- 1Tumor Microenvironment Laboratory, QIMR Berghofer Medical Research Institute, Herston, QLD, Australia
- 2Faculty of Health, School of Biomedical Sciences, Queensland University of Technology, Brisbane, QLD, Australia
Within the tumor microenvironment, there is an intricate communication happening between tumor and stromal cells. This information exchange, in the form of cytokines, growth factors, extracellular vesicles, danger molecules, cell debris, and other factors, is capable of modulating the function of immune cells. The triggering of specific responses, including phenotypic alterations, can ultimately result in either immune surveillance or tumor cell survival. Macrophages are a well-studied cell lineage illustrating the different cellular phenotypes possible, depending on the tumor microenvironmental context. While our understanding of macrophage responses is well documented in vitro, surprisingly, little work has been done to confirm these observations in the cancer microenvironment. In fact, there are examples of opposing reactions of macrophages to cytokines in cell culture and in vivo tumor settings. Additionally, it seems that different macrophage lineages, for example tissue-resident and monocyte-derived macrophages, respond differently to cytokines and other cancer-derived signals. In this review article, we will describe and discuss the diverging reports on how cancer cells influence monocyte-derived and tissue-resident macrophage traits in vivo.
Introduction
Macrophages are key immune cells involved in the phagocytosis of foreign factors and debris, and the production of cytokines (1–3). Classic macrophages can respond to cancer cells upon exposure to tumor-associated antigens (4). However, macrophages that are associated with established tumors are usually known to produce anti-inflammatory cytokines and support tumor progression (5). These cancer-associated types of macrophages have also been associated with metastasis, including early metastatic steps such as pre-metastatic niche formation (6).
Macrophages were first described by Elie Metchnikoff in 1882, as mononuclear phagocytic cells important for animals' defense against bacterial infection (1). In the context of cancer, initial studies focused on their phagocytic function, and how their secretory products could act as proinflammatory and anti-tumor agents (1, 7, 8). For example, breast cancer patients presenting with tumor masses highly infiltrated by macrophages had a reduced risk of metastasis (9). In contrast, during 1990s, proangiogenic and protumoral roles for macrophages started to be suggested in diverse cancer types, including breast (10), lung (11), and ovarian cancer (12).
Studies on the biology of monocyte differentiation to macrophage helped understand the opposing inflammatory and anti-inflammatory roles of macrophages (13, 14). These studies demonstrated how cytokines are involved in monocyte differentiation. For example, Interferon-gamma (IFN-γ) assists in the initiation of immune responses (15). Macrophage activation by lipopolysaccharide (LPS) results in an increase in IL-12 production, a cytokine commonly found in the inflammatory environment (16). When macrophages are treated with LPS in combination with IFN-γ, for example, IL-12 secretion is up-regulated, with subsequent promotion of Th1 inflammatory responses (17). On the other hand, macrophages exposed to IL-4 or IL-10 are known to promote Th2 anti-inflammatory responses (18). Charles Milles introduced that macrophages activated by LPS and IFN-γ are commonly termed M1 macrophages; while IL-4 or IL-10 treated macrophages are termed M2 macrophages (19). Nevertheless, this simple M1/M2 macrophage polarization, induced by only several cytokines, is not sufficient to describe the broad macrophage variability observed in different disease models (20), particularly in cancer (21).
So far, most work has been done on investigating macrophage responses to recombinant cytokines in in vitro cell culture settings. Mostly, murine and human macrophage cell lines, including RAW and THP-1 cells, respectively, have been used for those studies. Based on this data, we have generated a thorough understanding of signaling pathways in macrophages, in response to cytokines and other stimuli. Intriguingly, there is a paucity of studies on how macrophages in in vivo cancer microenvironments respond to cytokines. In fact, some reports highlight stark discrepancies between the responses of cell-cultured macrophages to a cue when compared to the macrophages in a tissue context (22). For example, comparison of bone marrow-derived macrophage (BMDM) and Raw 264.7 cells by RNA sequencing and proteomics uncovered dissimilarity in response to inflammation (23, 24). Additionally, there are at least two distinct macrophage populations, with different origins and functions, present in a tumor. Our knowledge of the different roles these populations have to play in different phases of tumor progression and metastasis are even more limited. We will now discuss the state of knowledge for these macrophage populations in in situ, in vivo or ex vivo cancer microenvironmental settings.
Monocyte-Derived Macrophages and Tissue-Resident Macrophages: Origins and Phenotypes
Based on their origin, macrophages are classified into monocyte-derived macrophages or tissue-resident macrophages (25). Monocyte-derived macrophages originate from adult hematopoietic stem cells in the bone marrow (26). These macrophages are firstly distributed to tissues as monocytes, which can then differentiate to macrophages depending on organ-specific cues and circumstance (27). On the other hand, tissue-resident macrophages are suggested to originate from progenitor cells during embryonic or fetal development, and are not dependent on adult hematopoiesis (28, 29). These macrophages have self-renewal properties, as well as distinct features and names that depend on the organ in which they reside (30). The tissue-resident macrophages' nomenclature includes historical names, such as bone marrow, microglia (brain) (31), alveolar (lung) (32), Kupffer (liver) (30), and kidney macrophage (33).
Tissue-resident macrophages are highly heterogeneous, showing more variable levels of transcription factors and surface markers compared to monocyte-derived macrophages (Figure 1). Regarding the expression of surface markers, monocyte-derived macrophages are generally F4/80intermediate/CD11bhigh/MHC class IIhigh/CCR2high, while tissue-resident macrophages are usually identified by the F4/80high/CD11blow/Cx3CR1high/MHC class IIhigh/low/CCR2low immunophenotype (30). In addition, it has been suggested that the responses triggered within the cancer microenvironment are different between monocyte-derived macrophage and tissue-derived macrophages. In pancreatic tumor, for example, tissue-resident macrophages proliferate, and promote tumor progression and pro-fibrotic activity, while monocyte-derived macrophages do not affect tumor progression, but have potent roles in antigen presentation (34). Conversely, monocyte-derived macrophages accumulate in high numbers during lung injury, whereas tissue-resident macrophages persist in their numbers (35). Moreover, in vivo injections of either LPS or IL-4 trigger different responses in monocyte-derived and tissue-derived macrophages, both functionally and phenotypically (35, 36). These studies show that monocyte-derived and tissue-resident macrophages can display distinct characteristics in different conditions (Figure 1). Therefore, it is important to clearly identify these two populations of macrophages when assessing their roles in the tumor microenvironment, particularly how both cell subsets are differentially affected by tumor-derived factors.
