- 1Department of Infection, Immunity and Cardiovascular Disease, Medical School, University of Sheffield, Sheffield, United Kingdom
- 2Bateson Centre, University of Sheffield, Firth Court, Western Bank, Sheffield, United Kingdom
- 3Molecular Immunity Unit, Department of Medicine, University of Cambridge, Francis Crick Avenue, Cambridge Biomedical, Cambridge, United Kingdom
- 4Cambridge Centre for Lung Infection, Royal Papworth Hospital, Cambridge, United Kingdom
Inflammation-related progressive lung destruction is the leading causes of premature death in cystic fibrosis (CF), a genetic disorder caused by a defective cystic fibrosis transmembrane conductance regulator (CFTR). However, therapeutic targeting of inflammation has been hampered by a lack of understanding of the links between a dysfunctional CFTR and the deleterious innate immune response in CF. Herein, we used a CFTR-depleted zebrafish larva, as an innovative in vivo vertebrate model, to understand how CFTR dysfunction leads to abnormal inflammatory status in CF. We show that impaired CFTR-mediated inflammation correlates with an exuberant neutrophilic response after injury: CF zebrafish exhibit enhanced and sustained accumulation of neutrophils at wounds. Excessive epithelial oxidative responses drive enhanced neutrophil recruitment towards wounds. Persistence of neutrophils at inflamed sites is associated with impaired reverse migration of neutrophils and reduction in neutrophil apoptosis. As a consequence, the increased number of neutrophils at wound sites causes tissue damage and abnormal tissue repair. Importantly, the molecule Tanshinone IIA successfully accelerates inflammation resolution and improves tissue repair in CF animal. Our findings bring important new understanding of the mechanisms underlying the inflammatory pathology in CF, which could be addressed therapeutically to prevent inflammatory lung damage in CF patients with potential improvements in disease outcomes.
Introduction
Cystic fibrosis (CF) is a genetic disease resulting from mutations in the cystic fibrosis transmembrane conductance regulator (CFTR) and causes premature death by progressive respiratory failure, itself caused by lung destruction from a vicious circle of infection and inflammation (1, 2). It is commonly assumed that the CF-related lung pathology is primarily an infectious disorder: susceptibility to invading pathogens results from airway mucus obstruction and collapse of mucociliary clearance and that the resultant persistent infection drives chronic inflammatory lung damage (3). However, in CF airways, there is an abnormal inflammatory phenotype often present in the absence of detectable infection (4, 5), suggesting that CFTR dysfunction might also cause primary defects in lung immunity, leading to an early pro-inflammatory state and raising the question of what drives non-infectious inflammation in CF.
So far, attempts to define a role for CFTR in host innate immune potential, using patient derived cells or mammalian models (6, 7), have yielded contradictory results and have not been able to dissociate direct effects of CFTR dysfunction from the consequences of chronic inflammation on cellular function. Consequently, the mechanisms by which CFTR directly regulates innate immunity and how CF mutations contribute to inflammatory pathogenesis in CF have remained obscure. There is therefore a pressing need to develop tractable in vivo models allowing observation of CFTR-dependent effects on innate immune responses in the absence of a pre-existing inflammatory environment.
Zebrafish innate immunity is closely homologous to that of humans (8), while their optical transparency allows non-invasive, real-time monitoring of inflammatory responses in the whole organism (9). In particular, zebrafish have emerged as a powerful model to investigate inflammatory processes, with the ability to recapitulate many aspects of human inflammatory disease (10). Remarkably, zebrafish CFTR retains close sequence identity with human (11, 12). Moreover, like mammals, zebrafish CFTR is expressed in epithelial surfaces and myeloid cells and plays an important role in homeostatic balance of fluid composition (13–15). Several phenotypes that mirror many aspects of human CF were also reported in CFTR-defective zebrafish (13, 14, 16). The high level of genetic and functional conservation between the zebrafish and mammalian CFTR and innate immune systems, as well as the lack of a pre-existent CF-related inflammation, make zebrafish a clinically-relevant system to investigate immune pathophysiology in CF. Exploiting CFTR-depleted zebrafish larvae as an innovative vertebrate organism, we show here that (i) there is a direct role for CFTR in regulation of host innate immunity and tissue repair, (ii) the inflammatory pathology of CF is determined by alterations in neutrophil behavior, and finally, (iii) the pro-resolution molecule, Tanshinone IIA (TIIA), restores normal levels of inflammation and tissue repair in CF, with important implications for the treatment of CF.
Materials and Methods
Zebrafish Husbandry and Ethic Statements
Experimental procedures were performed using the following zebrafish transgenic lines: TgBAC(mpx:eGFP)i114 and Tg(Lyz:DSred)nz5 labeling neutrophils (17, 18); Tg(mpx:gal4)sh267;Tg(UAS:kaede)i222 zebrafish were used for reverse migration assays (19, 20). To study gene expression, oxidative activity and tissue repair potential, the WT pigment-less nacre (21) larvae was used.
Fish were maintained in buffered reverse osmotic water systems at 28°C and were exposed on a 14:10-h light/dark cycle to maintain proper circadian conditions in UK Home Office-approved facilities in the Bateson Centre aquaria at the University of Sheffield, under AWERB (Animal Welfare and Ethical Review Bodies). All zebrafish experiments described in the present study were performed on larvae <5 days post-fertilization (dpf), to standards set by the UK Home Office. Maintenance of adult mutant fish was approved under home office license P1A4A7A5E.
Generation of a Stable cftr Null Mutant
Purified gRNA, Cas9 protein, and tracrRNA were purchased from Sigma-Aldrich. We used the following pair of gRNA to generate the cftr mutantsh540 reported here (PAM site is indicated in bold): CCCTCCATCGCGTCTCAGTAGAT and AATCGTCAACCCTCTTGGGGTGG. 1nl of gRNA pair was co-injected with Cas9 protein and tracrRNA into the yolk of TgBAC(mpx:eGFP)i114 or nacre larvae at one-cell-stage as described earlier (22). Genomic DNA was extracted and prepared from individual larvae at 2 dpf as previously described (23). PCR using Firepol® (Solis BioDyne) was used to amplify a 320 bp region into the cftr gene (ENSDARG00000041107). Primer sequences used were as follows: cftr_fw CCTTTCCTGAGCTTCAGTCAG cftr_rev CACCAGGGAGAACTTTCTGTC. WT Mutant forms produce 193-bp band (Supplementary Figure 1A). Each founder (F0) is outcrossed with a WT fish, and then embryos from each outcrossed pair (F1 offspring) are screened for germline transmission mutations. Genomic DNA was isolated from 72 h post-fertilization (dpf) tail biopsies (23) and heterozygotes mutants were identified via gel based genotyping targeted deletion in the cftr gene. Heterozygous larvae carrying mutations were used to achieve a stable population of cftr +/– adults. cftr–/– embryos were produced by crossing these heterozygous lines and screened for impaired Kupffer's vesicle inflation, as initially reported by Navis et al. in a TALEN-generated cftr-null mutant (24).
Morpholino Injection
Morpholino were purchased from Gene Tools. The knockdown of cftr was carried on by injecting the validated cftr splice-blocking morpholino (5′-GACACATTTTGGACACTCACACCAA-3′) into one-cell-stage zebrafish as previously described (14). The morpholino for cxcl8 knockdown (5′-TATTTATGCTTACTTGACAATGATC-3′) (25) and duox2 knockdown (5′- AGTGAATTAGAGAAATGCACCTTTT-3′) (26) were prepared and injected as described earlier. Neutrophil depleted-embryos were generated using the csf3r morpholino (5′-GAAGCACAAGCGAGACGGATGCCAT-3′) (27). A standard control morpholino (5′-CCTCTTACCTCAGTTACAATTTATA-3′) was used as a negative control.
Whole Body Neutrophil Counts
To assess total neutrophil number, 3 dpf TgBAC(mpx:eGFP)i114 larvae were mounted in 0.8% agarose supplemented with tricaine (0.168 mg/mL; Sigma-Aldrich) and imaged on an Eclipse TE2000 U inverted compound fluorescence microscope (Nikon UK Ltd., Kingston upon Thames, UK) with a 4x NA objective lens. Three images were taken per larvae: one of the head region, one of the trunk region and one of the tail region. Neutrophils were counted manually, from these images and combined to give a whole body neutrophil count.
Neutrophils Recruitment Assays
The f-Met-Leu-Phe (fMLP, Sigma-Aldrich) or recombinant human IL-8 (rhIL-8, R&D Systems, Inc.) were used to induce neutrophil chemotaxis pharmacologically, as previously described (14). Injured larvae were maintained at 28°C in sterile E3 media in a 24 well-plate. Neutrophils at the injection sites were quantified and imaged on a fluorescence microscope (Nikon) using a 20x NA objective lens.
In vivo Neutrophil Reverse Migration Assay
Inflammation was elicited by tail transection on Tg(mpx:gal4)sh267;Tg(UAS:kaede)i222 larvae following established protocols (22). Briefly, injured larvae were raised to 4 h post-injury (hpi), then neutrophils at the wound site was photoconverted by 120 pulses of the 405-nm laser at 40% laser power using an UltraVIEW PhotoKinesis device on an UltraVIEW VoX spinning disk confocal microscope (PerkinElmer Life and Analytical Sciences). Larvae were transferred to an Eclipse TE2000-U inverted compound fluorescence microscope (Nikon), then were time-lapsed with 2.5 min intervals between 5 and 9 hpi using a 1394 ORCA-ERA camera (Hamamatsu Photonics Inc).
