- 1Departamento de Microbiologia, Imunologia e Parasitologia, Escola Paulista de Medicina, Universidade Federal de São Paulo, São Paulo, Brazil
- 2Departamento de Farmacologia, Escola Paulista de Medicina, Universidade Federal de São Paulo, São Paulo, Brazil
The palladacycle complex DPPE 1.2 was previously shown to inhibit Leishmania (Leishmania) amazonensis infection in vitro and in vivo. The present study aimed to evaluate the effect of DPPE 1.2 associated with a recombinant cysteine proteinase, rLdccys1, and the adjuvant Propionibacterium acnes on L. (L.) amazonensis infection in two mouse strains, BALB/c, and C57BL/6. Treatment with this association potentiated the leishmanicidal effect of DPPE 1.2 resulting in a reduction of parasite load in both strains of mice which was higher compared to that found in groups treated with either DPPE 1.2 alone or associated with P. acnes or rLdccys1. The reduction of parasite load in both mice strains was followed by immunomodulation mediated by an increase of memory CD4+ and CD8+ T lymphocytes, IFN-γ levels and reduction of active TGF-β in treated animals. No infection relapse was observed 1 month after the end of treatment in mice which received DPPE 1.2 associated with rLdccys1 or rLdccys1 plus P. acnes in comparison to that exhibited by animals treated with DPPE 1.2 alone. Evaluation of serum levels of AST, ALT, urea, and creatinine showed no alterations among treated groups, indicating that this treatment schedule did not induce hepato or nephrotoxicity. These data indicate the potential use of this association as a therapeutic alternative for cutaneous leishmaniasis caused by L. (L) amazonensis.
Introduction
Leishmaniasis comprise a group of vector-borne diseases caused by protozoans of Leishmania genus and represent an important public health problem with a broad clinical spectrum and epidemiological diversity. The World Health Organization reports a worldwide annual incidence of 0.6–1.0 million of new cases of cutaneous leishmaniasis and 50,000–90,000 of visceral leishmaniasis with the occurrence of 20,000–30,000 deaths (1). Leishmaniasis are included in programs for the control and eradication of neglected tropical diseases.
Among several Leishmania species affecting humans, L. (L.) amazonensis is one of the causative agents of human cutaneous leishmaniasis in the Amazon region, Brazil, responsible for the development of nodular skin lesions that may evolved to diffuse form (2). Pentavalent antimonials are the first-line drug for the treatment of leishmaniasis, but the high toxicity and low efficacy in immunosuppressed patients have limited their use (3). Amphotericin B and Pentamidine are used to replace antimonials, however, problems such as high cost and toxicity have been reported (4). Miltefosine, initially developed as an antitumor drug, was effective for the treatment of cutaneous and visceral leishmaniasis, representing a promising candidate due to its oral administration, but its use is also limited by problems such as teratogenicity and parasite resistance (5–7). Therefore, it is imperative the development of new therapeutic alternatives for control of leishmaniasis and among them, the development of natural and synthetic products, molecular modifications of existing compounds and drug repositioning have shown different degrees of efficacy in the treatment of experimental leishmaniasis. Antitumor compounds have also shown activity against parasites, including palladacycle complexes that displayed trypanocidal and leishmanicidal effects (8–11). Recent findings identified immunomodulators able to increase the efficacy of leishmanicidal compounds (12, 13).
The activity of two palladacycle complexes, DPPE 1.1 and DPPE 1.2, was previously demonstrated on in vitro and in vivo L. (L.) amazonensis infection (14, 15). The leishmanicidal effect of these palladacycle complexes is followed by activation of immune responses mediated by an increase of CD4 + and CD8 +T lymphocytes in treated animals (15, 16). A significant increase in the leishmanicidal effect of DPPE 1.2 as well as of inflammatory responses was observed when this palladacycle complex was associated with heat-killed-P. acnes suspension for treatment of L. (L.) amazonensis-infected BALB/c and C57BL/6 mice (16). The adjuvant effect of P. acnes suspension is due to its ability to increase proinflammatory cytokine synthesis and modulation of Th2 to Th1 responses (17–20). DPPE 1.2 was also used in association with a recombinant cysteine proteinase from L. (L.) infantum chagasi, rLdccys1, for treatment of L. (L.) amazonensis-infected BALB/c mice. This recombinant protein cross-reacts with a cysteine proteinase of 30 kDa from L. (L.) amazonesnis amastigotes and treatment with DPPE 1.2 plus rLdccys1 resulted in a higher leishmanicidal and immunomodulatory effect of this palladacycle complex (21). This recombinant protein is implicated in Th1 lymphocyte-mediated immune responses in patients and dogs with visceral leishmaniasis (22), as well as in protective responses in mice and dogs previously immunized and challenged with L. (L.) infantum chagasi (23, 24). The efficacy of the treatment of canine visceral leishmaniasis with this recombinant protein plus P. acnes was also demonstrated (25). These findings led us to use DPPE 1.2 associated with rLdccys1 and P. acnes for the treatment of L. (L.) amazonensis-infected mice. This treatment schedule resulted in the exacerbation of the leishmanicidal effect of DPPE 1.2 followed by an increase of memory CD4+ and CD8+ T lymphocytes, IFN-γ levels and reduction of active TGF-β in treated animals.
Materials and Methods
Experimental Animals
Female BALB/c and C57BL/6 mice 6 to 8 weeks old were acquired from Universidade Federal de São Paulo (São Paulo, Brazil). Eight-week-old female Golden hamsters were obtained from Anilab Company, Paulínia (São Paulo, Brazil). All animals were maintained under specific pathogen-free conditions and fed a balanced diet. All animal procedures were carried out in accordance with the recommendations in the Guide for the Care and Use of Laboratory Animals of the Brazilian National Council of Animal Experimentation. The protocol was approved by the Committee on the Ethics of Animal Experiments of the Institutional Animal Care and Use Committee at the Federal University of São Paulo (Id # CEUA: 2957181016).
Parasites
The L. (L.) amazonensis strain used (MHOM/BR/1973/M2269) was kindly provided by Dr. Jeffrey Jon Shaw, Instituto Evandro Chagas, Belém, Pará, Brazil and maintained as amastigotes by inoculation into footpads of Golden hamsters every 4 to 6 weeks as previously described (26).
Biphosphinic Palladacycle Complex [Pd (C2, N-S(-)DMPA)(DPPE)]Cl (DPPE 1.2)
The palladacycle compound DPPE 1.2 was obtained from N,N-dimethyl-1-phenylethylamine (DMPA), complexed to 1,2-ethane-bis(diphenylphosphine; DPPE) ligand and synthesized as previously described (27). Stock solutions at 1.45 mM were prepared in phosphate-buffered saline (PBS; 137 mM NaCl in 10 mM phosphate buffer, pH 7.4) after solubilization in dimethylsulfoxide (final concentration of 0.1%).
Heat-Killed P. acnes Suspension
Propionibacterium acnes was obtained from Instituto Adolfo Lutz, São Paulo, S.P., Brazil and cultured in anaerobic medium (Hemobac, Probac, São Paulo, S.P., Brazil) for 3 days at 37°C and washed by centrifugation (28). The resulting pellets were suspended in 0.9% saline and subjected to continuous water vapor for 20 min at 120°C. The protein concentration of the suspension was determined by the Bradford method (29).
Expression and Purification of Recombinant Cysteine Proteinase (rLdccys1)
The PCR product corresponding to the open reading frame (ORF) of the L. (L.) infantum chagasi Ldccys1 gene previously obtained in our laboratory (30) was subcloned into the BamHI and EcoRI restriction sites of the parallel pHIS 3 expression vector containing a tail of six histidine residues in the amino-terminal region (31). Recombinant plasmids were used to transform Escherichia coli BL21 (DE3). Protein expression was performed by inoculating 25 ml of a bacterial culture into 500 ml LB medium containing 100 μg/ml ampicillin. The suspension was kept on a shaker at 37°C until reaching the log phase (absorbance at 600 nm = 0.6) and protein expression was induced with 1 mM IPTG for a further 3 h at 37°C. After growth, the recombinant bacteria was pelleted at 4,000 × g for 10 min and the recombinant antigen was purified from insoluble inclusion bodies treated with solubilization buffer (0.1 M NaH2PO4, 10 mM Tris–HCl, pH 8.0, 8M urea). Purification of the recombinant protein was performed by affinity chromatography using Ni-NTA Superflow Agarose Matrix (QIAGEN) as previously described (32). Purified protein was analyzed by SDS-PAGE and Western blotting using mouse serum anti-rLdccys1. Cross-reactivity of rLdccys1 and a 30 kDa cysteine proteinase from L. (L.) amazonensis was demonstrated in amastigote extracts of this parasite subjected to SDS-PAGE and Western blotting (21).
In vivo Antileishmanial Assays
In vivo leishmanicidal activity of DPPE 1.2 associated with rLdccys1 plus P. acnes was evaluated in female BALB/c and C57BL/6 mice infected subcutaneously at the right hind-foot pad with 1 × 105 L. (L.) amazonensis amastigotes freshly isolated from infected Golden hamsters as previously described (14). Twenty days after infection, the animals were separated into eight groups of 10 mice each. Treated animals received in the foot lesions every other day 21 doses of 160 μg/Kg of DPPE 1.2 (total of 3.36 mg/Kg) associated or not with 50 μg/animal of rLdccys1 and 100 μg/animal of P. acnes. The recombinant antigen and the adjuvant were administered on the 1st, 7th, and 14th days of treatment (total of 150 and 300 μg/animal, respectively). The infection was monitored once a week by measuring the diameter of foot lesions with a dial caliper (Mitutoyo Corp., Japan). Parasite burden was evaluated by limiting dilution in foot lesions of BALB/c and C57BL/6 mice after the end of the treatment, as previously described (33). Treatment longevity was evaluated in the remaining animals 30 days after the end of treatment. During this period, the groups treated with DPPE 1.2 associated with either the recombinant protein, P. acnes or both received once a week additional doses of either 50 μg of rLdccys1, 100 μg of P. acnes or 50 μg of rLdccys1 plus 100 μg of P. acnes.
