- 1Centre for the AIDS Programme of Research in South Africa, University of KwaZulu-Natal, Durban, South Africa
- 2Africa Health Research Institute, University of KwaZulu-Natal, Durban, South Africa
- 3HIV Pathogenesis Programme, The Doris Duke Medical Research Institute, Nelson R. Mandela School of Medicine, University of KwaZulu-Natal, Durban, South Africa
- 4Department of Medical Microbiology, University of KwaZulu-Natal, Durban, South Africa
- 5Institute of Infectious Diseases and Molecular Medicine (IDM), University of Cape Town, and National Health Laboratory Service, Cape Town, South Africa
- 6Department of Epidemiology, Mailman School of Public Health, Columbia University, New York, NY, United States
- 7Department of Microbiology and Immunology, The Peter Doherty Institute for Infection and Immunity, University of Melbourne, Melbourne, VIC, Australia
- 8Department of Public Health, University of KwaZulu-Natal, Durban, South Africa
The RV144 HIV-vaccine trial highlighted the importance of envelope-specific non-neutralizing antibody (nNAb) Fc-mediated functions as immune correlates of reduced risk of infection. Since pre-exposure prophylaxis (PrEP) and HIV-vaccines are being used as a combination prevention strategy in at risk populations, the effects of PrEP on nNAb functions both mucosally and systemically remain undefined. Previous animal and human studies demonstrated reduced HIV-specific antibody binding avidity post-HIV seroconversion with PrEP, which in turn may affect antibody functionality. In seroconverters from the CAPRISA 004 tenofovir gel trial, we previously reported significantly higher detection and titres of HIV-specific binding antibodies in the plasma and genital tract (GT) that distinguished the tenofovir from the placebo arm. We hypothesized that higher HIV-specific antibody titres and detection reflected corresponding increased antibody-dependent neutrophil-mediated phagocytosis (ADNP) and NK-cell-activated antibody-dependent cellular cytotoxic (ADCC) activities. HIV-specific V1V2-gp70, gp120, gp41, p66, and p24 antibodies in GT and plasma samples of 48 seroconverters from the CAPRISA 004 tenofovir gel trial were tested for ADCP and ADCC at 3, 6- and 12-months post-HIV-infection. GT gp41- and p24-specific ADNP were significantly higher in the tenofovir than the placebo arm at 6 and 12 months respectively (p < 0.05). Plasma gp120-, gp41-, and p66-specific ADNP, and GT gp41-specific ADCC increased significantly over time (p < 0.05) in the tenofovir arm. In the tenofovir arm only, significant inverse correlations were observed between gp120-specific ADCC and gp120-antibody titres (r = −0.54; p = 0.009), and gp41-specific ADNP and gp41-specific antibody titres at 6 months post-infection (r = −0.50; p = 0.015). In addition, in the tenofovir arm, gp41-specific ADCC showed significant direct correlations between the compartments (r = 0.53; p = 0.045). Certain HIV-specific nNAb activities not only dominate specific immunological compartments but can also exhibit diverse functions within the same compartment. Our previous findings of increased HIV specific antibody detection and titres in women who used tenofovir gel, and the limited differences in nNAb activities between the arms, suggest that prior PrEP did not modulate these nNAb functions post-HIV seroconversion. Together these data provide insight into envelope-specific-nNAb Fc-mediated functions at the site of exposure which may inform on ensuing immunity during combination HIV prevention strategies including PrEP and HIV vaccines.
Introduction
HIV continues to be a major global health challenge. In particular, young women in Africa (aged 15–24 years) remain vulnerable to HIV infection (1). Despite the advent and testing of several biomedical prevention strategies such as intra-vaginal rings containing antiretroviral drugs (2), topical tenofovir gel as pre-exposure prophylaxis (PrEP) (3), or oral PrEP (4, 5) levels of protection among women have been inconsistent, ranging from −49 to 76% (3–5). These data therefore underscore the urgent need for an efficacious HIV vaccine. In the current era of prevention, where future combination HIV prevention strategies will likely include both PrEP and HIV vaccines especially in at-risk populations, elucidating the effects of prior use of PrEP on immunity are important.
A non-human primate (NHP) study of oral tenofovir reported that animals with breakthrough SHIV infection had reduced CD4+ T cell loss and lowered immune activation compared to control animals (6). Women who experienced breakthrough HIV infections and were assigned to the tenofovir gel arm in the CAPRISA 004 trial had delayed antibody binding avidity (7) and preserved Gag-specific CD4+ T cells (8), which may likely have aided B cells to produce p24-specific or other antibodies. P24-specific antibodies have been associated with improved CD4+ T cell counts and lower viral loads (9) and has also been associated with HIV control (10). In addition, p66 antibodies have been associated with a decreased rate of disease progression (11, 12) and Fc-mediated antiviral activities (13). In seroconverters from the CAPRISA tenofovir gel trial, we previously reported significantly higher detection and titres of gp120-IgG, p66-IgG, and gp41-IgA in the plasma and genital tracts of women in the tenofovir arm, compared to those in the placebo arm (14). Whether these antibodies were capable of binding to infected cells and/or exerting Fc-mediated antiviral activities remains unknown [reviewed by Overbaugh and Morris (15)].
The ultimate goal of a vaccine is to induce robust antibody responses capable of neutralizing a variety of HIV strains to prevent HIV infection. To date, the RV144 HIV-vaccine is the only trial to show a 31.2% protective efficacy (16). The analyses of the correlates of reduced risk of infection were attributed to the presence of HIV-specific non-neutralizing binding antibodies (nNAb) which elicited enhanced Fc-mediated NK-cell-activated antibody-dependent cellular cytotoxicity (ADCC) in the presence of limited tier 1 nNAbs (17), but in the absence of IgA (18). Even in preclinical macaque studies, Fc-mediated antiviral activities were shown to protect animals from SHIV infections (19–21). Furthermore, in passive immunity studies in macaques, broadly neutralizing antibodies such as PGT121, were shown to exert viral clearance using Fc-mediated antiviral activities in distal tissues (22). Human cohort studies have also underscored the role of ADCC (9, 13, 23, 24) and antibody-dependent neutrophil-mediated phagocytosis (ADNP) activities in ameliorating HIV disease progression (25). Antibodies capable of effecting ADCC in the breastmilk have been associated with significant reductions in mother-to-child-transmissions of HIV (26) and decreased infant mortality (27). Given that the genital tract is the most common site of sexual HIV transmission, the presence of functional mucosal antibodies may aid in preventing local infection or delaying/halting disease progression through viral control. Additional evidence reporting ADCC activities in the female genital tract and subsequent decreases in genital tract viral loads, emphasizes the importance of Fc-mediated antiviral activities at vulnerable sites of transmission (28, 29).
The effects of PrEP however, on HIV-specific antibody (IgG) Fc-mediated antiviral activities remain unknown. Based on our previous findings, we posited that the higher titres and detection of certain HIV-specific antibodies in women who used the tenofovir gel (14), translated to a corresponding increase in HIV-specific nNAb activities in the genital tract and plasma relative to those in the placebo arm. In this study, we sought to elucidate the effects of prior PrEP on nNAb functional immune responses in the genital tracts and plasma of women who experienced breakthrough HIV infections.
Materials and Methods
Study Population
This sub-study included 48 women who acquired HIV infection while participating in the CAPRISA 004 tenofovir gel trial (NCT00441298) (3). Twenty-four women from the tenofovir arm were case matched to 24 women in the placebo arm according to their viral loads (VL) and CD4+ T-cell counts at six-months post-infection. Therefore, there were no significant differences in either the CD4+ T cell counts or HIV viral loads between the arms. Plasma and cervicovaginal lavages (CVL) were collected as previously described (3). Plasma and CVL samples from 3, 6-, and 12-months post-infection were included in this sub-study. These women remained antiretroviral treatment naïve during this period of observation post-HIV infection. Tenofovir drug levels were previously measured in the CVL aspirates of women from the CAPRISA 004 trial (30), and these women were HIV uninfected at the time of the tenofovir drug measurements. Ethics approvals for the original study (E111/06) and for this sub-study (BE241/16) were obtained from the University of KwaZulu-Natal Biomedical Research Ethics Committee (BREC).
Plasma IgG and CVL IgG Isolation and Quantification
Immunoglobulin (Ig) G from the plasma of women in both arms were positively selected using an IgG isolation kit (Thermofisher Scientific, Waltham, MA), as per the manufacturer's instructions. CVL samples for the mucosal experiments were used without further purifying out the IgGs, due to the limited volume of the CVLs available.