Tracking analysis of red-fluorescing neutrophils moving away from photoconverted site was assessed in Volocity 6 (Improvision; PerkinElmer Life and Analytical Sciences), using the intensity of fluorescence to identify individually labeled neutrophils over time course of inflammation resolution.
Neutrophil Apoptosis Assays
Rates of apoptotic neutrophils were assessed in 4 and 8 hpi 4% paraformaldehyde-fixed larvae after dual staining with Rhodamine-TUNEL (ApopTag Red; Millipore Corp.) to label apoptotic cells and FITC-TSA (TSAplus kit; Fluorescence Systems, PerkinElmer Life and Analytical Sciences) to label neutrophils as previously described (19).
Neutrophilic response at the wound was imaged by confocal microscopy (PerkinElmer Life), and the percentage of neutrophil apoptosis was calculated by comparing the total neutrophil number (TSA-positive) and number of apoptotic neutrophils (dual TSA/TUNEL-positive). Additionally, neutrophil death was assessed by staining with acridine orange (AO; Sigma-Aldrich) labeling death cells. Three dpf Tg(Lyz:DSred)nz5 zebrafish larvae were tail-injured and AO-stained as described previously (28).
In vivo Oxidative Activity Assay
Oxidative response at the wound sites was measured using CellROX® Deep Red (ThermoFisher) following established protocols (14). Larvae were incubated with CellROX® reagent prior tail fin amputation procedure, then immediately injured. ROS production at the wound was imaged by confocal microscopy (PerkinElmer Life) at 30 min. Oxidative response post injury at the wound was assessed in ImageJ 1.8 (National Institutes of Health) using the intensity of fluorescence and normalized to uninjured animal (WT or CF fish).
In vivo Sterile Inflammation Assays
All sterile injury-induced inflammation was performed following established methods (17).
Briefly, 3 dpf larvae were tricaine-anesthetized, then transection of the tail was performed with a microscalpel (5 mm depth; World Precision Instruments). Neutrophil chemotaxis was evaluated by assessing the number of cells at wound sites (the region posterior to the circulatory loop, Figure 1A) at various time points throughout inflammatory process on a fluorescence dissecting stereomicroscope (Leica).
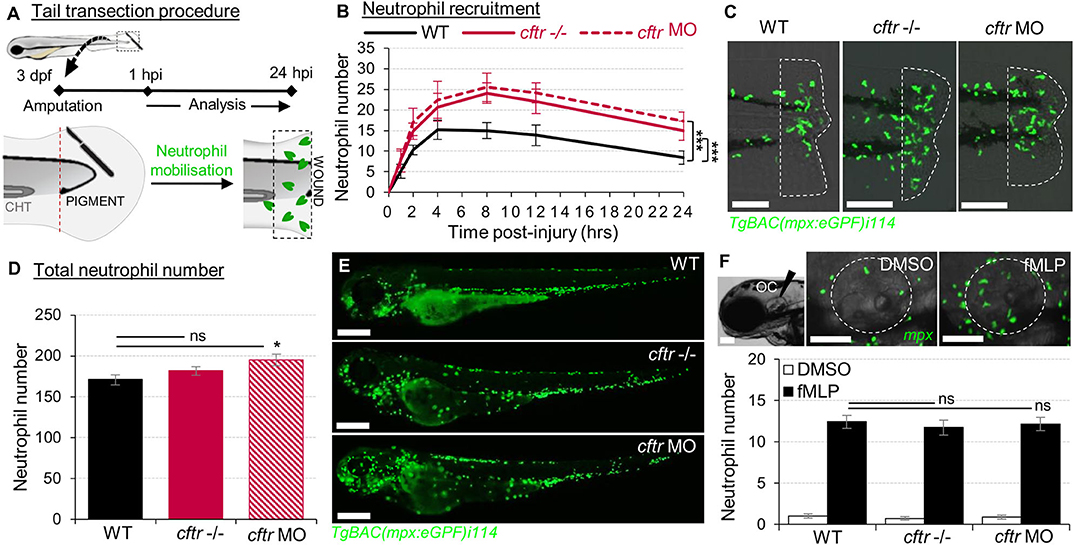
Figure 1. Sterile injury-induced neutrophilic inflammatory responses are exacerbated in the absence of CFTR. (A–C) Neutrophil chemotaxis to sterile tissue lesions in WT, cftr –/– mutant (cftr –/–) and cftr morphant (cftr MO) TgBAC(mpx:EGFP)i114 zebrafish larvae. (A) Larvae were tail amputated then the number of neutrophils mobilized to the site of injury (dotted lines) has been observed and counted by fluorescent microscopy throughout inflammation. The wound area is defined as the region between the amputation edge and caudal hematopoietic tissue end (CHT). (B) Dynamics of neutrophil recruitment towards the wound over 24 h (n = 30; two-way ANOVA with Tukey post-test). (C) Representative confocal images of injured tails at 4 hpi (scale bars, 200 μm). (D,E) Total number of neutrophils (D) in whole TgBAC(mpx:EGFP)i114 larvae (n = 18; one-way ANOVA with Dunnett's post-test). (E) Microscopy revealed a disorganized neutrophilic distribution in CFTR-depleted animal compared to control counterpart (scale bars, 200 μm). (F) Neutrophil mobilization into the otic cavity (oc, dotted lines) in response to DMSO or fMLP injection in TgBAC(mpx:EGFP)i114 larvae monitored at 2 h-post injection. Representative photomicrographs of fMLP-injected animal compared to DMSO-injected control (top panel; scale bars, 50 μm). Neutrophil counts (n = 18; one-way ANOVA with Dunnett's post-test) (bottom panel). See also Supplementary Figure 1.
In vivo Tissue Repair Assay
For regeneration assays, 2 dpf embryos were anesthetized and tail fins were transected at the region indicated in Figure 4A. Tissue repair performances were evaluated by assessing the regenerated tail fin area at 3 days post-injury (dpi). Percentage of regeneration was calculated by normalizing the regenerated tail fin area vs. fin areas of unamputated animal (WT or CF fish).
Pharmacological Treatments for Anti-inflammation and Pro-resolution Assays
Larvae were incubated in sterile E3 media supplemented with 25 μM Tanshinone IIA (TIIA, Sigma-Aldrich) (29), 100 μM Diphenyleneiodonium (DPI, Sigma-Aldrich) (26), H2O2 (ThermoFisher) (30) or 1% Dimethyl sulfoxide (DMSO, Sigma-Aldrich) as negative control.
For anti-inflammation procedures, larvae were pretreated with indicated compounds prior tail fin amputation challenge, then injured and immediately put back in treatments. Neutrophils at wounds were counted at 4 hpi at the peak of recruitment. For resolution of inflammation assays, injured larvae were raised to 4 hpi then incubated in E3 media supplemented with indicated compounds. Neutrophils at the wound sites were enumerated at 8 hpi for inflammation resolution.
qRT-PCR Analysis
Larvae were injured, then RNA from tails was extracted using TRIzol at time points indicated in Figure legends and cDNA synthesized using SuperScript II (Thermo Fisher Scientific). Real-time RT-PCRs were performed with a DyNAmo Flash SYBR green qPCR kit (Thermo Fisher Scientific) and gene expressions were detected with gene-specific primers listed as follows: ef1a_fw TCTGTTACCTGGCAAAGGG and ef1a_rev TTCAGTTTGTCCAACACCCA (31), cxcl8_fw CCTGGCATTTCTGACCATCAT and cxcl8_rev GATCTCCTGTCCAGTTGTCAT (31); duox2_fw GTTGGCTTTGGTGTAACTGTA and duox2_fw GCCCAGGCTGTGAGAG (26). Each experiment was run in triplicate. ΔCT was calculated using the housekeeping gene ef1α as a reference gene. Relative expression levels were calculated using the ΔΔCt method.
Quantification and Statistical Analysis
Statistical analysis was performed using R 3.5.0 (R core team) or Prism 7.0 (GraphPad Software, CA, USA) and detailed in each Figure legend. All data are presented as means from 3 independent experiments. All error bars indicate standard errors of means (SEM). ns, not significant (p ≥ 0.05); *p < 0.05; **p < 0.01; ***p < 0.001.
Results
Deletion of cftr Promotes Overactive Neutrophilic Inflammation to Tissue Injury
To assess the role of CFTR in regulating host inflammatory potential, expression of cftr was knocked-out using CRISPR-Cas9 technology and/or knocked-down by morpholino oligonucleotides (14) (Supplementary Figures 1A,B). In zebrafish larvae, tail amputation triggers leukocyte infiltration towards the wound, accurately mimicking the kinetics seen in mammalian inflammatory responses (17, 32, 33). At these stages, there is no infectious pathology in routine husbandry conditions. Using these “clean” CF zebrafish models, we sought to establish whether a dysfunctional CFTR could regulate non-infectious inflammation in vivo.
