct Biol. (1996) 3:842–8. doi: 10.1038/nsb1096-842
35. Dunphy SE, Guinan KJ, Chorcora CN, Jayaraman J, Traherne JA, Trowsdale J, et al. 2DL1, 2DL2 and 2DL3 all contribute to KIR phenotype variability on human NK cells. Genes Immun. (2015) 16:301–10. doi: 10.1038/gene.2015.15
36. Bari R, Bell T, Leung WH, Vong QP, Chan WK, Das Gupta N, et al. Significant functional heterogeneity among KIR2DL1 alleles and a pivotal role of arginine245. Blood. (2009) 114:5182–90. doi: 10.1182/blood-2009-07-231977
37. Béziat V, Traherne JA, Liu LL, Jayaraman J, Enqvist M, Larsson S, et al. Influence of KIR gene copy number on natural killer cell education. Blood. (2013) 121:4703–7. doi: 10.1182/blood-2012-10-461442
38. Le Luduec JB, Boudreau JE, Freiberg JC, Hsu KC. Novel approach to cell surface discrimination between KIR2DL1 subtypes and KIR2DS1 identifies hierarchies in NK repertoire, education, and tolerance. Front Immunol. (2019) 10:734. doi: 10.3389/fimmu.2019.00734
39. He Y, Tao S, Ying Y, He J, Zhu F, Lv H. Allelic polymorphism, mRNA and antigen expression of KIR2DL1 in the Chinese Han population. Hum Immunol. (2014) 75:245–9. doi: 10.1016/j.humimm.2013.12.005
40. Yawata M, Yawata N, Draghi M, Little A-M, Partheniou F, Parham P. Roles for HLA and KIR polymorphisms in natural killer cell repertoire selection and modulation of effector function. J Exp Med. (2006) 203:633–45. doi: 10.1084/jem.20051884
41. Wright PW, Li H, Huehn A, O'Connor GM, Cooley S, Miller JS, et al. Characterization of a weakly expressed KIR2DL1 variant reveals a novel upstream promoter that controls KIR expression. Genes Immun. (2014) 15:440–8. doi: 10.1038/gene.2014.34
42. Li H, Wright PW, McCullen M, Anderson SK. Characterization of KIR intermediate promoters reveals four promoter types associated with distinct expression patterns of KIR subtypes. Genes Immun. (2016) 17:66–74. doi: 10.1038/gene.2015.56
43. Robinson J, Halliwell JA, Hayhurst JD, Flicek P, Parham P, Marsh SGE. The IPD and IMGT/HLA database: Allele variant databases. Nucleic Acids Res. (2015) 43:D423–31. doi: 10.1093/nar/gku1161
44. Nemat-Gorgani N, Hilton HG, Henn BM, Lin M, Gignoux CR, Myrick JW, et al. Different selected mechanisms attenuated the inhibitory interaction of KIR2DL1 with C2 + HLA-C in two indigenous human populations in Southern Africa. J Immunol. (2018) 200:2640–55. doi: 10.4049/jimmunol.1701780
45. David G, Djaoud Z, Willem C, Legrand N, Rettman P, Gagne K, et al. Large spectrum of HLA-C recognition by killer Ig–like receptor (KIR)2DL2 and KIR2DL3 and restricted C1 specificity of KIR2DS2: dominant impact of KIR2DL2/KIR2DS2 on KIR2D NK cell repertoire formation. J Immunol. (2013) 191:4778–88. doi: 10.4049/jimmunol.1301580
46. Horowitz A, Djaoud Z, Nemat-Gorgani N, Blokhuis J, Hilton HG, Béziat V, et al. Class I HLA haplotypes form two schools that educate NK cells in different ways. Sci Immunol. (2016) 1:eaag1672. doi: 10.1126/sciimmunol.aag1672
47. Li H, Ivarsson MA, Walker-Sperling VE, Subleski J, Johnson JK, Wright PW, et al. Identification of an elaborate NK-specific system regulating HLA-C expression. PLoS Genet. (2018) 14:e1007163. doi: 10.1371/journal.pgen.1007163
48. Goodson-Gregg FJ, Krepel SA, Anderson SK. Tuning of human NK cells by endogenous HLA-C expression. Immunogenetics. (2020) 72:205–15. doi: 10.1007/s00251-020-01161-x
49. Moesta AK, Norman PJ, Yawata M, Yawata N, Gleimer M, Parham P. Synergistic polymorphism at two positions distal to the ligand-binding site makes KIR2DL2 a stronger receptor for HLA-C than KIR2DL3. J Immunol. (2008) 180:3969–79. doi: 10.4049/jimmunol.180.6.3969
51. Braun-Prado K, Vieira Mion AL, Farah Pereira N, Culpi L, Petzl-Erler ML. HLA class I polymorphism, as characterised by PCR-SSOP, in a Brazilian exogamic population. Tissue Antigens. (2000) 56:417–27. doi: 10.1034/j.1399-0039.2000.560504.x
52. Sanger F, Nicklen S, Coulson AR. DNA sequencing with chain-terminating inhibitors. Proc Natl Acad Sci USA. (1977) 74:5463–7. doi: 10.1073/pnas.74.12.5463