Antibody Dependent Neutrophil Mediated Phagocytosis (ADNP)
Neutrophil mediated phagocytosis assays were performed as previously described (31). Briefly, white blood cells were isolated from fresh whole blood of healthy volunteers with ACK lysis buffer (Life Technologies, Grand Island, NY), as per the manufacturer's instructions and then resuspended to ~50,000 cells/mL in the volume of R10 media required for the assays. Neutrophils were then activated using isolated IgG and CVL from HIV infected women from both the tenofovir and placebo arms. A 100 μl of 0.50 mg/ml purified IgG from the plasma was used for these experiments and for the genital tract experiments 100 μl of CVL. Neutravidin beads were biotinylated to one of five HIV antigens: Con6 gp120/B (Consensus Group M gp120), gp70_B CaseA_V1_V2 (V1V2-gp70), gp41 (Ectodomain HIV-1), p66 RT (Immune Technology, California, USA or ImmunoDX, Massachusetts, USA), and p24 (HIV-1/Clade B/C CN54). The assays were conducted as previously described in (31) and the data were analyzed according to the protocol established by Darrah et al. (32). CD3 A700 was used to stain for CD3 negative cells, CD14 PE-Cy7, CD66b V450 (BD Biosciences, New Jersey, USA) were used to stain for neutrophils and the negative controls, which were the normal human serum (NHS) and phosphate buffered saline (PBS), respectively. Following staining, the samples were acquired on a LSR Fortessa (BD Biosciences, California, USA) as previously described (31). PBS and NHS were used to gate on CD3−, CD66+, CD14− neutrophils that phagocytosed biotinylated antigen that was identified by the FITC+ population (Figure 1). Phagocytic scores were determined using an ADNP-specific gating strategy (Figure 1) and the integrated mean fluorescent intensity (MFI) with the following modification—% of neutrophils taking up beads x MFI/ 10,000 (32).
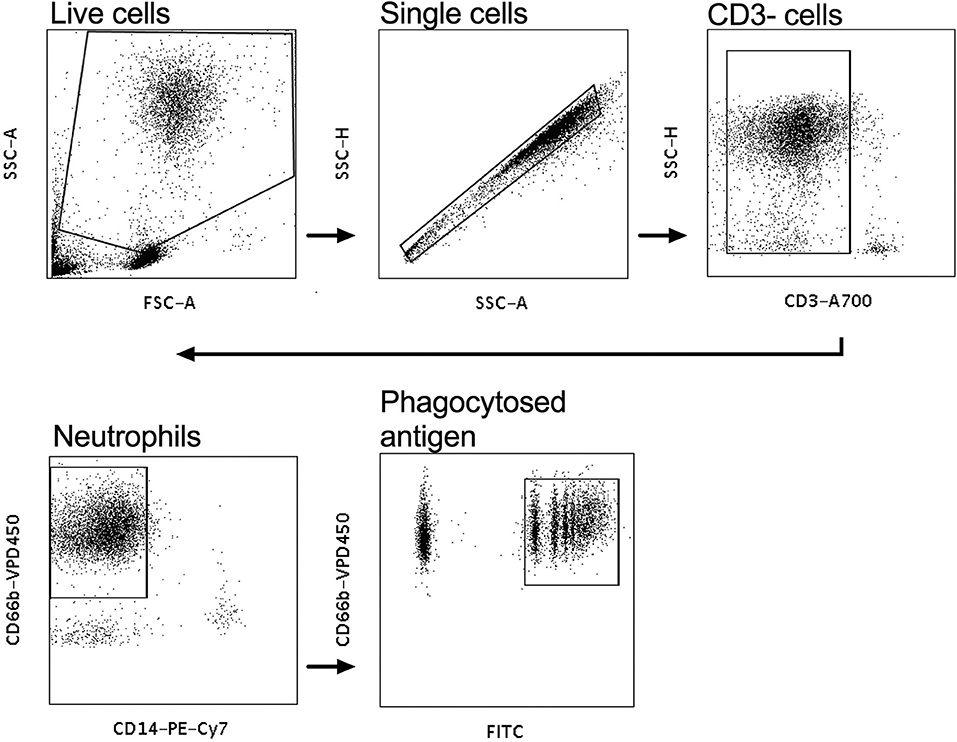
Figure 1. Gating strategy for antibody-dependent neutrophil phagocytic activity (ADNP). White blood cells were gated on, and the CD3− population selected. CD14−, CD66+ populations were gated on to identify neutrophils and the CD66+FITC+ cells were gated on to identify phagocytosed neutravidin bound antigen beads.
NK-Cell Activated Antibody Dependent Cellular Cytotoxicity (ADCC)
Intracellular staining was used to assess the effector functions of activated natural killer (NK) cells isolated by negative selection (RosetteSep kit, Stemcell technologies) from fresh whole blood from healthy donors, as previously described (24), with the following modifications – 50 μl of 0.25 mg/ml IgG purified from plasma (data not shown) or 50 μl CVL were incubated with the one of four HIV antigens Con6 gp120/B (Consensus Group M gp120), gp41 (Ectodomain) (HIV-1), p66 RT, and p24 (HIV-1/Clade B/C CN54) (Immune Technology, California, USA or ImmunoDX, Massachusetts, USA) and 50,000 NK cells/well. Following incubation with monensin and Brefeldin A as well as CD107a, cells were stained with CD3− A700, CD56 PE-Cy-7, CD16 APC-Cy7, IFN-γ APC, and MIP1-β PE (BD Biosciences, California, USA) and were then fixed with BD cell fix and then acquired on a LSR Fortessa (BD Biosciences, California, USA). Enriched NK cells were acquired and selected for singlets, followed by CD3 negative lymphocytes that were CD56+/CD16+ (Figure 2). The release of CD107a, IFN-γ and MIP-1β was used to assess frequency of activated NK cells to determine NK-cell activated ADCC (Figure 2) (9). When calculating HIV-specific antiviral activities, the non-specific ADCC activity obtained as background activity from PBS/NHS was subtracted from HIV-specific antibody-mediated ADCC activities.
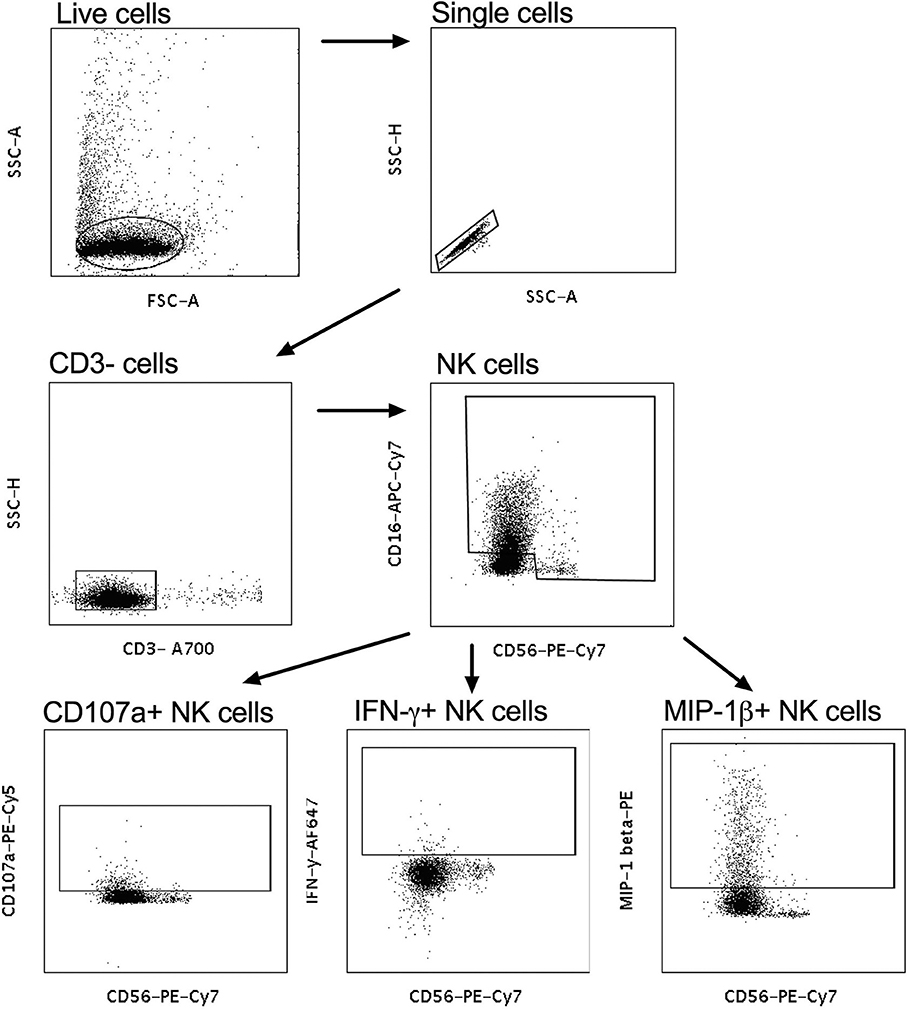
Figure 2. Gating strategy for NK-cell-activated antibody-dependent cellular cytotoxicity (ADCC). CD3− cells were gated on and subsequently CD56+CD16+ NK cells were selected. CD56+CD107a+, CD56+IFN-γ+, and CD56+MIP-1β+ were used to identify cytotoxic markers and antiviral cytokines for activated NK cells.
Statistical Analyses
Wilcoxon signed-rank test was used to compare differences in the plasma IgG and CVL phagoscores at different time points (i.e., 3–6 months, 6–12 months, and 3–12 months). Dunn's post hoc analyses could not be performed as there were limited matched sample availability at certain time points. Wilcoxon-Mann-Whitney test was used to compare two medians between tenofovir and placebo for %CD107a expression as a marker of NK cell ADCC activities, as well as %IFN-γ and %MIP-1β cytokine responses. No adjustments were made for multiple comparisons for either the ADNP or ADCC data. Spearman Rank correlation analyses were performed to determine inter-compartmental relationships, CD4+ T cell counts and VL in relation to Fc-mediated activities. Linear mixed models were used to analyse the change in ADNP and ADCC responses over time for each HIV protein and were adjusted for multiple comparisons using the false discovery rate method. Statistical analyses were performed using GraphPad Prism version 8 and SAS versions 9.4 (SAS Institute Inc., Cary) software.