Evaluation of Immune Responses
T lymphocyte populations were evaluated by flow cytometry. Popliteal and inguinal lymph nodes were isolated from BALB/c and C57BL/6 mice. After staining with Trypan Blue and counting in a Neubauer chamber, 1 × 106 lymphocytes were washed twice in PBS and incubated with monoclonal antibodies either anti-CD3 conjugated to allophycocyanin (APC), anti-CD4 conjugated to phycoerythrin cyanine 5 (PECy5), anti-CD8 conjugated to pacific blue (PB), anti-CD44 conjugated to phycoerythrin cyanine 7 (PeCy7) (Pharmingen) or anti-CD62L conjugated to phycoerythrin (PE) (Pharmingen). After incubation for 30 min at 4°C, the cells were washed in PBS and fixed in formalin 1%. Fluorescence intensity was analyzed by FACS (FACSCAN – Cell Sorter Becton–Dickinson). Detection of IFN-γ and active TGF-β was carried out in the supernatants of foot lesions from treated BALB/c and C57BL/6 mice. The animals were euthanized, and after homogenization of excised foot lesions in PBS, supernatants were collected, cleared by centrifugation and assayed for these cytokines using a double-sandwich ELISA assay according to the manufacturer instructions (eBioscience, Inc., San Diego, CA, United States). Supernatant concentrations higher than the minimal values obtained from standard IFN-γ and TGF-β were considered positive.
For evaluation of proliferation in response to rLdccys1, lymphocytes isolated from popliteal and inguinal lymph nodes were stained with 1.5 μM of carboxyfluorescein succinimidyl ester (CFSE) and cultured (5 × 105 cells/well) with 5 μg/ml of rLdccys1 peptides or medium (R10) alone for 3 days under an atmosphere of 5% CO2 at 37°C. Cells were then collected and labeled for 30 min at 4°C with anti-CD3 conjugated to allophycocyanin (APC), anti-CD4 conjugated to phycoerythrin cyanine 5 (PECy5), anti-CD8 conjugated to pacific blue (PB), anti-CD44 conjugated to phycoerythrin cyanine 7 (PeCy7) (Pharmingen) and anti-CD62L conjugated to phycoerythrin (PE) (Pharmingen). After washing, samples were analyzed using a FACSCantoTM II (BD) with appropriate compensation controls (single stained beads, CompBeads (BD), or CFSE-single stained cells). The frequency of proliferating T lymphocyte subpopulations (CFSElow) among populations was determined using FlowJo software, version 9.7.6 (Tree Star). The rLdccys1 specific population was determined by estimating the number of rLdccys1-stimulated CFSElow cells subtracted from numbers of unstimulated lymphocytes.
Toxicity Assays
Serum concentrations of urea, creatinine, and transaminases were determined in BALB/c and C57BL/6 mice at the end of treatment, using sets of commercial reagents (Labtest, Ltda, Brazil).
Statistical Analysis
The data were analyzed using GraphPad Version 8.0.0 software, applying one-way ANOVA and Tukey’s multiple comparison test (P < 0.001).
Results
Association With rLdccys1 Plus P. acnes Increased the Leishmanicidal Effect of DPPE 1.2 in L. (L.) amazonensis-Infected BALB/c and C57BL6 Mice
The treatment with DPPE 1.2 associated with rLdccys1 plus P. acnes, hereafter referred as triple association, was performed by administration of 3.36 mg/Kg of DPPE 1.2, 150 mg/animal of rLdccys1 and 300 mg/animal of P. acnes. The parasite load of BALB/c mice evaluated 3 (phase 1) and 30 days after the end of treatment (phase 2) was 14 and 19 times, respectively, higher than that found in C57BL/6 mice. In both mouse strains, there were no significant differences between the parasite load of animals treated with either PBS or P. acnes. The other treated groups from both strains evaluated in phase 1 showed a significant reduction of parasite load compared to controls. BALB/c and C57BL/6 mice treated with the triple association displayed a parasite reduction of 1935 and 1265 times, respectively, compared to the parasite load found in controls. Although the parasite load from the group treated with the triple association was lower than that observed in the other treated groups, this difference was not statistically significant (Figure 1). Control groups, as well as those treated either with DPPE 1.2 alone or DPPE 1.2 plus P. acnes from both mouse strains, displayed an increase of parasite load in phase 2. On the other hand, all groups treated with either rLdccys1 alone or associated with DPPE 1.2, P. acnes or both showed a parasite load very similar to that evaluated in phase 1 (Table 1).
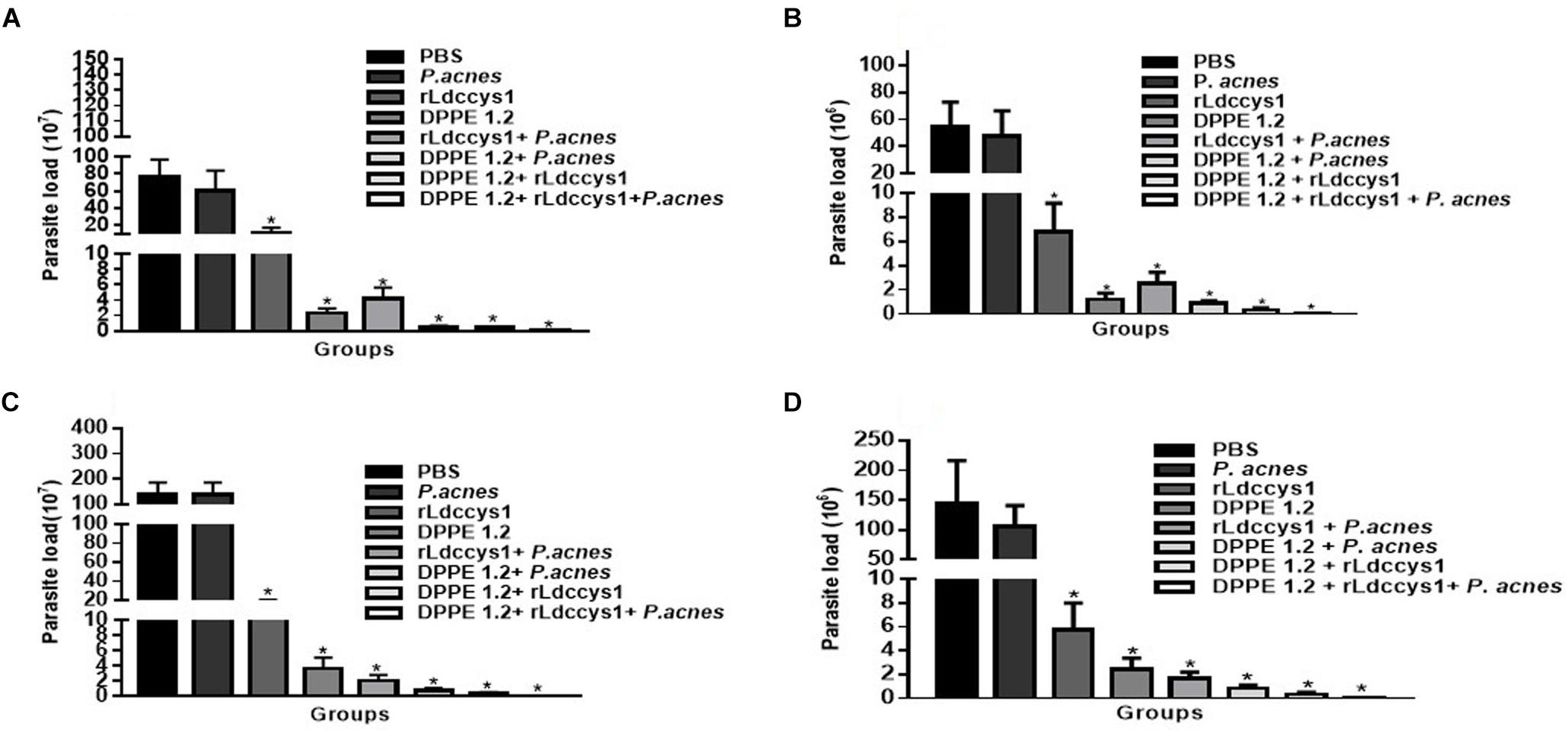
Figure 1. Effect of DPPE 1.2 associated with rLdccys1 plus P. acnes on L. (L.) amazonensis-infected BALB/c (A,C) and C57BL/6 mice (B,D). Parasite load in foot lesions evaluated 3 days (Phase 1) (A,B) and 30 days after the end of treatment (Phase 2) (C,D). ∗P < 0.001. Data are representative of three independent experiments.
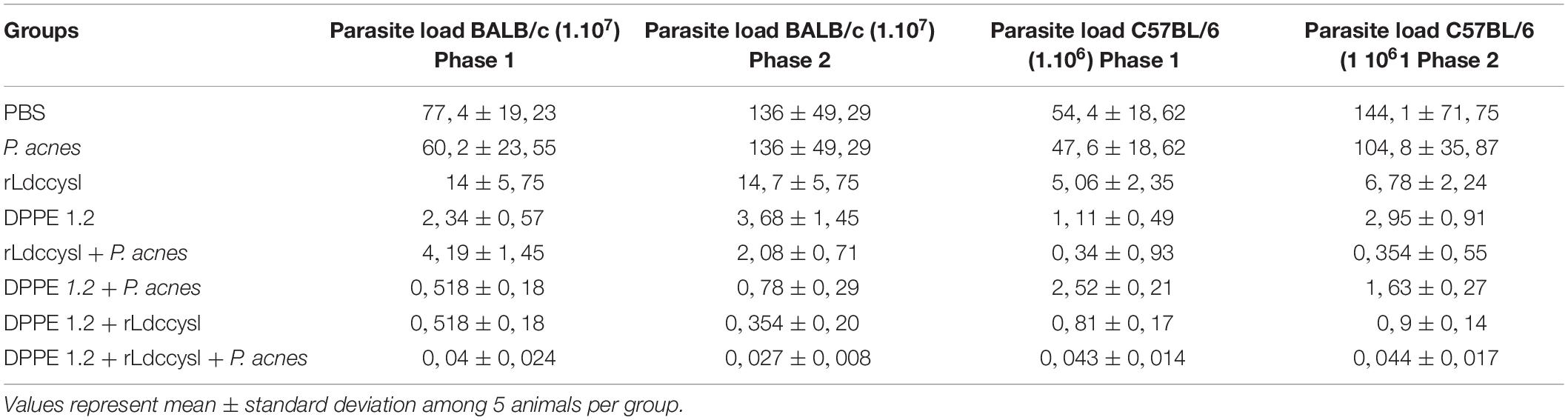
Table 1. Parasite load in foot lesions of L. (L.) amazonensis-infected BALB/c and C57BL/6 mice treated with DPPE 1.2 associated with rLdccys1 plus P. acnes evaluated in phases 1 and 2.