Neutrophil-mediated inflammation is the characteristic abnormality in the CF airways (34). Therefore, we first investigated the consequence of loss of CFTR on the neutrophilic response throughout an inflammatory process using the neutrophil-specific TgBAC(mpx:EGFP)i114 reporter line (17) (Figure 1A). Both cftr–/– mutant and cftr morphant larvae displayed overactive neutrophilic inflammation, typified by early increased, and sustained accumulation of neutrophils at wounds (Figures 1B,C). While WT fish exhibited a peak number of recruited neutrophils at 4 hpi, neutrophil influx reached a peak at 8 hpi in the absence of CFTR. Interestingly, although CF animals exhibited a slight increase in the global number of neutrophils (Figures 1D,E), fMLP-stimulated neutrophil chemotaxis revealed that CF neutrophil responses are indistinguishable from their control counterparts (14) (Figure 1F), suggesting that elevation in neutrophil numbers at wound is neither due to an overall increase in neutrophils within CF fish nor to a generalized upregulation of chemotaxis in CF neutrophils. Importantly, cftr morphants successfully phenocopy the cftr-null mutant inflammatory phenotypes, thus validating the use of both CRISPR-Cas9 and morpholino procedures to further investigate the effects of CFTR ablation on host inflammatory responses.
Collectively, these findings show that sterile injury-induced neutrophilic inflammation is exacerbated in the absence of CFTR, supporting the view that CFTR plays an essential role in control of host innate immunity and revealing a direct link between a defective CFTR and the inflammatory phenotype in CF in the absence of infection.
Enhanced ROS Production Promotes Exuberant Neutrophil Chemotaxis in the Absence of cftr
We next asked how CFTR orchestrates neutrophil trafficking during wound-induced inflammation. We first sought to identify the mechanisms driving increased neutrophil infiltration to injury. In CF lungs, high concentrations of chemotactic factors, including IL-8, are thought to be responsible for the accumulation of neutrophils (35). Despite increased cxcl8 (IL-8) expression following injury in CF fish compared with WT animals (Supplementary Figure 2A), neutrophil migration in response to exogenous IL-8 stimuli was similar in WT and CF larvae (Supplementary Figure 2B). These results suggest that early changes in neutrophil infiltration in CFTR-deficient zebrafish may be in part altered by IL-8, independently of surface receptor expression such as CXCR1 or CXCR2 in CF neutrophils. However, although cxcl8 was required for maximal neutrophil accumulation during inflammation, inhibition of cxcl8 signaling slightly affected recruitment of early neutrophils towards wounds (Supplementary Figure 2C), suggesting that CFTR likely regulates the earliest phase of neutrophil mobilization to injuries in an IL-8-independent manner.
Epithelial release of H2O2 through the NADPH oxidase DUOX2 has been identified as a potent trigger of neutrophilic inflammation (26, 36). While tail transection in WT animals consistently triggered normal oxidative response at wounds, there was higher ROS production at injured tissues in CF fish (Figures 2A,B). We next asked whether CFTR/ROS dependent innate immunity is required to orchestrate neutrophilic responses during inflammation. qRT-PCR analysis revealed similar duox2 expression in both injured-WT and CF fish (Figure 2C). Inhibition of ROS generation by genetic (Figures 2D,E) or pharmacological (Figure 2F) ablation of epithelial-specific DUOX2 oxidase, strongly reduced early neutrophil chemotaxis to tissue injury in CF animals (Figure 2G). In contrast, H2O2-treated WT larvae were characterized by increased numbers of neutrophils at the wound (Figure 2F). Interestingly, persistence of neutrophilic response did not appear to be ROS dependent, since blocking the late oxidative signaling did not reverse wound-associated neutrophil number at later time points (Figure 2H).
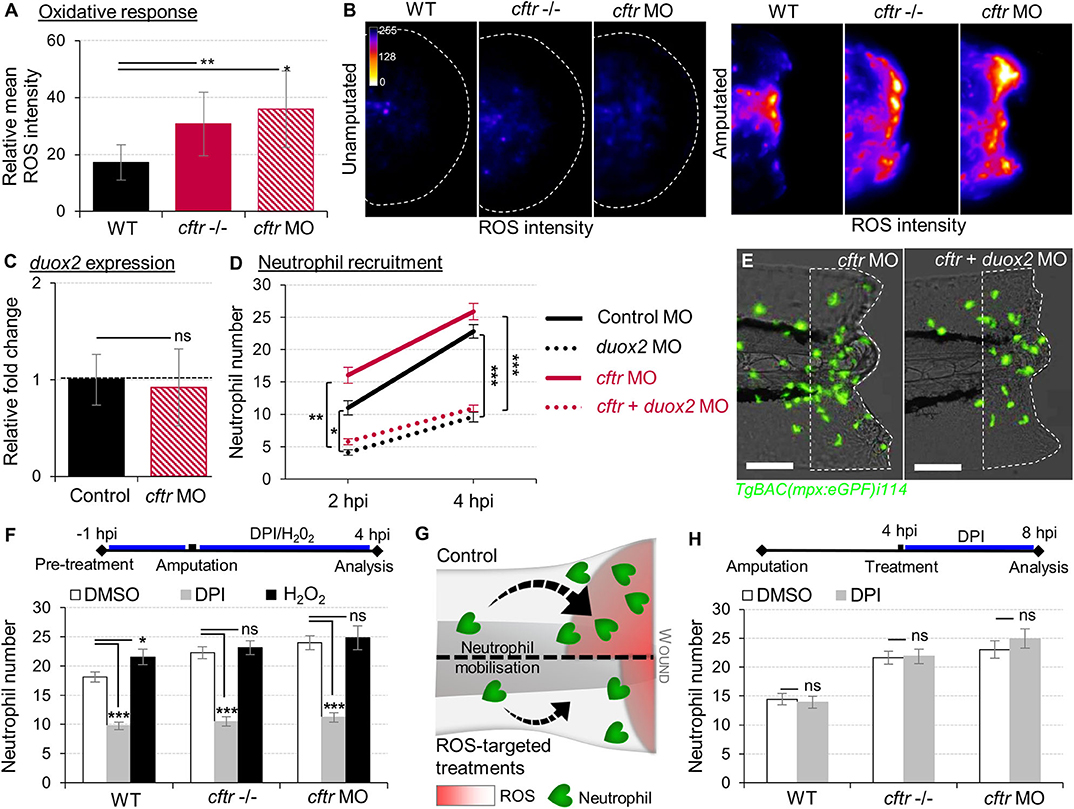
Figure 2. Exuberant wound-induced oxidative responses drive the overactive neutrophilic response in CF larvae. (A,B) WT, cftr –/– and cftr MO stained with CellROX® to label H2O2 production. Means ± SEM ROS intensity at 30 min post-injury (mpi) (A) and associated pseudocolored photomicrographs of uninjured and injured tails revealing ROS production (B) (n = 15; one-way ANOVA with Dunnett's post-test). (C) mRNA levels of duox2 gene in tail fin tissue at 30 mpi. Gene expression was expressed as fold change over tail fin tissue from uninjured larvae (30 fins per replicate; mean relative ± SEM gene expression; two-tailed Student t-test). (D) TgBAC(mpx:EGFP)i114 controls, cftr, duox2, and double cftr/duox2 morphants were tail amputated and neutrophils at wounds were enumerated at 2 and 4 hpi. (n = 21; two-way ANOVA with Tukey post-test). (E) Representative photomicrographs of injured tails at 4 hpi (scale bars, 200 μm). (F) WT, cftr –/– and cftr MO TgBAC(mpx:EGFP)i114 larvae were pretreated with DPI or H2O2 prior tail amputation procedure, then injured and immediately put back in treatments. Neutrophil counts at 4 hpi (n = 21; one-way ANOVA with Dunnett's post-test). (G) Schematic diagram showing inhibition of ROS signaling efficiently reduces neutrophil inflammation at wounds in CF animal. (H) TgBAC(mpx:EGFP)i114 larvae were treated with DPI at 4 hpi. Neutrophil counts at 8 hpi (n = 21; two-tailed Bonferroni t-test). See also Supplementary Figure 2.
Altogether, these findings indicate that functional CFTR is necessary to orchestrate the early phases of neutrophil recruitment through ROS-mediated mechanisms at sites of damage, and support that deleterious changes in epithelial oxidative responses in CF airway (37) are involved in part in the neutrophilic inflammation in CF.
Neutrophil Apoptosis and Reverse Migration Are Suppressed in CF Animals
The resolution of neutrophilic inflammation is brought about by pro-resolution events acting together to restore tissue homeostasis. These events include: (i) cessation of neutrophil infiltration and initiation of reverse migration, and (ii) promotion of neutrophil apoptosis (38). In order to elucidate the cellular processes underlying persistent neutrophilic inflammation in CF, we further investigated innate immune cell activity over the time course of inflammation resolution in CFTR-deficient animals.
Firstly, we compared WT and CF animals, to determine any difference in neutrophil apoptosis at injured sites. Confocal analysis revealed that apoptotic neutrophils are found in both the presence and absence of CFTR (Figures 3A,B, Supplementary Figure 3). However, more importantly, neutrophil apoptosis in CFTR-defective larvae was lower when compared with their control counterpart, implying that CFTR plays a crucial role in determining neutrophil lifespan during inflammation.