Results
Demographics of Study Participants From the CAPRISA 004 Tenofovir Gel Trial
As previously reported in Archary et al. (14), the women in both the tenofovir and placebo arms were similar in terms of their age, level of education, numbers of sexual partners, condom use, use of hormonal contraception, and disease progression markers such as CD4+ T cell counts and viral loads at 6 months post-infection (Table 1). The only difference was that women in the tenofovir arm had a significant 5 month delay in time to HIV infection, compared to those in the placebo arm (p < 0.02) (14). In the tenofovir arm, 58.3% of the women had detectable tenofovir drug levels in the CVL aspirates.
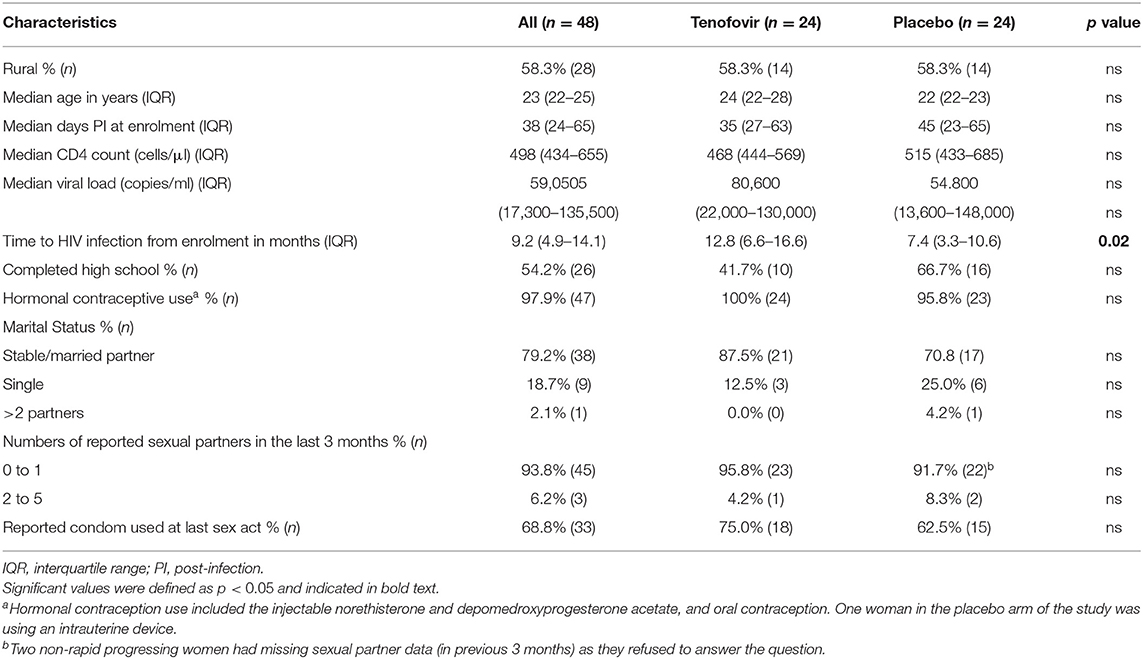
Table 1. Demographics and clinical characteristics of the sub-set of women who acquire HIV infections during the CAPRISA 004 trials (n = 48).
Gp41- and p24-Specific ADNP Increased Significantly in the GT of Women in the Tenofovir Compared to the Placebo Arm
In order to determine if topical tenofovir had an effect on the ADNP responses in the GT, we compared HIV-specific ADNP responses at 3, 6, and 12 months to those in the placebo arm. GT gp41-specific ADNP in the tenofovir arm were significantly higher at 6 months [median and interquartile range (IQR)] (37.92; IQR = 19.40–65.60) compared to those in the placebo arm (19.40; IQR = 9.43–28.93) (p = 0.014) (Figure 3A). In addition, p24-specific ADNP were significantly higher in the tenofovir arm at 12 months (20.15; IQR = 16.61–30.61) compared to the placebo arm (13.41; IQR = 4.16–18.11) (p = 0.007) (Figure 3B). P66-specific ADNP trended higher in the tenofovir arm (63.58; IQR = 33.48–83.09) compared to the placebo arm (24.03; IQR = 17.44–54.16) at 12 months (p = 0.063, Supplementary Table 1). No significant differences were found between the arms, at any of the other time points, for gp120-specific ADNP (Supplementary Table 1).
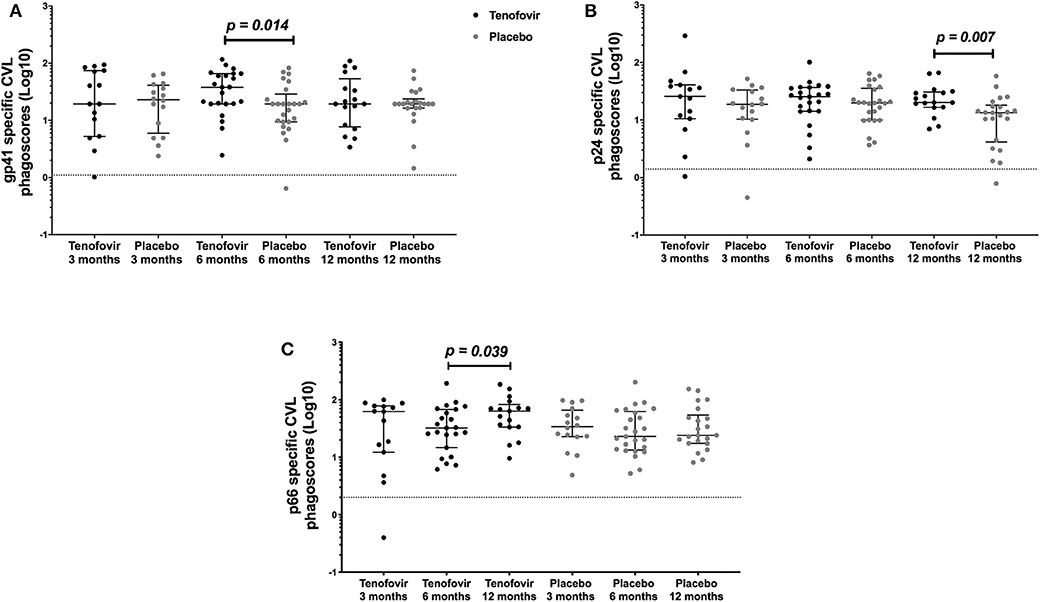
Figure 3. HIV-specific ADNP [phagoscores (Log10)] in the genital tract (CVL) in women from the tenofovir and placebo arms. Cross-sectional analyses between the tenofovir and placebo arms to HIV proteins (A) gp41 and (B) p24 and longitudinal analyses for tenofovir and placebo (C) p66. Solid lines indicate the minimum and maximum values of box and whisker plots, with black solid lines across the dots indicate the median at each time point for each study arm. Wilcoxon signed-rank test was used to analyse ADNP activity over time and Wilcoxon-Mann-Whitney test was used for cross sectional analysis. Statistically significant values were defined as p < 0.05 with no adjustments for multiple comparisons.
In order to determine if there were changes in HIV-specific ADNP over time, we investigated these responses longitudinally in the GT in both arms. In the tenofovir arm, only p66-specific ADNP in the GT increased significantly from 6 to 12 months [IQR of 6 months (32.33; IQR = 14.68–67.99); 12 months (63.58; IQR = 33.48–83.09)] (p = 0.039) (Figure 3C). In contrast, the p66-specific ADNP in the placebo arm remained similar from 3 to 12 months. In the GT, within the arms, gp120-, gp41-, and p24-specific ADNP remained similar across all time points (Supplementary Table 1).
Gp120- and p66-Specific ADNP Activities Increased in the Blood Over Time, Irrespective of Prior Topical Tenofovir Gel Use
Next, we aimed to understand the kinetics of the HIV-specific ADNP in the blood within each of the arms over time. Longitudinal analyses showed that in the tenofovir arm, gp120-specific ADNP consistently and significantly increased from 3 months (27.12; IQR = 18.49–39.85) to 12 months (41.99; IQR = 25.17–65.51) (p = 0.007) (Figure 4A) and from 6 (27.12; IQR = 21.32–49.47) to 12 months (41.99; IQR = 25.17–65.51) (p = 0.029). Similarly, a significant increase in gp120 ADNP was also observed in the placebo arm from 3 months (26.52; IQR = 10.08–29.56) to 12 months (37.52; IQR = 28.00–49.11, p = 0.007, Figure 4A). Gp41-specific ADNP in the tenofovir arm trended to increase from 6 months (51.52; IQR = 37.26–71.69) to 12 months, (64.10; IQR = 41.91–71.59) (p = 0.051, Figure 4B). In the tenofovir arm, p66-ADNP significantly increased from 3 months (42.35; IQR = 27.40–62.37) to 12 months (79.01; IQR = 60.21–107.50) (p = 0.002, Figure 4C). Similarly, in the placebo arm p66-specific ADNP significantly increased from 3 months (47.12; IQR = 32.40–77.85) to 12 months (80.89; IQR = 56.81–101.90) (p = 0.022, Figure 4C) and also from 6 months (42.41; IQR = 24.87–80.24) to 12 months (80.89; IQR = 56.81–101.90) (p = 0.008, Figure 4C). For p24-specific ADNP no significant differences were observed over time within the tenofovir or placebo arms (Figure 4D).
