- 1Section of Microbiology and Applied Pathology, Department of Life Science and Biotechnology, University of Ferrara, Ferrara, Italy
- 2Department of Anesthesia, Critical Care & Pain Medicine, Beth Israel Deaconess Medical Center, Harvard Medical School, Boston, MA, United States
- 3Department of Morphology, Surgery and Experimental Medicine and LTTA Centre, University of Ferrara, Ferrara, Italy
- 4Department of Pulmonology, Medical University of Vienna, Vienna, Austria
- 5Division of Pneumology, University Hospital RWTH Aachen, Aachen, Germany
Eosinophils are major effector cells against parasites, fungi, bacteria, and viruses. However, these cells also take part in local and systemic inflammation, which are central to eczema, atopy, rhinitis, asthma, and autoimmune diseases. A role for eosinophils has been also shown in vascular thrombotic disorders and in cancer. Many, if not all, above-mentioned conditions involve the release of intracellular nucleotides (ATP, ADP, UTP, etc.) and nucleosides (adenosine) in the extracellular environment. Simultaneously, eosinophils further release ATP, which in autocrine and paracrine manners, stimulates P2 receptors. Purinergic signaling in eosinophils mediates a variety of responses including CD11b induction, ROI production, release of granule contents and enzymes, as well as cytokines. Exposure to extracellular ATP also modulates the expression of endothelial adhesion molecules, thereby favoring eosinophil extravasation and accumulation. In addition, eosinophils express the immunosuppressive adenosine P1 receptors, which regulate degranulation and migration. However, pro-inflammatory responses induced by extracellular ATP predominate. Due to their important role in innate immunity and tissue damage, pharmacological targeting of nucleotide- and nucleoside-mediated signaling in eosinophils could represent a novel approach to alleviate eosinophilic acute and chronic inflammatory diseases. These innovative approaches might also have salutary effects, particularly in host defense against parasites and in cancer.
Introduction
Nucleotides and nucleosides are present at high concentrations within the cell where they exert multiple functions. However, they are not restricted to the intracellular compartments but they serve as extracellular mediators to eukaryotic cells (1, 2). A growing body of evidence indicates that released nucleotides represent important modulators to several cell and organ pathways under both physiological and pathological conditions. Their role in the cardiocirculatory and the nervous system, in tissue metabolism, respiration and immune function, as well as in gastrointestinal and hepatic disease pathogenesis has been described recently (3–7).
Extracellular purines and pyrimidines have been implicated in the regulation of ciliary beat frequency, chloride/liquid secretion, goblet cell degranulation, epithelial mucus secretion, transmission of the respiratory nervous stimuli and modulation of the airway vascular tone (8–10). Accordingly, inhaled ATP is a powerful bronchoconstrictor in both healthy and asthmatic individuals. Furthermore, extracellular ATP can act as a damage-associated molecular pattern molecule (DAMP, also known as alarmin or danger molecule) to activate the inflammasome with subsequent upregulation of IL-1β, IL-18, and release of other pro-mobilizing mediators like high molecular group box 1 (Hmgb1) and S100 calcium-binding protein A9 (S100A9) (11).
Under homeostatic conditions extracellular ATP levels are rather low. This is due to a moderate release and rapid degradation by extracellular ATP-metabolizing enzymes (ectonucleotidases) (12, 13). However, in the course of infection, inflammation, hypoxic conditions due to ischemia as well as necrotic and apoptotic cell death ATP is released from intracellular storage pools and can reach a concentration high enough to be sensed by surrounding cells expressing P2 receptors (14–16). Besides the unregulated ATP release as a consequence of cell damage, mediated secretion of this extracellular messenger occurs through plasma membrane molecules such as connexins, pannexins, and P2X7 receptors (17–20). Apart from ATP, uridine nucleotides (UTP, UDP and UDP-glucose) can also be released in the extracellular space (21).
Eosinophils are polymorphonuclear cells mainly involved in the immune defense, tissue remodeling and inflammation. Activation and migration of these cells to inflammatory sites are crucial to tissue defense. In addition to the classical immune activators (chemokines, cytokines, microbial products, allergens, complement components) eosinophils are also capable to sense nucleotides that can amplify responses induced by other stimuli (22). Thus, extracellular nucleotides contribute to eosinophilic inflammation and tissue damage both in human and animal models (23, 24). Therefore, nucleotides and nucleosides are under intense investigation for their capacity to activate and recruit eosinophils. In this regard, high levels of ATP are present in the bronchoalveolar lavage fluid of patients suffering from eosinophilic pneumonia. This mediator also correlates with uric acid and IL-33 concentration (24, 25).
P2 Receptors
P2 receptors are plasma membrane receptors for extracellular nucleotides. On the basis of cloning, functional and pharmacological data, two P2 receptor subfamilies have been described: P2X and P2Y receptors (2, 26). Differences in nucleotide sensitivity and specificity of the P2 receptor subtypes, allow the activation of distinct P2 receptor subsets depending on the nucleotide concentration and kind.
The P2X receptor subfamily represents ligand-gated ion channels selective for monovalent and divalent cations. These ion channels are homo- or in some cases hetero-multimers with carboxyl- and amino-terminal cytoplasmatic domains (27, 28). In mammals, seven different subunits have been identified and named P2X1-P2X7. Extracellular ATP is an agonist for all P2X subtypes and regulates their permeability to Na+, K+, Ca2+, Mg2+. While the majority of P2X receptors is rapidly desensitized (e.g., P2X1 and P2X3), the non-desensitizing P2X7 represents a peculiar subtype having a long carboxyl-terminal domain allowing the receptor to undergo a permeability transition from a plasma membrane channel to a large plasma membrane pore depending on ATP concentration and the way of stimulation (26).
Stimulation of the P2X7 subtype by high ATP concentrations is associated with a permeability transition due to the opening of a membrane pore with a cut-off of 900 Da (27). Transmembrane ion fluxes, driven by pore opening, induce transcription and secretion of different inflammatory cytokines such as IL-1β, IL-18, IL-6 (23). Pharmacological blocking, genetic ablation and attenuation of P2X7 function resulted in reduced inflammatory responses (29–31).
The P2Y receptors are seven transmembrane G-protein-coupled receptors with an extracellular amino-terminus and an intracellular carboxyl-terminus. Eight human P2Y subtypes have been identified and named: P2Y1, P2Y2, P2Y4, P2Y6, P2Y11, P2Y12, P2Y13 and P2Y14 (32). They differ in agonist specificity, coupled G-protein and transduced intracellular signaling. However, according to amino acid homology and presence of conserved motifs in the transmembrane α-helix 7, two groups have been described. The first group includes the P2Y1, P2Y2, P2Y4, P2Y6, and P2Y11 subtypes, having 25–52% amino acid identity and a Y-Q/K-X-X-R motif in the transmembrane α-helix 7 (33).
To the second group belong P2Y12, P2Y13 and P2Y14, with sequence homology of 47–48% and the presence of the K-E-X-X-L motif (2). Some evidence suggests that the two P2Y subgroups differ in G-protein coupling. Hence, the receptors of the first group couple to Gq/G11proteins, contributing to calcium release via phospholipase C/inositol-1,4,5-triphosphate activation; while receptors of the second group couple to Gi/0 proteins, inhibiting adenylate cyclase (AC) (34). Different P2Y agonists have been identified, among them both adenine and uridine nucleotides (35). P2Y1, P2Y12, and P2Y13 subtypes are preferentially activated by ADP (36), whereas UDP is an agonist at P2Y6. While P2Y2 can be activated by both UTP and ATP, P2Y4 and P2Y11 are selective for UTP and ATP, respectively. Last but not least, P2Y14 is activated by UDP-glucose (35).
In the last two decades, P2 receptors gained attention for their wide tissue distribution and number of modulated pathophysiological responses. This has also prompted several P2-based therapeutic approaches (37), as in the context of kidney disease, cardiovascular and metabolic disorders as well as central nervous system (CNS) inflammation.
P1 Receptors
Similar to the P2Y receptors, the P1 receptors are seven-transmembrane G-protein-coupled receptors but their natural agonist is adenosine (ADO) (38). ADO exerts ambiguous effects in different tissues, depending on cell type and P1 receptor subtypes predominantly expressed (39–41).
Four receptors subtypes have been identified and named: A1 (ADORA1), A2A (ADORA2A), A2B (ADORA2B), and A3 (ADORA3), respectively. The main differences between the subtypes concern the affinity to ADO, the coupled G-protein families and effects on AC. While A1 and A3 inhibit AC, A2A and A2B drive its activation (38). ADO concentration of the extracellular milieu ranges from 100 to 500 nM and increases to levels in the low micromolar range as a consequence of inflammation, hypoxia and ischemia. Among the subtypes, A2B shows the lowest affinity for ADO. Accordingly, A1, A2A and A3 are activated by lower ADO concentrations (10–50 nM); whereas A2B needs a rather high agonist concentration (1 mM) for stimulation.
