- 1Department of Pathobiology Diagnostic Investigation, College of Veterinary Medicine, Michigan State University, East Lansing, MI, United States
- 2Institute for Integrative Toxicology, Michigan State University, East Lansing, MI, United States
- 3Cell and Molecular Biology Program, Michigan State University, East Lansing, MI, United States
- 4Department of Microbiology and Molecular Genetics, Michigan State University, East Lansing, MI, United States
Successful pregnancy outcome is partially determined by the suppression of reactive effector T cells by maternal regulatory T cells (TRegs) at the maternal-fetal interface. While a large area of research has focused on the regulation of peripherally-induced TReg (pTReg) distribution and differentiation using transgenic mouse models and human samples, studies focusing on the role of TRegs derived from the thymus (tTRegs), and the potential role of central tolerance in maternal-fetal tolerance is less explored. The genome of the fetus is composed of both the tissue-specific and paternally-inherited antigens, and a break in maternal immune tolerance to either antigen may result in adverse pregnancy outcomes. Notably, “self”-antigens, including antigens that are highly restricted to the fetus and placenta, are promiscuously expressed by medullary thymic epithelial cells under the control of Autoimmune Regulator (Aire), which skews the tTReg T cell receptor (TCR) repertoire to be specific toward these antigens. TRegs that circulate in mothers during pregnancy may be comprised of TRegs that stem from the thymus as well as those induced in the periphery. Moreover, despite a wealth of research dedicated to elucidating the function of TRegs in maternal-fetal tolerance, little is understood about the origin of these cells, and whether/how tTRegs may contribute. Investigation into this question is complicated by the absence of reliable markers to distinguish between the two. In this review, we discuss how distinct types of fetal/placental antigens may determine the generation of different subtypes of TReg cells in the mother, and in turn how these may promote maternal tolerance to the fetus in pregnancy.
Regulatory T Cells Are Critical Players in Pregnancy Outcome
The immunological paradox of the semi-allogeneic fetus has been a key question in reproductive physiology and immunology for decades. There are few examples in which one molecule or cell type can account for maternal tolerance to the fetus; an exception is CD4+CD25+Foxp3+ regulatory T (TReg) cells. TReg cells were identified after years of controversy based on their expression of the IL2α receptor, CD25, and their critical role in protection against autoimmune disease (1). Many studies in mice have demonstrated the indispensable role of CD4+CD25+Foxp3+ TRegs for maintaining immune homeostasis (2); mice lacking functional Foxp3, the critical lineage-determining factor for TReg cells, develop a phenotype characterized by scaly, ruffled skin, and an enlarged spleen (3–5). In humans, Foxp3 mutations result in IPEX syndrome (immune dysregulation, polyendocrinopathy, enteropathy, X-linked). Both humans and mice lacking functional Foxp3 suffer severe autoimmune disease and are at high risk of early death due to autoimmune disease caused by a deficiency in TReg cells.
The additional role of TReg cells in pregnancy was identified in a seminal study that used a depletion/replacement experimental strategy in mice to show that these cells are critical for immunological protection of the semiallogeneic fetus (6, 7). This finding has been independently verified by many laboratories, with the additional finding that TReg cells are particularly important around the time of implantation (8–11); interestingly, Rowe et al. (12) cautioned that TReg cells could be detrimental to pregnancy in terms of potentially limiting the ability of mothers to fight infection. Information regarding TReg cells in human pregnancy has been obtained from peripheral blood and samples obtained at the maternal fetal interface from pregnant women: initial studies compared decidual and peripheral blood TReg cells in the first trimester of healthy pregnancy and pregnancies complicated by spontaneous abortion (13). The proportion of TReg cells in the decidua was ~3-fold higher than in peripheral blood, suggesting recruitment and/or in situ proliferation of these cells. Moreover, decidual TReg cells were significantly decreased in spontaneous abortion cases. Further studies of spontaneous abortion and pre-eclampsia have supported the notion that optimal TReg cell responses are necessary to avoid detrimental pregnancy outcomes in women (14, 15). Collectively, these studies suggest that generation and recruitment of TRegs to the maternal fetal interface are important in protecting optimal survival of the allogeneic fetus, while maintaining the ability of the mother to fight infection during pregnancy.
Origins of TReg Cells and Their Cognate Antigens
CD4+CD25+Foxp3+ TReg cells arise from two overarching mechanisms: during thymocyte development and differentiation in the thymus, or by differentiation of circulating peripheral CD4+ cells following their exit from the thymus. Peripherally induced TReg (pTReg) result from the conversion of mature circulating conventional CD4+CD25- T cells into TReg cells in response to low-dose foreign antigens (2). Such is the case in Gut-Associated Lymphoid Tissue (GALT) and lymph nodes (LNs) draining the intestines, where pTReg cells with T cell receptor (TCR) specific to gut microbiota are found (16). These TReg develop in response to TCR and TGF-ß signaling through binding of NFAT (Nuclear Factor of Activated T cells) and Smad3 (Mothers against decapentaplegic homolog 3) to the CNS1 (Conserved Noncoding Sequence 1) element in the promoter region of Foxp3 (17). CNS1 is indispensable for the generation of pTRegs: in CNS1-deficient mice, induction of Foxp3 in naïve CD4+ T cells and consequent generation of pTReg was impaired in vivo (18). Importantly, Foxp3 expression is not sustained in pTReg cells if TGF-ß is removed; thus, stability of Foxp3 expression and functional activity of pTReg cells are relatively low (2, 19).
The necessity of the CNS1 element was also investigated in pregnancy. It appears logical to expect that pTReg cells are key in pregnancy success: antigens inherited from the father could be neither present nor expressed by the maternal thymic genome—a key component of thymic T cell tolerance and generation of thymic TReg (tTReg). Instead, introduction of paternal alloantigens at coitus, and later as conceptus, could induce the generation of pTRegs. Intriguingly, the Foxp3 binding site within CNS1 is highly conserved among placental mammals, in which pregnancy involves long, sustained, direct contact between maternal and fetal cells; the binding site was not conserved in non-eutherian mammals (e.g., marsupials) and non-mammals (20). In the same study, expansion of TReg cells in allogeneically-mated females appeared to be dependent on CNS1, and rates of resorption, albeit relatively low overall, were higher in CNS1-deficient mice in comparison to CNS1-sufficient mice, as well as in comparison to syngeneically-bred controls. This work agrees with that of Rowe et al. who found that adoptively transferred naïve CD4+ T cells with specificity to a surrogate paternally-inherited antigen upregulated Foxp3 expression and gained protective function during pregnancy (21, 22).
Other studies, however, implicate the importance of TReg generated in the thymus—tTReg–in establishing maternal tolerance to the fetus. These cells commit to the TReg lineage as early as the CD4+CD8+ double-positive stage of T cell development in a manner dependent on TCR and IL-2 signaling (23). In the thymic medulla, single-positive CD4+ T cells with properly arranged TCRs can develop into Foxp3+ TReg after encountering self-antigen/MHC II complexes expressed by thymic antigen presenting cells (APC) (24). High affinity/avidity signals from self-antigen/MHC II through the TCR leads to upregulation of CD25 and increased sensitivity to IL-2 (25–28). In contrast to pTReg cells, in which Foxp3 expression is relatively unstable, Foxp3 expression in tTReg cells are highly stable due to sustained demethylation of the CNS2 region of the promoter (29–31). For a more detailed comparison between the developmental requirements of tTReg and pTReg, readers are referred to excellent reviews (2, 32).