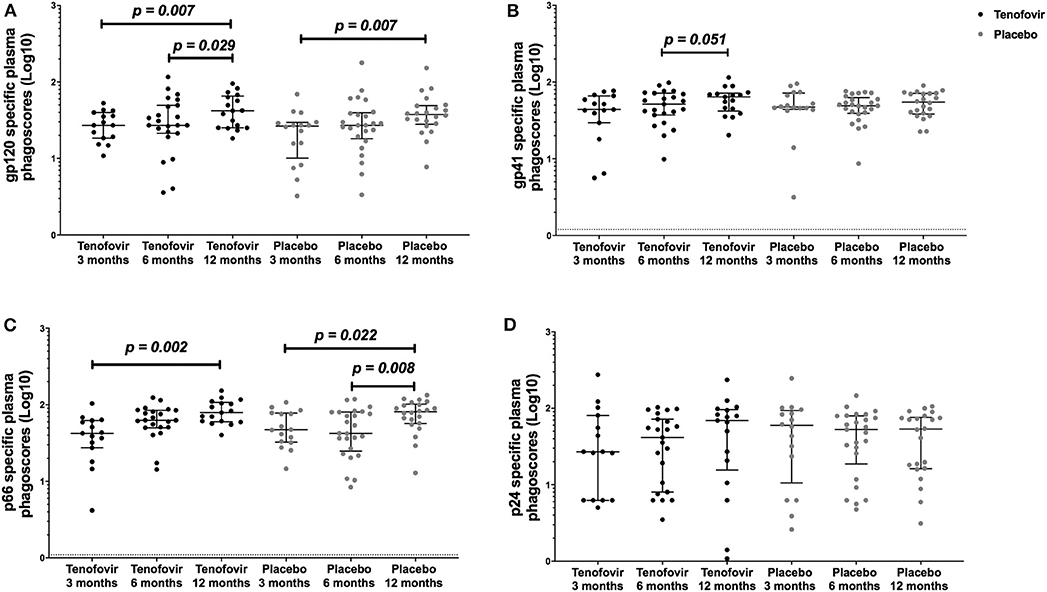
Figure 4. Longitudinal analyses of plasma HIV-specific ADNP [phagoscores (Log10)] in both the tenofovir and the placebo arms. Phagocytic activities [phagoscores (Log10)] to HIV proteins (A) gp120, (B) gp41, (C) p66, and (D) p24. Solid lines indicate the minimum and maximum values of box and whisker plots, with black solid lines across the dots indicate the median at each time point for each study arm. Statistical differences were defined as p < 0.05 and were determined by Wilcoxon signed-rank test. No adjustments were made for multiple comparisons.
P66-Specific ADNP Correlated Inversely Between the GT and Blood in the Placebo Arm
In order to determine if the magnitudes of ADNP activities in the blood mirrored those in the GT, cross-compartmental correlation analyses for both arms were conducted. In the placebo arm, only the p66-specific ADNP correlated inversely between the plasma and GT at 12 months post-infection (r = −0.48; p = 0.024, Supplementary Table 2). No other significant cross-compartment associations were found (Supplementary Table 2).
Gp41-Specific ADCC Were Significantly Higher in the Genital Tract in the Tenofovir Arm
Antibody mediated NK cell activation was measured by the % expression of CD107a positive (+) cells as a surrogate for ADCC. Longitudinal analyses in the tenofovir arm, showed that plasma gp41-specific ADCC significantly decreased at 12 months (1.75; IQR = 0.95–4.28) from the 6 month post-infection time-point (4.85; IQR = 2.40–9.60), (p = 0.025, Figure 5A). However, in the GT and in the tenofovir arm gp41-specific ADCC significantly increased at 12 months (5.20; IQR = 1.76–7.16) from 6 months post-infection (2.88; IQR = 1.17–6.39), (p = 0.032, Figure 5B). Plasma and GT gp120-, p66- or p24-specific ADCC were not different over time within each of the study arms (Supplementary Tables 3, 4).
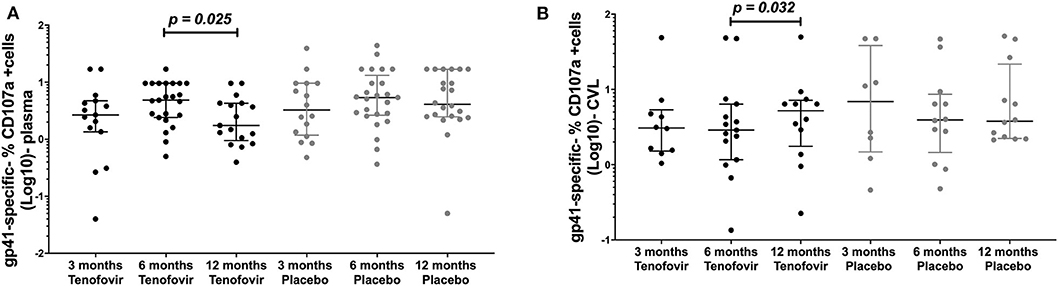
Figure 5. Longitudinal analyses of NK cell activated ADCC (antibody-specific- %CD107a+ cells [Log10]) in the plasma and GT (CVL) of women at 3 months, 6 months and 12 months, for the tenofovir and placebo arms for gp41. Analyses for cytotoxic activities (gp41-specific %CD107a+ cells [Log10]) in the plasma on the left (A) for gp41 and the genital tract on the right to (B) for gp41. Solid lines indicate the minimum and maximum values of box and whisker plots, with black solid lines across the dots indicate the median at each time point for each study arm. Wilcoxon signed-rank tests were done to determine statistical differences between NK cell activated ADCC activities within each arm of the study, for each protein. Statistically significant values were defined as p < 0.05. No adjustments were made for multiple comparisons.
Gp41 and p24-Specific ADCC Responses in the Plasma Were Greater at Particular Time Points, in the Placebo Arm
We then assessed whether there were any differences for ADCC between the arms in the plasma and GT. We report significant differences in the plasma but not in the GT (Supplementary Table 4). Plasma HIV-gp41 ADCC responses in the placebo arm 4.11 (IQR = 2.47–16.90), were significantly higher at 12 months compared to the tenofovir arm 1.75 (IQR = 0.95–4.28), p = 0.017) (Supplementary Table 3). In the placebo arm, plasma p24-specific responses at 3 months were greater 3.80 (IQR = 2.55–7.23), than those in the tenofovir arm 1.75 (IQR = 1.25–4.54, p = 0.027) (Supplementary Table 3). Gp120- and p66-specific ADCC in both the tenofovir and placebo arms were similar for all timepoints (Supplementary Table 3). In the absence of further differences between the arms for any of the remaining HIV-specific ADCC activities in the plasma, we then sought to determine if there was concordance between HIV-specific ADCC in the plasma and GT in both arms at 3, 6, and 12 months.
Gp41-Specific ADCC Responses in the Tenofovir Arm Correlated Between the Plasma and GT
To determine if HIV-specific ADCC in the blood mirrored those in the GT, cross-compartmental correlation analyses for both arms were conducted. At 6 months post-infection, gp41-specific ADCC in the plasma significantly and directly correlated with those in the GT of women in the tenofovir arm (r = 0.53; p = 0.045) (Table 2). No other significant correlations were observed for gp120, p66, or p24.
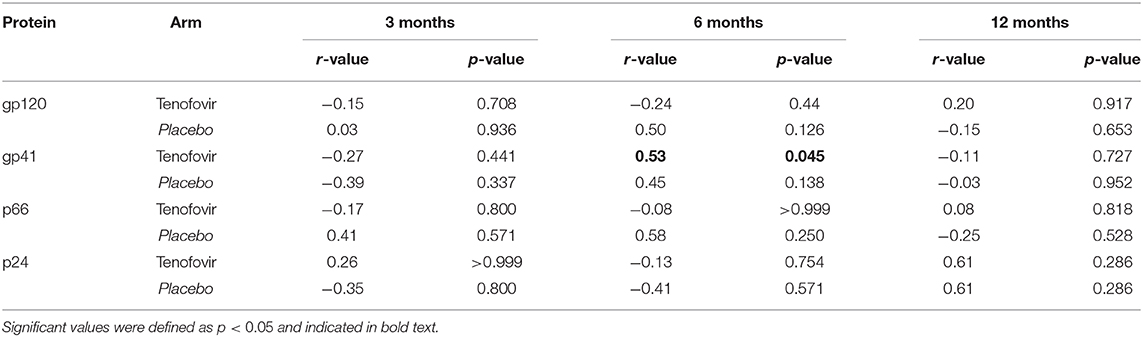
Table 2. Inter-compartmental correlations for the blood and genital tract for HIV-specific ADCC activities in the tenofovir and placebo arms.