Primarily, adenosine receptors have been associated with dampening acute inflammation and tissue injury. On the one hand the inhibition of pro-inflammatory cytokine production and on the other hand the induction of suppressive cytokines as well as regulatory immune cell differentiation are two known effects of the anti-inflammatory responses driven by P1 receptors. Nevertheless, in the context of rheumatoid arthritis or multiple sclerosis P1 receptors have also been implicated in inflammatory cell recruitment (42–44).
Ectonucleotidases
Four main groups of plasma membrane enzymes are endowed of the ability of hydrolyzing extracellular nucleotides, transforming ATP and ADP to ADO thus shifting purinergic receptor activation from P2 to P1 subtypes. Activity of ectonucleotidases is fundamental to avoid excessive accumulation of nucleotides in the extracellular milieu and to terminate P2 signaling (45). The following families have been described: ectonucleoside triphosphate diphosphohydrolases (NTPDases), ecto-5′-nucleotidase (CD73), ectonucleotide pyrophosphatase/phosphodiesterases (NPP) and alkaline phosphatases (12). NTPDases (among which NTPDase1 or CD39) catalyze the conversion of ATP or ADP to AMP and are highly expressed by immune cells and the vasculature (46–48). Extracellular AMP is further hydrolyzed to the anti-inflammatory ADO by CD73 (49, 50). However, the CD73 driven ADO generation has been associated with the potent suppression of anti-cancer immune responses. Thus, inhibitors of CD73 for the use in clinical practice are highly desired (51).
The proposed important immunoregulatory activities of ectonucleotidases are to prevent the development of autoimmune conditions. Accordingly, we recently observed that CD39 overexpression ameliorates experimental colitis and prevents hypoxia-related damage in vivo in a dextran-sulfate-sodium-induced colitis model. In addition, exogenous administration of a recombinant form of human CD39L3 (APT102) boosted the regulatory effects of endogenous CD39 in vivo and enhanced in vitro Treg functions in Crohn's disease (48). Likewise, the administration of apyrase, which has ectoenzymatic activity comparable to CD39, attenuated peribronchial eosinophilic inflammation and reduced the levels of Th2 cytokines in the bronchoalveolar lavage fluid of mice with allergic airway inflammation (52).
Eosinophil Granulocytes
Eosinophils are granulocytes deriving from CD34+ bone marrow precursors expressing CD38 and CD125. Thereby, IL-3, IL-5, and GM-CSF exposure have been reported to induce eosinophil differentiation (53, 54). The differentiation process occurs in about 8 days and is mainly driven by the transcription factors GATA-1, GATA-2, c/EBP, and XBP1 (55–58). Cytokines (particularly IL-5) and chemokines (CCL11, CCL24, CCL26) promote the release of eosinophils from the bone marrow (59). After circulating in the peripheral blood for 8–12 h, mature eosinophils home into tissues (mammary gland, adipose tissue, uterus, gut, lung) where they contribute to maintaining organ integrity and promote B and T cell immune function (60–64).
Under pathophysiological conditions such as atopic diseases, rhinitis, eczema, asthma and parasitic infections, chemokine-mediated CCR3 receptor activation on eosinophils as well as the stimulation with cytokines such as IL-4, IL-5, IL-9, IL-13, GM-CSF, RANTES, MCP-3, and MCP-4 have been linked to the recruitment and accumulation of eosinophils in tissues including nasal mucosa, lungs, heart, skin, liver and bile ducts, gut and nerves (65–69). In addition, eotaxin, IL-4, and IL-13 have been shown to induce the up-regulation of the adhesion molecules VCAM-1 and PSGL-1 on epithelial cells and fibroblasts thus further promoting eosinophil trafficking and recruitment (70, 71). In contrast, IL-6, and IL-11 decrease tissue infiltration by eosinophils through inhibiting VCAM-1 expression and decreasing production of type 2 cytokines. Pro-inflammatory cytokines such as IL-1, IL-12, and TNF-α up-regulate endothelium adhesion molecules, including VCAM-1, thereby favoring eosinophil diapedesis (67, 72, 73).
Eosinophils themselves produce different cytokines including IL-1, IL-3, IL-4, IL-5, IL-6, IL-8, TNF-α, TGF-β, GM-CSF and pro-inflammatory mediators such as leukotriene C4 (LTC4), platelet-activation factor (PAF) (71, 74), the granular cationic proteins, major basic protein (MBP) 1, MBP 2, eosinophil cationic protein (ECP), eosinophil-derived neurotoxin (EDN) and eosinophil peroxidase (EPO). MBP which is present in the crystal core of the specific granules has cytotoxic effects due to interference with electrical properties and permeability of the cell membrane. MBP also triggers degranulation of mast cells and basophils (75). ECP favors the entry of cytotoxic molecules by forming voltage-insensitive, non-selective pores in the membrane of target cells (54, 68). ECP and EDN, that belong to the ribonuclease A superfamily, kill single-stranded RNA pneumoviruses (76). In addition, eosinophils generate reactive oxygen species, hypohalous acids and lysosomal hydrolases that are toxic for bacteria and parasites but also for surrounding tissues (77–80) (Figure 1).
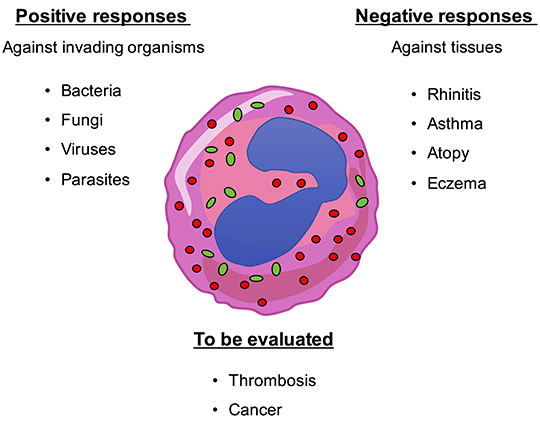
Figure 1. Eosinophils play multiple roles within the organism. Eosinophils are actively involved in the defense against multicellular parasites (e.g., worms) as well as fungi, bacteria, and viruses. However, they also show detrimental responses by damaging tissues and organs such as in rhinitis, asthma, atopy, eczema, etc. Involvement of eosinophils in lung tumor progression and in thrombosis have been also shown but their role has to be adequately evaluated.
P2 Receptors Expressed by Eosinophils
There is currently no systematic study on the expression of P2 receptor specific mRNAs and proteins in eosinophils. Another source of uncertainty is represented by the fact that the expression of individual P2 subtypes is not replicated in all studies. This bias can be due to the presence (or absence) of contaminating cells in different eosinophil preparations and/or to sensitivity of the techniques used. Different studies revealed that human, murine and rat eosinophils express mRNAs for different P2X and P2Y receptors including P2X1, P2X4, P2X5, P2X7, P2Y1, P2Y2, P2Y4, P2Y6, P2Y11 (81–85). An RNASeq study confirmed expression of P2Y6 and P2X5 mRNAs (86). Proteomic studies have shed light on expression of P2 receptor proteins, showing that human blood eosinophils express P2Y2, P2Y4, P2Y13 and P2Y14 as well as P2X1, P2X2 (87). Several pharmacologic studies performed with P2 receptor agonists and antagonists confirmed functionality of the receptors on eosinophils. Interestingly, currents evoked by the P2X agonist alpha, beta-methylene ATP were lower in eosinophils derived from asthmatic subjects compared to eosinophils derived from healthy donors, although P2X1 mRNA and protein expression was comparable in both groups. However, this effect in eosinophils isolated from asthmatics was negated by pharmacological degradation of extracellular ATP using apyrase, suggesting that P2X1 receptors were partially desensitized due to ATP release by eosinophils and raising the question why eosinophils from asthmatic subjects might release the nucleotide (88).
Nucleotide Mediated Responses in Eosinophils
Eosinophils are activated by a plethora of soluble mediators including cytokines such as IL-3, IL-5, IL-8, and GM-CSF, CC- chemokines, complement factors C3a and C5a, PAF, prostaglandin D2 (PGD2) and LPA, leukotriene B4 (LTB4) (59, 66, 89–91). Moreover, eosinophils respond to alarmins released by damaged tissue during infection or inflammation and stimulate immune responses and tissue remodeling (92). Nucleotide stimulation of human eosinophils was reported almost 30 years ago when it was shown that extracellular ATP secreted by thrombin-stimulated platelets exerted chemoattractant effects on human eosinophils (93, 94). Of note, the interaction of platelets and eosinophils contributing to tissue inflammation and remodeling was demonstrated in later studies (95–97).
In addition, eosinophils are also capable of secreting ATP which in turn autocrinally stimulates the release of different pro-inflammatory mediators by activating P2Y2 receptors (98) (Figure 2).
