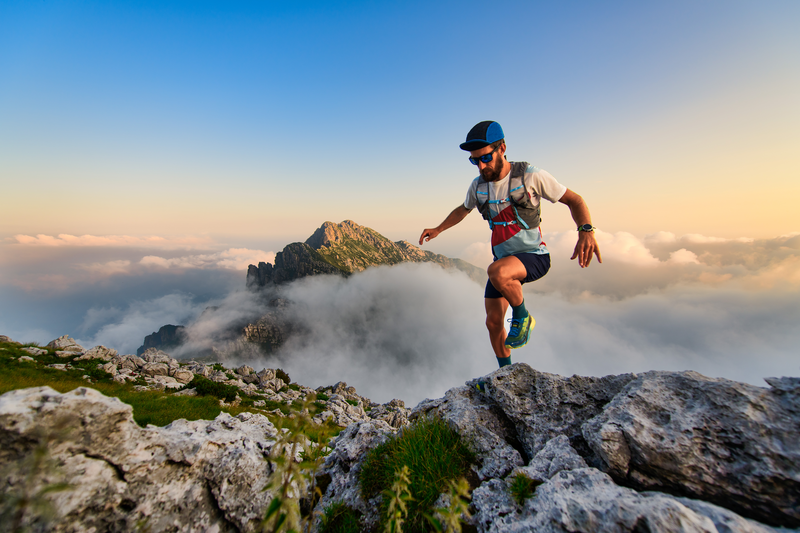
94% of researchers rate our articles as excellent or good
Learn more about the work of our research integrity team to safeguard the quality of each article we publish.
Find out more
ORIGINAL RESEARCH article
Front. Immunol. , 08 May 2020
Sec. Comparative Immunology
Volume 11 - 2020 | https://doi.org/10.3389/fimmu.2020.00904
The effects of the oral administration of Rehmannia glutinosa polysaccharide (RGP-1) on the immunoregulatory properties, antioxidant activity, and resistance against Aeromonas hydrophila in Cyprinus carpio L. were investigated. The purified RGP-1 (250, 500, and 1,000 μg/mL) was co-cultured with the head kidney cells of the common carp. The proliferation and phagocytosis activities of the head kidney cells, and the concentration of nitric oxide (NO) and cytokines in the culture medium were determined. Next, 300 common carps (47.66 ± 0.43 g) were randomly divided into five groups; the two control groups (negative and positive) were administered sterile PBS and the three treatment groups were administered different concentrations of RGP-1 (250, 500, and 1,000 μg/mL) for seven days. Subsequently, the positive and treatment groups were infected with A. hydrophila, and the negative group was administered sterile PBS for 24 h. The concentration of NO, cytokines, lysozyme (LZM), and alkaline phosphatase (AKP) in serum, the total antioxidant capacity (T-AOC), the levels of malonaldehyde (MDA) and glutathione (GSH), and the total activities of superoxide dismutase (T-SOD), catalase (CAT), and glutathione peroxidase (GSH-Px) in the hepatopancreas of the common carp were tested. We observed that RGP-1 could significantly enhance the proliferation and phagocytosis activities (P < 0.05), besides inducing the production of NO, pro-inflammatory cytokines (TNF-α, IL-1β, IL-6, IL-12) and anti-inflammatory cytokines (IL-10, TGF-β) (P < 0.05) in vitro. The in vivo experimental results revealed that RGP-1 significantly enhanced NO production, protein levels of pro-inflammatory cytokines (TNF-α, IL-1β, IL-6, IL-12), LZM and AKP activities, and the antioxidant content (T-AOC, SOD, CAT, GSH, GSH-Px, and MDA) compared to that observed in the negative group prior to A. hydrophila infection (P < 0.05). NO, pro-inflammatory cytokines, LZM and AKP activities were significantly lower than that in the positive group after infection (P < 0.05). However, whether infected or not, the expression of anti-inflammatory cytokines (IL-10, TGF-β) increased significantly in the RGP-1-treated groups (P < 0.05). Therefore, the results suggested that RGP-1 could enhance the non-specific immunity, antioxidant activity and anti-A. hydrophila activity of the common carp, and could be used as a safe and effective feed additive in aquaculture.
Cyprinus carpio is the major commercial fish species in China. In recent years, the increased frequency of diseases in the breeding process of the common carp have severely hindered industrial development. According to statistics, in 2017, the economic loss resulting from diseases in China's fishery industry reached 3.405 billion yuan, which increased by 24.3% compared to that in 2016 (1). Therefore, it is of significant importance to determine effective antibiotic substitutes that can help improve the immunity and disease resistance of aquaculture animals, and construct a healthy aquaculture system with safe aquatic products based on the idea of “no antibiotics and no worries” in the aquaculture industry. In the strategy of discovering alternative antibiotics, based on the large numbers of research foundations and application prospects, traditional Chinese medicine (TCM) and polysaccharides used in TCM are ideal choices.
In recent years, increasing attention has been paid to the effect of TCM on immune regulation (2–6), and several studies have demonstrated that polysaccharides are one of the important active ingredients. For example, the polysaccharide AEPS derived from the roots of Actinidia eriantha could significantly increase the expression of cytokines in rat medullary dendritic cells (BMDCs) (7). Smilax glabra Roxb polysaccharide SGRP1 promoted the phagocytic activity of macrophages and increased the expression of NO and cytokines (8). In addition, polysaccharides CRP from Collybia radicata mushroom, TLH-3 from Tricholoma lobayense, and HEP-S from Hericium erinaceus fruiting bodies all significantly promoted the proliferation and phagocytosis of RAW264.7 cells, and induced the expression of NO and cytokines (9–11). Moreover, polysaccharides also play a crucial role in aquaculture. For instance, the addition of Ganoderma lucidum polysaccharide to the basic diet significantly improved the survival and growth rates and the digestive enzyme activities of Macrobrachium rosenbergii (12). Both Ficus carica polysaccharide and Hericium caput-medusae polysaccharide stimulated the immune response in grass carp, and upregulated the immune-related genes, thereby enhancing the host's disease resistance ability (3, 13). Polysaccharides from Padina gymnospora and Astragalus sp. also improved the non-specific and specific immune response in the immunity-related organs in the common carp, and enhanced the immune regulation and disease resistance of the host (14, 15). In addition, polysaccharides from algae and other species are also widely used in aquaculture (16, 17).
As a part of TCM, Rehmannia glutinosa is widely cultivated in the Henan, Shandong, Shanxi, and Shaanxi provinces. Studies have revealed that the R. glutinosa polysaccharide (RGP) is one of its major functional components. For example, RGP could improve the vascular inflammation in diabetic mice induced by streptozotocin, and exerted positive therapeutic effects on hyperglycaemia and hyperlipidaemia in a mice model (18). RGP could also induce the maturation of BMDCs (19), the proliferation of splenic lymphocytes and the IL-12 and IFN-γ expression regulated by T cells (20). In addition, RGP liposomes could enhance the antigen presenting ability of mature DC cells and increase the number of splenic lymphocytes, IgGs, central and effector memory cells in vivo (21). However, to the best of our knowledge, no research has been conducted on the immune regulation, antioxidant and disease resistance effects exerted by RGP on the common carp. Based on previous research, the objective of this study was to determine the immunomodulatory, antioxidant and disease resistance effects exerted by RGP-1 derived from R. glutinosa, and to explore its feasibility as an immunomodulatory agent.
