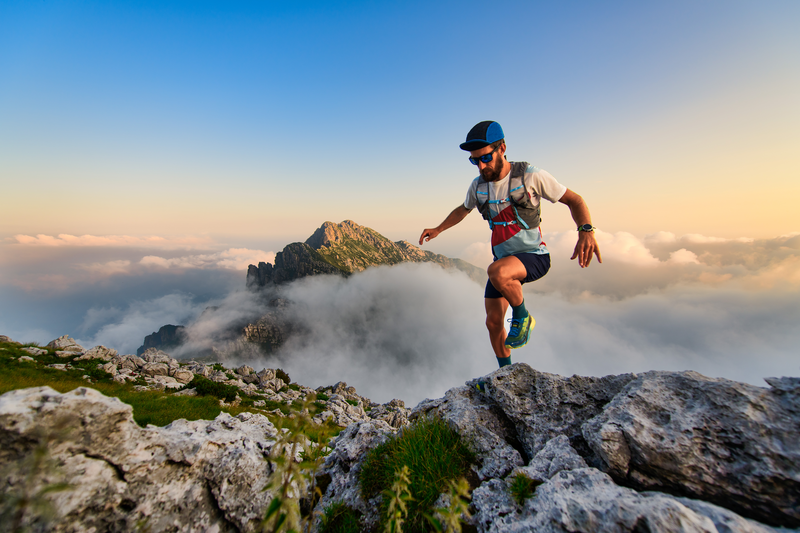
94% of researchers rate our articles as excellent or good
Learn more about the work of our research integrity team to safeguard the quality of each article we publish.
Find out more
MINI REVIEW article
Front. Immunol. , 24 March 2020
Sec. Molecular Innate Immunity
Volume 11 - 2020 | https://doi.org/10.3389/fimmu.2020.00534
This article is part of the Research Topic Hippo Signaling in the Immune System View all 7 articles
The nuclear Dbf2-related (NDR) kinases NDR1 and NDR2 belong to the NDR/LATS (large tumor suppressor) subfamily in the Hippo signaling pathway. They are highly conserved from yeast to humans. It is well-known that NDR1/2 control important cellular processes, such as morphological changes, centrosome duplication, cell proliferation, and apoptosis. Recent studies revealed that NDR1/2 also play important roles in the regulation of infection and inflammation. In this review, we summarized the roles of NDR1/2 in the modulation of inflammation induced by cytokines and innate immune response against the infection of bacteria and viruses, emphasizing on how NDR1/2 regulate signaling transduction through Hippo pathway-dependent and -independent manners.
The nuclear Dbf2-related (NDR) kinase NDR1 and NDR2 are also known as serine/threonine kinase 38 (STK38) and serine/threonine kinase 38 like (STK38L), respectively. They are two members of the NDR/LATS kinase family, a subfamily of the AGC (protein kinase A/G/C PKA/PKG/PKC-like) group of serine/threonine kinases, which are highly conserved from yeast to humans (1, 2). The first NDR serine/threonine kinase, Dbf2p, was discovered in budding yeast (3) then followed by the identification of the homologues in human cells (4). The mammalian genome encodes four members of the NDR/LATS kinase family: NDR1 (STK38), NDR2 (STK38L), LATS1 and LATS2 (1). The NDR orthologs are also found in different species: Cbk1p in Saccharomyces cerevisiae, Orb6p in Schizosaccharomyces pombe, sensory axon guidance-1 (SAX-1) in Caenorhabditis elegans, Tricornered (Trc) in Drosophila melanogaster (5). NDR1 (Stk38) mainly distributes in the nuclei. NDR2, on the other hand, is defined as a cytoplasmic kinase (4–7). In addition to a central kinase catalytic domain, NDR1 and NDR2 each has a conserved N-terminal regulatory domain (NTR) and a C-terminal hydrophobic motif (8). NDR1/2 have been regarded as protein kinases that are involved in a variety of biological processes, including morphological changes, centrosome duplication, cell cycle and apoptosis (9). Besides, studies also showed that NDR kinases are involved in embryonic development (10), neurodevelopment (11–14), and cancer biology (15, 16). Originally identified in Drosophila, the Hippo pathway is a highly conserved signaling pathway that controls organ size. The core components of the Hippo pathway in mammals include: mammalian STE20-like serine/threonine protein kinases 1 and 2 (MST1/2), the AGC serine/threonine protein kinases large tumor suppressor 1 and 2 (LAST1/2), Salvador family WW domain-containing protein 1 (SAV1), monopolar spindle-one-binder protein 1(MOB1), the transcriptional co-activator Yes-associated protein (YAP) and transcriptional co-activator with PDZ-binding motif (TAZ) (17–22). YAP/TAZ translocate to nuclei and bind to transcription factors, such as TEAD1/2/3/4, to induce the expression of target genes that control cell proliferation, survival, and migration (23–26). When Hippo pathway is activated, MST1/2 phosphorylate SAV1, MOB1, and