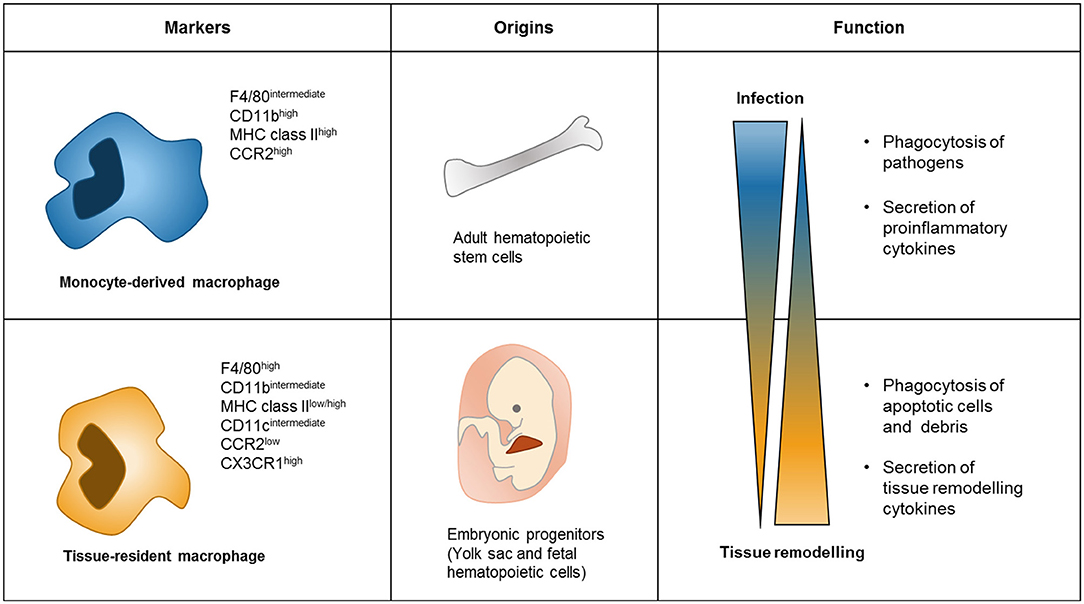
Figure 1. Difference between Monocyte-derived macrophages and tissue-resident macrophages. Monocyte-derived macrophages commonly express high levels of CD11b MHC class II and CCR2, while tissue-resident macrophages have high levels of F4/80 and CX3CR1 (30). Monocyte-derived macrophages begin from adult hematopoietic stem cells through monocyte differentiation. However, progenitors of tissue-resident macrophages are of embryonic origin and fetal hematopoietic cells, and maintain their number by self-renewal signaling in tissue (30). Functions of these two types of macrophages are different as well. Monocyte-derived macrophages act in infection conditions to phagocytosis pathogens and secretes cytokines related to proinflammatory conditions (35). In contrast, tissue-resident macrophages subsist in tissue for maintaining tissue homeostasis by phagocytosis apoptotic cells and secreting cytokines related to tissue remodeling (3).
Macrophages in the Cancer Microenvironment
The surrounding environment of macrophages has been reported to determine their polarization (37). For example, macrophages in either hypoxic or acidic conditions promote tumor progression more efficiently than cells derived from normoxic condition (38–40). This observation indicates that macrophage differentiation can be altered by extrinsic factors, hence impacting the cancer microenvironment. There is a vast difference between the cytokine profiles secreted from normal and cancer cells. It is known that many types of cancer cells secret a large range of extracellular mediators, including cytokines, chemokines and growth factors, such as the chemokine (C-C motif) ligand 2 (CCL2), IL-6, transforming growth factor beta (TGF-β), tumor necrosis factor alpha (TNF-α), matrix metalloproteinases (MMPs), and granulocyte-macrophage colony-stimulating factor (GM-CSF) (41, 42). These proteins play important roles in altering the phenotype of macrophages, but might affect monocyte-derived macrophages and tissue-resident macrophages differentially. The composition and amounts of cytokines secreted within the tumor microenvironment by both cancer and stromal cells partially determine the reaction of macrophages to a cancer, which could result in either promotion or suppression of immune responses, and consequent inhibited or sustained cancer growth, respectively.
Effects of Tumor-Derived Cytokines on Monocyte-Derived Macrophages
CCL2 is known to be highly abundant in the cancer microenvironment (43). It is shown to recruit CCR2+ highly inflammatory monocytes, which are then differentiated to F4/80+/CD11b+/Gr1− macrophages, promoting metastasis through VEGF-dependent mechanisms in breast cancer (44). Furthermore, F4/80+/CD11b+macrophages express higher levels of CCR2 than tissue resident macrophages, being recruited by CCL2 secreting tissues. CCL2, in turn, regulates CCR2+ macrophage signaling and induces, for example, secretion of CCL3 and consequent extravasation of cancer cells (45). Deletion of CCL3 in bone marrow-derived macrophages suppresses lung metastasis and reduces the recruitment of monocyte-derived macrophages to tumor site (45). In another study, CCL2 has been reported to play an important role in differentiating monocytes. CCL2 was incubated with human CD11b+ monocytes, causing them to differentiate to a CD14+/CD206+/CD11b+ population (46). These macrophages produce protumoral cytokines and are associated with a tumor promoting phenotype (46).