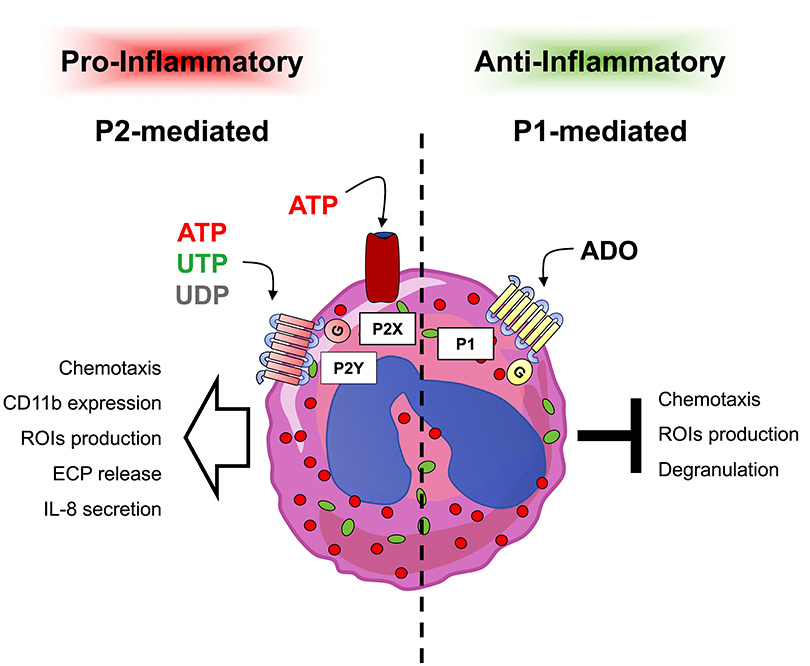
Figure 2. Responses induced by extracellular nucleotides and adenosine in eosinophils. Eosinophils express P1 and P2 receptor subtypes whose stimulation has been linked to different responses. In particular, pro-inflammatory P2-mediated responses (left part) confer to eosinophils a pro-inflammatory behavior, while on the contrary, anti-inflammatory P1-mediated responses (right part) induce anti-inflammatory effects.
Eosinophilic Tissue Infiltration
Airway infiltration by eosinophils is driven by binding of the cell surface molecule α4β1 integrin (VLA-4) on eosinophils to VCAM-1. Accordingly, mice deficient for VCAM-1 fail to develop pulmonary eosinophilia (99, 100). Extracellular nucleotides (ATP, UTP) have been implicated in modulating the expression and function of adhesion molecules including VCAM-1 (101, 102). In this context, P2Y2 receptor signaling might play an important role since it has been shown to modulate both membrane-bound and soluble VCAM-1 in a mouse model of OVA-induced lung inflammation. Furthermore, P2Y2-deficiency in the same model was associated with a reduced VCAM-1 up-regulation and lung eosinophilia compared to wild type animals (84).
In addition to VCAM-1, eosinophils express the integrin family member CD11b. CD11b interacts with CD18 to form the complement receptor 3 (CR3) heterodimer, which also contributes to eosinophil migration into inflamed tissue. Endothelial cells release ATP in response to different stimuli which might modulate the expression and function of CD11b and other adhesion molecules in circulating granulocytes (103, 104). Hence, in vitro stimulation of human eosinophils with ATP results in a fast (within seconds) and dose-dependent up-regulation of CD11b (105). In line with this, exposure of human eosinophils to pharmacological P2X and P2Y agonists induces CD11b expression (106). The fast kinetic suggests a nucleotide-mediated plasma membrane trafficking by intracellularly stored CD11b rather than an induced transcription of the cd11b gene which would be delayed. The P2X1 receptor subtype seems to be crucial in this context, since the P2X1 activation using alpha,beta-methylene ATP promotes αMβ2 integrin–dependent eosinophil adhesion. This effect was higher in eosinophils from healthy individuals compared to patients suffering from asthma (88).
Apart from modulating the expression of adhesion molecules, a direct chemotactic P2Y2-dependent effect of ATP on eosinophils has been demonstrated. Of note, eosinophils derived from asthmatic patients showed an up-regulation of P2Y2 receptor expression accompanied by an increased ATP-driven migration (22, 106) (Figure 2).
Release of Effector Molecules/Pro-Inflammatory Mediators
Activated by different stimuli such as eotaxin and complement proteins C3a and C5a, human eosinophils generate reactive oxygen intermediates (ROIs). Extracellular nucleotides (ATP, ADP, UTP, GTP, and BzATP) have also been implicated in the production of ROIs via activating both P2X and P2Y receptors (83, 106). In accordance, blood eosinophils isolated from asthmatics showed an increased expression of P2X7 receptors compared to healthy controls. Simultaneously, these eosinophils produced higher amounts of ROIs after stimulation with the P2X7 agonist BzATP (107).
Eosinophil granules contain different enzymatic and non-enzymatic proteins promoting host defense but also the pathogenesis of chronic diseases such as asthma, atopic dermatitis, prurigo nodularis and vasculitis, where they cause tissue damage associated with inappropriate release. Eosinophil cationic protein (ECP) is a known marker of eosinophil activation/participation under pathophysiological conditions (108). Stimulation of eosinophils with ATP, UTP and UDP, but not BzATP, ADP or alpha,beta-methylene ATP induces the release of ECP in a dose-dependent and pertussis toxin sensitive manner. This suggests the involvement of P2Y receptors, potentially of the P2Y2 subtype, in purine-driven ECP release. Similar observations have been made for the eosinophil derived neurotoxin, a protein closely related to ECP with cytotoxic properties, which is released following P2Y2 receptor activation (83, 109). Human and mouse eosinophils also express the P2Y12 receptor. Accordingly, ADP stimulated secretion of eosinophil peroxidase (EPO) in a P2Y12 dependent manner in human eosinophils has been shown (85).
Interleukin-8 (IL-8) or CXCL8 is a human chemokine produced by innate immune cells including eosinophils but also by endothelial or epithelial cells. Augmented IL-8 secretion has been observed in eosinophils from patients with asthma or atopic dermatitis (110, 111). Human eosinophils secrete IL-8 in response to stimulation with UDP, ATP, alpha,beta-meATP, and BzATP, while UTP or ADP show no effect. From the pharmacological profile of the response and use of P2 inhibitors, both P2Y and P2X receptor subfamilies could be involved in IL-8 secretion. A participation of P2Y6, P2X1 and P2X7 subtypes has been hypothesized (109). Moreover, a recent study demonstrated that release of pro-inflammatory cytokines by human eosinophils upon stimulation with the endogenous danger signal crystalline uric acid is dependent on autocrine secretion of ATP in the extracellular space and on the expression of purinergic receptors (Figure 2) (98).
P1 Receptors Expressed by Eosinophils and Their Responses
Adenosine P1 receptors have been shown to strongly suppress eosinophil pro-inflammatory functions. In asthma, the anti-inflammatory effects of the drug theophylline are enhanced by A3 receptors expressed on eosinophils. Accordingly, ADO administration boosts the beneficial effects of clinically relevant theophylline concentrations, while administration of the selective A3 antagonist MRS 1220 alleviates the anti-inflammatory effects of theophylline. However, A1 and A2 antagonists fail to inhibit theophylline treatment (112).
Furthermore, A3 activation in eosinophils triggers Ca2+ release from intracellular stores (113, 114). However, A3 activation does not appear to be a prime mechanism for free radical generation by human peripheral blood eosinophils and an inhibitory effect of A3 receptor subtypes on the degranulation of human eosinophils and O2− release has been suggested (115, 116). The same adenosine receptor has been found to have a regulatory function on the migration of eosinophils to the site of inflammation. In vitro experiments revealed that the A3 receptor signaling inhibits the migration of human eosinophils in response to PAF, RANTES, and LTB4 (117). This inhibitory effect has been confirmed in vivo, where A3 activation significantly reduces PAF-induced eosinophil migration to the lungs. This suggests the use of A3 receptor agonists as a therapeutic approach for asthma and rhinitis (118). Of note, an atypical form of the A3 receptor found in human eosinophils is positively coupled to AC and promotes anti-inflammatory responses by inducing cAMP (113). In some of these human studies, expression of A3 receptor in eosinophils was determined at the mRNA level (113, 118) or by immune-labeling (116); while in most of these earlier investigations presence of A3 receptor was indirectly proven by functional or inhibition studies using selective agents or antagonists (112, 115, 117), without the expression of the receptor being proven per se. In recent studies, expression of ADORA2B in human eosinophils was detected by RNA-seq analysis that, however, did not detect presence of A3 receptor in the same samples (86). Further, a subsequent proteomic study by Wilkerson and colleagues, did not detect presence of P1 receptors in human eosinophils obtained from the peripheral blood. These discrepancies might result from differences in the eosinophil purification protocols or, alternatively, in the specificity of the techniques used. Further studies are needed to combine eosinophil RNA and proteomic profiling along with functional investigations, to resolve these apparent incongruities.
Under certain circumstances, selected adenosine receptors are also responsive to inosine, a purine formed by deamination and breakdown of adenosine. It has been shown that inosine contributes to lung recruitment of eosinophils in a murine model of allergic OVA-induced respiratory inflammation in an A2A- and A3-dependent manner (119) (Figure 2).