LATS1/2. After LATS1/2 kinases get activated, they phosphorylate YAP/TAZ. This results in the cytoplasmic sequestration and degradation of YAP/TAZ, which inhibits YAP/TAZ-driven cell proliferation, survival, and migration. NDR1/2 are newly identified as members of the Hippo pathway (5, 18, 27), and were reported to play similar roles like LATS1/2 as upstream kinases of YAP (28–33). Recent studies demonstrated critical functions of the Hippo signaling in innate immunity (17, 24, 34). For example, Mst1/2 knockout mice are more susceptible to cecal ligation and puncture (CLP)-induced sepsis compared to wild-type mice (35). Emerging evidences have revealed that NDR1/2 also play pivotal roles in innate immunity. Here, we reviewed the roles of NDR1/2 in inflammation and antimicrobial innate immune response. We focused on their regulatory roles in innate immunity in Hippo pathway-dependent and -independent manners.
The innate immune system is the first line of host defense against the invasion of microbes, including bacteria, viruses and fungi. Innate immune response is initiated by the recognition of pathogen-associated molecular patterns (PAMPs) of pathogens and damage-associated molecular patterns (DAMPs) of damaged cells by pattern-recognition receptors (PRRs). The members of PRRs include Toll-like receptors (TLRs), C-type lectin receptors (CLRs), retinoic acid-inducible gene (RIG)-I-like receptors (RLRs), NOD-like receptors (NLRs) and DNA sensors. PAMPs are conserved components of pathogens, such as lipopolysaccharide (LPS), mannose, peptidoglycan (PGN), dextran, teichoic acid (LTA), nucleic acids (DNA, RNA), peptide substances (flagella, etc.), lipoproteins, etc. After PAMPs of invading microbes are recognized by PRRs, the downstream signaling pathways of PRRs are activated to elicit innate immune response, accompanied by the secretion of inflammatory cytokines and type I interferons (36, 37). CpG DNA is a typical ligand of TLR9 located on the membrane of endosomes. Our previous research demonstrated that NDR1 (Stk38) is a negative regulator of TLR9-mediated immune response in macrophages. Mechanistically, NDR1 binds with ubiquitin E3 ligase Smurf1. This interaction promotes Smurf1-mediated ubiquitination and degradation of mitogen-activated protein kinase kinase 2 (MEKK2), which is essential for CpG-induced ERK1/2 activation and subsequent production of TNF-α and IL-6. However, MEKK2 is not required for LPS-induced TNF-α and IL-6 production. Consequently, NDR1 inhibits ERK1/2 activation and decreases the production of TNF-α and IL-6 induced by CpG in macrophages. In contrast, NDR1 deficiency only slightly affects LPS-induced cytokine secretion. NDR1 deficiency also increases CpG-induced pro-inflammatory cytokine production in vivo. For instance, Stk38-deficient mice infected with Escherichia coli had been found to secrete higher levels of TNF-α, IL-6, and show a higher mortality rate than control wild-type mice. Stk38-deficiency also renders mice more susceptible to CLP-induced polymicrobial sepsis than control mice. Similarly, knockdown of NDR2 (Stk38L) with siRNA increased CpG-induced IL-6 secretion, suggesting that NDR2 is functionally similar to NDR1 in regulating the production of TLR9-mediated inflammatory cytokines. Taken together, our results showed that NDR1 prevents the excessive production of inflammatory cytokines by inhibiting TLR9-mediated innate immune response. Thus, NDR1 plays a significant role in protecting the host from TLR9-mediated inflammation (38).