Several publications have demonstrated that IL-6 is also associated with macrophage polarization. For example, in the glioblastoma microenvironment, IL-6 and CSF-1, which are produced by glioblastoma-associated endothelial cells, increase arginase-1 expression, which is mediated by HIF-2α activation in monocyte-derived macrophages (47). Therefore, when IL-6 is knocked out in glioblastoma-associated endothelial cells, glioblastoma-bearing mice display an increased survival rate (47). Moreover, macrophages generated from peripheral blood monocytes and incubated with macrophage colony-stimulating factor (M-CSF) induce high levels of DC-SIGN, which could also be achieved by incubation of monocytes with conditioned media from cancer cell lines containing high levels of IL-6 and IL-10 (48). The DC-SIGN protein expression is commonly observed in macrophages found in patient tumor stroma, and has been reported to be associated with high levels of VEGF, as well as a proangiogenic phenotype (48, 49). TGF-β is also known to be secreted by various cancer cells, including breast (50), lung (51), and liver cancer cells (52). Among the cytokines secreted from hepatocellular carcinoma, TGF-β induced Tim3 signal, activated NF-kB and STAT6, increased IL-6 and IL-10, and decreased IL-1 in macrophages (53). The IL-6 released from these macrophages, but not IL-10 and GM-CSF, suggests that cancer cells are promoting their proliferation in a paracrine manner (53). When TGF-β is blocked by antibodies, human monocyte-derived macrophages differentiated by M-CSF have increased secretion of IL-10 and decreased levels of IL-12 (54). High levels of TNF-α are found in cancer and non-cancer proinflammatory environments (55). TNF-α produced by the cancer affects macrophage SINGLEC1 expression, which is found in high levels on tumor-associated macrophages (56). SINGLEC1 and CCL8 expression on macrophages are independent prognostic markers for poor survival (56). Taken together, these observations show that monocyte-derived macrophages secrete cytokines associated with tumor promotion or contribute to the cancer progression in response to cancer-derived cytokines.
Effect of Tumor-Derived Cytokines on Tissue-Resident Macrophages
There has been less research done on the role and responses of tissue-resident macrophages in the tumor microenvironment, leaving us with a sketchier understanding. However, several studies show distinguishing features of monocyte-derived and tissue-resident macrophages in tissues (2, 57). Additionally, there are several reports showing that tissue-resident macrophages are involved in tissue remodeling rather than inflammatory conditions (58). Despite these reports, it is still not clear what the exact role(s) of this tissue-resident macrophage in the tumor microenvironment are. It has been reported that the recruitment of macrophages in CCR2−/− mice to pancreatic ductal adenocarcinoma tumors were reduced (34). However, it was noted that this reduction did not affect pancreatic ductal adenocarcinoma tumor growth (2). This result suggests that pancreatic cancer growth is regulated by CCR2+ monocyte/macrophage-independent mechanisms (2). In contrast, this report demonstrated that these tissue-resident macrophages are able to self-renew in tumors due to tumor-derived CSF-1 and promote tumor progression (2). A recent study partially explained this phenotype by showing that CSF1/2 regulates both proliferation and angiogenic capacity of cardiac tissue-resident macrophage by regulating KFL4 levels (59). In ovarian cancer, bioinformatics analyses showed that tumor-associated macrophages are similar to tissue-resident macrophages, but not monocyte macrophages (60). At the same time, the phenotype and function of tumor-associated macrophages accumulating in breast cancer tumors is different from that of breast tissue-resident macrophages (61). As such, macrophage characteristics in each organ are different, and it differs in distinct tumor microenvironments. How the different macrophage types respond in a given situation in a cancer would therefore require further detailed studies.
Tumor-Derived Extracellular Vesicles
Small extracellular vesicles (sEVs) are derived from cells without being able to self-replicate (62). Fusion of multi-vesicular bodies (MVBs) with the plasma membrane allows the release of sEVs into the extracellular environment (63). These sEVs play an important role in the intercellular communication within the tumor microenvironment. Depending on the origin of the cell, the extracellular vesicles have a different content, and therefore, the extracellular vesicles released from the cancer cells are different from the corresponding normal cells (64). sEVs contain various bioactive compounds such as proteins, lipids, mRNAs and microRNAs (65–67). It has been reported that tumor-derived sEVs can be distributed to various organs and lymph nodes through blood and/or lymphatic vessels (68). These vesicles are not only found to be retained in distal tissues, but have also shown to be taken up, for example, by cells in the brain and bone marrow (68, 69). Therefore, it is reasonable to suggest that tumor-derived EVs are capable of changing the characteristics and behavior of their target cells. As such, these compounds have been shown to affect the phenotype and cellular function of recipient cells in different organs, including immune cells, and especially macrophages.
Effects Tumor-Derived Extracellular Vesicles in Monocyte-Derived Macrophages
Breast cancer-derived sEVs containing high levels of gp130 activate STAT3 signaling in monocyte-derived macrophages (70). The proportion of CD163+CD206highHLA-DRlow macrophages, derived from human blood CD14+ monocytes, was shown to be increased after incubation with hypoxic lung cancer-derived extracellular vesicles enriched for microRNA-103a (71). MicroRNA-103a in turn targets PTEN to activate AKT and STAT3 pathways (71). Y RNA, hY4 is another small non-coding RNA found in sEVs from chronic lymphocytic leukemia (72). These sEVs induced secretion of CCL2, CCL4, and IL-6, as well as expression of PD-L1, in monocytes and macrophages (72). Furthermore, these macrophages with activated AKT and STAT3 signaling pathways, and increased levels of PD-L1 induce a reduced immune response and therapeutic resistance (73). Altogether, these studies suggest that sEVs derived from cancer cells are capable of polarizing monocyte-derived macrophages to tumor-associated macrophages.