The relative Foxp3 stability of tTReg over pTReg may have important implications for pregnancy: thymus-derived TReg cells may offer a distinct functional advantage over pTReg cells given the length of pregnancy and need for long-lasting tolerance. Further, tTRegs and pTRegs may possess dissimilar TCR repertoires due to the source of and mode of exposure of T cells to fetal/placental antigens. Indeed, one may categorize fetal antigens into two groups: those arising exogenously after inheritance from the father, and tissue-specific antigens. Exogenous fetal/placental antigens include paternally-inherited major and minor histocompatibility antigens that differ between parents (33); these are known to elicit maternal T cell reactivity (34). On the other hand, fetus- and/or placenta-specific antigens may arise not due to their mode of inheritance, but simply as a result of being highly tissue-specific, and as such, perceived as antigenic by the mother.
It is currently unknown whether fetus/placenta-specific antigens promote TReg cell generation in the mother during pregnancy. However, thymic generation of tissue-specific TReg cells is critical for tolerance to many tissues. As explained further below, the expression of tissue-specific proteins occurs in the thymus under the control of the transcriptional regulator Aire (Autoimmune Regulator), and leads to both deletion of autoreactive conventional T cells and generation of tissue-specific TReg cells (35–37). Absence of functional Aire is associated with severely reduced fertility and early pregnancy loss in both mice and in women, and a number of fetus- and placenta-specific antigens are expressed in the thymus in an Aire-dependent manner (38–41). Additionally, tissue-specific antigens may access the thymus via the bloodstream (42); studies using TCR transgenic mice show that fetal antigen may do so (43). The possibility that fetal/placental antigens access or are expressed in the thymus raises the possibility that tTReg arise as a result, and further, that they possess TCR repertoires distinct from pTReg cells. Thus, distinct types/sources of antigens may determine the type and relative stability of TReg cells that are formed and are thus responsible for tolerance to fetal/placental antigens during pregnancy. Whether these cells have additional distinct roles in the local environment (i.e., at the maternal-fetal interface) or systemically is unknown, but this warrants investigation.
Identification of pTreg And tTReg
Due to their distinct developmental mechanism, potential differences in TCR repertoire, antigen specificity, and differential stability, a mechanism is required to reveal their contribution to maternal-fetal tolerance in pregnancy. To this end, Helios, an ikaros transcription factor family (44), and neuropilin-1 (Nrp-1), a single transmembrane receptor (45, 46), offer tools for distinguishing tTReg cells from pTReg cells; however, our ability to do so remains imperfect. Using mouse models, Singh et al. demonstrated higher expression of Helios than Nrp1 in thymic CD4+CD8-CD25+ cells compared to pancreas draining LN and spleen, suggesting that Helios may be superior to Nrp1 to detect tTReg (47). However, this study did not rule out the possibility that Helios and Nrp1 could be induced in the periphery and thus may also identify T cells that are recirculating back into the thymus. Comparison of TCR repertoires between Helios-sufficient and Helios-deficient TReg from mesenteric LNs has shown both similarities (48) and differences (49) between the two populations, suggesting that Helios may not be a reliable marker for tTReg. Helios can also be induced in culture on CD4+ T conventional cells by inflammatory stimuli (50–53), and in humans, both Helios+ and Helios- Foxp3+ tTReg cell populations can be found (54). Collectively, these data suggest that using presence of Helios alone to discriminate between tTReg and pTReg is insufficient (32).
Nrp1 is a multifunctional single-pass transmembrane receptor that participates in axonal growth and angiogenesis as well as in immune regulatory functions (55). Nrp1 was shown in early studies to be highly expressed by CD4+CD25+ T cells with suppressive capability, but not on naïve CD4+CD25- T cells (56, 57). Nrp1 functions to increase sensitivity of TReg to antigen and permit prolonged interaction with immature dendritic cells (57). Further, its expression is associated with prolonged survival of allogeneic grafts in mice (58) as well as demethylation at the CNS2 region of the Foxp3 locus (59), which is characteristic of tTRegs. On the other hand, 90% of CD4+CD8+ DP cells in the thymus are Nrp1+ (60) and can also be induced in conventional CD4+ T cells in vitro (58). Thus, further studies are required to discern whether expression of Nrp1 is constitutive or inducible in tTRegs in vivo and in vitro.
Our inability to discriminate tTReg cells from recirculating pTReg cells within the thymus has hampered our ability to study these subpopulations. A recent discovery, however, has provided a fresh approach (61). Using CD73, an ectonuclease receptor that converts adenosine monophosphate to adenosine (62), together with Rag2-GFP animals, CD73-expressing cells were found to represent mature peripheral recirculating cells, whereas CD73-negative cells represented newly made thymic T cells. This study also showed that tTReg progenitors initially possess an immature CD24hiQa-2lo phenotype (61), subsequently progressing to more mature CD24loQa-2hi profile. Interestingly, one of the first studies to identify Nrp1 as a potential marker for tTReg showed that the Nrp1lo population in the thymus is of the same immature CD24hiQa-2lo phenotype while the vast majority of Nrp1hi cells represented the more mature CD24loQa-2hi subset (45). Thus, the findings of these two studies suggest that immature CD73-negative tTReg progenitors may acquire Nrp1 expression as they mature through progenitor stages in the thymus.
Similarly, using a dual reporter mouse model for Foxp3 and Helios, Thornton et al. simultaneously measured both markers in T cells isolated from mesenteric LN, and found lower expression of CD73 in Helios-deficient TRegs compared to Helios-sufficient TRegs (49). Therefore, while CD73 expression can confidently be used to distinguish between newly generated thymic cells from recirculating peripheral cells, we still lack conclusive markers to differentiate between peripheral and thymic TReg cells in the peripheral lymphoid organs or peripheral blood (63). It is worthwhile to investigate whether CD73 expression can be gained by tTRegs as they exit the thymus, and whether CD73-negative cells in the thymus express Nrp1 and/or Helios. These findings may provide effective means to distinguish tTRegs and pTRegs.
Could Tolerance to the Fetus be Driven by tTReg Cells in Pregnancy?
In both mice and women, changing dynamics of TReg cells are evident early in pregnancy. In women, abundance of TReg cells in the uterus fluctuates with the menstrual cycle (64), and during pregnancy, TReg cell accumulation in the first trimester decidua is associated with their decrease in the peripheral blood (65). Similarly, in mice, TReg cells accumulate in the uterus as early as coitus (9). While these early trafficking patterns suggest that TReg cells migrate to the maternal-fetal interface from the peripheral circulation, they do not reveal the origin of TReg cells that accumulate there.