The roots of R. glutinosa were collected from the Wuzhi County, Jiaozuo, China. The polysaccharides from the roots of R. glutinosa (RGP-1) were prepared and characterized, as previously described (22, 23). In brief, 100 g of the dried root powder of R. glutinosa were extracted to prepare the aqueous extract of the crude polysaccharide based on the following steps: 100°C (extraction temperature), 2 h (extraction time), 20.0 mL/g (ratio of water to raw material) and three extractions (number of extractions). The extraction solution was centrifuged and filtered through a 0.45 μm filter membrane. The filtrate was then concentrated to a 1 Lvolume by decompression at 70°C in a rotary evaporator. The aqueous fraction was added to four times volume of 95% ethyl alcohol to form a precipitate at 4°C overnight. The precipitate was isolated through centrifugation at 12,000 × g for 10 min at 25°C, and rinsed with absolute ethanol, acetone and diethyl ether twice in turn, and then lyophilized to obtain the crude polysaccharide with a yield of 12.6 g. The crude polysaccharide was dissolved in deionized water and centrifuged to remove the extra insoluble constituents, and 10 mL of the supernatant was introduced into a DEAE-cellulose 32 column (60 × 6 cm, Cl− form), followed by stepwise elution with deionized water and 0.2 mol/L NaCl at a flow rate of 0.23 BV/h. The eluate was then collected and loaded onto a Sephacryl S-200HR column (2.6 × 100 cm), followed by elution with 0.2 mol/L NaCl at a flow rate of 0.32 BV/h. Finally, the eluate was collected, dialyzed with water, and lyophilized to obtain the pure RGP-1 with a yield of 3.1 g.
The head kidney cells were isolated from healthy common carps (24) and cultured in RPMI-1640 medium (GIBCO, USA) with 10% fetal bovine serum (GIBCO, USA), 100 unit/mL penicillin (Sigma, USA) and 100 μg/mL streptomycin (Sigma, USA) in 24-well tissue culture plates (Corning, USA; 2 × 106 cells/mL). Following this, the cells were co-cultured with different concentrations of RGP-1 (0, 250, 500, 1,000 μg/mL) at 28°C for 24 h. The production of cytokines and NO in the culture supernatants were detected.
To determine the effect of RGP-1 on the proliferation of head kidney cells, RGP-1 (0, 250, 500, 1,000 μg/mL) was added to the 24-well tissue culture plates (2 × 106 cells/mL). After incubating for 20 h, 20 μL of MTT (5 mg/mL) was added to each well, and the cells were re-incubated for another 4 h. The supernatant was then discarded and DMSO (150 μL) was added to each well. The absorbance values were measured at 570 nm using an EnSpire 2300 microplate reader (PE EnSpire, USA) (9, 25).
For phagocytosis, similar to the method mentioned previously, the supernatants were discarded after the cells were incubated with the RGP-1 (0, 250, 500, 1,000 μg/mL) for 20 h. One hundred microlitres of neutral red solution (0.1 %) was added to each well. The supernatants were then discarded after 4 h, and the cells were washed thrice with PBS to remove the neutral red. One hundred microlitres of a cell lysis buffer [ethanol: glacial acetic acid = 1:1 (v/v)] was added to each well, and the plates were incubated for 2 h to extract the phagocytosed neutral red. The absorbance values at 540 nm were measured using an EnSpire 2300 microplate reader (PE EnSpire) (9).
After a 24 h incubation period, the levels of NO in the culture medium were determined using the Total Nitric Oxide Assay Kit (Beyotime, Shanghai, China), according to the manufacturer's instructions. The absorbance values at 490 nm were determined using an EnSpire 2300 microplate reader (PE EnSpire). The NO concentrations were estimated by referring to a standard curve for a 5-fold serial dilution of NaNO2.
The concentration of cytokines in the culture supernatant was determined using the ELISA with polyclonal antibodies prepared in our lab that evaluated the in vitro immune regulatory activity of RGP-1 in common carps (26). Briefly, 96-well plates (Corning, USA) were coated with culture supernatants in a coating buffer (0.05 M carbonate buffer, pH 9.6) and maintained overnight at 4°C. The plates were blocked with 10% skimmed milk powder and washed. The first antibodies (TNF-α, IL-1β, IL-6, IL-12, IL-10, and TGF-β, 1: 2000) were added to the plates, and incubated for 2 h at 37°C. Next, the plates were washed again, and the second antibody (goat anti-rabbit, conjugated with horseradish peroxidise, 1:10000) was added, followed by incubation for 1 h at 37°C and an additional wash. The chromogenic signals were developed at 37°C for 30 min using 3, 3′, 5, 5′-tetramethylbenzidine (TMB) as a substrate. The reactions were terminated using 50 μL of 2 N H2SO4, and the absorbance values at 450 nm were measured using a Microplate Reader (PerkinElmer, USA). The equivalent levels of TNF-α, IL-1β, IL-6, IL-12, IL-10, and TGF-β were determined by comparing to reference curves constructed using the corresponding standards. The results were expressed as the concentration of cytokines in the culture medium (pg/mL).
To determine the effect of RGP-1 on immunity, antioxidants and disease resistance in vivo, we adopted the following experimental design (22). Cyprinus carpio L. were obtained from the “Aquaculture Breeding Base of Henan Aquatic Technology Extension Station,” Henan Province, China. Thereafter, the fish, which were normal feeding, disease-free, and non-injured, were subjected to routine physical and microbiological examination to confirm the absence of bacterial diseases or any abnormal clinical signs, and the 300 common carps (47.66 ± 0.43 g) were randomly divided into 15 300-L tanks (20 fish per tank). The fish were fed commercial diets twice daily to apparent satiation (10:00 and 17:00) (Table 1).
After 2 weeks of acclimation, the 15 tanks were divided into five groups, with three parallel subgroups for each treatment. Group 1 (G1, negative control) and 2 (G2, positive control) were administered 0.1 mL PBS; G3, G4, and G5 were administered 0.1 mL of different concentrations (250, 500, and 1,000 μg/mL) of RGP-1, respectively, once daily for 7 days. For sample collection, six fish per tank (except for the G2 tank) were randomly selected and anesthetized using ethyl 3-aminobenzoate (MS-222, 40 mg/L) after 7 days of the gavage trial. During the trial, ~30% of the culture water was replaced once a day with fresh, dechlorinated water at a similar temperature and the oxygen–saturation was maintained by aeration. The tanks were maintained under a natural light/dark regime. During the experimental period, the water quality parameters [mean ± standard deviation (SD)] monitored were as follows: water temperature, 26.0 ± 0.5°C; pH, 6.5–7.1, dissolved oxygen > 6.1 ± 0.5 mg/L; -N < 0.5 mg/L; NO2-N < 0.05 mg/L.
After the feeding trial, the fish remaining from each replicate (except in G1) were injected with 100 μL of A. hydrophila (Ah 01) (LD50 = 5 × 106 CFU/mL) intraperitoneally, which was provided by our laboratory, and cultured as previously described (26). In the negative control group (G1), the fish were injected with 100 μL of sterilized PBS. The culture conditions were the same as previously mentioned. After 24 h of the infection, six fish were randomly selected per tank and anesthetized for the sample collection.
After 7 days of gavage and 24 h of injection, six fish were anesthetized per tank using MS-222 (40 mg/L), and blood samples were collected from the caudal vein by puncturing with a syringe, and the serum was separated by centrifugation (4°C, 3,000 × g, 30 min). Finally, the fish were dissected to isolate the hepatopancreas. All samples were stored at −80°C until further analysis.