A previous study suggested that NDR1 and NDR2 kinases were incorporated into HIV-1 particles. Furthermore, NDR1 and NDR2 can be cleaved by the HIV-1 protease, which inhibits the activity of NDR1/2 (39). This finding draws our attention to the connection between NDR1/2 and viral infection. MiR146a inhibits TLR signaling by targeting IRAK1, TRAF6, STAT1, and IRAK2 (40–44), which are important for antiviral immune response. A recent study showed that NDR1 acts as a transcriptional regulator by binding to the intergenic region of miR146a, which dampens miR146a transcription to promote the translation of STAT1. This takes place independently of the NDR kinase activity. STAT1 translation subsequently increases the production of type I IFN, pro-inflammatory cytokines and interferon-stimulated genes (ISGs) for the antiviral immune response. These findings revealed that NDR1 positively regulates type I and type II IFN pathways and enhances antiviral immune response (6). Glycogen synthase kinase 3β (GSK3β) and STAT1 are important participants in the antiviral immune response. GSK3β promotes IFN-induced STAT1 activation (45–47). While GSK3β inhibits NDR1 activation, NDR1 decreases the phosphorylation of GSK3β, promotes GSK3β activation and facilitates the production of type I IFNs induced by poly (I:C) (7, 48). Meanwhile, NDR2 was reported to promote RIG-I-mediated antiviral immune response by directly associating with RIG-I and TRIM25, thus facilitating the forming of RIG-I/TRIM25 complex and enhancing K63-linked polyubiquitination of RIG-I (49) (Figure 1). Overall, these findings demonstrated that NDR1/2 down-regulates TLR-mediated inflammation but positively regulates RIG-I-mediated antiviral immune response. It is unclear why NDR1 inhibits CpG-induced inflammatory cytokine production but increases virus-induced inflammatory cytokine production. It is possible that the target of NDR1, MEKK2, which promotes CpG-induced inflammatory cytokine production, plays a different role in antiviral innate immunity. As reported, MEKK2 in tumor-derived exosomes antagonizes innate antiviral immunity (50). In addition, CpG triggers TLR signal transduction and inflammatory cytokine production much more rapid than virus infection. It can't be ruled out that alteration of miR146a and STAT1 by NDR1 are not as efficient in upregulating CpG-induced inflammatory cytokine production as in upregulating virus-induced inflammatory cytokine production. The negative or positive role of NDR1 in CpG and virus induced innate immunity might be the net results of its regulation of MEKK2, STAT1, GSK3, and other unknown molecules under different conditions.
Figure 1. NDR1/2 regulate RIG-I-mediated innate immunity. RIG-I senses virus nucleic acids of viruses and activates downstream signaling pathways to initiate immune response. NDR2 directly associate with RIG-I and TRIM25, thus facilitating the formation of RIG-I/TRIM25 complex and enhancing the polyubiquitination of RIG-I. The ubiquitination of RIG-I further promotes the production of type I IFNs, so the antiviral immune response is enhanced. NDR1 promotes the activity of GSK3β. GSK3β promotes the activation of STAT1, then facilitates the expression of IFN-stimulated genes (ISGs). STAT1 is inhibited by miR146a. Binding to miR146a promoter, NDR1 inhibits NF-κB-mediated miR146a expression, and subsequently releases the inhibition of STAT1 expression by miR146a.
Infection and tissue injury are the two main causes of inflammation. In these circumstances, the immune system releases pro-inflammatory cytokines to eliminate pathogens or damaged cells and releases anti-inflammatory cytokines to balance inflammatory response, preventing immune injury. Both previous cytokines form the delicate balance of the immune system. Excessive secretion of pro-inflammatory cytokines can cause serious inflammatory diseases (51). Emerging evidences uncovered that major inflammatory cytokines tumor necrosis factor alpha (TNF-α) and interleukin 17 (IL-17) are associated with autoimmune diseases (52–55). Specifically, IL-17 participates in encephalomyelitis (EAE), rheumatoid arthritis (RA) and IBD. Moreover, IL-17 levels were found to be elevated in patients with multiple sclerosis (MS) and ulcerative colitis (UC) (56–60). NDR1 promotes TNFα-induced NF-κB activation via its kinase activity by interacting with multiple signal components in NF-κB signaling pathway. Thus, it acts as a positive regulator in TNFα-induced inflammation (61). A study from Ma C demonstrated that NDR1 promotes the pathological process of IBD and EAE in vivo by facilitating IL-17-mediated and TNF-α-mediated inflammation. NDR1 competitively binds to TRAF3, thus functions as a positive regulator of IL-17 signal transduction (62). It was reported that the suppressor of cytokine signaling 2 (SOCS2) is an E3 ligase for NDR1, and the overexpression of SOCS2 inhibits NDR1-induced TNFα-stimulated NF-κB activity (63). Nevertheless, a recent study reported that NDR2 inhibits IL-17 signaling by promoting the ubiquitination and degradation of Smurf1-mediated MEKK2. Therefore, knockdown of NDR2 enhances IL-17-induced MAPK and NF-κB activation and significantly increases IL-17-induced expression of IL-6, CXCL2, and CCL20. These results suggest that NDR2 alleviates IL-17-associated inflammation (64). In conclusion, NDR1 promotes IL-17- and TNF-α-mediated inflammation while NDR2 suppresses IL-17-associated inflammation (Figure 2). Due to the crucial roles of IL-17 and TNF-α in autoimmune diseases and the contribution of NDR1 in IL-17 signaling, NDR1 could be a potential target for drug discovery of autoimmune diseases like EAE, RA, IBD, MS, and UC.