Although there is evidence supporting a role for pTRegs in pregnancy (20), emerging evidence also suggests that tTReg are important. There are conspicuous changes that occur in the thymus during pregnancy, even as early as the first trimester in the mouse. Under the control of ovarian hormones, the murine thymus gradually declines in size and cellularity, ultimately losing up to 90% of its cells by late gestation (66–68). The thymus normally exports millions of new T cells daily; however, during pregnancy, thymocyte output is reduced (69, 70). Older studies suggest that similar pregnancy-associated reductions in thymic mass also occur in women (63, 71). Loss of thymocytes in mice is accompanied by decreased recruitment of thymocyte progenitors from the bone marrow, loss of proliferative activity by early stage CD4-CD8- double negative thymocytes, and reduced production of chemokines and growth factors regulating immigration, proliferation, and migration of thymocytes and their precursors (68, 70, 72). All populations of CD4+ and CD8+ T cells appear to be similarly affected, including TReg cells (72). These changes have been postulated to be necessary to diminish the output of effector T cells with fetal/paternal antigen specificity and/or to promote production of lymphocytes beneficial for pregnancy (68, 73). One study showed that thymic involution and pregnancy outcome influence each other; When thymus involution is prevented in dams during pregnancy, the number of viable implantation sites decreased and number of resorbed sites increased (68). Deletion of fetal/paternal antigens in the thymus is possible but to our knowledge, no studies have shown this to be the case. More studies are needed to elucidate the role of the thymus in pregnancy outcome.
One possibility is that the thymic changes during pregnancy result in altered/increased output of highly stable tTReg cells with antigenic specificity to the fetus. Evidence supporting this is, however, conflicting. In mice, absolute numbers of CD4+CD25hi T cells in the thymus are reduced; however, their relative proportions are maintained or increased (70, 72). In women, Heliburg et al. reported maintained output of tTReg cells during pregnancy (74), while Wagner et al. found a decrease in circulating recent thymic emigrant (RTE) tTReg (75). Despite this decrease, however, these authors found increased proliferative activity of RTE-tTRegs with superior suppressive activity compared to more mature TReg cells. Consistent with this idea, in mice, tTReg cells as defined by Nrp1 expression and demethylated status of the CNS2, proliferate in the uterus-draining para-aortic LNs as early as GD3.5 (59). The presence of organ-specific antigens can trigger local expansion of organ-specific TReg cells in draining LNs with considerable inter-organ differences in TCR usage by TReg (16). As such, tTReg in uterus-draining LNs are likely responding specifically to fetal/paternal antigens in the para-aortic LNs by proliferation (59). The notion of early recruitment of tTReg cells to the decidua was also suggested by Teles et al. who demonstrated increased Foxp3+ Helios+ TReg cells in the thymus, uterus-draining LNs, and uterus by day 2 of murine pregnancy (76). Collectively, these studies in women and in mice support the idea that tTReg cells may expand and proliferate in the peripheral LNs during pregnancy and may provide strong suppressive activity.
Is There a Role of Maternal Thymic Aire in Immune Tolerance to Fetal antigens?
An individual's capacity to tolerate self and respond to foreign antigen is determined by the TCR repertoire of conventional CD4+ and CD8+ T cells, and Foxp3+ TReg cells in the periphery. An important determinant of the CD4+ T cell and TReg TCR repertoire is Aire, which is expressed primarily by medullary thymic epithelial cells (mTECs) (35) and has an unusual mechanism of action that promotes transcription of genes marked with transcriptional silencers. As a result, Aire induces the expression of a wide range of chromosomally clustered genes that are otherwise restricted to certain tissues (77–79). This expression results in direct or indirect presentation of tissue-restricted antigens in the context of major histocompatibility complex (MHC) molecules by mTEC or resident dendritic cells, respectively (80). Thymocytes with TCR specificity for Aire-regulated self-antigens may then undergo one of two possible fates: negative selection, wherein the autoreactive cells undergo apoptosis, or differentiation into self-antigen-specific tTReg cells (37, 81, 82).
Aire-mediated tolerance provided by this mechanism is pivotal: in humans, genomic mutations in Aire gene result in a devastating autosomal recessive monogenic disease called Autoimmune Polyglandular Syndrome type 1 (APS-1), with prototypical clinical manifestations that include hypoparathyroidism, primary adrenocortical insufficiency, and chronic mucocutaneous candidiasis (83, 84). Mice lacking Aire vary in phenotype according to their genetic background strain: those on the C57BL/6 background experience only mild autoimmune disease with only few affected organs, whereas those on the SJL/J and NOD backgrounds succumb to fatal disease. Aire-deficient mice on the Balb/c backgrounds experience intermediate disease (85).
Autoimmunity in Aire-deficient mice is at least partially explained by alterations in tTReg development. Knockout mice show reductions in tTReg cell numbers (86), and autoimmune disease in neonatal NOD mice lacking Aire can be prevented by donor TReg from Aire-sufficient mice (87). Malchow et al. demonstrated that T cells infiltrating the prostate in knockouts possessed TCR repertoires preferentially expressed by TReg cells in Aire-sufficient mice, suggesting that Aire enforces tolerance to self-antigen by driving autoreactive T cells toward the tTReg lineage (37).
Genes regulated by Aire represent virtually all tissues in the body, including reproductive tissues such as the ovary, uterus, and male reproductive tract. Gene expression profile studies of human and murine TEC reveal that Aire also regulates genes restricted to developmentally important tissues, including the pre- and post-implantation embryo, fetus, and placenta (41, 88). RNA sequencing analysis of mTECs from 6 to 8 week old WT and Aire-deficient mice revealed representation of placental antigens within the top 10 represented organs overall (88). Additionally, Gotter et al. showed that human mTECs express a highly diverse selection of tissue-specific genes colocalized within chromosomal clusters: encompassing autoantigens and placenta-associated antigens including CGA, PRG2, SDC1, SEMA3B, CHS2, and CLDN4 (89). Recently, HLA-G was postulated to be regulated by Aire, consistent with earlier findings that identified a subset of TECs sharing expression of this placenta-specific gene with placental trophoblast cells (90, 91). Therefore, it is possible that these antigens, when expressed by mTEC, help shape the mature T cell repertoire, including that of tTRegs that are recruited to the maternal-fetal interface and uterus-draining LN to monitor and provide tolerance to the developing fetus.
Sources of TReg Stimulation in Pregnancy
Most studies in mice agree that both syngeneic and allogeneic pregnancies, in which the dams and sire are of identical or dissimilar genotypes, respectively, involve early and local expansion of TReg cells, with an earlier and higher degree of expansion occurring in allogeneic pregnancy (6, 22, 92, 93). These responses may be mediated by multiple stimuli, including endocrine changes and/or “foreign” antigens that are inherited from the father. A recent study suggested that extravillous trophoblast cells can induce Foxp3 expression in naïve CD4+ T cells via a contact-independent mechanism, suggesting non-antigenic stimulation and further, that these cells may not be specific for fetal antigen (94). Fetal trophoblast cells that lack MHC do not have the ability to present antigen to T cells directly; further, since all trophoblast cells lack MHC class II, it would seem that they would be incapable of directly stimulating CD4+ TReg cells. In agreement with this, mice lacking MHC revealed that presentation of fetal antigens in vivo is predominantly or entirely accomplished through cross presentation by maternal antigen presenting cells (APCs) (42, 95).
Paternally-inherited antigens provided by the fetus to which the mother may react can include major and minor histocompatibility antigens (89). This has been confirmed in human pregnancy: minor antigen-specific T cells, as well as antibodies to male antigens, can be identified in multiparous women (96, 97). Further, maternal reactivity to paternally-inherited antigens appears to be of clinical significance: in hematopoietic cell transplant cases in humans, male recipients of cells from parous females have increased risk of graft vs. host disease, possibly due to co-transferred anti-male antigen specific T cells resulting from prior pregnancy (97).