After 7 days of gavage and 24 h of injection, the NO levels in the serum were determined according to the method described in nitrite assay for the estimation of NO.
After the 7 days gavage, the antioxidant stress ability of RGP-1 in common carps was measured. In brief, the tissue homogenate of hepatopancreas (10%) was prepared using sterile saline, the supernatant was collected after centrifugation (4°C, 8,000 × g, 10 min), and the total antioxidant capacity (T-AOC), malonaldehyde (MDA) and glutathione (GSH) levels and the total activities of superoxide dismutase (T-SOD), catalase (CAT), and glutathione peroxidase (GSH-Px) in the supernatant were determined according to the kit instructions (Nanjing Jiancheng Bioengineering Institute, Nanjing, China).
After the 7 days gavage and 24 h of injection, the levels of TNF-α, IL-1β, IL-6, IL-12, IL-10, and TGF-β in the serum were measured according to the method described in Enzyme-Linked Immunosorbent Assay (ELISA) for the quantitative analysis of cytokines. The serum lysozyme (LZM) and alkaline phosphatase (AKP) activities were measured according to the kit instructions (Nanjing Jiancheng Bioengineering Institute).
The SPSS statistical package version 25.0 (SPSS Inc., Chicago, IL, USA) was used for statistical analysis, and the normality and homogeneity of data were checked and confirmed. The data were reported as mean ± SD, and subjected to the one-way analysis of variance (ANOVA), followed by Duncan's multiple range test as a post-hoc test to evaluate the significant difference between treatments at P < 0.05.
The effects exerted by RGP-1 on the proliferation and phagocytosis of the head kidney cells are presented in Figures 1, 2. The proliferation and phagocytosis in the treatment groups were higher than that in the control group. As the RGP-1 concentration increased from 250 to 1,000 μg/mL, cell proliferation and phagocytosis increased gradually (P < 0.05). Particularly at 1,000 μg/mL, RGP-1 treatment increased cell proliferation and phagocytosis by 2.07 and 3.42 times, compared that in the control group (administered sterile PBS), respectively. In general, RGP-1 was non-toxic to the head kidney cells at the tested concentrations.
Figure 1. The effect of RGP-1 on the proliferation of the head kidney cells of common carps. The head kidney cells (2 × 106 cells/well in 96-well plates) were incubated with the indicated concentrations of RGP-1 in DMEM at 28°C for 20 h; the proliferation activity of the head kidney cells was determined using an MTT kit. The data are expressed as the mean ± standard deviation (SD) (n = 6). The error bars represent the SDs; values marked with different letters are significantly different between the treatment groups (P < 0.05).
Figure 2. The effect of RGP-1 on the phagocytosis of the head kidney cells of common carps. The head kidney cells (2 × 106 cells/well in 96-well plates) were incubated with the indicated concentrations of RGP-1 in DMEM at 28°C for 20 h; the phagocytosis activity of the head kidney cells was determined using the neutral red method. The data are expressed as the mean ± SD (n = 6). The error bars represent the SDs; values marked with different letters are significantly different between the treatment groups (P < 0.05).
The results of NO detection are presented in Figure 3 and Table 2. After exposure or gavage using different concentrations of RGP-1, the levels of NO in the head kidney cells and the serum of common carps in the groups that were only treated with RGP-1 increased in a dose-dependent manner both in vitro (Figure 3) and in vivo (Table 2), and were significantly higher than those in the control group (P < 0.05). The NO content in the serum of common carps increased significantly in the G2 group (only infected with A. hydrophila), whereas it reduced significantly in the groups pre-treated with RGP-1 after infection with A. hydrophila (P < 0.05, Table 2), and the ability of reducing NO production increased with the increase in RGP-1 concentration. Particularly, when the RGP-1 concentration was 1,000 μg/mL, the NO production reduced by 69.0%, and there was no significant difference with the control group (P > 0.05, Table 2).
Figure 3. The effect of RGP-1-induced NO production in the head kidney cells of common carps. The head kidney cells (2 × 106 cells/well in 96-well plates) were incubated with the indicated concentrations of RGP-1 in DMEM at 28°C for 24 h; the levels of NO in the culture medium were determined using the Total Nitric Oxide Assay Kit. The data are expressed as mean ± SD (n = 6). The error bars represent the SDs; values marked with different letters are significantly different between the treatment groups (P < 0.05).
Table 2. Effects of RGP-1 or/and A. hydrophila on NO levels, and the LZM and AKP activities in the serum of common carps.
As shown in Figure 4, at the end of the 7 days feeding experiment, the T-AOC and GSH-Px activity in hepatopancreas appeared to follow a dose-dependent pattern, and were significantly higher at a higher concentration (1,000 μg/mL of RGP-1) than in the control (P < 0.05, Figures 4A, 5C). The T-SOD activity and GSH levels were also higher in the treatment groups than in the control group (P < 0.05), and peaked at 500 μg/mL of RGP-1 (P < 0.05, Figures 4A, 5E). Similarly, the CAT activity peaked at the RGP-1 concentration of 250 μg/mL, and was significantly higher than that in the control group (P < 0.05, Figure 4D). Conversely, the MDA levels were significantly lower in the RGP-1 treatment groups, with the lowest activity recorded at 1,000 μg/mL of RGP-1 (P < 0.05, Figure 4F).
Figure 4. The effects of RGP-1 on the levels of antioxidant substances in the hepatopancreas of common carps. Common carps (47.66 ± 0.43 g) were gavaged with the indicated concentrations of RGP-1 for 7 days. (A) T-AOC, (B) T-SOD, and the levels of (C) GSH-Px, (D) CAT, (E) GSH, and (F) MDA in hepatopancreas were measured according to the kit instructions. The data are expressed as mean ± SD (n = 6). The error bars represent the SDs; values marked with different letters are significantly different between the treatment groups (P < 0.05).
Figure 5. The effects of RGP-1 on the cytokine secretion from the head kidney cells. (A) The pro-inflammatory cytokines TNF-α, IL-1β, IL-6, and IL-12; (B) the anti-inflammatory cytokines IL-10 and TGF-β. Head kidney cells (2 × 106 cells/well in 96-well plates) were incubated with the indicated concentrations of RGP-1 in DMEM at 28°C for 24 h; the cytokine levels in the culture medium were determined using the ELISA. The data are expressed as mean ± SD (n = 6). The error bars represent the SDs; values marked with different letters are significantly different between the treatment groups (P < 0.05).
The levels of inflammatory cytokines TNF-α, IL-1β, IL-6, IL-12, IL-10, and TGF-β in head kidney cells were used to evaluate the in vitro immunoregulatory activity of RGP-1. The results indicated that RGP-1 significantly stimulated the secretion of cytokines TNF-α, IL-6, IL-10, and TGF-β in a dose-dependent manner (Figure 5), and at 1,000 μg/mL concentration of RGP-1, the enhancement of TNF-α, IL-6, IL-10, and TGF-β production was ~1.28, 1.71, 1.45, and 1.70 times higher than that in the control group, respectively (P < 0.05). Meanwhile, the expression trend for IL-1β and IL-12 exhibited a “hump pattern” with increasing RGP-1 concentration. When the concentration of RGP-1 was 250 μg/mL, the expression levels of IL-1β and IL-12 were the highest, with the expression levels in the treatment groups higher than that in the control group (P < 0.05, Figure 5).