Nucleotide Metabolizing Enzymes Expressed by Eosinophils
Information on expression and function of ectonucleotidases in human eosinophils are lacking.
Although expression of CD39 (ectonucleotidase-1) was demonstrated in human leukocytes from sputum and BALF, and its activity was shown to be modulated by smoking and increased in chronic obstructive pulmonary disease (COPD) (120), no clear attribution of CD39 protein to human eosinophil cells has been done so far.
In the context of asthma, some studies suggest a protective effect of global (or on regulatory T cells) CD39 expression in the modulation of eosinophil functions.
In the settings of ovalbumin-induced allergic airway inflammation, systemic CD39 inhibition by ARL67156 or through genetic deletion in regulatory T cells, worsens animal clinical conditions. Interestingly, control mice (i.e., mice with normal CD39 expression levels), present milder airway inflammation, associated with significantly lower eosinophil counts in BALF (121).
A correlation between CD39 levels in the thymus and eosinophil infiltration in the BALF has been recently observed also in experimental house dust mite (HDM)-induced allergic asthma. Results from this study suggest the beneficial effects of multiple doses of adipose tissue-derived mesenchymal stromal cells (MSCs). Notably, animals exposed to three doses of MSCs present significantly reduced inflammation in the lungs, this being associated to increased levels of CD39 in the thymus and lower eosinophil counts in the BALF (122).
Conclusions
While in healthy subjects the number of eosinophils in the peripheral blood is low, it can increase dramatically under pathophysiological conditions such as atopic dermatitis, bronchial asthma, eosinophilic esophagitis, gastritis, gastroenteritis, colitis or hematological malignancies (54, 62, 71, 80, 123). The large body of evidence supporting the critical role of eosinophils in parasitic and inflammatory diseases has prompted and intensified investigations on potential targets to modulate cellular responses of eosinophils.
Inflammation is associated with the release of nucleotides in the extracellular space, where they serve as ligands for purinergic receptors. Purinergic signaling represents an ubiquitous signal transduction and regulatory system (7). Eosinophils express a wide range of purinergic receptors and purinergic receptor activation is associated with the recruitment of eosinophils into inflamed tissue, ROIs production, the release of effector molecules and the secretion of pro-inflammatory cytokines. Thus, the inhibition of purinergic receptors on eosinophils would be highly desirable for reducing detrimental immune responses and tissue damage related to various disorders. In accordance, blocking P2 receptors signaling using specific inhibitors or P2 receptor deficiency could be associated with decreased eosinophilic inflammation in diverse animal models. Given the wide range of specific P2 receptor inhibitors available and the successful application in clinical trials, further research in P2 inhibition as therapeutic strategy for treating eosinophilic diseases in humans is warranted.
Besides targeting eosinophil migration and activation, in inflammation another potential approach is the modulation of eosinophil-platelets interactions. In asthma patients it has been demonstrated that platelets bind to eosinophils in the blood. This event directly correlates with the occurrence of spontaneous or clinically induced (e.g., allergen challenge) asthmatic attacks (124). One randomized, placebo-controlled clinical study on the use of the anti-P2Y12 platelet inhibitor “Prasugrel” in asthmatic patients, has shown a slight, although not significant reduction, in the bronchial inflammatory burden (125).
However, other P2Y12 inhibitors (used for the treatment of thrombosis) have failed to control eosinophil recruitment in animal models of allergic inflammation (126), suggesting different levels in platelet activity in response to vascular injury, when compared to allergic responses (127). Furthermore, different studies confirmed the involvement of P2Y1 and P2Y14 receptors in platelet-dependent eosinophil recruitment in the lungs (126, 128, 129).
New developments of effective treatments for eosinophilic diseases, like asthma or allergy, are also important because eosinophils are a major source of intravascular tissue factor, a key initiator of blood coagulation (130). Disorders characterized by eosinophil accumulation have been associated with an increased risk of thrombosis. A study conducted on a cohort of patients affected by hypereosinophilia confirmed the presence of increased tissue factor expression in eosinophils from these patients compared to healthy controls (131). However, further investigation is needed to confirm whether this finding is truly associated with an increased risk of thrombosis. A comprehensive profiling of eosinophil P1 and P2 receptor expression pattern at both mRNA and protein levels would shed light on the function of these receptors in eosinophils, as well as on their biology and contribution to the regulation of pathologically relevant eosinophil responses.
Moreover, differences in the purinergic signaling of different eosinophil subpopulations could exist and be important for diseases where eosinophil participation is predominant (132, 133). Therefore, isolation of eosinophils subpopulations and analysis of their purinergic network would be requested. Another prerequisite is the characterization of the complete panel of cytokine/chemokines released by eosinophils in response to nucleotide stimulation. In future studies, it would be relevant to check the effect of ATP and other nucleotides on production of eosinophil preeminent cytokines and chemokines such as IL-5, eotaxin and RANTES. Further efforts should be done to elucidate expression and function of ectonucleotidases CD39 and CD73 in human eosinophils; this would give a more complete picture of the purinergic signaling of these cells and would help to interpret relationships between purinergic signaling in eosinophils and other cell types involved in the immune response and tissue remodeling. Eosinophils are thought to play either positive or negative roles in cancer, depending on type of tumor (59). Since nucleosides and nucleotides are present in the tumor microenvironment and heavily affect immune response against cancer, it would be worthy to check whether stimulation of the purinergic network of eosinophils modulate their responses against tumors.
Author Contributions
DF, MV, ML, SR, AZ, TM, and MI conceived the review and wrote the manuscript. FC prepared the figures, contribute and revise the manuscript. EM and PS checked and revised the manuscript. All authors contributed to the article and approved the submitted version.
Funding
This manuscript was supported by local founds of the University of Ferrara.
Conflict of Interest
The authors declare that the research was conducted in the absence of any commercial or financial relationships that could be construed as a potential conflict of interest.
Abbreviations
AC, adenylate cyclase; ADO, adenosine; ADP, adenosine diphosphate; ATP, adenosine triphosphate; BALF, bronchoalveolar lavage fluid; BzATP, 2',3'-O-(4-benzoyl-benzoyl)ATP; C3a, complement factor 3a; C5a, complement factor 5a; CCL11, (eotaxin-1); CCL24, (eotaxin-2); CCL26, (eotaxin-3); CNS, central nervous system; COPD, chronic obstructive pulmonary disease; CR, complement receptor; DAMP, damage-associated molecular pattern; ECP, eosinophil cationic protein; EDN, eosinophil-derived neurotoxin; EPO, eosinophil peroxidase; EPO, eosinophil peroxidase; GM-CSF, granulocyte macrophage-colony stimulating factor; GTP, guanosine triphosphate; HDM, house dust mite; ICAM-1, intercellular adhesion molecule-1; IL, interleukin; LPS, lipopolysaccharides; LT, leukotriene; MBP, major basic protein; MCP, monocyte chemoattractant protein; OVA, ovalbumin; PAF, platelet-activating factor; PGD2, prostaglandin D2; MSCs, mesenchymal stromal cells; PSGL-1, P-selectin Glycoprotein Ligand-1; ROIs, reactive oxygen intermediates; TGF-β, transforming growth factor β; TNF-α, tumor necrosis factor α; UDP, uridine diphosphate; UTP, uridine triphosphate; VCAM-1, vascular cell adhesion molecule 1.