Determination of hepato and nephrotoxicity of treated animals by detection of serum levels of transaminases, urea and creatinine showed no statistically significant alterations among controls and treated groups (Figures 2, 3).
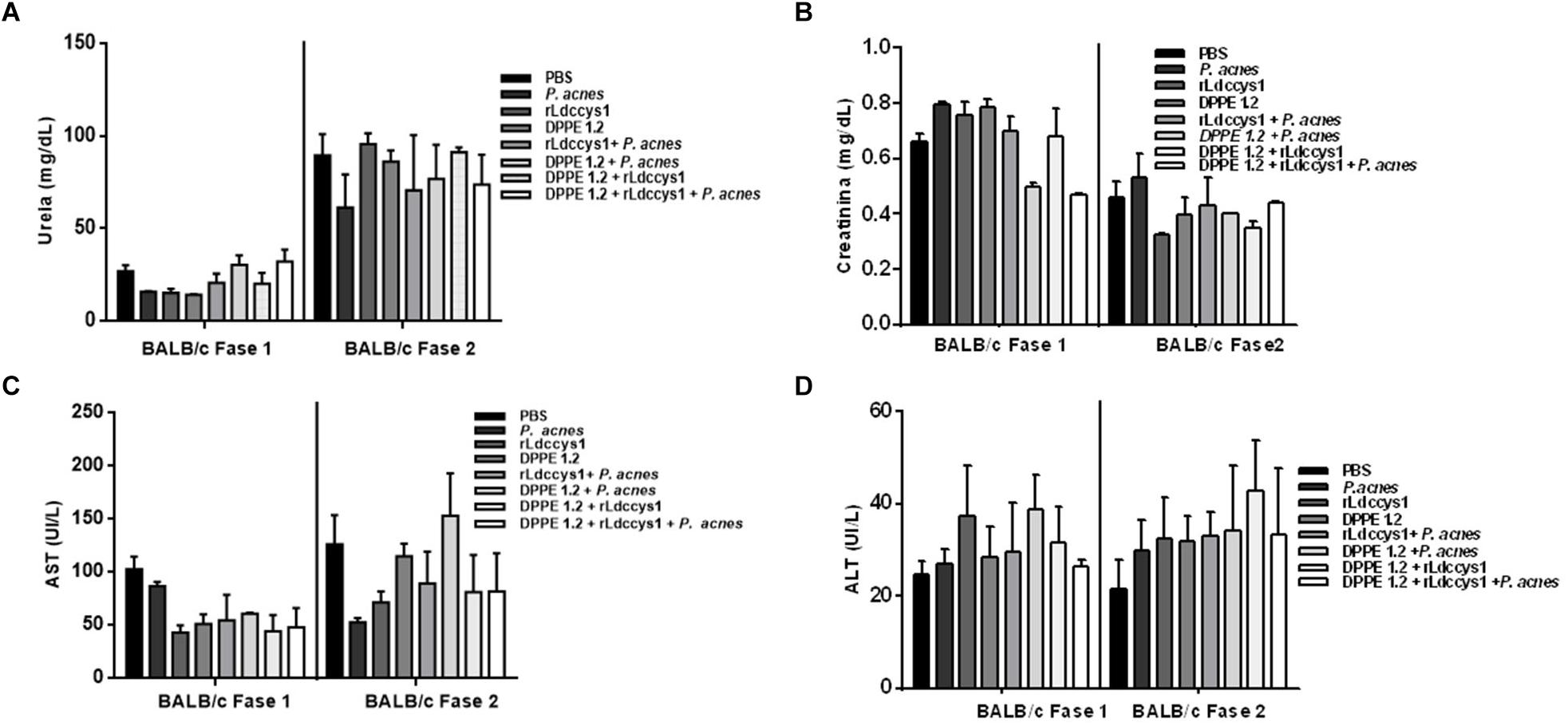
Figure 2. Evaluation of toxicity in L. (L.) amazonensis-infected BALB/c mice after treatment with DPPE 1.2 associated with rLdccys1 plus P. acnes. Serum concentrations of urea (A), creatinine (B), AST (C), and ALT (D) in phases 1 and 2. The reference values are: urea: 42–55 mg/dL; creatinine: 0,44–0,55 mg/dL; AST: 70–100 UI/L; ALT: 30–35 UI/L.
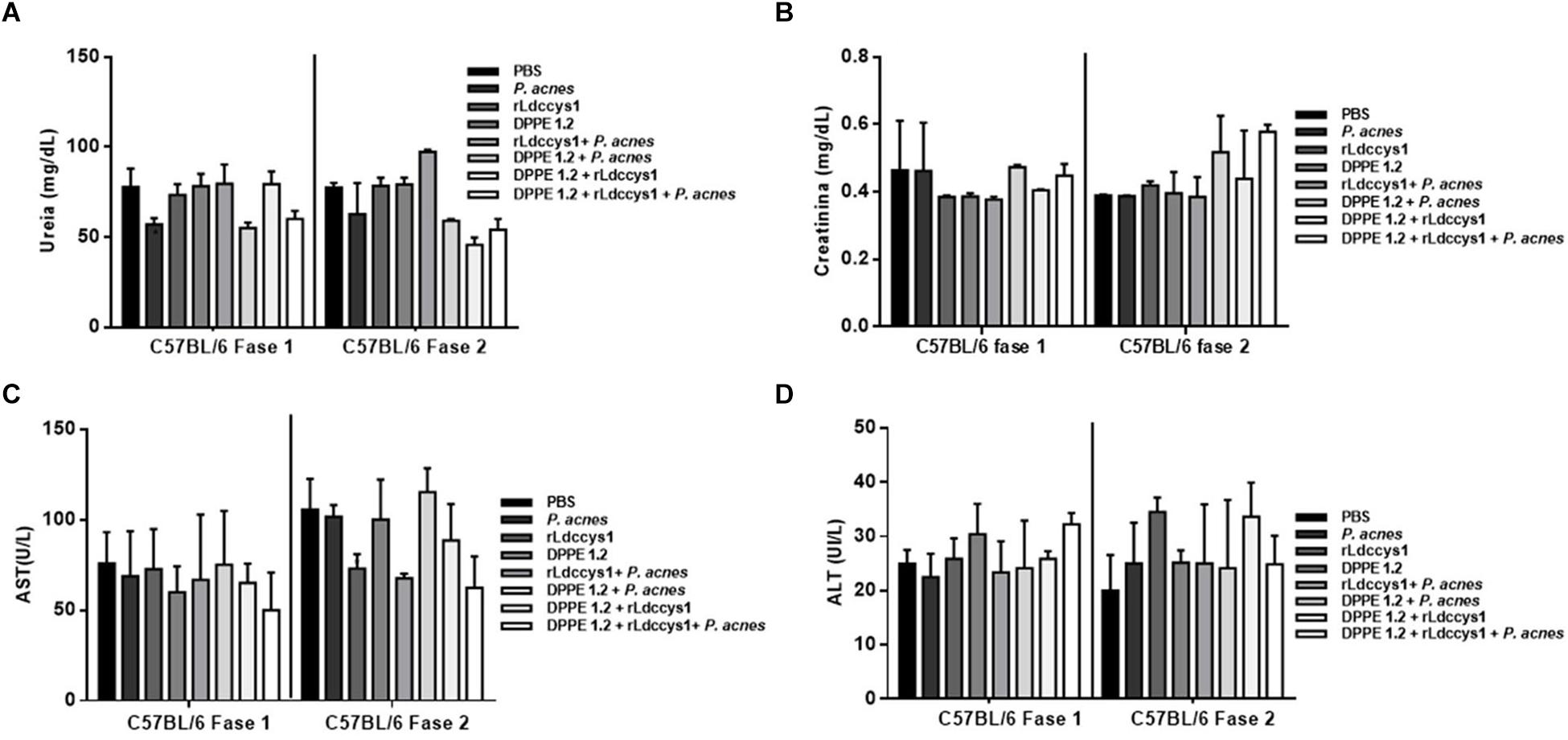
Figure 3. Evaluation of toxicity in L. (L.) amazonensis-infected C57BL/6 mice after treatment with DPPE 1.2 associated with rLdccys1 plus P. acnes. Serum concentrations of urea (A), creatinine (B), AST (C), and ALT (D) in phases 1 and 2. The reference values are: urea: 42–55 mg/dL; creatinine: 0,44–0,55 mg/dL; AST: 70–100 UI/L; ALT: 30–35 UI/L.
Increase of CD4+ CD8+ and Memory T lymphocytes in L. (L.) amazonensis-Infected BALB/c and C57BL/6 Mice After Treatment With DPPE 1.2 Associated With rLdccys1 Plus P. acnes
Data on analysis of CD4+ and CD8+ T lymphocyte expression are shown in Figures 4, 5, and Supplementary Figures S1–S5, respectively. There was an increase of CD4+ T lymphocytes in some groups of treated BALB/c mice compared to controls and this increase was significantly higher in those treated with the triple association in both evaluation phases. Central memory CD4+ T lymphocytes increased only in BALB/c mice treated with the triple association in the two evaluation phases, while in this group memory effectors increased significantly only in phase 2 (Figures 4A,C).