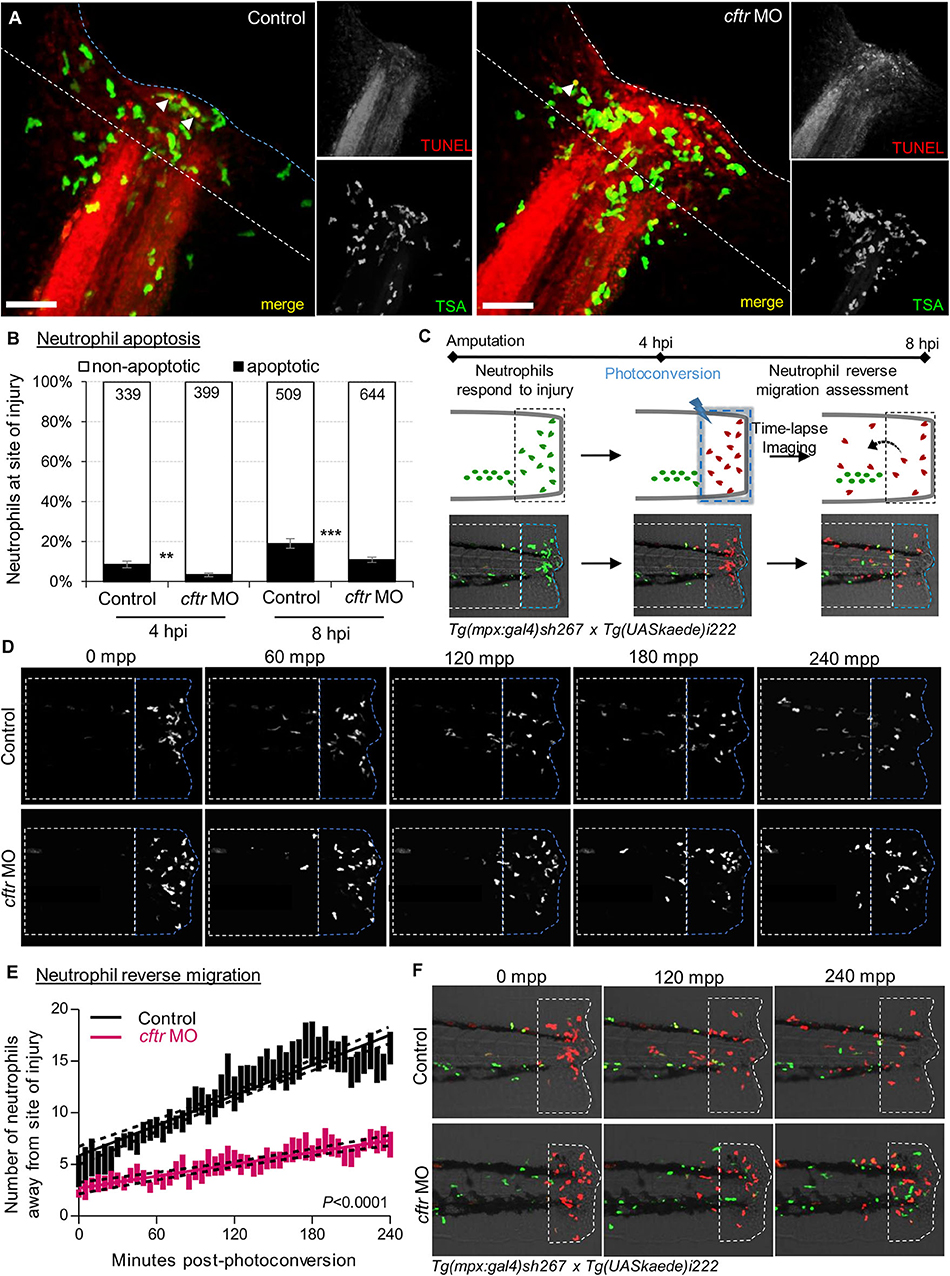
Figure 3. CFTR deficiency delays inflammation resolution in vivo both by reducing neutrophil apoptosis and reverse migration of neutrophils. (A,B) Control and cftr MO larvae were amputated and stained with TUNEL/TSA to label apoptotic cells. (A) Representative confocal pictures of injured tails at 8 hpi (scale bars, 60 μm) revealing the proportion of apoptotic neutrophils at the wound (white arrow). (B) Quantification of neutrophil apoptosis rate at site of injury at 4 and 8 hpi. (n = 18–22, Fisher t-test). (C–F) Neutrophil reverse migration in control and cftr MO larvae. (C) Tail transection was performed on 3 dpf Tg(mpx:gal4)sh267;Tg(UASkaede)i222 larvae. The site of injury was photoconverted at 4 hpi, then the number of photoconverted neutrophils (red) moving away (white dotted box) from the wound (blue dotted box) were time-lapse imaged and quantified over 4 h post-photoconversion by confocal microscopy. (D) Representative confocal imaging of amputated tails showing the kinetics of photoconverted neutrophils that migrate away from photoconverted region over inflammation resolution. (E) Plot showing the number of photoconverted neutrophils leaving the area of injury over 4 h post photoconversion in control and cftr MO. Line of best fit shown is calculated by linear regression. P-value shown is for the difference between the 2 slopes (n = 12, performed as 3 independent experiments). (F) Representative confocal imaging of amputated tails showing the kinetics of new neutrophils (green) recruited towards site of injury after photoconversion. See also Supplementary Figure 3.
We next addressed if the absence of CFTR affected reverse migration of neutrophils from lesions, as a potential contributor delaying resolution of inflammation in CF. To do that, cftr was knocked-down in Tg(mpx:Gal4)sh267;Tg(UAS:Kaede)i222 zebrafish, a neutrophil-specific transgenic line expressing a photoconvertable pigment which can be used to track specific groups of cells (Figure 3C). We observed a reduced number of neutrophils migrating away from the injury site in cftr morphants (Figures 3D,E), demonstrating the importance of CFTR for permitting reverse migration and subsequent resolution of inflammation. Additionally, microscopy analysis showed the presence of more late-migrating neutrophils (Figure 3F), suggesting that defective CFTR activity also delays cessation of neutrophil recruitment.
Collectively, these findings indicate that loss of CFTR delays resolution of inflammation in vivo by reducing neutrophil apoptosis and their reverse migration in the context of sterile inflammation, and thus linking a CFTR-related defect in both neutrophil apoptosis and reverse migration of neutrophils as pathogenic mechanisms leading to persistent neutrophilic inflammation in CF.
CF-Related Inflammation Impedes Successful Tissue Repair
Resolution of inflammation plays a pivotal role preventing chronic inflammation and excessive tissue damage, as well as initiating tissue repair (39, 40). The profound inflammatory phenotype, prompted us to analyze tissue repair in CF animals. As a developing organism, tissue repair responses in zebrafish are robust, with larval tail fins repairing completely over 72 hpi (Figure 4A) (41). We therefore studied the role of CFTR in tissue repair potential and interrogated how unresolved neutrophilic inflammation could be involved in defective tissue repair in CF. Our results showed that CF fish undergo tail fin regrowth by a reduction of 30% (Figures 4A,B). Moreover, regenerated tissues in CF animals consistently showed abnormal shape and evidence of damage (Figure 4B). We hypothesized that harmful neutrophilic activity at sites of injury might contribute to a non-healing wound. Therefore, in order to evaluate the influence of neutrophilic responses on tissue repair, we ablated neutrophils in zebrafish embryos using the csf3r morpholino (27). Removal of neutrophils at wounds during inflammation significantly improved fin repair in injured csf3r morphants in CF (Figure 4C). Interestingly, reducing early neutrophil infiltration by DPI partially restored tissue regeneration in CFTR-depleted animals (Figure 4D). Defective tissue repair was not reversed by genetic inhibition of DUOX2 (Figure 4E). In addition, our results showed that regenerated fin area in WT animals is significantly reduced in the presence of exogenous H2O2 (Figure 4D), supporting our hypothesis that deleterious neutrophilic inflammation contributes to defective tissue repair in CF.