Based on the in vitro experiment, we also determined the concentration of cytokines in the serum to further assess the immunoregulatory effects of RGP-1 in vivo. As shown in Table 3, RGP-1 significantly stimulated the secretion of cytokines TNF-α and IL-6 in a dose-dependent manner, whereas the expression trend for IL-1β and IL-12 exhibited a “hump pattern” with increasing RGP-1 concentration. However, the in vivo expression patterns of anti-inflammatory cytokines IL-10 and TGF-β were different from those in vitro. Compared to that in G1, RGP-1 could significantly increase the expression levels of IL-10 and TGF-β in the treatment groups (P < 0.05); however, there were no significant differences between the groups with different concentrations (P > 0.05).
The results of cytokine detection in serum upon A. hydrophila infection are presented in Table 3. Compared with that in G1, the expression of pro-inflammatory cytokines TNF-α, IL-1β, IL-6, and IL-12 and anti-inflammatory cytokines IL-10 and TGF-β increased significantly in G2 (P < 0.05), which was only infected with A. hydrophila. Meanwhile, compared to G2, the expression of pro-inflammatory cytokines TNF-α, IL-1β, IL-6, and IL-12 in the RGP-1 treatment groups reduced significantly in a dose-dependent manner (P < 0.05). Particularly, for IL-12, the expression patterns in the RGP-1 (500 and 1,000 μg/mL) treatment groups and G1 had no significant difference (P > 0.05). With respect to the anti-inflammatory cytokines, RGP-1 significantly increased the IL-10 and TGF-β levels in the G3 group (250 μg/mL) (P < 0.05), while in the G4 and G5 groups (500 and 1,000 μg/mL), the expression levels of IL-10 and TGF-β reduced, and there were no significant differences with the levels in G1 (P > 0.05). In summary, it was observed that anti-inflammatory cytokines were more sensitive to RGP-1 (Table 3).
The LZM and AKP activities in the serum were also determined after RGP-1 feeding and A. hydrophila infection. As shown in Table 2, the activities of LZM and AKP could be significantly altered by RGP-1, and seemed to follow an upward trend (P < 0.05) with the increase in RGP-1 concentrations before infection. The LZM activity increased in a dose-dependent manner, while the AKP activity peaked at 500 μg/mL feeding. However, after infection, RGP-1 could affect the activities of LZM and AKP and reduce their functions significantly (P < 0.05), and these inhibitory effects followed a dose-dependent pattern. In particular, when the RGP-1 concentration was 1,000 μg/mL, the LZM and AKP activities in the treatment and negative control groups had no significant difference (P > 0.05).
Owing to their broad spectrum of therapeutic properties and relatively low toxicity, polysaccharides isolated from several species of fungi, algae, and fruits among others have attracted significant attention in biomedical research (9–11, 16, 27–29). In addition, along with the increasing emphasis on food safety, a large number of natural immunostimulants have been developed as feed additives or medicines to reduce the use of chemotherapeutic drugs in animal production (30), thereby reducing the development of drug-resistant bacteria, drug residues in organisms and other side effects caused by the excessive use of antibiotics (31). R. glutinosa polysaccharide (RGP) is a natural polysaccharide, which is the major active component in the Chinese herbal medicine species R. glutinosa, and it exhibited positive immune-enhancing and antioxidant properties in mammals, insects and fish by activating their non-specific and specific immune system (32–34). According to the reports, RGP treatment induced the maturation of natural killer (NK) cells and exerted an anticancer effect (35); RGP also could be used as a mucosal adjuvant for inducing the activation of immune responses in the lungs of mice (34). Furthermore, RGP could promote the activation of human dendritic cells by up-regulating co-stimulatory molecule expression and pro-inflammatory cytokine production (36). In addition, a dietary supplement of RGP effectively enhanced growth performance, increasing the activities of LZM, acid phosphatase (ACP), SOD, AKP and total protein (TP), up-regulating immune- (TNF-α, IL-1β, IL-8, IFN-γ) and growth-related (growth hormone, GH, insulin-like growth factor, IGF-I) genes, and reducing the mortality in Luciobarbus capita (33). However, reports on the application of RGP in common carp production are still limited. Moreover, immune regulation, antioxidant and anti-infection capacities against pathogens are often assessed while determining the effects of immunostimulants on the host (37–40). Therefore, the present study was conducted to investigate the effect of RGP-1 on the immunity, antioxidant capacity and disease resistance of common carps in vitro and in vivo.
As marker cells in the immune system that measure non-specific immune function, the proliferative and phagocytic activities of monocytes/macrophages are frequently used to determine the immunomodulatory effects of natural immunostimulants in vitro (9, 41). In this study, the proliferative and phagocytic activities of the head kidney cells increased with increasing dietary RGP-1 levels, and were higher than those in the negative control (Figures 1, 2). These results indicated that RGP-1 could improve the survival status of immune cells in the common carp and affect the non-specific immune function and response strength of the host (42), thereby guiding host inflammation and other immune processes (43, 44). Similar results were also observed in T cells (41, 45) and RAW264.7 cells (9, 46).
Macrophages are known to play a critical role in innate immunity, and attack, destroy and ingest foreign substances through the production of cytokines and NO (47), primarily because NO can activate the immune system, and can react with the superoxide anions produced by the respiratory burst of phagocytes to generate a strong oxidant-peroxynitrite (ONOOH), which can kill or inhibit the growth of several pathogenic microorganisms. Therefore, NO serves as a critical cellular defense factor in the anti-infective immunity of an organism (48, 49), and NO synthesis is an important mechanism underlying the non-specific immunity of macrophages (50). For teleosts, which rely on non-specific immune processes to enhance their immune response and resist pathogen infection (51), cytokines such as TNF-α, IL-1β, IL-6, IL-10, IL-12, and TGF-β, primarily produced by activated macrophages, also play an important role in the immune system. Once induced, they trigger a disease-resistant response of the immune system and help enhance the immune response. Therefore, NO and cytokines are frequently detected in immune responses, an observation being reported by an increasing number of immunologists (37, 52). For instance, dietary loquat leaf extract supplementation significantly upregulated immune-related genes in the intestine and improves innate immune responses (53). The Ziziphus jujube fruit extract ZJFE exhibited immune regulation potential in the skin mucosa of carps by regulating cytokine expression (54). In this study, RGP-1 treatment groups exhibited a significant increase in the production of NO and cytokines in vitro and in vivo (Figures 3, 5, Tables 2, 3). However, after infection with A. hydrophila, there was an effective reduction in the expression of NO and pro-inflammatory cytokines in vivo in the treatment groups (Tables 2, 3). Similar results were also observed for NO in mice (42), Japanese eel (Anguilla japonica) (55) and abalone (Haliotis discus hannai Ino) (56), and for cytokines in the common carp (22), yellow catfish (Pelteobagrus fulvidraco) (57), and mice (9, 46). These results suggested that RGP-1 could induce non-specific immunity by regulating the production of NO and cytokines, which promoted the immunity against pathogenic infection in common carps and improved their survival rate. However, some extracts significantly down-regulated the anti-inflammatory cytokines in the process of regulating the immune response of common carps (54), which may be attributed to the different types of extracts, dosage and treatment methods used. Furthermore, it has been reported that the common carp and A. hydrophila also contain NO. The proportion of this part NO in the entire test results and its effect on the results remain unknown; therefore, further studies are required to draw effective conclusions on this.