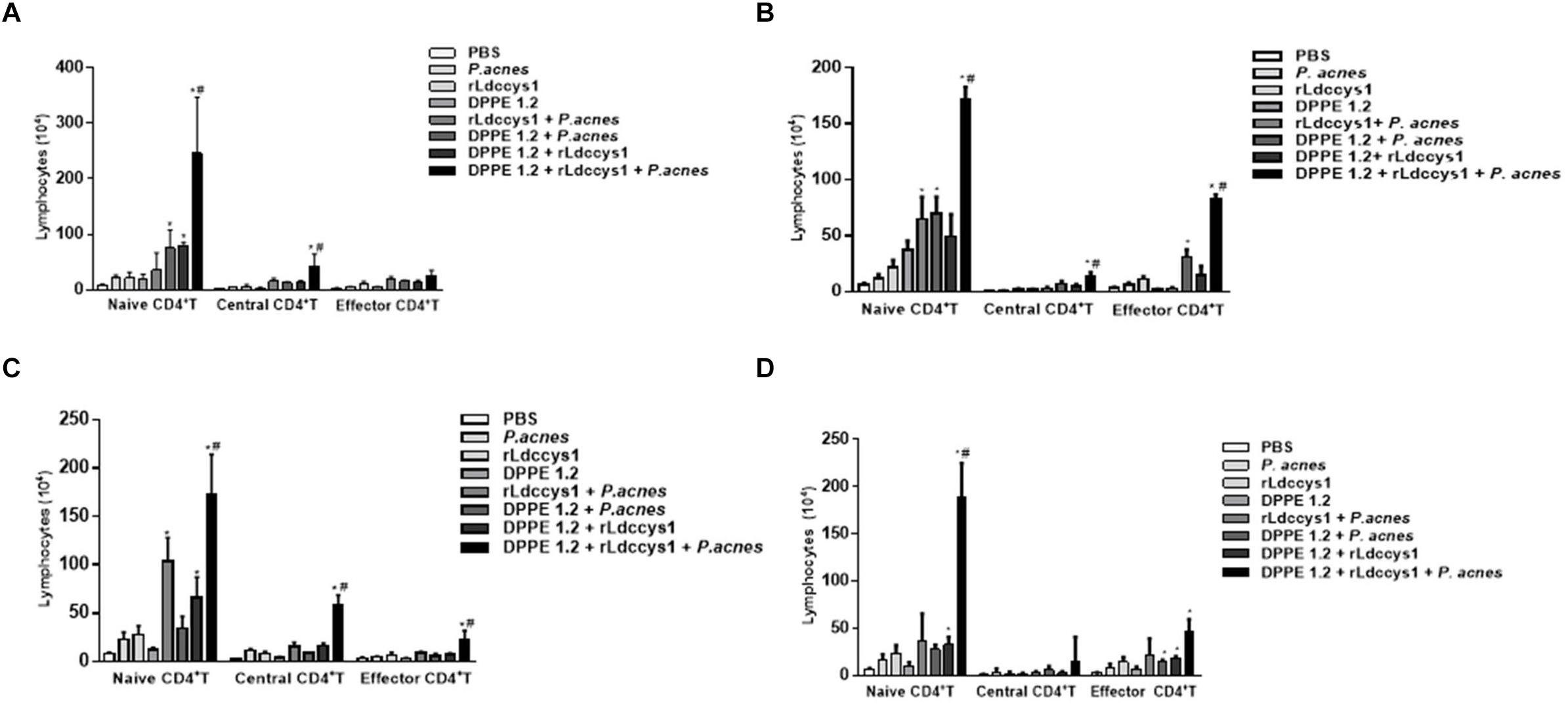
Figure 4. Analysis of central and effector memory CD4+ T lymphocytes in L. (L.) amazonensis-infected BALB/c (A,C) and C57BL/6 (B,D) mice treated with DPPE 1.2 associated with rLdccys1 plus P. acnes evaluated in phases 1 (A,B) and 2 (C,D). ∗P < 0.001 compared to control; #P < 0.001 compared to the other treated groups.
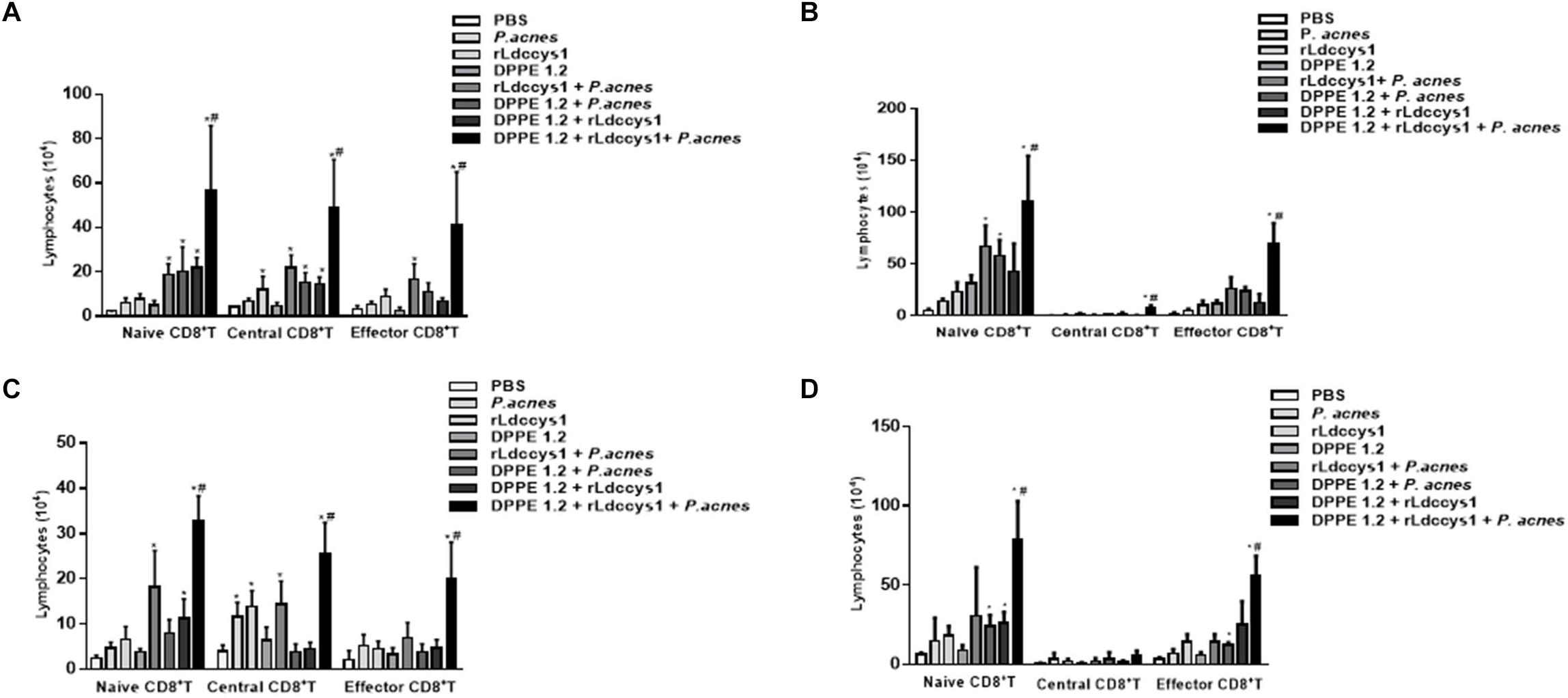
Figure 5. Analysis of central and effector memory CD8+ T lymphocytes in L. (L.) amazonensis-infected BALB/c (A,C) and C57BL 6 (B,D) mice treated with DPPE 1.2 associated with rLdccys1 plus P. acnes in phases 1 (A,B) and 2 (C,D). ∗P < 0.001 compared to control groups; #P < 0.001 compared to the other treated groups.
Some groups of treated C57BL/6 mice displayed an increase of CD4+ T lymphocytes compared to control groups and this increase was significantly higher in those treated with the triple association in both phases of evaluation. There was an increase of central memory CD4+ T lymphocytes during phase 1 in the group treated with the triple association, whereas this group displayed a significant increase of effector memory CD4+ T lymphocytes in both evaluation phases (Figures 4B,D).
Regarding CD8+ T lymphocytes, although there was an increase of this population in some groups of treated BALB/c mice, this increase was significantly higher in those treated with the triple association in both phases of parasite evaluation. The central and effector memory CD8+ T lymphocytes also increased only in the group treated with the triple association in both evaluation phases (Figures 5A,C).
In C57BL/6 strain there was a significant increase of CD8+ T population in some of the treated groups which was significantly higher in those treated with the triple association. A significant increase of central memory CD8+ T lymphocytes was observed in the group treated with the triple association during phase 1, whereas effector memory CD8+ T lymphocytes increased in both phases of evaluation (Figures 5B,D). It was also demonstrated that in phase 2 the C57BL/6 strain displayed a higher number of effector memory CD8+ T lymphocytes compared to that found in BALB/c mice (Figures 5C,D).
Lymphoproliferative Responses to rLdccys1 From L. (L.) amazonensis-Infected BALB/c Mice Treated With DPPE 1.2 Associated With rLdccys1 Plus P. acnes Resulted in an Increase of CD4+, CD8+, and Memory T Lymphocytes
Proliferative responses were evaluated in lymphocytes isolated from inguinal and popliteal lymph nodes of L. (L.) amazonensis-infected BALB/c mice 30 days after treatment with DPPE 1.2 associated with rLdccys1 plus P. acnes by stimulation in vitro with rLdccys1. These data are shown in Figure 6 and Supplementary Figures S6, S7. Cells from animal group treated with the triple association measured 3 days after rLdccys1 stimulation resulted in a significant increase of CD4+ T and central memory CD4+ T lymphocytes while increase of effector memory CD4+ T was observed in cell cultures from groups treated with rLdccys1 plus either P. acnes or DPPE 1.2, besides from that treated with DPPE 1.2 plus P. acnes, but this increase was significantly higher in lymphocytes from mice treated with the triple association (Figure 6A).