53. Hilton HG, Norman PJ, Nemat-Gorgani N, Goyos A, Hollenbach JA, Henn BM, et al. Loss and gain of natural killer cell receptor function in an African hunter-gatherer population. PLoS Genet. (2015) 11:e1005439. doi: 10.1371/journal.pgen.1005439
54. Augusto DG, Piovezan BZ, Tsuneto LT, Callegari-Jacques SM, Petzl-Erler ML. KIR gene content in Amerindians indicates influence of demographic factors. PLoS ONE. (2013) 8:e56755. doi: 10.1371/journal.pone.0056755
55. Kulkarni S, Martin MP, Carrington M. KIR genotyping by multiplex PCR-SSP. Methods Mol Biol. (2010) 612:365–375. doi: 10.1007/978-1-60761-362-6_25
56. Bunce M, O'Neill CM, Barnardo MCNM, Krausa P, Browning MJ, Morris PJ, et al. Phototyping: comprehensive DNA typing for HLA-A, B, C, DRB1, DRB3, DRB4, DRB5 & DQB1 by PCR with 144 primer mixes utilizing sequence-specific primers (PCR-SSP). Tissue Antigens. (1995) 46:355–67. doi: 10.1111/j.1399-0039.1995.tb03127.x
57. Vilches C, Castaño J, Gómez-Lozano N, Estefanía E. Facilitation of KIR genotyping by a PCR-SSP method that amplifies short DNA fragments. Tissue Antigens. (2007) 70:415–22. doi: 10.1111/j.1399-0039.2007.00923.x
58. Gross J, Ligges U. Nortest: Tests for Normality. (2015). Available online at: https://cran.r-project.org/package=nortest (accessed May 20, 2020).
59. R Core Team. R: A Language and Environment for Statistical Computing. Vienna: R Foundation for Statistical Computing (2019). Available online at: https://www.r-project.org/ (accessed July 06, 2020).
60. Dinno A. dunn. test: Dunn's test of multiple comparisons using rank sums. (2017). Available online at: https://cran.r-project.org/package=dunn.test (accessed July 06, 2020).
61. Jr FEH, Dupont C. Hmisc: Harrell Miscellaneous. (2019). Available online at: https://cran.r-project.org/package=Hmisc (accessed July 06, 2020).
62. Warnes G, Gorjanc G, Leisch F, Man M. Genetics: population genetics. (2019). Available online at: https://cran.r-project.org/package=genetics (accessed May 20, 2020).
63. Shin J-H, Blay S, Graham J, McNeney B. LDheatmap : an R function for graphical display of pairwise linkage disequilibria between single nucleotide polymorphisms. J Stat Softw. (2006) 16. doi: 10.18637/jss.v016.c03
64. Eklund A. Beeswarm: The Bee Swarm Plot, An Alternative To Stripchart. R package version 0.2.0. (2015). Available online at: http://cran.r-project.org/package=beeswarm (accessed July 06, 2020).
65. Sabeti PC, Reich DE, Higgins JM, Levine HZP, Richter DJ, Schaffner SF, et al. Detecting recent positive selection in the human genome from haplotype structure. Nature. (2002) 419:832–7. doi: 10.1038/nature01140
66. Gautier M, Klassmann A, Vitalis R. rehh 2.0: a reimplementation of the R package rehh to detect positive selection from haplotype structure. Mol Ecol Resour. (2017) 17:78–90. doi: 10.1111/1755-0998.12634
Keywords: NK cells, KIR, natural selection, linkage disequilibrium, coevolution, expression, population genetics
Citation: Vargas LB, Dourado RM, Amorim LM, Ho B, Calonga-Solís V, Issler HC, Marin WM, Beltrame MH, Petzl-Erler ML, Hollenbach JA and Augusto DG (2020) Single Nucleotide Polymorphism in KIR2DL1 Is Associated With HLA-C Expression in Global Populations. Front. Immunol. 11:1881. doi: 10.3389/fimmu.2020.01881
Received: 22 May 2020; Accepted: 13 July 2020;
Published: 21 August 2020.
Edited by:
Martin Maiers, National Marrow Donor Program, United StatesReviewed by:
Julian Vivian, Monash University, AustraliaStephen K. Anderson, National Cancer Institute at Frederick, United States
Copyright © 2020 Vargas, Dourado, Amorim, Ho, Calonga-Solís, Issler, Marin, Beltrame, Petzl-Erler, Hollenbach and Augusto. This is an open-access article distributed under the terms of the Creative Commons Attribution License (CC BY). The use, distribution or reproduction in other forums is permitted, provided the original author(s) and the copyright owner(s) are credited and that the original publication in this journal is cited, in accordance with accepted academic practice. No use, distribution or reproduction is permitted which does not comply with these terms.
*Correspondence: Danillo G. Augusto, ZGFuaWxsbyYjeDAwMDQwO2F1Z3VzdG8uYmlvLmJy