Effects of Tumor-Derived Extracellular Vesicles on Tissue-Resident Macrophages
Recently, there have been a number of reports suggesting that tumor-derived EVs induce premetastatic niche formation by altering myeloid cell phenotypes, including that of monocytes and macrophages (74, 75). Apart from these publications, there has not been much research reported on the impact of tumor-derived sEVs on tissue-resident macrophages. A few examples on the role of sEVs come from glioblastoma and microglia work, possibly due to brain-resident macrophages being mostly of embryonic origin (30). Glioblastoma stem-like cells secrete EVs, which induce membrane type 1-MMP (MT1-MMP) in microglia with strong tumor-supportive functions (76). Similarly, these vesicles are enriched for miR-451 and miR-21, which are transferred to microglia. In these cells, the microRNAs decrease c-myc levels, inducing gene expression alterations in favor of protumoral phenotypes (77). Additionally, sEVs might change extracellular matrix composition via MT1-MMP and c-myc, which induces cancer cells migration and invasion (78, 79). However, more research is required to understand the interactions and roles cancer-secreted EVs exert on tissue-resident macrophages.
Conclusion
Although much research has been conducted on understanding the responses of macrophages to cytokines and EVs, most of these studies were done in cell culture settings. While very informative, more recent literature suggests that the tissue-context dimension, which cannot be mimicked in cell culture, has an enormous impact on macrophage responses. Without a detailed knowledge of the macrophage-lineage specific response to certain stimuli in the tumor microenvironment context, it is very difficult to ascertain the roles and therefore, the best macrophage-targeting interventions in a cancer setting. This review described how tumors affect monocyte-derived and tissue-derived macrophages by dividing the roles of soluble factors and EVs (Figure 2). This summary and interpretation highlight some areas of cancer macrophage biology requiring further research so we can better understand the intricate relationship between cancer cells and macrophages. Many studies have evaluated the tumor microenvironment by using conditioned media, focusing on the role of single or small sets of cytokines and/or sEVs. However, regional differences within the microenvironment as well as spatial/regional regulation mechanisms of macrophages are not understood at any depth (69). It will be very exciting, using more comprehensive approaches in animal models and patient samples, to interrogate on single cell levels in situ how macrophages phenotypes are differentially regulated with important implications for our understanding of cancer progression and developing novel therapeutic approaches. Recently, many studies have been conducted targeting tumor-associated macrophages. For example, it has been reported that activation of CD206 by RP-182 on tumor-associated macrophages reprograms these cells and increases their anti-tumor activity (80). In addition, increased legumain, a lysosomal peptidase, in tumor-associated macrophages increases CD8 T cell activity (81). Understanding the macrophage origin and how these types of macrophages respond to the tumor microenvironment will be essential to develop better cancer therapies in the future.
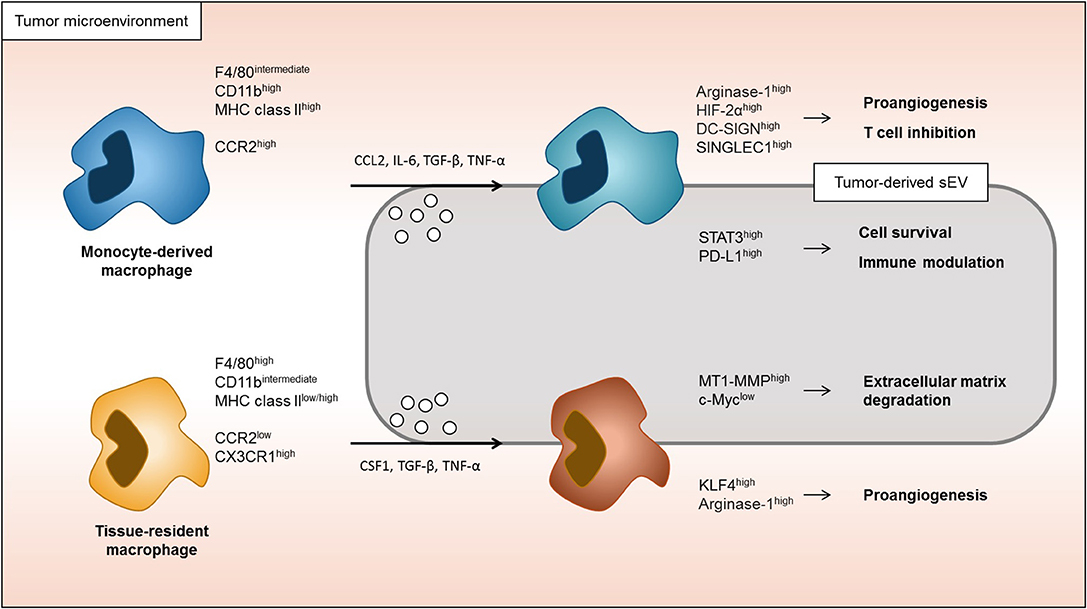
Figure 2. Response of monocyte-derived macrophages and tissue-resident macrophages in tumor microenvironment. Both monocyte-derived macrophages and tissue-resident macrophages are able to be associated to tumor progression. Monocyte-derived macrophages in tumor microenvironment are more induced to immune modulation and macrophage cells survival signaling. On the other hand, tissue-resident macrophages are more associated to the change in extracellular matrix and proangiogenic signaling.
Author Contributions
SH and AM wrote and reviewed the manuscript. LL and EL edited and reviewed the manuscript. All authors contributed to the article and approved the submitted version.
Funding
This work was funded by a grant from the National Breast Cancer Foundation Australia (IIRS-18-159) and the National Health and Medical Research Council Australia (APP1185907) to AM.
Conflict of Interest
The authors declare that the research was conducted in the absence of any commercial or financial relationships that could be construed as a potential conflict of interest.
Acknowledgments
The authors would like to thank the members of the Tumor Microenvironment Laboratory of QIMR Berghofer Medical Research Institute for critical input and proofreading of the manuscript.