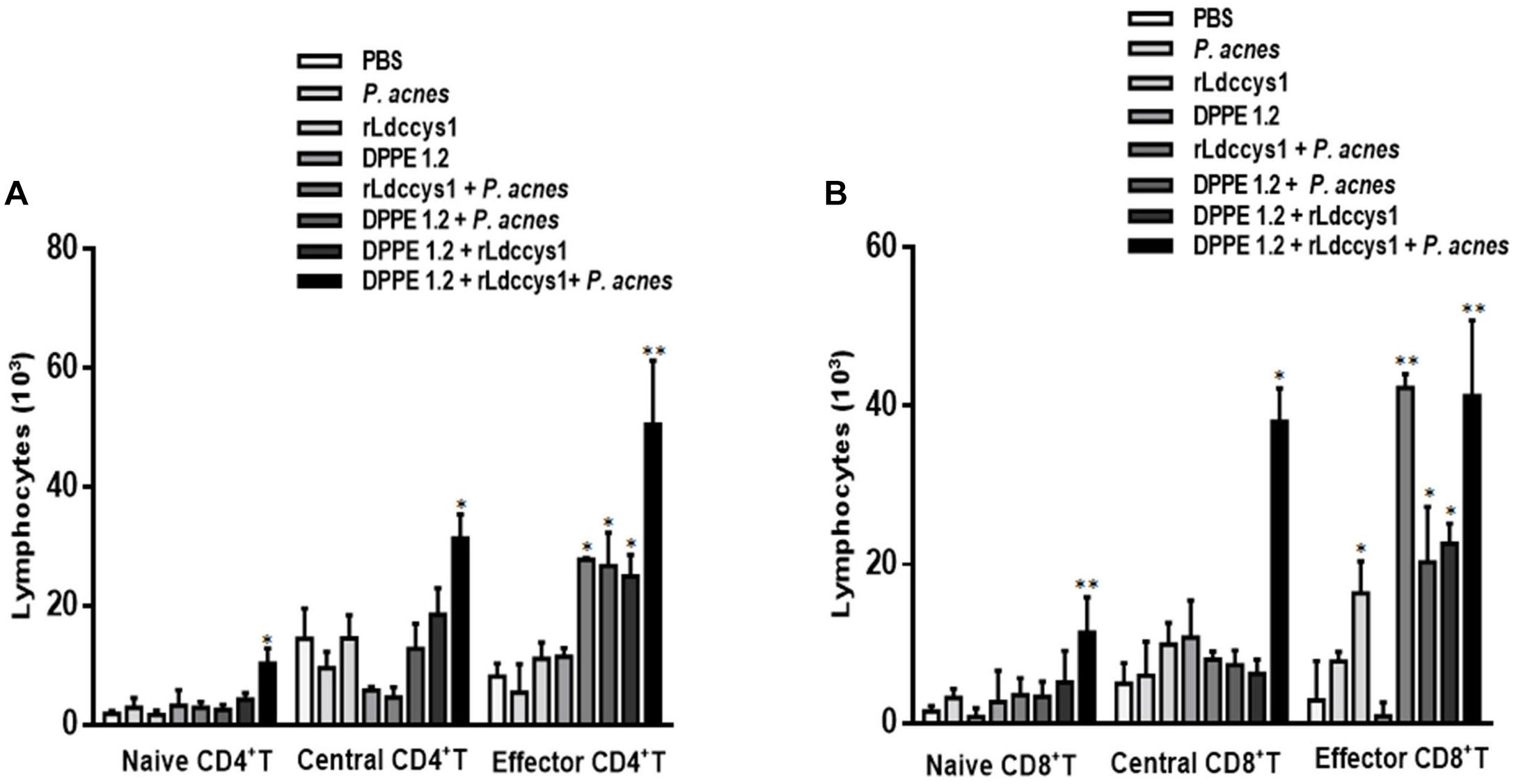
Figure 6. Proliferative responses to rLdccys1 of lymphocytes isolated from inguinal and popliteal lymph nodes of L. (L.) amazonensis-infected BALB/c mice 1 month after the end of treatment with DPPE 1.2 associated with rLdccys1 plus P. acnes. Data are representative of two experiments and were defined by CFSE dilution in gated CD3+CD4+ and CD3+CD8+ cells by flow cytometry. Memory T population was defined in gated CD44low CD62Lhigh for naive, CD44high CD62Lhigh for central and CD44high CD62Llowfor effectors. Results are presented as values (mean ± SD) for each group. ∗P < 0.001.
The proliferative responses under rLdccys1 stimulation increased CD8+ T and central CD8+ T lymphocytes from the group treated with the triple association. Although there was also proliferation of effector CD8+ T lymphocytes stimulated with rLdccys1 in groups treated with either rLdccys1 alone or associated with DPPE 1.2, as well as with DPPE 1.2 plus P. acnes, these lymphoproliferative responses were significantly higher in groups treated with either rLdccys1 plus P. acnes or the triple association (Figure 6B).
Treatment of L. (L.) amazonensis-Infected BALB/c and C57BL/6 Mice With DPPE 1.2 Associated With rLdccys1 Plus P. acnes Resulted in an Increase of IFN-γ and Reduction of TGF-β Secretion
Treated groups from BALB/c and C57BL/6 mice, in both evaluation phases, displayed high levels of IFN-γ in foot lesions compared to controls and in mice treated with the triple association IFN-γ secretion was significantly higher compared to that secreted by the other treated groups (Figure 7). It was also observed that C57BL/6 mice treated with DPPE 1.2 plus P. acnes exhibited levels of IFN-γ comparable to those found in the group treated with the triple association (Figure 7D).
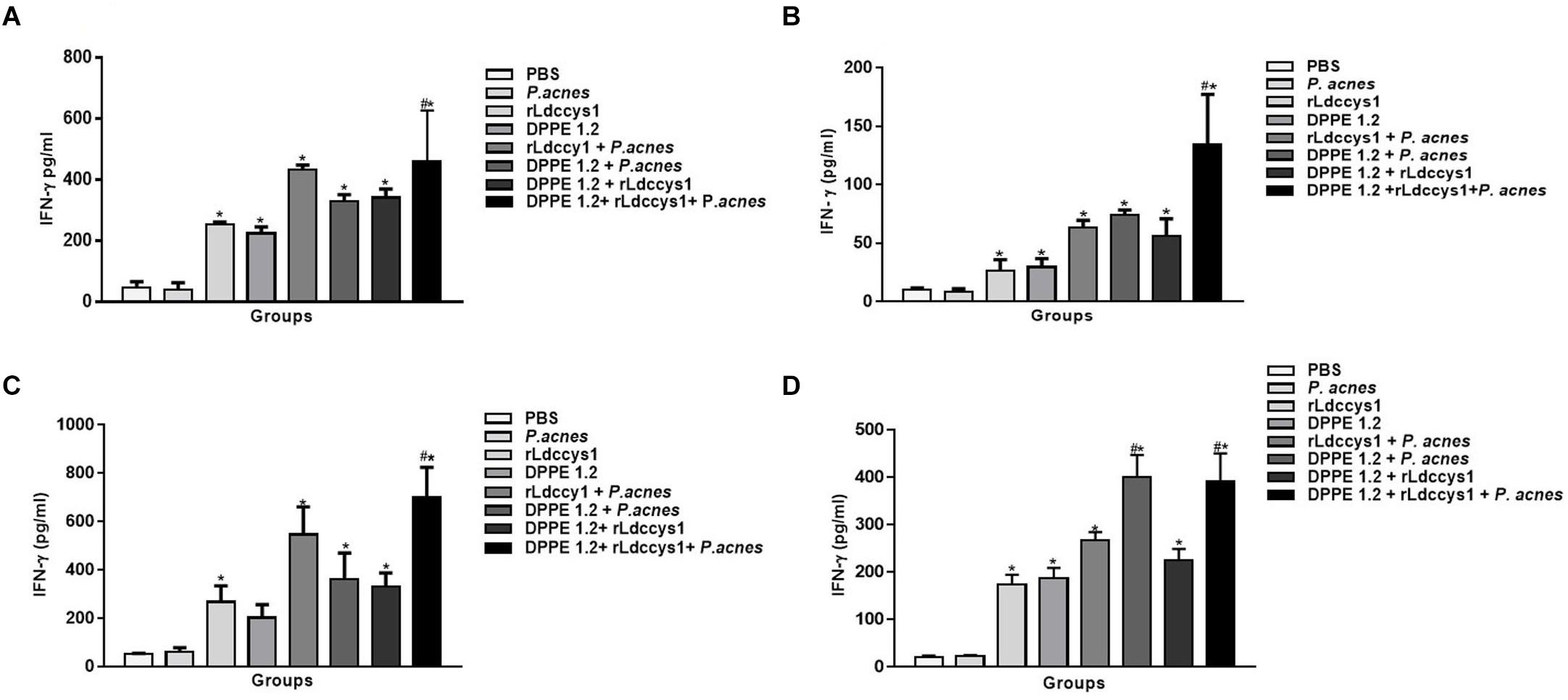
Figure 7. Secretion of IFN-γ in treated mice. Levels of IFN-γ were determined by ELISA assay in supernatants of foot lesions from L. (L.) amazonensis-infected BALB/c (A,C) and C57BL/6 (B,D) mice treated with DPPE 1.2 associated with rLdccys1 plus P. acnes in phases 1 (A,B) and 2 (C,D). ∗P < 0.001 compared to control groups; #P < 0.001 compared to the other treated groups.
As can be seen in Figure 8 there was a significant decrease of TGF-β secretion in treated animals from both mouse strains in the two evaluation phases compared to that detected in controls. Although the reduction of TGF-β was more pronounced in groups treated with the tripled association in comparison to the other treated groups, this difference was not statistically significant. It was also noted that in both evaluation phases TGF-β levels were higher in all groups of BALB/c mice compared to those found in C57BL/6 mice.