References
1. Ferrari D, Mcnamee EN, Idzko M, Gambari R, Eltzschig HK. Purinergic signaling during immune cell trafficking. Trends Immunol. (2016) 37:399–411. doi: 10.1016/j.it.2016.04.004
2. Abbracchio MP, Burnstock G, Boeynaems JM, Barnard EA, Boyer JL, Kennedy C, et al. International Union of Pharmacology LVIII: update on the P2Y G protein-coupled nucleotide receptors: from molecular mechanisms and pathophysiology to therapy. Pharmacol Rev. (2006) 58:281–341. doi: 10.1124/pr.58.3.3
3. Burnstock G. Purinergic signaling in the cardiovascular system. Circ Res. (2017) 120:207–28. doi: 10.1161/CIRCRESAHA.116.309726
4. Burnstock G, Gentile D. The involvement of purinergic signalling in obesity. Purinergic Signal. (2018) 14:97–108. doi: 10.1007/s11302-018-9605-8
5. Ferrari D, Vitiello L, Idzko M, la Sala A. Purinergic signaling in atherosclerosis. Trends Mol Med. (2015) 21:184–92. doi: 10.1016/j.molmed.2014.12.008
6. Ferrari D, Gambari R, Idzko M, Muller T, Albanesi C, Pastore S, et al. Purinergic signaling in scarring. FASEB J. (2016) 30:3–12. doi: 10.1096/fj.15-274563
7. Idzko M, Ferrari D, Riegel AK, Eltzschig HK. Extracellular nucleotide and nucleoside signaling in vascular and blood disease. Blood. (2014) 124:1029–37. doi: 10.1182/blood-2013-09-402560
8. Davis CW, Lazarowski E. Coupling of airway ciliary activity and mucin secretion to mechanical stresses by purinergic signaling. Respir Physiol Neurobiol. (2008) 163:208–13. doi: 10.1016/j.resp.2008.05.015
9. Funk GD, Huxtable AG, Lorier AR. ATP in central respiratory control: a three-part signaling system. Respir Physiol Neurobiol. (2008) 164:131–42. doi: 10.1016/j.resp.2008.06.004
10. Burnstock G, Brouns I, Adriaensen D, Timmermans JP. Purinergic signaling in the airways. Pharmacol Rev. (2012) 64:834–68. doi: 10.1124/pr.111.005389
11. Ratajczak MZ, Adamiak M, Thapa A, Bujko K, Brzezniakiewicz-Janus K, Lenkiewicz AM. NLRP3 inflammasome couples purinergic signaling with activation of the complement cascade for the optimal release of cells from bone marrow. Leukemia. (2019) 33:815–25. doi: 10.1038/s41375-019-0436-6
12. Zimmermann H, Zebisch M, Strater N. Cellular function and molecular structure of ecto-nucleotidases. Purinergic Signal. (2012) 8:437–502. doi: 10.1007/s11302-012-9309-4
13. Yegutkin GG. Nucleotide-and nucleoside-converting ectoenzymes: important modulators of purinergic signalling cascade. Biochim Biophys Acta. (2008) 1783: 673–94. doi: 10.1016/j.bbamcr.2008.01.024
14. Chekeni FB, Elliott MR, Sandilos JK, Walk SF, Kinchen JM, Lazarowski ER, et al. Pannexin 1 channels mediate 'find-me' signal release and membrane permeability during apoptosis. Nature. (2010) 467:863–7. doi: 10.1038/nature09413
15. Ferrari D, Idzko M, Müller T, Manservigi R, Marconi P. Purinergic Signaling: a new pharmacological target against viruses? Trends Pharmacol Sci. (2018) 39:926–36. doi: 10.1016/j.tips.2018.09.004
16. Savio LEB, Coutinho-Silva R. Immunomodulatory effects of P2X7 receptor in intracellular parasite infections. Curr Opin Pharmacol. (2019) 47:53–8. doi: 10.1016/j.coph.2019.02.005
17. Eltzschig HK, Eckle T, Mager A, Kuper N, Karcher C, Weissmuller T, et al. ATP release from activated neutrophils occurs via connexin 43 and modulates adenosine-dependent endothelial cell function. Circ Res. (2006) 99:1100–8. doi: 10.1161/01.RES.0000250174.31269.70
18. Junger WG. Immune cell regulation by autocrine purinergic signalling. Nat Rev Immunol. (2011) 11:201–12. doi: 10.1038/nri2938
19. Lohman AW, Billaud M, Isakson BE. Mechanisms of ATP release and signalling in the blood vessel wall. Cardiovasc Res. (2012) 95:269–80. doi: 10.1093/cvr/cvs187
20. Lazarowski ER. Vesicular and conductive mechanisms of nucleotide release. Purinergic Signal. (2012) 8:359–73. doi: 10.1007/s11302-012-9304-9
21. Lazarowski ER, Sesma JI, Seminario-Vidal L, Kreda SM. Molecular mechanisms of purine and pyrimidine nucleotide release. Adv Pharmacol. (2011) 61:221–61. doi: 10.1016/B978-0-12-385526-8.00008-4
22. Müller T, Robaye B, Vieira RP, Ferrari D, Grimm M, Jakob T, et al. The purinergic receptor P2Y2 receptor mediates chemotaxis of dendritic cells and eosinophils in allergic lung inflammation. Allergy. (2010) 65:1545–53. doi: 10.1111/j.1398-9995.2010.02426.x
23. Idzko M, Ferrari D, Eltzschig HK. Nucleotide signalling during inflammation. Nature. (2014) 509:310–7. doi: 10.1038/nature13085
24. Uratsuji H, Tada Y, Kawashima T, Kamata M, Hau CS, Asano Y, et al. P2Y6 receptor signaling pathway mediates inflammatory responses induced by monosodium urate crystals. J Immunol. (2012) 188:436–44. doi: 10.4049/jimmunol.1003746
25. Kouzaki H, Iijima K, Kobayashi T, O'grady SM, Kita H. The danger signal, extracellular ATP, is a sensor for an airborne allergen and triggers IL-33 release and innate Th2-type responses. J Immunol. (2011) 186:4375–87. doi: 10.4049/jimmunol.1003020
26. North RA, Surprenant A. Pharmacology of cloned P2X receptors. Annu Rev Pharmacol Toxicol. (2000) 40:563–80. doi: 10.1146/annurev.pharmtox.40.1.563
27. North RA. Molecular physiology of P2X receptors. Physiol Rev. (2002) 82:1013–67. doi: 10.1152/physrev.00015.2002
28. Nicke A, Kerschensteiner D, Soto F. Biochemical and functional evidence for heteromeric assembly of P2X1 and P2X4 subunits. J Neurochem. (2005) 92:925–33. doi: 10.1111/j.1471-4159.2004.02939.x
29. Manthei DM, Jackson DJ, Evans MD, Gangnon RE, Tisler CJ, Gern JE, et al. Protection from asthma in a high-risk birth cohort by attenuated P2X(7) function. J Allergy Clin Immunol. (2012) 130:496–502. doi: 10.1016/j.jaci.2012.05.040
30. Weber FC, Esser PR, Muller T, Ganesan J, Pellegatti P, Simon MM, et al. Lack of the purinergic receptor P2X(7) results in resistance to contact hypersensitivity. J Exp Med. (2010) 207:2609–19. doi: 10.1084/jem.20092489
31. Labasi JM, Petrushova N, Donovan C, Mccurdy S, Lira P, Payette MM, et al. Absence of the P2X7 receptor alters leukocyte function and attenuates an inflammatory response. J Immunol. (2002) 168:6436–45. doi: 10.4049/jimmunol.168.12.6436
32. Von Kugelgen I, Wetter A. Molecular pharmacology of P2Y-receptors. Naunyn Schmiedebergs Arch Pharmacol. (2000) 362:310–23. doi: 10.1007/s002100000310
33. D'ambrosi N, Iafrate M, Vacca F, Amadio S, Tozzi A, Mercuri NB, et al. The P2Y(4) receptor forms homo-oligomeric complexes in several CNS and PNS neuronal cells. Purinergic Signal. (2006) 2:575–82. doi: 10.1007/s11302-006-9014-2
34. Jacobson KA, Balasubramanian R, Deflorian F, Gao ZG. G protein-coupled adenosine (P1) and P2Y receptors: ligand design and receptor interactions. Purinergic Signal. (2012) 8:419–36. doi: 10.1007/s11302-012-9294-7
35. Lazarowski ER, Boucher RC. UTP as an extracellular signaling molecule. News Physiol Sci. (2001) 16:1–5. doi: 10.1152/physiologyonline.2001.16.1.1
36. Chhatriwala M, Ravi RG, Patel RI, Boyer JL, Jacobson KA, Harden TK. Induction of novel agonist selectivity for the ADP-activated P2Y1 receptor versus the ADP-activated P2Y12 and P2Y13 receptors by conformational constraint of an ADP analog. J Pharmacol Exp Ther. (2004) 311:1038–43. doi: 10.1124/jpet.104.068650
37. Burnstock G. Pathophysiology and therapeutic potential of purinergic signaling. Pharmacol Rev. (2006) 58:58–86. doi: 10.1124/pr.58.1.5
38. Fredholm BB, Irenius E, Kull B, Schulte G. Comparison of the potency of adenosine as an agonist at human adenosine receptors expressed in Chinese hamster ovary cells. Biochem Pharmacol. (2001) 61:443–8. doi: 10.1016/s0006-2952(00)00570-0
39. Antonioli L, Blandizzi C, Pacher P, Hasko G. Immunity, inflammation and cancer: a leading role for adenosine. Nat Rev Cancer. (2013) 13:842–57. doi: 10.1038/nrc3613
40. Lasley RD. Adenosine receptor-mediated cardioprotection-current limitations and future directions. Front Pharmacol. (2018) 9:310. doi: 10.3389/fphar.2018.00310
41. Alencar AKN, Montes GC, Barreiro EJ, Sudo RT, Zapata-Sudo G. Adenosine receptors as drug targets for treatment of pulmonary arterial hypertension. Front Pharmacol. (2017) 8:858. doi: 10.3389/fphar.2017.00858
42. Salmon JE, Cronstein BN. Fc gamma receptor-mediated functions in neutrophils are modulated by adenosine receptor occupancy. A1 receptors are stimulatory and A2 receptors are inhibitory. J Immunol. (1990) 145:2235–40.