Plasma HIV-gp41 and p66-Specific ADNP Correlated to ADCC Activities in the Tenofovir Arm
Whether HIV-specific antibodies mediating ADNP and ADCC occupy the same immunological space and can correspond in terms of magnitude remains less well defined. We, therefore, sought to determine if ADNP and ADCC activities correlated with each other within each of the compartments. In the tenofovir arm, gp41-specific ADNP inversely correlated with gp41-specific ADCC activities in the plasma at 3 months (r = −0.68; p = 0.005) (Figure 6A). Plasma p66-specific ADNP and ADCC correlated significantly at 12 months (r = 0.67; p = 0.006) in the tenofovir arm (Figure 6B). No correlations were observed for gp120 or p24-specific activities in the plasma. Additional linear mixed effects models were performed to also determine if these correlations were predictive of the effect of tenofovir on specific nNAb functions over time. These analyses yielded no significant findings (data not shown).
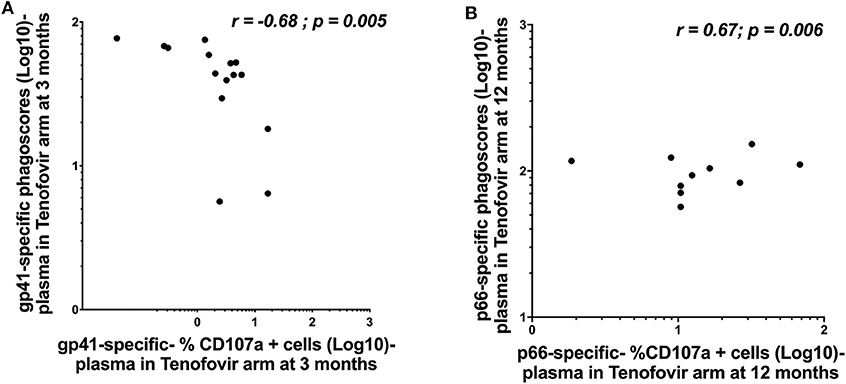
Figure 6. Correlations between ADNP (Log 10 Phagoscores) and NK cell activated ADCC (antibody-specific- %CD107a+ cells [Log10]) activities in the plasma of women in the tenofovir arm. (A) gp41-specific- phagoscores (Log10) at 3 months in the tenofovir arm, (B) p66-specific- %CD107a+ cells (Log10) at 12 months. Spearman R correlation analyses were performed. Significant values were identified as p < 0.05.
Fc-Mediated ADCC and ADNP Correlated Inversely With gp120- and gp41 Specific Antibody Titres in the Tenofovir Arm Only
Next, we investigated whether the titres of the HIV-specific antibodies from our previous study of this cohort [Log10 (MFI *dilution factor)] (14), directly correlated with the magnitude of ADNP and ADCC found in this study. In the tenofovir arm, significant inverse correlations were observed between the plasma gp41-specific ADNP and gp41-specific antibody titres at 6 months post-infection (r = −0.50; p = 0.015, Supplementary Table 5). Gp120-specific antibody titres in the tenofovir arm inversely correlated with gp120-specific ADCC in the plasma (r = −0.54; p = 0.009) (Supplementary Table 6) at 6 months. No further correlations for ADNP or ADCC were observed in relation to any of the other HIV-specific antibody titres in either compartments or study arms (Supplementary Tables 5, 6).
V1V2-gp70-Specific ADNP and p24-Specific ADCC Correlated With CD4+ T Cell Counts in the Tenofovir Arm
In order to understand the impact of nNAb functions on disease progression, we investigated the relationship between HIV-specific ADNP/ADCC activities and CD4+ T cell counts and viral loads. In the tenofovir arm at 6 months post-infection, V1V2-gp70-specific ADNP and p24-specific ADCC activities in the plasma directly correlated with CD4+ T cell counts [r = 0.35; p = 0.020—Figure 7A and r = 0.41; p = 0.055—Figure 7B, respectively], and no further associations were found.
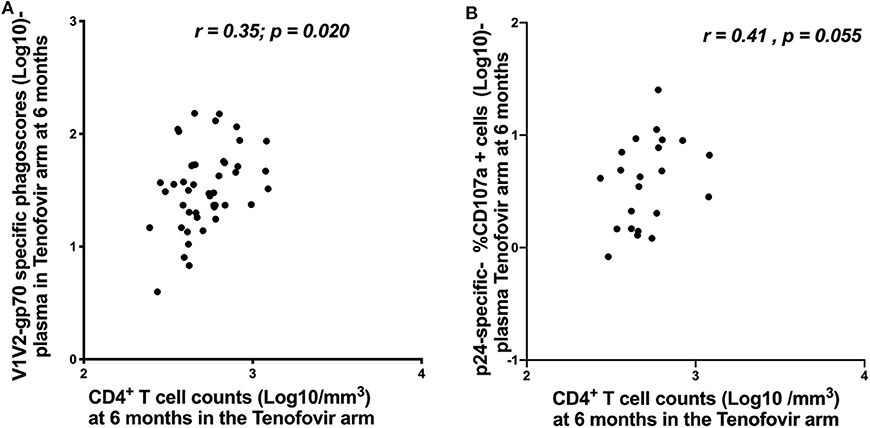
Figure 7. Correlations between CD4+ T cell counts and ADNP (Log 10 Phagoscores) and NK cell activated ADCC in the plasma for the tenofovir arm at 6 months. (A) V1V2-gp70-specific phagoscores (Log10) correlation with CD4+ T cell counts and (B) p24-specific- %CD107a+ cells (Log10]) correlation with CD4+ T cell counts. Significant values were identified as p < 0.05.
Discussion
Previous findings by our group demonstrated that topical PrEP use modulated HIV-1 antibody avidity post-seroconversion (7) underscoring potential public health implications for confirming incident HIV infections. Subsequently we showed higher detectability and titres of particular HIV-specific antibodies (14) in the genital tracts and blood of women who used the tenofovir gel prior to HIV-1 infection compared to those of the placebo gel users. We tested the hypothesis that the higher detectability and titres showed a corresponding increase in HIV-specific antibody Fc-mediated ADCC and ADNP functions in the genital tracts and the blood despite the reduced HIV-1 binding avidity (7) associated with tenofovir gel. We found gp120-and gp41-specific Fc-mediated antiviral activities in both compartments, regardless of prior topical tenofovir gel use.
HIV-specific antibodies from the genital tract and blood displayed nNAb functionality despite a prior study from our group having demonstrated that topical PrEP modulated plasma antibody binding avidity in these seroconverters (7). Although the HIV-specific nNAb activities were mostly similar between the tenofovir and placebo gel users, there were a few differences that distinguished the tenofovir from the placebo arm during the first 12 months post-infection. Despite gp41- and p24-specific ADNP in the blood being higher in the placebo compared to the tenofovir arm, genital tract gp41- and p24-specific ADNP were significantly higher in the tenofovir compared to the placebo arm at 6 and 12 months post-infection. The magnitudes of gp41- and p24-specific ADNP in the genital tract were also mirrored in the plasma. These data suggest the preservation of immune responses/function (8) in both compartments at least in the primary phase of HIV infection in women who used tenofovir. NNAbs isolated from vaginal samples of HIV infected women and more specifically gp41-specific IgG3 were shown to facilitate the phagocytosis of infectious HIV virions (33). The significantly increased p66-specific ADNP over time post-infection in the genital tract in the tenofovir arm, may be indicative of a developing immune response (11). Our data suggest that the significant delay in time to infection in the women who used tenofovir, an inhibitor of HIV reverse transcriptase (comprised of p66/p51 subunits) may have allowed for priming of p66-specific antibody immunity leading to the higher titres (14), and magnitude of ADNP over time. The functional significance of p66-specific antibodies is more challenging as p66 is not usually expressed on the surfaces of infected cells (13). P66-specific antibodies are likely elicited through the antigenic stimulation of infected cells undergoing subsequent cell lysis. Pol proteins may also be scavenged by antigen presenting cells, which then present these peptides subsequently targeted for degradation through ADNP or ADCC (13). These data provide evidence at least in vitro for the potential use of conserved viral proteins such as Pol and Gag as vaccine immunogens that elicit HIV-specific antibodies (12, 34) able to augment diverse antiviral activities.
In the tenofovir arm only, and in the blood, we also found significant inverse associations between ADNP and ADCC for gp41, and direct associations between ADNP and ADCC for p66. With prior PrEP use, these functions may be differentially regulated peripherally, as we found no such correlations between the two antiviral functions in the genital tract. Our data suggests that various nNAb functions may occupy specific compartmental spaces in pathogenic control, possibly to avoid redundancies. Whether both ADCC and ADNP need to be equally exerted in the genital tract and systemically to control infection, remains unknown.
We previously showed that HIV-specific IgG titres in the genital tract were considerably lower than those in the plasma in women from this study (14), the amount of antibodies in the genital tract depends on a combination of local IgG production (35) and transudation across the epithelial cell layer (36). Gp120-specific IgG titres inversely correlated with gp120-specific ADCC in the plasma, of women in the tenofovir arm. Env gp41-specific antibody titres also correlated inversely with gp41-specific ADNP. NHP studies have shown a strong and direct association between the Env IgG titres and ADCC function (in Env prime boost strategies) which protected against SHIV infection in the blood, vaginal and rectal mucosae (37, 38). Our data argue that the quality and not necessarily the quantity of these antibodies may be paramount in eliciting effective nNAb and neutralizing antiviral functions (39–41) and are analogous to the immune correlate studies in the RV144 trial where the quality of antibody activities were suggested to be a better surrogate indicator of reduced risk of infection (17, 42–44).