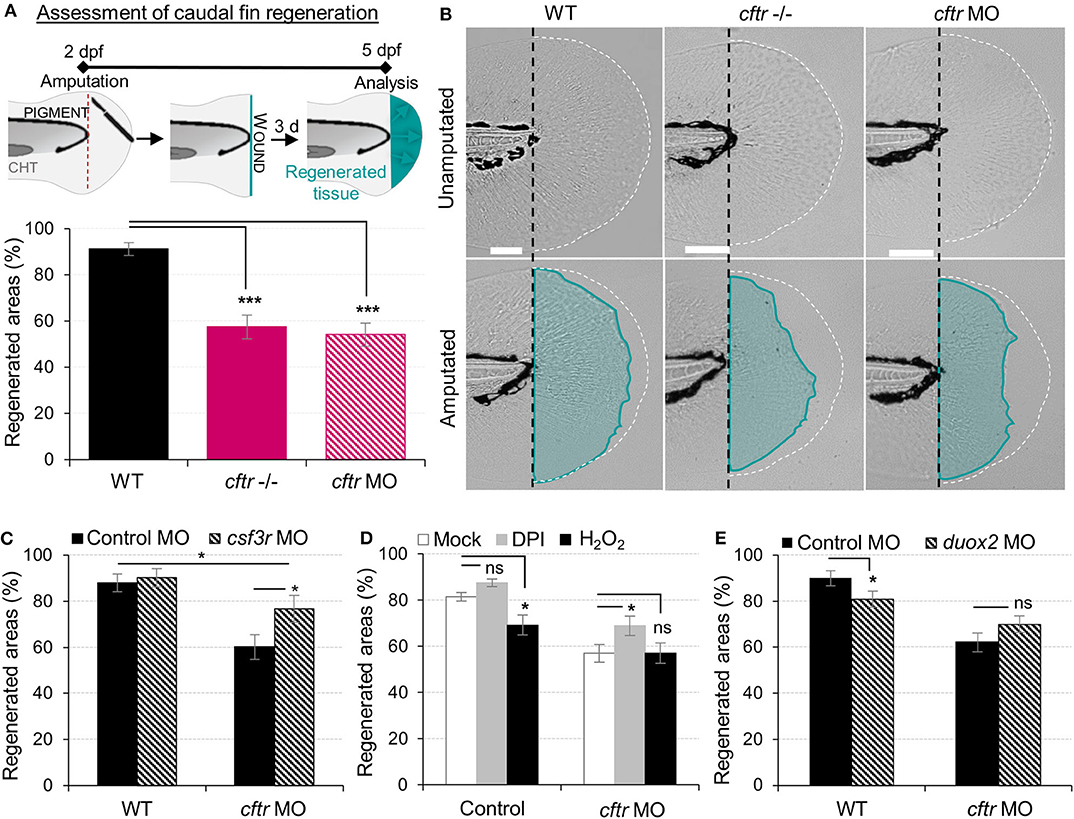
Figure 4. Neutrophilic response in CF hampers tissue repair in vivo. (A,B) Tail fin regeneration assessment in WT, cftr –/– and cftr MO zebrafish. Two dpf embryos were tail fin amputated then the potential of tail fin regeneration is evaluated by measuring regenerated fin areas at 3 dpi (blue). (A) Measurement of regenerated fin areas (n = 30, one-way ANOVA with Dunnett's post-test). (B) Representative imaging of injured tail fin at 3 dpi (scale bars, 200 μm). (C) Selective ablation of neutrophils has been carried out in WT and CF animals by injecting csf3r-MO. Measurement of regenerated fin areas in the presence or absence of neutrophils (n = 21, one-way ANOVA with Tukey post-test). (D) TgBAC(mpx:EGFP)i114 larvae were pretreated with DPI or H2O2 prior to tail fin amputation procedures, then injured and immediately put back in treatments for 4 h. Regenerated fin areas are measured at 3 dpi (n = 21, one-way ANOVA with Dunnett's post-test). (E) Inhibition of NADPH oxidase activity by injecting duox2-MO has been carried out in WT and CF animals. Embryos were tail fin injured and regenerated fin areas measured at 3 dpi (n = 21, two-tailed Bonferroni t-test).
Collectively, these results indicate that functional CFTR is required for tissue repair. Additionally, our findings demonstrate that local inflammation mediated by injury and its resolution are both crucial for successful tissue repair. These results suggest that the deleterious inflammatory processes impeding tissue performance might be a potential therapeutic target in CF.
Tanshinone IIA Promotes Resolution of Neutrophilic Inflammation and Subsequent Tissue Repair in a CF Model
Reducing the impact of inflammation-mediated tissue damage is a major concern in CF therapy. We have previously identified the potential of TIIA to accelerate resolution of inflammation by enhancing both reverse migration and apoptosis of neutrophils (29). We therefore investigated whether TIIA treatment could resolve wound-induced inflammation and initiate regenerative responses in a CF context. Interestingly, while some reports have highlighted the anti-oxidative properties of TIIA (42), our results showed that early neutrophil mobilization towards wounds was not affected by TIIA exposure in CF zebrafish (Figure 5A), and are reminiscent of our results in WT fish (29). However, when TIIA was used during the resolution phase of inflammation, TIIA treatment strongly reduced wound-associated inflammation in CF animals, as determined by neutrophil numbers (Figures 5B,C). In order to further understand the mechanism of inflammation resolution mediated by TIIA in a CFTR-deficient context, we next studied neutrophil activity during inflammation resolution. Our results showed that TIIA enhanced both neutrophil apoptosis (Figure 5D) and migration of neutrophils away from wounds (Figure 5E) in injured CF larvae. Remarkably, CFTR-deficient larvae treated with TIIA exhibited markedly enhanced tissue repair of the tail fin (Figures 5F,G).
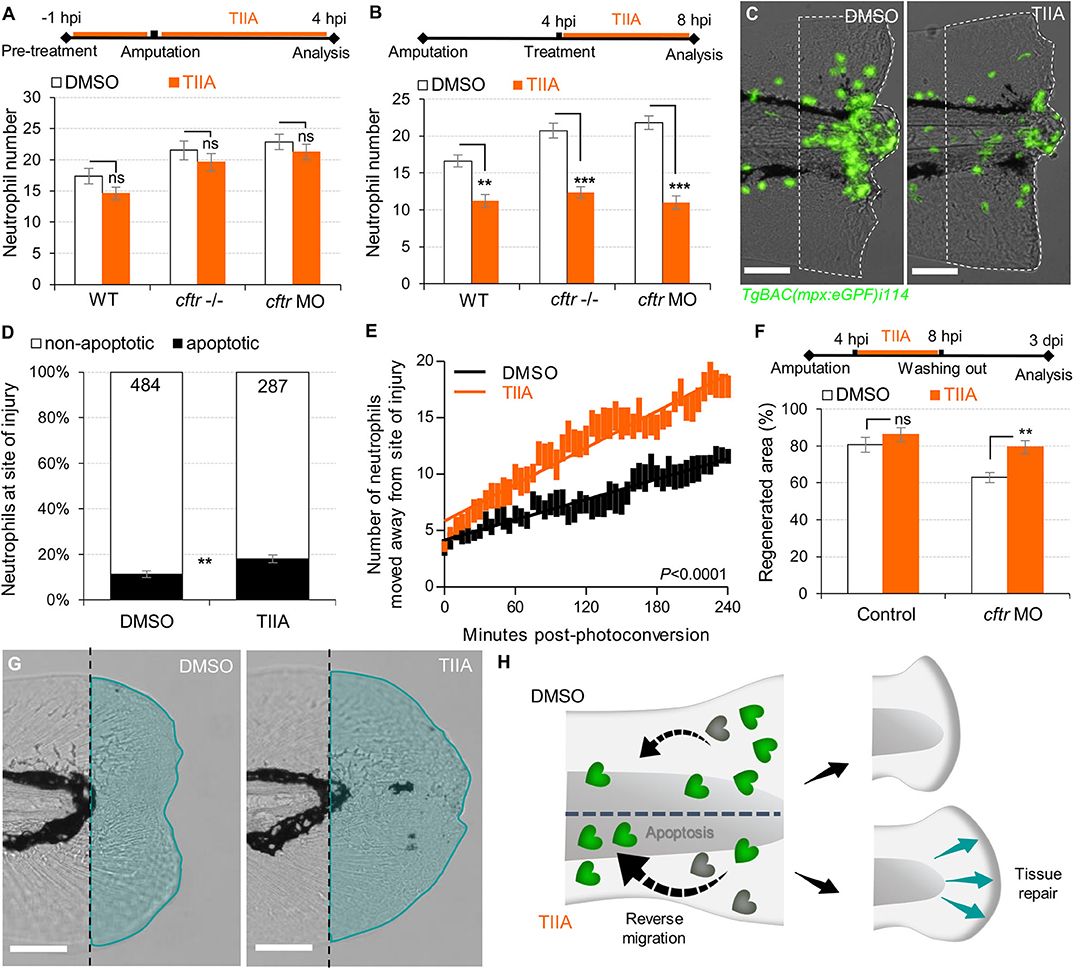
Figure 5. TIIA-driven neutrophil apoptosis and reverse migration accelerate inflammation resolution in CF. (A) TgBAC(mpx:EGFP)i114 larvae were pretreated with 25 μM of TIIA prior to tail fin amputation procedure, then injured and immediately put back in treatments for 4 h. Neutrophil number at the wound was counted at 8 hpi (n = 21, two-tailed Bonferroni t-test). (B,C) TgBAC(mpx:EGFP)i114 larvae were injured and treated from 4 hpi with 25 μM of TIIA. (B) Neutrophil number at wound was counted at 8 hpi (n = 21, two-tailed Bonferroni t-test). (C) representative number of neutrophils remaining at wounds at 8 hpi (scale bars, 200 μm). (D) Neutrophil apoptosis quantification at 8 hpi in cftr MO treated with 25 μM of TIIA from 4 hpi and stained with TUNEL/TSA. (n = 40, Fisher t-test). (E) Reverse-migration assay in cftr MO Tg(mpx:gal4)sh267;Tg(UASkaede)i222. At 4 hpi fish were treated with 25 μM of TIIA and neutrophils at site of injury were photoconverted. The numbers of photoconverted cells that moved away from the wound were time-lapse imaged and quantified over 4 h. (F,G) Regenerative performance after TIIA treatment. (F) Regenerated fin areas are measured at 3 dpi (n = 21, two-tailed Bonferroni t-test). (G) Representative imaging of injured tail fin at 3 dpi (scale bars, 200 μm). (H) Schematic diagram showing TIIA efficiently accelerates inflammation resolution by inducing neutrophil apoptosis and reverse migration at wounds and improves tissue repair in CF animal.