Evidence for antigen specificity of pTReg cells was provided by Rowe et al. which showed that naïve CD4+ cells with paternal-antigen specificity became Foxp3+ after transfer into TReg-deficient hosts (19). Schumacher et al. used the abortion prone CBA/J x DBA/2J model to show that minor histocompatibility antigens are likely key to induce maternal CD4+CD25+ TReg cells that promote pregnancy success: whereas DBA/2J and Balb/c sires possess the same major histocompatibility haplotype, they likely differ in a number of minor histocompatibility antigens—yet pregnancy is compromised with only the DBA/2J sires and can be rescued by pre-treatment of the dams with TRegs obtained from CBA/J x Balb/c matings (98).
While pTReg cells play a role in maternal tolerance to the fetus (20, 21), a possible role of central tolerance in pregnancy is less well understood and explored. Fetal/placental antigens may access the thymus via the vasculature; using a surrogate antigen, we showed evidence that this may occur. Fetal antigens may be able to access the thymus, thereby inducing TReg at that location (43), in the same way that the central nervous system protein myelin basic protein induces negative selection of CD4+ T cells despite its lack of synthesis in the thymus (99). Alternatively, tissue restricted antigens that are expressed in the thymus, including those regulated by Aire, may induce tTReg development. Indeed, Aire-deficient mice have impaired fertility, and while this is associated with autoimmune-mediated oophoritis, we recently showed that peri-embryonic loss can occur as well (39). Further studies will determine whether Aire-mediated central tolerance via tTReg generation is necessary for maternal tolerance to the fetus.
Conclusion
The fetus is a semi-allograft wherein half of the genome is self and the other half, non-self. Therefore, we must consider the possibility that the suppressive maternal immune component that ensures pregnancy success stems from both tTReg cells, which may tolerize toward paternally-inherited antigens, fetus/placenta-specific antigens, or both, and pTReg cells, which may tolerize toward paternally-inherited antigen (Figure 1). Exposure of antigen to maternal T cells likely occurs via release of placental and fetal cells, vesicles, and free protein into the maternal circulation and lymphatics. Priming of naïve maternal conventional T cells and induction/differentiation of TReg cells could occur locally within the decidua, draining LN, and in thymus; alternatively, expression of placenta- and fetus-specific antigens within the thymus could also mediate de novo generation of tTReg cells.
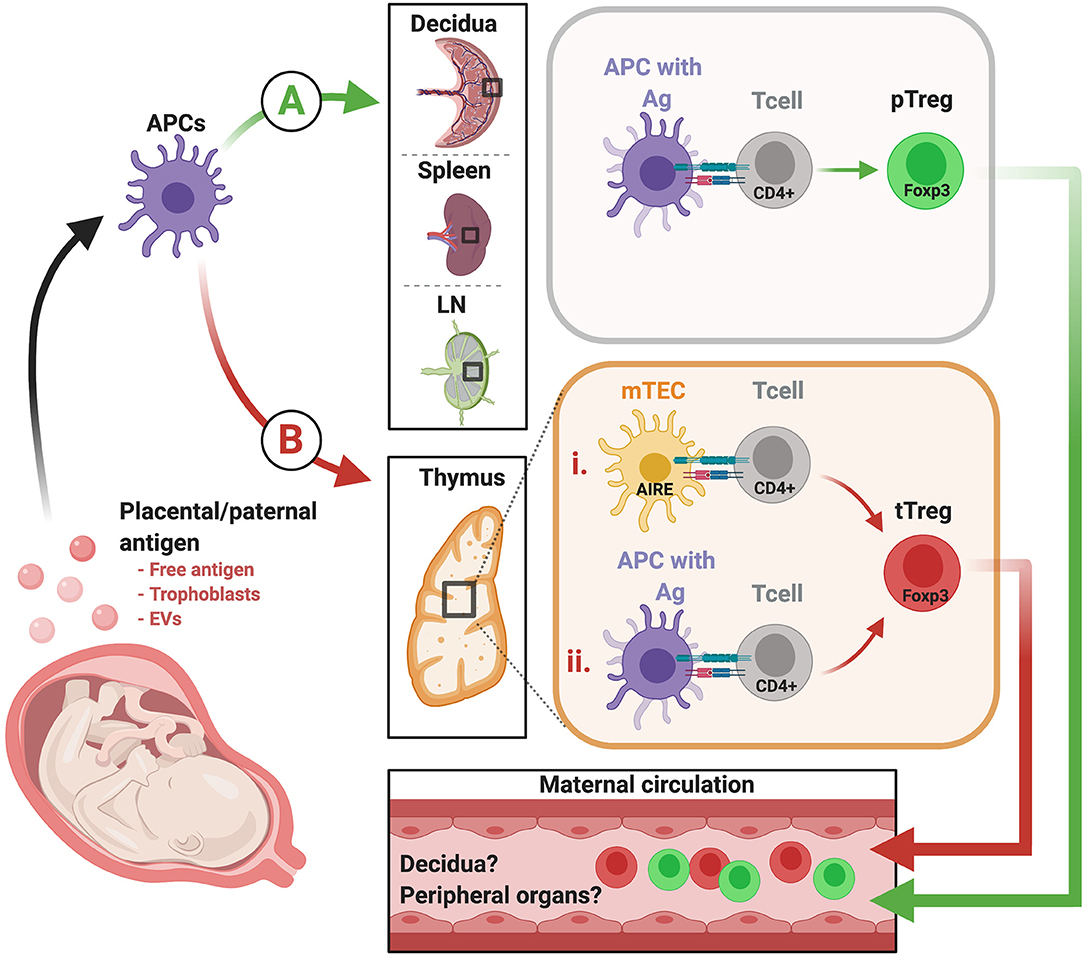
Figure 1. Proposed model of how fetus-specific and paternally-inherited antigens can contribute to pTReg and tTReg development. Fetal and placental antigens that elicit maternal T cell reactivity include those that are paternally-inherited and thus foreign to the maternal immune system, and may also include antigens restricted to fetal and placental tissues. These antigens may arise from chorionic villi, extravillous trophoblast cells, or both, and can be released in the form of whole cells (microchimerism), EVs, and/or as free soluble antigen. From there, placental antigens may prompt TReg development in either or both of two possible pathways. (A) Placental antigens may be presented by maternal APCs in the decidua, and/or drain into maternal blood and lymphatic vessels to access the spleen and uterus-draining LN. At these sites, they can be processed and indirectly presented in the context of maternal MHC to elicit fetal antigen-specific pTReg. (B) It is also possible that tTReg are elicited in the thymus via two potential mechanisms. (i) Placenta/fetus-specific antigens may be expressed and presented directly by mTECs under the control of Aire. (ii) Alternatively, these antigens, and/or placentally-derived antigens accessing the thymus via the vasculature, may be indirectly presented via resident APC in the thymus. EVs, extracellular vesicles; APC, antigen presenting cells; LN, lymph nodes; MHC, major histocompatibility complex; pTReg, peripherally-induced TReg; tTReg, thymus-derived TReg. Created with BioRender.