Viral control in elite controllers (45), reductions in the genital tract viral loads (28, 29) and delayed HIV disease progression (46) have been associated with increased ADCC. V1V2-gp70 loop specific antibodies identified as immune correlates of reduced risk of infection in the RV144 trial vaccinees (17, 18, 47, 48) elicited ADCC, virion capture and limited tier 1 virus neutralization (49–51). P24-specific IgG1 responses were associated with reduced viral loads (10, 52, 53) and improved CD4+ T cell counts in HIV-1 subtype C infected participants (9). The direct associations between V1V2-gp70-specific ADNP, p24-specific ADCC and CD4+ T cell counts in the plasma of HIV infected women from the tenofovir arm suggest that poly-functional antibodies may be needed to synergise nNAb activities to not only control viral load (44) and impact HIV disease progression but also to confer protection. We show that in infected women exposed to a topical PrEP, genital tract gp41, and gp120-specific IgG are able to mediate diverse functional activities such as ADCC. In HIV uninfected women from the HPTN035 microbicide trial only gp120-specific IgA could be detected in the genital tract (17). Whether the gp120-specific IgA of women from the HPTN035 trial were able to effect functional activity remains unknown. Our data suggest that prior topical PrEP did not modulate gp120 or gp41-specific IgG Fc mediated ADCC and that PrEP may be used in parallel as an HIV prevention strategy with vaccination that aims to induce envelope antibodies (17). Although these nNAbs did not prevent these women from acquiring HIV in our study, antibodies directed toward V1V2-gp70, gp120 (10, 18, 44, 54) and p24 that elicit ADCC have been correlated with slower disease progression (9, 46) or elite control (55) and a functional immune response (8, 9).
One of the limitations of this study is that we showed limited differences in HIV-specific nNAb functions between the arms despite having previously shown that higher HIV-specific antibody titres and detection associated with tenofovir gel (14). Indeed, compared to topical PrEP, oral PrEP may have a markedly different impact on immune responses. The lack of more distinct differences between the arms may in part be attributed to just over half of the tenofovir gel users, having detectable tenofovir levels in their CVLs likely indicative of women using the tenofovir gel inconsistently or not at all [reviewed by Baxter and Abdool Karim (56)]. Alternatively, tenofovir levels were dependent on women anticipating or having coitus with their partners which may or may not have coincided with the follow-up clinic visits where specimen collection was performed. It should be noted that these tenofovir levels in the genital tract were measured prior to HIV infection, and once women tested positive for HIV, the use of the topical tenofovir gel was discontinued. Furthermore, limited volumes of genital samples available, and the low concentrations of IgGs inherent in the CVL samples through dilution precluded further enrichment and verification of the specific IgG subclass or subclasses that mediated the ADCC activities (28). Antibodies from these diluted genital tract specimens contain a heterogenous pool of immunoglobulins which may have modulated or interfered with nNAb function in contrast to the purified IgG from the plasma specimens. Additionally, we further acknowledge that differences in the concentrations in the IgGs between the plasma and GT precluded comparisons for the nNAb functions between the compartments. Despite the shortcomings of the CVL specimens with the lower antibody concentrations and other factors that may interfere with nNAb functions than the plasma purified IgG, the CVL does represent more accurately the milieu in vivo and contains antibodies that facilitate antiviral functions. This is more especially for gp-120 specific antibodies in the genital tract that despite having lower titres (14), still exerted ADNP and ADCC. A further limitation is that we did not evaluate the impact of HIV-specific IgA-mediated nNAb activities in the blood or genital tracts of women. Env-specific IgAs in the blood have been shown to block ADCC activities mediated by IgG (57) whereas gp41-specific IgAs inhibited HIV-viral transcytosis across the mucosal tissue (58), mediated phagocytosis and blocked HIV-1 binding to host cell receptors (59). The contribution of IgAs on IgG mediated effector functions in the genital tract requires further investigation. Together these data further emphasize that in high risk populations where combination prevention strategies are required to prevent HIV, antibodies capable of diverse antiviral functions can be elicited at mucosal portals of entry.
Conclusions
The limited differences in HIV-specific nNAb functions between the tenofovir and placebo arms suggest that prior topical PrEP did not modulate humoral immune functions post HIV-seroconversion. Moreover, the simultaneous assessment of these two nNAb functions in both the compartments allowed us to gauge the discrepant and common HIV antibody-specific antiviral activities in different immunological spaces. These nNAb functions may be compartment-specific possibly to avoid immunological redundancies or to avert extra inflammation associated with nNAb-mediated activities. Together, the data provide further evidence for the role of nNAbs as quintessential to a diverse functional immune profile, especially in the area of vulnerability, the female genital tract.
Data Availability Statement
The datasets generated for this study are available on request to the corresponding author.
Ethics Statement
The studies involving human participants were reviewed and approved by University of KwaZulu-Natal Biomedical Research Ethics Committee. The patients/participants provided their written informed consent to participate in this study.
Author Contributions
KF designed and ran experiments, acquired and analyzed the data, and wrote and edited the manuscript. DA conceptualized, designed and acquired funding for the study, and wrote and edited the manuscript with CB. JM, BN, and DA assisted in study design. JM and AS assisted in experiments and JM and FO assisted in and verified the data analysis. DA, JM, SN, QA, and SA provided laboratory space for experimental procedures, samples to run the experiments, and edited the manuscript along with J-AP and AC. All authors contributed to the article and approved the submitted version.
Funding
We would like to acknowledge Dr. Galit Alter, from the Ragon Institute, and Dr. Georgia Tomaras, from The Duke Human Vaccine Institute, who shared their ADCC and ADNP protocols respectively for this study. We would like to thank the CAPRISA 004 and CAPRISA 002 teams as well as the study participants for their participation in these studies. Gilead Sciences supplied the tenofovir gel that was manufactured by CONRAD for the CAPRISA 004 trial. The CAPRISA 002 study team has received support from the National Institute of Allergy and Infectious Disease (NIAID), National Institutes of Health (NIH) (grants #AI51794, #AI104387, #AI115981, and #AI116086), from CONRAD (USAID co-operative grant #GP00-08-00005-00, subproject agreement #PPA-09-046), from the National Research Foundation (grant #67385), the Medical Research Council of South Africa, the Technology Innovation Agency, and the Columbia University-Southern African Fogarty AIDS International Training and Research Programme (AITRP) funded by the Fogarty International Centre, NIH (grant #D43TW00231). DA was supported through the Medical Research Council of South Africa Self-Initiated Grant (MRC SIR), the National Research Foundation (NRF) of South Africa Thuthuka (grant #TTK160517165310), the NRF Research Career Advancement Fellowship (grant #RCA13101656388), the Poliomyelitis Research Foundation (PRF17/02), and a senior fellowship through the European and Developing Countries Clinical Trials Partnership (EDCTP) (grant #TMA2017SF-1960) funds. KF was funded through the South African Department of Science and Technology and the National Research Foundation's DST-NRF Centre of Excellence in HIV prevention (grant #UID:96354), the University of KwaZulu-Natal College of Health Sciences, the Poliomyelitis Research Foundation (grant #18/95) and supported through the Sub-Saharan African Network for TB/HIV Research Excellence (SANTHE), a DELTAS Africa Initiative [grant #DEL-15-006]. The DELTAS Africa Initiative is an independent funding scheme of the African Academy of Sciences (AAS)'s Alliance for Accelerating Excellence in Science in Africa (AESA) and supported by the New Partnership for Africa's Development Planning and Coordinating Agency (NEPAD Agency) with funding from the Wellcome Trust [grant #107752/Z/15/Z] and the UK government. The views expressed in this publication are those of the author(s) and not necessarily those of AAS, NEPAD Agency, Wellcome Trust, or the UK government.
Conflict of Interest
The authors declare that the research was conducted in the absence of any commercial or financial relationships that could be construed as a potential conflict of interest.
The reviewer JP declared a past co-authorship with one of the authors SA to the handling editor.
Supplementary Material
The Supplementary Material for this article can be found online at: https://www.frontiersin.org/articles/10.3389/fimmu.2020.01274/full#supplementary-material
References
1. UNAIDS. UNAIDS Global HIV and AIDS statistics-2018 fact sheet. Geneva, Switzerland: The Joint United Nations Programme on HIV/AIDS 2018. (2019).