43. Merrill JE, Murphy SP. Inflammatory events at the blood brain barrier: regulation of adhesion molecules, cytokines, and chemokines by reactive nitrogen and oxygen species. Brain Behav Immun. (1997) 11:245–63. doi: 10.1006/brbi.1997.0496
44. Salmon JE, Brogle N, Brownlie C, Edberg JC, Kimberly RP, Chen BX, et al. Human mononuclear phagocytes express adenosine A1 receptors. A novel mechanism for differential regulation of Fc gamma receptor function. J Immunol. (1993) 151:2775–85.
45. Antonioli L, Pacher P, Vizi ES, Hasko CD39 G. and CD73 in immunity and inflammation. Trends Mol Med. (2013) 19:355–67. doi: 10.1016/j.molmed.2013.03.005
46. Pinsky DJ, Broekman MJ, Peschon JJ, Stocking KL, Fujita T, Ramasamy R, et al. Elucidation of the thromboregulatory role of CD39/ectoapyrase in the ischemic brain. J Clin Invest. (2002) 109:1031–40. doi: 10.1172/JCI10649
47. Kohler D, Eckle T, Faigle M, Grenz A, Mittelbronn M, Laucher S, et al. CD39/ectonucleoside triphosphate diphosphohydrolase 1 provides myocardial protection during cardiac ischemia/reperfusion injury. Circulation. (2007) 116:1784–94. doi: 10.1161/CIRCULATIONAHA.107.690180
48. Robles RJ, Mukherjee S, Vuerich M, Xie A, Harshe R, Cowan PJ, et al. Modulation of CD39 and exogenous APT102 correct immune dysfunction in experimental colitis and Crohn's disease. J Crohns Colitis. (2019) jjz182. doi: 10.1093/ecco-jcc/jjz182
49. Ohta A, Sitkovsky M. Role of G-protein-coupled adenosine receptors in downregulation of inflammation and protection from tissue damage. Nature. (2001) 414:916–20. doi: 10.1038/414916a
50. Flogel U, Burghoff S, Van Lent PL, Temme S, Galbarz L, Ding Z, et al. Selective activation of adenosine A2A receptors on immune cells by a CD73-dependent prodrug suppresses joint inflammation in experimental rheumatoid arthritis. Sci Transl Med. (2012) 4:146ra108. doi: 10.1126/scitranslmed.3003717
51. Beavis PA, Stagg J, Darcy PK, Smyth MJ. CD73: a potent suppressor of antitumor immune responses. Trends Immunol. (2012) 33:231–7. doi: 10.1016/j.it.2012.02.009
52. Li P, Cao J, Chen Y, Wang W, Yang J. Apyrase protects against allergic airway inflammation by decreasing the chemotactic migration of dendritic cells in mice. Int J Mol Med. (2014) 34:269–75. doi: 10.3892/ijmm.2014.1771
53. Mori Y, Iwasaki H, Kohno K, Yoshimoto G, Kikushige Y, Okeda A, et al. Identification of the human eosinophil lineage-committed progenitor: revision of phenotypic definition of the human common myeloid progenitor. J Exp Med. (2009) 206:183–93. doi: 10.1084/jem.20081756
54. Ramirez GA, Yacoub MR, Ripa M, Mannina D, Cariddi A, Saporiti N, et al. Eosinophils from physiology to disease: a comprehensive review. Biomed Res Int. (2018) 2018:9095275. doi: 10.1155/2018/9095275
55. Bettigole SE, Lis R, Adoro S, Lee AH, Spencer LA, Weller PF, et al. The transcription factor XBP1 is selectively required for eosinophil differentiation. Nat Immunol. (2015) 16:829–37. doi: 10.1038/ni.3225
56. Du J, Stankiewicz MJ, Liu Y, Xi Q, Schmitz JE, Lekstrom-Himes JA, et al. Novel combinatorial interactions of GATA-1, PU.1, and C/EBPepsilon isoforms regulate transcription of the gene encoding eosinophil granule major basic protein. J Biol Chem. (2002) 277:43481–94. doi: 10.1074/jbc.M204777200
57. Katsumura KR, Bresnick EH, GATA Factor Mechanisms Group. The GATA factor revolution in hematology. Blood. (2017) 129:2092–102. doi: 10.1182/blood-2016-09-687871
58. Fulkerson PC. Transcription factors in eosinophil development and as therapeutic targets. Front Med. (2017) 4:115. doi: 10.3389/fmed.2017.00115
59. Klion AD, Ackerman SJ, Bochner BS. Contributions of eosinophils to human health and disease. Annu Rev Pathol. (2020) 15:179–209. doi: 10.1146/annurev-pathmechdis-012419-032756
60. Shi HZ, Humbles A, Gerard C, Jin Z, Weller PF. Lymph node trafficking and antigen presentation by endobronchial eosinophils. J Clin Invest. (2000) 105:945–53. doi: 10.1172/JCI8945
61. Sugawara R, Lee EJ, Jang MS, Jeun EJ, Hong CP, Kim JH, et al. Small intestinal eosinophils regulate Th17 cells by producing IL-1 receptor antagonist. J Exp Med. (2016) 213:555–67. doi: 10.1084/jem.20141388
62. Lee JJ, Jacobsen EA, Mcgarry MP, Schleimer RP, Lee NA. Eosinophils in health and disease: the LIAR hypothesis. Clin Exp Allergy. (2010) 40:563–75. doi: 10.1111/j.1365-2222.2010.03484.x
63. Mesnil C, Raulier S, Paulissen G, Xiao X, Birrell MA, Pirottin D, et al. Lung-resident eosinophils represent a distinct regulatory eosinophil subset. J Clin Invest. (2016) 126:3279–95. doi: 10.1172/JCI85664
64. Marichal T, Mesnil C, Bureau F. Homeostatic eosinophils: characteristics and functions. Front Med. (2017) 4:101. doi: 10.3389/fmed.2017.00101
65. Denburg JA, Sehmi R, Saito H, Pil-Seob J, Inman MD, O'byrne PM. Systemic aspects of allergic disease: bone marrow responses. J Allergy Clin Immunol. (2000) 106(Suppl. 5):S242–6. doi: 10.1067/mai.2000.110156
66. Foster PS, Mould AW, Yang M, Mackenzie J, Mattes J, Hogan SP, et al. Elemental signals regulating eosinophil accumulation in the lung. Immunol Rev. (2001) 179:173–81. doi: 10.1034/j.1600-065x.2001.790117.x
67. Wang S, Fan Y, Han X, Yang J, Bilenki L, Yang X. IL-12-dependent vascular cell adhesion molecule-1 expression contributes to airway eosinophilic inflammation in a mouse model of asthma-like reaction. J Immunol. (2001) 166:2741–9. doi: 10.4049/jimmunol.166.4.2741
68. Hogan SP, Rosenberg HF, Moqbel R, Phipps S, Foster PS, Lacy P, et al. Eosinophils: biological properties and role in health and disease. Clin Exp Allergy. (2008) 38:709–50. doi: 10.1111/j.1365-2222.2008.02958.x
69. Chen R, Smith SG, Salter B, El-Gammal A, Oliveria JP, Obminski C, et al. Allergen-induced increases in sputum levels of group 2 innate lymphoid cells in subjects with asthma. Am J Respir Crit Care Med. (2017) 196:700–12. doi: 10.1164/rccm.201612-2427OC
70. Bochner BS, Schleimer RP. Mast cells, basophils, and eosinophils: distinct but overlapping pathways for recruitment. Immunol Rev. (2001) 179:5–15. doi: 10.1034/j.1600-065x.2001.790101.x
71. Nagata M, Nakagome K, Soma T. Mechanisms of eosinophilic inflammation. Asia Pac Allergy. (2020) 10:e14. doi: 10.5415/apallergy.2020.10.e14
72. Wang J, Homer RJ, Hong L, Cohn L, Lee CG, Jung S, et al. IL-11 selectively inhibits aeroallergen-induced pulmonary eosinophilia and Th2 cytokine production. J Immunol. (2000) 165:2222–31. doi: 10.4049/jimmunol.165.4.2222
73. Wang J, Homer RJ, Chen Q, Elias Endogenous JA. and exogenous IL-6 inhibit aeroallergen-induced Th2 inflammation. J Immunol. (2000) 165:4051–61. doi: 10.4049/jimmunol.165.7.4051
74. Tavares LP, Peh HY, Tan WSD, Pahima H, Maffia P, Tiligada E, et al. Granulocyte-targeted therapies for airway diseases. Pharmacol Res. (2020) 157:104881. doi: 10.1016/j.phrs.2020.104881
75. Klion AD, Nutman TB. The role of eosinophils in host defense against helminth parasites. J Allergy Clin Immunol. (2004) 113:30–7. doi: 10.1016/j.jaci.2003.10.050
76. Jonsson UB, Blom K, Stalenheim G, Hakansson LD, Venge P. The production of the eosinophil proteins ECP and EPX/EDN are regulated in a reciprocal manner. APMIS. (2014) 122:283–91. doi: 10.1111/apm.12142
77. Mcbrien CN, Menzies-Gow A. The biology of eosinophils and their role in asthma. Front Med. (2017) 4:93. doi: 10.3389/fmed.2017.00093
78. Manohar M, Verma AK, Venkateshaiah SU, Mishra A. Role of eosinophils in the initiation and progression of pancreatitis pathogenesis. Am J Physiol Gastrointest Liver Physiol. (2018) 314:G211–22. doi: 10.1152/ajpgi.00210.2017