Altogether, these data demonstrate that redirecting neutrophils to apoptosis and reserve migration using TIIA may be a targeted therapeutic strategy to restore tissue repair (Figure 5H) and thus prevent inflammatory lung damage in CF.
Discussion
Despite improvement in the care of inflammatory pathology in CF patients, inflammation-related progressive pulmonary destruction remains the leading causes of premature death in CF. Our understanding of the mechanistic links between CFTR mutations and the pathogenesis of inflammatory pulmonary disease in CF is far from complete. The in vivo evaluation of biological functions of CFTR in currently available CF models suffers from several limitations, predominantly the evaluation of phenomena in a pre-existing inflammatory environment. To overcome this, we established and exploited a transparent zebrafish larval model, as a physiologically-relevant sterile tissue damage model in vivo. While the absence of lungs might at first sight appear to reduce the translational relevance of this model, our data suggest that changes to CFTR function in epithelial and myeloid cells are conserved across tissues and species. Using CFTR-depleted zebrafish, we have investigated the effects of CFTR dysfunction on host innate immune response to tissue injury (Figure 6).
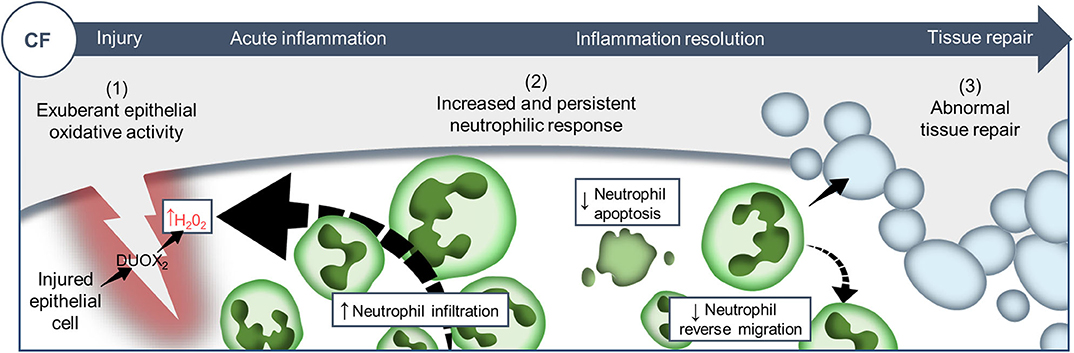
Figure 6. Proposed model showing CF-related inflammation immuno-pathogenesis. The spatiotemporal events associated with CFTR ablation reveal a mechanism whereby CFTR participates in the adjustment of innate immunity, conditioning key regulatory mediators involved in the regulation of inflammation and tissue repair. A sterile lesion is characterized by early release of epithelial H2O2 through DUOX2, leading to neutrophil recruitment towards wounds; coordination of neutrophil apoptosis and reverse migration promoting efficient inflammation resolution, and allowing to restore tissue homeostasis and initiate tissue repair. In contrast, in CF zebrafish sterile injury leads to extensive inflammation, typified by increased then sustained accumulation of neutrophils at wounds: (1) excessive epithelial ROS release drives increased neutrophil recruitment towards wounds; (2) reduction of neutrophil apoptosis and impaired retrograde migration of neutrophils resulting in delayed resolution of inflammation. (3) Therefore, the increased number of neutrophils that mobilize in an uncontrolled manner at wound sites causes persistent inflammation, severe tissue damage, and abnormal tissue repair.
This study suggests that inflammation, irrespective of the initiating stimulus is exacerbated in the absence of CFTR. This is in marked contrast to the classical explanations linking excessive inflammation to chronic infection, providing a new potential explanation for how CFTR directly regulates host inflammatory potential in vivo. This observation suggests that targeting ROS and neutrophils directly might have a much larger effect on inflammatory tissue damage than previously supposed. Without neutrophilic inflammation to generate increased extracellular DNA release from the dead pulmonary cells, this might result in major improvements in mucus viscosity and hence pathogen handling in CF.
H2O2 produced in epithelia is a potent chemoattractant source for neutrophils (26, 43) and has been proposed as an important component in the neutrophilic inflammatory pathogenesis of CF lung disease (37). Our work highlights that loss of CFTR leads to an overactive neutrophilic response to tissue damage, mediated by excessive epithelial ROS generation, and demonstrates that CFTR/DUOX2 axis-dependent ROS production is instrumental in efficiently orchestrating neutrophil chemotaxis. Surprisingly, such exuberant neutrophil mobilization is not observed when CFTR-defective zebrafish are infected with CF pathogens (13, 14), strongly implying tissue damage as the primary initiator in increasing inflammation rather than infection in CF. This observation is of particular interest in light of clinical reports of human patients showing early elevation of neutrophil infiltration in CF airway in the absence of detectable infection (4, 5). Whether CFTR/DUOX2 NADPH axis differentially regulates sterile or infection-induced neutrophilic inflammation remains to be addressed.
While progress has been made, the mechanistic link between dysfunctional CFTR and abnormal ROS generation is still elusive with evidence for both negative and positive regulation depending on the cell type. For example, our recent study shows that CF zebrafish exhibit increased susceptibility to Mycobacterium abscessus in part due to an inability to generate effective oxidative immunity in professional phagocytes (14), thus indicating opposite effects on oxidative responses in myeloid cells and in epithelial cells as shown in this present work. Further investigation is warranted to find the as yet unidentified molecular basis and to delineate the differential role of the CFTR/ROS axis in both epithelial inflammation and immunity to infection, and the link with CF phenotype in patients.
Mechanisms leading to efficient inflammation resolution, tissue healing and repair depend on suppression of inflammatory signaling pathways, orchestrated by a tightly regulated innate immune response. CF lung disease is characterized by an unresolved inflammatory response. Corroborating data from other CF ex vivo models (34, 44), we show that CF neutrophils that have migrated towards inflamed sites display molecular changes associated with reductions in both apoptosis and reverse migration, and thus have enhanced potential to drive tissue damage and impair tissue repair because of their prolonged survival and extended activity at the wound. Although it is unclear whether these are primary neutrophil defects or a response to wound-mediated inflammation in CF zebrafish, these results emphasize that CFTR plays an important role in the maintenance of tissue homeostasis by determining neutrophil behavior and lifespan during inflammation process.
Tissue repair after injury depends on both host regenerative capacity and the quality of the inflammatory response (39, 40). Reminiscent of previous studies suggesting that CFTR plays a critical role in wound repair (45, 46), we show here that CF zebrafish exhibit incomplete tissue repair after tail fin amputation, which can be improved by genetic ablation of neutrophils. These results indicate the deleterious role of neutrophil activity contributing to tissue damage and impaired tissue repair in the context of CF. repair. Importantly, this also appears to be ROS dependent, since therapeutic approaches modulating oxidative responses markedly reduce neutrophilic inflammation to tissue injury as well as greatly decrease collateral damage from inflammation and improve tissue repair. However, if oxidative stress is considered a causal mediator of damage and inflammatory disease, ROS are crucial regulators of regenerative inflammation (47, 48), as suggested by genetic inhibition of the DUOX2 NADPH oxidase pathway modulating neutrophil response at wounds but interfering with tissue repair processes. These findings emphasize that balancing the positive and negative effects of the inflammatory process should be considered for the design of clinical treatments for the management of inflammatory disease in CF. Importantly, while tissue repair in CF zebrafish can be improved by re-balancing neutrophilic activity at wounds, their regenerated tissue area remains smaller than in CF controls. This suggests that CF-mediated alterations other than unresolved inflammation are responsible for the impaired tissue repair in CF fish, potentially explaining the severe nature of lung destruction in CF compared to other forms of ciliary dysfunction, and thus indicates that additional CFTR-mediated mechanisms are likely to participate in host tissue repair capacity. The molecular basis of defective tissue repair in CF is particularly intriguing and deserves further attention, and undoubtedly will be crucial to support optimal tissue repair in CF.
Controlling injurious effects of inflammation is an essential component in the management of CF. Overall, our findings indicating that CF neutrophils have prolonged survival and activity due to dysfunctional apoptosis (44, 49) and reverse migration, providing an explanation as to how CFTR mutations may lead to unresolved neutrophilic responses, impaired tissue repair resulting in scar formation or fibrosis in CF lung, and highlighting various approaches that aim at modulating these mechanisms to limit inflammation-driven tissue damage and promote tissue repair. Assuming that this dysregulated innate immunity, prior to bacterial colonization, disrupts both inflammatory responses and tissue repair in CF, therapeutic strategies to normalize harmful inflammation might simultaneously promote resolution of inflammation and tissue repair, and thus prevent pulmonary destruction. Current strategies based on anti-inflammatory treatments in CF have not yet proven effective in the clinic and carry persistent long-term use safety concerns (50). TIIA, derived from the traditional Chinese medicinal herb Salvia miltiorrhiza widely used for the treatment of patients with cancers, inflammatory or cardiovascular diseases (51–53), was also found to be effective as a pro-resolution compound by inducing apoptosis of neutrophils and promoting their reverse migration in both zebrafish model and human neutrophils (29). Considering evidence regarding the dysfunctional responses to apoptosis as a driver of persistent neutrophilic inflammation in the CF lung, we proposed here to study efficiency of TIIA on inflammation outcomes in a context of CF, attempting to restore dysregulated inflammation and epithelial integrity in CF. We show that TIIA can effectively rebalance neutrophilic inflammation in CF animal by counteracting signaling pathways associated with neutrophil persistence and survival. Consequently, TIIA can efficiently prevent inflammatory tissue damages and improve tissue repair. These findings have significant therapeutic implications for potently targeting neutrophilic inflammation in CF, while minimizing risk of blocking host immunity, and thus may support existing therapeutic strategies or could be an alternative to existing anti-inflammatory approaches. TIIA is currently used in clinical trials in other respiratory diseases (54), and its effects are conserved in human neutrophils (29), suggesting it might be possible to directly test this hypothesis.