2. Baeten JM, Palanee-Phillips T, Brown ER, Schwartz K, Soto-Torres LE, Govender V, et al. Use of a vaginal ring containing dapivirine for HIV-1 prevention in women. N Engl J Med. (2016) 375:2121–32. doi: 10.1056/NEJMoa1506110
3. Abdool Karim Q, Abdool Karim SS, Frohlich JA, Grobler AC, Baxter C, Mansoor LE, et al. Effectiveness and safety of tenofovir gel, an antiretroviral microbicide, for the prevention of HIV infection in women. Science. (2010) 2010:1168–74. doi: 10.1126/science.1193748
4. Van Damme L, Corneli A, Ahmed K, Agot K, Lombaard J, Kapiga S, et al. Preexposure prophylaxis for HIV infection among African women. N Engl J Med. (2012) 367:411–22. doi: 10.1056/NEJMoa1202614
5. Marrazzo JM, Ramjee G, Richardson BA, Gomez K, Mgodi N, Nair G, et al. Tenofovir-based preexposure prophylaxis for HIV infection among African women. N Engl J Med. (2015) 372:509–18. doi: 10.1056/NEJMoa1402269
6. Kersh EN, Luo W, Zheng Q, Adams DR, Hanson D, Youngpairoj AS, et al. Reduced inflammation and CD4 loss in acute SHIV infection during oral pre-exposure prophylaxis. J Infect Dis. (2012) 206:770–9. doi: 10.1093/infdis/jis422
7. Laeyendecker O, Redd AD, Nason M, Longosz AF, Karim QA, Naranbhai V, et al. Antibody maturation in women who acquire HIV infection while using antiretroviral preexposure prophylaxis. J Infect Dis. (2015) 212:754–9. doi: 10.1093/infdis/jiv110
8. Mureithi MW, Poole D, Naranbhai V, Reddy S, Mkhwanazi NP, Sibeko S, et al. Preservation HIV-1-specific IFNγ+ CD4+ T cell responses in breakthrough infections following exposure to Tenofovir Gel in the CAPRISA 004 microbicide trial. J Acquir Immune Defici Syndr. (2012) 60:124–7. doi: 10.1097/QAI.0b013e31824f53a9
9. Chung AW, Makuba JM, Ndlovu B, Licht A, Robinson H, Ramlakhan Y, et al. Viral control in chronic HIV-1 subtype C infection is associated with enrichment of p24 IgG1 with Fc effector activity. AIDS. (2018) 32:1207. doi: 10.1097/QAD.0000000000001812
10. Tjiam MC, Morshidi MA, Sariputra L, Martin JN, Deeks SG, Tan DB, et al. The association of HIV-1 Gag-specific IgG antibodies with natural control of HIV-1 infection in individuals not carrying HLA-B* 57:01 is only observed in viremic controllers. J Acqu Immune Defic Syndr. (2017) 76:e90. doi: 10.1097/QAI.0000000000001477
11. Moja P, Cheynet V, Bourlet T, Mallet F, Lucht F, Pozzetto B, et al. Prognostic value of antibodies to p66 during the course of HIV1 infection. Immunol Lett. (1997) 1:133. doi: 10.1016/S0165-2478(97)85532-0
12. French MA, Center RJ, Wilson KM, Fleyfel I, Fernandez S, Schorcht A, et al. Isotype-switched immunoglobulin G antibodies to HIV Gag proteins may provide alternative or additional immune responses to 'protective'human leukocyte antigen-B alleles in HIV controllers. AIDS. (2013) 27:519–28. doi: 10.1097/QAD.0b013e32835cb720
13. Isitman G, Chung AW, Navis M, Kent SJ, Stratov I. Pol as a target for antibody dependent cellular cytotoxicity responses in HIV-1 infection. Virology. (2011) 412:110–6. doi: 10.1016/j.virol.2010.12.044
14. Archary D, Seaton K, Passmore J, Werner L, Deal A, Dunphy L, et al. Distinct genital tract HIV-specific antibody profiles associated with tenofovir gel. Muc Immunol. (2016) 9:821–33. doi: 10.1038/mi.2015.145
15. Overbaugh J, Morris L. The antibody response against HIV-1. Cold Spring Harbor Persp Med. (2012) 2:a007039. doi: 10.1101/cshperspect.a007039
16. Rerks-Ngarm S, Pitisuttithum P, Nitayaphan S, Kaewkungwal J, Chiu J, Paris R, et al. Vaccination with ALVAC and AIDSVAX to prevent HIV-1 infection in Thailand. N Engl J Med. (2009) 361:2209–20. doi: 10.1056/NEJMoa0908492
17. Yates NL, Liao H-X, Fong Y, Vandergrift NA, Williams WT, Alam SM, et al. Vaccine-induced Env V1-V2 IgG3 correlates with lower HIV-1 infection risk and declines soon after vaccination. Sci Trans Med. (2014) 6:228ra39–ra39. doi: 10.1126/scitranslmed.3007730
18. Haynes BF, Gilbert PB, McElrath MJ, Zolla-Pazner S, Tomaras GD, Alam SM, et al. Immune-correlates analysis of an HIV-1 vaccine efficacy trial. N Engl J Med. (2012) 366:1275–86. doi: 10.1056/NEJMoa1113425
19. Tuero I, Mohanram V, Musich T, Miller L, Vargas-Inchaustegui DA, Demberg T, et al. Mucosal B cells are associated with delayed SIV acquisition in vaccinated female but not male rhesus macaques following SIVmac251 rectal challenge. PLoS Pathog. (2015) 11:8. doi: 10.1371/journal.ppat.1005101
20. Barouch DH, Stephenson KE, Borducchi EN, Smith K, Stanley K, McNally AG, et al. Protective efficacy of a global HIV-1 mosaic vaccine against heterologous SHIV challenges in rhesus monkeys. Cell. (2013) 155:531–9. doi: 10.1016/j.cell.2013.09.061
21. Barouch DH, Alter G, Broge T, Linde C, Ackerman ME, Brown EP, et al. Protective efficacy of adenovirus/protein vaccines against SIV challenges in rhesus monkeys. Science. (2015) 349:320–4. doi: 10.1126/science.aab3886
22. Liu J, Ghneim K, Sok D, Bosche WJ, Li Y, Chipriano E, et al. Antibody-mediated protection against SHIV challenge includes systemic clearance of distal virus. Science. (2016) 353:1045–9. doi: 10.1126/science.aag0491
23. Chung AW, Rollman E, Center RJ, Kent SJ, Stratov I. Rapid degranulation of NK cells following activation by HIV-specific antibodies. J Immunol. (2009) 182:1202–10. doi: 10.4049/jimmunol.182.2.1202
24. Chung AW, Isitman G, Navis M, Kramski M, Center RJ, Kent SJ, et al. Immune escape from HIV-specific antibody-dependent cellular cytotoxicity (ADCC) pressure. Proc Natl Acad Sci. (2011) 108:7505–10. doi: 10.1073/pnas.1016048108
25. Worley MJ, Fei K, Lopez-Denman AJ, Kelleher AD, Kent SJ, Chung AW. Neutrophils mediate HIV-specific antibody-dependent phagocytosis and ADCC. J Immunol Methods. (2018) 457:41–52. doi: 10.1016/j.jim.2018.03.007
26. Mabuka J, Nduati R, Odem-Davis K, Peterson D, Overbaugh J. HIV-specific antibodies capable of ADCC are common in breastmilk and are associated with reduced risk of transmission in women with high viral loads. PLoS Pathog. (2012) 8:e1002739. doi: 10.1371/journal.ppat.1002739
27. Milligan C, Richardson BA, John-Stewart G, Nduati R, Overbaugh J. Passively acquired antibody-dependent cellular cytotoxicity (ADCC) activity in HIV-infected infants is associated with reduced mortality. Cell Host Microbe. (2015) 17:500–6. doi: 10.1016/j.chom.2015.03.002
28. Nag P, Kim J, Sapiega V, Landay AL, Bremer JW, Laingam S, et al. Women with cervicovaginal antibody-dependent cell-mediated cytotoxicity have lower genital HIV-1 RNA loads. J Infect Dis. (2004) 190:1970–8. doi: 10.1086/425582
29. Battle-Miller K, Eby CA, Landay AL, Cohen MH, Sha BE, Baum LL. Antibody-dependent cell-mediated cytotoxicity in cervical lavage fluids of human immunodeficiency virus type 1-infected women. J Infect Dis. (2002) 185:439–47. doi: 10.1086/338828
30. Abdool Karim SS, Kashuba AD, Werner L, Abdool Karim Q. Drug concentrations after topical and oral antiretroviral pre-exposure prophylaxis: implications for HIV prevention in women. Lancet. (2011) 378:279–81. doi: 10.1016/S0140-6736(11)60878-7
31. Ackerman ME, Moldt B, Wyatt RT, Dugast A-S, McAndrew E, Tsoukas S, et al. A robust, high-throughput assay to determine the phagocytic activity of clinical antibody samples. J Immunol Methods. (2011) 366:8–19. doi: 10.1016/j.jim.2010.12.016
32. Darrah PA, Patel DT, De Luca PM, Lindsay RW, Davey DF, Flynn BJ, et al. Multifunctional TH 1 cells define a correlate of vaccine-mediated protection against Leishmania major. Nat Med. (2007) 13:843–50. doi: 10.1038/nm1592
33. Tay MZ, Liu P, Williams LD, McRaven MD, Sawant S, Gurley TC, et al. Antibody-mediated internalization of infectious HIV-1 virions differs among antibody isotypes and subclasses. PLoS Pathog. (2016) 12:e1005817. doi: 10.1371/journal.ppat.1005817
34. Moog C, Dereuddre-Bosquet N, Teillaud J, Biedma M, Holl V, Van Ham G, et al. Protective effect of vaginal application of neutralizing and nonneutralizing inhibitory antibodies against vaginal SHIV challenge in macaques. Mucosal Immunol. (2014) 7:46–56. doi: 10.1038/mi.2013.23