Figure 2. NDR1/2 regulate IL-17-induced inflammatory response. NDR1 competitively binds with TRAF3 and consequently dampens TRAF3-inhibited combination between Act1and TRAF6. This results in the enhanced IL-17 signaling and the increased production of inflammatory cytokines. NDR2 promotes the ubiquitination and degradation of MEKK2 to inhibit IL-17 signaling, thus preventing the excessive secretion of inflammatory cytokines.
Taken together, we summarized the role of NDR1/2 in innate immunity by elucidating their roles in inflammation and antimicrobial immune response. Although the important roles of NDR1/2 in innate immunity have been revealed, the precise mechanism by which they regulate innate immunity are not fully illuminated. Besides that, NDR1/2 have been found to phosphorylate YAP and promote the degradation of YAP. It is reported that YAP antagonizes the antiviral innate immune response by directly binding to interferon regulatory factor 3 (IRF3) or TANK binding kinase 1 (TBK1) (65, 66). In the context of viral infection, whether NDR1/2 inhibit the production of type I interferon through YAP to enhance the antiviral immune response remains unclear. YAP impairs M2 macrophage polarization and promotes M1 macrophage activation (67). It remains unclear whether NDR1 regulates CpG-induced inflammation through modulating YAP phosphorylation and degradation. Furthermore, given the role of NDR1 in the expression and activation of STAT1 and YAP, it might be worth investigating whether NDR1 regulates macrophage polarization via phosphorylating YAP. NDR1 ablated mice are known to be more likely to develop T cell lymphoma (68). A recent study reported that NDR2 facilitates TCR-induced LFA-1 activation in T cells (69). It is intriguing to investigate the role of NDR1/2 in the adaptive immune response. Finally, both in vitro and in vivo experiments showed that NDR1/2 regulate inflammation and immune response. However, further investigation is required to ascertain the participation of NDR1/2 in human inflammation and immune response and if they could be used as therapeutic targets for immune-related diseases.
XY, HA, and YZ designed and reviewed the paper and contributed in drafting the manuscript. NO edited and reviewed the manuscript. All the authors approved the final version of the manuscript.
This study was supported by National Natural Science Foundation of China (81471537) and the Program for Professor of Special Appointment (Eastern Scholar) at Shanghai Institutions of Higher Education and Interdisciplinary Project of Clinical Immunology of Traditional Chinese Medicine in Shanghai (30304113598).
The authors declare that the research was conducted in the absence of any commercial or financial relationships that could be construed as a potential conflict of interest.