We report here a direct stepwise dissection of the inflammatory response in an animal lacking CFTR, providing a more comprehensive delineation of the cellular basis linking CFTR deficiency with inflammatory pathogenesis of the CF airways, and consequently insights for development of specific therapies aimed at restoring innate immune potential of CF patients and thus identify novel treatment approaches to alleviate neutrophil inflammation-driven tissue damage, with improvement in both quality of life and life expectancy.
Data Availability Statement
The raw data supporting the conclusions of this article will be made available by the authors, without undue reservation.
Ethics Statement
The animal study was reviewed and approved by UK Home Office.
Author Contributions
AB, RF, and SR designed the study and analyzed the data. AB performed all experiments with assistance from CL. AB wrote the manuscript with input from RF and SR. All authors contributed to the article and approved the submitted version.
Funding
This study was supported by the European Community's Horizon 2020-Research and Innovation Framework Program (H2020-MSCA-IF-2016) under the Marie-Curie IF CFZEBRA (751977) to AB, MRC Program Grants (MR/M004864/1) to SR, the MRC AMR Theme award (MR/N02995X/1) to AB and SR, and CF Trust workshop funding (160161) to AB, RF, and SR.
Conflict of Interest
The authors declare that the research was conducted in the absence of any commercial or financial relationships that could be construed as a potential conflict of interest.
Acknowledgments
We acknowledge the Bateson Centre aquarium and Wolfson Light Microscopy Facility [MRC grant (G0700091) and Wellcome Trust grant (GR077544AIA)] at the University of Sheffield. We would like to thank David Drew for technical assistance. This manuscript has been released as a pre-print at Bernut et al. (55).
Supplementary Material
The Supplementary Material for this article can be found online at: https://www.frontiersin.org/articles/10.3389/fimmu.2020.01733/full#supplementary-material
References
1. Rommens JM, Iannuzzi MC, Kerem BS, Drumm ML, Melmer G, Dean M, et al. Identification of the cystic fibrosis gene: chromosome walking and jumping. Science. (1989) 245:1059–65. doi: 10.1126/science.2772657
2. Gadsby DC, Vergani P, Csanády L. The ABC protein turned chloride channel whose failure causes cystic fibrosis. Nature. (2006) 440:477–83. doi: 10.1038/nature04712
3. Donaldson SH, Boucher RC. Cystic fibrosis. In: Runge MS, Patterson C, editors. Principles of Molecular Medicine. Totowa, NJ: Humana Press, Inc. (2005). p. 251–8. doi: 10.1007/978-1-59259-963-9_2
4. Verhaeghe C, Delbecque K, de Leval L, Oury C, Bours V. Early inflammation in the airways of a cystic fibrosis foetus. J Cyst Fibros. (2007) 6:304–8. doi: 10.1016/j.jcf.2006.12.001
5. Rosen BH, Evans TIA, Moll SR, Gray JS, Liang B, Sun X, et al. Infection is not required for mucoinflammatory lung disease in CFTR-Knockout ferrets. Am J Respir Crit Care Med. (2018) 197:1308–18. doi: 10.1164/rccm.201708-1616OC
6. Aldallal N, McNaughton EE, Manzel LJ, Richards AM, Zabner J, Ferkol TW, et al. Inflammatory response in airway epithelial cells isolated from patients with cystic fibrosis. Am J Respir Crit Care Med. (2002) 166:1248–56. doi: 10.1164/rccm.200206-627OC
7. Lavelle GM, White MM, Browne N, McElvaney NG, Reeves EP. Animal models of cystic fibrosis pathology: phenotypic parallels and divergences. Biomed Res Int. (2016) 2016:5258727. doi: 10.1155/2016/5258727
8. Renshaw SA, Trede NS. A model 450 million years in the making: Zebrafish and vertebrate immunity. DMM Dis Model Mech. (2012) 5:38–47. doi: 10.1242/dmm.007138
9. Renshaw SA, Loynes CA, Elworthy S, Ingham PW, Whyte MKB. Modeling inflammation in the zebrafish: how a fish can help us understand lung disease. Exp Lung Res. (2007) 33:549–54. doi: 10.1080/01902140701756778
10. Martin JS, Renshaw SA. Using in vivo zebrafish models to understand the biochemical basis of neutrophilic respiratory disease. Biochem Soc Trans. (2009) 37:830–7. doi: 10.1042/BST0370830
11. Zhang Z, Chen J. Atomic structure of the cystic fibrosis transmembrane conductance regulator. Cell. (2016) 167:1586–597.e9. doi: 10.1016/j.cell.2016.11.014
12. Liu F, Zhang Z, Csanády L, Gadsby DC, Chen J. Molecular structure of the human CFTR ion channel. Cell. (2017) 169:85–95.e8. doi: 10.1016/j.cell.2017.02.024
13. Phennicie RT, Sullivan MJ, Singer JT, Yoder JA, Kim CH. Specific resistance to pseudomonas aeruginosa infection in zebrafish is mediated by the cystic fibrosis transmembrane conductance regulator. Infect Immun. (2010) 78:4542–50. doi: 10.1128/IAI.00302-10
14. Bernut A, Dupont C, Ogryzko NV, Neyret A, Herrmann JL, Floto RA, et al. CFTR protects against mycobacterium abscessus infection by fine-tuning host oxidative defenses. Cell Rep. (2019) 26:1828–40.e4. doi: 10.1016/j.celrep.2019.01.071
15. Guh YJ, Lin CH, Hwang PP. Osmoregulation in zebrafish: Ion transport mechanisms and functional regulation. Excli J. (2015) 14:627–59. doi: 10.17179/excli2015-246
16. Navis A, Bagnat M. Loss of cftr function leads to pancreatic destruction in larval zebrafish. Dev Biol. (2015) 399:237–48. doi: 10.1016/j.ydbio.2014.12.034
17. Renshaw SA, Loynes CA, Trushell DMI, Elworthy S, Ingham PW, Whyte MKB. Atransgenic zebrafish model of neutrophilic inflammation. Blood. (2006) 108:3976–8. doi: 10.1182/blood-2006-05-024075
18. Hall C, Flores M, Storm T, Crosier K, Crosier P. The zebrafish lysozyme C promoter drives myeloid-specific expression in transgenic fish. BMC Dev Biol. (2007) 7:42. doi: 10.1186/1471-213X-7-42
19. Elks PM, Van Eeden FJ, Dixon G, Wang X, Reyes-Aldasoro CC, Ingham PW, et al. Activation of hypoxia-inducible factor-1α (hif-1α) delays inflammation resolution by reducing neutrophil apoptosis and reverse migration in a zebrafish inflammation model. Blood. (2011) 118:712–22. doi: 10.1182/blood-2010-12-324186
20. Holmes GR, Anderson SR, Dixon G, Robertson AL, Reyes-Aldasoro CC, Billings SA, et al. Repelled from the wound, or randomly dispersed? Reverse migration behaviour of neutrophils characterized by dynamic modelling. J R Soc Interface. (2012) 9:3229–39. doi: 10.1098/rsif.2012.0542
21. Lister JA, Robertson CP, Lepage T, Johnson SL, Raible DW. Nacre encodes a zebrafish microphthalmia-related protein that regulates neural-crest-derived pigment cell fate. Development. (1999) 126:3757–67.