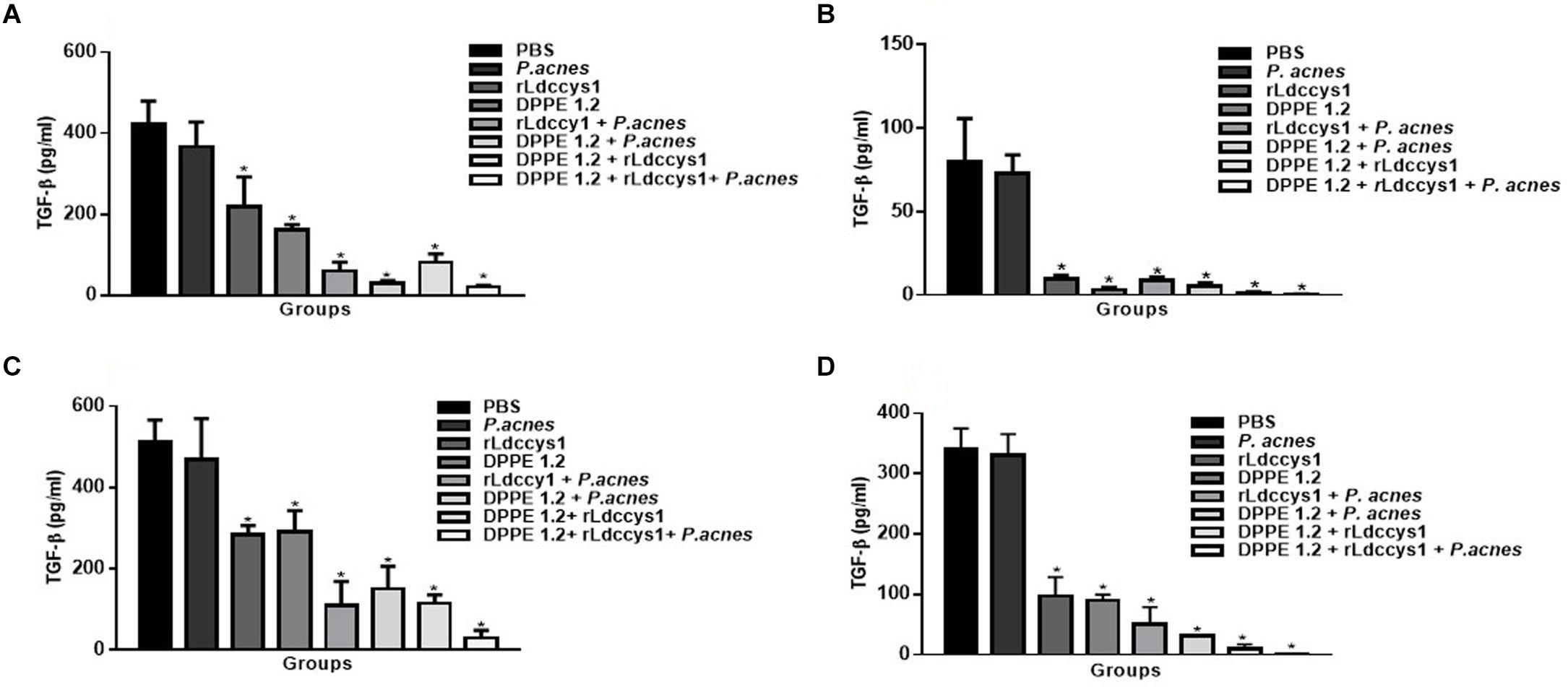
Figure 8. Evaluation of active TGF-β in treated mice. Levels of active TGF-β were determined by ELISA assay in supernatants of foot lesions from L. (L.) amazonensis-infected BALB/c (A,C) and C57BL/6 mice (B,D) treated with DPPE 1.2 associated with rLdccys1 plus P. acnes in phases 1 (A,B) and 2 (C,D). ∗P < 0.001 compared to control groups.
Discussion
In the present study, the potentiation of the leishmanicidal effect of DPPE 1.2 was demonstrated in L. (L.) amazonensis-infected BALB/c and C57BL/6 mice after treatment with this palladacycle complex associated with rLdccys1 plus P. acnes. The reduction of parasite load in treated groups was followed by immunomodulation evidenced in these animals by the significant increase of CD4+ and CD8+ T lymphocytes and IFN-γ levels and a concomitant reduction of active TGF-β. Increase of CD4 + T lymphocytes, as well as IFN-γ production in groups treated with P. acnes associated with either DPPE 1.2, rLdccys1 or both corroborate previous data showing that the treatment with this adjuvant induces immune responses mediated by Th1 lymphocytes with IFN-γ production (17). In experimental leishmaniasis, P. acnes was used as an adjuvant with native and recombinant Leishmania antigens for mice vaccination that resulted in protective immunity mediated by CD4+ Th1 lymphocytes (23, 34). More recently, the potentiation of the leishmanicidal effect of DPPE 1.2, as well as immune responses mediated by CD4+ T lymphocytes with increased IFN-γ production, was demonstrated in mice treated with P. acnes associated with DPPE 1.2 (16). The treatment with rLdccys1 alone or associated with P. acnes, DPPE 1.2, or both led to IFN-γ production, corroborating our previous findings which demonstrated a predominance of inflammatory responses in mice immunized with rLdccys1, as well as in L. (L.) infantum chagasi-infected dogs treated with this recombinant antigen (23, 25). Furthermore, although not statistically significant, the treatment with rLdccys1 induced an increase of CD8+ T lymphocytes and this increase was significantly higher in mice treated with either rLdccys1 plus P. acnes or the triple association. These data are in accordance with previous findings on analysis of the predicted amino acid sequence of the cloned L. (L.) infantum chagasi Ldccys1 gene which showed one potential MHC class I epitope for CD8+ T lymphocytes besides two MHC class II epitopes (30). In addition, parallel to its inflammatory property, P. acnes increases MHCI, MHCII, TLR2.4.9 molecules expression in cell surface as dendritic cells macrophages and B lymphocytes (20, 35, 36). The IFN-γ production suggests its involvement in the destruction of parasites in treated animals. Although the participation of CD4+ Th1 lymphocytes with IFN-γ production has been demonstrated in mice protected against L. (L.) amazonensis infection (37, 38), it is important to emphasize that the immune responses induced against L. (L.) amazonensis have shown a distinct pattern from that described for L. (L.) major. While mice that are resistant or susceptible to infection with L. (L.) major exhibit immune responses polarized to Th1 and Th2, respectively, susceptibility to L. (L.) amazonensis cannot be correlated to an increased Th2 response (39). Furthermore, BALB/c mice infected with L. (L.) amazonensis develop Th1 responses (39–41) and the treatment with either IFN-γ or IL-12 did not affect the outcome of L. (L.) amazonensis infection in BALB/c and C57BL/6 mice (42, 43). These data and recent findings that showed the resistance of the L. (L.) amazonensis strain used in the present study to the action of nitric oxide secreted by activated macrophages (44) permit to ascribe to cytotoxic CD8 + T lymphocytes a more relevant role in reduction of parasitic load. Other literature data have also shown that the susceptibility of L. (L.) amazonensis to nitric oxide secreted by activated macrophages is controversial (45, 46). The hypothesis of the relevance of cytotoxic CD8 + T lymphocytes in the destruction of parasites in treated animals is corroborated by previous data showing an increase of this lymphocyte population in parallel with its cytotoxic activity on L. (L.) amazonensis infected macrophages from partially protected BALB/c mice against homologous infection (47). Furthermore, the relevant role of cytotoxic CD8+ T lymphocytes producing perforin and IFN-γ on L. (L.) amazonensis infection has been demonstrated by other authors (48–50).
The specificity to rLdccys1 of the immune responses observed in treated groups was demonstrated by the evaluation of proliferative responses of lymphocytes from L. (L.) amazonensis-infected mice 30 days after the end of treatment stimulated in vitro by the recombinant antigen. Animal group treated with the triple association showed a significant lymphoproliferative responses mediated by CD4+, central CD4+ memory T cells, while all groups that received the recombinant protein displayed proliferative responses mediated by effector CD4+ memory T lymphocytes. These results are probably related to the permanent presence of the antigen in vivo during the treatment. The group treated with DPPE 1.2 plus P. acnes also exhibited effector CD4+ memory T responses possibly due to the immunological properties of this adjuvant previously discussed. Similar proliferative responses mediated by CD8+ T lymphocytes stimulated by rLdccys1 were also observed.
The implication of TGF-β in increasing infection by cutaneous and visceralizing Leishmania species has been demonstrated (51, 52). Data from the present study corroborate these results, as low levels of TGF-β were detected in lesions of treated mice that presented reduced parasitic load, a concomitant increase of CD4+T and CD8+T lymphocytes and a significant IFN-γ secretion. BALB/c mice displayed about 10 times higher parasite load compared to C57BL/6. These results reflect the different susceptibility to L. (L.) amazonensis of these strains since BALB/c mice develop the diffuse anergic form of cutaneous leishmaniasis characterized by metastatic and non-curable lesions with the presence of a high number of amastigotes (53). On the other hand, infection with L. (L.) amazonensis in the C57BL/6 strain leads to the development of chronic lesions (54). IFN-γ secretion was higher in BALB/c mice compared to C57BL/6, but in both strains there was a significant increase of this cytokine in animals treated with the triple association. On the other hand, in phase 1 of evaluation the TGF-β levels of C57BL/6 mice were about 5 times lower compared to those exhibited by BALB/c mice, possibly indicating that a lower immunosuppressive environment leads to the higher resistance of the C57BL/6 strain to L. (L.) amazonensis infection.
Regarding the treatment longevity, the data showed that all animals treated with DPPE 1.2 which received rLdccys1, P. acnes, or both for more 30 days displayed a parasite load similar to that observed in the first phase of evaluation, whereas there was a relapse in those animals treated with DPPE 1.2 alone. The increase of memory CD4+ T lymphocytes was only observed in animals treated with the triple association and among them, effector memory CD4+ T lymphocytes were detected only in C57BL/6 mice in both phases, whereas in BALB/c mice this set of lymphocytes increased only in phase 2 of evaluation. In the two mouse strains treated with the triple association, there was an increase of central and effector memory CD8+ T lymphocytes in both evaluation phases. In BALB/c mice the number of central memory CD8+ T lymphocytes was similar in both evaluation phases, whereas in C57BL/6 mice there was a higher proportion of effector memory CD8+ T lymphocytes in phase 1 of evaluation and in phase 2 only the effectors persisted. These findings are in accordance with the significant secretion of IFN-γ and low levels of TGF-β in mice treated with the triple association, indicating the maintenance of an inflammatory response in these groups which was more quickly induced in the C57BL/6 strain. The involvement of memory lymphocytes in the elimination of microorganisms is closely related to the prompt and specific nature of their responses and they are generated according to the intensity and maintenance of the inflammatory stimulus. Thus, the association of DPPE 1.2 with rLdccys1 plus P. acnes was able to potentiate and keep this stimulus due to the pro-inflammatory nature of this adjuvant and rLdccys1. Recently we also detected specific memory and effector CD4+ and CD8+ T lymphocytes increased in a HIV vaccine associated with P. acnes in experimental model including immune response longevity (55). In animals that received only DPPE 1.2, the inflammatory environment was not sustained, allowing parasite replication and disease relapse.
The present data are supported by literature reports which have shown the advantage of immunochemotherapy over monotherapy both in murine models of cutaneous and visceral leishmaniasis, as well as in patients suffering from American cutaneous leishmaniasis treated with leishmanicidal drugs associated with different Leishmania antigens (56–61).
Conclusion
In conclusion, data from the present study strengthened the effective inhibitory action of DPPE 1.2 on in vivo L. (L.) amazonensis infection at non-toxic concentrations. The association of this palladacycle complex with rLdccys1 plus P. acnes potentiated the leishmanicidal effect of DPPE 1.2, opening perspectives to further explore this association as a therapeutic alternative for cutaneous leishmaniasis caused by L. (L.) amazonensis.
Data Availability Statement
All datasets generated for this study are included in the article/Supplementary Material.