1. Cornils H, Kohler RS, Hergovich A, Hemmings BA. Downstream of human NDR kinases: impacting on c-myc and p21 protein stability to control cell cycle progression. Cell Cycle. (2011) 10:1897–904. doi: 10.4161/cc.10.12.15826
2. Hergovich A, Lamla S, Nigg EA, Hemmings BA. Centrosome-associated NDR kinase regulates centrosome duplication. Mol Cell. (2007) 25:625–34. doi: 10.1016/j.molcel.2007.01.020
3. Johnston LH, Eberly SL, Chapman JW, Araki H, Sugino A. The product of the Saccharomyces cerevisiae cell cycle gene DBF2 has homology with protein kinases and is periodically expressed in the cell cycle. Mol Cell Biol. (1990) 10:1358–66. doi: 10.1128/MCB.10.4.1358
4. Millward T, Cron P, Hemmings BA. Molecular cloning and characterization of a conserved nuclear serine (threonine) protein kinase. Proc Natl Acad Sci USA. (1995) 92:5022–6. doi: 10.1073/pnas.92.11.5022
5. Hergovich A, Stegert MR, Schmitz D, Hemmings BA. NDR kinases regulate essential cell processes from yeast to humans. Nat Rev Mol Cell Biol. (2006) 7:253–64. doi: 10.1038/nrm1891
6. Liu Z, Qin Q, Wu C, Li H, Shou J, Yang Y, et al. Downregulated NDR1 protein kinase inhibits innate immune response by initiating an miR146a-STAT1 feedback loop. Nat Commun. (2018) 9:2789. doi: 10.1038/s41467-018-05176-7
7. Xu K, Xie X, Qi G, Weng P, Hu Z, Han K, et al. Grass carp STK38 regulates IFN I expression by decreasing the phosphorylation level of GSK3beta. Dev Comp Immunol. (2019) 99:103410. doi: 10.1016/j.dci.2019.103410
8. Hergovich A. Regulation and functions of mammalian LATS/NDR kinases looking beyond canonical Hippo signaling. Cell Biosci. (2013) 3:32. doi: 10.1186/2045-3701-3-32
9. Cornils H, Kohler RS, Hergovich A, Hemmings BA. Human NDR kinases control G(1)/S cell cycle transition by directly regulating p21 stability. Mol Cell Biol. (2011) 31:1382–95. doi: 10.1128/MCB.01216-10
10. Schmitz-Rohmer D, Probst S, Yang ZZ, Laurent F, Stadler MB, Zuniga A, et al. NDR kinases are essential for somitogenesis and cardiac looping during mouse embryonic development. PLoS ONE. (2015) 10:e0136566. doi: 10.1371/journal.pone.0136566
11. Emoto K. The growing role of the Hippo-NDR kinase signalling in neuronal development disease. J Biochem. (2011) 150:133–41. doi: 10.1093/jb/mvr080
12. Du Y, Ge MM, Xue W, Yang QQ, Wang S, Xu Y, et al. Chronic lead exposure and mixed factors of genderxagexbrain regions interactions on dendrite growth, spine maturity and NDR kinase. PLoS ONE. (2015) 10:e0138112. doi: 10.1371/journal.pone.0138112
13. Demiray YE, Rehberg K, Kliche S, Stork O. Ndr2 kinase controls neurite outgrowth and dendritic branching through α 1 integrin expression. Front Mol Neurosci. (2018) 11:66. doi: 10.3389/fnmol.2018.00066
14. Leger H, Santana E, Leu NA, Smith ET, Beltran WA, Aguirre GD, et al. Ndr kinases regulate retinal interneuron proliferation and homeostasis. Sci Rep. (2018) 8:12544. doi: 10.1038/s41598-018-30492-9
15. Sharif AAD, Hergovich A. The NDR/LATS protein kinases in immunology and cancer biology. Semin Cancer Biol. (2018) 48:104–14. doi: 10.1016/j.semcancer.2017.04.010
16. Zhang L, Tang F, Terracciano L, Hynx D, Kohler R, Bichet S, et al. NDR functions as a physiological YAP1 kinase in the intestinal epithelium. Curr Biol. (2015) 25:296–305. doi: 10.1016/j.cub.2014.11.054
17. Hong L, Li X, Zhou D, Geng J, Chen L. Role of Hippo signaling in regulating immunity. Cell Mol Immunol. (2018) 15:1003–9. doi: 10.1038/s41423-018-0007-1
18. Hergovich A. The roles of NDR protein kinases in hippo signalling. Genes. (2016) 7:21. doi: 10.3390/genes7050021
19. Pearce LR, Komander D, Alessi DR. The nuts and bolts of AGC protein kinases. Nat Rev Mol Cell Biol. (2010) 11:9–22. doi: 10.1038/nrm2822