References
1. Adams DO, Hamilton TA. The cell biology of macrophage activation. Annu Rev Immunol. (1984) 2:283–318. doi: 10.1146/annurev.iy.02.040184.001435
2. Zhu Y, Herndon JM, Sojka DK, Kim KW, Knolhoff BL, Zuo C, et al. Tissue-Resident macrophages in pancreatic ductal adenocarcinoma originate from embryonic hematopoiesis and promote tumor progression. Immunity. (2017) 47:323–38 e6. doi: 10.1016/j.immuni.2017.07.014
3. A-Gonzalez N, Quintana JA, Garcia-Silva S, Mazariegos M, Gonzalez de la Aleja A, Nicolas-Avila JA, et al. Phagocytosis imprints heterogeneity in tissue-resident macrophages. J Exp Med. (2017) 214:1281–96. doi: 10.1084/jem.20161375
4. van der Laan LJ, Dopp EA, Haworth R, Pikkarainen T, Kangas M, Elomaa O, et al. Regulation and functional involvement of macrophage scavenger receptor mARCO in clearance of bacteria in vivo. J Immunol. (1999) 162:939–47.
5. Haque A, Moriyama M, Kubota K, Ishiguro N, Sakamoto M, Chinju A, et al. CD206(+) tumor-associated macrophages promote proliferation and invasion in oral squamous cell carcinoma via eGF production. Sci Rep. (2019) 9:14611. doi: 10.1038/s41598-019-51149-1
6. Sharma SK, Chintala NK, Vadrevu SK, Patel J, Karbowniczek M, Markiewski MM. Pulmonary alveolar macrophages contribute to the premetastatic niche by suppressing antitumor t cell responses in the lungs. J Immunol. (2015) 194:5529–38. doi: 10.4049/jimmunol.1403215
7. Nathan CF. Secretory products of macrophages. J Clin Invest. (1987) 79:319–26. doi: 10.1172/JCI112815
8. Takemura R, Werb Z. Secretory products of macrophages and their physiological functions. Am J Physiol. (1984) 246(1 Pt 1):C1–9. doi: 10.1152/ajpcell.1984.246.1.C1
9. Lauder I, Aherne W, Stewart J, Sainsbury R. Macrophage infiltration of breast tumours: a prospective study. J Clin Pathol. (1977) 30:563–8. doi: 10.1136/jcp.30.6.563
10. O'Sullivan C, Lewis CE, Harris AL, McGee JO. Secretion of epidermal growth factor by macrophages associated with breast carcinoma. Lancet. (1993) 342:148–9. doi: 10.1016/0140-6736(93)91348-P
11. Vignaud JM, Marie B, Klein N, Plenat F, Pech M, Borrelly J, et al. The role of platelet-derived growth factor production by tumor-associated macrophages in tumor stroma formation in lung cancer. Cancer Res. (1994) 54:5455–63.
12. Erroi A, Sironi M, Chiaffarino F, Chen ZG, Mengozzi M, Mantovani A. IL-1 and iL-6 release by tumor-associated macrophages from human ovarian carcinoma. Int J Cancer. (1989) 44:795–801. doi: 10.1002/ijc.2910440508
13. Melief SM, Geutskens SB, Fibbe WE, Roelofs H. Multipotent stromal cells skew monocytes towards an anti-inflammatory interleukin-10-producing phenotype by production of interleukin-6. Haematologica. (2013) 98:888–95. doi: 10.3324/haematol.2012.078055
14. Sanford DE, Belt BA, Panni RZ, Mayer A, Deshpande AD, Carpenter D, et al. Inflammatory monocyte mobilization decreases patient survival in pancreatic cancer: a role for targeting the cCL2/CCR2 axis. Clin Cancer Res. (2013) 19:3404–15. doi: 10.1158/1078-0432.CCR-13-0525
15. Shirayoshi Y, Burke PA, Appella E, Ozato K. Interferon-induced transcription of a major histocompatibility class i gene accompanies binding of inducible nuclear factors to the interferon consensus sequence. Proc Natl Acad Sci USA. (1988) 85:5884–8. doi: 10.1073/pnas.85.16.5884
16. Lapara NJ, 3rd, Kelly BL. Suppression of lPS-induced inflammatory responses in macrophages infected with leishmania. J Inflamm (Lond). (2010) 7:8. doi: 10.1186/1476-9255-7-8
17. Dobashi K, Aihara M, Araki T, Shimizu Y, Utsugi M, Iizuka K, et al. Regulation of lPS induced iL-12 production by iFN-gamma and iL-4 through intracellular glutathione status in human alveolar macrophages. Clin Exp Immunol. (2001) 124:290–6. doi: 10.1046/j.1365-2249.2001.01535.x
18. Makita N, Hizukuri Y, Yamashiro K, Murakawa M, Hayashi Y. IL-10 enhances the phenotype of m2 macrophages induced by iL-4 and confers the ability to increase eosinophil migration. Int Immunol. (2015) 27:131–41. doi: 10.1093/intimm/dxu090
19. Mills CD, Kincaid K, Alt JM, Heilman MJ, Hill AM. M-1/M-2 macrophages and the th1/Th2 paradigm. J Immunol. (2000) 164:6166–73. doi: 10.4049/jimmunol.164.12.6166
20. Martinez FO, Gordon S. The m1 and m2 paradigm of macrophage activation: time for reassessment. F1000Prime Rep. (2014) 6:13. doi: 10.12703/P6-13
21. Benner B, Scarberry L, Suarez-Kelly LP, Duggan MC, Campbell AR, Smith E, et al. Generation of monocyte-derived tumor-associated macrophages using tumor-conditioned media provides a novel method to study tumor-associated macrophages in vitro. J Immunother Cancer. (2019) 7:140. doi: 10.1186/s40425-019-0622-0
22. Svedberg FR, Brown SL, Krauss MZ, Campbell L, Sharpe C, Clausen M, et al. The lung environment controls alveolar macrophage metabolism and responsiveness in type 2 inflammation. Nat Immunol. (2019) 20:571–80. doi: 10.1038/s41590-019-0352-y
23. Guo M, Hartlova A, Dill BD, Prescott AR, Gierlinski M, Trost M. High-resolution quantitative proteome analysis reveals substantial differences between phagosomes of rAW 264.7 and bone marrow derived macrophages. Proteomics. (2015) 15:3169–74. doi: 10.1002/pmic.201400431
24. Das A, Yang CS, Arifuzzaman S, Kim S, Kim SY, Jung KH, et al. High-Resolution mapping and dynamics of the transcriptome, transcription factors, and transcription co-Factor networks in classically and alternatively activated macrophages. Front Immunol. (2018) 9:22. doi: 10.3389/fimmu.2018.00022
25. Parwaresch MR, Wacker HH. Origin and kinetics of resident tissue macrophages. Parabiosis studies with radiolabelled leucocytes. Cell Tissue Kinet. (1984) 17:25–39. doi: 10.1111/j.1365-2184.1984.tb00565.x