It is clear in both mice and women that TReg cells are required and critically necessary for successful, uncomplicated pregnancy outcome. However, future studies are required to dissect the heterogeneity and antigen specificity of these cells, and the contribution of central tolerance to pregnancy. Further requirements will include identification of clear markers that distinguish between tTReg and pTReg by using novel thymus-specific identifiers such as CD73. These tools can then be used to our advantage to finally understand the makeup of TReg population in pregnancy and their overall contribution to the success of pregnancy outcome in both mice and women.
Author Contributions
SA and MP conceptualized and wrote the article. SN provided editorial assistance and conceptualized the figure. All authors contributed to the article and approved the submitted version.
Funding
This work was supported by NIH grants R21AI142173, R21HD083058, and R21HD091429. Additional support was provided by Michigan State University and AgBioResearch. The authors are grateful to lab members for the helpful discussions that contributed to this manuscript.
Conflict of Interest
The authors declare that the research was conducted in the absence of any commercial or financial relationships that could be construed as a potential conflict of interest.
References
1. Vignali DAA, Collison LW, Workman CJ. How regulatory T cells work. Nat Rev Immunol. (2008) 8:523–32. doi: 10.1038/nri2343
2. Josefowicz SZ, Lu L-F, Rudensky AY. Regulatory T cells: mechanisms of differentiation and function. Annu Rev Immunol. (2012) 30:531–64. doi: 10.1146/annurev.immunol.25.022106.141623
3. Fontenot JD, Rasmussen JP, Williams LM, Dooley JL, Farr AG, Rudensky AY. Regulatory T cell lineage specification by the forkhead transcription factor foxp3. Immunity. (2005) 22:329–41. doi: 10.1016/j.immuni.2005.01.016
4. Wildin RS, Ramsdell F, Peake J, Faravelli F, Casanova J-L, Buist N, et al. X-linked neonatal diabetes mellitus, enteropathy and endocrinopathy syndrome is the human equivalent of mouse scurfy. Nat Genet. (2001) 27:18–20. doi: 10.1038/83707
5. Bennett CL, Christie J, Ramsdell F, Brunkow ME, Ferguson PJ, Whitesell L, et al. The immune dysregulation, polyendocrinopathy, enteropathy, x-linked syndrome (IPEX) is caused by mutations of fOXP3. Nat Genet. (2001) 27:20–1. doi: 10.1038/83713
6. Aluvihare VR, Kallikourdis M, Betz AG. Regulatory T cells mediate maternal tolerance to the fetus. Nat Immunol. (2004) 5:266–71. doi: 10.1038/ni1037
7. Care AS, Bourque SL, Morton JS, Hjartarson EP, Robertson SA, Davidge ST. Reduction in regulatory T cells in early pregnancy causes uterine artery dysfunction in mice. Hypertension. (2018) 72:177–87. doi: 10.1161/HYPERTENSIONAHA.118.10858
8. Shima T, Sasaki Y, Itoh M, Nakashima A, Ishii N, Sugamura K, et al. Regulatory T cells are necessary for implantation and maintenance of early pregnancy but not late pregnancy in allogeneic mice. J Reprod Immunol. (2010) 85:121–9. doi: 10.1016/j.jri.2010.02.006
9. Guerin LR, Moldenhauer LM, Prins JR, Bromfield JJ, Hayball JD, Robertson SA. Seminal fluid regulates accumulation of fOXP3+ regulatory T cells in the preimplantation mouse uterus through expanding the fOXP3+ cell pool and cCL19-Mediated recruitment. Biol Reprod. (2011) 85:397–408. doi: 10.1095/biolreprod.110.088591
10. Robertson SA, Guerin LR, Bromfield JJ, Branson KM, Ahlström AC, Care AS. Seminal fluid drives expansion of the cD4+CD25+ t Regulatory cell pool and induces tolerance to paternal alloantigens in mice1. Biol Reprod. (2009) 80:1036–45. doi: 10.1095/biolreprod.108.074658
11. Zenclussen AC, Gerlof K, Zenclussen ML, Sollwedel A, Bertoja AZ, Ritter T, et al. Abnormal t-Cell reactivity against paternal antigens in spontaneous abortion. Am J Pathol. (2005) 166:811–22. doi: 10.1016/S0002-9440(10)62302-4
12. Rowe JH, Ertelt JM, Aguilera MN, Farrar MA, Way SS. Foxp3 + regulatory T cell expansion required for sustaining pregnancy compromises host defense against prenatal bacterial pathogens. Cell Host Microbe. (2011) 10:54–64. doi: 10.1016/j.chom.2011.06.005
13. Sasaki Y, Sakai M, Miyazaki S, Higuma S, Shiozaki A, Saito S. Decidual and peripheral blood cD4 +CD25 + regulatory T cells in early pregnancy subjects and spontaneous abortion cases. Mol Hum Reprod. (2004) 10:347–53. doi: 10.1093/molehr/gah044
14. Santner-Nanan B, Peek MJ, Khanam R, Richarts L, Zhu E, Fazekas de St, Groth B, et al. Systemic increase in the ratio between foxp3 + and iL-17-Producing cD4 + T cells in healthy pregnancy but not in preeclampsia. J Immunol. (2009) 183:7023–30. doi: 10.4049/jimmunol.0901154
15. Somerset DA, Zheng Y, Kilby MD, Sansom DM, Drayson MT. Normal human pregnancy is associated with an elevation in the immune suppressive cD25 1 cD4 1 regulatory t-cell subset. Immunology. (2004) 112:38–43. doi: 10.1111/j.1365-2567.2004.01869.x
16. Lathrop SK, Santacruz NA, Pham D, Luo J, Hsieh CS. Antigen-specific peripheral shaping of the natural regulatory T cell population. J Exp Med. (2008) 13:3105–17. doi: 10.1084/jem.20081359
17. Tone Y, Furuuchi K, Kojima Y, Tykocinski ML, Greene MI, Tone M. Smad3 and nFAT cooperate to induce foxp3 expression through its enhancer. Nat Immunol. (2008) 9:194–202. doi: 10.1038/ni1549
18. Zheng Y, Josefowicz S, Chaudhry A, Peng XP, Forbush K, Rudensky AY. Role of conserved non-coding dNA elements in the foxp3 gene in regulatory t-cell fate. Nature. (2010) 463:808–12. doi: 10.1038/nature08750
19. Chen WJ, Jin W, Hardegen N, Lei KJ, Li L, Marinos N, et al. Conversion of peripheral cD4 + cD25 - naive T cells to cD4 + cD25 + regulatory T cells by tGF-β induction of transcription factor foxp3. J Exp Med. (2003) 198:1875–86. doi: 10.1084/jem.20030152
20. Samstein RM, Josefowicz SZ, Arvey A, Treuting PM, Rudensky AY. Extrathymic generation of regulatory T cells in placental mammals mitigates maternal-fetal conflict. Cell. (2012) 150:29–38. doi: 10.1016/j.cell.2012.05.031
21. Rowe JH, Ertelt JM, Xin L, Way SS. Pregnancy imprints regulatory memory that sustains anergy to fetal antigen. Nature. (2012) 490:102–6. doi: 10.1038/nature11462