20. Avruch J, Zhou D, Fitamant J, Bardeesy N, Mou F, Barrufet LR. Protein kinases of the Hippo pathway: regulation and substrates. Semin Cell Dev Biol. (2012) 23:770–84. doi: 10.1016/j.semcdb.2012.07.002
21. Hergovich A. MOB control: reviewing a conserved family of kinase regulators. Cell Signal. (2011) 23:1433–40. doi: 10.1016/j.cellsig.2011.04.007
22. Sudol M, Harvey KF. Modularity in the Hippo signaling pathway. Trends Biochem. Sci. (2010) 35:627–33. doi: 10.1016/j.tibs.2010.05.010
23. Meng Z, Moroishi T, Guan KL. Mechanisms of Hippo pathway regulation. Genes Dev. (2016) 30:1–17. doi: 10.1101/gad.274027.115
24. Zhang Y, Zhang H, Zhao B. Hippo signaling in the immune system. Trends Biochem Sci. (2018) 43:77–80. doi: 10.1016/j.tibs.2017.11.009
25. Xu X, Jaeger ER, Wang X, Lagler-Ferrez E, Batalov S, Mathis NL, et al. Mst1 directs Myosin IIa partitioning of low and higher affinity integrins during T cell migration. PLoS ONE. (2014) 9:e105561. doi: 10.1371/journal.pone.0105561
26. Nishikimi A, Ishihara S, Ozawa M, Etoh K, Fukuda M, Kinashi T, et al. Rab13 acts downstream of the kinase Mst1 to deliver the integrin LFA-1 to the cell surface for lymphocyte trafficking. Sci Signal. (2014) 7:ra72. doi: 10.1126/scisignal.2005199
27. Manning G, Whyte DB, Martinez R, Hunter T, Sudarsanam S. The protein kinase complement of the human genome. Science. (2002) 298:1912–34. doi: 10.1126/science.1075762
28. Liu B, Zheng Y, Yin F, Yu J, Silverman N, Pan D. Toll receptor-mediated hippo signaling controls innate immunity in Drosophila. Cell. (2016) 164:406–19. doi: 10.1016/j.cell.2015.12.029
29. Du X, Yu A, Tao W. The non-canonical Hippo/Mst pathway in lymphocyte development and functions. Acta Biochimica et Biophysica Sinica. (2014) 47:60–4. doi: 10.1093/abbs/gmu112
30. Kurz ARM, Catz SD, Sperandio M. Noncanonical Hippo signalling in the regulation of leukocyte function. Trends Immunol. (2018) 39:656–69. doi: 10.1016/j.it.2018.05.003
31. Gundogdu R, Hergovich A. The possible crosstalk of MOB2 with NDR1/2 kinases in cell cycle and DNA damage signaling. J Cell Signal. (2016) 1:125. doi: 10.4172/2576-1471.1000125
32. Tang F, Gill J, Ficht X, Barthlott T, Cornils H, Schmitz-Rohmer D, et al. The kinases NDR1/2 act downstream of the Hippo homolog MST1 to mediate both egress of thymocytes from the thymus and lymphocyte motility. Sci Signal. (2015) 8:ra100. doi: 10.1126/scisignal.aab2425
33. Gundogdu R, Hergovich A. MOB (Mps one Binder) proteins in the Hippo pathway and cancer. Cells. (2019) 8:56. doi: 10.3390/cells8060569
34. Taha Z, Janse van Rensburg HJ, Yang X. The Hippo pathway: immunity and cancer. Cancers. (2018) 10:94. doi: 10.3390/cancers10040094
35. Geng J, Sun X, Wang P, Zhang S, Wang X, Wu H, et al. Kinases Mst1 and Mst2 positively regulate phagocytic induction of reactive oxygen species and bactericidal activity. Nat Immunol. (2015) 16:1142–52. doi: 10.1038/ni.3268
36. Kawai T, Akira S. The role of pattern-recognition receptors in innate immunity: update on Toll-like receptors. Nat. Immunol. (2010) 11:373–84. doi: 10.1038/ni.1863
37. Takeuchi O, Akira S. Pattern recognition receptors and inflammation. Cell. (2010) 140:805–20. doi: 10.1016/j.cell.2010.01.022
38. Wen M, Ma X, Cheng H, Jiang W, Xu X, Zhang Y, et al. Stk38 protein kinase preferentially inhibits TLR9-activated inflammatory responses by promoting MEKK2 ubiquitination in macrophages. Nat Commun. (2015) 6:7167. doi: 10.1038/ncomms8167
39. Devroe E, Silver PA, Engelman A. HIV-1 incorporates and proteolytically processes human NDR1 and NDR2 serine-threonine kinases. Virology. (2005) 331:181–9. doi: 10.1016/j.virol.2004.10.023