T-AOC, T-SOD, GSH-Px, and CAT are important antioxidant enzymes that form the first level of defense by preventing free radical formation (58), and their activities are critically connected (59). In addition, GSH and MDA are also commonly detected in antioxidant indexes (39, 60). For example, dietary Ferula (Ferula asafoetida) significantly increased antioxidant gene expression in the common carp (61), and Myrtle (Myrtus communis L., Myrtaceae) in zebrafish (62). Dose-dependent seaweed supplementation increased the plasma T-AOC as well as the GSH levels, and the CAT and SOD activities in the liver of the Atlantic salmon (Salmo salar) (63). Allium mongolicum Regel polysaccharide supplementation exerted significant protective effects against LPS challenge by preventing alterations in the SOD and GST levels in Channa argus (64). In the present study, RGP-1 treatment significantly enhanced the activities of T-SOD, CAT, and GSH-Px, increased the concentration of T-AOC and GSH, and reduced the concentration of MDA in the hepatopancreas (Figure 4). Similar results were also observed in mice (65, 66), suggesting that RGP-1 may reduce the levels of reactive oxygen free radicals and exert positive antioxidant effect on the common carp.
LZM and AKP are the key components in defense against pathogens and oxidative stress in species that lack of an adaptive immune system (67), and are the important innate immune parameters indicating the stress or disease condition (68, 69). Therefore, the LZM and AKP activities in serum were detected at the end of the experiment. Several immunostimulants (such as Thymus vulgaris essential oils, Sargassum horneri, and Psidium guajava) reportedly enhance the LZM and AKP levels in fish (70–72). For example, the inclusion of Heracleum persicum and Myrtle (Myrtus communis L., Myrtaceae) in the diet significantly elevated the LZM activity in the common carp (73) and zebrafish (62), respectively, and oral exopolysaccharide from Lactococcus lactis Z-2 significantly up-regulated the LZM and AKP activities in common carp (74). In this study, we also observed that the LZM and AKP activities in the serum of common carps fed with RGP-1 increased significantly and remained stable after infection with A. hydrophila (Table 2), which indicated that RGP-1, as an effective immune enhancer, could improve the LZM and AKP activities in the common carp.
In conclusion, RGP-1 exerts potent immunoregulatory effects at the cellular and host levels. It could improve the cellular proliferation and phagocytosis, besides inducing the release of cytokine and NO from cells, and could enhance the levels of antioxidant substances and the LZM and AKP activities in common carps after 7 days of gavage. Moreover, during the pathogen infection, RGP-1 also exerted anti-A. hydrophila effects in vivo. These results indicated that RGP-1 can be used as an immunostimulant for enhancing the immunity of common carps.
All datasets generated for this study are included in the article.
This study conformed to the guidance of animal ethical treatment for the care and use of experimental animals, and was approved by the Institutional Animal Care and Use Committee of Henan Normal University. The fishes were anesthetized with diluted MS-222 before been euthanized, and all efforts were made to minimize suffering.
JF and GN conceived and designed the experiments, prepared the manuscript. JF, ZC, and YC performed the majority of the experiments, evaluated the data. XY, XZ, and XC participated in immune related index assay. XM, YC, and XW participated in antioxidant assay. JF, GN, JZ, and CQ revised the manuscript.
This work was supported by the Innovation Scientists and Technicians Troop Construction Projects of Henan Province (Grant no. CXTD2016043); Key Technology Research Project of Henan Province (182102110022, 182102110235, 192102310138); Youth Program of National Natural Science Foundation of China (31902361, 31702358, 31902384, 31902371); Cultivation fund project of Henan Normal University (2017PL19).
The authors declare that the research was conducted in the absence of any commercial or financial relationships that could be construed as a potential conflict of interest.
1. Fisheries and Fisheries Administration Bureau of the Ministry of Agriculture. China Fishery Statistical Yearbook. Beijing: China Agriculture Press (2018).
2. Wang JL, Meng XL, Lu RH, Wu C, Luo YT, Yan X, et al. Effects of Rehmannia glutinosa on growth performance, immunological parameters and disease resistance to Aeromonas hydrophila in common carp (Cyprinus carpio L.). Aquaculture. (2015) 435:293–300. doi: 10.1016/j.aquaculture.2014.10.004
3. Yang X, Guo JL, Ye JY, Zhang YX, Wang W. The effects of Ficus carica polysaccharide on immune response and expression of some immune-related genes in grass carp, Ctenopharyngodon idella. Fish Shellfish Immun. (2015) 42:132–7. doi: 10.1016/j.fsi.2014.10.037
4. Li JL, Chen Y, Zhang L, Xing LP, Xu H, Wang YJ, et al. Total saponins of panaxnotoginseng promotes lymphangiogenesis by activation VEGF-C expression of lymphatic endothelial cells. J Ethnopharmacol. (2016) 193:293–302. doi: 10.1016/j.jep.2016.08.032
5. Wang EL, Chen X, Wang KY, Wang J, Chen DF, Geng Y, et al. Plant polysaccharides used as immunostimulants enhance innate immune response and disease resistance against Aeromonas hydrophila infection in fish. Fish Shellfish Immun. (2016) 59:196–202. doi: 10.1016/j.fsi.2016.10.039
6. Zhou W, Cheng X, Zhang Y. Effect of Liuwei Dihuang decoction, a traditional Chinese medicinal prescription, on the neuroendocrine immunomodulation network. Pharmacol Therapeut. (2016) 162:170–8. doi: 10.1016/j.pharmthera.2016.02.004
7. Du J, Chen X, Wang C, Sun HX. Pathway analysis of global gene expression change in dendritic cells induced by the polysaccharide from the roots of Actinidia eriantha. J Ethnopharmacol. (2018) 214:141–52. doi: 10.1016/j.jep.2017.12.009
8. Wang M, Yang XB, Zhao JW, Lu CJ, Zhu W. Structural characterization and macrophage immunomodulatory activity of a novel polysaccharide from Smilax glabra Roxb. Carbohyd Polym. (2017) 156:390–402. doi: 10.1016/j.carbpol.2016.09.033
9. Wang YF, Tian YQ, Shao JJ, Shu X, Jia JX, Ren XJ, et al. Macrophage immunomodulatory activity of the polysaccharide isolated from Collybia radicata mushroom. Int J Biol Macromol. (2018) 108:300–6. doi: 10.1016/j.ijbiomac.2017.12.025