26. van Furth R, Cohn ZA. The origin and kinetics of mononuclear phagocytes. J Exp Med. (1968) 128:415–35. doi: 10.1084/jem.128.3.415
27. Jakubzick C, Gautier EL, Gibbings SL, Sojka DK, Schlitzer A, Johnson TE, et al. Minimal differentiation of classical monocytes as they survey steady-state tissues and transport antigen to lymph nodes. Immunity. (2013) 39:599–610. doi: 10.1016/j.immuni.2013.08.007
28. Gomez Perdiguero E, Klapproth K, Schulz C, Busch K, Azzoni E, Crozet L, et al. Tissue-resident macrophages originate from yolk-sac-derived erythro-myeloid progenitors. Nature. (2015) 518:547–51. doi: 10.1038/nature13989
29. Sheng J, Ruedl C, Karjalainen K. Most tissue-Resident macrophages except microglia are derived from fetal hematopoietic stem cells. Immunity. (2015) 43:382–93. doi: 10.1016/j.immuni.2015.07.016
30. Mass E, Ballesteros I, Farlik M, Halbritter F, Gunther P, Crozet L, et al. Specification of tissue-resident macrophages during organogenesis. Science. (2016) 353:4238. doi: 10.1126/science.aaf4238
31. Tischer J, Krueger M, Mueller W, Staszewski O, Prinz M, Streit WJ, et al. Inhomogeneous distribution of iba-1 characterizes microglial pathology in alzheimer's disease. Glia. (2016) 64:1562–72. doi: 10.1002/glia.23024
32. Misharin AV, Morales-Nebreda L, Mutlu GM, Budinger GR, Perlman H. Flow cytometric analysis of macrophages and dendritic cell subsets in the mouse lung. Am J Respir Cell Mol Biol. (2013) 49:503–10. doi: 10.1165/rcmb.2013-0086MA
33. Munro DAD, Hughes J. The origins and functions of tissue-Resident macrophages in kidney development. Front Physiol. (2017) 8:837. doi: 10.3389/fphys.2017.00837
34. Zhu Y, Herndon JM, Sojka DK, Kim KW, Knolhoff BL, Zuo C, et al. Tissue-Resident macrophages in pancreatic ductal adenocarcinoma originate from embryonic hematopoiesis and promote tumor progression. Immunity. (2017) 47:597. doi: 10.1016/j.immuni.2017.08.018
35. Janssen WJ, Barthel L, Muldrow A, Oberley-Deegan RE, Kearns MT, Jakubzick C, et al. Fas determines differential fates of resident and recruited macrophages during resolution of acute lung injury. Am J Respir Crit Care Med. (2011) 184:547–60. doi: 10.1164/rccm.201011-1891OC
36. Gundra UM, Girgis NM, Ruckerl D, Jenkins S, Ward LN, Kurtz ZD, et al. Alternatively activated macrophages derived from monocytes and tissue macrophages are phenotypically and functionally distinct. Blood. (2014) 123:e110–22. doi: 10.1182/blood-2013-08-520619
37. Haas L, Obenauf AC. Allies or enemies-The multifaceted role of myeloid cells in the tumor microenvironment. Front Immunol. (2019) 10:2746. doi: 10.3389/fimmu.2019.02746
38. Casazza A, Laoui D, Wenes M, Rizzolio S, Bassani N, Mambretti M, et al. Impeding macrophage entry into hypoxic tumor areas by sema3A/Nrp1 signaling blockade inhibits angiogenesis and restores antitumor immunity. Cancer Cell. (2013) 24:695–709. doi: 10.1016/j.ccr.2013.11.007
39. Ohashi T, Aoki M, Tomita H, Akazawa T, Sato K, Kuze B, et al. M2-like macrophage polarization in high lactic acid-producing head and neck cancer. Cancer Sci. (2017) 108:1128–34. doi: 10.1111/cas.13244
40. El-Kenawi A, Gatenbee C, Robertson-Tessi M, Bravo R, Dhillon J, Balagurunathan Y, et al. Acidity promotes tumour progression by altering macrophage phenotype in prostate cancer. Br J Cancer. (2019) 121:556–66. doi: 10.1038/s41416-019-0542-2
41. Kano A. Tumor cell secretion of soluble factor(s) for specific immunosuppression. Sci Rep. (2015) 5:8913. doi: 10.1038/srep08913
42. Chen W, Qin Y, Wang D, Zhou L, Liu Y, Chen S, et al. CCL20 triggered by chemotherapy hinders the therapeutic efficacy of breast cancer. PLoS Biol. (2018) 16:e2005869. doi: 10.1371/journal.pbio.2005869
43. Kersten K, Coffelt SB, Hoogstraat M, Verstegen NJM, Vrijland K, Ciampricotti M, et al. Mammary tumor-derived cCL2 enhances pro-metastatic systemic inflammation through upregulation of iL1beta in tumor-associated macrophages. Oncoimmunology. (2017) 6:e1334744. doi: 10.1080/2162402X.2017.1334744
44. Qian BZ, Li J, Zhang H, Kitamura T, Zhang J, Campion LR, et al. CCL2 recruits inflammatory monocytes to facilitate breast-tumour metastasis. Nature. (2011) 475:222–5. doi: 10.1038/nature10138
45. Kitamura T, Qian BZ, Soong D, Cassetta L, Noy R, Sugano G, et al. CCL2-induced chemokine cascade promotes breast cancer metastasis by enhancing retention of metastasis-associated macrophages. J Exp Med. (2015) 212:1043–59. doi: 10.1084/jem.20141836
46. Roca H, Varsos ZS, Sud S, Craig MJ, Ying C, Pienta KJ. CCL2 and interleukin-6 promote survival of human cD11b+ peripheral blood mononuclear cells and induce M2-type macrophage polarization. J Biol Chem. (2009) 284:34342–54. doi: 10.1074/jbc.M109.042671
47. Wang Q, He Z, Huang M, Liu T, Wang Y, Xu H, et al. Vascular niche iL-6 induces alternative macrophage activation in glioblastoma through hIF-2alpha. Nat Commun. (2018) 9:559. doi: 10.1038/s41467-018-03050-0
48. Dominguez-Soto A, Sierra-Filardi E, Puig-Kroger A, Perez-Maceda B, Gomez-Aguado F, Corcuera MT, et al. Dendritic cell-specific iCAM-3-grabbing nonintegrin expression on M2-polarized and tumor-associated macrophages is macrophage-CSF dependent and enhanced by tumor-derived iL-6 and iL-10. J Immunol. (2011) 186:2192–200. doi: 10.4049/jimmunol.1000475
49. Merlotti A, Malizia AL, Michea P, Bonte PE, Goudot C, Carregal MS, et al. Aberrant fucosylation enables breast cancer clusterin to interact with dendritic cell-specific iCAM-grabbing non-integrin (DC-SIGN). Oncoimmunology. (2019) 8:e1629257. doi: 10.1080/2162402X.2019.1629257
50. Lucke CD, Philpott A, Metcalfe JC, Thompson AM, Hughes-Davies L, Kemp PR, et al. Inhibiting mutations in the transforming growth factor beta type 2 receptor in recurrent human breast cancer. Cancer Res. (2001) 61:482–5.