40. Taganov KD, Boldin MP, Chang KJ, Baltimore D. NF-kappaB-dependent induction of microRNA miR-146, an inhibitor targeted to signaling proteins of innate immune responses. Proc Natl Acad Sci USA. (2006) 103:12481–6. doi: 10.1073/pnas.0605298103
41. Hou ZH, Han QJ, Zhang C, Tian ZG, Zhang J. miR146a impairs the IFN-induced anti-HBV immune response by downregulating STAT1 in hepatocytes. Liver Int. (2014) 34:58–68. doi: 10.1111/liv.12244
42. Lu LF, Boldin MP, Chaudhry A, Lin LL, Taganov KD, Hanada T, et al. Function of miR-146a in controlling Treg cell-mediated regulation of Th1 responses. Cell. (2010) 142:914–29. doi: 10.1016/j.cell.2010.08.012
43. Tang Y, Luo X, Cui H, Ni X, Yuan M, Guo Y, et al. MicroRNA-146A contributes to abnormal activation of the type I interferon pathway in human lupus by targeting the key signaling proteins. Arthritis Rheum. (2009) 60:1065–75. doi: 10.1002/art.24436
44. Hou J, Wang P, Lin L, Liu X, Ma F, An H, et al. MicroRNA-146a feedback inhibits RIG-I-dependent Type I IFN production in macrophages by targeting TRAF6, IRAK1, and IRAK2. J. Immunol. (2009) 183:2150–8. doi: 10.4049/jimmunol.0900707
45. Tsai CC, Kai JI, Huang WC, Wang CY, Wang Y, Chen CL, et al. Glycogen synthase kinase-3beta facilitates IFN-gamma-induced STAT1 activation by regulating Src homology-2 domain-containing phosphatase 2. J. Immunol. (2009) 183:856–64. doi: 10.4049/jimmunol.0804033
46. Wang H, Brown J, Garcia CA, Tang Y, Benakanakere MR, Greenway T, et al. The role of glycogen synthase kinase 3 in regulating IFN-β-mediated IL-10 production. J. Immunol. (2011) 186:675–84. doi: 10.4049/jimmunol.1001473
47. Wang H, Garcia CA, Rehani K, Cekic C, Alard P, Kinane DF, et al. IFN-beta production by TLR4-stimulated innate immune cells is negatively regulated by GSK3-beta. J. Immunol. (2008) 181:6797–802. doi: 10.4049/jimmunol.181.10.6797
48. Enomoto A, Kido N, Ito M, Takamatsu N, Miyagawa K. Serine-threonine kinase 38 is regulated by glycogen synthase kinase-3 and modulates oxidative stress-induced cell death. Free Radic Biol Med. (2012) 52:507–15. doi: 10.1016/j.freeradbiomed.2011.11.006
49. Liu Z, Wu C, Pan Y, Liu H, Wang X, Yang Y, et al. NDR2 promotes the antiviral immune response via facilitating TRIM25-mediated RIG-I activation in macrophages. Sci Adv. (2019) 5:eaav0163. doi: 10.1126/sciadv.aav0163
50. Gao L, Wang L, Dai T, Jin K, Zhang Z, Wang S, et al. Tumor-derived exosomes antagonize innate antiviral immunity. Nat Immunol. (2018) 19:233–45. doi: 10.1038/s41590-017-0043-5
51. Palladino MA, Bahjat FR, Theodorakis EA, Moldawer LL. Anti-TNF-alpha therapies: the next generation. Nat Rev Drug Discov. (2003) 2:736–46. doi: 10.1038/nrd1175
52. Balkwill F. TNF-alpha in promotion and progression of cancer. Cancer Metastasis Rev. (2006) 25:409–16. doi: 10.1007/s10555-006-9005-3
53. Amatya N, Garg AV, Gaffen SL. IL-17 Signaling: The Yin and the Yang. Trends Immunol. (2017) 38:310–22. doi: 10.1016/j.it.2017.01.006
54. Beringer A, Noack M, Miossec P. IL-17 in chronic inflammation: from discovery to targeting. Trends Mol Med. (2016) 22:230–41. doi: 10.1016/j.molmed.2016.01.001
55. Xu S, Cao X. Interleukin-17 and its expanding biological functions. Cell Mol Immunol. (2010) 7:164–74. doi: 10.1038/cmi.2010.21
56. Park H, Li Z, Yang XO, Chang SH, Nurieva R, Wang YH, et al. A distinct lineage of CD4 T cells regulates tissue inflammation by producing interleukin 17. Nat Immunol. (2005) 6:1133–41. doi: 10.1038/ni1261
57. Li N, Wang JC, Liang TH, Zhu MH, Wang JY, Fu XL, et al. Pathologic finding of increased expression of interleukin-17 in the synovial tissue of rheumatoid arthritis patients. Int J Clin Exp Pathol. (2013) 6:1375–9.