10. Zhang MZ, Tian XH, Wang Y, Wang DD, Li W, Chen L, et al. Immunomodulating activity of the polysaccharide TLH-3 from Tricholomalobayense in RAW264.7 macrophages. Int J Biol Macromol. (2018) 107:2679–85. doi: 10.1016/j.ijbiomac.2017.10.165
11. Wu FF, Zhou CH, Zhou DD, Ou SY, Zhang XA, Huang HH. Structure characterization of a novel polysaccharide from Hericium erinaceus fruiting bodies and its immunomodulatory activities. Food Funct. (2018) 9:294–306. doi: 10.1039/c7fo01389b
12. Mohan K, Padmanaban AM, Uthayakumar V, Chandirasekar R, Muralisankar T, Santhanam P. Effect of dietary Ganoderma lucidum polysaccharides on biological and physiological responses of the giant freshwater prawn Macrobrachium rosenbergii. Aquaculture. (2016) 464:42–9. doi: 10.1016/j.aquaculture.2016.05.046
13. Gou CL, Wang JZ, Wang YQ, Dong WL, Shan XF, Lou YJ, et al. Hericium caput-medusae (Bull.:Fr.) Pers. polysaccharide enhance innate immune response, immune-related genes expression and disease resistance against Aeromonas hydrophila in grass carp (Ctenopharyngodon idella). Fish Shellfish Immun. (2018) 72:604–10. doi: 10.1016/j.fsi.2017.11.027
14. Rajendran P, Subramani PA, Michael D. Polysaccharides from marine macroalga, Padina gymnospora improve the nonspecific and specific immune responses of Cyprinus carpio and protect it from different pathogens. Fish Shellfish Immun. (2016) 58:220–8. doi: 10.1016/j.fsi.2016.09.016
15. Yuan CT, Pan XP, Gong Y, Xia AJ, Wu GH, Tang JQ, et al. Effects of Astragalus polysaccharides (APS) on the expression of immune response genes in head kidney, gill and spleen of the common carp, Cyprinus carpio L. Int. Immunopharmacol. (2008) 8:51–8. doi: 10.1016/j.intimp.2007.10.009
16. Hindu SV, Chandrasekaran N, Mukherjee A, Thomas J. A review on the impact of seaweed polysaccharide on the growth of probiotic bacteria and its application in aquaculture. Aquacult Internat. (2019) 27:227–38. doi: 10.1007/s10499-018-0318-3
17. Paria A, Zahra A. Effect of polysaccharides extracts of algae Ulva rigida on growth, antioxidant, immune response and resistance of shrimp, Litopenaeus vannamei against Photobacterium damselae. Aquac Res. (2018) 49:2503–10. doi: 10.1111/are.13710
18. Zhou J, Xu G, Yan JY, Li KC, Bai ZS, Cheng WN, et al. Rehmannia glutinosa (Gaertn.) DC. Polysaccharide ameliorates hyperglycemia, hyperlipemia and vascular inflammation in streptozotocin-induced diabetic mice. J Ethnopharmacol. (2015) 164:229–38. doi: 10.1016/j.jep.2015.02.026
19. Zhang ZJ, Meng YM, Guo YX, He X, Liu QR, Wang XF, et al. Rehmannia glutinosa polysaccharide induces maturation of murine bone marrow derived Dendritic cells (BMDCs). Int J Biol Macromol. (2013) 54:136–43. doi: 10.1016/j.ijbiomac.2012.12.005
20. Huang Y, Jiang CM, Hu YL, Zhao XJ, Shi C, Yu Y, et al. Immunoenhancement effect of Rehmannia glutinosa polysaccharide on lymphocyte proliferation and dendritic cell. Carbohyd Polym. (2013) 96:516–21. doi: 10.1016/j.carbpol.2013.04.018
21. Huang Y, Qin T, Huang YF, Liu ZG, Bo R, Hu YL, et al. Rehmannia glutinosa polysaccharide liposome as a novel strategy for stimulating an efficient immune response and their effects on dendritic cells. Int J Nanomed. (2016) 11:6795–808. doi: 10.2147/IJN.S119108
22. Feng JC, Chang XL, Zhang YR, Lu RH, Meng XL, Song DY, et al. Characterization of a polysaccharide HP-02 from Honeysuckle flowers and its immunoregulatory and anti-Aeromonas hydrophila effects in Cyprinus carpio L. Int J Biol Macromol. (2019) 140:477–483. doi: 10.1016/j.ijbiomac.2019.08.041
23. Wang PP, Liao WF, Fang JP, Liu Q, Yao J, Hu MH, et al. A glucan isolated from flowers of Lonicera japonica Thunb. Inhibits aggregation and neurotoxicity of Aβ42, Carbohyd Polym. (2014) 110:142–7. doi: 10.1016/j.carbpol.2014.03.060
24. Jiang J, Zhao WY, Xiong QX, Wang KY, He Y, Wang J, et al. Immune responses of channel catfish following the stimulation of three recombinant flagellins of Yersinia ruckeri in vitro and in vivo. Dev Comp Immunol. (2017) 73:61–71. doi: 10.1016/j.dci.2017.02.015
25. Geng Y, Xing L, Sun MM, Su FU. Immunomodulatory effects of sulfated polysaccharides of pine pollen on mouse macrophages. Int J Biol Macromol. (2016) 91:846–55. doi: 10.1016/j.ijbiomac.2016.06.021
26. Feng JC, Chang XL, Zhang YR, Yan X, Zhang JX, Nie GX. Effects of Lactococcus lactis from Cyprinus carpio L. as probiotics on growth performance, innate immune response and disease resistance against Aeromonas hydrophila. Fish Shellfish Immun. (2019) 93:73–81. doi: 10.1016/j.fsi.2019.07.028
27. Zhao RQ, Hu QH, Ma GX, Su AX, Xie MH, Li XF, et al. Effects of Flammulina velutipes polysaccharide on immune response and intestinal microbiota in mice. J Funct Foods. (2019) 56:255–64. doi: 10.1016/j.jff.2019.03.031
28. Wu Y, Zhu CP, Zhang Y, Li Y, Sun JR. Immunomodulatory and antioxidant effects of pomegranate peel polysaccharides on immunosuppressed mice. Int J Biol Macromol. (2019) 137:504–11. doi: 10.1016/j.ijbiomac.2019.06.139
29. Song Y, Zhu MQ, Hao HL, Deng J, Li MY, Sun YM, et al. Structure characterization of a novel polysaccharide from Chinese wild fruits (Passiflora foetida) and its immune-enhancing activity. Int J Biol Macromol. (2019) 136:324–31. doi: 10.1016/j.ijbiomac.2019.06.090
30. Hasan MT, Jang WJ, Lee JM, Lee BJ, Hur SW, Lim SG, et al. Effects of Immunostimulants, Prebiotics, Probiotics, Synbiotics, and Potentially Immunoreactive Feed Additives on Olive Flounder (Paralichthys olivaceus): a review. Rev Fish Sci Aquac. (2019) 27:417–37. doi: 10.1080/23308249.2019.1622510
31. Vieco-Saiz N, Belguesmia Y, Raspoet R, Auclair E, Gancel F, Kempf I, et al. Benefits and inputs from lactic acid bacteria and their bacteriocins as alternatives to antibiotic growth promoters during food-animal production. Front Microbiol. (2019) 10:57. doi: 10.3389/fmicb.2019.00057
32. Yuan YY, Kang NX, Lu YH, Miao XZ, Zhang X, Liu YG, et al. The effects of polysaccharides from Rehmannia glutinosa on Caenorhabditis elegans. Pharmacogn Mag. (2019) 15:385–91. doi: 10.4103/pm.pm_618_18
33. Wu C, Shan JF, Feng JC, Wang JL, Qin CB, Nie GX, et al. Effects of dietary Radix Rehmanniae Preparata polysaccharides on the growth performance, immune response and disease resistance of Luciobarbus capito. Fish Shellfsh Immunol. (2019) 89:641–6. doi: 10.1016/j.fsi.2019.04.027