22. Xin L, Ertelt JM, Rowe JH, Jiang TT, Kinder JM, Chaturvedi V, et al. Cutting edge: committed th1 cD4 + T cell differentiation blocks pregnancy-Induced foxp3 expression with antigen-Specific fetal loss. J Immunol. (2014) 192:2970–4. doi: 10.4049/jimmunol.1302678
23. Cabarrocas J, Cassan C, Magnusson F, Piaggio E, Mars L, Derbinski J, et al. Foxp3+ cD25+ regulatory T cells specific for a neo-self-antigen develop at the double-positive thymic stage. Proc Natl Acad Sci USA. (2006) 103:8453–8. doi: 10.1073/pnas.0603086103
24. Aschenbrenner K, D'Cruz LM, Vollmann EH, Hinterberger M, Emmerich J, Swee LK, et al. Selection of foxp3+ regulatory T cells specific for self antigen expressed and presented by aire+ medullary thymic epithelial cells. Nat Immunol. (2007) 8:351–58. doi: 10.1038/ni1444
25. Burchill MA, Yang J, Vang KB, Moon JJ, Chu HH, Lio CWJ, et al. Linked T cell receptor and cytokine signaling govern the development of the regulatory T cell repertoire. Immunity. (2008) 28:112–21. doi: 10.1016/j.immuni.2007.11.022
26. Lio CWJ, Hsieh CS. A two-Step process for thymic regulatory T cell development. Immunity. (2008) 28:100–11. doi: 10.1016/j.immuni.2007.11.021
27. Klein L, Robey EA, Hsieh CS. Central cD4 + T cell tolerance: deletion versus regulatory T cell differentiation. Nat Rev Immunol. (2019) 19:7–18. doi: 10.1038/s41577-018-0083-6
28. Weist BM, Kurd N, Boussier J, Chan SW, Robey EA. Thymic regulatory T cell niche size is dictated by limiting iL-2 from antigen-bearing dendritic cells and feedback competition. Nat Immunol. (2015) 16:631–41. doi: 10.1038/ni.3171
29. Floess S, Freyer J, Siewert C, Baron U, Olek S, Polansky J, et al. Epigenetic control of the foxp3 locus in regulatory T cells. PLoS Biol. (2007) 5:e38. doi: 10.1371/journal.pbio.0050038
30. Kim HP, Leonard WJ. CREB/ATF-dependent T cell receptor-induced foxP3 gene expression: a role for dNA methylation. J Exp Med. (2007) 204:1543–1551. doi: 10.1084/jem.20070109
31. Toker A, Engelbert D, Garg G, Polansky JK, Floess S, Miyao T, et al. Active demethylation of the foxp3 locus leads to the generation of stable regulatory T cells within the thymus. J Immunol. (2013) 190:3180–8. doi: 10.4049/jimmunol.1203473
32. Dhamne C, Chung Y, Alousi AM, Cooper LJN, Tran DQ. Peripheral and thymic foxp3+ regulatory T cells in search of origin, distinction, and function. Front Immunol. (2013) 4:253. doi: 10.3389/fimmu.2013.00253
33. Linscheid C, Petroff MG. Minor histocompatibility antigens and the maternal immune response to the fetus during pregnancy. Am J Reprod Immunol. (2013) 69:304–314. doi: 10.1111/aji.12075
34. Petroff MG. Review: fetal antigens - identity, origins, and influences on the maternal immune system. Placenta. (2011) Suppl 2:S176–81. doi: 10.1016/j.placenta.2010.12.014
35. Anderson MS, Venanzi ES, Klein L, Chen Z, Berzins SP, Turley SJ, et al. Projection of an immunological self shadow within the thymus by the aire protein. Science. (2002) 298:1395–401. doi: 10.1126/science.1075958
36. Liston A, Lesage S, Wilson J, Peltonen L, Goodnow CC. Aire regulates negative selection of organ-specific T cells. Nat Immunol. (2003) 4:350–4. doi: 10.1038/ni906
37. Malchow S, Leventhal DS, Lee V, Nishi S, Socci ND, Savage PA. Aire enforces immune tolerance by directing autoreactive T cells into the regulatory T cell lineage. Immunity. (2016) 44:1102–13. doi: 10.1016/j.immuni.2016.02.009
38. Jasti S, Warren BD, McGinnis LK, Kinsey WH, Petroff BK, Petroff MG. The autoimmune regulator prevents premature reproductive senescence in female mice. Biol Reprod. (2012) 86:110. doi: 10.1095/biolreprod.111.097501
39. Warren BD, Ahn SH, McGinnis LK, Grzesiak G, Su RW, Fazleabas AT, et al. Autoimmune regulator is required in female mice for optimal embryonic development and implantation†. Biol Reprod. (2019) 100:1492–504. doi: 10.1093/biolre/ioz023
40. Sansom SN, Shikama-Dorn N, Zhanybekova S, Nusspaumer G, Macaulay IC, Deadman ME, et al. Population and single-cell genomics reveal the aire dependency, relief from polycomb silencing, and distribution of self-antigen expression in thymic epithelia. Genome Res. (2014) 24:1918–31. doi: 10.1101/gr.171645.113
41. Derbinski J, Schulte A, Kyewski B, Klein L. Promiscuous gene expression in medullary thymic epithelial cells mirrors the peripheral self. Nat Immunol. (2001) 2:1032–9. doi: 10.1038/ni723
42. Erlebacher A, Vencato D, Price KA, Zhang D, Glimcher LH. Constraints in antigen presentation severely restrict T cell recognition of the allogeneic fetus. J Clin Invest. (2007) 117:1399–411. doi: 10.1172/JCI28214
43. Perchellet AL, Jasti S, Petroff MG. Maternal cD4+ and cD8+ T cell tolerance towards a fetal minor histocompatibility antigen in T cell receptor transgenic mice1. Biol Reprod. (2013) 89:1–12. doi: 10.1095/biolreprod.113.110445
44. Thornton AM, Korty PE, Tran DQ, Wohlfert EA, Murray PE, Belkaid Y, et al. Expression of helios, an ikaros transcription factor family member, differentiates thymic-Derived from peripherally induced foxp3<sup>+</sup> t Regulatory cells. J Immunol. (2010) 184:3433–41. doi: 10.4049/jimmunol.0904028
45. Yadav M, Louvet C, Davini D, Gardner JM, Martinez-Llordella M, Bailey-Bucktrout S, et al. Neuropilin-1 distinguishes natural and inducible regulatory T cells among regulatory T cell subsets in vivo. J Exp Med. (2012) 209:1713–22. doi: 10.1084/jem.20120822
46. Weiss JM, Bilate AM, Gobert M, Ding Y, de Lafaille MAC, Parkhurst CN, et al. Neuropilin 1 is expressed on thymus-derived natural regulatory T cells, but not mucosagenerated induced foxp3+ t reg cells. J Exp Med. (2012) 209:1723–42. doi: 10.1084/jem.20120914
47. Singh K, Hjort M, Thorvaldson L, Sandler S. Concomitant analysis of helios and neuropilin-1 as a marker to detect thymic derived regulatory T cells in naïve mice. Sci Rep. (2015) 5:7767. doi: 10.1038/srep07767