58. Zhang Z, Zheng M, Bindas J, Schwarzenberger P, Kolls JK. Critical role of IL-17 receptor signaling in acute TNBS-induced colitis. Inflamm Bowel Dis. (2006) 12:382–8. doi: 10.1097/01.MIB.0000218764.06959.91
59. Venken K, Hellings N, Hensen K, Rummens JL, Stinissen P. Memory CD4+CD127high T cells from patients with multiple sclerosis produce IL-17 in response to myelin antigens. J Neuroimmunol. (2010) 226:185–91. doi: 10.1016/j.jneuroim.2010.05.025
60. Fonseca-Camarillo G, Mendivil EJ, Mendivil-Rangel E, Furuzawa-Carballeda J, Yamamoto-Furusho JK. Interleukin 17 gene and protein expression are increased in patients with ulcerative colitis. Inflamm Bowel Dis. (2011) 17:E135–6. doi: 10.1002/ibd.21816
61. Shi DD, Shi H, Lu D, Li R, Zhang Y, Zhang J. NDR1/STK38 potentiates NF-kappaB activation by its kinase activity. Cell Biochem Funct. (2012) 30:664–70. doi: 10.1002/cbf.2846
62. Ma C, Lin W, Liu Z, Tang W, Gautam R, Li H, et al. NDR1 protein kinase promotes IL-17- and TNF-alpha-mediated inflammation by competitively binding TRAF3. EMBO Rep. (2017) 18:586–602. doi: 10.15252/embr.201642140
63. Paul I, Batth TS, Iglesias-Gato D, Al-Araimi A, Al-Haddabi I, Alkharusi A, et al. The ubiquitin ligase Cullin5(SOCS2) regulates NDR1/STK38 stability and NF-kappaB transactivation. Sci Rep. (2017) 7:42800. doi: 10.1038/srep42800
64. Ma X, Wang D, Li N, Gao P, Zhang M, Zhang Y. Hippo kinase NDR2 inhibits IL-17 signaling by promoting Smurf1-mediated MEKK2 ubiquitination and degradation. Mol Immunol. (2019) 105:131–6. doi: 10.1016/j.molimm.2018.10.005
65. Wang S, Xie F, Chu F, Zhang Z, Yang B, Dai T, et al. YAP antagonizes innate antiviral immunity and is targeted for lysosomal degradation through IKKε-mediated phosphorylation. Nat Immunol. (2017) 18:733–43. doi: 10.1038/ni.3744
66. Zhang Q, Meng F, Chen S, Plouffe SW, Wu S, Liu S, et al. Hippo signalling governs cytosolic nucleic acid sensing through YAP/TAZ-mediated TBK1 blockade. Nat Cell Biol. (2017) 19:362–74. doi: 10.1038/ncb3496
67. Zhou X, Li W, Wang S, Zhang P, Wang Q, Xiao J, et al. YAP aggravates inflammatory bowel disease by regulating M1/M2 macrophage polarization and gut microbial homeostasis. Cell Rep. (2019) 27:1176–89.e5. doi: 10.1016/j.celrep.2019.03.028
68. Cornils H, Stegert MR, Hergovich A, Hynx D, Schmitz D, Dirnhofer S, et al. Ablation of the kinase NDR1 predisposes mice to the development of T cell lymphoma. Sci Signal. (2010) 3:ra47. doi: 10.1126/scisignal.2000681
Keywords: NDR1, NDR2, innate immunity, infection, inflammation, Hippo signaling pathway
Citation: Ye X, Ong N, An H and Zheng Y (2020) The Emerging Roles of NDR1/2 in Infection and Inflammation. Front. Immunol. 11:534. doi: 10.3389/fimmu.2020.00534
Received: 02 December 2019; Accepted: 09 March 2020;
Published: 24 March 2020.
Edited by:
Lanfen Chen, Xiamen University, ChinaReviewed by:
Chunfu Zheng, Fujian Medical University, ChinaCopyright © 2020 Ye, Ong, An and Zheng. This is an open-access article distributed under the terms of the Creative Commons Attribution License (CC BY). The use, distribution or reproduction in other forums is permitted, provided the original author(s) and the copyright owner(s) are credited and that the original publication in this journal is cited, in accordance with accepted academic practice. No use, distribution or reproduction is permitted which does not comply with these terms.
*Correspondence: Huazhang An, YW5oekBpbW11bm9sLm9yZw==; Yuejuan Zheng, MTM2NDE3NzY0MTJAMTYzLmNvbQ==
Disclaimer: All claims expressed in this article are solely those of the authors and do not necessarily represent those of their affiliated organizations, or those of the publisher, the editors and the reviewers. Any product that may be evaluated in this article or claim that may be made by its manufacturer is not guaranteed or endorsed by the publisher.
Research integrity at Frontiers
Learn more about the work of our research integrity team to safeguard the quality of each article we publish.