22. Loynes CA, Lee JA, Robertson AL, Steel MJG, Ellett F, Feng Y, et al. PGE2 production at sites of tissue injury promotes an anti-inflammatory neutrophil phenotype and determines the outcome of inflammation resolution in vivo. Sci Adv. (2018) 4:eaar8320. doi: 10.1126/sciadv.aar8320
23. Isles HM, Herman KD, Robertson AL, Loynes CA, Prince LR, Elks PM, et al. The CXCL12/CXCR4 signaling axis retains neutrophils at inflammatory sites in zebrafish. Front Immunol. (2019) 10:1784. doi: 10.3389/fimmu.2019.01784
24. Bagnat M, Navis A, Marjoram L. Cftr controls lumen expansion and function of kupffer's vesicle in zebrafish. Development. (2013) 140:1703–12. doi: 10.1242/dev.091819
25. Sarris M, Masson JB, Maurin D, Van Der Aa LM, Boudinot P, Lortat-Jacob H, et al. Inflammatory chemokines direct and restrict leukocyte migration within live tissues as glycan-bound gradients. Curr Biol. (2012) 22:2375–82. doi: 10.1016/j.cub.2012.11.018
26. Niethammer P, Grabher C, Look AT, Mitchison TJ. A tissue-scale gradient of hydrogen peroxide mediates rapid wound detection in zebrafish. Nature. (2009) 459:996–9. doi: 10.1038/nature08119
27. Palha N, Guivel-Benhassine F, Briolat V, Lutfalla G, Sourisseau M, Ellett F, et al. Real-Time whole-body visualization of chikungunya virus infection and host interferon response in zebrafish. PLoS Pathog. (2013) 9:e1003619. doi: 10.1371/journal.ppat.1003619
28. Bernut A, Herrmann JL, Kissa K, Dubremetz JF, Gaillard JL, Lutfalla G, et al. Mycobacterium abscessus cording prevents phagocytosis and promotes abscess formation. Proc Natl Acad Sci USA. (2014) 111:E943–52. doi: 10.1073/pnas.1321390111
29. Robertson AL, Holmes GR, Bojarczuk AN, Burgon J, Loynes CA, Chimen M, et al. A zebrafish compound screen reveals modulation of neutrophil reverse migration as an anti-inflammatory mechanism. Sci Transl Med. (2014) 6:225ra29. doi: 10.1126/scitranslmed.3007672
30. Mugoni V, Camporeale A, Santoro MM. Analysis of oxidative stress in Zebrafish embryos. J Vis Exp. (2014) 7:51328. doi: 10.3791/51328
31. Bernut A, Nguyen-Chi M, Halloum I, Herrmann JL, Lutfalla G, Kremer L. mycobacterium abscessus-induced granuloma formation is strictly dependent on TNF signaling and neutrophil trafficking. PLoS Pathog. (2016) 12:e1005986. doi: 10.1371/journal.ppat.1005986
32. Mathias JR, Dodd ME, Walters KB, Yoo SK, Ranheim EA, Huttenlocher A. Characterization of zebrafish larval inflammatory macrophages. Dev Comp Immunol. (2009) 33:1212–7. doi: 10.1016/j.dci.2009.07.003
33. Li L, Yan B, Shi YQ, Zhang WQ, Wen ZL. Live imaging reveals differing roles of macrophages and neutrophils during zebrafish tail fin regeneration. J Biol Chem. (2012) 287:25353–60. doi: 10.1074/jbc.M112.349126
34. Downey DG, Bell SC, Elborn JS. Neutrophils in cystic fibrosis. Thorax. (2009) 64:81–8. doi: 10.1136/thx.2007.082388
35. Dean TP, Dai Y, Shute JK, Church MK, Warner JO. Interleukin-8 concentrations are elevated in bronchoalveolar lavage, sputum, and sera of children with cystic fibrosis. Pediatr Res. (1993) 34:159–61. doi: 10.1203/00006450-199308000-00010
36. Gamaley IA, Klyubin I V. Roles of reactive oxygen species: signaling and regulation of cellular functions. Int Rev Cytol. (1999) 188:203–55. doi: 10.1016/S0074-7696(08)61568-5
37. Galli F, Battistoni A, Gambari R, Pompella A, Bragonzi A, Pilolli F, et al. Oxidative stress and antioxidant therapy in cystic fibrosis. Biochim Biophys Acta. (2012) 1822:690–713. doi: 10.1016/j.bbadis.2011.12.012
38. Ortega-Gómez A, Perretti M, Soehnlein O. Resolution of inflammation: an integrated view. EMBO Mol Med. (2013) 5:661–74. doi: 10.1002/emmm.201202382
39. Karin M, Clevers H. Reparative inflammation takes charge of tissue regeneration. Nature. (2016) 529:307–15. doi: 10.1038/nature17039
40. Mescher AL, Neff AW, King MW. Inflammation and immunity in organ regeneration. Dev Comp Immunol. (2017) 66:98–110. doi: 10.1016/j.dci.2016.02.015
41. Iovine MK. Conserved mechanisms regulate outgrowth in zebrafish fins. Nat Chem Biol. (2007) 3:613–8. doi: 10.1038/nchembio.2007.36
42. Fu J, Huang H, Liu J, Pi R, Chen J, Liu P. Tanshinone IIA protects cardiac myocytes against oxidative stress-triggered damage and apoptosis. Eur J Pharmacol. (2007) 568:213–21. doi: 10.1016/j.ejphar.2007.04.031
43. Brothers KM, Gratacap RL, Barker SE, Newman ZR, Norum A, Wheeler RT. NADPH oxidase-driven phagocyte recruitment controls candida albicans filamentous growth and prevents mortality. PLoS Pathog. (2013) 9:e1003634. doi: 10.1371/journal.ppat.1003634
44. Gray RD, Hardisty G, Regan KH, Smith M, Robb CT, Duffin R, et al. Delayed neutrophil apoptosis enhances NET formation in cystic fibrosis. Thorax. (2018) 73:134–44. doi: 10.1136/thoraxjnl-2017-210134
45. Trinh NTN, Bardou O, Privé A, Maillé E, Adam D, Lingée S, et al. Improvement of defective cystic fibrosis airway epithelial wound repair after CFTR rescue. Eur Respir J. (2012) 40:1390–400. doi: 10.1183/09031936.00221711
46. Schiller KR, Maniak PJ, O'Grady SM. Cystic fibrosis transmembrane conductance regulator is involved in airway epithelial wound repair. Am J Physiol Cell Physiol. (2010) 299:C912–21. doi: 10.1152/ajpcell.00215.2010
47. Santabárbara-Ruiz P, López-Santillán M, Martínez-Rodríguez I, Binagui-Casas A, Pérez L, Milán M, et al. ROS-Induced JNK and p38 signaling is required for unpaired cytokine activation during drosophila regeneration. PLoS Genet. (2015) 11:e1005595. doi: 10.1371/journal.pgen.1005595
48. André-Lévigne D, Modarressi A, Pepper MS, Pittet-Cuénod B. Reactive oxygen species and NOX enzymes are emerging as key players in cutaneous wound repair. Int J Mol Sci. (2017) 18:2149. doi: 10.3390/ijms18102149
49. McKeon DJ, Condliffe AM, Cowburn AS, Cadwallader KC, Farahi N, Bilton D, et al. Prolonged survival of neutrophils from patients with ΔF508 CFTR mutations. Thorax. (2008) 63:660–1. doi: 10.1136/thx.2008.096834
50. Dinwiddie R. Anti-inflammatory therapy in cystic fibrosis. J Cyst Fibros. (2005) 4(2 Suppl.):45–8. doi: 10.1016/j.jcf.2005.05.010
51. Stumpf C, Fan Q, Hintermann C, Raaz D, Kurfürst I, Losert S, et al. Anti-inflammatory effects of danshen on human vascular endothelial cells in culture. Am J Chin Med. (2013) 41:1065–77. doi: 10.1142/S0192415X13500729
52. Chen X, Guo J, Bao J, Lu J, Wang Y. The anticancer properties of salvia miltiorrhiza bunge (danshen): a systematic review. Med Res Rev. (2014) 34:768–94. doi: 10.1002/med.21304
53. Gao S, Liu Z, Li H, Little PJ, Liu P, Xu S. Cardiovascular actions and therapeutic potential of tanshinone IIA. Atherosclerosis. (2012) 220:3–10. doi: 10.1016/j.atherosclerosis.2011.06.041
54. Wu CY, Cherng JY, Yang YH, Lin CL, Kuan FC, Lin YY, et al. Danshen improves survival of patients with advanced lung cancer and targeting the relationship between macrophages and lung cancer cells. Oncotarget. (2017) 8:90925–47. doi: 10.18632/oncotarget.18767
Keywords: cystic fibrosis, CFTR, neutrophilic inflammation, apoptosis, tissue repair, neutrophil reverse migration, zebrafish, Tanshione IIA
Citation: Bernut A, Loynes CA, Floto RA and Renshaw SA (2020) Deletion of cftr Leads to an Excessive Neutrophilic Response and Defective Tissue Repair in a Zebrafish Model of Sterile Inflammation. Front. Immunol. 11:1733. doi: 10.3389/fimmu.2020.01733
Received: 15 May 2020; Accepted: 29 June 2020;
Published: 31 July 2020.
Edited by:
Haichao Wang, Feinstein Institute for Medical Research, United StatesReviewed by:
Nicoletta Pedemonte, Giannina Gaslini Institute (IRCCS), ItalyCarlos Miguel Farinha, University of Lisbon, Portugal
Copyright © 2020 Bernut, Loynes, Floto and Renshaw. This is an open-access article distributed under the terms of the Creative Commons Attribution License (CC BY). The use, distribution or reproduction in other forums is permitted, provided the original author(s) and the copyright owner(s) are credited and that the original publication in this journal is cited, in accordance with accepted academic practice. No use, distribution or reproduction is permitted which does not comply with these terms.
*Correspondence: Audrey Bernut, YXVkcmV5LmJlcm51dCYjeDAwMDQwO3V2c3EuZnI=
†Present address: Audrey Bernut, Infection and Inflammation Unit, INSERM, Université Versailles Saint-Quentin, Montigny le Bretonneux, France