51. de Jonge RR, Garrigue-Antar L, Vellucci VF, Reiss M. Frequent inactivation of the transforming growth factor beta type iI receptor in small-cell lung carcinoma cells. Oncol Res. (1997) 9:89–98.
52. Shirai Y, Kawata S, Tamura S, Ito N, Tsushima H, Takaishi K, et al. Plasma transforming growth factor-beta 1 in patients with hepatocellular carcinoma. Comparison with chronic liver diseases. Cancer. (1994) 73:2275–9. doi: 10.1002/1097-0142(19940501)73:9<2275::AID-CNCR2820730907>3.0.CO;2-T
53. Yan W, Liu X, Ma H, Zhang H, Song X, Gao L, et al. Tim-3 fosters hCC development by enhancing tGF-beta-mediated alternative activation of macrophages. Gut. (2015) 64:1593–604. doi: 10.1136/gutjnl-2014-307671
54. Kelly A, Gunaltay S, McEntee CP, Shuttleworth EE, Smedley C, Houston SA, et al. Human monocytes and macrophages regulate immune tolerance via integrin alphavbeta8-mediated tGFbeta activation. J Exp Med. (2018) 215:2725–36. doi: 10.1084/jem.20171491
55. Martinez-Reza I, Diaz L, Garcia-Becerra R. Preclinical and clinical aspects of tNF-alpha and its receptors tNFR1 and tNFR2 in breast cancer. J Biomed Sci. (2017) 24:90. doi: 10.1186/s12929-017-0398-9
56. Cassetta L, Fragkogianni S, Sims AH, Swierczak A, Forrester LM, Zhang H, et al. Human tumor-Associated macrophage and monocyte transcriptional landscapes reveal cancer-Specific reprogramming, biomarkers, and therapeutic targets. Cancer Cell. (2019) 35:588–602 e10. doi: 10.1016/j.ccell.2019.02.009
57. Misharin AV, Morales-Nebreda L, Reyfman PA, Cuda CM, Walter JM, McQuattie-Pimentel AC, et al. Monocyte-derived alveolar macrophages drive lung fibrosis and persist in the lung over the life span. J Exp Med. (2017) 214:2387–404. doi: 10.1084/jem.20162152
58. Wynn TA, Vannella KM. Macrophages in tissue repair, regeneration, and fibrosis. Immunity. (2016) 44:450–62. doi: 10.1016/j.immuni.2016.02.015
59. Liao X, Shen Y, Zhang R, Sugi K, Vasudevan NT, Alaiti MA, et al. Distinct roles of resident and nonresident macrophages in nonischemic cardiomyopathy. Proc Natl Acad Sci USA. (2018) 115:E4661–E9. doi: 10.1073/pnas.1720065115
60. Finkernagel F, Reinartz S, Lieber S, Adhikary T, Wortmann A, Hoffmann N, et al. The transcriptional signature of human ovarian carcinoma macrophages is associated with extracellular matrix reorganization. Oncotarget. (2016) 7:75339–52. doi: 10.18632/oncotarget.12180
61. Franklin RA, Liao W, Sarkar A, Kim MV, Bivona MR, Liu K, et al. The cellular and molecular origin of tumor-associated macrophages. Science. (2014) 344:921–5. doi: 10.1126/science.1252510
62. Théry C, Witwer KW, Aikawa E, Alcaraz MJ, Anderson JD, Andriantsitohaina R, et al. Minimal information for studies of extracellular vesicles 2018 (MISEV2018): a position statement of the international society for extracellular vesicles and update of the mISEV2014 guidelines. J Extrac Ves. (2018) 7:1535750. doi: 10.1080/20013078.2018.1461450
63. Hessvik NP, Llorente A. Current knowledge on exosome biogenesis and release. Cell Mol Life Sci. (2018) 75:193–208. doi: 10.1007/s00018-017-2595-9
64. Kosaka N, Kogure A, Yamamoto T, Urabe F, Usuba W, Prieto-Vila M, et al. Exploiting the message from cancer: the diagnostic value of extracellular vesicles for clinical applications. Exp Mol Med. (2019) 51:31. doi: 10.1038/s12276-019-0219-1
65. Valadi H, Ekstrom K, Bossios A, Sjostrand M, Lee JJ, Lotvall JO. Exosome-mediated transfer of mRNAs and microRNAs is a novel mechanism of genetic exchange between cells. Nat Cell Biol. (2007) 9:654–9. doi: 10.1038/ncb1596
66. Jakobsen KR, Paulsen BS, Baek R, Varming K, Sorensen BS, Jorgensen MM. Exosomal proteins as potential diagnostic markers in advanced non-small cell lung carcinoma. J Extracell Vesicles. (2015) 4:26659. doi: 10.3402/jev.v4.26659
67. Sansone P, Savini C, Kurelac I, Chang Q, Amato LB, Strillacci A, et al. Packaging and transfer of mitochondrial dNA via exosomes regulate escape from dormancy in hormonal therapy-resistant breast cancer. Proc Natl Acad Sci USA. (2017) 114:E9066–E75. doi: 10.1073/pnas.1704862114
68. Wiklander OP, Nordin JZ, O'Loughlin A, Gustafsson Y, Corso G, Mager I, et al. Extracellular vesicle in vivo biodistribution is determined by cell source, route of administration and targeting. J Extracell Vesicles. (2015) 4:26316. doi: 10.3402/jev.v4.26316