34. Kwak M, Yu K, Lee PCW, Jin JO. Rehmannia glutinosa polysaccharide functions as a mucosal adjuvant to induce dendritic cell activation in mediastinal lymph node. Int J Biol Macromol. (2018) 120:1618–23. doi: 10.1016/j.ijbiomac.2018.09.187
35. Xu L, Zhang W, Zeng L, Jin JO. Rehmannia glutinosa polysaccharide induced an anti-cancer effect by activating natural killer cells. Int J Biol Macromol. (2017) 105:680–5. doi: 10.1016/j.ijbiomac.2017.07.090
36. Wang YH, Kwak M, Lee PCW, Jin JO. Rehmannia glutinosa polysaccharide promoted activation of human dendritic cells. Int J Biol Macromol. (2018) 116:232–8. doi: 10.1016/j.ijbiomac.2018.04.144
37. Habijanic J, Berovic M, Boh B, Plankl M, Wraber B. Submerged cultivation of Ganoderma lucidum and the effects of its polysaccharides on the production of human cytokines TNF-α, IL-12, IFN-γ, IL-2, IL-4, IL-10, and IL-17. New Biotechnol. (2015) 32:85–95. doi: 10.1016/j.nbt.2014.07.007
38. Inturri R, Mangano K, Santagati M, Intrieri M, Di Marco R, Blandino G. Immunomodulatory effects of Bifidobacterium longum W11 produced exopolysaccharide on cytokine production. Curr Pharm Biotechnol. (2017) 18:883–9. doi: 10.2174/1389201019666171226151551
39. Chen SJ, Yu YY, Gao YJ, Yin P, Tian LX, Niu J, et al. Exposure to acute ammonia stress influences survival, immune response and antioxidant status of pacific white shrimp (Litopenaeus vannamei) pretreated with diverse levels of inositol. Fish Shellfish Iimmunol. (2019) 89:248–56. doi: 10.1016/j.fsi.2019.03.072
40. Liu ZQ, Dong LY, Jia KY, Zhan H, Zhang ZH, Shah NP, et al. Sulfonation of Lactobacillus plantarum WLPL04 exopolysaccharide amplifies its antioxidant activities in vitro and in a Caco-2 cell model. J Dairy Sci. (2019) 102:5922–32. doi: 10.3168/jds.2018-15831
41. Teixeira D, Ishimura ME, Apostolico JD, Viel JM, Passarelli VC, Cunha-Neto E, et al. Propionibacterium acnes enhances the immunogenicity of HIVBr18 human immunodeficiency virus-1 vaccine. Front Immnol. (2018) 9:177. doi: 10.3389/fimmu.2018.00177
42. Shu Y, Liu XB, Ma XH, Gao J, He W, Cao XY, et al. Immune response mechanism of mouse monocytes/macrophages treated with κ-carrageenan polysaccharide. Environ Toxicol Phar. (2017) 53:191–8. doi: 10.1016/j.etap.2017.06.010
43. Shin MS, Hwang SH, Yoon TJ, Kim SH, Shin KS. Polysaccharides from ginseng leaves inhibit tumor metastasis via macrophage and NK cell activation. Int J Biol Macromol. (2017) 103:1327–33. doi: 10.1016/j.ijbiomac.2017.05.055
44. Martins VMR, Simões J, Ferreira I, Cruz MT, Domingues MR, Coimbra MA. In vitro macrophage nitric oxide production by Pterospartum tridentatum (L.) Willk. inflorescence polysaccharides. Carbohydr Polym. (2017) 157:176–84. doi: 10.1016/j.carbpol.2016.09.079
45. Bloem K, Garcia-Vallejo JJ, Vuist IM, Cobb BA, van Vliet SJ, van Kooyk Y. Interaction of the capsular polysacmaride A from Bacteroides fragilis with DC-SIGN on human dendritic cells is necessary for its processing and presentation to T cells. Front Immnol. (2013) 4:103. doi: 10.3389/fimmu.2013.00103
46. Xiu L, Zhang HC, Hu ZP, Liang YC, Guo S, Yang M, et al. Immunostimulatory activity of exopolysaccharides from probiotic Lactobacillus casei WXD030 strain as a novel adjuvant in vitro and in vivo. Food Agr Immunol. (2018) 29:1086–105. doi: 10.1080/09540105.2018.1513994
47. Jeong SH, Kim J, Min H. In vitro anti-inflammatory activity of the Artemisia Montana leaf ethanol extract in macrophage RAW 264.7 cells. Food Agr Immunol. (2018) 29:688–98. doi: 10.1080/09540105.2018.1439454
48. Milosevic M, Milicevic K, Bozic I, Lavrnja I, Stevanovic I, Bijelic D, et al. Immunoglobulins G from sera of amyotrophic lateral sclerosis patients induce oxidative stress and upregulation antioxidative system in BV-2 microglial cell line. Front Immnol. (2017) 8:1619. doi: 10.3389/fimmu.2017.01619
49. Castellanos-Martinez S, Gestal C. Immune parameters in the common octopus (Octopus vulgaris Cuvier, 1797) naturally infected by the gastrointestinal protozoan parasite Aggregata octopiana. Aquaculture. (2018) 497:487–93. doi: 10.1016/j.aquaculture.2018.08.022
50. Abiodun OO, Rodríguez-Nogales A, Algieri F, Gomez-Caravaca AM, Segura-Carretero A, Utrilla MP, et al. Antiinflammatory and immunomodulatory activity of an ethanolic extract from the stem bark of Terminalia catappa L. (Combretaceae): In vitro and in vivo evidences. J Ethnopharmacol. (2016) 192:309–19. doi: 10.1016/j.jep.2016.07.056
51. Sowmya R, Sachindra NM. Enhancement of non-specific immune responses in common carp, Cyprinus carpio, by dietary carotenoids obtained from shrimp exoskeleton. Aquac Res. (2015) 46:1562–72. doi: 10.1111/are.12310
52. Hu ZY, Wu BQ, Meng FH, Zhou ZJ, Lu H, Zhao H. Impact of molecular hydrogen treatments on the innate immune activity and survival of zebrafish (Danio rerio) challenged with Aeromonas hydrophila. Fish Shellfish Immunol. (2017) 67:554–60. doi: 10.1016/j.fsi.2017.05.066
53. Hoseinifar SH, Zou HK, Van Doan H, Miandare HK, Hoseini SM. Evaluation of some intestinal cytokines genes expression and serum innate immune parameters in common carp (Cyprinus carpio) fed dietary loquat (Eriobotrya japonica) leaf extract. Aquac. Res. (2017) 9:120–7. doi: 10.1111/are.13440
54. Hoseinifar SH, Zou HK, Van Doan H, Harikrishnan R, Yousefi M, Paknejad H, et al. Can dietary jujube (Ziziphus jujuba Mill.) fruit extract alter cutaneous mucosal immunity, immune related genes expression in skin and growth performance of common carp (Cyprinus carpio)? Fish Shellfish Immunol. (2019) 94:705–10. doi: 10.1016/j.fsi.2019.09.016
55. Birhanu BT, Lee JS, Lee SJ, Choi SH, Hossain MA, Park JY, et al. Immunomodulation of Lactobacillus pentosus PL11 against Edwardsiella tarda infection in the head kidney cells of the Japanese eel (Anguilla japonica). Fish Shellfish Immunol. (2016) 54:466–72. doi: 10.1016/j.fsi.2016.04.023
56. Gao XL, Zhang M, Li X, Han Y, Wu FC, Liu Y. The effects of feeding Lactobacillus pentosus on growth, immunity, and disease resistance in Haliotis discus hannai Ino. Fish Shellfish Immunol. (2018) 78:42–51. doi: 10.1016/j.fsi.2018.04.010
57. Zhu WT, Zhang YQ, Zhang JC, Yuan GL, Liu XL, Ai TS, et al. Astragalus polysaccharides, chitosan and poly(I:C) obviously enhance inactivated Edwardsiella ictaluri vaccine potency in yellow catfish Pelteobagrus fulvidraco. Fish Shellfish Immunol. (2019) 87:379–85. doi: 10.1016/j.fsi.2019.01.033