Ethics Statement
This study was carried out in strict accordance with the National Institutes of Health Guide for the Care Use of Laboratory Animals and the Brazilian National Law (11.794/2008). The protocol was approved by the Committee on the Ethics of Animal Experiments of the Institutional Animal Care and Use Committee at the Federal University of São Paulo (Id # CEUA: 2957181016).
Author Contributions
DS, FS, DT, and SK designed and performed the experiments, and analyzed and interpreted the data. DG designed, synthesized, and analyzed the compounds. IL-M analyzed and interpreted the data, and contributed to reviewing of the manuscript. CB and DG conceived the work, contributed to interpretation of the data, and wrote and reviewed the manuscript.
Funding
This work was supported by grant #2018/10.373-2, São Paulo Research Foundation (FAPESP). FS (2017/06350-4) was supported by FAPESP fellowship. DS (88882.330528/2019-01) was supported by Coordenação de Aperfeiçoamento de Pessoal de Nível Superior (CAPES) fellowship.
Conflict of Interest
The authors declare that the research was conducted in the absence of any commercial or financial relationships that could be construed as a potential conflict of interest.
Acknowledgments
We are grateful to Priscila D. Motta and Cristina M. Orikaza Toqueiro for technical assistance.
Supplementary Material
The Supplementary Material for this article can be found online at: https://www.frontiersin.org/articles/10.3389/fimmu.2020.00345/full#supplementary-material
References
1. WHO. Leishmaniasis Fact Sheet Updated April 2017. (2017). Available online at: http://www.who.int/mediacentre/factsheets/fs375/en/ (accessed December 2019).
2. Lainson R, Shaw JJ. New world leishmaniasis – the neotropical Leishmania species. In: Cox FEG, Kreier JP, Wakelin D, editors. Topley & Wilson’s Microbiology and Microbial Infections. Parasitology. New York, NY: Oxford University Press (1998). p. 242–66.
3. Nagle AS, Khare S, Kumar AB, Supek F, Buchynskyy A, Mathison CJ, et al. Recent developments in drug discovery for leishmaniasis and human African trypanosomiasis. Chem Rev. (2014) 114:11305–47. doi: 10.1021/cr500365f
4. Goto H, Lindoso JA. Current diagnosis and treatment of cutaneous and mucocutaneous leishmaniasis. Expert Rev Anti Infect Ther. (2010) 8:419–33. doi: 10.1586/eri.10.19
5. Croft SL, Coombs GH. Leishmaniasis – current chemotherapy and recent advances in the search for novel drugs. Trends Parasitol. (2003) 9:502–8. doi: 10.1016/j.pt.2003.09.008
6. Soto J, Arana BA, Toledo J, Rizzo N, Vega JC, Diaz A, et al. Miltefosine for New World cutaneous leishmaniasis. Clin Infect Dis. (2004) 38:1266–72. doi: 10.1086/383321
7. Sundar S, Jha TK, Thakur CP, Bhattacharya SK, Rai M. Oral miltefosine for Indian visceral leishmaniasis. N Engl J Med. (2002) 347:1739–46. doi: 10.1056/NEJMoa021556
8. Caires ACF. Recent advances involving palladium (II) complexes for cancer therapy. Anticancer Agents Me Chem. (2007) 7:484–91. doi: 10.2174/187152007781668661
9. Navarro M, Betancourt A, Hernández C, Marchán E. Palladium polypyridyl complexes: synthesis, characterization, DNA interaction and biological activity on Leishmania (L.) mexicana. J Braz Chem Soc. (2008) 19:1355–60. doi: 10.1590/S0103-50532008000700018
10. Matsuo AL, Silva LS, Torrecilhas AC, Pascoalino BS, Ramos TC, Rodrigues EG, et al. In vitro and in vivo trypanocidal effects of the cyclopalladated compound 7a, a drug candidate for treatment of Chagas’ disease. Antimicrob Agents Chemother. (2010) 54:3318–25. doi: 10.1128/AAC.00323-10
11. Velásquez AMA, Souza RA, Passalacqua TG, Ribeiro AR, Scontri M, Chin CM, et al. Antiprotozoal activity of the cyclopalladated complexes against Leishmania amazonensis and Trypanosoma cruzi. J Braz Chem Soc. (2016) 27:1032–9. doi: 10.5935/0103-5053.20150360
12. Gupta S, Sane SA, Shakya N, Vishwakarma P, Hag W. CpG oligodeoxynucleotide 2006 and miltefosine, a potential combination for treatment of experimental visceral leishmaniasis. Antimicrob Agents Chemother. (2011) 55:3461–4. doi: 10.1128/AAC.00137-11
13. Seifert K, Juhls C, Salguero FJ, Croft SL. Sequential chemoimmunotherapy of experimental visceral leishmaniasis using a single low dose of liposomal amphotericin B and a novel DNA vaccine candidate. Antimicrob Agents Chemother. (2015) 59:5819–23. doi: 10.1128/AAC.00273-15
14. Paladi SP, Pimentel IAS, Katz S, Cunha RL, Judice WA, Caires AC, et al. In vitro and in vivo activity of a palladacycle complex on Leishmania (Leishmania) amazonensis. PLoS Negl Trop Dis. (2012) 6:e1626. doi: 10.1371/journal.pntd.0001626
15. Santos IB, Silva DAM, Paz FACR, Garcia DM, Carmona AK, Teixeira D, et al. Leishmanicidal and immunomodulatory activities of the palladacycle complex DPPE 1.1, a potential candidate for treatment of cutaneous leishmaniasis. Front Microbiol. (2018) 9:1427. doi: 10.3389/fmicb.2018.01427
16. Paladi CS, Silva DAM, Motta PD, Garcia DM, Teixeira D, Longo-Maugéri IM, et al. Treatment of Leishmania (Leishmania) amazonensis-infected mice with a combination of a palladacycle complex and heat-killed Propionibacterium acnes triggers protective cellular immune responses. Front Microbiol. (2017) 8:333. doi: 10.3389/fmicb.2017.00333
17. Matsui K, Yoshimoto T, Tsutsui H, Hyodo Y, Hayashi N, Hiroishi K, et al. Propionibacterium acnes treatment diminishes CD4+ NK1.1+ T cells but induces type I T cells in the liver by induction of IL-12 and IL-18 production from Kupffer cells. J Immunol. (1997) 159:97–106.
18. Braga EG, Ananias RZ, Mussalem JS, Squaiella CC, Longhini ALF, Mariano M, et al. Treatment with Propionibacterium acnes modulates the late phase reaction of immediate hypersensitivity in mice. Immunol Lett. (2003) 88:163–9. doi: 10.1016/S0165-2478(03)00079-8
19. Squaiella CC, Longhini ALF, Braga EG, Mussalem JS, Ananias RZ, Yendo TM, et al. Modulation of the type I hypersensitivity late phase reaction to OVA by Propionibacterium acnes-soluble polysaccharide. Immunol Lett. (2008) 121:157–66. doi: 10.1016/j.imlet.2008.10.005
20. Squaiella CC, Teixeira D, Mussalem JS, Ishimura ME, Longo-Maugéri IM. Modulation of Th1/Th2 immune responses by killed Propionibacterium acnes and its soluble polysaccharide fraction in a type I hypersensitivity murine model: induction of different activation status of antigen-presenting cells. J Immunol Res. (2015) 2015:132083. doi: 10.1155/2015/132083
21. Silva DM. Efeito do Tratamento Com o Complexo Paladaciclo DPPE 1.2 Associado à Cisteína Proteinase Recombinante rLdccys1 Sobre a Infecção por Leishmania (Leishmania) Amazonensis em Modelo Murino. Ph.D. thesis, Universidade Federal de São Paulo, São Paulo (2016).
22. Pinheiro PHC, Dias SS, Eulálio KD, Mendonça IL, Katz S, Barbiéri CL. Recombinant cysteine proteinase from Leishmania (L.) chagasi implicated in human and dog T cell responses. Inf Immun. (2005) 73:3787–9. doi: 10.1128/IAI.73.6.3787-3789.2005
23. Ferreira JHL, Gentil LG, Dias SS, Fedeli CEC, Katz S, Barbiéri CL. Immunization with the cysteine proteinase Ldccys1 gene from Leishmania (Leishmania) chagasi and the recombinant Ldccys1 protein elicits protective immune responses in a murine model of visceral leishmaniasis. Vaccine. (2008) 26:677–85. doi: 10.1016/j.vaccine.2007.11.044
24. Pinheiro PHC. A Cisteína Proteinase Recombinante de Leishmania (Leishmania) Chagasi (rLdccys1): Alvo Para Diagnóstico e Imunização Protetora de Cães de Área Endêmica de Leishmaniose Visceral, Teresina/PI. Ph.D. thesis, Universidade Federal de São Paulo, São Paulo (2009).