79. Gudbjartsson DF, Bjornsdottir US, Halapi E, Helgadottir A, Sulem P, Jonsdottir GM, et al. Sequence variants affecting eosinophil numbers associate with asthma and myocardial infarction. Nat Genet. (2009) 41:342–7. doi: 10.1038/ng.323
80. Diny NL, Rose NR, Cihakova D. Eosinophils in autoimmune diseases. Front Immunol. (2017) 8:484. doi: 10.3389/fimmu.2017.00484
81. Ferrari D, Idzko M, Dichmann S, Purlis D, Virchow C, Norgauer J, et al. P2 purinergic receptors of human eosinophils: characterization and coupling to oxygen radical production. FEBS Lett. (2000) 486:217–24. doi: 10.1016/s0014-5793(00)02306-1
82. Alberto AV, Faria RX, De Menezes JR, Surrage A, Da Rocha NC, Ferreira LG, et al. Role of P2 receptors as modulators of rat eosinophil recruitment in allergic inflammation. PLoS ONE. (2016) 11:e0145392. doi: 10.1371/journal.pone.0145392
83. Kobayashi T, Soma T, Noguchi T, Nakagome K, Nakamoto H, Kita H, et al. ATP drives eosinophil effector responses through P2 purinergic receptors. Allergol Int. (2015) 64:S30–6. doi: 10.1016/j.alit.2015.04.009
84. Vanderstocken G, Bondue B, Horckmans M, Di Pietrantonio L, Robaye B, Boeynaems JM, et al. P2Y2 receptor regulates VCAM-1 membrane and soluble forms and eosinophil accumulation during lung inflammation. J Immunol. (2010) 185:3702–7. doi: 10.4049/jimmunol.0903908
85. Muniz VS, Baptista-Dos-Reis R, Benjamim CF, Mata-Santos HA, Pyrrho AS, Strauch MA, et al. Purinergic P2Y12 receptor activation in eosinophils and the schistosomal host response. PLoS ONE. (2015) 10:e0139805. doi: 10.1371/journal.pone.0139805
86. Shen ZJ, Hu J, Esnault S, Dozmorov I, Malter JS. RNA Seq profiling reveals a novel expression pattern of TGF- β target genes in human blood eosinophils. Immunol Lett. (2015) 167:1–10. doi: 10.1016/j.imlet.2015.06.012
87. Wilkerson EM, Johansson MW, Hebert AS, Westphall MS, Mathur SK, Jarjour NN, et al. The peripheral blood eosinophil proteome. J Proteome Res. (2016) 15:1524–33. doi: 10.1021/acs.jproteome.6b00006
88. Wright A, Mahaut-Smith M, Symon F, Sylvius N, Ran S, Bafadhel M, et al. Impaired P2X1 receptor-mediated adhesion in eosinophils from asthmatic patients. J Immunol. (2016) 196:4877–84. doi: 10.4049/jimmunol.1501585
89. Hirai H, Tanaka K, Yoshie O, Ogawa K, Kenmotsu K, Takamori Y, et al. Prostaglandin D2 selectively induces chemotaxis in T helper type 2 cells, eosinophils, and basophils via seven-transmembrane receptor CRTH2. J Exp Med. (2001) 193:255–61. doi: 10.1084/jem.193.2.255
90. Lukacs NW. Role of chemokines in the pathogenesis of asthma. Nat Rev Immunol. (2001) 1:108–16. doi: 10.1038/35100503
91. Lynch OT, Giembycz MA, Daniels I, Barnes PJ, Lindsay MA. Pleiotropic role of lyn kinase in leukotriene B(4)-induced eosinophil activation. Blood. (2000) 95:3541–7. doi: 10.1182/blood.V95.11.3541
92. Yang D, Chen Q, Su SB, Zhang P, Kurosaka K, Caspi RR, et al. Eosinophil-derived neurotoxin acts as an alarmin to activate the TLR2-MyD88 signal pathway in dendritic cells and enhances Th2 immune responses. J Exp Med. (2008) 205:79–90. doi: 10.1084/jem.20062027
93. Saito H, Ebisawa M, Reason DC, Ohno K, Kurihara K, Sakaguchi N, et al. Extracellular ATP stimulates interleukin-dependent cultured mast cells and eosinophils through calcium mobilization. Int Arch Allergy Appl Immunol. (1991) 94:68–70. doi: 10.1159/000235327
94. Burgers JA, Schweizer RC, Koenderman L, Bruijnzeel PL, Akkerman JW. Human platelets secrete chemotactic activity for eosinophils. Blood. (1993) 81:49–55. doi: 10.1182/blood.V81.1.49.49
95. Johansson MW, Mckernan EM, Fichtinger PS, Angulo EL, Bagley JL, Lee KE, et al. αIIb-Integrin (CD41) associated with blood eosinophils is a potential biomarker for disease activity in eosinophilic esophagitis. J Allergy Clin Immunol. (2020) 145:1699–701. doi: 10.1016/j.jaci.2020.01.022
96. McCarty OJ, Tien N, Bochner BS, Konstantopoulos K. Exogenous eosinophil activation converts PSGL-1-dependent binding to CD18-dependent stable adhesion to platelets in shear flow. Am J Physiol Cell Physiol. (2003) 284:C1223–34. doi: 10.1152/ajpcell.00403.2002
97. Shah SA, Page CP, Pitchford SC. Platelet-eosinophil interactions as a potential therapeutic target in allergic inflammation and asthma. Front Med. (2017) 4:129. doi: 10.3389/fmed.2017.00129
98. Kobayashi T, Kouzaki H, Kita H. Human eosinophils recognize endogenous danger signal crystalline uric acid and produce proinflammatory cytokines mediated by autocrine ATP. J Immunol. (2010) 184:6350–8. doi: 10.4049/jimmunol.0902673
99. Gonzalo JA, Lloyd CM, Kremer L, Finger E, Martinez AC, Siegelman MH, et al. Eosinophil recruitment to the lung in a murine model of allergic inflammation. The role of T cells, chemokines, adhesion receptors. J Clin Invest. (1996) 98:2332–45. doi: 10.1172/JCI119045
100. Chin JE, Hatfield CA, Winterrowd GE, Brashler JR, Vonderfecht SL, Fidler SF, et al. Airway recruitment of leukocytes in mice is dependent on alpha4-integrins and vascular cell adhesion molecule-1. Am J Physiol. (1997) 272(2 Pt 1):L219–29. doi: 10.1152/ajplung.1997.272.2.L219
101. Stachon P, Geis S, Peikert A, Heidenreich A, Michel NA, Unal F, et al. Extracellular ATP induces vascular inflammation and atherosclerosis via purinergic receptor Y2 in mice. Arterioscler Thromb Vasc Biol. (2016) 36:1577–86. doi: 10.1161/ATVBAHA.115.307397
102. Seye CI, Yu N, Gonzalez FA, Erb L, Weisman GA. The P2Y2 nucleotide receptor mediates vascular cell adhesion molecule-1 expression through interaction with VEGF receptor-2 (KDR/Flk-1). J Biol Chem. (2004) 279:35679–86. doi: 10.1074/jbc.M401799200
103. Faigle M, Seessle J, Zug S, El Kasmi KC, Eltzschig HK. ATP release from vascular endothelia occurs across Cx43 hemichannels and is attenuated during hypoxia. PLoS ONE. (2008) 3:e2801. doi: 10.1371/journal.pone.0002801
104. Yamamoto K, Shimizu N, Obi S, Kumagaya S, Taketani Y, Kamiya A, et al. Involvement of cell surface ATP synthase in flow-induced ATP release by vascular endothelial cells. Am J Physiol Heart Circ Physiol. (2007) 293:H1646–53. doi: 10.1152/ajpheart.01385.2006
105. Dichmann S, Idzko M, Zimpfer U, Hofmann C, Ferrari D, Luttmann W, et al. Adenosine triphosphate-induced oxygen radical production and CD11b up-regulation: Ca(++) mobilization and actin reorganization in human eosinophils. Blood. (2000) 95:973–8. doi: 10.1182/blood.V95.3.973.003k47_973_978
106. Idzko M, Dichmann S, Panther E, Ferrari D, Herouy Y, Virchow C, et al. Functional characterization of P2Y and P2X receptors in human eosinophils. J Cell Physiol. (2001) 188:329–36. doi: 10.1002/jcp.1129
107. Muller T, Vieira RP, Grimm M, Durk T, Cicko S, Zeiser R, et al. A potential role for P2X7R in allergic airway inflammation in mice and humans. Am J Respir Cell Mol Biol. (2011) 44:456–64. doi: 10.1165/rcmb.2010-0129OC
108. Pronk-Admiraal CJ, Bartels PC. Total amount of ECP per eosinophil as indicator for the activity state of eosinophils. Scand J Clin Lab Invest. (2001) 61:453–7. doi: 10.1080/00365510152567095
109. Idzko M, Panther E, Bremer HC, Sorichter S, Luttmann W, Virchow CJ, et al. Stimulation of P2 purinergic receptors induces the release of eosinophil cationic protein and interleukin-8 from human eosinophils. Br J Pharmacol. (2003) 138:1244–50. doi: 10.1038/sj.bjp.0705145
110. Yousefi S, Hemmann S, Weber M, Holzer C, Hartung K, Blaser K, et al. IL-8 is expressed by human peripheral blood eosinophils. Evidence for increased secretion in asthma. J Immunol. (1995) 154:5481–90.