69. Wen SW, Sceneay J, Lima LG, Wong CS, Becker M, Krumeich S, et al. The biodistribution and immune suppressive effects of breast cancer-Derived exosomes. Cancer Res. (2016) 76:6816–27. doi: 10.1158/0008-5472.CAN-16-0868
70. Ham S, Lima LG, Chai EPZ, Muller A, Lobb RJ, Krumeich S, et al. Breast cancer-Derived exosomes alter macrophage polarization via gp130/STAT3 signaling. Front Immunol. (2018) 9:871. doi: 10.3389/fimmu.2018.00871
71. Hsu YL, Hung JY, Chang WA, Jian SF, Lin YS, Pan YC, et al. Hypoxic lung-Cancer-Derived extracellular vesicle microRNA-103a increases the oncogenic effects of macrophages by targeting pTEN. Mol Ther. (2018) 26:568–81. doi: 10.1016/j.ymthe.2017.11.016
72. Haderk F, Schulz R, Iskar M, Cid LL, Worst T, Willmund KV, et al. Tumor-derived exosomes modulate pD-L1 expression in monocytes. Sci Immunol. (2017) 2:5509. doi: 10.1126/sciimmunol.aah5509
73. Irey EA, Lassiter CM, Brady NJ, Chuntova P, Wang Y, Knutson TP, et al. JAK/STAT inhibition in macrophages promotes therapeutic resistance by inducing expression of protumorigenic factors. Proc Natl Acad Sci USA. (2019) 116:12442–51. doi: 10.1073/pnas.1816410116
74. Plebanek MP, Angeloni NL, Vinokour E, Li J, Henkin A, Martinez-Marin D, et al. Pre-metastatic cancer exosomes induce immune surveillance by patrolling monocytes at the metastatic niche. Nat Commun. (2017) 8:1319. doi: 10.1038/s41467-017-01433-3
75. Chen XW, Yu TJ, Zhang J, Li Y, Chen HL, Yang GF, et al. CYP4A in tumor-associated macrophages promotes pre-metastatic niche formation and metastasis. Oncogene. (2017) 36:5045–57. doi: 10.1038/onc.2017.118
76. de Vrij J, Maas SL, Kwappenberg KM, Schnoor R, Kleijn A, Dekker L, et al. Glioblastoma-derived extracellular vesicles modify the phenotype of monocytic cells. Int J Cancer. (2015) 137:1630–42. doi: 10.1002/ijc.29521
77. van der Vos KE, Abels ER, Zhang X, Lai C, Carrizosa E, Oakley D, et al. Directly visualized glioblastoma-derived extracellular vesicles transfer rNA to microglia/macrophages in the brain. Neuro Oncol. (2016) 18:58–69. doi: 10.1093/neuonc/nov244
78. Perentes JY, Kirkpatrick ND, Nagano S, Smith EY, Shaver CM, Sgroi D, et al. Cancer cell-associated mT1-MMP promotes blood vessel invasion and distant metastasis in triple-negative mammary tumors. Cancer Res. (2011) 71:4527–38. doi: 10.1158/0008-5472.CAN-10-4376
79. Schaub FX, Dhankani V, Berger AC, Trivedi M, Richardson AB, Shaw R, et al. Pan-cancer alterations of the mYC oncogene and its proximal network across the cancer genome atlas. Cell Syst. (2018) 6:282–300 e2. doi: 10.1016/j.cels.2018.03.003
80. Jaynes JM, Sable R, Ronzetti M, Bautista W, Knotts Z, Abisoye-Ogunniyan A, et al. Mannose receptor (CD206) activation in tumor-associated macrophages enhances adaptive and innate antitumor immune responses. Sci Transl Med. (2020) 12:530. doi: 10.1126/scitranslmed.aax6337
Keywords: tumor microenvironment, tissue-resident macrophage, monocyte-derived macrophage, small extracellular vesicles, tumor-derived cytokines
Citation: Ham S, Lima LG, Lek E and Möller A (2020) The Impact of the Cancer Microenvironment on Macrophage Phenotypes. Front. Immunol. 11:1308. doi: 10.3389/fimmu.2020.01308
Received: 14 February 2020; Accepted: 22 May 2020;
Published: 23 June 2020.
Edited by:
Baoli Hu, University of Pittsburgh, United StatesReviewed by:
Amorette Barber, Longwood University, United StatesJulie Tabiasco, Institut National de la Santé et de la Recherche Médicale (INSERM), France
Copyright © 2020 Ham, Lima, Lek and Möller. This is an open-access article distributed under the terms of the Creative Commons Attribution License (CC BY). The use, distribution or reproduction in other forums is permitted, provided the original author(s) and the copyright owner(s) are credited and that the original publication in this journal is cited, in accordance with accepted academic practice. No use, distribution or reproduction is permitted which does not comply with these terms.
*Correspondence: Andreas Möller, YW5kcmVhcy5tb2xsZXImI3gwMDA0MDtxaW1yYmVyZ2hvZmVyLmVkdS5hdQ==