48. Szurek E, Cebula A, Wojciech L, Pietrzak M, Rempala G, Kisielow P, et al. Differences in expression level of helios and neuropilin-1 do not distinguish thymus-derived from extrathymically-induced cD4+Foxp3+ regulatory T cells. PLoS ONE. (2015) 10:1–16. doi: 10.1371/journal.pone.0141161
49. Thornton AM, Lu J, Korty PE, Kim YC, Martens C, Sun PD, et al. Helios + and helios – treg subpopulations are phenotypically and functionally distinct and express dissimilar tCR repertoires. Eur J Immunol. (2019) 49:398–412. doi: 10.1002/eji.201847935
50. Gottschalk RA, Corse E, Allison JP. Expression of helios in peripherally induced Foxp3+ regulatory T cells. J Immunol. (2012) 188:976 LP −80. doi: 10.4049/jimmunol.1102964
51. Schliesser U, Chopra M, Beilhack A, Appelt C, Vogel S, Schumann J, et al. Generation of highly effective and stable murine alloreactive treg cells by combined anti-CD4 mAb, tGF-β, and rA treatment. Eur J Immunol. (2013) 43:3291–305. doi: 10.1002/eji.201243292
52. Akimova T, Beier UH, Wang L, Levine MH, Hancock WW. Helios expression is a marker of T cell activation and proliferation. PLoS ONE. (2011) 6:e24226. doi: 10.1371/journal.pone.0024226
53. Adeegbe DO, Nishikawa H. Natural and induced t regulatory cells in cancer. Front Immunol. (2013) 4:190. doi: 10.3389/fimmu.2013.00190
54. Himmel ME, MacDonald KG, Garcia R V, Steiner TS, Levings MK. Helios+ and helios-Cells coexist within the natural fOXP3+ t Regulatory cell subset in humans. J Immunol. (2013) 190:2001–8. doi: 10.4049/jimmunol.1201379
55. Prud'homme GJ, Glinka Y. Neuropilins are multifunctional coreceptors involved in tumor initiation, growth, metastasis and immunity. Oncotarget. (2012) 3:921–39. doi: 10.18632/oncotarget.626
56. Bruder D, Probst-Kepper M, Westendorf AM, Geffers R, Beissert S, Loser K, et al. Frontline: neuropilin-1: a surface marker of regulatory T cells. Eur J Immunol. (2004) 34:623–30. doi: 10.1002/eji.200324799
57. Sarris M, Andersen KG, Randow F, Mayr L, Betz AG. Neuropilin-1 expression on regulatory T cells enhances their interactions with dendritic cells during antigen recognition. Immunity. (2008) 28:402–13. doi: 10.1016/j.immuni.2008.01.012
58. Campos-Mora M, Contreras-Kallens P, Gálvez-Jirón F, Rojas M, Rojas C, Refisch A, et al. CD4+Foxp3+T regulatory cells promote transplantation tolerance by modulating effector cD4+ T cells in a neuropilin-1-dependent manner. Front Immunol. (2019) 10:882. doi: 10.3389/fimmu.2019.00882
59. Moldenhauer LM, Schjenken JE, Hope CM, Green ES, Zhang B, Eldi P, et al. Thymus-Derived regulatory T cells exhibit foxp3 epigenetic modification and phenotype attenuation after mating in mice. J Immunol. (2019) 203:647–57. doi: 10.4049/jimmunol.1900084
60. Corbel C, Lemarchandel V, Thomas-Vaslin V, Pelus AS, Agboton C, Roméo PH. Neuropilin 1 and cD25 co-regulation during early murine thymic differentiation. Dev Comp Immunol. (2007) 31:1082–94. doi: 10.1016/j.dci.2007.01.009
61. Owen DL, Mahmud SA, Sjaastad LE, Williams JB, Spanier JA, Simeonov DR, et al. Thymic regulatory T cells arise via two distinct developmental programs. Nat Immunol. (2019) 20:195–205. doi: 10.1038/s41590-018-0289-6
62. Colgan SP, Eltzschig HK, Eckle T, Thompson LF. Physiological roles for ecto-5′-nucleotidase (CD73). Purinergic Signal. (2006) 2:351–60. doi: 10.1007/s11302-005-5302-5
64. Kallikourdis M, Betz AG. Periodic accumulation of regulatory T cells in the uterus: preparation for the implantation of a semi-allogeneic fetus? PLoS One. (2007) 2:e382. doi: 10.1371/journal.pone.0000382
65. Tilburgs T, Roelen DL, van der Mast BJ, de Groot-Swings GM, Kleijburg C, Scherjon SA, et al. Evidence for a selective migration of fetus-Specific cD4 + cD25 bright regulatory T cells from the peripheral blood to the decidua in human pregnancy. J Immunol. (2008) 180:5737–5745. doi: 10.4049/jimmunol.180.8.5737
66. Clarke AG, Kendall MD. The thymus in pregnancy: the interplay of neural, endocrine and immune influences. Immunol Today. (1994) 15:545–52. doi: 10.1016/0167-5699(94)90212-7
67. Zoller AL, Kersh GJ. Estrogen induces thymic atrophy by eliminating early thymic progenitors and inhibiting proliferation of β-Selected thymocytes. J Immunol. (2006) 176:7371–8. doi: 10.4049/jimmunol.176.12.7371
68. Tibbetts TA, Demayo F, Rich S, Conneely OM, Malley BWO. Progesterone receptors in the thymus are required for thymic involution during pregnancy and for normal fertility. Proc Natl Acad Sci USA. (1999) 96:12021–6. doi: 10.1073/pnas.96.21.12021
69. den Braber I, Mugwagwa T, Vrisekoop N, Westera L, Mögling R, Bregje de Boer A, et al. Maintenance of peripheral naive T cells is sustained by thymus output in mice but not humans. Immunity. (2012) 36:288–97. doi: 10.1016/j.immuni.2012.02.006
70. Zoller AL, Schnell FJ, Kersh GJ. Murine pregnancy leads to reduced proliferation of maternal thymocytes and decreased thymic emigration. Immunology. (2007) 121:207–15. doi: 10.1111/j.1365-2567.2006.02559.x
71. Hammer JA. Die menschen thymus in gesundheit und krankenheit teil II: das organ unter anormalen korerverhaltnissen. Ztschr F Mikr-Anat Forsch. (1926) 16:733.
72. Laan M, Haljasorg U, Kisand K, Salumets A, Peterson P. Pregnancy-induced thymic involution is associated with suppression of chemokines essential for t-lymphoid progenitor homing. Eur J Immunol. (2016) 46:2008–17. doi: 10.1002/eji.201646309
73. Clarke AG. Pregnancy-induced involution of the thymus can be prevented by immunizing with paternal skin grafts: a strain-dependent effect. Clin Exp Immunol. (1979) 35:421–4.
74. Hellberg S, Mehta RB, Forsberg A, Berg G, Brynhildsen J, Winqvist O, et al. Maintained thymic output of conventional and regulatory T cells during human pregnancy. J Allergy Clin Immunol. (2019) 143:771–5.e7. doi: 10.1016/j.jaci.2018.09.023
75. Wagner MI, Mai C, Schmitt E, Mahnke K, Meuer S, Eckstein V, et al. The role of recent thymic emigrant-regulatory t-cell (RTE-Treg) differentiation during pregnancy. Immunol Cell Biol. (2015) 10:858–67. doi: 10.1038/icb.2015.51
76. Teles A, Thuere C, Wafula PO, El-Mousleh T, Zenclussen ML, Zenclussen AC. Origin of foxp3(+) cells during pregnancy. Am J Clin Exp Immunol. (2013) 2:222–33.