111. Sugihara R, Kumamoto T, Ito T, Ueyama H, Toyoshima I, Tsuda T. Human muscle protein degradation in vitro by eosinophil cationic protein (ECP). Muscle Nerve. (2001) 24:1627–34. doi: 10.1002/mus.1198
112. Ezeamuzie CI. Involvement of A(3) receptors in the potentiation by adenosine of the inhibitory effect of theophylline on human eosinophil degranulation: possible novel mechanism of the anti-inflammatory action of theophylline. Biochem Pharmacol. (2001) 61:1551–9. doi: 10.1016/s0006-2952(01)00613-x
113. Kohno Y, Ji X, Mawhorter SD, Koshiba M, Jacobson KA. Activation of A3 adenosine receptors on human eosinophils elevates intracellular calcium. Blood. (1996) 88:3569–74. doi: 10.1182/blood.V88.9.3569.bloodjournal8893569
114. Fukuda T, Ackerman SJ, Reed CE, Peters MS, Dunnette SL, Gleich GJ. Calcium ionophore A23187 calcium-dependent cytolytic degranulation in human eosinophils. J Immunol. (1985) 135:1349–56.
115. Ezeamuzie CI, Philips E. Adenosine A3 receptors on human eosinophils mediate inhibition of degranulation and superoxide anion release. Br J Pharmacol. (1999) 127:188–94. doi: 10.1038/sj.bjp.0702476
116. Reeves JJ, Harris CA, Hayes BP, Butchers PR, Sheehan MJ. Studies on the effects of adenosine A3 receptor stimulation on human eosinophils isolated from non-asthmatic or asthmatic donors. Inflamm Res. (2000) 49:666–72. doi: 10.1007/s000110050644
117. Knight D, Zheng X, Rocchini C, Jacobson M, Bai T, Walker B. Adenosine A3 receptor stimulation inhibits migration of human eosinophils. J Leukoc Biol. (1997) 62:465–8. doi: 10.1002/jlb.62.4.465
118. Walker BA, Jacobson MA, Knight DA, Salvatore CA, Weir T, Zhou D, et al. Adenosine A3 receptor expression and function in eosinophils. Am J Respir Cell Mol Biol. (1997) 16:531–7. doi: 10.1165/ajrcmb.16.5.9160835
119. Da Rocha Lapa F, De Oliveira AP, Accetturi BG, De Oliveira Martins I, Domingos HV, De Almeida Cabrini D, et al. Anti-inflammatory effects of inosine in allergic lung inflammation in mice: evidence for the participation of adenosine A2A and A3 receptors. Purinergic Signal. (2013) 9:325–36. doi: 10.1007/s11302-013-9351-x
120. Lazar Z, Mullner N, Lucattelli M, Ayata CK, Cicko S, Yegutkin GG, et al. NTPDase1/CD39 and aberrant purinergic signalling in the pathogenesis of COPD. Eur Respir J. (2016) 47:254–63. doi: 10.1183/13993003.02144-2014
121. Li P, Gao Y, Cao J, Wang W, Chen Y, Zhang G, et al. CD39+ regulatory T cells attenuate allergic airway inflammation. Clin Exp Allergy. (2015) 45:1126–37. doi: 10.1111/cea.12521
122. Castro LL, Kitoko JZ, Xisto DG, Olsen PC, Guedes HLM, Morales MM, et al. Multiple doses of adipose tissue-derived mesenchymal stromal cells induce immunosuppression in experimental asthma. Stem Cells Transl Med. (2020) 9:250–60. doi: 10.1002/sctm.19-0120
123. Butterfield JH, Leiferman KM, Gleich Nodules GJ. Nodules, eosinophilia, rheumatism, dermatitis and swelling (NERDS): a novel eosinophilic disorder. Clin Exp Allergy. (1993) 23:571–80. doi: 10.1111/j.1365-2222.1993.tb00896.x
124. Pitchford SC, Yano H, Lever R, Riffo-Vasquez Y, Ciferri S, Rose MJ, et al. Platelets are essential for leukocyte recruitment in allergic inflammation. J Allergy Clin Immunol. (2003) 112:109–18. doi: 10.1067/mai.2003.1514
125. Lussana F, Di Marco F, Terraneo S, Parati M, Razzari C, Scavone M, et al. Effect of prasugrel in patients with asthma: results of PRINA, a randomized, double-blind, placebo-controlled, cross-over study. J Thromb Haemost. (2015) 13:136–41. doi: 10.1111/jth.12779
126. Amison RT, Momi S, Morris A, Manni G, Keir S, Gresele P, et al. RhoA signaling through platelet P2Y(1) receptor controls leukocyte recruitment in allergic mice. J Allergy Clin Immunol. (2015) 135:528–38. doi: 10.1016/j.jaci.2014.09.032
127. Page CP. The involvement of platelets in non-thrombotic processes. Trends Pharmacol Sci. (1988) 9:66–71. doi: 10.1016/0165-6147(88)90120-4
128. Amison RT, Arnold S, O'shaughnessy BG, Cleary SJ, Ofoedu J, Idzko M, et al. Lipopolysaccharide (LPS) induced pulmonary neutrophil recruitment and platelet activation is mediated via the P2Y1 and P2Y14 receptors in mice. Pulm Pharmacol Ther. (2017) 45:62–8. doi: 10.1016/j.pupt.2017.05.005
129. Pan D, Amison RT, Riffo-Vasquez Y, Spina D, Cleary SJ, Wakelam MJ, et al. P-Rex and Vav Rac-GEFs in platelets control leukocyte recruitment to sites of inflammation. Blood. (2015) 125:1146–58. doi: 10.1182/blood-2014-07-591040
130. Moosbauer C, Morgenstern E, Cuvelier SL, Manukyan D, Bidzhekov K, Albrecht S, et al. Eosinophils are a major intravascular location for tissue factor storage and exposure. Blood. (2007) 109:995–1002. doi: 10.1182/blood-2006-02-004945
131. Cugno M, Marzano AV, Lorini M, Carbonelli V, Tedeschi A. Enhanced tissue factor expression by blood eosinophils from patients with hypereosinophilia: a possible link with thrombosis. PLoS ONE. (2014) 9:e111862. doi: 10.1371/journal.pone.0111862
132. Mateus Medina EF, Osuna Gomez R, Ujaldón Miró C, Crespo Lessman A, García Moral A, Curto Sánchez E, et al. Identification of a distinct eosinophil subpopulation in induced sputum from atopic asthmatic. Eur Respir J. (2018) 52(Suppl. 62):PA4457. doi: 10.1183/13993003.congress-2018.PA4457
Keywords: eosinophils, extracellular ATP, extracellular adenosine, CD39, CD73, P1 receptors, P2 receptors, inflammation
Citation: Ferrari D, Vuerich M, Casciano F, Longhi MS, Melloni E, Secchiero P, Zech A, Robson SC, Müller T and Idzko M (2020) Eosinophils and Purinergic Signaling in Health and Disease. Front. Immunol. 11:1339. doi: 10.3389/fimmu.2020.01339
Received: 02 April 2020; Accepted: 26 May 2020;
Published: 08 July 2020.
Edited by:
Detlef Neumann, Hannover Medical School, GermanyReviewed by:
Mats W. Johansson, University of Wisconsin-Madison, United StatesPaige Lacy, University of Alberta, Canada
Copyright © 2020 Ferrari, Vuerich, Casciano, Longhi, Melloni, Secchiero, Zech, Robson, Müller and Idzko. This is an open-access article distributed under the terms of the Creative Commons Attribution License (CC BY). The use, distribution or reproduction in other forums is permitted, provided the original author(s) and the copyright owner(s) are credited and that the original publication in this journal is cited, in accordance with accepted academic practice. No use, distribution or reproduction is permitted which does not comply with these terms.
*Correspondence: Davide Ferrari, ZGF2aWRlLmZlcnJhcmlAdW5pZmUuaXQ=; Fabio Casciano, ZmFiaW8uY2FzY2lhbm9AdW5pZmUuaXQ=