25. Ferreira JHL, Silva LS, Longo-Maugéri IM, Katz S, Barbiéri CL. Use of a recombinant cysteine proteinase from Leishmania (Leishmania) infantum chagasi for the immunotherapy of canine visceral leishmaniasis. PLoS Negl Trop Dis. (2014) 8:e2729. doi: 10.1371/journal.pntd.0002729
26. Barbiéri CL, Doine AI, Freymüller E. Lysosomal depletion in macrophages from spleen and foot lesions of Leishmania-infected hamster. Exp Parasitol. (1990) 71:218–28. doi: 10.1016/0014-4894(90)90024-7
27. Rodrigues EG, Silva LS, Fausto DM, Hayashi MS, Dreher S, Santos EL, et al. Cyclopalladated compounds as chemotherapeutic agents: antitumor activity against a murine melanoma cell line. Int J Cancer. (2003) 107:498–504. doi: 10.1002/ijc.11434
28. Mussalem JS, Squaiella CC, Teixeira D, Yendo TM, Thies FG, Popi AF. Adjuvant effect of killed Propionibacterium acnes on mouse peritoneal B-1 lymphocytes and their early phagocyte differentiation. PLoS One. (2012) 7:e33955. doi: 10.1371/journal.pone.0033955
29. Bradford MM. A rapid and sensitive method for the quantification of microgram quantities of protein utilizing the principle of protein-dye binding. Anal Biochemm. (1976) 7:248–54. doi: 10.1006/abio.1976.9999
30. Dias SS, Pinheiro PHC, Katz S, dos Santos MR, Barbiéri CL. A recombinant cysteine proteinase from Leishmania (Leishmania) chagasi suitable for serodiagnosis of American visceral leishmaniasis. Am J Trop Med Hyg. (2005) 72:126–32. doi: 10.4269/ajtmh.2005.72.126
31. Sheffield P, Garrard S, Derewenda Z. Overcoming expression and purification problems of RhoGDI using a family of “parallel” expression vectors. Prot Expres Purif. (1999) 15:34–9. doi: 10.1006/prep.1998.1003
32. Skeiky YAW, Coler RN, Brannon M, Stromberg E, Greeson K, Thomas-Crane R, et al. Protective efficacy of a tandemly linked, multi-subunit recombinant leishmanial vaccine (Leish-111f) formulated in MPL adjuvant. Vaccine. (2002) 20:3292–303. doi: 10.1016/s0264-410x(02)00302-x
33. Titus RG, Marchand M, Boon T, Louis JA. A limiting dilution assay for quantifying Leishmania major in tissues of infected mice. Parasite Immunol. (1985) 7:545–55. doi: 10.1111/j.1365-3024.1985.tb00098.x
34. Pinto AR, Beyrodt CGP, Lopes RAM, Barbiéri CL. Identification of a 30kDa antigen from Leishmania (L.) chagasi amastigotes implicated in protective cellular responses in a murine model. Int J Parasitol. (2000) 30:599–607. doi: 10.1016/s0020-7519(00)00037-0
35. Mussalem JS, Vasconcelos JR, Squaiella CC, Ananias RZ, Braga EG, Rodrigues MM, et al. Adjuvant effect of the Propionibacterium acnes and its purified soluble polysaccharide on the immunization with plasmidial DNA containing a Trypanosoma cruzi gene. Microbiol Immunol. (2006) 50:53–63. doi: 10.1111/j.1348-0421.2006.tb03791.x
36. Squaiella CC, Ananias RZ, Mussalem JS, Braga EG, Rodrigues EG, Travassos LR, et al. In vivo and in vitro effect of killed Propionibacterium acnes and its purified soluble polysaccharide on mouse bone marrow stem cells and dendritic cell differentiation. Immunobiology. (2006) 211:105–16. doi: 10.1016/j.imbio.2005.10.013
37. Coelho EA, Tavares CA, Carvalho FA, Chaves KF, Teixeira KN, Rodrigues RC, et al. Immune responses induced by the Leishmania (Leishmania) donovani A2 antigen, but not by the LACK antigen, are protective against experimental Leishmania (Leishmania) amazonensis infection. Infect Immun. (2003) 71:3988–94. doi: 10.1128/iai.71.7.3988-3994.2003
38. Pinto EF, Pinheiro RO, Rayol A, Larraga V, Rossi-Bergmann B. Intranasal vaccination against cutaneous leishmaniasis with a particulated leishmanial antigen or DNA encoding LACK. Infect Immun. (2004) 72:4521–7. doi: 10.1128/IAI.72.8.4521-4527.2004
39. Afonso LCC, Scott P. Immune responses associated with susceptibility of C57BL/10 mice to Leishmania amazonensis. Infect Immun. (1993) 61:2952–9.
40. Ji J, Sun J, Qi H, Soong L. Analysis of T helper cell responses during infection with Leishmania amazonensis. Am J Trop Med Hyg. (2002) 66:338–45. doi: 10.4269/ajtmh.2002.66.338
41. Soong L, Duboise SM, Kima P, McMahon-Pratt D. Leishmania pifanoi amastigote antigens protect mice against leishmaniasis. Infect Immun. (1995) 63:3559–66.
42. Barral-Neto M, Von Sohsten RL, Teixeira M, Santos WL, Pompeu ML, Moreira RA, et al. In vivo protective effect of the lectin from Canavalia brasiliensis on BALB/c mice infected by Leishmania amazonensis. Acta Tropica. (1996) 60:237–50. doi: 10.1016/0001-706x(95)00120-4
43. Jones DE, Buxbaum LU, Scott P. IL-4-independent inhibition of IL-12 responsiveness during Leishmania amazonensis infection. J Immun. (2000) 165:364–72. doi: 10.4049/jimmunol.165.1.364
44. Carmo EVS, Katz S, Barbiéri CL. Neutrophils reduce the parasite burden in Leishmania (Leishmania) amazonensis-infected macrophages. PLoS One. (2010) 5:e1381. doi: 10.1371/journal.pone.0013815
45. Qi H, Wanasen N, Soong L. Enhanced replication of Leishmania amazonensis amastigotes in gamma interferon-stimulated murine macrophages: implications for the pathogenesis of cutaneous leishmaniasis. Infect Immun. (2004) 72:988–95. doi: 10.1128/iai.72.2.988-995.2004
46. Mukbel RM, Patten C, Gibson K, Ghosh M, Petersen C, Jones DE. Macrophage killing of Leishmania amazonensis amastigotes requires both nitric oxide and superoxide. Am J Trop Med Hyg. (2007) 76:669–75. doi: 10.4269/ajtmh.2007.76.669
47. Fedeli CE, Ferreira JH, Mussalem JS, Longo-Maugéri IM, Gentil LG, dos Santos MR, et al. Partial protective responses induced by a recombinant cysteine proteinase from Leishmania (Leishmania) amazonensis in a murine model of cutaneous leishmaniasis. Exp Parasitol. (2010) 124:153–8. doi: 10.1016/j.exppara.2009.09.003
48. Colmenares M, Kima PE, Samoff E, Soong L, McMahon-Pratt D. Perforin and gamma interferon are critical CD8+ T-cell-mediated responses in vaccine-induced immunity against Leishmania amazonensis infection. Infect Immun. (2003) 71:3172–82. doi: 10.1128/iai.71.6.3172-3182.2003
49. Alves CR, Benévolo-de-Andrade TC, Alves JL, Pirmez C. Th1 and Th2 immunological profile induced by cysteine proteinase in murine leishmaniasis. Parasite Immunol. (2004) 26:127–35. doi: 10.1111/j.0141-9838.2004.00691.x
50. Pereira BAS, Alves CR. Immunological characteristics of experimental murine infection with Leishmania (Leishmania) amazonensis. Vet Parasitol. (2008) 158:239–55. doi: 10.1016/j.vetpar.2008.09.015
51. Barral-Netto M, Barral A, Brownell CE, Skeiky YA, Ellingsworth LR, Twardzik DR, et al. Transforming growth factor-beta in leishmanial infection: a parasite escape mechanism. Science. (1992) 257:545–8. doi: 10.1126/science.1636092
52. Wilson ME, Young BM, Davidson BL, Mente KA, McGowan SE. The importance of TGF-β in murine visceral leishmaniasis. J Immunol. (1998) 161:6148–55.
53. Andrade ZA, Reed SB. Sadigursky M. Immunopathology of experimental cutaneous leishmaniasis. Am. J. Pathol. (1984) 114:137–48.
54. Calabrese KS, Costa SCG. Enhancement of Leishmania amazonensis infection in BCG non-responder mice by BCG-antigen specific vaccine. Mem Inst Oswaldo Cruz. (1992) 87:49–56. doi: 10.1590/s0074-02761992000500010
55. Teixeira D, Ishimura ME, Apostólico JS, Viel JM, Passarelli VC, Cunha-Neto E, et al. Propionibacterium acnes enhances the immunogenicity of HIVBr18. human immunodeficiency virus-1 vaccine. Front Immunol. (2018) 9:177. doi: 10.3389/fimmu.2018.00177
56. Da-Cruz AM, Filgueiras DV, Coutinho Z, Mayrink W, Grimaldi G, Luca PM, et al. Atypical mucocutaneous leishmaniasis caused by Leishmania braziliensis in an acquired immunodeficiency syndrome patient: T-cell responses and remission of lesions associated with antigen immunotherapy. Mem Inst Oswaldo Cruz. (1999) 94:537–42. doi: 10.1590/S0074-02761999000400020
57. Daftarian PM, Stone GW, Kovalski L, Kumar M, Vosoughi A, Urbieta M, et al. A targeted and adjuvanted nanocarrier lowers the effective dose of liposomal amphotericin B and enhances adaptive immunity in murine cutaneous leishmaniasis. J Infect Dis. (2013) 208:1914–22. doi: 10.1093/infdis/jit378
58. Gary SN, Afonso LCC, Farrell JP, Scott PJAYP. Switch from a type 2 to a type 1 T helper cell response and cure of established Leishmania major infection in mice is induced by combined therapy with interleukin 12 and Pentostam. Proc Natl Acad Sci USA. (1995) 92:3142–6. doi: 10.1073/pnas.92.8.3142
59. Joshi J, Kaur S. Studies on the protective efficacy of second-generation vaccine along with standard antileishmanial drug in Leishmania donovani infected BALB/c mice. Parasitology. (2013) 141:554–62. doi: 10.1017/s0031182013001959
60. Pinto JM, Pinto J, Costa CA, Genaro O, Marques MJ. Modabber F, et al. Immunochemotherapy for cutaneous leishmaniasis: a controlled trial using killed Leishmania (Leishmania) amazonensis vaccine plus antimonial. Int J Derm. (2002) 41:73–8. doi: 10.1046/j.1365-4362.2002.01336.x
Keywords: Leishmania (Leishmania) amazonensis, immunochemotherapy, palladacycle complex, rLdccys1, adjuvant Propionibacterium acnes
Citation: da Silva DAM, Santana FR, Katz S, Garcia DM, Teixeira D, Longo-Maugéri IM and Barbiéri CL (2020) Protective Cellular Immune Response Induction for Cutaneous Leishmaniasis by a New Immunochemotherapy Schedule. Front. Immunol. 11:345. doi: 10.3389/fimmu.2020.00345
Received: 23 October 2019; Accepted: 12 February 2020;
Published: 03 March 2020.
Edited by:
Wanderley De Souza, Federal University of Rio de Janeiro BrazilReviewed by:
Prashant Khare, All India Institute of Medical Sciences Bhopal IndiaValeria Borges, Oswaldo Cruz Foundation (Fiocruz), Brazil
Copyright © 2020 da Silva Santana Katz Garcia Teixeira Longo-Maugéri and Barbiéri. This is an open-access article distributed under the terms of the Creative Commons Attribution License (CC BY). The use, distribution or reproduction in other forums is permitted, provided the original author(s) and the copyright owner(s) are credited and that the original publication in this journal is cited, in accordance with accepted academic practice. No use, distribution or reproduction is permitted which does not comply with these terms.
*Correspondence: Clara L. Barbiéri, barbieri.clara@unifesp.br