77. Heino M, Kudoh J, Nagamine K, Lagerstedt A, Ovod V, Ranki A, et al. Autoimmune regulator is expressed in the cells regulating immune tolerance in thymus medulla. Biochem Biophys Res Commun. (1999) 825:821–5. doi: 10.1006/bbrc.1999.0308
78. Org T, Rebane A, Kisand K, Laan M, Haljasorg U, Andreson R, et al. AIRE activated tissue specific genes have histone modifications associated with inactive chromatin. Hum Mol Genet. (2009) 24:4699–710. doi: 10.1093/hmg/ddp433
79. Org T, Chignola F, Hetényi C, Gaetani M, Rebane A, Liiv I, et al. The autoimmune regulator pHD finger binds to non-methylated histone h3K4 to activate gene expression. EMBO Rep. (2008) 9:370–6. doi: 10.1038/sj.embor.2008.11
80. Hubert FX, Kinkel SA, Davey GM, Phipson B, Mueller SN, Liston A, et al. Aire regulates the transfer of antigen from mTECs to dendritic cells for induction of thymic tolerance. Blood. (2011) 118:2462–72. doi: 10.1182/blood-2010-06-286393
81. Kishimoto H, Sprent J. Negative selection in the thymus includes semimature T cells. J Exp Med. (1997) 185:263–72. doi: 10.1084/jem.185.2.263
82. Mathis D, Benoist C. Aire. Annu Rev Immunol. (2009) 27:287–312. doi: 10.1146/annurev.immunol.25.022106.141532
83. Ahonen P, Myllärniemi S, Sipilä I, Perheentupa J. Clinical variation of autoimmune polyendocrinopathy-Candidiasis-Ectodermal dystrophy (APECED) in a series of 68 patients. N Engl J Med. (1990) 332:1829–36. doi: 10.1056/NEJM199006283222601
84. Husebye ES, Anderson MS, Kampe O. Autoimmune polyendocrine syndromes. N Engl J Med. (2018) 378:1132–41. doi: 10.1056/NEJMra1713301
85. Jiang W, Anderson MS, Bronson R, Mathis D, Benoist C. Modifier loci condition autoimmunity provoked by aire deficiency. J Exp Med. (2005) 202:805–15. doi: 10.1084/jem.20050693
86. Anderson MS, Venanzi ES, Chen Z, Berzins SP, Benoist C, Mathis D. The cellular mechanism of aire control of T cell tolerance. Immunity. (2005) 23:227–39. doi: 10.1016/j.immuni.2005.07.005
87. Yang S, Fujikado N, Kolodin D, Benoist C, Mathis D. Regulatory T cells generated early in life play a distinct role in maintaining self-tolerance. Science. (2015) 348:589–94. doi: 10.1126/science.aaa7017
88. St-Pierre C, Trofimov A, Brochu S, Lemieux S, Perreault C. Differential features of aIRE-Induced and aIRE-Independent promiscuous gene expression in thymic epithelial cells. J Immunol. (2015) 195:498–506. doi: 10.4049/jimmunol.1500558
89. Gotter J, Brors B, Hergenhahn M, Kyewski B. Medullary epithelial cells of the human thymus express a highly diverse selection of tissue-specific genes colocalized in chromosomal clusters. J Exp Med. (2004) 199:155–66. doi: 10.1084/jem.20031677
90. Crisa L, McMaster MT, Ishii JK, Fisher SJ, Salomon DR. Identification of a thymic epithelial cell subset sharing expression of the class ib hLA-G molecule with fetal trophoblasts. J Exp Med. (1997) 186:289–98. doi: 10.1084/jem.186.2.289
91. Melo-Lima BL, Poras I, Passos GA, Carosella ED, Donadi EA, Moreau P. The autoimmune regulator (Aire) transactivates hLA-G gene expression in thymic epithelial cells. Immunology. (2019) 158:121–35. doi: 10.1111/imm.13099
92. Chen T, Darrasse-Jèze G, Bergot A-S, Courau T, Churlaud G, Valdivia K, et al. Self-Specific memory regulatory T cells protect embryos at implantation in mice. J Immunol. (2013) 191:2273–81. doi: 10.4049/jimmunol.1202413
93. Kahn DA, Baltimore D. Pregnancy induces a fetal antigen-specific maternal t regulatory cell response that contributes to tolerance. Proc Natl Acad Sci USA. (2010) 107:9299–304. doi: 10.1073/pnas.1003909107
94. Salvany-Celades M, van der Zwan A, Benner M, Setrajcic-Dragos V, Bougleux Gomes HA, Iyer V, et al. Three types of functional regulatory T cells control T cell responses at the human maternal-Fetal interface. Cell Rep. (2019) 27:2537–47.e5. doi: 10.1016/j.celrep.2019.04.109
95. Moldenhauer LM, Diener KR, Thring DM, Brown MP, Hayball JD, Robertson SA. Cross-Presentation of male seminal fluid antigens elicits T cell activation to initiate the female immune response to pregnancy. J Immunol. (2009) 182:8080–93. doi: 10.4049/jimmunol.0804018
96. Christiansen OB, Pedersen B, Nielsen HS, Andersen AMN. Impact of the sex of first child on the prognosis in secondary recurrent miscarriage. Hum Reprod. (2004) 19:2946–51. doi: 10.1093/humrep/deh516
97. Miklos DB, Kim HT, Miller KH, Guo L, Zorn E, Lee SJ, et al. Antibody responses to h-Y minor histocompatibility antigens correlate with chronic graft-versus-host disease and disease remission. Blood. (2005) 105:2973–2978. doi: 10.1182/blood-2004-09-3660
98. Schumacher A, Wafula PO, Bertoja AZ, Sollwedel A, Thuere C, Wollenberg I, et al. Mechanisms of action of regulatory T cells specific for paternal antigens during pregnancy. Obstet Gynecol. (2007) 110:1137–1145. doi: 10.1097/01.AOG.0000284625.10175.31
Keywords: central tolerance, peripheral tolerance, Aire, thymic TRegs peripheral TRegs, fetal antigens, paternal antigens, pregnancy
Citation: Ahn SH, Nguyen SL and Petroff MG (2020) Exploring the Origin and Antigenic Specificity of Maternal Regulatory T Cells in Pregnancy. Front. Immunol. 11:1302. doi: 10.3389/fimmu.2020.01302
Received: 20 March 2020; Accepted: 22 May 2020;
Published: 25 June 2020.
Edited by:
Ana Claudia Zenclussen, University Hospital Magdeburg, GermanyReviewed by:
Tippi C. MacKenzie, University of California, San Francisco, United StatesSing Sing Way, Cincinnati Children's Hospital Medical Center, United States
Copyright © 2020 Ahn, Nguyen and Petroff. This is an open-access article distributed under the terms of the Creative Commons Attribution License (CC BY). The use, distribution or reproduction in other forums is permitted, provided the original author(s) and the copyright owner(s) are credited and that the original publication in this journal is cited, in accordance with accepted academic practice. No use, distribution or reproduction is permitted which does not comply with these terms.
*Correspondence: Margaret G. Petroff, cGV0cm9mMTBAbXN1LmVkdQ==