58. Wolonciej M, Milewska E, Roszkowska-Jakimiec W. Trace elements as an activator of antioxidant enzymes. Postep Hig Med Dosw. (2016) 70:1483–98. doi: 10.5604/17322693.1229074
59. Yu J, Cui PJ, Zeng WL, Xie XL, Liang WJ, Lin GB, et al. Protective effect of selenium-polysaccharides from the mycelia of Coprinus comatus on alloxan-induced oxidative stress in mice. Food Chem. (2009) 117:42–7. doi: 10.1016/j.foodchem.2009.03.073
60. Zafar N, Khan MA. Growth, feed utilization, mineralization and antioxidant response of stinging catfish Heteropneustes fossilis fed diets with different levels of manganese. Aquaculture. (2019) 509:120–8. doi: 10.1016/j.aquaculture.2019.05.022
61. Safari R, Hoseinifar SH, Nejadmoghadam S, Jafar A. Transciptomic study of mucosal immune, antioxidant and growth related genes and non-specific immune response of common carp (Cyprinus carpio) fed dietary Ferula (Ferula assafoetida). Fish Shellfish Immunol. (2016) 55:242–8. doi: 10.1016/j.fsi.2016.05.038
62. Safari R, Hoseinifar SH, Van Doan H, Dadar M. The effects of dietary Myrtle (Myrtus communis) on skin mucus immune parameters and mRNA levels of growth, antioxidant and immune related genes in zebrafish (Danio rerio). Fish Shellfish Immunol. (2017) 66:264–9. doi: 10.1016/j.fsi.2017.05.007
63. Kamunde C, Sappal R, Melegy TM. Brown seaweed (AquaArom) supplementation increases food intake and improves growth, antioxidant status and resistance to temperature stress in Atlantic salmon, Salmo salar. PLoS ONE. (2019) 14:e0219792. doi: 10.1371/journal.pone.0219792
64. Li MY, Zhu XM, Niu XT, Chen XM, Tian JX, Kong YD, et al. Effects of dietary Allium mongolicum Regel polysaccharide on growth, lipopolysaccharide-induced antioxidant responses and immune responses in Channa argus. Mol Biol Rep. (2019) 46:2221–30. doi: 10.1007/s11033-019-04677-y
65. Guo YX, Pan DD, Li H, Sun YY, Zeng XQ, Yan BX. Antioxidant and immunomodulatory activity of selenium exopolysaccharide produced by Lactococcus lactis subsp. lactis, Food Chem. (2013) 26:248–59. doi: 10.1016/j.foodchem.2012.10.029
66. Pan DD, Mei XM. Antioxidant activity of an exopolysaccharide purified from Lactococcus lactis subsp. lactis 12. Carbohyd Polym. (2010) 80:908–14. doi: 10.1016/j.carbpol.2010.01.005
67. Callewaert L, Michiels CW. Lysozymes in the animal kingdom. J Biosciences. (2010) 35:127–60. doi: 10.1007/s12038-010-0015-5
68. Abdel-Tawwab M, Abbass FE. Turmeric powder, Curcuma longa L., in common carp, Cyprinus carpio L., diets: growth performance, innate immunity, and challenge against pathogenic Aeromonas hydrophila infection. J World Aquacult Soc. (2017) 48:303–12. doi: 10.1111/jwas.12349
69. Hoseinifar SH, Zou HK, Paknejad H, Ahmadifar E, Doan HV. Non-specific immune responses and intestinal immunity of common carp (Cyprinus carpio) fed jujube (Ziziphus jujube) fruit extract. Aquac Res. (2018) 49:2995–3003. doi: 10.1111/are.13759
70. Zargar A, Rahimi-Afzal Z, Soltani E, Mirghaed AT, Ebrahimzadeh-Mousavi HA, Soltani M, et al. Growth performance, immune response and disease resistance of rainbow trout (Oncorhynchus mykiss) fed Thymus vulgaris essential oils. Aquac Res. (2019) 50:3097–106. doi: 10.1111/are.14243
71. Shi QC, Rong H, Hao ML, Zhu DS, Aweya JJ, Li SK, et al. Effects of dietary Sargassum horneri on growth performance, serum biochemical parameters, hepatic antioxidant status, and immune responses of juvenile black sea bream Acanthopagrus schlegelii. J Appl Phycol. (2019) 31:2103–13. doi: 10.1007/s10811-018-1719-4
72. Hoseinifar SH, Sohrabi A, Paknejad H, Jafari V, Paolucci M, Van Doan H. Enrichment of common carp (Cyprinus carpio) fingerlings diet with Psidium guajava: the effects on cutaneous mucosal and serum immune parameters and immune related genes expression. Fish Shellfish Immunol. (2019) 86:688–94. doi: 10.1016/j.fsi.2018.12.001
73. Hoseinifar SH, Zoheiri F, Lazado CC. Dietary phytoimmunostimulant Persian hogweed (Heracleum persicum) has more remarkable impacts on skin mucus than on serum in common carp (Cyprinus carpio). Fish Shellfish Immunol. (2016) 59:77–82. doi: 10.1016/j.fsi.2016.10.025
74. Feng JC, Cai ZL, Chen YY, Zhu HY, Chang XL, Wang XF, et al. Effects of an exopolysaccharide from Lactococcus lactis Z-2 on innate immune response, antioxidant activity, and disease resistance against Aeromonas hydrophila in Cyprinus carpio L. Fish Shellfish Immunol. (2020) 98:324–333. doi: 10.1016/j.fsi.2020.01.037
Keywords: Cyprinus carpio L, polysaccharide, Rehmannia glutinosa, immunoregulatory, antioxidant, disease resistance
Citation: Feng J, Cai Z, Zhang X, Chen Y, Chang X, Wang X, Qin C, Yan X, Ma X, Zhang J and Nie G (2020) The Effects of Oral Rehmannia glutinosa Polysaccharide Administration on Immune Responses, Antioxidant Activity and Resistance Against Aeromonas hydrophila in the Common Carp, Cyprinus carpio L. Front. Immunol. 11:904. doi: 10.3389/fimmu.2020.00904
Received: 06 January 2020; Accepted: 20 April 2020;
Published: 08 May 2020.
Edited by:
Brian Dixon, University of Waterloo, CanadaReviewed by:
Seyed Hossein Hoseinifar, Gorgan University of Agricultural Sciences and Natural Resources, IranCopyright © 2020 Feng, Cai, Zhang, Chen, Chang, Wang, Qin, Yan, Ma, Zhang and Nie. This is an open-access article distributed under the terms of the Creative Commons Attribution License (CC BY). The use, distribution or reproduction in other forums is permitted, provided the original author(s) and the copyright owner(s) are credited and that the original publication in this journal is cited, in accordance with accepted academic practice. No use, distribution or reproduction is permitted which does not comply with these terms.
*Correspondence: Jun-chang Feng, ZmpjMTUyOTAwMjJAMTI2LmNvbQ==; Guo-xing Nie, bmllZ3hAaHR1LmNu
Disclaimer: All claims expressed in this article are solely those of the authors and do not necessarily represent those of their affiliated organizations, or those of the publisher, the editors and the reviewers. Any product that may be evaluated in this article or claim that may be made by its manufacturer is not guaranteed or endorsed by the publisher.
Research integrity at Frontiers
Learn more about the work of our research integrity team to safeguard the quality of each article we publish.