35. Bélec L, Dupré T, Prazuck T, Tévi-Bénissan C, Kanga J-M, Pathey O, et al. Cervicovaginal overproduction of specific IgG to human immunodeficiency virus (HIV) contrasts with normal or impaired IgA local response in HIV infection. J Infect Dis. (1995) 172:691–7. doi: 10.1093/infdis/172.3.691
36. Li Z, Palaniyandi S, Zeng R, Tuo W, Roopenian DC, Zhu X. Transfer of IgG in the female genital tract by MHC class I-related neonatal Fc receptor (FcRn) confers protective immunity to vaginal infection. Proc Natl Acad Sci. (2011) 108:4388–93. doi: 10.1073/pnas.1012861108
37. Xiao P, Zhao J, Patterson LJ, Brocca-Cofano E, Venzon D, Kozlowski PA, et al. Multiple vaccine-elicited nonneutralizing antienvelope antibody activities contribute to protective efficacy by reducing both acute and chronic viremia following simian/human immunodeficiency virus SHIV89. 6P challenge in rhesus macaques. J Virol. (2010). 84:7161–73. doi: 10.1128/JVI.00410-10
38. Florese RH, Demberg T, Xiao P, Kuller L, Larsen K, Summers LE, et al. Contribution of nonneutralizing vaccine-elicited antibody activities to improved protective efficacy in rhesus macaques immunized with Tat/Env compared with multigenic vaccines. J Immunol. (2009) 182:3718–27. doi: 10.4049/jimmunol.0803115
39. Hessell AJ, Poignard P, Hunter M, Hangartner L, Tehrani DM, Bleeker WK, et al. Effective, low-titer antibody protection against low-dose repeated mucosal SHIV challenge in macaques. Nat Med. (2009) 15:951–4. doi: 10.1038/nm.1974
40. Hessell AJ, Rakasz EG, Poignard P, Hangartner L, Landucci G, Forthal DN, et al. Broadly neutralizing human anti-HIV antibody 2G12 is effective in protection against mucosal SHIV challenge even at low serum neutralizing titers. PLoS Pathog. (2009) 5:e1000433. doi: 10.1371/journal.ppat.1000433
41. Mascola JR, Stiegler G, VanCott TC, Katinger H, Carpenter CB, Hanson CE, et al. Protection of macaques against vaginal transmission of a pathogenic HIV-1/SIV chimeric virus by passive infusion of neutralizing antibodies. Nat Med. (2000) 6:207–10. doi: 10.1038/72318
42. Chung AW, Ghebremichael M, Robinson H, Brown E, Choi I, Lane S, et al. Polyfunctional Fc-effector profiles mediated by IgG subclass selection distinguish RV144 and VAX003 vaccines. Sci Trans Med. (2014) 6:228ra38–ra38. doi: 10.1126/scitranslmed.3007736
43. Choi I, Chung AW, Suscovich TJ, Rerks-Ngarm S, Pitisuttithum P, Nitayaphan S, et al. Machine learning methods enable predictive modeling of antibody feature:function relationships in RV144 vaccinees. PLoS Comput Biol. (2015) 11:e1004185. doi: 10.1371/journal.pcbi.1004185
44. Ackerman ME, Mikhailova A, Brown EP, Dowell KG, Walker BD, Bailey-Kellogg C, et al. Polyfunctional HIV-specific antibody responses are associated with spontaneous HIV control. PLoS Pathog. (2016) 12:e1005315. doi: 10.1371/journal.ppat.1005315
45. Lambotte O, Ferrari G, Moog C, Yates NL, Liao H-X, Parks RJ, et al. Heterogeneous neutralizing antibody and antibody-dependent cell cytotoxicity responses in HIV-1 elite controllers. AIDS. (2009) 23:897–906. doi: 10.1097/QAD.0b013e328329f97d
46. Baum LL, Cassutt KJ, Knigge K, Khattri R, Margolick J, Rinaldo C, et al. HIV-1 gp120-specific antibody-dependent cell-mediated cytotoxicity correlates with rate of disease progression. J Immunol. (1996) 157:2168–73.
47. Zolla-Pazner S, deCamp AC, Cardozo T, Karasavvas N, Gottardo R, Williams C, et al. Analysis of V2 antibody responses induced in vaccinees in the ALVAC/AIDSVAX HIV-1 vaccine efficacy trial. PLoS ONE. (2013) 8:53629. doi: 10.1371/journal.pone.0053629
48. Chung AW, Kumar MP, Arnold KB, Yu WH, Schoen MK, Dunphy LJ, et al. Dissecting polyclonal vaccine-induced humoral immunity against HIV using systems serology. Cell. (2015) 163:988–98. doi: 10.1016/j.cell.2015.10.027
49. Liao H-X, Bonsignori M, Alam SM, McLellan JS, Tomaras GD, Moody MA, et al. Vaccine induction of antibodies against a structurally heterogeneous site of immune pressure within HIV-1 envelope protein variable regions 1 and 2. Immunity. (2013) 38:176–86. doi: 10.1016/j.immuni.2012.11.011
50. Pollara J, Bonsignori M, Moody MA, Liu P, Alam SM, Hwang K-K, et al. HIV-1 vaccine-induced C1 and V2 Env-specific antibodies synergize for increased antiviral activities. J Virol. (2014) 88:7715–26. doi: 10.1128/JVI.00156-14
51. Liu P, Williams LD, Shen X, Bonsignori M, Vandergrift NA, Overman RG, et al. Capacity for infectious HIV-1 virion capture differs by envelope antibody specificity. J Virol. (2014) 88:5165–70. doi: 10.1128/JVI.03765-13
52. Banerjee K, Klasse P, Sanders RW, Pereyra F, Michael E, Lu M, et al. IgG subclass profiles in infected HIV type 1 controllers and chronic progressors and in uninfected recipients of Env vaccines. AIDS Res Hum Retrovir. (2010) 26:445–58. doi: 10.1089/aid.2009.0223
53. Tjiam MC, Taylor JP, Morshidi MA, Sariputra L, Burrows S, Martin JN, et al. Viremic HIV controllers exhibit high plasmacytoid dendritic cell-reactive opsonophagocytic IgG antibody responses against HIV-1 p24 associated with greater antibody isotype diversification. J Immunol. (2015) 194:5320–8. doi: 10.4049/jimmunol.1402918
54. Seaton KE, Ballweber L, Lan A, Donathan M, Hughes S, Vojtech L, et al. HIV-1 specific IgA detected in vaginal secretions of HIV uninfected women participating in a microbicide trial in Southern Africa are primarily directed toward gp120 and gp140 specificities. PloS ONE. (2014) 9:e101863. doi: 10.1371/journal.pone.0101863
55. Madhavi V, Wines BD, Amin J, Emery S, Lopez E, Kelleher A, et al. HIV-1 Env-and Vpu-specific antibody-dependent cellular cytotoxicity responses associated with elite control of HIV. J Virol. (2017) 91:e00700–17. doi: 10.1128/JVI.00700-17
56. Baxter C, Abdool Karim S. Combination HIV prevention options for young women in Africa. Afric J AIDS Res. (2016) 15:109–21. doi: 10.2989/16085906.2016.1196224
57. Tomaras GD, Ferrari G, Shen X, Alam SM, Liao H-X, Pollara J, et al. Vaccine-induced plasma IgA specific for the C1 region of the HIV-1 envelope blocks binding and effector function of IgG. Proc Natl Acad Sci USA. (2013) 110:9019–24. doi: 10.1073/pnas.1301456110
58. Tudor D, Derrien M, Diomede L, Drillet A, Houimel M, Moog C, et al. HIV-1 gp41-specific monoclonal mucosal IgAs derived from highly exposed but IgG-seronegative individuals block HIV-1 epithelial transcytosis and neutralize CD4+ cell infection: an IgA gene and functional analysis. Muc Immunol. (2009) 2:412–26. doi: 10.1038/mi.2009.89
Keywords: Fc-mediated activity, tenofovir, HIV, women, ADCC–antibody dependent cellular cytotoxicity, ADNP, genital tract
Citation: Fisher KL, Mabuka JM, Sivro A, Ngcapu S, Passmore J-AS, Osman F, Ndlovu B, Abdool Karim Q, Abdool Karim SS, Chung AW, Baxter C and Archary D (2020) Topical Tenofovir Pre-exposure Prophylaxis and Mucosal HIV-Specific Fc-Mediated Antibody Activities in Women. Front. Immunol. 11:1274. doi: 10.3389/fimmu.2020.01274
Received: 17 September 2019; Accepted: 20 May 2020;
Published: 06 July 2020.
Edited by:
Mats Bemark, University of Gothenburg, SwedenReviewed by:
Charles Kelly, King's College London, United KingdomJustin Pollara, Duke University, United States
Copyright © 2020 Fisher, Mabuka, Sivro, Ngcapu, Passmore, Osman, Ndlovu, Abdool Karim, Abdool Karim, Chung, Baxter and Archary. This is an open-access article distributed under the terms of the Creative Commons Attribution License (CC BY). The use, distribution or reproduction in other forums is permitted, provided the original author(s) and the copyright owner(s) are credited and that the original publication in this journal is cited, in accordance with accepted academic practice. No use, distribution or reproduction is permitted which does not comply with these terms.
*Correspondence: Derseree Archary, ZGVzaC5hcmNoYXJ5QGNhcHJpc2Eub3Jn